- 1Savanna Agricultural Research Institute, CSIR, Tamale, Ghana
- 2Department of Soil Science, School of Agriculture, College of Basic and Applied Sciences, University of Ghana, Accra, Ghana
- 3International Fertilizer Development Center (IFDC), Muscle Shoals, AL, United States
- 4Fertilizer Research and Responsible Implementation Program, International Fertilizer Development Center, Accra, Ghana
The nitrates produced after mineralization from compost may be prone to leaching, especially in tropical sandy soils, because of the increased rate of nitrification and the porous nature of such soils. This may result in low nitrogen (N) use efficiency and adverse environmental effects. Inorganic nitrification inhibitors are costly and mostly unavailable in Ghana. Research on simple but effective local materials for use as nitrification inhibitors is therefore a priority. Two such materials are neem materials and biochar. Neem materials can suppress nitrifying bacteria due to their antimicrobial properties. Biochar can hold ammonium in the soil, making it temporarily unavailable to nitrifying bacteria. This study aimed to determine the efficacy of neem materials and biochar as nitrification inhibitors and their influence on nitrate leaching. In preliminary studies: 1) pot incubation was conducted for 60 days to estimate the nitrification rate with manure, compost, and NH4Cl as the N source (150 kg N/ha) in one set and neem seeds, bark, and leaves (1.25 µg azadirachtin/g) in another set, using nitrate concentrations; and 2) the ammonium sorption and desorption capacities of sawdust, rice husk, and groundnut husk biochar were determined. In the main study, pot incubation with compost as the N source but treated with milled neem seeds or bark (1.25 µg azadirachtin/g) or sawdust biochar (20 t/ha) was conducted for 60 days, in which the nitrification inhibition was determined using nitrate concentrations. A leaching experiment in columns with similar treatments and maize sown was then conducted to quantify the nitrate in leachates. A high nitrification rate was recorded in compost-amended soil, almost half that of the standard (NH4Cl). The use of sawdust biochar, which showed the highest ammonium sorption and desorption capacity, resulted in 40% nitrification inhibition that lasted the entire incubation period. The use of neem seeds with an azadirachtin concentration of 3.92 mg/g resulted in a similar nitrification inhibition, but this only lasted 40 days. Inhibition caused by both materials resulted in about a 60% reduction in nitrate leached. Thus, neem seeds (498 kg/ha) and sawdust biochar (20 mt/ha) could be used to control nitrate leaching for short-duration and long-duration crops, respectively.
1 Introduction
Soils in sub-Saharan Africa are low in nitrogen (1), but the plant N requirement, as compared to many other nutrients, is very high. The management of soil N is therefore critical for crop production in sub-Saharan Africa, where soil fertility decline, primarily due to soil nutrient mining, has been identified as a key constraint to agricultural productivity (2). The low N in these soils has been attributed to a number of reasons; key among them is the rapid rate of mineralization due to increased atmospheric temperature, which leads to significant N losses. Thus, a normal farming practice is to supply N to soils through organic or inorganic fertilizer application or other agronomic cultural techniques (e.g., cultivation of legumes) in order to achieve high crop yields (3).
Concerns have been raised, however, about the cost, availability, and environmental friendliness of inorganic fertilizers (4, 5). Consequently, the application of organic fertilizers to farmlands has become an economical alternative and provides a more sustainable mechanism for increasing crop production (5). Organic fertilizers increase crop yields by providing large amounts of nutrients and organic matter (5). These organic fertilizers contain both mineral N (which may be immediately available) and a large amount of organically bound N, which undergo a microbially mediated mineralization process in which ammonium () and nitrate () are released in inorganic and soluble forms that can be utilized by plants (6). The soluble and mobile N components of organic fertilizers are important for consideration in terms of leachability in the soil, especially during rain events (7). When water infiltrates 2.5 cm of soil, it moves the 2.5 and 6.5 cm downward in clayey loam and sandy soils, respectively (8). Thus, during periods of heavy rainfall, leaching may move out of the effective rooting zone of plants (8). Nitrate leaching after organic fertilizer application may even be more intense in soils such as Ferric Luvisols due to the rapid rate of mineralization caused by soil and climatic conditions. A wide variation (from 0.2 to over 200 kg N/ha) in the amount of soil N leached on the African continent has been found, and these values are inconsistent with the N inputs, e.g., high leaching even under zero fertilizer application (9). Fertilized fields have also been found to contain a three to four times higher concentration in underground water than under the unfertilized control (9). This results in a low N use efficiency, which affects crop production and causes some environmental issues. Like other sub-Saharan African countries, Ghana is faced with the problem of low soil N (10), and attempts to improve the situation may bring up the issue of soil N leaching.
Many researchers have seen this as a problem when inorganic fertilizer is involved, but not with organic fertilizer, with the explanation of a slow N release (11). However, this may not always be the case because nitrate leaching from agricultural soils is a complex process closely related to local environmental factors, such as soil characteristics and climatic variables (12), and farm management practices in intensive agriculture. The use of inorganic nitrification inhibitors applied elsewhere to control such situations may not be practical in Ghana because of cost and availability concerns. Accordingly, investigation into the use of local materials that are readily available with few or no competing demands is needed. Two such materials are neem materials and biochar. Neem materials delay the bacterial oxidation of to (nitrification) (13, 14) by suppressing the nitrifying bacteria involved in the process due to their antimicrobial properties (azadirachtin). In microorganisms, azadirachtin inhibits proliferation and monolayer formation and reduces the rates of protein synthesis, which finally leads to cell death (15). Recent studies by Altayb etal. (16) and Kebede etal. (17) have reported on the strong antibacterial activity of neem materials against different types of bacteria. Several studies have also been conducted to evaluate the efficacy of aromatic plant materials (18), neem seed cake and oil (19), and karanja seed powder (20) in suppressing the nitrification of urea. Meena etal. (21) even reported that the Government of India has mandated the production and distribution of neem-coated urea in place of normal urea across the country, as a responsible and sustainable model. Biochar has also been shown to significantly influence almost all forms of N, especially , either directly or indirectly (22, 23). Several mechanisms have been proposed for biochar– interaction, including higher pore space and surface area (24), oxygen-containing functional groups due to short- and long-term oxidation (25, 26), sorption due to ion exchange, sorption via chemosorption ammonia fixation, ion exchange, with columbic forces or an association with S-functional groups (27, 28), chemistry of surface functional groups (29), and the physical adsorption (van der Waals adsorption) of onto the biochar surface (30). Based on these established interactions, biochar can hold in the soil to make it temporarily unavailable to nitrifying bacteria, thus slowing the rate of nitrification and subsequently reducing the amount of nitrate leached.
The reduction of soil leaching using these simple materials is therefore a priority area of research due to its agricultural, financial, health, and environmental relevance. The main objective of this study was to determine the efficacy of either neem seeds, bark, and leaves or biochar when each is used as a nitrification inhibitor, their influence on the amount of nitrate leached from a compost-amended Ferric Luvisol, and their impact on some agronomic performance indices with maize as a test crop and on the residual soil.
2 Materials and methods
2.1 Soil used
This study used a Varempere series soil. Adu and Asiamah (31) classified this soil as a Ferric Luvisol. Ferric Luvisol is the most dominant soil in the Ghanaian portion of the Black Volta River basin, which lies between latitude 7°00'00'' N and 14°30'00'' N and longitude 5°30'00'' W and 1°30'00'' W (32). The soil is very deep (>150 cm) and sedentary and developed from granite. It is moderately well drained, brownish yellow to yellowish red in color, with a sandy clay loam to a sandy clay texture, and is found on gentle slopes. The top soil (0–25 cm) is dark brown, a weak fine granular loamy sand, is non-sticky and non-plastic, and is overlying the thick (25–155 cm) brownish yellow to yellowish red subsoil. The subsoil has a moderate to strong medium subangular blocky structure, is faintly mottled, has a sandy clay to gritty clay texture, is slightly sticky, and is slightly plastic in consistency. At 155–175 cm, it has a firm structure, with a few to more common iron, manganese dioxide nodules, and quartz stones and gravels.
