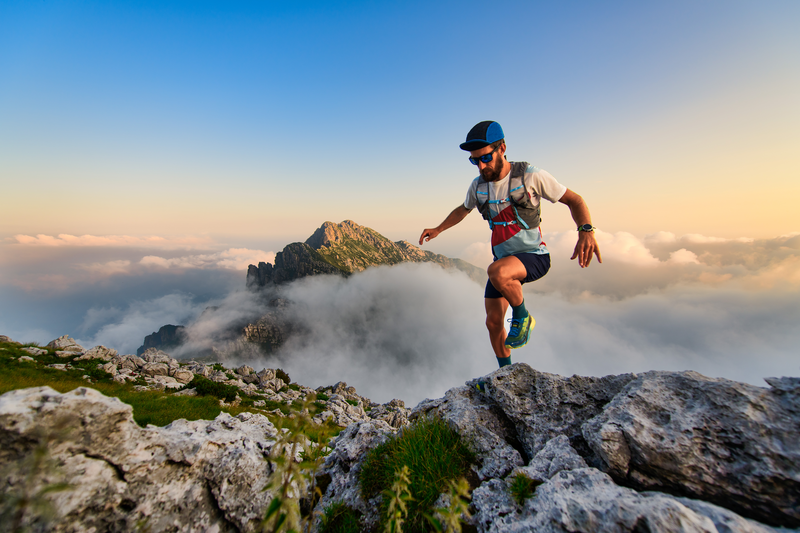
95% of researchers rate our articles as excellent or good
Learn more about the work of our research integrity team to safeguard the quality of each article we publish.
Find out more
ORIGINAL RESEARCH article
Front. Soil Sci. , 06 January 2022
Sec. Plant-Soil Interactions
Volume 1 - 2021 | https://doi.org/10.3389/fsoil.2021.709507
This article is part of the Research Topic Sustainable Phosphorus Use in Agriculture View all 6 articles
Development of local P fertilizers using low-grade phosphate rock (PR) is expected to overcome the low-stagnated crop yield in Sub-Saharan Africa. Calcination and partial acidulation methods have been proposed to increase the phosphate (P) solubility of PRs. However, the effects of fertilization with calcinated PR (CPR) and partially acidulated PR (PAPR) on sorghum [Sorghum bicolor (L.)] and cowpea [Vigna unguiculata (L.) Walp.] cultivation are poorly understood. Therefore, we conducted a 2-year field experiment in Burkina Faso to identify the differences in sorghum and cowpea responses to CPR and PAPR application. The following eight treatments were applied with six replicates using a complete randomized block design: control without P fertilization, two types of CP (CPs), triple superphosphate (TSP) as a positive control for CPs, three types of PAPR with different degrees of acidulation (PAPRs), and single superphosphate (SSP) as a positive control for PAPRs. SSP mostly comprised of water-soluble P fraction (WP), TSP and PAPRs of WP and alkaline ammonium citrate-soluble P fraction (SP), and CPRs of SP and 2% citric acid-soluble P fraction (CP). Their solubility was in the order WP > SP > CP. The fertilization effects were evaluated by P use efficiency (PUE). In 2019, the biomass and P uptake of sorghum was decreased by the low available soil water at the early growth stage. On the contrary, cowpea survived the low available soil water because of its shorter growing period compared to sorghum. P fertilization significantly increased the grain yields. However, the effect size differed according to the crop and fertilizer types. The SP, along with WP, significantly contributed to the PUE and grain yield of sorghum, whereas only WP contributed to the PUE of cowpea. Therefore, CPs, mainly consisting of SP and CP, had a disadvantage compared to TSP, especially for cowpea. We thus concluded that PAPRs are effective for sorghum and would be effective for cowpea when the acidulation level is sufficiently high. We also conclude that the long growing period of sorghum is favorable for absorbing slow-release P, but is unfavorable for the variable rainfall often observed in this region.
Sub-Saharan Africa (SSA) has the highest percentage of food insecurity globally (1). In rural households, most food is produced and consumed locally (2); thus, household agricultural productivity is critical for improving food security (3). According to previous studies, crop production in SSA is restricted by low water availability, low soil fertility, and low fertilizer input (4, 5). Agricultural production in SSA is mainly conducted under rain-fed conditions and is particularly vulnerable to rainfall variability (6). Furthermore, SSA has a wide distribution of soils with low phosphorus (P) content, mainly due to the high degree of weathering, and these are recognized as one of the significant constraints for rice production (7). Although P fertilizer application is the most promising approach to overcome this problem (8), the total fertilizer application rate [sum of nitrogen (N), P, and potassium (K)] in SSA is only 8 kg ha−1 which is far below the global average of 93 kg ha−1 mainly due to the high costs incurred from long-distance transportation (9).
A vast amount of geological phosphate rock (PR) deposits has been found throughout SSA (10). According to the Burkina Faso Government, there are 100 million metric tons of PR in the Kodjari region of Burkina Faso (11), which could serve as an alternative source of P fertilizer (12). However, most of these have not been utilized because of their low solubility and reactivity (13). Methods for increasing the solubility of PR by partial acidulation and calcination have been reported previously (14–17). The International Fertilizer Development Center has promoted partial acidulation with sulfuric acid. Acidulation of PR produces the phosphate component monocalcium phosphate, small amounts of dicalcium phosphate, and residual apatite depending on the degree of acidulation (16). However, the viscosity and acidity of the product increase with increasing amounts of sulfuric acid because of the unbound sulfuric acid (14, 15). Therefore, to secure adequate solubility and usability, it is necessary to optimize the amount of sulfuric acid added.
Akiyama et al. (14) developed a calcination procedure for PR at a temperature of 900°C, using alkaline additives to convert fluoride apatite to α-tricalcium phosphate and Rhenania phosphate. Nakamura et al. (17) modified this method for the low-grade PR from Kodjari region and achieved high solubility in 2% citric acid.
