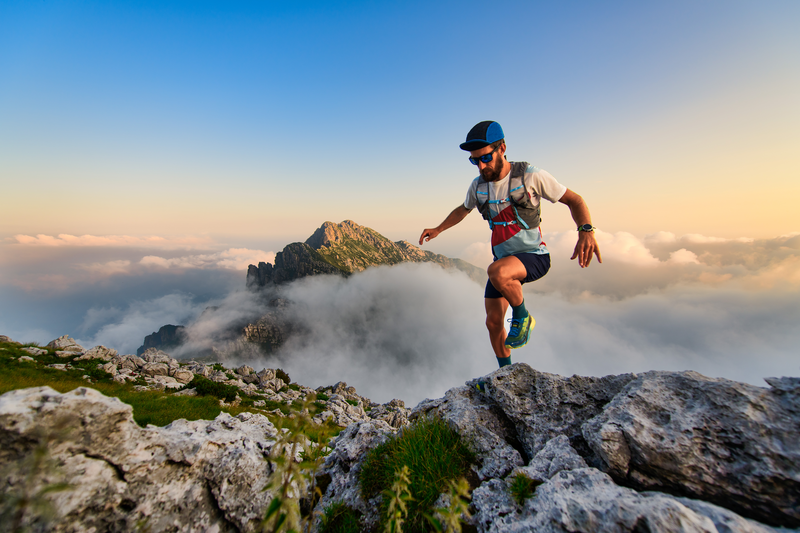
94% of researchers rate our articles as excellent or good
Learn more about the work of our research integrity team to safeguard the quality of each article we publish.
Find out more
PERSPECTIVE article
Front. Water , 12 February 2025
Sec. Water and Critical Zone
Volume 7 - 2025 | https://doi.org/10.3389/frwa.2025.1547094
Beavers (Castor canadensis) have not been adequately included in critical zone research, yet they can affect multiple critical zone processes across the terrestrial-aquatic interface of river corridors. River corridors (RC) provide a disproportionate amount of ecosystem services. Over time, beaver activity, including submersion of woody vegetation, burrowing, dam building, and abandonment, can impact critical zone processes in the river corridor by influencing landscape evolution, biodiversity, geomorphology, hydrology, primary productivity, and biogeochemical cycling. In particular, they can effectively restore degraded riparian areas and improve water quality and quantity, causing implications for many important ecosystem services. Beaver-mediated river corridor processes in the context of a changing climate require investigation to determine how both river corridor function and critical zone processes will shift in the future. Recent calls to advance river corridor research by leveraging a critical zone perspective can be strengthened through the explicit incorporation of animals, such as beavers, into research projects over space and time. This article illustrates how beavers modify the critical zone across different spatiotemporal scales, presents research opportunities to elucidate the role of beavers in influencing Western U.S. ecosystems, and, more broadly, demonstrates the importance of integrating animals into critical zone science.
The river corridor– comprised of the channel, floodplain, and hyporheic zone (Harvey and Gooseff, 2015; Wymore et al., 2023) – plays a fundamental role in landscape evolution, material transport, and resource provisioning. These are all important processes in the critical zone (CZ), which extends from the underlying bedrock to the vegetation canopy (Brantley et al., 2007). Approaching the study of the river corridor through the lens of CZ science is useful for conducting effective research across spatiotemporal scales. Indeed, the CZ exerts strong controls on river corridor processes – such as interactions of the geologic template with climate dictating river corridor structure and function (Wymore et al., 2023). While CZ research in the river corridor has advanced significantly in recent years, more CZ studies should incorporate the role of animals, particularly in shaping the river corridor.
The North American Beaver (Castor canadensis) is an exemplary species for exploring how animals can create fundamental changes to CZ processes in the river corridor. Beavers modify geomorphological, hydrological, ecological, and biogeochemical processes over time and their activity creates amplifying feedbacks. For example, when beaver dams split river flows, they create multiple flow paths for other beavers to dam and split again (Polvi and Wohl, 2012). Here, we argue that macrofauna exert important controls on CZ processes, and we use beavers in the river corridor as an example. Joining previous research highlighting the importance of animals (Schmitz et al., 2014), we present a framework for integrating beavers into CZ science. We first, briefly describe the ways that beavers modify the CZ across spatial and temporal scales, and then present opportunities for incorporating beavers into CZ studies within Western U.S. ecosystems. Although we do not present a full review of the impacts of beavers on the critical zone (e.g., Larsen et al., 2021; Brazier et al., 2021), this perspective aims to highlight the need for including animals in integrative CZ studies.