2.2 Soil sampling
Surface soil (0–20 cm) was sampled from an uncultivated land at Bawku, the capital town of Bawku Municipal District in the Upper East Region of North Ghana. Bulk soil samples were brought to the laboratory, air-dried, and then sieved with a 2-mm sieve for chemical and physical analyses.
2.3 Preliminary studies
2.3.1 Incubation studies
Each incubation study was carried out in Sinna’s garden of the College of Basic and Applied Sciences of the University of Ghana. The objective of the first incubation study was to determine whether the potential rate of nitrification in a compost or cow dung manure-amended soil is rapid enough to warrant considering leaching control measures. The compost was prepared basically from market and household waste materials, and the cow dung manure was collected from the Livestock and Poultry Research Centre of the University of Ghana. A total of 500 g of soil samples was weighed into plastic pots, and substances such as stones and roots were removed. The soil was neither air-dried nor sieved in order to minimize disturbance of microbial activity. The soils were either mixed with 2 g of the compost (150 kg N/ha), 2 g of the manure (150 kg N/ha), or 0.12 g of NH4Cl (150 kg N/ha); the control treatment received no amendment. Soil moisture content was maintained at 70% water-holding capacity and incubated. The incubation was carried out in a dark chamber at a temperature range of 28°C–32°C under aerobic conditions for 60 days. This experiment was conducted according to a completely randomized design with three replications. Values of mineralized were determined at the start of the experiment and after 10, 20, 30, 40, 50, and 60 days of incubation by destructive sampling.
The equation developed by Crawford and Chalk (33) was used to calculate potential nitrification rates (n), as follows:
where Dt2 is the number of days from the start of incubation to time 2 and Dt1 is the number of days from the start of incubation to time 1.
A similar 60-day incubation study was set up after the first one to determine and account for potential mineralizable nitrate from neem leaves, bark, and seeds to be used as inhibitors in a subsequent study. The results were also used to establish the suitability or otherwise of neem leaves and bark for use as substitutes for the well-documented neem seeds when out of season. The materials harvested from neem trees on the Legon Campus of the University of Ghana were completely air-dried and milled into a fine form. Methanolic extraction of azadirachtin was performed, and filtrates were sent to the Ghana Standard Authority Pesticide Laboratory for the determination of azadirachtin concentration using the 6420 Triple Quad Liquid Chromatography Mass Spectrometer (LC/MS) and a MassHunter WorkStation software (Agilent Technologies, Santa Clara, CA, USA). The pots of soil were treated with 1) 0.16 g of milled neem seeds, 2) 12.50 g of milled neem bark, or 3) 20.80 g of neem leaves, each containing 1.25 µg azadirachtin/g soil, based on the conclusion made by Sarawaneeyaruk etal. (34) that 1.25 µg azadirachtin/ml is the minimum concentration of azadirachtin needed for microbial inhibition. The unamended soil served as the control. Nitrate () was determined at 10-day intervals.
2.3.2 Sorption and desorption study
An ammonium sorption and desorption study was conducted by preparing concentrations of 0, 5, 10, 15, 20, 25, 30, and 40 mg /L from (NH4)2SO4 in a CaCl2 background solution to attain an ionic strength of 0.01 M CaCl2. The purpose of this study was to determine the biochar type with the highest sorption and desorption ability that can be used as a nitrification inhibitor in the subsequent inhibition study. Three feedstocks with no local competing demand—rice husk, sawdust, and groundnut husk—were selected for pyrolysis from the Soil and Irrigation Research Centre (SIREC) of the University of Ghana, a sawmill, and a farm at Bawku in the Northern Region of Ghana, respectively. The feedstocks were air-dried to a moisture content of less than 10%, and all foreign materials were removed. Charring was done in batches for each feedstock using a locally manufactured kiln (kuntan kiln), and a series of charring temperatures were recorded during each batch using an infrared thermometer. The average of these temperatures was calculated as the charring temperature for each biochar type. A total of 2 g of rice husk biochar (RHB), groundnut husk biochar (GHB), and sawdust biochar (SDB) (charred at 480°C, 440°C, and 460°C, respectively) was weighed into each solution and shaken for 30 min twice a day (at 9 a.m. and 4 p.m.) at 125 oscillations per minute for 6 days. The solutions were filtered, and 5 ml of 40% NaOH was added to a 5-ml aliquot from each and distilled. The distillates were titrated against 0.01 M HCl. The amount of sorbed by the various biochar types was calculated as follows:
where C0 and Ce are the initial and equilibrium ammonium concentrations, respectively (in milligrams per liter); v is the volume of the solution (in liters); and m is the mass of biochar (in grams) (35).
A desorption study was also conducted using the residual samples at the highest concentration level (40 mg/L). After centrifugation at 3,500 rpm for 10 min at room temperature, wet residues were shaken with 20 ml of 0.01 M KCl solution for 3 h. A 5-ml aliquot of the supernatant was taken for the determination of . The extraction was repeated two more times, and the released into the supernatant at each extraction period was measured. The total amount desorbed was the summation of the amount desorbed at each of the three extractions.
2.3.3 Inhibition study
A third similar 60-day incubation study was conducted with 2 g of compost (as the N source) mixed with 1) 0.16 g of milled neem seeds (1.25 µg azadirachtin/g soil), 2) 12.50 g of milled neem bark (1.25 µg azadirachtin/g soil), 3) 30.1 g sawdust biochar (20 mt/ha) (36), or 4) 5.9 g dicyandiamide (DCD; 1.25 µg a.i./g soil). The purpose of this study was to determine the percentage of nitrification inhibition caused in a compost-amended soil treated with either neem seeds, neem bark, or sawdust biochar. The neem leaves were dropped at this stage because the results from the earlier study revealed a high amount of N mineralized from the leaves. This defeats the basic principle underlining the inhibition (i.e., reducing the amount of available N susceptible to leaching). Soil mixed with compost only served as a control. Mineralized nitrate () was determined at the start of the experiment and after 10, 20, 30, 40, 50, and 60 days of incubation by destructive sampling.
The percentage of nitrification inhibition (NI%) was also calculated using the equation by Crawford and Chalk (33).
NI is either neem seed, neem bark, or biochar.
2.4 Main study
2.4.1 Setup for leaching
Acrylic cylinder columns with a diameter of 16 cm (radius, 8 cm) and height of 40 cm were used. The bottoms of the columns were covered with Whatman no. 42 filter paper, followed by a nylon mesh of 25 µm pore size. The filter paper and nylon mesh were secured at the mouth with circular metal clips to prevent soil particles from falling. A depth of 10 cm from the top of the height of each column was left for water after saturation. The remaining 30 cm was divided into two exact parts (15 cm each) for soil sampled from 20–40 cm (at the bottom) and from 0–20 cm (on top). A soil mass of 4.9 kg was poured first into the columns (according to its bulk density of 1.54 Mg/m3) and was packed to the 15-cm mark by gently tapping the sides of the cylinders carefully. Water was added to reach 70% field moisture capacity. Then, 4.7 kg (according to its bulk density of 1.56 Mg/m3) of topsoil (0–20 cm) was thoroughly mixed with compost at 150 kg N/ha and treated with 1) milled neem seeds (1.25 µg azadirachtin/g soil), 2) milled neem bark (1.25 µg azadirachtin/g soil), 3) sawdust biochar (20 mt/ha), and 4) DCD (1.25 µg a.i./g soil) or 5) left unamended. Each was packed to the 15-cm mark by gently tapping the sides of the cylinder carefully. Treatments were left for 1 week (7 days) to equilibrate (during this period, the moisture content was maintained at 70% field capacity), after which five seeds of the Obaatanpa maize variety (test crop) per cylinder were sown and thinned to three plants per cylinder after germination. All treatments were replicated four times and completely randomized. On days 30 and 50 after amendment, the moisture content of the soils was brought above 100% field capacity in order to leach soils completely with deionized water, and the leachate was collected into a 1,000-ml conical flask placed under the columns for the determination of concentration in each.