The Sudan Savanna (annual rainfall, 600–900 mm) in West Africa is a climatic transition zone between the Sahel (annual rainfall, 200–600 mm) and the Guinea Savanna (annual rainfall, 900–1200 mm). Owing to its semi-arid conditions, sorghum [Sorghum bicolor (L.)] and cowpea [Vigna unguiculata (L.) Walp.] are widely cultivated in the Sudan Savanna. Sorghum is an important food source for human consumption (13). Sorghum is known as drought, heat, and waterlogging tolerant crop, making it the second most grown cereal crop in SSA (18). However, water stress at different growth stages is also reported to affect grain yield and yield components (19–21). Cowpea is a legume crop of vital importance to the livelihood of millions of people in West and Central Africa, providing a nutritious grain and an inexpensive protein source (22). However, its average yield is substantially below the biological potential (23).
Previous studies have shown a positive effect of partial acidulated PR (PAPR) application on upland crops (24, 25). Moreover, Nakamura et al. (17) showed good performance of calcinated PR (CPR) on rice and maize production in a pot experiment. Furthermore, Iwasaki et al. (26) and Fukuda et al. (27) reported that CPR showed comparable performance with imported P fertilizers. However, the fertilization effect of CPR on upland crops under rainfed conditions has never been studied. The P applied to soil undergoes a series of physicochemical (dissolution-precipitation and desorption-adsorption) transformations (28, 29). Thus, the P solubility of local P fertilizer could be a critical controlling factor of the P fertilization effect. However, the fertilization effects of PAPR and CPR with different P solubility on sorghum and cowpea cultivation under rainfed conditions remain poorly understood, especially in the Sudan Savanna.
Therefore, this study aimed to identify differences in the responses of sorghum and cowpea to P fertilization and soil moisture conditions under rainfed conditions with the hypothesis that the P fertilization effect of local P fertilizers differs according to crop type and climatic conditions. We selected the Central Plateau of Burkina Faso as the study site as it is a major sorghum and cowpea producing area in the Sudan Savanna. Suppose PAPRs and CPRs improve upland crops yield in fields with low productivity, they could facilitate the efficient use of local P resources and the addressing of frequent food shortages in the Sudan Savanna.
A field experiment was conducted at the Saria station of the Institute of Environment and Agricultural Research (Institut de l'Environnement et de Recherches Agricoles: INERA) located at the Central Plateau region of Burkina Faso. The study area is characterized by a long dry season from November to April and a short rainy season from May to October. The mean annual precipitation is 800 mm yr−1, and the mean annual temperature is 28°C, as recorded at INERA Saria station. Meteorological data were recorded at 10-min intervals using an automatic weather station with temperature and relative humidity sensor (HygroVUE™5, Campbell Scientific) and a rain gauge (TE525MM-L, Campbell Scientific).
According to the EU (30) and Ikazaki et al. (31), Ferric Lixisols (Lxfr), Petric Plinthosols (PTpt), and Pisoplinthic Petric Plinthosols (PTpt.px) are dominantly distributed in relation to the local topography. In this study, a field of approximately 1.2 ha (80 m × 150 m) on LXfr was selected. The morphological, physical, and chemical properties of the soil were previously reported by Ikazaki et al. (31) (open access, see profile 1). Soil samples were collected at randomly 106 selected points to evaluate the initial soil fertility and spatial variation in soil physico-chemical properties. The total P content was 319.8 ± 42.8 [mean ± sd (mg P kg−1)], and the available P contents measured using the Bray-1 and Bray-2 methods was 8.4 ± 3.3 and 9.7 ± 6.5, respectively (Table 1).
Two types of local P fertilizer were produced by calcination and partial acidulation methods using PR from the Kodjari deposit (12°1′ N; 1°55′ E) in Burkina Faso (BPR). BPR contained 113 g P kg−1, and its 2% citric acid-solubility was 31.1% of the total P. The calcination procedure described by Nakamura et al. (17) was employed to prepare calcinated PR (CPs). Fine powdered BPR was mixed well with the respective additives as follows: with K2CO3 to produce CPk (17) and with K2CO3 + CaCO3 to produce CPkca (Nakamura et al., unpublished data). The components were mixed with distilled water, pressed to form pellets, and then calcinated at 900°C for 10 min using a muffle furnace (FP32; Yamato Scientific Co., Ltd., Japan).
To prepare partially acidulated PR (PAPR), we followed the acidulation procedure described by Frederick and Roth (15). The addition rate of sulfuric acid was determined based on the mineral composition of BPR. To produce 100% acidulated phosphate rock (PAPR100), 326.8 mL of H2SO4 was added to 1 kg of BPR. We also prepared 50% and 75% acidulated phosphate rock (PAPR50 and PAPR75). The chemical properties of CPs, PAPRs, TSP, and SSP were analyzed as described by the Food and Agricultural Materials Inspection Center (32). Water-solubility, alkaline ammonium citrate-solubility, and 2% citric acid-solubility were measured. The water-soluble P fraction (WP: equal to water solubility), alkaline ammonium citrate-soluble P fraction (SP: alkaline ammonium citrate-solubility with water solubility subtracted), 2% citric acid-soluble P fraction (CP: 2% citric acid-solubility with alkaline ammonium citrate-solubility subtracted), and residual P fraction (RP: the P fraction not soluble in 2% citric acid) were calculated. Solubility and availability for plants are generally in the order WP > SP > CP > RP.
In 2017, sorghum (Sorghum bicolor (L.) Moench, var. Kapelga) was cultivated without fertilization to reduce soil heterogeneity and to obtain a uniform cropping history. In 2018, 48 plots were established both for sorghum and cowpea, with a plot size of 6.0 m × 4.0 m for sorghum and 4.0 m × 4.0 m for cowpea. According to the results of soil analysis (Table 1), areas with high spatial variability in soil chemical properties were excluded when preparing the 96 above-mentioned plots.
