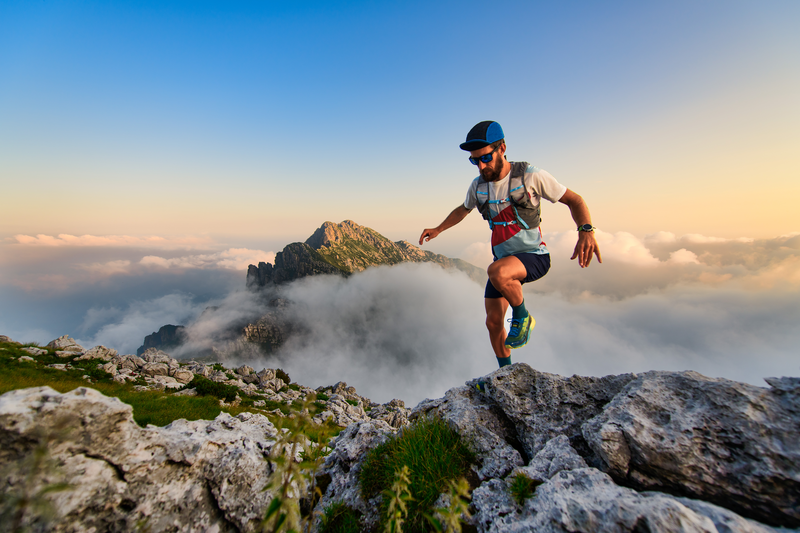
94% of researchers rate our articles as excellent or good
Learn more about the work of our research integrity team to safeguard the quality of each article we publish.
Find out more
ORIGINAL RESEARCH article
Front. Soil Sci. , 22 February 2022
Sec. Soil Pollution & Remediation
Volume 2 - 2022 | https://doi.org/10.3389/fsoil.2022.814924
Ciprofloxacin is a broad-spectrum antibiotic of the fluoroquinolone class that is used to treat bacterial infections in both humans and animals. Antibiotics released into the environment can select for antibiotic-resistant microorganisms, which negatively affects human and animal healthcare. Understanding soil factors that govern the mobility of ciprofloxacin can facilitate the development of targeted efforts to mitigate potential negative impacts. The objectives were (1) to determine the sorption capacity of ciprofloxacin in soils under acidic conditions; and (2) to reveal relative importance of key soil factors that influence sorption of ciprofloxacin. Evaluations were conducted using 20 soil samples with diverse properties and different cultivation/vegetation history. Sorption capacity ranged from 8 to 141 g kg−1; distribution coefficient (Kd) ranged from 23 to 200 mL kg−1 soil; and soil organic carbon-water partitioning coefficient (Koc) values ranged from 54 to 2,146 mL g−1 organic carbon. Clay content and cation exchange capacity were the most significant factors that influenced sorption capacity and Kd of ciprofloxacin with r values of 0.92*** and 0.64***, respectively. Soil pH had little effect on ciprofloxacin sorption parameters with r < 0.25. pH-independent charges played a predominant role dictating sorption parameters of ciprofloxacin in soil. Cation exchange via interlayer adsorption was a primary sorption mechanism under acidic conditions. Sorption parameters were significantly correlated with organic carbon content in cultivated soils only, resulting in r values of 0.97*** (with sorption capacity) and 0.72*** (with Kd). Cultivation led to changes in the quality of soil organic matter, resulting in changes in the sorption behavior and altered mechanisms of ciprofloxacin sorption in soil. Soils are effective in restraining the mobility of ciprofloxacin through adsorption and the effectiveness increases with clay content.
Environmental contamination by ciprofloxacin and the influence of soil properties on its sorption in soil are receiving increasing attention. The widespread use of antibiotics prescribed for therapeutic, prophylactic, and methaphylactic treatment to fight infectious diseases and as growth promotors in livestock production since the 1940's (1) has led to the extensive release of these compounds into the environment. Van Boeckel et al. (2) estimated that global consumption of antibiotics in meat production was 63,151 tons in 2010, and are expected to increase to 105,596 tons by 2030. Fluoroquinolones (FQs) are some of the most commonly used antibiotics. FQs treat bacterial infections by inhibiting DNA replication and can enter the environment through direct discharge of wastewater from wastewater treatment plants (WWTPs) and aquaculture facilities, through the application of manure, biosolids, runoff and leaching from agricultural fields and landfills, and leakage of septic tanks (3, 4). FQs have been reported to be as high as 31 mg L−1 in WWTP effluent (5), 6.5 mg L−1 in surface waters and 14 μg L−1 in groundwater (6). Manure amended soil have been detected having FQs at 9.8 mg kg−1 (7). Currently, there are no regulations on the application of animal manure as soil amendments to prevent antibiotic release.
Ciprofloxacin, a type of FQs, was first introduced to treat bacterial infections in humans and animals in 1987. According to the United States Center for Disease Control and Prevention (US CDC), approximately 62 million prescriptions were given to outpatients between 2013 and 2015 in the US (8). Nearly 90% of the consumed ciprofloxacin could be released into the environment because 45–62% of ciprofloxacin is released unmetabolized through urine while an additional 15–25% is released through feces (9).
The prevalence of antibiotics in the environment has caused increasing global concerns, attributing to increased antibiotic-resistant bacterial strains (3). Among antibiotic-treated chicken manure isolates, up to 100% of Campylobacter resisted antibiotics, compared to <12% from the untreated group (10). Consequently, the United States Food and Drug Administration (US FDA) in 2005 and the European Union (EU) in 2006 banned the use of FQ antibiotics in poultry production.