2.4.2 Plant materials
The maize plants were harvested 10 weeks after planting. The chlorophyll content in the leaves was determined using the Apogee CCM-200 plus chlorophyll content meter (Apogee Instruments, Logan, UT, USA) before harvesting. The harvested plants were separated into shoots and roots and dried in an oven at a temperature of 68°C for 48 h. The weight of the dried samples was then taken as the dry matter weight.
2.5 Statistical analysis
All data were subjected to analysis of variance (ANOVA). The statistical package used was GenStat 2012 version. The least significance difference method was used for the mean separation at the 5% level of probability.
3 Results and discussion
3.1 Preliminary studies
3.1.1 Soil used
Some of the physiochemical properties of the soil used for the study are shown in Table 1. The soil had a high sand content of 80.8%, with silt and clay contents of 12.9% and 6.3%, respectively. The soil is classified as a loamy sand according to the U.S. Department of Agriculture (USDA) system. It has a high bulk density of 1.59 Mg/m3 and a low moisture content at field capacity of 16.1%. The pH of the soil in water was near neutral, with a value of 6.5. A low organic carbon content of 0.34% was recorded, with very low total nitrogen and available phosphorus contents of 0.02% and 7.41 mg/kg, respectively. The low value of organic carbon would not maintain a sustainable crop yield, as Garten and Wullschleger (37) documented that the critical value is 1%. The low organic carbon value for the soil could be attributed to the sparse grass vegetation covering the soil. The low available nitrogen recorded could be due to the effect of the intense leaching of nitrate as a result of the sandy nature of the soil. The calcium concentration was 1.34 cmol/kg, and sodium had the lowest concentration of 0.01 cmol/kg. All of these physical and chemical characteristics are consistent with what have been reported by numerous researchers, including Nketia etal. (38) and Adu and Asiamah (31).
3.1.2 Potential nitrification rate after compost or manure amendment
The results for the nitrate concentrations at 10-day intervals for the incubation period were used to calculate the overall potential nitrification rate, as shown in Figure 1. The highest rate of nitrification was recorded in the NH4Cl-amended soil (10.4 µg g−1 day−1). This was expected because of the readily available released into the solution upon the application of NH4Cl. The manure- and compost-amended soils had insignificantly (p > 0.05) different rates of 4.4 and 4.6 µg g−1 day−1, respectively, representing about half the rate of the NH4Cl-amended soil. The similarity in the properties (e.g., total N, CN ratio, and lignin and phenol contents) of the two materials, as shown in Table 2, may have accounted for their nitrification rates not being significantly different.
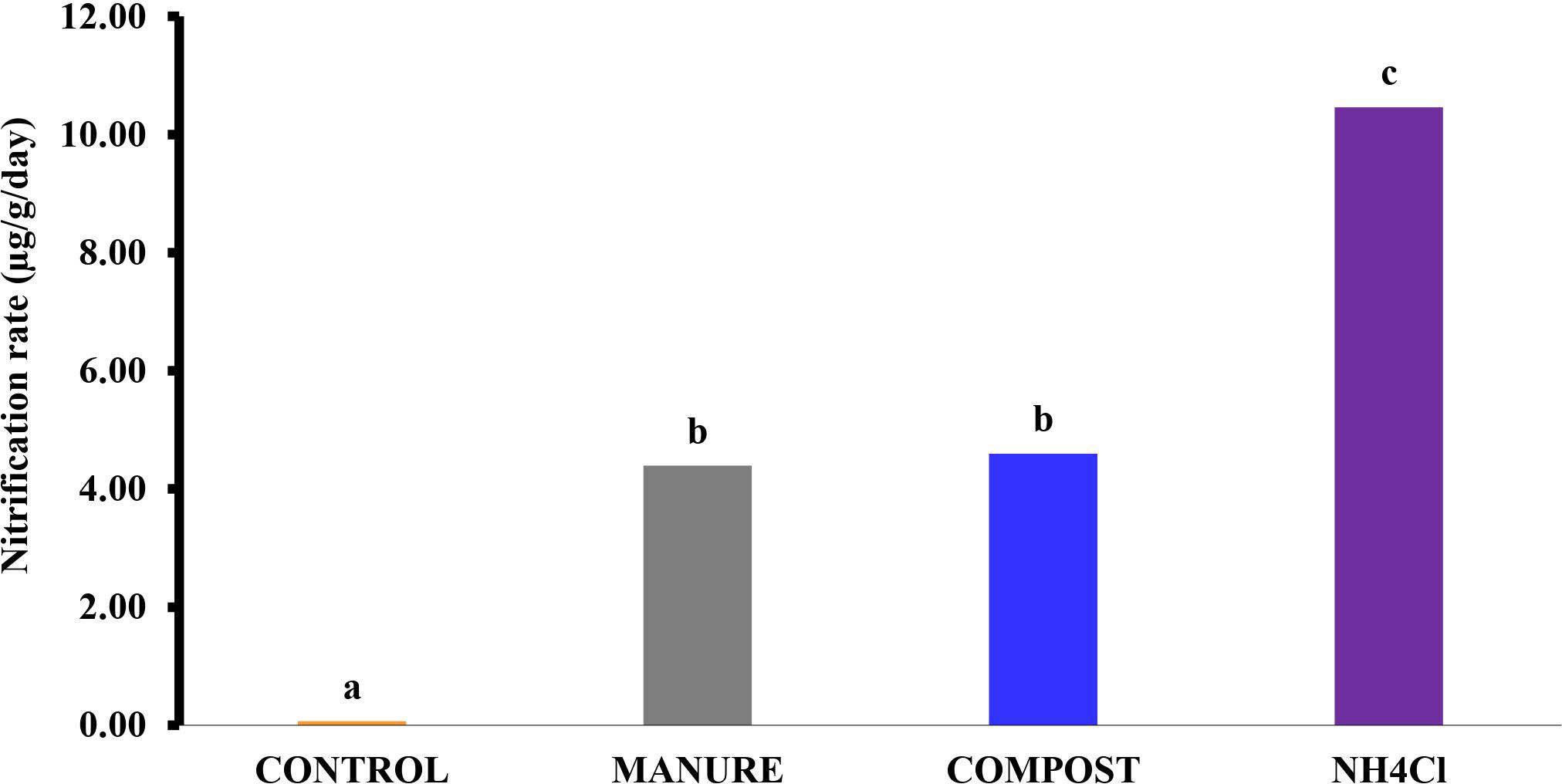
Figure 1 Potential nitrification rate in each amended soil after the incubation period. *Values followed by different letters above the bar are significantly different at the p < 0.05 level.
This suggests a relatively high-potential nitrification rate in the manure- and compost-amended soils. The physiochemical properties of the soil used, as shown in Table 1 (especially pH and texture), could be attributed to the rapid mineralization of the materials. This supports the findings of Chèneby etal. (39), who reported a high amount of labile N fractions of compost (34%) in sandy soils. The quality of the compost and manure (Table 2) may have also contributed to this observation. Similarly, a high rate of mineralization was observed by He etal. (40), who recommended that the application rates of composts similar to biosolids, which contain a high N concentration, should be adjusted for high N release in order to minimize the risk of leaching into groundwater. This result therefore formed the basis for consideration of N leaching control.
3.1.3 Azadirachtin concentrations and mineralizable N of neem materials
The azadirachtin concentration was found to be highest in the neem seeds (3.92 mg/g), which was significantly different from that in the leaves (0.03 mg/g) and the bark (0.05 mg/g), as shown in Table 3. The higher azadirachtin concentration in the seeds, as compared to the leaves and bark, is consistent with what has been widely reported in the literature (41): the secretory cells for the synthesis of azadirachtin are more abundant in the seeds than in the other parts. Therefore, based on the active ingredient, one would need 80 times as much leaves or bark, compared to seeds, to perform the same function.
