- 1International Research Centre for Environmental Membrane Biology & Department of Horticulture, Foshan University, Foshan, China
- 2College of Resource and Environment , Huazhong Agricultural University, Wuhan, China
- 3Tasmanian Institute of Agriculture, University of Tasmania, Hobart, TAS, Australia
Root border cells (RBCs) are a group of cells that originated from the root cap meristem, which are developed by genetic regulation and play a variety of biological functions. Being composed of a homologous single cell population with high metabolic activity and intact cell walls, RBCs represent a highly useful tool for studying various aspects of plant mineral absorption and utilization, as well as plant-soil-microbiome interactions in the rhizosphere. Research on RBCs also promise to become a hotspot in the context of understanding root adaptive responses to hostile environments. In order to take advantage of RBCs as an ideal single cell system in plant-environmental interactions, we summarized the production and function of RBCs and built-up the methodology for RBCs culturing, purification, and quantity control for plant research. The latter is done by using a case study of the application of RBCs to study mechanisms of Al toxicity in plants. This work offers plant scientists a new cognition of adopting RBCs as a convenient single cell system for the multidisciplinary research including (but not limited to) plant physiology, development and genetics, nutrition, and stress and adaptation. Root border cells (RBCs) are derived from the root cap and represent a population of living cells with special physiological activity and biological roles that are different from the root cap cells per se. After being separated from the root cap, RBCs become more active in metabolism than the progenitor root cap cells; for example, they incorporate labeled amino acids into protein 2.6-fold more efficiently than the cells of the root cap. In addition, mRNA and protein were differentially expressed between root cap cells and RBCs. Since the production of RBCs is genetically regulated and RBCs played a variety of biological functions in resistance to biotic and abiotic stresses occurred in the rhizosphere, RBCs were suggested as an ideal single cell system for the study the response of plant root cells to nutrient availability, environmental stresses, and in plant-microbial interactions. Some studies revealed that RBCs, which development is regulated by endogenous and exogenous signals, are biologically viable in the majority of higher plant species. This work reviews the research on RBCs in plant environment interaction and describes the case study of RBCs as a convenient single cell system to study plant responses to Al toxicity.
1 Production of RBCs and its Advantages as Plant Single Cell Line
1.1 Production of RBCs
RBCs are a group of specialized cells that dissociate from the epidermis of the root cap and gather around the root tip, which were previously called sloughed root cap cells. As early as 1919, Knudson had pointed out that some sloughed root cap cells were metabolically active; however, this had not attracted much attention from researchers at that time. In fact, more than 90% of the sloughed root cap cells in most species are alive, and their development is genetically regulated and shown to maintain a variety of biological functions even under adverse conditions (1–3). Hawes (4) redefined these cells as RBCs, due to that they are located between the root surface and the soil, and emphasized that these cells were bioactive biotic boundary layers.
Root cap cells differentiate from root cap meristem through mitosis, and then go through a series of developmental stages such as starch synthesis stage, gravity sensing stage, and mucilage secretion stage to produce RBCs. The separation of border cells is the final stage of root cap cell development (5), and the total number of RBCs don’t increase indefinitely but tends to saturate. For example, during seed germination, the number of RBCs is positively correlated with the root elongation, but when the root length reaches about 2.5cm, the number of RBCs reaches the maximum value (5, 6).
The efficiency of RBCs production differs among species. Based on the previous studies, we analyzed the proportion of RBCs from 11 families that contains nearly 30 different species. The number and viability of RBCs are different not only among plant families, but also within one family. The RBCs number varies greatly among different plant families, ranging from 20 in Solanaceae to ~12,000 in Leguminous (Figure 1; 3, 7). As shown in Figure 1, RBCs number also varies greatly within one family, e.g. from about 5,000 in soybean (Glycine Max) up to 12,000 in pea (Pisum sativum) (8, 9). The environmental factors such as metal ions, the ratio of CO2/O2, etc., also affect the production of RBCs (8, 10, 11). For example, the RBCs number increased from approximately 10,000 per root tip in the control to 12,000 after Al exposure (Figure 1; 7). Figure 1 and Table 1 list the range of RBCs number among families and species affected by different metal ions. There is little doubt that RBCs function essentially at least among these plant species and respond actively to environmental signals especially stresses. Therefore, they represent natural homologous root cell lines that may be used in studying various aspects of plant-environmental interactions.
1.2 Functions of RBCs
When RBCs are generated from the root cap, they build a biological interface between the root surface and soil, and play many functions, such as directly affecting the rhizosphere micro-ecosystem, inhibiting the invasion of the pathogen, reducing root mechanical resistance and protecting the root tip (4, 7, 8, 10, 12–18). It has been reported that RBCs can quickly release antibiotics, specific enzymes, sugars, and other chemicals to regulate the growth of bacteria, fungi, viruses, and nematodes around the rhizosphere by changing gene expression under stress (18–20). Some scientists also called the RBCs as root extracellular traps (RET) and their associated secretions form a protective structure, which plays a major role in the interaction between roots and soil-borne microorganisms(21, 22). In addition, as RBCs separation from root tip proceeds, the cells synthesize and secrete a hydrated mucilage that contains polysaccharides, secondary metabolites, antimicrobial proteins and extracellular DNA (exDNA), and this exDNA-based matrix seems to function in root defense in a way similar to that of recently characterized neutrophil extracellular traps (NETs) in mammalian cells (23). Therefore, the change of the rhizosphere can be communicated through the interaction between RBCs, various rhizosphere microorganisms, and toxic substances (such as Al toxicity), thereby generating practical significance.