The following eight treatments were assigned to the plots with six replicates using a complete randomized block design. (1) control without P fertilization (CT), (2) CPk, 3) CPkca, (4) TSP as a positive control for CPs, (5) PAPR50, (6) PAPR75, (7) PAPR100, (8) SSP as a positive control for PAPRs. According to the blanket recommendation in Burkina Faso (IRAT 1978), the application rate of N, P, and K was set at 37 kg N ha−1, 23 Kg P2O5 ha−1, and 14 kg K2O ha−1 for sorghum, and 14 kg N ha−1, 23 kg P2O5 ha−1, and 14 kg K2O ha−1 for cowpea. N and K were applied as urea and potassium chloride. Planting density was set at 3.1 hills m−2 (80 cm by 40 cm) following the recommendations. We employed the improved varieties developed by INERA: Kapelga for Sorghum and Nafi for cowpea. The land was prepared by ox plowing for about 2 weeks before sowing. After harrowing using a hoe, earth mounds of 10 cm height were established around the plot to prevent water and nutrient contamination from other plots.
Sorghum was planted manually on July 7 in 2018, and on June 29 in 2019. If there were empty pockets in the plot, replanting was conducted as required. After germination, plants were thinned to three plants per hill. One-third of N and the complete amount of P and K were applied at 2 weeks after sowing (WAS). Another one-third of N was applied at 4 WAS and 6 WAS, respectively. Sorghum was harvested on November 4 in 2018, and on November 7 in 2019. Cowpea was planted on July 14 in 2018 and on July 16 in 2019. After germination, plants were thinned to two plants per hill. Insecticide was applied 2–3 times as needed. The harvest date was October 4 in 2018 and October 5 in 2019.
Soil volumetric water content (VWC) was continuously measured every 10 min using time domain reflectometry (TDR) probes (CS616; Campbell Scientific, Logan, UT, USA) installed at 0–10 and 10–25 cm. Soil temperature at the same depth was also recorded using thermistor probes (107; Campbell Scientific, Logan, UT, USA) to correct the temperature dependence of the TDR probe. The estimated VWC was calibrated according to the manufacturer's instructions (33). Undisturbed soil cores were collected every 2 weeks from each layer and subjected to oven-drying at 105°C to determine the gravimetric VWC.
All sorghum plants (39 hills plot−1) except those on the border were harvested and subjected to the yield and yield components survey. Panicles were harvested and then divided into rachises and grains. The air-dried weights of stovers, rachises, and grains were measured. The number of stems (m−2), number of panicles (stem−1), ripening rate, number of grains (good panicle−1), and 1000-grain weight (g) were measured as yield components.
All cowpea plants (24 hills plot−1) except those on the border were harvested and subjected to the yield and yield components survey. Pods were threshed and divide into cobs and grains. The air-dried weights of shoots, pods, and grains were measured. The number of stems (m−2), number of grains (pod−1), and 100-grain weight (g) were measured as yield components. For both sorghum and cowpea, 100–200 g of air-dried stovers and shoots from every plot were used to determine the oven-dried weight. The grain moisture content was measured using a portable grain moisture tester (MT-16, Agratronix). The harvest index was determined as a portion of the grain mass in the total above-ground biomass (hereafter biomass indicates the sum of stover, rachis, and grain for sorghum and that of the shoot, cob, and grain for cowpea). Grain yield was represented as Mg ha−1 at a 12% seed moisture content.
The P concentration of sorghum and cowpea plant parts was determined using a Handheld X-Ray Fluorescence Analyzer [XRF (VANTA, OLYMPUS Scientific Solutions Americas Corp., MA, USA)] in 2018 and by a dry-combustion method in 2019. First, plant samples were oven-dried at 70°C and finely ground. In 2018, the P concentration was measured using XRF according to the manufacturer's instruction (34). In 2019, the samples were dry-ashed using a muffle furnace at 550°C for 2 h and then dissolved in 0.5 M HCl. The concentration of P in the extract was determined using inductively coupled plasma atomic emission spectrophotometry (ICPE-9000, Shimadzu, Japan). The values determined in 2018 and 2019 were corrected using the equations obtained from the relationships with values calculated using the wet-digestion method to fill the discrepancies between the two methods used as follows:
where Pcorrected is the P concentration after the correction (mg P kg−1), PXRF and Pdry−combustion are the P concentrations measured by the XRF and dry-combustion method, respectively.
The available soil water (mm) in each soil layer was calculated according to Iseki et al. (35):
where VWCt is VWC (%) at time t and VWCthreshold is VWC (%) at pF 3.0 for each soil layer. The threshold of soil water potential below which plant growth starts to be suppressed was set as pF 3.0. Then, the available soil water at 0–25 cm was calculated. The growing period was divided into four stages for sorghum and into three stages for cowpea. For sorghum, we used the early growth stage until the growing point differentiation (0–5 WAS, SW1), middle growth stage until the flowering time (6–10 WAS, SW2), late growth stage until physiological maturity (10–15 WAS, SW), and the remain stage until harvest (~16 WAS, SW4). For cowpea, we used the early growth stage until flowering (0–4 WAS, SW1), middle growth stage until maximum vegetation (5–8 WAS, SW2), and late growth stage until harvest (~9 WAS, SW3).
The plant P uptake (kg P ha−1) was calculated by multiplying the above-ground biomass and P concentration. The P transfer efficiency (PTE in %) was calculated as a ratio of grain P to total P (sum of stover, rachis, and grain for sorghum, and that of the shoot, cob, and grain for cowpea).
The fertilization effects were evaluated by the increase in grain yield and P use efficiency (PUE in %). PUE was calculated as described by Gitari et al. (36):
where TUPTreatments is the total P uptake in the P fertilization treatments (kg P ha−1) and TUPCT is the total P uptake in CT treatment (kg P ha−1).