The concentrations of in the soil after amendment with neem leaves, bark, or seeds are shown in Figure 2. There was a significantly (p< 0.05) higher concentration of in the soil amended with neem leaves (57.8 µg/g) compared to those amended with seeds (25.6 µg/g) and bark (16.7 µg/g) and the control (4.1 µg/g).
The highest concentration of total N found in the neem leaves, as well as the relatively low values of some of the other properties, such as the C/N ratio and the lignin and phenol contents (as shown in Table 2), might account for the high mineralizable N. Therefore, for the purpose of nitrification inhibition, neem leaves may not be appropriate as the high amount of mineralizable N from the material defeats the principle involved in its use. This result formed the basis for the exclusion of neem leaves as an inhibitor in the subsequent incubation study.
3.1.4 Sorption and desorption study
The properties of the three biochar types are shown in Table 4. The results revealed similarities in the key sorption- and desorption-determining properties, such as pyrolysis temperature and cation exchange capacity (CEC), among the biochar types.
Differences in the pH of the equilibrium solution of the three biochar types could thus have contributed to the differences in the amount of sorbed. These different pH values might have resulted in either a competitive action (low pH of RHB), volatilization (high pH of GHB), or enhanced sorption (near-neutral pH of SDB). SDB sorbed the highest amount of , while RHB sorbed the least (Figure 3). The woody nature of sawdust (making it more porous) relative to its agricultural waste counterparts may also explain the relatively higher sorption capacity of SDB. The higher porous nature of SDB further corroborated its relatively higher ease of desorbability. Thus, upon amendment to soils, SDB would make more bioavailable by acting as a buffer for storage and release into the soil solution. Based on this, SDB was selected for use as the nitrification inhibitor in the subsequent inhibition study.
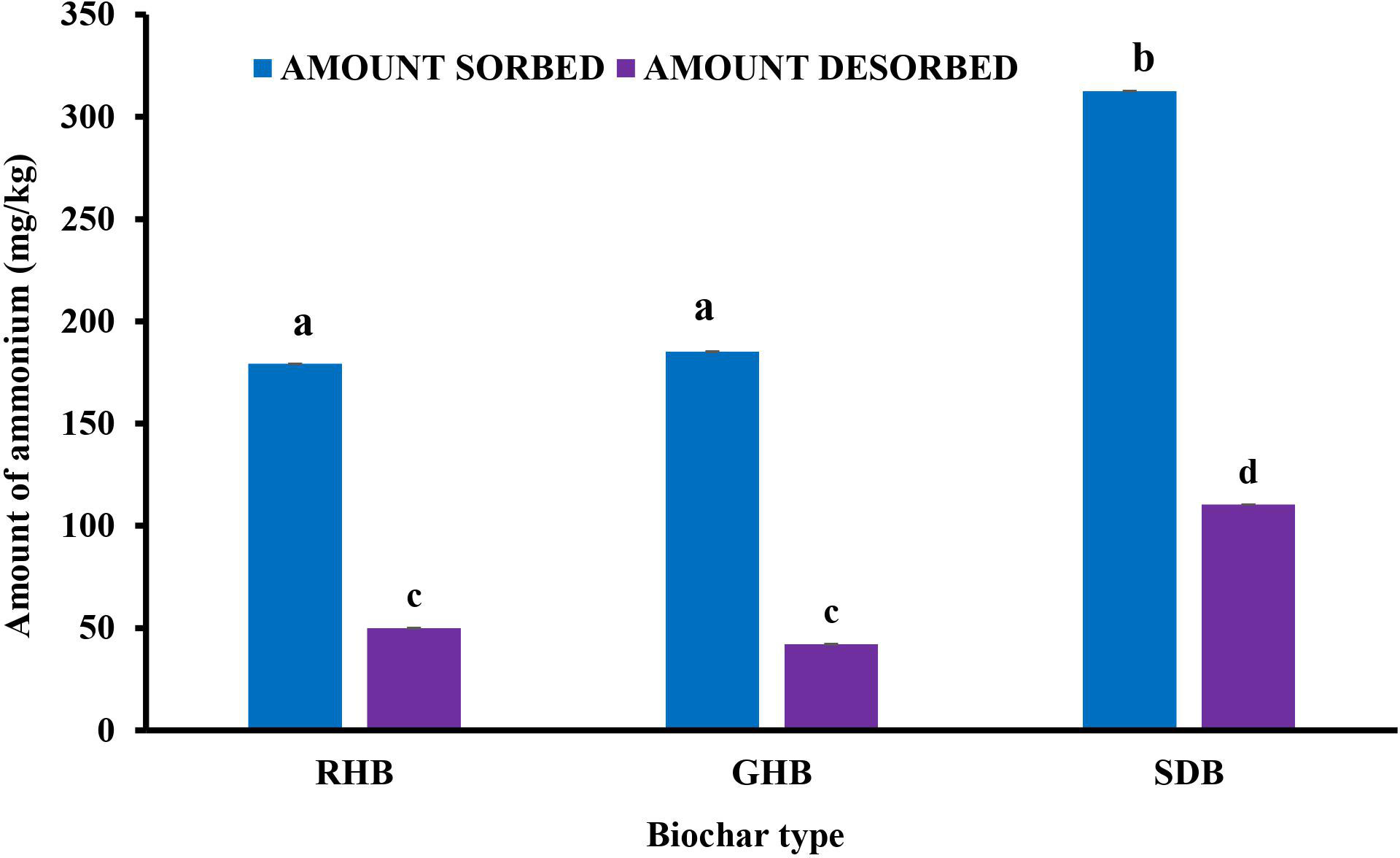
Figure 3 Amount of sorbed and desorbed for the three biochar types at 40 mg/L. *Values followed by different letters above the bar are significantly different at the p < 0.05 level.
3.1.5 Inhibition study
Figures 4, 5 show the effects of neem seeds, neem bark, SDB, and DCD treatments on and mineralization in a compost-amended soil. The greatest effects were observed in the neem seed and SDB treatments.
These results, translated into evidential similar percentage inhibition in each treatment, are shown in Figure 6. The highest percentage of inhibition was recorded for the seed treatment until day 30, after which the SDB treatment took the lead. The inhibition caused by the neem materials may be attributed to the delay they cause in the bacterial oxidation of to (nitrification) (14) by suppressing the nitrifying bacteria involved in the process due to the azadirachtin.