Beavers forage and fell large wood and other organic material to use as their food supply, as well as in the construction of dams and lodges (Brazier et al., 2021). These structures are supplemented with sediment from their ponds and the surrounding floodplain (Larsen et al., 2021). Beaver dams can attenuate river flows and retain water, sediment, nutrients, and carbon; reverse channel incision; and increase channel-floodplain connectivity (Naiman et al., 1994; Pollock et al., 2014; Rees et al., 2024). Ponds force water across the floodplain, increasing the potential for anoxic soil conditions and hydrological connectivity both laterally and vertically (Westbrook et al., 2006). Together, these changes can promote the formation of more abundant niche habitats and increase concentrations of bioavailable nutrients (Larsen et al., 2021). Over decadal timescales, beavers can create meadows, which are typically low-gradient valleys with multi-thread channels and connected ponds (Levine and Meyer, 2014; Polvi and Wohl, 2012). Changing river corridor morphology increases the capacity of streams to attenuate extreme flows and release stored water via base flow during dry periods (Jordan and Fairfax, 2022; Macfarlane et al., 2017; Wegener et al., 2017). Beavers commonly create a complex mosaic of eco-geomorphic units, or patches with distinctive elevations relative to the channel, grain size characteristics, and vegetation assemblages (Fryirs and Brierley, 2022; Laurel and Wohl, 2018).
Beaver populations have rebounded since their near extirpation in the 19th century and their return comes under a unique set of conditions. Beavers are now a part of landscapes where human activities have drastically modified the vast majority of U.S. river corridors, and climate change has altered precipitation and streamflow patterns (Reuss, 2004; Morrison et al., 2023). Water resources are strained from intensive demand in water-scarce regions, and water quality degradation is associated with fertilizer and pesticide dispersal (Reuss, 2004; Wohl et al., 2017). Human impacts have caused deleterious effects on river corridors, contributing to an increase in river restoration efforts (Bernhardt et al., 2005; Whigham, 1999). Beavers, and their associated impact on river corridors, often coincide with river restoration goals (Bernhardt et al., 2005; Curran and Cannatelli, 2014) and are being introduced for rewilding and restoring riverways across the U.S. (Cluer and Thorne, 2014; Larsen et al., 2021; Law et al., 2017; Puttock et al., 2017; Westbrook et al., 2020). Despite a growing body of research across the fields of ecology, hydrology, biogeochemistry, and geomorphology that includes beavers, their role in the evolution of, and linkages within, the CZ has received insufficient attention in interdisciplinary CZ research, particularly at the regional scale. The challenges associated with large-scale research have limited a holistic understanding of the potential benefits and challenges associated with a beaver-dominated landscape. Expansion of beaver populations can result in human-beaver conflicts (Pilliod et al., 2018; Siemer et al., 2013). Studies are mixed, but beaver dams may negatively impact some fish populations by impeding migration (Mitchell and Cunjak, 2007) or creating thermal stressors (Johnson-Bice et al., 2018); other studies have shown beaver dams do not negatively impact fish passage and can reduce thermal stress by increasing groundwater inputs (Johnson-Bice et al., 2018; Dittbrenner et al., 2022; Weber et al., 2017). Although there may be some limited negative impacts of beaver reintroduction and population expansion, landscape changes caused by the loss of beavers in the 19th century, including river incision, depleted aquifers, lower plant and animal biodiversity, erosion, and changes in element balances (Law et al., 2016; Wohl et al., 2017), indicate the return of beavers will likely have significant positive effects on river function.