Statistical analysis and figure drawing were performed using R version 4.0.0 (37). The difference in the mean available soil water between the 2 years was analyzed using an unpaired t-test in each period. Pearson's correlation coefficient was employed to show the correlation of the yield components with the grain yield. The effects of year and treatment on the biomass, harvest index, grain yield, total P uptake, PTE, and grain P were analyzed by two-way analysis of variance (ANOVA). The effects of year, crop, and treatment on PUE were analyzed by three-way ANOVA. Differences in PUE were evaluated by comparison with the positive controls (TSP for CPs, and SSP for PAPRs). Multiple comparisons using Shaffer's Modified Sequentially Rejective Bonferroni Procedure (38) were conducted if a significant difference was detected. The effect size of the sources was evaluated using the eta squared (η2). High η2 suggests a high contribution to the total variance. The effects of P fractions (WP, SP, CP, and RP) on the PUE and grain yield were analyzed using stepwise multiple regression analysis. Standardized partial regression coefficients were estimated. Selection of explanatory variables was based on Akaike's information criterion (AIC) (39). In this analysis, the 2-year averages of PUE and grain yields in each treatment were used.
Partial acidulation and calcination methods resulted in increased solubility compared with that of raw BPR (Table 2). The RP fraction decreased from 68.9% in BPR to 25.8% (CPk), 3.8% (CPkca), 21.4% (PAPR50), 21.1% (PAPR75), and 18.0% (PAPR100). PAPR mainly consisted of WP and SP, whereas CPR contained almost equal amounts of SP and CP. Among the CPs, CPkca contained higher total P and lower RP compared to CPk. A higher degree of acidulation increased the water-, alkaline ammonium citrate-, and 2% citric acid-solubility. The pH (H2O) values at a solid: liquid extraction ratio of 1:10 for BPR, TSP, SSP, CPs, and PAPRs were 7.4, 3.0, 3.0, 12.3, and 3.4–2.7, respectively.
Time-course changes in solar radiation and available soil water (mm) at 0–25 cm depth are shown in Figure 1 (raw data of precipitation and VWC are provided in Supplementary Figure S1). The solar radiation in 2019 decreased to 400 (W m−1) when compared to that in 2018, which was a 2-fold decrease. For sorghum, the mean available soil water during SW1 and SW4 was significantly different between the 2 years. Notably, the mean available soil water was particularly low in the first 2 weeks of 2019 (ranging from 0 to 4.2 mm). On the contrary, for cowpea, no significant difference was observed in the mean available soil water between 2 years.
Figure 1. Solar radiation (upper panel) and available soil water at 0–25 cm depth (lower panel) during the growing season The cropping season was divided depending on the plant growth stage. Sorghum: SW1, early growth stage until the growing point differentiation (0–5 WAS); SW2, middle growth stage until the flowering time (6–10 WAS); and SW3, late growth stage until physiological maturity (10–15 WAS); SW4, until the harvest (16~ WAS). Cowpea: SW1, early growth stage until the flowering time (0–4 WAS); SW2, middle growth stage until maximum vegetation (5–8 WAS); and SW3, late growth stage until harvest (9~ WAS). The difference between the 2 years was analyzed in each stage. DAS, days after sowing; **, p < 0.01; ns, not significant.
Pearson's correlation coefficients of yield components to grain yield are shown in Table 3. For sorghum, the number of panicles, number of grains per panicle, and 1000-grain weight were positively correlated with grain yield in 2018, whereas only the ripening rate showed a significant correlation with grain yield in 2019. For cowpea, the number of pods positively correlated with the grain yield in both years, and a significant contribution of the 100-grain weight was observed only in 2019.
The results of two-way ANOVA are summarized in Table 4. For sorghum, the year effect was significant for all properties with high values of η2. The treatment effect showed an increasing trend in grain yield, total P uptake, and grain P uptake (p < 0.1), but this was not significant. For cowpea, the year effect was significant in all properties except for biomass. The η2 values were generally lower than those for sorghum except for the harvest index. In contrast with sorghum, the treatment effect was significant for all properties of cowpea, except for PTE with high values of η2, suggesting that the effect of P fertilization was more obvious for cowpea than for sorghum. Significant interactions between the year and treatment were observed in biomass, harvest index, and total P uptake for cowpea.
Table 4. Effects of the annual difference (year) and treatments on biomass, harvest index, grain yield, total P uptake, PTE, and grain P uptake.
Annual differences in parameters concerning the yield and P uptake are shown in Figure 2. The grain yield of sorghum was 1.47 ± 0.24 [mean ± sd (Mg ha−1)] in 2018 and 0.34 ± 0.11 in 2019; the other components were similarly lower in 2019 than in 2018 (p < 0.001). In contrast, the grain yield of cowpea was 0.99 ± 0.14 in 2018 and 0.60 ± 0.09 in 2019, and the other components except for biomass were similarly lower in 2019 than in 2018 (p < 0.05). The annual difference was more prominent in PTE (p < 0.001) than in the total P uptake (p < 0.05) for cowpea.
Figure 2. Annual differences in biomass, harvest index, grain yield, total phosphorus (P) uptake, PTE, and grain P uptake. Lowercase letters indicate a significant difference between the treatments. PTE, P transfer efficiency; *, p < 0.05; ***, p < 0.001; ns, not significant.
The increased grain yield of sorghum and cowpea was positively correlated with the PUE (0.43 < R2 < 0.97) (Figure 3). The result of three-way ANOVA for PUE are summarized in Table 5. The effects of plants and treatments were significant with significant interactions between the year and plant, and the plant and treatment. The highest η2 value was observed in the treatment effect. According to the three-way ANOVA, Figures 4A,B were prepared to compare the PUE between two crops and between 2 years, respectively. Figure 4C was also prepared to evaluate the effect of P treatment on the PUE. Although there was no significant difference in the PUE between the plants in 2018, the PUE for cowpea was significantly higher than that for sorghum in 2019 (Figure 4A). The PUE for sorghum was significantly higher in 2018 than in 2019 (Figure 4B). In contrast, there was no significant difference in the PUE for cowpea between 2 years (Figure 4B). The PUE for sorghum was significantly different among the CPs but not among the PAPRs (Figure 4C). However, the PUE for cowpea differed significantly among the treatments for both CPs and PAPRs (Figure 4C). The PUE in CPs was significantly smaller than that in TSP for both sorghum and cowpea. PAPR50 and 75 had a significantly smaller PUE than SSP, whereas PAPR100 showed a comparable performance with SSP for cowpea (Figure 4C).