At present, the role of RBCs in improving plant stress resistance, especially in resisting heavy metal ion toxicity, has become a new hotspot in the field of plant physiology. We summarized this research on the range of RBCs number in different families which were affected by metal stresses with the citation lists in Figure 1. Among these studies, more attention was paid to Al toxicity. Under Al stress, the elongation of the root is significantly inhibited in a few minutes, and the root tip is the main target of Al stress sensing and toxicity (32–35). However, the most sensitive zone to Al toxicity is not the root cap and meristem, but root transition zone, a zone 2-3 mm away from the root tip (36). RBCs, produced and gathered around the root cap and meristem (Figure 2), were assumed to not only prevent the protruding root tip from mechanical damage, but also act as a protective barrier for the root tip to absorb Al. When the Al concentration increased to 200 µM, the thickness of the adhesive layer exuded by RBCs increased, reducing the toxic effect of Al on roots (37). In addition, the removal of RBCs leads to the increase of Al absorption by root tip (7, 38). As shown in Figure 2, in-depth research on the response of RBCs in situ or in vitro to Al toxicity was carried out (which will be discussed later), indicating RBCs have been successfully applied in Al research and can be an example of RBCs as a highly convenient single cell system for the study of plant-rhizosphere interactions.
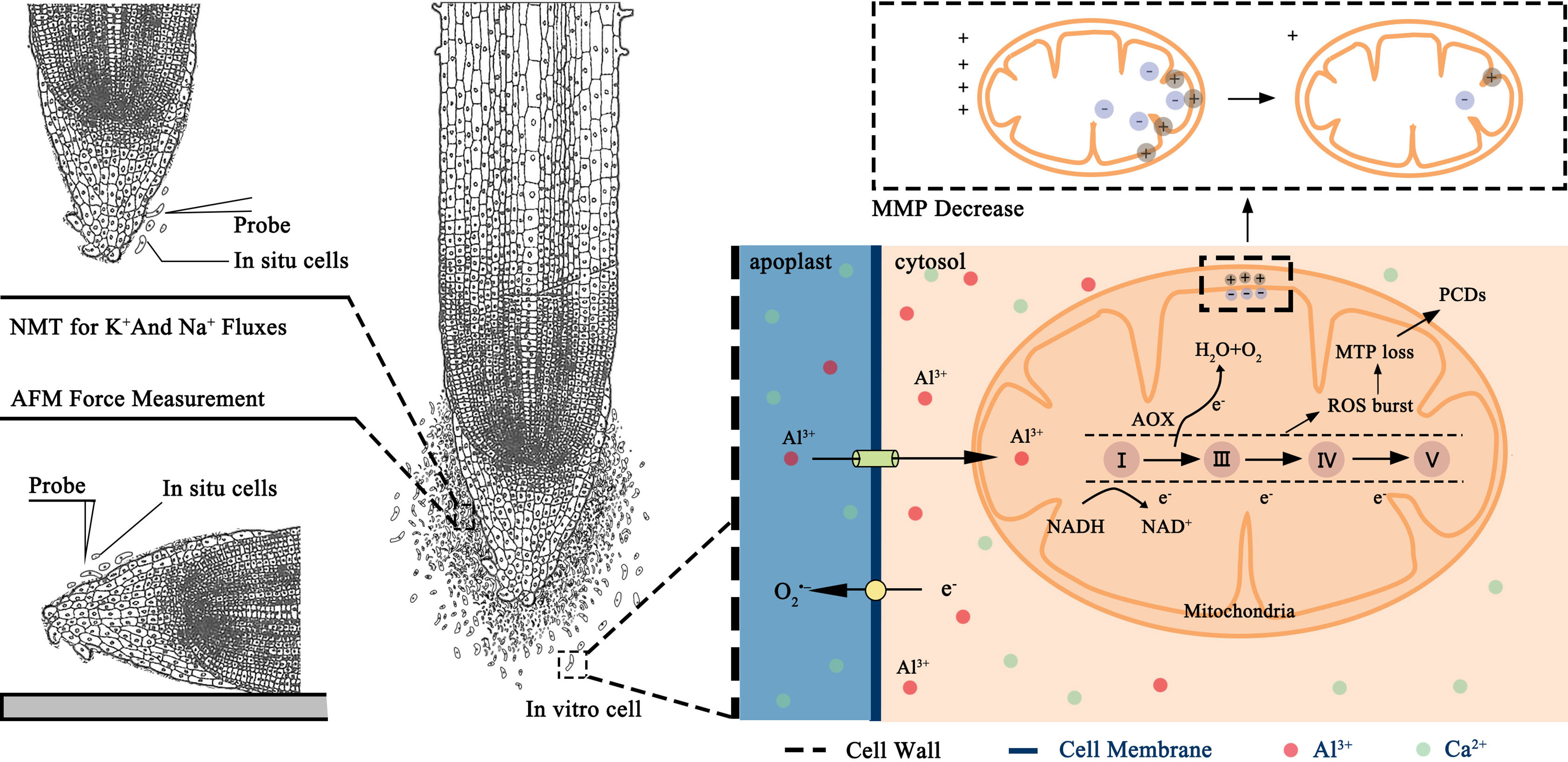
Figure 2 Schematic diagram of mechanism of response system of root border cells under Al toxicity stress.
1.3 The Ideal Plant Single Cell System of RBCs
As a homologous single cell group with metabolic activity and complete cell wall, the discovery of RBCs provides a useful tool for studying many interesting research topics including (but not limited to) Al toxicity and tolerance, transport of nutrients and toxins through cell wall and plasma membrane, and a broad range of interactions (e.g. Si-Al, B-Al interaction) in the cell wall matrix. As RBCs are naturally produced in root tips of a large number of plant species (7, 39), research on RBCs can reflect the real events that occurred in root tips, and the results are more reliable and applicable to root research in comparison to the other cell lines (e.g. suspension cells) or protoplast (without cell wall) and organelles (vacuoles), although they have other merits.
The second advantage is that RBCs can be acquired simply just by collection in water or solution, or even directly be wiped onto the glass slides for observation. It is well-known that the process of producing a new plant cell line generally takes a few years. The uniform population of protoplasts can be produced quicker (within several hours) but requires a prior optimization of the cocktail of enzymatic solution which is time consuming and may take months (3, 40). Protoplasts are also highly artificial as they lack cell walls (and hence many wall-anchored proteins) as well as have non-physiological resting membrane potential values that questions applicability of findings on protoplasts to explain planta responses. There are no other plant tissues that can be distinguished and dissected so easily without any complex instrumentation and isolation processes. Also, RBCs can survive for a long time in a large osmotic potential range including distilled water without disintegration or visible cell damage (13, 41).