Introduction of ciprofloxacin could also lead to degradation of environmental health through altering bacterial function and processes. The growth of Pseudomonas putida in WWTPs was inhibited by 50% at merely 0.08 mg ciprofloxacin L−1 (11). In wastewater containing as little as 0.2 mg ciprofloxacin L−1, microbial consumption of nitrogen and phosphorus in wastewater processing was reduced by about 11 and 5%, respectively (12). Fu et al. (13) found 2.7 mg L−1 of ciprofloxacin in bench scale wastewater treatment reactors increased multidrug antibiotic resistance in isolates, decreased overall microbial community diversity, and strongly shifted the microbial community toward the Burkholderiales. In soil, Girardi et al. (14) reported an inhibition rate of 70% in cumulative respiration at 0.2 mg ciprofloxacin kg−1 soil, while Cui et al. (15) found that potential nitrification rates were significantly inhibited at 1 mg kg−1 soil. Ciprofloxacin negatively influenced microbial processes and function by altering community composition and diversity. Córdova-Kreylos and Scow (16) reported selective influence of ciprofloxacin on sediment microbial community, which favored Gram-negative and sulfate-reducing bacteria at 20 mg L−1. Naslund et al. (17) found that the presence of ciprofloxacin as low as 0.1 μg kg−1 sediment led to decreased bacterial community diversity.
The degree of ciprofloxacin influence on the microbial community is inversely related to its sorption to soils (16). Adsorption can regulate the fate of antibiotics in soil to a certain degree (18). Charges and properties of soil particles such as cation exchange capacity (CEC) and pH are key factors that influence its adsorption. Charges in soil are largely originated from clay minerals and organic materials. pH determines the charges of ionizable molecules. Thus, the content and type of clay and organic compounds as well as soil pH are expected to play dominant roles governing sorption of ciprofloxacin in soil. However, research findings on their influence on sorption of ciprofloxacin in soil have been inconsistent. Vasudevan et al. (19) reported that ciprofloxacin sorption was pH-dependent. In their study, ciprofloxacin adsorption peaked at pH 5.5 and decreased at pH 7-8. However, pH dependence of ciprofloxacin sorption to dissolved organic carbon (DOC) from biosolids was not observed by Carmosini and Lee (20) based on tests in the pH range of 5.5–7.6. Cation exchange has been suggested as a dominant pathway for ciprofloxacin adsorption in soil (19, 21, 22). Soil organic carbon (SOC) content has been reported to demonstrate a positive (23), a negative (24, 25), or have little effect (26, 27) on the sorption of ciprofloxacin in soil. The observed inconsistencies could be in part due to the complex chemical nature that hinders laboratory evaluations. Since ciprofloxacin is not soluble in alkaline conditions, it is challenging to determine its sorption capacity in alkaline soils and/or under alkaline conditions. Vasudevan et al. (19) concluded that ciprofloxacin sorption was pH-dependent based on initial sorption rates. Data interpretation and validation in their study were further complicated by the use of four different buffers and the potential influences of varied ionic strength (20). Further, limited studies have been conducted on revealing the effect of soil clay content on sorption of ciprofloxacin. Evaluations of purified clay minerals showed that 2:1 type of clays was hundreds times more effective in adsorbing ciprofloxacin than 1:1 clays (18, 28). Although CEC of organic matter is at least several times of those of clay minerals, clay content is order of magnitude higher than organic carbon content in soil. We hypothesized that clay content could be a major player governing sorption ciprofloxacin in soil. The objectives of this study were (1) to determine the sorption capacity of ciprofloxacin in soils under an acidic condition; and (2) to reveal relative importance of key soil factors that influence sorption of ciprofloxacin through examining sorption behavior in soils with diverse intrinsic soil properties and varied nature of SOC resulting from unique cultivation/vegetation history.
Twenty surface soil samples (0–10 cm for croplands and 0–5 cm for pasture and uncultivated soils) of diverse properties and vegetation covers were used in this study (Table 1). These soils included five major soil series in the United States and one from Hungary that were either cultivated with major crops (soybean, corn, wheat, cotton) and pasture, or uncultivated prairie, woodland, and pine forest. Following the removal of large plant debris and stones, soils were air-dried, ground to pass a 2-mm sieve, and kept in sealed containers at 23°C until analysis. Soil pH (soil:0.01M CaCl2 ratio = 1:2.5) was determined using a Basic® pH meter (Denver Instruments, Denver, CO, USA) equipped with Accumet combination glass electrode (Fisher, Pittsburgh, PA, USA). SOC was determined using a LECO CN 2000 (LECO Corp., St. Joseph, MI, USA) by dry combustion as described by Nelson and Sommers (29). Texture was determined using the hydrometer method (30). CEC was measured by summation of base cations (Ca, K, Mg, and Na) extracted in Mehlich-3 and quantified by Inductively Coupled Plasma (ICP) Spectroscopy (31).
Table 1. The taxonomy, location, vegetative cover, and key physical and chemical properties of the 20 soils used in this study.
Ciprofloxacin (1-cyclopropyl-6-fluoro-4-oxo-7-piperazin-1-ylquinoline-3-carboxylic acid, C17H18FN3O3) was purchased from Sigma-Aldrich (catalog # 17850, HPLC grade; St. Louis, MO, USA). Acetonitrile, hydrochloric acid and other solvents were purchased from Pharmco-Aaper (Brookfield, CT, USA). Water and all solvents were HPLC grade. Other chemicals were purchased from Sigma-Aldrich (St. Louis, MO, USA) or Fisher Scientific (Pittsburgh, PA, USA) and were at least of analytical grade. The ciprofloxacin had the following physical-chemical properties: molecular weight = 331.34 g mol−1, log Kow = 0.28, water solubility = 0.09 M, pKa1 = 6.09, and pKa2 = 8.74. The ciprofloxacin stock solution (1.0 mg mL−1) was prepared by dissolving 0.5 g of ciprofloxacin into 500 mL of 0.1 N HCl in a volumetric flask. The phosphate buffer (0.02 M) was prepared by dissolving 2.84 g of Na2HPO4 in about 700 mL of deionized water and adjusted to pH 2.7 with phosphoric acid (H3PO4).