Figure 3. Relationship between phosphorus (P) use efficiency (PUE) and grain yield. Error bar indicates standard deviation (n = 6). CPk, phosphate rock calcinated with potassium carbonate (K2CO3); CPkca, phosphate rock calcinated with potassium carbonate (K2CO3) and calcium carbonate (CaCO3); TSP, triple superphosphate; SSP, single superphosphate. PAPR50, 75, and 100, partially acidulated phosphate rock with different degrees of acidulation. The degree of acidulation increases with an increase in the number.
Figure 4. Effects of fertilizer types on phosphorus use efficiency (PUE). Comparison between the year (A), crop type (B), and fertilizer type (C). Lowercase letters indicate a significant difference between the treatments. ns, not significant; *, p < 0.05; **, p < 0.01; ***, p < 0.001. CPs, calcinated phosphate rocks; PAPRs, partial acidulated phosphate rocks; CPk, phosphate rock calcinated with potassium carbonate (K2CO3); CPkca, phosphate rock calcinated with potassium carbonate (K2CO3) and calcium carbonate (CaCO3); TSP, triple superphosphate; SSP, single superphosphate. PAPR50, 75, and 100, partially acidulated phosphate rock with different degrees of acidulation. The degree of acidulation increases with an increase in the number.
The results of multiple regression for the PUE and grain yield are shown in Table 6. All regression equations were significant (p < 0.05) with a high R2 (0.59–0.88). However, the contribution of P fractions differed between the crops. For sorghum, the standardized partial regression coefficients suggest that the WP and SP fractions contributed equally to the PUE and grain yield. On the other hand, for cowpea, the contribution of SP to the PUE and grain yield was only 43 and 54% that of WP, respectively. Moreover, the contribution of SP to the grain yield was not significant.
A strong positive correlation between the PUE and increased grain yield (Figure 3) emphasized the importance of P uptake on increasing the grain yield (36, 40). Therefore, the fertilization effect of local P fertilizers is hereafter discussed using the PUE.
The significantly smaller PUE of CPs compared to TSP and the comparable performance of PAPRs with SSP both for sorghum and cowpea (Figure 4C) can be explained by the effective P fractions (Table 6). CP with relatively low solubility (41) did not significantly contribute to the PUE and grain yield compared with WP and SP. For sorghum, WP and SP had an almost equal contribution to the PUE and grain yield whereas the contribution was much smaller than that of WP for cowpea. Therefore, CPs, which mainly consisted of SP and CP fractions, had a disadvantage compared to TSP (Table 2), especially for cowpea. In contrast, PAPRs contained WP and SP, and their composition increased with increasing acidulation levels (Table 2) as described by Chien and Menon et al. (25) and Mizane and Rehamnia (16). From these results, we concluded that PAPRs were effective for sorghum, and that when the acidulation level is sufficiently high, they would be effective for cowpea as well. This conclusion was supported by the difference in the period of demand for P between sorghum and cowpea. First, the growing period of sorghum, 130 days (128 and 131 days in this study), is much longer than that of cowpea, 80 days (79 and 82 days in this study). Second, the highest correlation with grain yield was observed in the number of grains in 2018 and the ripening rate in 2019 (Table 2) for sorghum. These yield components are determined in the later growth stage. In contrast, the grain yield of cowpea was strongly controlled by the pod number, which is determined in the early growth stage (42, 43). Therefore, sorghum is more favorable for absorbing the slow-release P fraction i.e., SP. Moreover, Gambín and Borrás, (44) documented the plasticity of one-grain weight of sorghum to increase assimilate availability, suggesting that even if the sorghum plant fails to establish a sufficient number of kernels, it could recover the grain yield by increasing the one-grain weight. From these results, we concluded that the effects of local P fertilizer application were generally higher for sorghum than for cowpea (Figure 4C). The results of the present study could facilitate the development of local fertilizers in Burkina Faso, as well as in other countries in the Sudan Savanna. Further research using locally developed P fertilizers in different regions could also facilitate the determination of optimal P fertilization methods and guide the development of fertilizers adapted to the local conditions.
The annual difference in grain yield was observed both for sorghum and cowpea (Figure 2), and the difference for sorghum was clearer than that for cowpea (η2 in Table 4). In 2019, the sorghum and cowpea yields were reduced by 77 and 39%, respectively, compared with those in 2018. These results may also be attributed to the different growth periods between two crops. The rainy season generally starts from May in this region; however, in 2019, only a small amount of rainfall was observed in June (Supplementary Figure S1) and the available soil water in SW1 for sorghum was significantly lower in 2019 than in 2018 (Figure 1) and was particularly low at 0–2 WAS in 2019 (<4.2 mm). As a result, a substantial number of plants died (0–41%), resulting in a small grain yield (Figure 2). Sorghum is known as a drought-tolerant crop (18). However, yield depression by water stress at some growth stages has also been documented (19–21, 45, 46). Mastrorilli et al. (20) reported that the impact of water stress on grain yield was the largest in the early flowering period. The different sensitivity against water stress was explained by the different water requirement at each growth stage (19, 46). In this study, water stress was only observed in SW1 during which water requirement is generally low (47), but sorghum plants were suffering from intense water stress. This might be because a sufficient amount of water is generally irrigated before sowing in the previous studies in US and thus, water stress was not observed at 0–2 WAS. In India, Yadav et al. (21) showed that the influence of water deficit was the highest at the vegetative stage (corresponding to SW1 in this study) compared to the flowering and grain filling stages.