Another advantage is the homogeneity of the RBCs that responds consistently to the chemicals, stresses, or signals which has a great advantage over root heterogeneity as cells from different root zones (root caps, meristem, transition, elongation, and mature zones) function and respond differently (19). Protoplasts derived from a specific cell type also possess this feature but creating a homogenous protoplast population may require cell-specific fluorescent labeling and the use of highly expensive and sophisticated techniques (e.g., fluorescence-activated cell sorting). Most critically, lacking cell walls, protoplasts cannot be used to study the response of whole single cells to chemicals, stresses, and signals in the apoplast (42–44) limiting their applicability in stress physiology.
Last but not the least, previous studies have shown that the RBCs and their mucilage could form a protective sheath at the root tip, which prevented Al from diffusing into the root tip, thereby alleviating Al stress in plant root tip (7, 28, 45, 46). The mucilage consists of a variety of molecules including cell wall polysaccharides and proteoglycans, secondary metabolites, anti-microbial proteins and peptides, and extracellular DNA (13, 47–50). Mucilage can strongly bind Al in the rhizosphere (46). The mucilage can protect root tips from Al toxicity by tightly binding Al and sequestrating Al in mucilage, which consequently reduced the inhibition of root growth induced by Al and the absorption of Al by roots (51). Therefore, RBCs are considered to be the front of plant response and defense to stresses. Considering RBCs are easier to be harvested in large quantities and easily purified, they are widely used in studies on Al toxicity (7, 8, 15, 39, 52). Of specific interest is pea (Pisum sativum) - Mendel’s model genetic material that are capable to produce up to 10,000 RBCs from one root tip (8).
2 Methodological Aspects of RBCs Production and Use: A Case Study for Al Response and Tolerance
2.1 Production of RBCs
2.1.1 Modified Solid Media Culture
Pea seeds are generally germinated in a Petri dish with sterilized 1.0% agar, and then a small amount of RBCs derived from the pea roots is harvested for further analysis (53, 54). If required, the chemicals can be applied to the agar preparation. To maximize the cell yield, the growth procedure was modified as follows. Firstly, pea seeds are soaked in 0.1-2.0 mmol/L CaCl2 for 10-48 h after sterilization in 7.5% sodium-hypochlorite solution. Then, the fully soaked seeds are transferred to the solid agar using tweezers, and a layer of filter paper is put on the culture medium to prevent the root tip to penetrate the culture medium. The cells are then cultured in a 20-32°C incubator for 24-72 h (depending on plant growth rate) and collected when the root length reaches 1.0-5.0 cm. Cells can be collected by immersing root tips in water and gently rotating to detach the RBCs from the root (3, 4, 12). The solid media culture was previously widely used for pathology studies as it can be completely sterilized and can conveniently incorporate the treatment of microorganisms (3, 4, 12). However, it is difficult to count the number of RBCs since RBCs will fall off when they are touched during the sampling process, which affects the experimental results. Also, a large number of RBCs could be potentially obtained by cutting off many root tips (one by one) from plants grown in multiple Petri dishes (3, 8, 19). However, it is practically not feasible since it is time and labor-consuming and the viability of the RBCs will drop significantly if separated from the root tips for more than 3 h. So the modified solid media culture is most suitable for the research requiring a small amount of RBCs and studies that require strict sterility.
2.1.2 Mist Culture
Mist culture was invented for the mass harvest of RBCs by Yu’s group and it was found that mist culture could quickly collect a large number of RBCs with high metabolic activity (8). The method involves the use of a mist incubator (depicted in Figure 3) composed of a sealed box to keep the humidity, a plastic mesh rack to support seeds, a mist generator to produce fine mist for seed germination, a timer to control the operation of the mist generator. Seeds are put on the mesh rack and a mist generator is regularly used to keep the humidity in the sealed box at a constant level to ensure the normal growth and development of seeds. The mist production is controlled by a timer with a 15 s spaying time and at the time interval of 5 min, which can ensure the humidity in the box of 98%, and the temperature is controlled at 22-24°C. Under the plant gravitropism, the germinated root tips are neatly distributed under the mesh. After the roots are elongated to 2-3 cm, the mesh rack can be removed from the sealed box and put into water to collect RBCs. In addition, during the culture process, the nutrient composition may be modified as per purpose of the experiment, and various signaling and chemical agents could be also dispersed by mist generator (7, 8, 15, 39, 52, 55).