All solutions were filtered through a 0.22 μm filter prior to be used in the HPLC analysis. For the determination of ciprofloxacin concentrations in aqueous solutions, HPLC analysis was performed using a modified method described by Vella et al. (32), employing a Waters HPLC Breeze system equipped with 1500 Series HPLC pump, 2487 dual wavelength absorbance detector and 717 plus autosampler (Waters, Milford, MA, USA). Separations were carried out using an Xterra Shield RP18 reverse phase 5 μm 4.6 × 150 mm column (Waters, Milford, MA, USA) under isocratic elution with a flow rate of 1 mL min−1. The mobile phase consists of 80% phosphate buffer (pH 2.7) and 20% acetonitrile. The 0.02 M phosphate buffer was made with Na2HPO4 and H3PO4 and titrated with HCl. The analysis was conducted by the injection of 50 μL of sample solution and recording UV signals at 244 nm (8 min run time and ~4.35 min retention time for ciprofloxacin). Ciprofloxacin concentration in the solution was calculated based on peak area in reference to a calibration curve that was constructed using standard ciprofloxacin solutions in the range of 0.0–10.0 μg ciprofloxacin mL−1 and prepared in 0.1 N HCl.
Sorption of the ciprofloxacin to the soil was determined by batch sorption. Sorption equilibrium of ciprofloxacin between aqueous phase and soil was evaluated by shaking 0.5 g of soil with 100 mL ciprofloxacin solution in 250 mL Nalgene bottles. Ciprofloxacin concentrations tested included 0, 20, 40, 80, 120, 160, 200, 300, 400, and 500 mg ciprofloxacin L−1. A preliminary experiment indicated that ciprofloxacin adsorption by soils was not significantly different when shaken for 30 min or 24 hrs. Since ciprofloxacin is only soluble in acidic solution and all ciprofloxacin solutions were made with 0.1 N HCl, the batch sorption reaction mixtures were adjusted to contain a final concentration of 0.05 N HCl to limit the effect of varying ionic strength on sorption. Samples were shaken for 30 min on an orbital shaker at 200 rpm, and then filtered through a Whatman 42 ashless filter to quickly separate the ciprofloxacin solution from soil. A portion of the ciprofloxacin solution was further filtered through a 0.22 μm membrane using a 25 mm corning sterile syringe filtration setup for HPLC analysis. Dilutions were performed when ciprofloxacin concentrations exceeded the range of the calibration curve. To validate the method and monitor its performance, quality control samples (QCs) were employed. The QCs were blank matrix samples that were spiked with known concentrations of ciprofloxacin designed to cover the range of concentrations in the samples. These QCs were placed at the beginning and end of each sample set and were also inserted randomly through the analytical run. Retention time was also monitored. At the end of the analyses, the QC data were examined against a set of predefined criteria that enabled us to decide whether or not to accept or reject the batch.
Ciprofloxacin adsorption isotherms were generated to estimate how much ciprofloxacin was adsorbed by soil (mg kg−1) and how much remained in the solution (mg L−1). The obtained adsorption data were fitted to two isotherm equations, the Langmuir and Freundlich.
The Langmuir equation is expressed as follows:
where qe is the amount of ciprofloxacin adsorbed (mg kg−1 soil), Q0 is the sorption capacity of the soil (mg kg−1 soil), KL is the distribution coefficient (mL kg−1) and Ce is the concentration of ciprofloxacin in the solution (mg L−1). The Langmuir equation was transformed into three linearized forms; Eadie-Hofstee, Lineweaver-Burk, and Hanes-Woolf all as follows:
The Freundlich isotherm is as follows:
where Kf and n are empirical constants derived from the intercept and slope, respectively. Kf is a measure of quantity of adsorbate on the absorbent, while 1/n measures the intensity of sorption. 1/n value above 1 indicates corporative adsorption while 1/n values below 1 indicate normal adsorption. The Freundlich isotherm can be linearized to the following equation:
To determine which transformation of the isotherms was the best fit, r values were calculated based on a qe vs. qe/Ce plot for Eadie-Hofstee, 1/qe vs. 1/Ce plot for Lineweaver-Burk, Ce/qe vs. Ce plot for Hanes-Woolf and ln qe vs. ln Ce for linearized Freundlich isotherm. Sorption capacity for each soil was calculated by averaging three sorption capacities determined from the three linear transformations of the Langmuir isotherms. Ciprofloxacin sorption distribution coefficient (Kd) was determined based on sorption of ciprofloxacin concentration up to 80 μg mL−1. The linear correlation coefficient, r values, between ciprofloxacin adsorbed to soil and those remained in the solution in this concentration range were 0.82*** to 1.00*** for the 20 soils tested. The Kd value is the slope of the linear regressions expressed as:
Ciprofloxacin sorption the soil organic matter partition coefficient (Koc) was calculated to normalize Kd values by the amount of organic carbon (OC) in the soil. This was done to reduce the variability of data due to the variability of OC found within the tested soils. Koc was calculated by dividing a Kd by the OC fraction, fOC, of a specific soil:
All results are averages of duplicate analyses expressed on soil dry weight basis. Soil moisture was determined based on weight loss after drying at 105°C for 48 h. Statistical analyses were performed employing Statistical Analysis System (SAS) 9.4 utilizing PROC REG and PROC PRINCOMP. Principal component analysis (PCA) was used to reveal the complex relationships among multiple soil variables engaging multidimensional data sets. Because input data were expressed in different units, PCA was performed using correlation coefficients rather than covariance matrix. Such an approach allowed reduction in the number of variables, limited redundancy, and revealed the dominant variance that drove the system.