On the contrary, cowpea was sowed at 2 WAS of sorghum when a sufficient amount of rainfall was obtained (Supplementary Figure S1), and the available soil water in SW1 for cowpea was constantly high in both 2018 and 2019 (Figure 1). Furthermore, cowpea was harvested from the end of September to the beginning of October when the soil had enough moisture (Figure 1). The cowpea could avoid water stress because of its short growth duration, indicating that the cowpea is more tolerant to water shortage at the beginning of the rainy season. However, annual differences in the grain yield and grain P uptakes were still observed for cowpea, and the difference was more obvious in the harvest index rather than in the biomass and in PTE rather than the total P uptake (Figure 2). Thus, water stress might not be the factor that decreased the harvest index and PTE in cowpea. Among the yield components, the number of pods (m2) had the highest contribution to the grain yield of cowpea (r = 0.97 and 0.94 in 2018 and 2019, respectively) as reported in previous studies (48–50). Despite the similar biomass in 2018 and 2019 (Figure 2), the pod number was significantly smaller in 2019 than in 2018 (Table 3; Supplementary Figure S2). Ziska and Hall (50) indicated the importance of solar radiation during the flowering period on the pod number. The solar radiation around the 50% flowering (40 and 42 DAS in 2018 and 2019, respectively) in this study was relatively lower in 2019 than in 2018. Therefore, solar radiation can be an important factor limiting the pod number and consequently the grain yield as described by Tesfaye et al. (51), even though the annual yield variation of cowpea in this region was reported to be primarily caused by a difference in the amount of available soil water (35).
P fertilization significantly increased the grain yield of sorghum and cowpea. The WP and SP equally contributed to the PUE and grain yield for sorghum. However, only the WP significantly contributed to these parameters for cowpea. Therefore, CPs, which mainly consisted of SP and CP fractions, had a disadvantage, especially for cowpea. The annual difference in grain yield was clearly observed for sorghum compared with cowpea. Sorghum yield was significantly decreased by water stress at the early growth stage in the 2nd year. In contrast, cowpea could avoid water stress owing to its short growing period. We thus concluded that PAPRs were effective for sorghum cultivation, and would be effective for cowpea as well, when the acidulation level is increased. We also conclude that the long growing period for sorghum is favorable for absorbing slow-release P (i.e., SP fraction), but is unfavorable for the variable rainfall often observed in this region. The results of the present study could facilitate the development of local fertilizers and establishment of optimal P fertilization approaches in Burkina Faso, as well as in other countries in the Sudan Savanna.
The original contributions presented in the study are included in the article/Supplementary Material, further inquiries can be directed to the corresponding author.
SI: methodology, management of the field experiment and data collection, formal analysis, investigation, writing—original draft preparation, and writing—review and editing. KI: conceptualization, methodology, investigation, and writing—review and editing. AB: management of the field experiment and data collection, methodology, and writing—review and editing. FN: conceptualization and writing—review and editing. All authors contributed to the article and approved the submitted version.
This work was financially supported by the Science and Technology Research Partnership for Sustainable Development (SATREPS), Japan Science and Technology Agency (JST)/Japan International Cooperation Agency (JICA) (Project on establishment of the model for fertilizing cultivation promotion using Burkina Faso phosphate rock).
The authors declare that the research was conducted in the absence of any commercial or financial relationships that could be construed as a potential conflict of interest.
All claims expressed in this article are solely those of the authors and do not necessarily represent those of their affiliated organizations, or those of the publisher, the editors and the reviewers. Any product that may be evaluated in this article, or claim that may be made by its manufacturer, is not guaranteed or endorsed by the publisher.
The authors thank technical staff members (Mr. Mohamed Ouedraogo and Mr. Kafando Serge Placide) for supporting our field trials, as well as JIRCAS's Soil Team for soil chemical analysis. Soil samples were imported with a plant phytosanitary certificate of the Ministry of Agriculture, Forestry and Fisheries, Japan.
The Supplementary Material for this article can be found online at: https://www.frontiersin.org/articles/10.3389/fsoil.2021.709507/full#supplementary-material
1. FAO IFAD and WFP. The State of Food Insecurity in the World. Strengthening the Enabling Environment For Food Security and Nutrition. Rome: FAO (2014).
2. Garrity DP, Akinnifesi FK, Ajayi OC, Weldesemayat SG, Mowo JG, Kalinganire A, et al. Evergreen agriculture: a robust approach to sustainable food security in Africa. Food Sec. (2010) 2:197–214. doi: 10.1007/s12571-010-0070-7
3. Schmidhuber J, Tubiello FN. Global food security under climate change. Proc Natl Acad Sci USA. (2007) 104:19703–8. doi: 10.1073/pnas.0701976104
4. Callo-Concha D, Gaiser T, Webber H, Tischbein B, Müller M, Ewert F. Farming in the West African Sudan Savanna: Insights in the context of climate change. Afr J Agric Res. (2013) 8:4693–705. doi: 10.5897/AJAR2013.7153
5. Niang A, Becker M, Ewert F, Dieng I, Gaiser T, Tanaka A, et al. Variability and determinants of yields in rice production systems of West Africa. Field Crops Res. (2017) 207:1–12. doi: 10.1016/j.fcr.2017.02.014
6. Sanfo S, Barbier B, Dabiré IWP, Vlek PLG, Fonta WM, Ibrahim B, et al. Rainfall variability adaptation strategies: an ex-ante assessment of supplemental irrigation from farm ponds in southern Burkina. Faso Agric Syst. (2017) 152:80–9. doi: 10.1016/j.agsy.2016.12.011
7. Saito K, Vandamme E, Johnson J-M, Tanaka A, Senthilkumar K, Dieng I, et al. Yield-limiting macronutrients for rice in sub-Saharan Africa. Geoderma. (2018) 338:546–54. doi: 10.1016/j.geoderma.2018.11.036
8. Chien SH, Prochnow LI, Tu S, Snyder CS. Agronomic and environmental aspects of phosphate fertilizers varying in source and solubility: an update review. Nutr Cycl Agroecosyst. (2011) 89:229–55. doi: 10.1007/s10705-010-9390-4
9. Kelly VA. Factors affecting demand for fertilizer in sub-Saharan Africa. Agric Rural Dev. (2006) 23:89.
10. Zapata F, Roy RN. Use of phosphate rocks for sustainable agriculture. FAO Fertilizer Plant Nutr Bull. (2004) 1–48.
11. Van Straaten P. Rocks for crops: Agrominerals of sub-Saharan Africa. ICRAF; University of Guelph, Nairobi, Kenya: Guelph, Canada (2002).