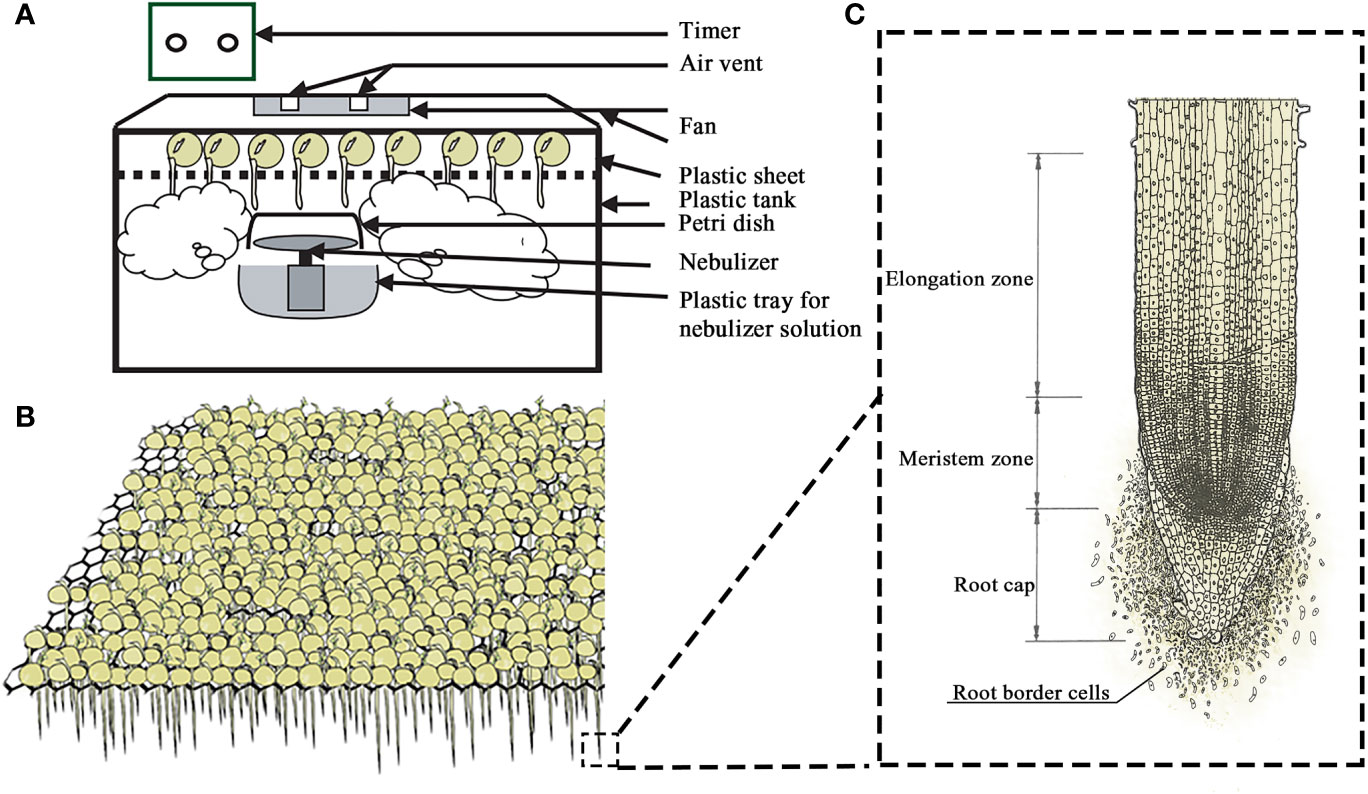
Figure 3 Scheme of the mist-culture device (A) for easy mass production and harvesting of pea seeds (B) and root border cells (C) with their root tips exposed to the humid atmosphere.
2.1.3 Quality and Quantity Control of Harvested RBCs
The RBCs are generally harvested (or collected) in the solution of the same chemical composition as for germination (0.1-2.0 mmol/L CaCl2). Cells are then purified by centrifugation at a centrifugal force of less than 500 (×g) to 1-3 times depending on the experiment requirement. Then the viability and number of RBCs are generally checked under a microscope for the quality and quantity control of the RBCs for further research. Generally, the total number of the pelleted RBCs is used to control the next experiment under the same amount of cell numbers. Some of these issues are discussed in more detail in the case study of Al tolerance below.
2.2 The Case Study of RBCs in Research of Al Tolerance
2.2.1 The Detection of the Number and Viability of RBCs Under Al Stress
It is a direct method to study the response of RBCs to Al stress by detecting the cell activity or quantity/proportion of viable cells. The number of cells can be counted by hemocytometer or by visual microscopic observations. For activity detection, many methods have been used, such as FDA (Fluorescent Diacetate) - PI (Propidium Iodide) viability staining (56), TTC (Triphenylte-trazolium Chloride) staining (19) and trypan blue exclusion staining (8, 15). Trypan blue is a cell reactive dye, and is commonly used to detect the activity of RBCs. In a living cell, the intact cell membrane can prevent trypan blue from entering the cells, so it will not be stained. If the membrane integrity is broken, the dead cells will be stained in blue due. During the operation of the trypan blue exclusion method, the solution containing RBCs and 0.5% trypan blue staining solution is mixed at 1:1 (V/V) and stained in the dark for 15 min. The blue-stained cells are dead cells and the non-stained cells are living cells. The cell survival rate is calculated according to the distribution of cells of the two colors under the microscope. RBCs in the majority of species examined are >90% viable (19). However, RBCs separated from the root cap died within 24 h under Al3+ stress while the in vitro RBCs maintained 85% viability at 48 h after treatment (25).
2.2.2 Removing Extracellular Al Ions From RBCs
RBCs can also be used to study the absorption kinetics of specific ions by single cells. When RBCs are treated in vitro, some metal ions treated outside or secreted by cells are required to be removed because of the reaction conditions of each element in the cells to be determined, as to purify RBCs. Generally, two methods are frequently used; one is microporous membrane filtration, and the second one is purification by centrifugation. Microporous membrane purification uses a 0.4~5 µm filter membrane to purify cells (10, 53, 57–59). The advantage of this method is that it is fast, accurate, and will not wash off cells. However, the method comes with the danger of mechanically damaging cells thus affecting their operation and viability. The centrifugal purification method is gentler. RBCs are centrifugated with a centrifugal force of less than 500 (×g), and lots machines, such as ICP(Inductive Coupled Plasma Emission Spectrometer), can be used to measure the degree of extracellular Al washing of each cell. The extracellular Al is completely removed after the 3-4 times centrifugation.
2.2.3 Changes of Cell Wall Components of RBCs Under Al Stress
The cell wall is in the outermost layer of the cell. As the initial part of Al contact, it is the first barrier for plants to deal with Al stress, both the initial site of Al toxicity, and the main part of Al accumulation. The accumulation of Al in the cell wall is a prerequisite for the toxicity of Al to plant root tips (32, 35, 48, 60). Pectin in the cell wall can promote the tolerance of plants to Al by regulating its distribution, so the combination of Al and cell wall polysaccharides plays an important role in the expression of Al toxicity and tolerance in plants (27, 61). In the analysis of cell wall components, The extraction of cell wall and the separation of cell wall polysaccharide components were carried out according to Takayuki etal. (62). The total sugar of cell wall can be determined by the phenol-sulfuric acid method based on glucose (63); uronic acid is determined by pyrocatechol colorimetry (64); and pectin content is analyzed and determined according to the method of Li etal. (45). Through the study of the interaction between cell wall and Al, the experimental conditions for the tolerance of root border cells to Al ion stress were established and optimized, so as to better study the physiological and biochemical mechanism of Al toxicity at the cell level and provide a direct method for the screening of Al tolerant germplasm in the future.