Increasing beaver populations further underscores the importance of highlighting animal influences on the CZ. To our knowledge, there has not been a comprehensive, large-scale population survey of beaver populations in North America, South America, or Eurasia, but there have been significant efforts to estimate population growth using mapping, literature reviews, field observations, and modeling. In North America, beaver populations have been increasing since the 19th century (Naiman et al., 1988) with some estimates indicating approximately 30 million individuals in 2000 (Whitfield et al., 2015). In South America, 50 North American beavers were introduced to Tierra Del Fuego in 1940 by the Argentine government. That initial population has grown to an estimated 100,000 individuals in 2008, and their activity promotes the encroachment of invasive species (Choi, 2008), although there is evidence that they can provide ecological benefits to some native species (Arismendi et al., 2020). Skewes et al. (2006) estimated that the beaver population on the Chilean side of Tierra Del Fuego was 60,000 individuals with a land colonization rate of 2.6–6.3 km/year. Beaver populations in Eurasia are estimated at 1.5 million individuals in 2020 and have rapidly expanded their range since 2000 (Halley et al., 2021). Poland saw an increase from 1,000 individuals in 1987 to around 120,000 in 2017 (Wróbel and Krysztofiak-Kaniewska, 2020). Together, the research suggests that beaver populations are expanding on all continents, creating ecological implications both for newly colonized and recolonized areas.
Beavers change molecular, pond, and reach scale river processes, creating the foundation for changes that, over time, can lead to the evolution of CZ processes, and even the structure of the CZ, at watershed and regional scales (Figure 1). Past review articles have summarized the effects beavers have on the river corridor (Brazier et al., 2021; Larsen et al., 2021). Here, we focus on how molecular-to-reach scale beaver activity has the potential to affect regional scale CZ processes. The cumulative, multi-scalar effects of beaver activity could play a key role in buffering severe climate and human pressures.
Figure 1. Conceptual model of the scales of influence (far left column) beavers have on the critical zone. The center left column depicts landscapes at different scales without beavers and the center right column with beavers. Example research opportunities are highlighted in the far-right column.
Beaver ponds function as maintained wetlands, where inundation and enhanced microbial activity deplete dissolved oxygen, stimulating anaerobic microbial metabolisms and the use of alternate electron acceptors (Larsen et al., 2021). The reduced redox state alters the production, storage, and export of nutrients, carbon, and metals, with potential implications for water quality (Briggs et al., 2013, 2019). Additionally, beaver activity in the river corridor enhances geomorphic complexity, creating a diverse mosaic of eco-geomorphic units that provide variable redox conditions (Murray et al., 2023). The dominant drivers of biogeochemical change are the submersion and retention of organic material, attenuation of water and sediment flows, expansion of anoxic and reducing conditions, increased lateral and vertical hydrological connectivity, and enhanced microbial activity (Battin et al., 2016; Cirmo and Driscoll, 1993, 1996; Driscoll et al., 1998; Janzen and Westbrook, 2013; Laurel and Wohl, 2018; Levanoni et al., 2015; Roy et al., 2009a). Biologically mediated processes combined with submerged organic matter stimulate the reduction of reactive elements such as nitrate and sulfate (Cirmo and Driscoll, 1993; Larsen et al., 2021). Murray et al. (2023) demonstrated that beaver ponds can be a sink or source of nitrate depending on the season, while others have shown that ponds limit the inflow of nitrate but export ammonium (Cirmo and Driscoll, 1993). In regions where nitrate is in excess (e.g., 1,000 kg km−2), beaver ponds can significantly reduce concentrations via denitrification, acting to improve water quality (Lazar et al., 2015).