12. Nakamura S, Fukuda M, Nagumo F, Tobita S. Potential utilization of local phosphate rocks to enhance rice production in sub-Saharan Africa. JARQ. (2013) 47:353–63. doi: 10.6090/jarq.47.353
14. Akiyama T, Tsumita K, Wada Y. Constituent minerals and solubility of calcinated phosphate of Na[[sb]]2[[/s]]O-CaO-MgO-P[[sb]]2[[/s]]O[[sb]]5[[/s]]-SiO[[sb]]2[[/s]] system made from high-silica phosphate ore. Jpn J Soil Sci Plant Nutr. (1992) 63:658–63.
15. Frederick ED, Roth EN. Sulfuric acid-based partially acidulated phosphate rock: its production, cost, and use, Technical bulletin / IFDC. International Fertilizer Development Center, Muscle Shoals, Ala., U.S.A (1986).
16. Mizane A, Rehamnia R. Study of some parameters to obtain the P[[sb]]2[[/s]]O[[sb]]5[[/s]] water- soluble from partially acidulated phosphate rocks (PAPRs) by sulfuric acid. Phosphorus Res Bull. (2012) 27:18–22. doi: 10.3363/prb.27.18
17. Nakamura S, Kanda T, Imai T, Sawadogo J, Nagumo F. Solubility and application effects of African low-grade phosphate rock calcinated with potassium carbonate. Soil Sci Plant Nutr. (2019) 65:267–73. doi: 10.1080/00380768.2019.1598236
18. Hadebe ST, Mabhaudhi T, Modi AT. Water use of sorghum (Sorghum bicolor L. Moench) in response to varying planting dates evaluated under rainfed conditions. Water SA. (2017) 43:91. doi: 10.4314/wsa.v43i1.12
19. Bell JM, Schwartz R, McInnes KJ, Howell T, Morgan CLS. Deficit irrigation effects on yield and yield components of grain sorghum. Agric Water Manag. (2018) 203:289–96. doi: 10.1016/j.agwat.2018.03.002
20. Mastrorilli M, Katerji N, Rana G. Water efficiency and stress on grain sorghum at different reproductive stages. Agric Water Manag. (1995) 28:23–34. doi: 10.1016/0378-3774(95)01160-K
21. Yadav SK, Lakshmi NJ, Maheswari M, Vanaja M, Venkateswarlu B. Influence of water deficit at vegetative, anthesis and grain filling stages on water relation and grain yield in sorghum. Indian J Plant Physiol. (2005) 10:5.
22. Kamara AY, Omoigui LO, Kamai N, Ewansiha SU, Ajeigbe HA. Improving cultivation of cowpea in West Africa. Burleigh Dodds Science Publishing (2018) p. 235–52.
23. FAOSTAT. Food and Agriculture Organization of the United Nations Statistics Database, FAOSTAT. (2021). Available online at: http://www.fao.org/faostat/en
24. Bationo A, Mokwunye AU. Alleviating soil fertility constraints to increased crop production in West Africa: the experience in the Sahel. Dev Plant Soil Sci. (1991) 47. doi: 10.1007./978-94-011-3224-4_18
25. Chien SH, Menon RG. Factors affecting the agronomic effectiveness of phosphate rock for direct application. Fertilizer Res. (1995) 41:227–34. doi: 10.1007/BF00748312
26. Iwasaki S, Fukuda M, Ikazaki K, Nakamura S, Ouattara K, Nagumo F. Optimal P fertilization using low-grade phosphate rock-derived fertilizer for rice cultivation under different ground-water conditions in the Central Plateau of Burkina Faso. Soil Sci Plant Nutr. (2021) 1–11. doi: 10.1080/00380768.2021.1932584
27. Fukuda M, Soma DM, Iwasaki S, Nakamura S, Kanda T, Ouattara K, et al. Site-specific responses of lowland rice to acidulated and calcined phosphate rock fertilizers in the Center-West region of Burkina Faso. PLoS ONE. (2021) 16:e0250240. doi: 10.1371/journal.pone.0250240
28. González Jiménez JL, Healy M, Daly K. Effects of fertiliser on phosphorus pools in soils with contrasting organic matter content: a fractionation and path analysis study. Geoderma. (2019) 338:128–35. doi: 10.1016/j.geoderma.2018.11.049
29. Rafael RBA, Fernández-Marcos ML, Cocco S, Ruello ML, Weindorf DC, Cardelli V, et al. Assessment of potential nutrient release from phosphate rock and dolostone for application in acid soils. Pedosphere. (2018) 28:44–58. doi: 10.1016/S1002-0160(17)60437-5
30. Jones A, Breuning-Madsen H, Brossard M, Dampha A, Deckers J, Dewitte O, et al. EU. Soil Atlas of Africa. (eds). Luxembourg: European Commission, Publications Office of the European Union (2013).
31. Ikazaki K, Nagumo F, Simporé S, Barro A. Soil toposequence, productivity, and a simple technique to detect petroplinthites using ground-penetrating radar in the Sudan Savanna. Soil Sci Plant Nutr. (2018) 64:623–31. doi: 10.1080/00380768.2018.1502604
32. FAMIC. Testing methods for fertilizers. Saitama, Japan: Incorporated Administrative Agency, Food and Agricultural Materials Inspection Center (FAMIC) (2013). p. 370
33. Campbell Scientific. CS616 and CS625 Water Content Reflectometers Instruction Manual. Logan, Utah, USA: Campbell Scientific (2020).