The 20 soils had diverse properties, with ranges of pH from 3.8 to 7.7 (avg. 6.0), OC from 0.4 to 12.6% (avg. 2.7%), clay content from 12.5 to 60.0% (avg. 26.0%), and sand content from 7.5 to 67.5% (avg. 33.8%) (Table 1).
Example sorption isotherms for four of the 20 soils are shown in Figure 1. Data obtained fit both the Langmuir and Freundlich isotherms with statistically significant r values ranging from 0.61 to 1.00 (Supplementary Table 1 and Figure 2). Kf values ranged from 0.40 to 1.94 g kg−1; and n values ranged from 1.22 to 2.94 mL kg−1 for the Freundlich isotherm (Table 2). The 1/n values (data not shown) in the Freundlich isotherms were <1, suggesting that the adsorption follows an L-shaped curve and that sorption of ciprofloxacin to these soils were strongly favored at lower concentrations and decreased with increasing concentration. Sorption capacity varied considerably among soils, ranging from 8 to 141 g ciprofloxacin kg−1 soil (Table 2 and Supplementary Table 1). Sorption coefficients based on linear transformations of Langmuir (KL) ranged from 52 to 653 mL kg−1 soil; those based on the initial linear sorption range (Kd) ranged from 23 to 200 mL kg−1 soil; while those standardized by soil organic carbon content (Koc) ranged from 54 to 2,146 mL g−1 OC (Table 2).
Figure 1. Example sorption isotherms for four (soil 6, 12, 17, and 20) of the 20 soils tested. All 20 soils followed both the Freundlich and Langmuir isothermal shape as determined by correlation (r) values.
Figure 2. Example linear transformations of Freundlich and Langmuir isotherms for soil 17 in Table 1. qe is the concentration of ciprofloxacin adsorbed in the soil (in g kg−1) and Ce is the concentration of ciprofloxacin in the solution (mg L−1). Each individual soil tested were linearly transformed by Freundlich and the Langmuir linear transformations as described in the materials and methods to obtained adsorption parameters.
Table 2. Sorption capacity and sorption coefficients Kd and Koc of ciprofloxacin in soils used in this study.
Sorption capacity was significantly correlated with OC (r = 0.46**, Figure 3A), clay content (r = 0.92***, Figure 3B), and CEC (r = 0.64***, Figure 3C), but not with soil pH (Figure 3C). Relationships between Kd and the tested soil properties showed similar trend to those observed for sorption capacity, although the relationship between Kd and OC was not statistically significant (r = 0.35, Figure 3E). Again, soil clay content was the most significant factor influencing Kd values with a r value of 0.64*** (Figure 3F), while significant relationship was not observed between Kd and soil pH (Figure 3G). When the Kd values were expressed based on OC content as the Koc values, there were little relationships observed between Koc and the selected soil properties (Figures 3J–L), with the exception of OC content that was significantly negatively correlated with Koc (r = 0.60***, Figure 3I).
Figure 3. Relationships between selected soil properties and ciprofloxacin sorption parameters. Soil 18 from Table 1 was excluded from correlation analysis for subplots (A,E,I), while Soil 16 from Table 1 was excluded from correlation analysis for subplots (D,E,L). Linear regression is significant (p < 0.01) for (B,E) and (p < 0.05) for (A).
The relationships between sorption parameters and soil properties were further examined based on whether the soils were cultivated (Data not shown). Interestingly, sorption capacity was not significantly correlated with OC in uncultivated soil (r = 0.16), but strongly significant in cultivated soils (r = 0.96***). However, the relationships between sorption capacity and clay content, pH, or CEC were not altered by cultivation history, which were still significantly positively correlated with clay content and CEC but not with soil pH. Again, the relationships between Kd and soil properties showed similar trends to those observed for sorption capacity. Cultivation altered relationships between Kd and OC, which showed little relationship in the uncultivated soil (r = 0.03) but significant positive relationship in cultivated soils (r = 0.72**). Regardless of cultivation history, Kd values were significantly influenced by clay content and CEC but not by soil pH. The Koc values were significantly negatively correlated with OC in uncultivated soils (r = 0.90***), but not in the cultivated soils (r = 0.45). When grouped soils by cultivation, none of the Koc values were significantly correlated with clay content, pH, or CEC of the soils.
To further elucidate the relative importance of the evaluated parameters to sorption behavior of ciprofloxacin to soil, principle component analysis (PCA) was conducted to reduce the multidimensional data set into three principle components (PCs) (Table 3). Principle component 1 (PC1) accounted for 40.1% of the total variance and was loaded positively by sorption capacity, CEC, clay content, and Kd, and negatively by sand content. Principle component 2 (PC2) accounted for 19.1% of the total variance and was loaded positively by OC and negatively by silt and Koc. Principle component 3 (PC3) accounted for 17.8% of the total variance and was loaded positively by Koc and pH and negatively by silt content. Additionally, CEC, Kd, sorption capacity, and Koc values were each grouped into three ranges that included low, medium, and high values, to reveal potential clustering in regards to PC1 and PC2. PCA showed clear clustering according to clay content (Figure 4A), but limited separation according to soil OC (Figure 4B).
Figure 4. Principal component score plot of selected soil properties and sorption parameters against the first two principle components. (A) clustering of clay content based on low (<20%), medium (20–30%), and high >30%) values plotted against the first two PCs. Similar trends were observed for sorption capacity and distribution coefficient (Kd) (data not shown). (B) clustering of OC content based on low (<1%), medium (1–2%), and high (>2%) values plotted against the first two PCs.