Conversely, the reduction of sulfate and availability of inorganic mercury can stimulate the production of methylmercury (MeHg), a neurotoxin that bioaccumulates and magnifies in food webs (Driscoll et al., 1998, 2013). In an unimpounded, aerated stream, mercury predominately stays in its oxidized form and is rapidly transported downstream. In a beaver-dominated stream, the increased water residence time facilitates the production of MeHg in the anoxic sediments, remobilization of MeHg into the water column, and assimilation of dissolved and particulate MeHg into biota (Figure 1). Further, the growth and decomposition of autochthonous vegetation in the beaver ponds results in increased availability of inorganic mercury and labile carbon that can lead to mercury methylation (Figure 1). However, variable rates of MeHg production have been observed in ponds of different ages (Driscoll et al., 1998; Levanoni et al., 2015; Roy et al., 2009a,b). Enhanced MeHg production, storage, export, and bioavailability may have negative implications for water quality and ecosystem health (Painter et al., 2015). Mercury methylation is not unique to beaver ponds, but rather all water bodies that host similar conditions, including wetlands, lakes, reservoirs, and estuaries. These aquatic environments can experience higher rates of mercury methylation, particularly because the global mercury pool has been increasing since the preindustrial era (Streets et al., 2019). Importantly, the magnitude of demethylation rates (i.e., MeHg removal) in beaver ponds are not well quantified and could significantly reduce the health risk of mercury exposure for wildlife and people (Levanoni et al., 2015). Other elements have similarly complex cycles in beaver ponds, and quantifying the dynamics will enhance our understanding of the controls that beavers have on the molecular scale, with potential impacts for downstream water quality.
Beaver dams change hydrological and geomorphic connectivity within the river corridor by attenuating incoming fluxes of water, sediment, organic material, and nutrients (Larsen et al., 2021). These changes can increase the physical complexity of the river corridor and change element balances. Past studies demonstrate that beavers can reduce peak discharge by up to 30% (Puttock et al., 2017) and annual discharge by 8% (Correll et al., 2000). Beaver dams force water onto the floodplain, increasing lateral connectivity (Brazier et al., 2021) and elevating evapotranspiration; the latter can be by as much as 50–150% in riparian areas ranging in size from 476 to 1,029 km2 (Fairfax and Small, 2018; Woo and Waddington, 1990).
Sediment retention, saturation of floodplain soils, and increased pressure gradients from pond creation can promote hyporheic exchange between the groundwater and surface flows (Janzen and Westbrook, 2013; Lautz et al., 2006; Tonina and Buffington, 2009). These physical changes can increase solute residence time by up to 30% (Lautz et al., 2006). Enhanced vertical connectivity and water residence time can raise the riparian water table via increased infiltration (Briggs et al., 2012; Polvi and Wohl, 2013; Westbrook et al., 2006; Wohl et al., 2017) and promote recharge pathways (Larsen et al., 2021). The formation of these ephemeral ponds on the landscape mediates the confluence of intersecting flow paths and the retention of complementary reactants. The result is biogeochemical hotspots within the river corridor that create novel pathways for element cycling compared to an unimpacted stream reach (McClain et al., 2003).
Beaver ponds promote deposition of sediment and associated constituents (e.g., organic material, nutrients, solutes) by reducing flow velocities within the channel (Pollock et al., 2007; Rees et al., 2024). Flow deflection due to pond creation can also promote bank erosion. Beavers can displace significant amounts of soil into the floodplain via excavation of canals and burrows (Hood and Larson, 2015). Naiman et al. (1986) found significantly greater sediment retention in a beaver pond (125 kg m−2) compared to a riffle without beaver ponds (1 kg m−2). Pond sedimentation rates are variable, with estimates of 0.4–47 cm year−1 (Butler, 2012; Meentemeyer and Butler, 1999; Naiman et al., 1986; Nummi et al., 2018; Rees et al., 2024). Associated with sediment deposition, McCreesh et al. (2019) showed that ponds were sinks for carbon and nitrogen Naiman and Melillo (1984) estimated that a beaver pond accumulated ~103 times more nitrogen than an undammed riffle. A summary of the effects of ponding are illustrated in Figure 1, where the beaver pond has greater evaporation, gaseous emission, sedimentation rates, organic matter deposition, enhanced vertical and lateral hydrologic connectivity, and mixing and mineralization compared with the unimpounded stream. The process changes that beavers make to river corridors at the pond scale, combined with the amplification of these changes across multiple ponds, establish the foundation for regional scale alterations to CZ processes.