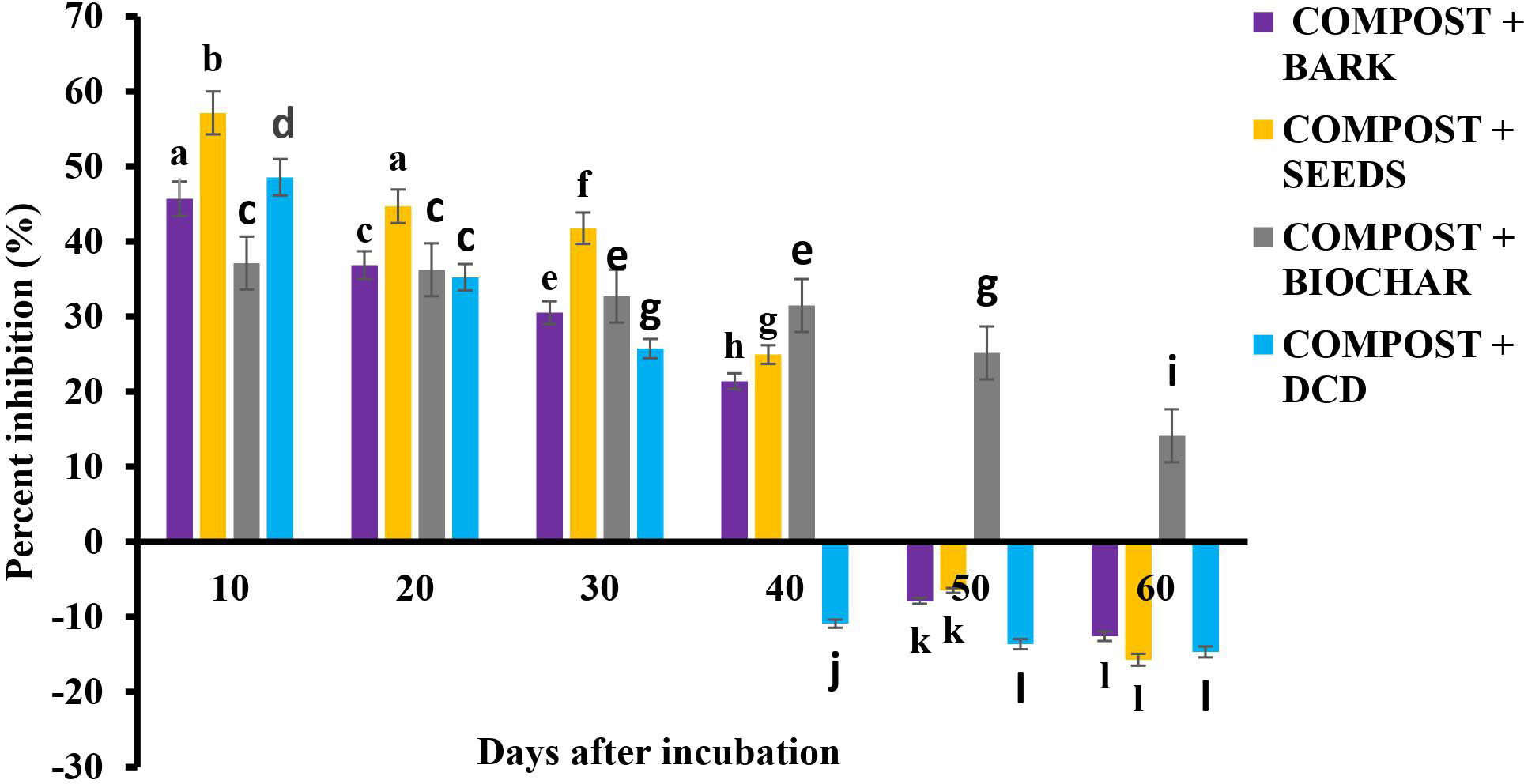
Figure 6 Percentage of nitrification inhibition over time after amendment. *Values with different letters are significantly different at the p < 0.05 level.
The antimicrobial effect of the treatments using neem materials was evident in the results showing the total nitrifying bacteria population after application (Figure 7). A similar nitrification inhibition by blending neem cake with urea has also been observed and reported by several researchers (42, 43). The combined effect of azadirachtin and the oil in the neem seeds might account for the highest rate of inhibition. However, the inhibitory property of the neem materials was short-lived (40 days), after which negative inhibition rates were recorded. The inhibitory effect of SDB, on the other hand, continued throughout the incubation period. This could be attributed to the inert or recalcitrant nature of biochar in the soil, which gives it the ability to resist decomposition for a long period of time. The high amount of recorded in the residual soil (Table 6) explains its inhibition mechanism of holding onto . Therefore, neem seeds could be used for nitrification inhibition in short-duration crops, such as cereals, vegetables, and other grain crops. Sawdust biochar, however, may be appropriate for nitrification inhibition in long-duration crops, such as tree crops.
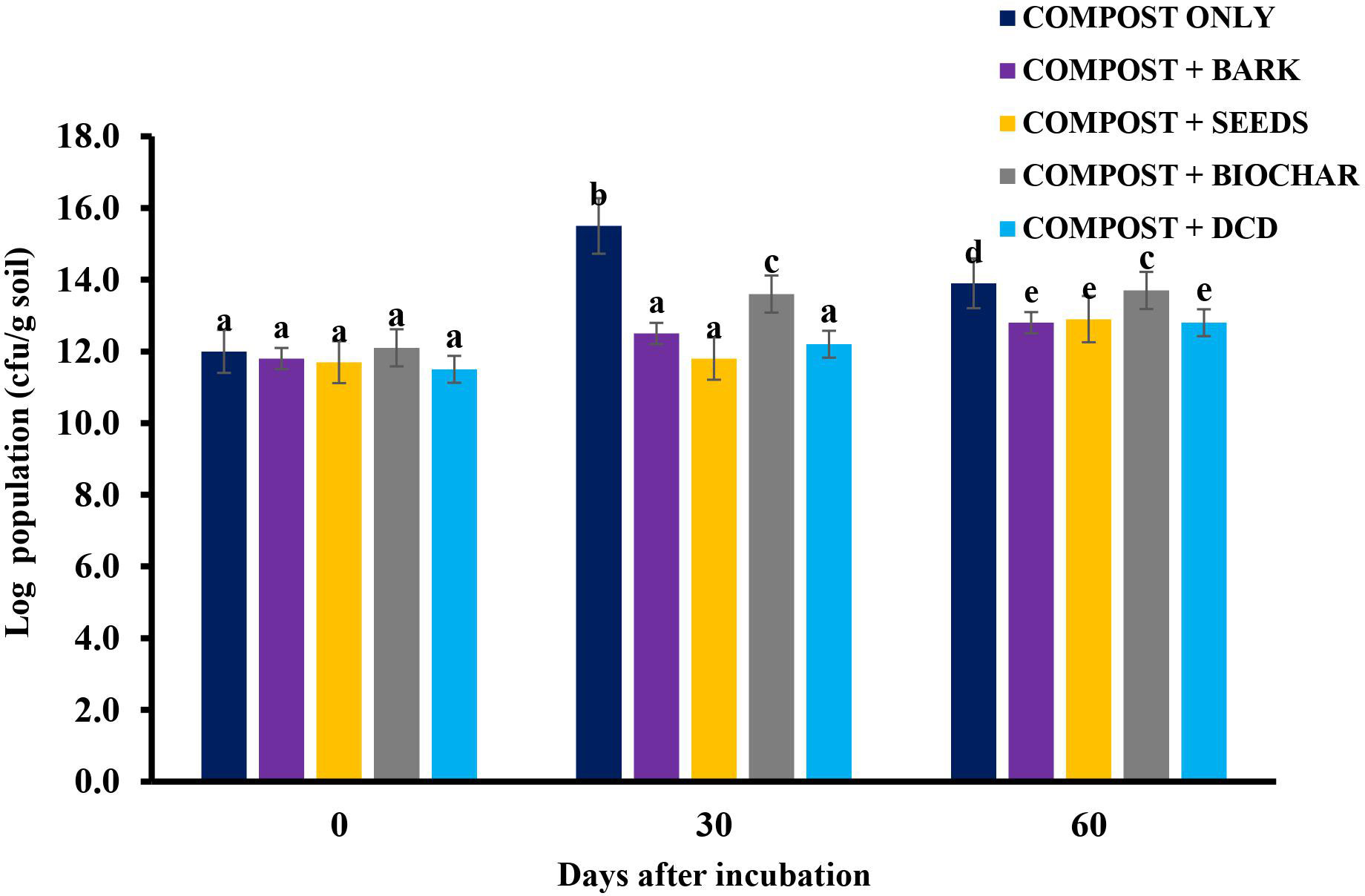
Figure 7 Total nitrifier population in the soil over time after amendment. *Values with different letters are significantly different at the p < 0.05 level.
3.2 Leaching study
The results from both leaching days (3 and 6 weeks after sowing) are shown in Figure 8. The results indicated that the highest amount of nitrate in the leachates came from the compost-only soil. This result was contrary to the conclusion drawn by Amlinger et al. (44) that, in lysimeter experiments, there is no increase in the leaching of N as a result of compost application. The contradictory results might be due to differences in the climatic (Irish) and soil (loamy) conditions of their work. The results further revealed similar nitrate contents of 28.48, 28.67, and 27.32 µg/g in the leachates from the soils treated with neem seeds, neem bark, and SDB, respectively, on the first leaching day. These values were not significantly (p > 0.05) different from each other, but not the standard (DCD). On the second leaching day, however, significantly lower amounts of nitrate were recorded in the neem seed and SDB treatments relative to the other treatments. The inhibitory ability of these materials, as discussed earlier, explains these observations. Additionally, characteristics such as the oil present in neem seeds (45) and the high water-holding capacity of biochar (46, 47) could also have played a role in enhancing their effectiveness as leaching control materials.