Most studies in the literature used a single ciprofloxacin concentration to determine sorption coefficients of organic chemicals in soil (Kd) (19, 21, 23). The use of three concentrations in this study would improve accuracy of the obtained Kd values considerably. Sorption coefficients obtained based on linear transformations of Langmuir (KL) were marked higher (2-3-fold) than those determined based on the initial linear phase of the sorption isotherms, which could influence data interpretation, or at minimum increase sensitivity in differentiating sorption coefficients in soils of different systems. To be consistent and relevant to data reported in the literature, Kd values were used in further analysis on relationships of interest in this study.
Sorption of organic molecules to soil particles is driven by hydrophobic effect, coulombic attraction resulting in van der Waals forces, or cation bridging. Mechanisms include cation exchange, outer- & inner-sphere adsorption, and precipitation on mineral surfaces (33, 34). Sorption in soil is affected by the chemical nature of the compound and the soil, including functional groups, charge and pH of the reaction mixture, soil CEC, and quantity and types of clay minerals, organic compounds, and metal ions in the soil.
Data obtained in this study suggests that sorption of ciprofloxacin in soil under acidic reaction conditions occurs largely through cation exchange and adsorption driven primarily by cation bridging, evidenced by the predominant influence of clay content to sorption capacity in the tested soils (Figure 3). The three soils (Vaiden, Sumter, and Canisteo) that demonstrated the highest sorption capacity coincided with high clay contents (60, 52.5, and 37.5%, respectively), and high activity clays for two of the soils as reflected by the ratios of CEC to clay fraction (CEC/clay content*100). These ratios were 0.59, 4.1, and 2.1 for Vaiden, Sumter, and Canisteo, respectively (data not shown). The lower ratio in Vaiden may indicate higher proportion of kaolinite in the clay fraction of the soil (34).
The predominant influence of clay minerals through surface complexation, cation-exchange, and cation-bridging to ciprofloxacin sorption is supported by other studies (18, 26). The type of clay plays a critical role. Chen et al. (35) reported that the maximum adsorption of ciprofloxacin for kaolinite (1:1 clay) was 0.026 g kg−1, while montmorillonite (2:1 clay) was 23 g kg−1. The significantly higher (almost 900-fold) sorption capacity of montmorillonite than kaolinite indicated the major role of pH-independent charges over pH-dependent charges to ciprofloxacin sorption in soil. It has been shown that most of the charges in 2:1 clay is due to isomorphous substitution, containing 95% permanent charge and 5% pH-dependent charge, while most of the charges in 1:1 clay is due to broken edges that results in 5% permanent charge and 95% pH-dependent charge (26). Based on the sorption of enrofloxacin, another FQ, to four different clay minerals, montmorillonite had the highest sorption coefficients, and kaolinite had the lowest sorption coefficients (18). However, despite containing a higher proportion of kaolinite in the clay fraction of Vaiden soil, high ciprofloxacin sorption was detected, indicating that clay content also played a critical role in ciprofloxacin sorption in soil.
Ciprofloxacin adsorption to soil particles is closely associated with its functional groups (–COOH and –NH2) and their charges, which are primarily in either a cationic (< pH 6.18), zwitterionic (pH 6.18–8.76), or anionic (>pH 8.76) forms. Under acidic conditions, the cationic form of ciprofloxacin was predominant and the pH-dependent charges of soil organic matter would be predominantly neutral or positive, while those of the broken edges of silica and alumina sheets of clay minerals would be largely neutral. Therefore, the observed strong correlations between sorption capacity and clay contents were contributed mostly by pH-independent charges resulting from isomorphous substitution in the crystalline sheet of one atom by an atom of similar size with lower valence, such as Si4+ is replaced by Al3+. Aside from charges, the influence of clay content and mineralogy on the adsorption of ciprofloxacin in soils can also be attributed to their high specific surface area that facilitated surface complexation. Sorption of ciprofloxacin to illite, another 2:1 clay mineral, was found to be restricted to the external surface and dominated by cation exchange (36). Several studies suggested that cation exchange was a dominant pathway for ciprofloxacin adsorption in soils (19, 21, 26, 27). Due to isomorphic substitution, FQ antibiotics can be exchanged with cations found in the interlayer of expandable clays by the same process as surface cation exchange (37). Evidence of interlayer adsorption of ciprofloxacin has been observed in montmorillonite (22), rectorite (36), and fluorohectorite (38).
Of soil factors, CEC has been identified as a primary factor that influence sorption of organic compounds to soil (19, 27, 39). The CEC for soil organic matter ranges from 250 to 400 meq 100 g−1 while that of pure smectite is 100 cmol(+) kg−1 soil and of pure kaolinite 8 cmol(+) kg−1 soil (40). Since organic matter has CEC more than twice of 2:1 clay minerals and over 25 times of 1:1 clay minerals, a strong relationship between OC and sorption parameters is expected. The relatively greater influence of clay rather than OC to ciprofloxacin sorption in the tested soil signifies that surface complexation, cation-bridging, and interlayer adsorption contributed significantly to ciprofloxacin sorption in soil.
Research suggested that the relationship between OC and sorption of FQs remains unclear (19, 26, 27, 39). Sorption of norfloxacin in soils with SOM removed was higher than that of soils where SOM was retained (24). The observations occurred among diverse soils. The 30 soils used in the studies by Vasudevan et al. (19), Carrasquillo et al. (26), and Jones et al. (39) originated from five different soil series with OC ranging from 0.5 to 89.3 g kg−1. The soils used by Leal et al. (27) originated from six soil orders with OC range of 6.7–213.4 g kg−1. However, the influence of OC is intricate to organic sorption to soil. SOM contains large numbers of the carboxylic functional groups that promotes antibiotic adsorption through hydrogen-bonding, ion-dipole, and aromatic electron donor-acceptor processes. The high CEC from soil organic matter can contribute 30–60% of all CEC sites in mineral soils (41). The inconsistent relationships observed could be due to the presence of multidimensional sorption mechanisms that are influenced by complicated factors, which is further complicated by experimental challenges in investigating sorption behavior, as ciprofloxacin is soluble only in acidic conditions. Conclusions from several previous studies were derived from sorption behavior of the organic compounds in low concentration ranges (19, 26, 39). Although organic compounds in lower solution concentrations might exhibit linear sorption behavior, non-linear behavior is often observed in higher concentrations, which could alter the measured sorption capacity (33). Data from this study is consistent with studies showing a positive relationship between OC and ciprofloxacin sorption with pH-independent charges playing a critical role. The limited relationship between OC and sorption parameters in this and several other studies discussed above could be due to predominant influence of clay minerals as well as organic compounds occupying or physically blocking the sorption sites on clay minerals as suggested by Zhang et al. (24).