2.2.4 Biomineralization of Al in RBCs
The biomineralization process of cell induced self-assembly to form nanostructures has been a great interest subject to biologists and material chemists for many years. It is a process in which a small number of organic macromolecules (polysaccharides, proteins, and glycoproteins) are used as templates for a molecular operation to control the nucleation and growth of mineral phases and combine them into inorganic materials in a highly orderly manner (15, 65). Inspired by nature, many researchers imitate the sheath structure outside biological cells by physical, chemical, or biochemical methods, especially the chemical modification method, which is a technology to prepare polyelectrolyte multilayer coating by alternately depositing anionic and cationic polyelectrolytes on the solid surface through electrostatic interaction (66, 67). Among them, layer-by-layer self-assembly (LBL) and polyethyleneimine (PEI) induction technology are common chemical modification methods. In recent years, chemical modification methods have been applied to yeast cells and rice suspension cells. The results show that the deposition of nano silicon can inhibit the entry of Cd2+ into cells and effectively alleviate cadmium toxicity (68, 69). For RBCs, Feng etal. (15) applied layer-by-layer self-assembly technology to pea RBCs, showing that nanosilicon can keep the mitochondrial function of RBCs normal under Al stress, reduce the burst of reactive oxygen species (ROS), and finally reduce the programmed cell death, so that RBCs can maintain high viability without significant difference from the control group. This mechanism is like the RBCs in response to Al toxicity described in Figure 2. Briefly, nano silicon deposition confers cells with high Al resistance. In addition, in situ RBCs can be used to study the change of cell wall mechanical properties and cell ion homeostasis under Al stress. Thus, RBCs offer material scientists a new single cell system for studing biomineralization.
3 Prospects
The use of RBCs provides significant competitive advantages for plant cell physiology research compared with the other plant cell lines or protoplast-based methods. Studies involving RBCs are becoming a hot spot in the research of developmental biology, plant nutrition, and plant-environmental interactions. Adopting RBCs in research of Al toxicity provides opportunities for understanding the mechanisms underlying Al tolerance making the use of the cutting edge techniques such as microelectrode ion flux estimation(MIFE). There is also an exciting possibility of utilizing RBCs in research on biomineralization. We believe that RBCs, as an ideal single cell system, may disclose more molecular mechanisms of the response of cells to surrounding signals, if taken in combination with modern molecular tools such as single cell omics techniques.
Author Contributions
Y-MF: Conceptualization, Methodology, Software, Investigation, Formal Analysis, Writing - Original Draft. X-YC: Data Curation, Writing - Original Draft. X-WL: Supervision, Writing - Review & Editing. Y-LL: Resources, Supervision. WN: Software, Validation. JT: Visualization, Writing - Review & Editing. H-XH: Software, Validation. LS: Conceptualization, Editing. SS: Resources, Supervision, Writing. MY: Conceptualization, Funding Acquisition, Resources, Supervision, Writing - Review & Editing. All authors contributed to the article and approved the submitted version.
Funding
This study was supported by the National Natural Science Foundation of China (42077150, 32172672, 32002130, 31172038), the Science and Technology Department of Guangdong Province (2018A050506085, 163-2018-XMZC-0001-05-0049), the Provincial National Science Foundation of Guangdong Province (2019A1515110059, 2015A030313637, 2016A030313379), and the Higher Education Department of Guangdong Province (2020KCXTD025, 2018KQNCX286).
Conflict of Interest
The authors declare that the research was conducted in the absence of any commercial or financial relationships that could be construed as a potential conflict of interest.
Publisher’s Note
All claims expressed in this article are solely those of the authors and do not necessarily represent those of their affiliated organizations, or those of the publisher, the editors and the reviewers. Any product that may be evaluated in this article, or claim that may be made by its manufacturer, is not guaranteed or endorsed by the publisher.
References
1. Feldman LJ. The Development and Dynamics of the Root Cap Meristem. Am J Bot (1984) 71:1038–314. doi: 10.1002/j.1537-2197.1984.tb11987.x
2. Hawes MC, Lin HJ. Correlation of Pectolytic Enzymes Activity With the Programmed Release of Cells From Root Caps of Pea (Pisum Sativum). Plant Physiol (1990) 94:1855–9. doi: 10.1104/pp.94.4.1855
3. Brigham LA, Woo HH, Hawes M. Root Border Cells as Tools in Plant Cells Studies. Methods Cell Biol (1995) 49:377–87. doi: 10.1016/S0091-679X(08)61467-3
4. Hawes MC. Sloughed Root Cap Cells: A Regulator of Microbial Populations in the Rhizophere? Plant Soil (1990) 129:19–27. doi: 10.1007/BF00011687
5. Pan JW, Ye D, Wang LL, Hua J, Zhao GF. Root Border Cell Development is a Temperature-Insensitive and Al-Sensitive Process in Barley. Plant Cell Physiol (2004) 45(6):751–60. doi: 10.1093/pcp/pch090
6. Arriola L, Niemira BA, Safir GR. Border Cells and Arbuscular Mycorrhizae in Four Amaranthaceae Species. Phytopathology (1997) 12:1240–2. doi: 10.1094/PHYTO.1997.87.12.1240
7. Yu M, Shen RF, Liu JY, Chen RF, Xu MM, Yang Y, et al. The Role of Root Border Cells in Aluminum Resistance of Pea (Pisum Sativum) Grown in Mist Culture. J Plant Nutr Soil Sci (2009) 172(4):528–34. doi: 10.1002/jpln.200800039
8. Yu M, Feng YM, Goldbach HE. Mist Culture for Mass Harvesting of Root Border Cells: Aluminum Effects. J Plant Nutr Soil Sci (2006) 169(5):670–4. doi: 10.1002/jpln.200620604
9. Ropitaux M, Bernard S, Schapman D, Follet-Gueye ML, Vicre M, Boulogne I, et al. Root Border Cells and Mucilage Secretions of Soybean, Glycine Max (Merr) L.: Characterization and Role in Interactions With the Oomycete Phytophthora Parasitica. Cells (2020) 9(10):2215. doi: 10.3390/cells9102215
10. Hawes MC, Brigham LA, Wen F, Woo HH, Zhu Y. Function of Root Border Cells in Plant Health: Pioneers in the Rhizosphere. Annu Rev Phytopathol (1998) 36:311–27. doi: 10.1146/annurev.phyto.36.1.311
11. Knox OGG, Curlango-Rivera G, Huskey DA, Hawes MC. Border Cell Counts of Bollgard3 Cotton and Extracellular DNA Expression Levels. Euphytica (2020) 216(9):142. doi: 10.1007/s10681-020-02678-8
12. Hawes MC, Brigham LA. Impact of Root Border Cells on Microbial Populations in Rhizosphere. Adv Plant Pathol (1992) 8:119–48
13. Hawes MC, Curlango-Rivera G, Xiong ZG, Kessler JO. Roles of Root Border Cells in Plant Defense and Regulation of Rhizosphere Microbial Populations by Extracellular DNA 'Trapping'. Plant Soil (2012) 355:1–16. doi: 10.1007/s11104-012-1218-3
14. Darshan K, Singh J, Yadav S, Venugopala KM, Aggarwal R. Root Border Cells: A Pioneer's of Plant Defence in Rhizosphere. Indian J Agric Sci (2020) 90(10):14–9.