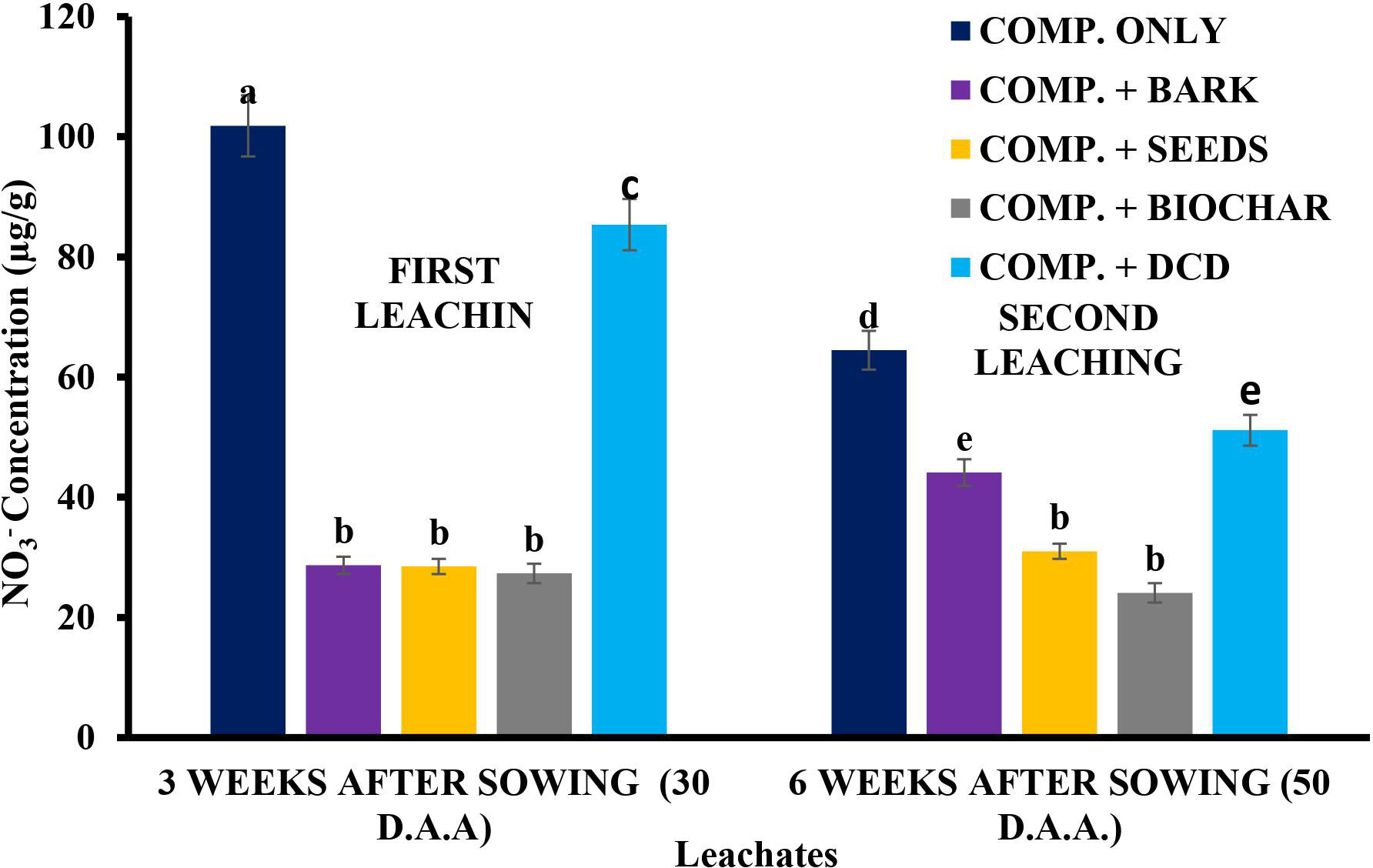
Figure 8 Nitrate concentration in leachates from the first and second leaching determinations. *Values with different letters are significantly different at the p < 0.05 level. D.A.A., days after amendment.
3.2.1 Chlorophyll content of leaves and the shoot and root dry weight of maize
The results for the chlorophyll content of leaves, as well as the shoot and root dry weight, of the maize plants 10 weeks after planting are shown in Table 5. For each of these agronomic parameters, maize plants that received neem seed and SDB treatments registered higher values relative to those given other treatments. The lower amount of lost in these treatments, which may have resulted in a higher uptake, could explain these results. This is because the chlorophyll content has been found to be approximately proportional to the shoot nitrogen content (48) since nitrogen is directly involved in the synthesis of chlorophyll (49). Nitrogen has also been found in molecules such as adenosine triphosphate (ATP), nicotinamide adenine dinucleotide hydrogen (NADH), nicotinamide adenine dinucleotide phosphate hydrogen (NADPH), storage proteins, nucleic acids and enzymes (50), cytochrome molecules, and chlorophyll (51), providing evidence that nitrogen is directly related to plant development and productivity. Figure 9 gives a visual evidence of treatment induced differences in plant development.
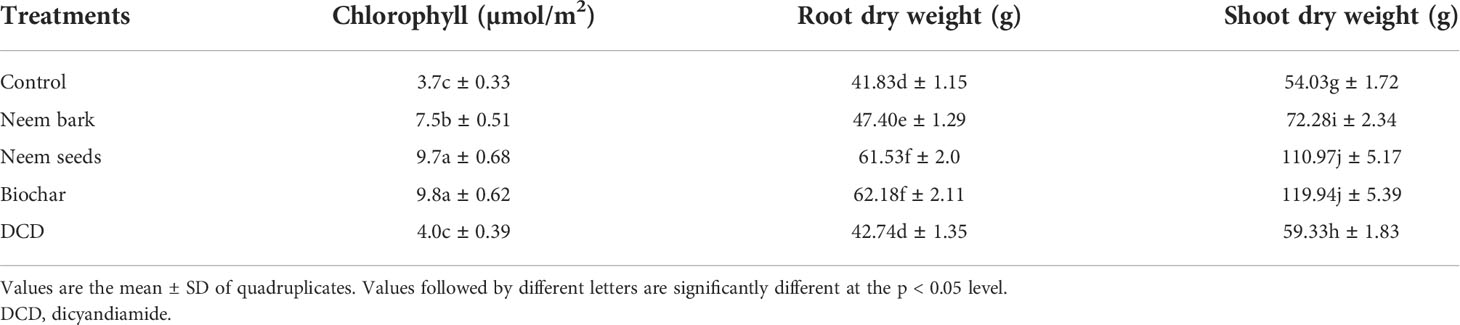
Table 5 Leaf chlorophyll content and root and shoot dry weight of maize plants 10 weeks after planting.
3.2.2 Residual soil available N
The nitrate and ammonium concentrations in the residual soil of all treatments are shown in Table 6. All treatments had a significantly higher amount of relative to the control. This may be attributed to the lower amount of loss recorded compared to the control. The increase in release because of the reduced inhibitory effect of these materials may also explain this observation. Soil from the SDB treatment, however, had the least amount of , but nearly double the amount of , compared to soils from the other treatments. This supports the reported ability of biochar to interact with by sorption (30), making it temporarily unavailable to nitrifying bacteria.
4 Conclusion and recommendations
1. A high rate of nitrification occurs in manure- or compost-amended Ferric Luvisol soils; thus, possible ways of controlling nitrate leaching must be considered.
2. Neem seeds are recommended as a nitrification inhibitor in the soil. However, inhibition with this treatment only lasted 40 days after amendment; due to this, the application of neem seed may be recommended for use in short-duration crops, such as some cereals, vegetables, and legumes.