Soil management and cultivation history influence SOC content and quality, resulting in influence of sorption behavior of organics in soil. Cultivation generally reduces SOC, while the incorporation of crop residues may lead to increase SOC. Grasslands, pastures, and forests generally have higher SOC than their cultivated counterparts. However, grasslands have less recalcitrant organic materials such as lignin compared to forest litter. Therefore, it is not surprising that ciprofloxacin sorption behavior differed in cultivated and uncultivated soils. The relationship between SOC and sorption capacity or Kd values was significant in cultivated cropland soils but not in the uncultivated ones. This observation is contrary to the sorption behavior of phosalone in soil. Popova et al. (42) reported that uncultivated soils exhibited a linear increase of phosalone binding strength to SOC with the increase of the reciprocal OC content (r = 0.95***), while this relationship was not observed in cultivated soils. The observed divergence cannot be explained by OC content because both uncultivated and cultivated soils had a wide range of OC content in the two studies (0.5–12.6 and 0.4–5.6%, respectively). Therefore, OC quality might have played a critical role. As cultivation progresses, proportion of recalcitrant OC increases. Popova et al. (42) suggested that cultivation led to changes in the sorption behavior of phosalone in soil and altered mechanisms of pesticide sorption in soil. NMR spectra revealed that the quantity and composition of SOC differed considerably between cultivated and uncultivated soils. Cultivation led to increase in O-alkyl carbon fraction of SOC, and proportional decrease in fractions of aryl carbon, O-aryl carbon, and ketones/aldehydes carbon (42). Aside from OC quality, the nature of the sorbed organic compound could be a key player leading to the observed differences in the sorption behavior of ciprofloxacin and phosalone in soil. Ciprofloxacin is primarily in cationic forms under the testing condition, while phosalone is a non-ionic compound. Further, the OC in uncultivated soils contain significant portion of easily decomposable small organic molecules which could bind with negatively charged soil particles and physically blocking the sorption sites as suggested by Zhang et al. (24).
Sorption of ciprofloxacin under acidic reaction conditions occurs largely through surface complexation and cation exchange driven primarily by cation bridging, evidenced by the predominant influence of clay content to sorption capacity in the tested soils. The sorption parameters of ciprofloxacin in soil varied widely, with capacity ranging from 8 to 141 g kg−1, Kd from 23 to 200 mL kg−1 soil, and Koc from 54 to 2,146 mL g−1 OC. For the Freundlich isotherm, Kf ranged from 0.40 to 1.94 g kg−1; and n values ranged from 1.22 to 2.94 mL kg−1. Clay content was the most significant factor that influenced the sorption of ciprofloxacin in the studied soil. CEC had a significant relationship with sorption capacity and Kd in all soils, while SOC had a significant relationship with sorption capacity in cultivated soils only. pH-independent charges in soil played a predominant role dictating sorption parameters of ciprofloxacin. Cation exchange via interlayer adsorption was a primary sorption mechanism under acidic conditions. Cultivation led to changes in the sorption behavior and altered the mechanisms of ciprofloxacin sorption in soil, resulted in a strongly significant relationship between SOC and sorption capacity in cultivated soils, but not in the uncultivated soils. Targeted investigations are warranted to reveal how the nature of organic matter changes through cultivation and influences the sorption of ciprofloxacin in soil. Nevertheless, soils are effective in restraining the mobility of ciprofloxacin and the effectiveness increases with increasing clay content.
The raw data supporting the conclusions of this article will be made available by the authors, without undue reservation.
SD conceived of the presented idea and designed the experiments. MK, JG, and HZ encouraged to investigate this subject and contributed to the experimental design. SD and HZ verified the analytical methods. AP and YW conducted the laboratory analyses. AP and SD performed data analysis, illustration, and interpretation. All authors discussed the results and contributed to the final manuscript.
This work was supported in part by Oklahoma Agricultural Experiment Station (OAES) under project h-OKLO3136, and by OSU Vice President for Research's office. This work was also partially supported by SD Santelmann-Worth endowed professorship award and HZ, Arthur L. Reed endowed chair award.
The authors declare that the research was conducted in the absence of any commercial or financial relationships that could be construed as a potential conflict of interest.
All claims expressed in this article are solely those of the authors and do not necessarily represent those of their affiliated organizations, or those of the publisher, the editors and the reviewers. Any product that may be evaluated in this article, or claim that may be made by its manufacturer, is not guaranteed or endorsed by the publisher.