Beaver activity can modify river corridor form, sediment and carbon storage, water dynamics, and vegetation communities at the reach scale. Beavers can create beaver meadows, which are typically low-gradient, multi-thread areas with numerous ponds, rich organic soils, and wetland vegetation (Gurnell, 2016; Ruedemann and Schoonmaker, 1938). Beaver meadows are hydrologically complex (Grudzinski et al., 2019; Hood and Larson, 2015; Larsen et al., 2021; Woo and Waddington, 1990), with changing surface and subsurface connectivity that varies with seasonal flows (Gurnell, 2016). Figure 1 illustrates how a beaver-dominated reach will differ from an unimpounded reach, with the former hosting multi-thread channels, a greater diversity of vegetation, and a heterogeneous landscape with ponded water disconnected from the main channel. Beaver dams increase reach-scale water residence time (Puttock et al., 2017), which promotes sediment trapping (Levine and Meyer, 2014). Dams are permeable; for example, through overtopping, flow through porous dams, or seepage below the structure, rivers may experience increased base flows during dry periods (Hood and Bayley, 2008; Puttock et al., 2021).
Together, the changing water and sediment dynamics can promote river meandering, resulting in multi-thread channel patterns (Levine and Meyer, 2014; Polvi and Wohl, 2012). According to Polvi and Wohl (2012), distributed flow paths and lower velocities can lead to greater fine sediment deposition in the banks and floodplains, increasing bank cohesion via fine sediment deposition and riparian vegetation growth. In addition, beaver dams can cause avulsions and side channel formation, resulting in multiple surface flow paths (Polvi and Wohl, 2013).
Sequential beaver dams promote feedback loops that further enhance dissipation of stream energy, hydrologic connectivity, and sediment/organic matter retention (Gurnell, 2016). Dissolved and particulate nutrients are also retained and increase primary productivity (Gurnell, 2016). Niche aquatic habitats form in beaver-dominated river corridors due to pooling, warmer water, increased nutrients, and enhanced light availability, thereby increasing biodiversity (Pollock et al., 2003; Polvi and Wohl, 2013; Rosell et al., 2005; Westbrook et al., 2011; Wohl et al., 2017). For example, both McDowell and Naiman (1986) and Ford and Naiman (1988) found a greater density of invertebrates in beaver ponds compared to an unimpacted riffle due to the soft bottom sediments in the pond promoting growth. Together, the impacts of beavers on the river corridor – wetter reaches, increased plant and animal diversity, and the creation of refugia – strengthen landscape resilience against drought, floods, and wildfire (Fairfax and Whittle, 2020; Nyssen et al., 2011).
Beavers manipulate reach scale carbon distribution by their direct inputs into the ponds, including submersion and trapping of woody material, thereby promoting vegetation growth within the ponds and trapping upstream inputs. Naiman et al. (1986) showed that a beaver pond receives nearly twice as much sediment carbon than a riffle per unit of channel length, the turnover time of which is nearly seven-fold greater than the riffle. Carbon transported or produced in beaver ponds can be stored longer due to reduced decomposition rate associated with increased anoxia (McDowell and Naiman, 1986; Wohl, 2013a). Beavers also increase carbon storage in the adjacent floodplain (Correll et al., 2000; Naiman et al., 1986; Wohl, 2013b) but the magnitude and timescales are not well quantified. Importantly, the magnitude of stored carbon is partially offset by emissions of carbon dioxide (CO2) and methane (CH4) which are enhanced in wetland environments. Researchers have shown that CO2 (0.14 to 11.2 g CO2 m−2 day−1, Roulet et al., 1997; Yavitt and Fahey, 1994), and CH4 emissions (27 to 919 mg CH4 m−2 day−1, Bubier et al., 1993; Ford and Naiman, 1988; Nummi et al., 2018), can be elevated in beaver ponds. However, the net carbon balance in beaver ponds is not well quantified over varying spatial and temporal scales and is further complicated by landscape heterogeneity, and the conditions that give rise to storage versus loss are not well known (Nummi et al., 2018).