3. The application of sawdust biochar resulted in nitrification inhibition throughout the entire incubation period and, thus, may be recommended for use in long-duration crops, such as trees.
4. Replication of these studies is recommended under field conditions.
Data availability statement
The raw data supporting the conclusions of this article will be made available by the authors, without undue reservation.
Author contributions
HA, IL, and EN: Conception and design of study. HA and IL: Carrying out experiments and data collection. HA, IL, EN, TA, and SA-B: Analysis and/or interpretation of data. HA and IL: Drafting the manuscript. HA, IL, PB, and WA: Revising the manuscript critically for important intellectual content. HA, IL, PB, and WA: Approval of the version of the manuscript to be published.
Funding
This work was fully funded by USAID (Capacity Building of the Feed the Future Project) as part of a PhD scholarship.
Acknowledgments
The authors are grateful for the financial support provided by the U.S. Agency for International Development (USAID)-funded Feed the Future Project. The authors express their appreciation for the administrative support offered by the Department of Soil Science, College of Basic and Applied Sciences, University of Ghana, Legon, and the CSIR-Savanna Agricultural Research Institute. Finally, we wish to express our profound gratitude to the reviewers whose contributions have led to a major improvement in this manuscript.
Conflict of interest
The authors declare that the research was conducted in the absence of any commercial or financial relationships that could be construed as a potential conflict of interest.
Publisher’s note
All claims expressed in this article are solely those of the authors and do not necessarily represent those of their affiliated organizations, or those of the publisher, the editors and the reviewers. Any product that may be evaluated in this article, or claim that may be made by its manufacturer, is not guaranteed or endorsed by the publisher.
Supplementary Material
The Supplementary Material for this article can be found online at: https://www.frontiersin.org/articles/10.3389/fsoil.2022.1023743/full#supplementary-material
References
1. Lal R. Soil carbon sequestration impacts on global climate change and food security. Science (2004) 304:1623–7. doi: 10.1126/science.1097396
2. Sanchez PA, Jama BA. Integrated plant nutrient management in Sub-Saharan Africa: From concept to practice. Vanlauwe B, Diels J, Sanginga N, Merckx R, editors. Wallingford, UK: CABI (2002) p. 23–45.
3. FAO. Plant nutrition for food security. a guide for integrated nutrient management. In: FAO fertilizer and plant nutrition bulletin 16. Rome: FAO (2006).
4. Adjognon SG, Liverpool-Tasie LS, Reardon TA. Agricultural input credit in Sub-Saharan Africa: Telling myth from facts. Food Policy (2017) 67:93–105. doi: 10.1016/j.foodpol.2016.09.014
5. Peyvast G, Ramezani Kharazi P, Tahernia S, Nosratierad Z, Olfati JA. Municipal solid waste compost increased yield and decreased nitrate amount of broccoli (Brassica oleracea var. italica). J Appl Horticult (2008) 10(2):129–32. doi: 10.37855/jah.2008.v10i02.27
6. Brady NC, Weil RR. The nature and properties of soils. 14th Edition. Upper Saddle River, New Jersey: Pearson Education, Inc. (2008).
7. D’Haene K, Salomez J, De Neve S, De Waele J, Hofman G. Environmental performance of nitrogen fertilizer limits imposed by the EU nitrates directive. Agricult Ecosyst Environ (2014) 192:67–79. doi: 10.1016/j.agee.2014.03.049
8. Nelson DW, Huber D. Nitrification inhibitors for corn production. In: National corn handbook 55. file agronomy 2-2. Ames: Iowa State University. (2001).
9. Kihara J, Bolo P, Kinyua M, Nyawira SS, Sommer R. Soil health and ecosystem services: Lessons from sub-Sahara Africa (SSA). Geoderma (2020) 370:114342. doi: 10.1016/j.geoderma.2020.114342
10. Atakora W, Kwakye P, Weymann D, Brüggemann N. Stimulus of nitrogen fertilizers and soil characteristics on maize yield and nitrous oxide emission from ferric luvisol in the Guinea savanna agro-ecological zone of Ghana. Sci African (2019) 6:3–4. doi: 10.1016/j.sciaf.2019.e00141
11. Amlinger F, Gotz B, Dreher P, Geszti J, Weissteiner C. Nitrogen in biowaste and yard waste compost: dynamics of mobilization and availability - a review. Eur Soil Biol (2003) 39:107–16. doi: 10.1016/S1164-5563(03)00026-8
12. Arauzo M, Valladolid M. Drainage and n-leaching in alluvial soils under agricultural land uses: Implications for the implementation of the EU nitrates directive. Agric Ecosyst Environ (2013) 179:94–107. doi: 10.1016/j.agee.2013.07.013
13. Chaves B, Opoku A, De Neve S, Boeckx P, Van Cleemput O, Hofman G. Influence of DCD and DMPP on soil n dynamics after incorporation of vegetable crop residues. Biol Fertil Soils (2006) 43:62–8. doi: 10.1007/s00374-005-0061-6
14. Zerulla W, Erhardt K, Pasda G, Dressel J, Barth T, von Locquenghien KH, et al. DMPP (3,4-dimethylpyrazole phosphate): A new nitrification inhibitor for agriculture and horticulture. Biol Fertil Soil (2001) 34:79–84. doi: 10.1007/s003740100380
15. Rembold H, Annadurai RS. Azadirachtin inhibits proliferation of sf 9 cells in monolayer culture. Z Naturforsch C J Biosci (1993) 48(5-6):495–9. doi: 10.1515/znc-1993-5-615
16. Altayb HN, Yassin NF, Hosawi S, Imran K. In-vitro and in-silico antibacterial activity of Azadirachta indica (Neem), methanolic extract, and identification of beta.d-mannofuranoside as a promising antibacterial agent. BMC Plant Biol (2022) 22:262. doi: 10.1186/s12870-022-03650-5
17. Kebede T, Gadisa E, Tufa A. Antimicrobial activities evaluation and phytochemical screening of some selected medicinal plants: A possible alternative in the treatment of multidrug-resistant microbes. PloS One (2021) 16(3):e0249253. doi: 10.1371/journal.pone.0249253
18. Kiran U, Patra DD. Medicinal and aromatic plant materials as nitrification inhibitors for augmenting yield and nitrogen uptake of Japanese mint (Mentha arvensis l. var. piperascens). Biores Technol (2003) 86:267–76. doi: 10.1016/S0960-8524(02)00143-8
19. Tyagi SK, Bhagwat VR, Nimesh A, Khatkar AB. Improving nutrient efficiency of soil using urea coats by mustard byproducts in wheat. Journal of Pharmacognosy and Phytochemistry (2019) 8(5):1148–50.
20. Majumdar D. Suppression of nitrification and N2O emission by karanjin - a nitrification inhibitor prepared from karanja (Pongamia glabra vent.). Chemosphere (2002) 47:845–50. doi: 10.1016/S0045-6535(01)00287-9
21. Meena BP, Biswas AK, Shirale AO, Ramesh K, Jha P, Lakaria BL, et al. Ecofriendly neem coated urea ecofriendly neem coated urea: A boon for farmers. Indian Farming (2017) 67(06):11–4.
22. Clough TJ, Condron LM. Biochar and the nitrogen cycle. J Environ Qual (2010) 39:1218–23. doi: 10.2134/jeq2010.0204
23. Pan Y, Yang M, Dong D, Wei-Xiang W. Effects of biochar on soil nitrogen cycle and related mechanisms: A review. J Appl Ecol (2013) 24(9):2666–73.