15. Feng YM, Li XW, Guo SX, Chen XY, Chen TX, He YM, et al. Extracellular Silica Nanocoat Formed by Layer-By-Layer (LBL) Self-Assembly Confers Aluminum Resistance in Root Border Cells of Pea (Pisum Sativum). J Nanobiotechnol (2019) 17:53. doi: 10.1186/s12951-019-0486-y
16. Huskey DA, Curlango-Rivera G, Root RA, Wen FS, Amistadi MK, Chorover J, et al. Trapping of Lead (Pb) by Corn and Pea Root Border Cells. Plant Soil (2018) 430:205–17. doi: 10.1007/s11104-018-3716-4
17. Huskey DA, Curlango-Rivera G, Hawes MC. Use of Rhodizonic Acid for Rapid Detection of Root Border Cell Trapping of Lead and Reversal of Trapping With DNase. Appl Plant Sci (2019) 7(4):1240. doi: 10.1002/aps3.1240
18. Hawes MC, Gunawardena U, Miyasaka S, Zhao XW. The Role of Root Border Cells in Plant Defense. Trends In Plant Science (2000) 5(3):128–33. doi: 10.1016/S1360-1385(00)01556-9
19. Pan JW, Zhu MY, Peng HZ, Wang LL. Developmental Regulation and Biological Functions of Root Border Cells in Higher Plants. Acta Botanica Sin (2002) 44(1):1–8. doi: 10.3321/j.issn:1672-9072.2002.01.001
20. Ropitaux M, Bernard S, Follet-Gueye ML, Vicre M, Boulogne I, Driouich A. Xyloglucan and Cellulose Form Molecular Cross-Bridges Connecting Root Border Cells in Pea (Pisum Sativum). Plant Physiol Biochem (2019) 139:191–6. doi: 10.1016/j.plaphy.2019.03.023
21. Carreras A, Bernard S, Durambur G, Gugi B, Loutelier C, Pawlak B, et al. In Vitro Characterization of Root Extracellular Trap and Exudates of Three Sahelian Woody Plant Species. Planta (2020) 251:19. doi: 10.1007/s00425-019-03302-3
22. Driouich A, Gaudry A, Pawlak B, Moore JP. Root Cap-Derived Cells and Mucilage: A Protective Network at the Root Tip. Protoplasma (2021) 258(6):1179–85. doi: 10.1007/s00709-021-01660-y
23. Driouich A, Follet-Gueye ML, Vicre-Gibouin M, Hawes M. Root Border Cells and Secretions as Critical Elements in Plant Host Defense. Curr Opin Plant Biol (2013) 16(4):489–95. doi: 10.1016/j.pbi.2013.06.010
24. Sun DL, Cui JC, Xu GD, Liu P. Biological Characteristics of Border Cell of Tomato and Effect of Aluminum on its Viability. Subtropical Plant Sci (2006) 35(2):4. doi: 10.3969/j.issn.1009-7791.2006.02.001
25. Chen WR, Liu P, Xu G, Cai MZ, Yu HN, Chen MY. Effects of Al3+ on the Biological Characteristics of Cowpea Root Border Cells. Acta Physiologiae Plantarum (2008) 30(3):303–8. doi: 10.1007/s11738-007-0121-7
26. Cai MZ, Zhang SN, Xing CH, Wang FM, Zhu L, Ning W, et al. Interaction Between Iron Plaque and Root Border Cells Ameliorates Aluminum Toxicity of Oryza Sativa Differing in Aluminum Tolerance. Plant Soil (2012) 353:155–67. doi: 10.1007/s11104-011-1019-0
27. Nagayama T, Nakamura A, Yamaji N, Satoh S, Furukawa J, Iwai H. Changes in the Distribution of Pectin in Root Border Cells Under Aluminum Stress. Front Plant Sci (2019) 10:1206. doi: 10.3389/fpls.2019.01216
28. Xiao ZX, Liang YC. Silicon Prevents Aluminum From Entering Root Tip by Promoting Formation of Root Border Cells in Rice. Plant Physiol Biochem (2022) 175:12–22. doi: 10.1016/j.plaphy.2022.02.003
29. Sun LJ, Song J, Peng C, Xu C, Yuan XF, Shi JY. Mechanistic Study of Programmed Cell Death of Root Border Cells of Cucumber (Cucumber Sativus L.) Induced by Copper. Plant Physiol Biochem (2015) 97:412–9. doi: 10.1016/j.plaphy.2015.10.033
30. Cheng P, Yi W, Sun L, Chen X, Zhang L, Shi J. Distribution and Speciation of Cu in the Root Border Cells of Rice by STXM Combined With NEXAFS. Bull Environ Contamination Toxicol (2016) 96(3):408–14. doi: 10.1007/s00128-015-1716-0
31. Fu Q, Lai JL, Li C, Ji XH, Luo XG. Phytotoxicity Mechanism of the Natural Radionuclide Thorium in Vicia Faba. J Hazardous Mater (2021) 424:127718. doi: 10.1016/j.jhazmat.2021.127718
32. Delhaize E, Ryan PR. Aluminum Toxicity and Tolerance in Plants. Plant Physiol (1995) 107:315–21. doi: 10.1104/pp.107.2.315
33. Horst WJ. The Role of the Apoplast in Aluminum Toxicity and Resistance of Higher Plants: A Review. J Plant Nutr Soil Sci (1995) 158(5):419–28. doi: 10.1002/jpln.19951580503
34. Kochian LV. Cellular Mechanisms of Aluminum Toxicity and Resistance in Plants. Plant Physiol Plant Mol Biol (1995) 46:237–60. doi: 10.1146/annurev.pp.46.060195.001321