34. Borges CS, Weindorf DC, Carvalho GS, Guilherme LRG, Takayama T, Curi N, et al. Foliar elemental analysis of Brazilian crops via portable x-ray fluorescence spectrometry. Sensors. (2020) 20:2509. doi: 10.3390/s20092509
35. Iseki K, Ikazaki K, Batieno JB. Cowpea yield variation in three dominant soil types in the Sudan Savanna of West Africa. Field Crops Res. (2021) 261:108012. doi: 10.1016/j.fcr.2020.108012
36. Gitari HI, Karanja NN, Gachene CKK, Kamau S, Sharma K, Schulte-Geldermann E. Nitrogen and phosphorous uptake by potato (Solanum tuberosum L.) and their use efficiency under potato-legume intercropping systems. Field Crops Res. (2018) 222:78–84. doi: 10.1016/j.fcr.2018.03.019
37. R Core Team. A language environment for statistical computing. Vienna, Austria: R Foundation for Statistical Computing (2020). Available online at: https://www.R-project.org/.
38. Donoghue JR. Implementing Shaffer's multiple comparison procedure for a large number of groups. IMS Lecture Notes Monogr Ser. (2004) 47:1–23. doi: 10.1214/lnms/1196285622
40. Oyeyiola YB, Omueti JAI. Phosphorus uptake and use efficiency by cowpea in phosphocompost and chemical fertilizer treated nutrient degraded acid soils. ARTOAJ. (2016) 2:555578. doi: 10.19080/ARTOAJ.2016.02.555578
41. Santos WO, Mattiello EM, Barreto MSC, Cantarutti RB. Acid ammonium citrate as P extractor for fertilizers of varying solubility. Rev Bras Ciênc Solo. (2019) 43:e0180072. doi: 10.1590/18069657rbcs20180072
42. Ismail AM, Hall AE. Reproductive-stage heat tolerance, leaf membrane thermostability and plant morphology in cowpea. Crop Sci. (1999) 39:1762–8. doi: 10.2135/cropsci1999.3961762x
43. Nielsen CL, Hall AE. Responses of cowpea (Vigna unguiculata (L.) Walp.) in the field to high night air temperature during flowering. II. Plant responses. Field Crops Res. (1985) 10:181–196. doi: 10.1016/0378-4290(85)90025-5
44. Gambín BL, Borrás L. Plasticity of sorghum kernel weight to increased assimilate availability. Field Crops Res. (2006) 100:272–84. doi: 10.1016/j.fcr.2006.08.002
45. Jabereldar AA, Naim AME, Abdalla AA, Dagash YM. Effect of water stress on yield and water use efficiency of sorghum (Sorghum bicolor L. Moench) in semi-arid environment. Int J Agric Forest. (2017) 7:1–6. doi: 10.5923/j.ijaf.20170701.01
46. Bell JM, Schwartz RC, McInnes KJ, Howell TA, Morgan CLS. (2020). Effects of irrigation level and timing on profile soil water use by grain sorghum. Agric Water Manag. 232:106030. doi: 10.1016/j.agwat.2020.106030
47. Stichler C, McFarland ML, Coffman CG. Irrigated and dryland sorghum production in south and southwest Texas. (1997). Texas Farmer Collection. Available online at: http://hdl.handle.net/1969,1./87776
48. Eriksen FI, Whitney AS. Effects of solar radiation regimes on growth and N[[sb]]2[[/s]] fixation of soybean, cowpea, and bushbean. Agronomy J. (1984) 76:7. doi: 10.2134/agronj1984.00021962007600040006x
49. Mbuma NW, Gerrano AS, Lebaka N, Mofokeng A, Labuschagne M. The evaluation of a southern African cowpea germplasm collection for seed yield and yield components. Crop Sci. (2021) 61:466–89. doi: 10.1002/csc2.20336
50. Ziska LH, Hall AE. Seed yields and water use of cowpeas (Vigna unguiculata [L.] walp.) subjected to planned-water-deficit irrigation. Irrig Sci. (1983). 3:237–45. doi: 10.1007/BF00272839
Keywords: Lixisols, Sub-Saharan Africa (SSA), phosphorus use efficiency, upland crops, water stress
Citation: Iwasaki S, Ikazaki K, Bougma A and Nagumo F (2022) Appropriate Use of Local Phosphate Rock Increases Phosphorus Use Efficiency and Grain Yield of Sorghum and Cowpea in the Sudan Savanna. Front. Soil Sci. 1:709507. doi: 10.3389/fsoil.2021.709507
Received: 14 May 2021; Accepted: 13 December 2021;
Published: 06 January 2022.
Edited by:
Tales Tiecher, Federal University of Rio Grande do Sul, BrazilReviewed by:
Djalma Eugênio Schmitt, Federal University of Santa Catarina, BrazilCopyright © 2022 Iwasaki, Ikazaki, Bougma and Nagumo. This is an open-access article distributed under the terms of the Creative Commons Attribution License (CC BY). The use, distribution or reproduction in other forums is permitted, provided the original author(s) and the copyright owner(s) are credited and that the original publication in this journal is cited, in accordance with accepted academic practice. No use, distribution or reproduction is permitted which does not comply with these terms.
*Correspondence: Shinya Iwasaki, aXdhc2FraXNAamlyY2FzLmFmZnJjLmdvLmpw
Disclaimer: All claims expressed in this article are solely those of the authors and do not necessarily represent those of their affiliated organizations, or those of the publisher, the editors and the reviewers. Any product that may be evaluated in this article or claim that may be made by its manufacturer is not guaranteed or endorsed by the publisher.
Research integrity at Frontiers
Learn more about the work of our research integrity team to safeguard the quality of each article we publish.