24. Ippolito JA, Ducey TF, Cantrell KB, Novak JM, Lentz RD. Designer, acidic biochar influences calcareous soil characteristics. Chemosphere (2016) 142:184–91. doi: 10.1016/j.chemosphere.2015.05.092
25. Borchard N, Wolf A, Laabs V, Aeckersberg R, Scherer HW, Moeller A, et al. Physical activation of biochar and its meaning for soil fertility and nutrient leaching – a greenhouse experiment. Soil Use Manage (2012) 28:177–84. doi: 10.1111/j.1475-2743.2012.00407.x
26. Quilliam RS, Marsden K, Gertler G, Rousk J, DeLuca TH, Jones DL. Nutrient dynamics, microbial growth and weed emergence in biochar amended soil are influenced by time since application and reapplication rate. Agric Ecosyst Environ (2012) 158:192–9. doi: 10.1016/j.agee.2012.06.011
27. Morales MM, Comerford N, Guerrini IA, Falcão NPS, Reeves JB. Sorption and desorption of phosphate on biochar and biochar-soil mixtures. Soil Use Manage (2013) 29:306–14. doi: 10.1111/sum.12047
28. Yao Y, Gao B, Zhang M, Inyang M, Zimmerman AR. Effect of biochar amendment on sorption and leaching of nitrate, ammonium, and phosphate in a sandy soil. Chemosphere (2012) 89:1467–71. doi: 10.1016/j.chemosphere.2012.06.002
29. Spokas KA, Novak JM, Venterea RT. Biochar’s role as an alternative n-fertilizer: Ammonia capture. Plant Soil (2012) 350:35–42. doi: 10.1007/s11104-011-0930-8
30. Zhang Y, Li Z, Zhang L. Adsorption characters of ammonium-nitrogen in aqueous solution by modified corn cob biochars. CIESC J (2014) 65(3):960–6. doi: 10.3969/j.issn.0438-1157.2014.03.028
31. Adu SV, Asiamah RD. Soils of the lawra-wa region, upper West region, Ghana. Kwadaso, Kumasi: Soil Research Institute, CSIR (2003).
32. Iddrisu WA, Nokoe KS, Luguterah A, Antwi EO. Generalized additive mixed modelling of river discharge in the black Volta river. Open J Stat (2017) 7:621–32. doi: 10.4236/ojs.2017.74043
33. Crawford DM, Chalk PM. Mineralization and immobilization of soil and fertilizer nitrogen with nitrification inhibitors and solvents. Soil Biol Biochem (1992) 24(6):559–68. doi: 10.1016/0038-0717(92)90081-8
34. Sarawaneeyaruk S, Krajangsang S, Pringsulaka O. The effects of neem extract and azadirachtin on soil microorganisms. J Soil Sci Plant Nutr (2015) 15(4):1071–83. doi: 10.4067/S0718-95162015005000075
35. Fox RL, Kamprath EJ. Phosphate sorption isotherms for evaluating the phosphate requirements of soils. Soils Sci Am Proc (1970) 34:902–6. doi: 10.2136/sssaj1970.03615995003400060025x
36. Yang X, Vancov T, Peñuelas J, Sardans J, Singla A, Alrefaei A, et al. Optimal biochar application rates for mitigating global warming and increasing rice yield in a subtropical paddy field. Exp Agric (2021) 57(5-6):283–99. doi: 10.1017/S0014479721000259
37. Garten CT Jr, Wullschleger SD. Soil carbon dynamics beneath switchgrass as indicated by stable isotope analysis. J Environ Qual (2000) 29:645–53. doi: 10.2134/jeq2000.00472425002900020036x
38. Nketia KA, Adjadeh TT, Adiku SGK. Evaluation of suitability of some soils in the forest-savanna transition and the Guinea savanna zones of Ghana for maize production. West Afr J Appl Ecol (2018) 26(1):61+.
39. Chèneby D, Nicolardot B, Godden B, Penninckx M. Mineralization of composted 15N–labelled farmyard manure during soil incubations, Biol.Agric. Horticulture (1994) 10:255–64. doi: 10.1080/01448765.1994.9754677
40. He ZL, Alva AK, Yan P, Li YC, Calvert DV, Stoffella PJ, et al. Nitrogen mineralization and transformation from composts and biosolids during field incubation in a sandy soil. Soil Sci (2000) 165(2):161–9. doi: 10.1097/00010694-200002000-00007
41. Mordue AJ, Nisbet AJ. Azadirachtin from the neem tree azadirachta indica: Its action against the insects. anais de sociedade entomológica do brasil. Anais Da Sociedade Entomol Do Bras (2000) 29(4):615–32. doi: 10.1590/S0301-80592000000400001
42. Joseph PA, Prasad R. The effect of dicyandiamide and neem cake on the nitrification of urea-derived ammonium under field conditions. Biol Fertil Soils (1993) 15(2):149–52. doi: 10.1007/BF00336434
43. Muni R, Patra DD, Subramanyam K, Singh DV. Nitrification inhibitory properties in mentha-spent and pyrethrum flowers. J Indian Soc Soil Sci (1993) 41:176–7. doi: 10.1016/B978-0-12-394275-3.00001-8
44. Amlinger F, Gotz B, Dreher P, Geszti J, Weissteiner C. Nitrogen in biowaste and yard waste compost: dynamics ofmobilisation and availability - a review. Eur Soil Biol (2003) 39:107–16. doi: 10.1016/S1164-5563(03)00026-8
45. Johnson S, Morgan ED. Supercritical fluid extraction of oil and triterpenoids from neem seeds. Phytochem Anal (1997) 8:228–32. doi: 10.1002/(SICI)1099-1565(199709/10)8:5<228:AID-PCA367>3.0.CO;2-C
46. Xu R, Qafoku NP, Van Ranst E, Li J, Jiang J. Chapter one - adsorption properties of subtropical and tropical variable charge soils: Implications from climate change and biochar amendment. In: Sparks DL, editor. Advances in agronomy. Amsterdam-Holland: Academic Press (2016) 135:1–58.
47. Yoo G, Kim H, Chen J, Kim Y. Effects of biochar addition on nitrogen leaching and soil structure following fertilizer application to rice paddy soil. Soil Sci Soc Am J (2014) 78:852–60. doi: 10.2136/sssaj2013.05.0160
48. Evans JR. Nitrogen and photosynthesis in the flag leaf of wheat (Triticum aestivum l.). Plant Physiol (1983) 72:297–302. doi: 10.1104/pp.72.2.297
49. Bindraban PS. Impact of canopy nitrogen profile in wheat on growth. Field Crops Res (1999) 63:63–77. doi: 10.1016/S0378-4290(99)00030-1
50. Harper JE, Bennett JM, Sinclair TR, Paulsen GM. Nitrogen metabolism. In: Boote KJ, et al, editors. Physiology and determination of crop yield, vol. 11. Madison, Wisconsin-USA: American Society of Agronomy (1994). p. 285–302.
Keywords: leaching, nitrification, azadirachtin, inhibition, biochar, neem, nitrate, amendment
Citation: Abeka H, Lawson IYD, Nartey E, Adjadeh T, Asuming-Brempong S, Bindraban P and Atakora WK (2022) Effectiveness of neem materials and biochar as nitrification inhibitors in reducing nitrate leaching in a compost-amended Ferric Luvisol. Front. Soil Sci. 2:1023743. doi: 10.3389/fsoil.2022.1023743
Received: 20 August 2022; Accepted: 03 October 2022;
Published: 02 November 2022.
Edited by:
Sunita K. Meena, Dr. Rajendra Prasad Central Agricultural University, IndiaReviewed by:
Carlos Garcia, Center for Edaphology and Applied Biology of Segura (CSIC), SpainMuhammad Shaaban, Bahauddin Zakariya University, Pakistan
H. P. Parewa, Agriculture University, India
Copyright © 2022 Abeka, Lawson, Nartey, Adjadeh, Asuming-Brempong, Bindraban and Atakora. This is an open-access article distributed under the terms of the Creative Commons Attribution License (CC BY). The use, distribution or reproduction in other forums is permitted, provided the original author(s) and the copyright owner(s) are credited and that the original publication in this journal is cited, in accordance with accepted academic practice. No use, distribution or reproduction is permitted which does not comply with these terms.
*Correspondence: Hammond Abeka, oyewu.abeka07@gmail.com
†These authors share first authorship