35. Matsumoto H. Cell Biology of Aluminum Toxicity and Tolerance in Higher Plants. Int Rev Cytol (2000) 200:1–46. doi: 10.1016/S0074-7696(00)00001-2
36. Sivaguru M, Horst WJ. The Distal Part of the Transition Zone is the Most Aluminum-Sensitive Apical Root Zone of Maize. Plant Physiol (1998) 116(1):155–63. doi: 10.1104/pp.116.1.155
37. Brigham LA, Hawes MC, Miyasaka SC. Avoidance of Aluminium Toxicity: Roles of Root Border Cells. https://link.springer.com/book/10.1007/0-306-47624-X Plant Nutrition (2001) 92:452–3. doi: 10.1007/0-306-47624-X_218
38. Hawes MC, McLain J, Ramirez-Andreotta M, Curlango-Rivera G, Flores-Lara Y, Brigham LA. Extracellular Trapping of Soil Contaminants by Root Border Cells: New Insights Into Plant Defense. Agronomy-Basel (2016) 6(1):5. doi: 10.3390/agronomy6010005
39. Yang J, Qu M, Fang J, Shen RF, Fong YM, Liu JY, et al. Alkali-Soluble Pectin Is the Primary Target of Aluminum Immobilization in Root Border Cells of Pea (Pisum sativum). Frontiers In Plant Science (2016) 7:1297. doi: 10.3389/fpls.2016.01297
40. Kumar N, Iyer-Pascuzzi AS. Shedding the Last Layer: Mechanisms of Root Cap Cell Release. Plants-Basel (2020) 9(3):308. doi: 10.3390/plants9030308
41. Driouich A, Smith C, Ropitaux M, Chambard M, Boulogne I, Bernard S, et al. Root Extracellular Traps Versus Neutrophil Extracellular Traps in Host Defence, a Case of Functional Convergence? Biol Rev (2019) 94(5):1685–700. doi: 10.1111/brv.12522
42. Pan WH, Shou JX, Zhou XR, Zha XJ, Guo TR, Zhu MY, et al. Al-Induced Cell Wall Hydroxyproline-Rich Glycoprotein Accumulation is Involved in Alleviating Al Toxicity in Rice. Acta Physiologiae Plantarum (2011) 33(2):601–8. doi: 10.1007/s11738-010-0684-6
43. Wan LC, Zhang HY. Cadmium Toxicity: Effects on Cytoskeleton, Vesicular Trafficking and Cell Wall Construction. Plant Signaling Behav (2012) 7(3):345–8. doi: 10.4161/psb.18992
44. Ganie SA, Ahammed GJ. Dynamics of Cell Wall Structure and Related Genomic Resources for Drought Tolerance in Rice. Plant Cell Rep (2021) 40(3):437–59. doi: 10.1007/s00299-020-02649-2
45. Li XW, Liu JY, Fang J, Tao L, Shen RF, Li YL, et al. Boron Supply Enhances Aluminum Tolerance in Root Border Cells of Pea (Pisum Sativum) by Interacting With Cell Wall Pectins. Front Plant Sci (2017) 8. doi: 10.3389/fpls.2017.00742
46. Geng MJ, Xu MM, Xiao HD, Wang HZ, He LL, Zhao ZQ, et al. Protective Role of Mucilage Against Al Toxicity to Root Apex of Pea (Pisum Sativum). Acta Physiol Plantarum (2012) 34(4):1261–6. doi: 10.1007/s11738-011-0919-1
47. Barilli E, Rubiales D, Amalfitano C, Evidente A, Prats E. BTH and BABA Induce Resistance in Pea Against Rust (Uromyces Pisi) Involving Differential Phytoalexin Accumulation. Planta (2015) 242:1095–106. doi: 10.1007/s00425-015-2339-8
48. Mravec J, Guo XY, Hansen AR, Schuckel J, Kracun SK, Mikkelsen MD, et al. Pea Border Cell Maturation and Release Involve Complex Cell Wall Structural Dynamics. Plant Physiol (2017) 174(2):1051–66. doi: 10.1104/pp.16.00097
49. Weiller F, Moore JP, Young P, Driouich A, Vivier MA. The Brassicaceae Species Heliophila Coronopifolia Produces Root Border-Like Cells That Protect the Root Tip and Secrete Defensin Peptides. Ann Bot (2017) 119:803–13. doi: 10.1093/aob/mcw141
50. Wen F, Curlango-Rivera G, Huskey DC, Xiong Z, Hawes MC. Visualization of Extracellular DNA Released During Border Cell Separation From the Root Cap. Am J Bot (2017) 104:1–9. doi: 10.3732/ajb.1700142
51. Cai MZ, Wang N, Xing CH, Wang FM, Wu K, Du X. Immobilization of Aluminum With Mucilage Secreted by Root Cap and Root Border Cells is Related to Aluminum Resistance in Glycine Max L. Environ Sci pollut Res (2013) 20(12):8924–33. doi: 10.1007/s11356-013-1815-6
52. Li CX, Yan JY, Ren JY, Sun L, Xu C, Li GX, et al. A WRKY Transcription Factor Confers Aluminum Tolerance via Regulation of Cell Wall Modifying Genes. J Integr Plant Biol (2020) 62(8):1176–92. doi: 10.1111/jipb.12888
53. Hawes MC, Pueppke SG. Isolated Peripheral Root Cap Cells: Yield From Different Plants, and Callus Formation From Single Cells. Am J Bot (1986) 73:1466–73. doi: 10.1002/j.1537-2197.1986.tb10892.x
54. Brigham LA, Woo HH, Nicoll SM, Hawes MC. Differential Expression of Proteins and mRNAs From Border Cells and Root Tips of Pea. Plant Physiol (1995) 109:457–63. doi: 10.1104/pp.109.2.457