The Supplementary Material for this article can be found online at: https://www.frontiersin.org/articles/10.3389/fsoil.2022.814924/full#supplementary-material
1. Gustafson RH, Bowen RE. Antibiotic use in animal agriculture. J Appl Microbiol. (1997) 83:531–41. doi: 10.1046/j.1365-2672.1997.00280.x
2. Van Boeckel TP, Brower C, Gibert M, Grenfell BT, Levin SA, Robinson TP, et al. Global trends in antimicrobial use in food animals. PNAS. (2015) 112:5649–54. doi: 10.1073/pnas.1503141112
3. Wang S, Wang H. Adsorption behavior of antibiotic in soil environment: A critical review. Front Environ Sci Eng. (2015) 9:565–74. doi: 10.1007/s11783-015-0801-2
4. Kummerer K. Antibiotics in the aquatic environment—a review—part I. Chemosphere. (2009) 75:417–34. doi: 10.1016/j.chemosphere.2008.11.086
5. Larsson DGJ, de Pedro C, Paxeus N. Effluent from drug manufactures contains extremely high levels of pharmaceuticals. J Hazard Mater. (2007) 148:751–5. doi: 10.1016/j.jhazmat.2007.07.008
6. Fick J, Soderstrom H, Lindberg RH, Phan C, Tysklind M, Larrson DGJ. Contamination of surface, ground, and drinking water from pharmaceutical production. Envion Toxicol Chem. (2009) 28:2522–7. doi: 10.1897/09-073.1
7. Morales-Munoz S, Leque-Garcia JL, Luque de Castro MD. Continuous microwave-assisted extraction coupled with derivatization and fluorimetric monitoring for the determination of fluoroquinolone antibacterial agents from soil samples. J Chromatogr A. (2004) 1059:25–31. doi: 10.1016/j.chroma.2004.09.086
8. US CDC U,.S. (Center for Disease Control, Prevention). Outpatient Antibiotic Prescriptions — United States. (2015). Available online at: https://www.cdc.gov/antibiotic-use/community/pdfs/Annual-Report-2015.pdf (accessed October 9, 2021).
9. Golet EM, Xifra I, Siegrist H, Alder AC, Giger W. Environmental exposure assessment of fluoroquinolone antibacterial agents from sewage to soil. Environ Sci Technol. (2003) 37:3243–9. doi: 10.1021/es0264448
10. Humphrey TJ, Jorgensen F, Frost JA, Wadda H, Domingue G, Elviss NC, et al. Prevalence and subtypes of ciprofloxacin-resistant Campylobacter spp. in commercial poultry flocks before, during, and after treatment with fluoroquinolones. Antimicrob Agents Chemother. (2005) 49:690–8. doi: 10.1128/AAC.49.2.690-698.2005
11. Al-Ahmad A, Daschner FD, Kümmerer K. Biodegradability of cefotiam, ciprofloxacin, meropenem, penicillin g, and sulfamethoxazole and inhibition of waste water bacteria. Arch Environ Contam Toxicol. (1999) 37:158–63. doi: 10.1007/s002449900501
12. Yi K, Wang D, Yang Q, Li X, Chen H, Sun J, et al. Effect of ciprofloxacin on biological nitrogen and phosphorus removal from wastewater. Sci Total Environ. (2017) 605–6:368–75. doi: 10.1016/j.scitotenv.2017.06.215
13. Fu X, Johnson WL, Gustafson JE, Wang Y, Wang X, Chitica I, et al. Ciprofloxacin prophylaxis in emergency response plans would alter bacterial communities in wastewater treatment infrastructure. Environ Eng Sci. (2021) 38:211–23. doi: 10.1089/ees.2020.0316
14. Girardi C, Greve J, Lamshöft M, Fetzer I, Miltner A, Kästner AM. Biodegradation of ciprofloxacin in water and soil and its effects on the microbial communities. J Hazard Mater. (2011) 30:22–30. doi: 10.1016/j.jhazmat.2011.10.004
15. Cui H, Wang SP, Fu J, Zhao ZQ, Zhang N, Guo L. Influence of ciprofloxacin on microbial community structure and function in soils. Biol Fert Soils. (2014) 50:939–47. doi: 10.1007/s00374-014-0914-y
16. Córdova-Kreylos AL, Scow KM. Effects of ciprofloxacin on salt marsh sediment microbial communities. ISME J. (2007) 1:585–95. doi: 10.1038/ismej.2007.71
17. Naslund J, Hedman JE, Agestrand C. Effects of the antibiotic ciprofloxacin on the bacterial community structure and degradation of pyrene in marine sediment. Aquatic Toxicol. (2008) 90:223–7. doi: 10.1016/j.aquatox.2008.09.002
18. Tolls J. Sorption of veterinary pharmaceuticals in soils: a review. Environ Sci Tech. (2001) 35:3397–406. doi: 10.1021/es0003021
19. Vasudevan D, Bruland GL, Torrance BS, Upchurch VG, MacKay AA. pH-dependent ciprofloxacin sorption to soils: interaction mechanisms and soil factors influencing sorption. Geoderma. (2009) 151:68–76. doi: 10.1016/j.geoderma.2009.03.007
20. Carmosini N, Lee LS. Ciprofloxacin sorption by dissolved organic carbon from reference and bio-waste materials. Chemosphere. (2009) 77:813–20. doi: 10.1016/j.chemosphere.2009.08.003
21. Figueroa-Diva RA, Vasudevan D, Mackay AA. Trends in soil sorption coefficients within common antimicrobial families. Chemosphere. (2010) 79:786–93. doi: 10.1016/j.chemosphere.2010.03.017
22. Roca Jalil ME, Baschini M, Sapag K. Influence of pH and antibiotic solubility on the removal of ciprofloxacin from aqueous media using montmorillonite. Appl Clay Sci. (2015) 114:69–76. doi: 10.1016/j.clay.2015.05.010
23. Teixidó M, Medeiros J, Beltrán J, Prat M. Sorption of enrofloxacin and ciprofloxacin in agricultural soils: effect of organic matter. Adsorp Sci Tech. (2014) 32:153–64. doi: 10.1260/0263-6174.32.2-3.153
24. Zhang J, Li ZJ, Ge GF, Sun WC, Liang YC, Wu LS. Impacts of soil organic matter, pH and exogenous copper on sorption behavior of norfloxacin in three soils. J. Environ. Sci. (2009) 21:632–40. doi: 10.1016/S1001-0742(08)62318-9
25. Pan B, Wang P, Wu M, Li J, Zhang D, Xiao D. Sorption kinetics of ofloxacin in soils and mineral particles. Environ Pollut. (2012) 171:185–90. doi: 10.1016/j.envpol.2012.07.037
26. Carrasquillo AJ, Bruland GL, Mackay AA, Vasudevan D. Sorption of ciprofloxacin and oxytetracycline zwitterions to soils and soil minerals: influence of compound structure. Environ Sci Technol. (2008) 42:7634–42. doi: 10.1021/es801277y
27. Leal RMP, Alleoni LRF, Tornisielo VL, Regitano JB. Sorption of fluoroquinolones and sulfonamides in 13 Brazilian soils. Chemosphere. (2013) 92:979–85. doi: 10.1016/j.chemosphere.2013.03.018
28. Bloesch PM. Prediction of the CEC to clay ratio using mid-infrared spectroscopy. Soil Res. (2011) 50:1–6. doi: 10.1071/SR11137
29. Nelson DW, Sommers LE. Total carbon, organic carbon, organic matter. In: Sparks DL, editor. Methods of Soil Analysis. Part 3. Madison, WI: SSSA Book Series (1996). doi: 10.2134/agronmonogr9.2.2ed.c29
30. Gee GW, Or D. Particle size analysis. In Dane JH. Topp GC, editors. Methods of Soil Analysis, Part. 4: Physical Methods. Madison, WI: Soils Science Society of America (2002).