55. Cai MZ, Zhang SN, Xing CH, Wang FM, Wang N, Zhu L. Developmental Characteristics and Aluminum Resistance of Root Border Cells in Rice Seedlings. Plant Sci (2011) 180(5):702–8. doi: 10.1016/j.plantsci.2011.01.017
56. Ishikawa S, Wagatsuma T. Plasma Membrane Permeability of Root-Tip Cells Following Temporary Exposure to Al Ions is a Rapid Measure of A1 Tolerance Among Plant Species. Plant Cell Physiol (1998) 39:516–25. doi: 10.1093/oxfordjournals.pcp.a029399
57. Zhao XW, Misaghi IJ, Hawes MC. Stimulation of Border Cell Production in Response to Increased Carbon Dioxide Levels. Plant Physiol (2000) 122:181–8. doi: 10.1104/pp.122.1.181
58. Brigham LA, Woo HH, Wen F, Hawes MC. Meristem-Specific Suppression of Mitosis and a Global Switch in Gene Expression in the Root Cap of Pea by Endogenous Signals. Plant Physiol (1998) 118:1223–31. doi: 10.1104/pp.118.4.1223
59. Zhu YM, Pierson LSIII, Hawes MC. Induction of Microbial Genes for Pathogenesis and Symbiosis by Chemicals From Root Border Cells. Plant Physiol (1997) 115:1691–8. doi: 10.1104/pp.115.4.1691
60. Horst WJ, Wang YX, Eticha D. The Role of the Root Apoplast in Aluminium-Induced Inhibition of Root Elongation and in Aluminium Resistance of Plants: A Review. Annals Of Botany (2010) 106(1):185–97. doi: 10.1093/aob/mcq053
61. Liu JY, Yu M, Liu LP, Xiao HD. Differences of Cell Wall Polysaccharides in Border Cells and Root Apices of Pea (Pisum Sativum) Under Aluminum Stress. Scientia Agricultura Sin (2009) 42(6):1963–71. doi: 10.3864/j.issn.0578-1752.2009.06.011
62. Takayuki H, Kouichi S, Kazuyuki W, Seiichiro K, Eiichi T. Growth and Cell Wall Changes in Rice Roots During Spaceflight. Plant Soil (2003) 255:19–26. doi: 10.1023/A:1026105431505
63. Dubios M, Gilles KA, Hamilton JK, Rebers PA, Smith F. Colorimetric Method for Determination of Sugars and Related Substances. Anal Chem (1956) 28:350–6. doi: 10.1021/ac60111a017
64. Blumenkrantz N, Asboe-Hansen G. New Method for Quantitative Determination of Uronic Acids. Anal Biochem (1973) 54:484–9. doi: 10.1016/0003-2697(73)90377-1
65. Wang LJ, Putnis CV, Ruiz-Agudo E, Hovelmann J, Putnis A. In Situ Imaging of Interfacial Precipitation of Phosphate on Goethite. Environ Sci Technol (2015) 10:1021. doi: 10.1021/acs.est.5b00312
66. Decher G. Fuzzy Nanoassemblies: Toward Layered Polymeric Multicomposites. Science (1997) 277(5330):1232–7. doi: 10.1126/science.277.5330.1232
67. Galloway JM, Senior L, Fletcher JM, Beesley JL, Hodgson LR, Harniman RL, et al. Bioinspired Silicification Reveals Structural Detail in Self-Assembled Peptide Cages. ACS NANO (2018) 12(2):1420–32. doi: 10.1021/acsnano.7b07785
68. Lee J, Choi J, Park JH, Kim MH, Hong D, Cho H, et al. Cytoprotective Silica Coating of Individual Mammalian Cells Through Bioinspired Silicification. Angewandte Chemie (2014) 53(31):8056. doi: 10.1002/anie.201402280
Keywords: root border cells, aluminum toxicity, model cells, plant-environment interaction, mist culture
Citation: Feng Y, Chen X, Li X, Li Y, Nong W, Tang J, Han H, Shi L, Shabala S and Yu M (2022) Root Border Cells as a Convenient Single Cell System to Study Plant-Environmental Interactions: A Case Study for Aluminum Tolerance. Front. Soil Sci. 2:909530. doi: 10.3389/fsoil.2022.909530
Received: 31 March 2022; Accepted: 17 May 2022;
Published: 22 June 2022.
Edited by:
Jiang Tian, South China Agricultural University, ChinaReviewed by:
Zhijian Chen, Chinese Academy of Tropical Agricultural Sciences, ChinaLi Li Sun, Fujian Agriculture and Forestry University, China
Copyright © 2022 Feng, Chen, Li, Li, Nong, Tang, Han, Shi, Shabala and Yu. This is an open-access article distributed under the terms of the Creative Commons Attribution License (CC BY). The use, distribution or reproduction in other forums is permitted, provided the original author(s) and the copyright owner(s) are credited and that the original publication in this journal is cited, in accordance with accepted academic practice. No use, distribution or reproduction is permitted which does not comply with these terms.
*Correspondence: Min Yu, eXVtaW5AZm9zdS5lZHUuY24=; Xue-wen Li, eHVld2VuLmxpQGZvc3UuZWR1LmNu