31. Ross D, Ketterings Q. Recommended methods for determining soil cation exchange capacity. in Recommended Soil Testing Procedures for the Northeastern United States, 3rd ed.; Northeastern Regional Publication No. 493. Newark, DE: Agricultural Experiment Station, University of Delaware (2011).
32. Vella J, Busuttil F, Bartolo NS, Sammut C, Ferrito V, Serracino-Inglott A, et al. A simple HPLC-UV method for the determination of ciprofloxacin in human plasma. J Chromatogr B. (2015) 989:80–5. doi: 10.1016/j.jchromb.2015.01.006
33. Strawn DG. Sorption mechanisms of chemicals in soils. Soil Syst. (2021) 5:13. doi: 10.3390/soilsystems5010013
34. Thompson A, Goyne KW. Introduction to the sorption of chemical constituents in soils. Nat Educ Knowl. (2012) 4:7. Available online at: https://www.nature.com/scitable/knowledge/library/introduction-to-the-sorption-of-chemical-constituents-94841002/
35. Chen H, Gao B, Yang LY, Ma LQ. Montmorillonite enhanced ciprofloxacin transport in saturated porous media with sorbed ciprofloxacin showing antibiotic activity. J Contam Hydrol. (2015) 173:1–7. doi: 10.1016/j.jconhyd.2014.11.010
36. Wang CJ, Li Z, Jiang WT. Adsorption of ciprofloxacin on 2:1 dioctahedral clay minerals. Appl Clay Sci. (2011) 53:723–8. doi: 10.1016/j.clay.2011.06.014
37. Sposito G, Skipper NT, Sutton R, Park S.-H., Soper AK, et al. Surface geochemistry of the clay minerals. PNAS. (1999) 96:3358–64. doi: 10.1073/pnas.96.7.3358
38. dos Santos EC, Gates WP, Michels L, Juranyi F, Mikkelsen A, da Silva GJ, et al. The pH influence on the intercalation of the bioactive agent ciprofloxacin in fluorohectorite. Appl Clay Sci. (2018) 166:288–98. doi: 10.1016/j.clay.2018.09.029
39. Jones AD, Bruland GL, Agrawal SG, Vasudevan D. Factors influencing the sorption of oxytetracycline to soils. Environ Toxicol Chemm. (2005) 24:761–70. doi: 10.1897/04-037R.1
40. Moore G. Soilguide: A Handbook for Understanding and Managing Agricultural Soils. Agriculture Western Australia (1998).
41. Loveland P, Webb J. Is there a critical level of organic matter in the agricultural soils of temperate regions? A review. Soil Till Res. (2003) 70:1–18. doi: 10.1016/S0167-1987(02)00139-3
Keywords: antibiotic, ciprofloxacin, sorption, soil, cultivation, organic matter
Citation: Pasket A, Zhang H, Wang Y, Krzmarzick M, Gustafson JE and Deng S (2022) Clay Content Played a Key Role Governing Sorption of Ciprofloxacin in Soil. Front. Soil Sci. 2:814924. doi: 10.3389/fsoil.2022.814924
Received: 14 November 2021; Accepted: 27 January 2022;
Published: 22 February 2022.
Edited by:
Shan-Li Wang, National Taiwan University, TaiwanReviewed by:
Ali El-Naggar, Ain Shams University, EgyptCopyright © 2022 Pasket, Zhang, Wang, Krzmarzick, Gustafson and Deng. This is an open-access article distributed under the terms of the Creative Commons Attribution License (CC BY). The use, distribution or reproduction in other forums is permitted, provided the original author(s) and the copyright owner(s) are credited and that the original publication in this journal is cited, in accordance with accepted academic practice. No use, distribution or reproduction is permitted which does not comply with these terms.
*Correspondence: Shiping Deng, c2hpcGluZy5kZW5nQG9rc3RhdGUuZWR1
Disclaimer: All claims expressed in this article are solely those of the authors and do not necessarily represent those of their affiliated organizations, or those of the publisher, the editors and the reviewers. Any product that may be evaluated in this article or claim that may be made by its manufacturer is not guaranteed or endorsed by the publisher.
Research integrity at Frontiers
Learn more about the work of our research integrity team to safeguard the quality of each article we publish.