Over decades to centuries, widespread beaver activity has the capacity to exert controls on watershed and regional mass balances of carbon (Nummi et al., 2018), water (Karran et al., 2018), and sediment (Pollock et al., 2007); increase landscape resilience (Hood and Larson, 2015); and influence the effect of changing climate forcing (i.e., precipitation patterns) on the CZ (Larsen et al., 2021). However, over broad spatial extents and long temporal scales, the effects of beavers have been poorly characterized. Assessing their cumulative effects over larger spatial extents, such as on water quality and quantity, is particularly important in the drought-prone Western U.S. (Harvey and Gooseff, 2015). Beaver-dominated watersheds enhance water storage in the surface and subsurface and can have more consistent streamflow (Scamardo et al., 2022; Wegener et al., 2017). Increased plant productivity in the margins of beaver-active areas, as well as higher carbon storage in beaver ponds, could promote long-term carbon storage in watersheds (Laurel and Wohl, 2018; Naiman et al., 1986; Rees et al., 2024; Wohl, 2013a). At the same time, the extent of open water, anoxia, and submerged organic matter may result in significant evaporation, as well as emission of greenhouse gases, which would reduce carbon storage (Cazzolla Gatti et al., 2018). As discussed for the reach scale, the degree of carbon burial and storage versus emissions of carbon gases will determine whether beaver-active areas at the watershed scale are net sinks or net sources of carbon over short versus longer timescales; this is an important, open question.
The increased vegetation cover and primary production within beaver-active reaches likely increases transpiration (Fairfax and Small, 2018), but the increased diversity of plant and animal species also generates ecological stability (Johnson-Bice et al., 2022), which further promotes landscape resilience. To predict regional scale impacts of widespread beaver activity we need to reconcile the complex interactions and feedbacks among local scale beaver activity (e.g., geomorphology, biogeochemistry, hydrology), the changing climate, and human stressors. Creating a more holistic understanding of beavers’ impact on the CZ and its resource output at large scales is a challenging but critical objective. In addition to ecosystem mass balance changes, beavers can play an important role in restoring river corridor function (Brazier et al., 2021; Law et al., 2017; Rosell et al., 2005). With human assistance, beaver populations could expand significantly and help to achieve river corridor restoration goals. With appropriate environmental conditions for their survival, they have the potential to colonize large swaths of river corridors over decadal time scales (Johnston and Naiman, 1990; Tape et al., 2018). Although the modern human-dominated river corridor will prevent beaver populations from expanding to their pre-European colonization size, research using the Beaver Restoration Assessment Tool (BRAT) in Colorado suggests that the state’s stream network could support approximately 1.3 million beaver dams (Scamardo et al., 2022). The potential impacts of regional scale beaver activity may include significant increases in landscape resilience, sediment and water storage, biodiversity, and reduced nutrient export (Figure 1). Indeed, Figure 1 shows how a watershed dominated by beaver has the potential to improve landscape resilience against disturbances. In the beaver-dominated panel, the watershed is more resistant to wildfire due to greater water inundation, filtering out burned particulates because of the increased water residence time, and higher groundwater levels because of greater vertical connectivity compared to the watershed without beavers. As researchers look to understand how river corridor function will respond to intensifying human and climate pressures, quantifying the complex spatial and temporal effects beavers have on the CZ will be an important component.
Animal activity influences CZ processes and landscape evolution, yet animals are relatively absent from the CZ literature. The dearth of studies integrating animal controls on CZ processes presents an opportunity for researchers to advance knowledge of the CZ by investigating the consequences of beavers and other animals over different spatial and temporal scales. Beyond beavers, a diverse group of animals, including pocket gophers (Gabet et al., 2014), wild boars (Mauri et al., 2019), elephants (Haynes, 2012), hippopotamus (Schoelynck et al., 2019), and others (Gabet et al., 2014; Jones et al., 1996; Mauri et al., 2019; Schmitz et al., 2014) are considered ecosystem engineers and have been impacting a variety of CZ processes. Together, organisms such as these influence the evolution of the physical template on which ecological processes occur (Law et al., 2016), yet we lack the research needed to predict how landscapes will evolve under the combined forces of animal activity, anthropogenic stressors, and the changing climate. As we consider research opportunities for the CZ community, we again use beavers as an example of how animal activity has direct implications for understanding CZ structure and function. Specifically, we discuss interdisciplinary research opportunities that we view as useful to determine beaver’s influence on mediating disturbances and promoting storage of water and other constituents. We also highlight the need to understand human-animal interactions and potential conflicts within the CZ framework. Although there are many possible studies that will contribute to understanding how beaver activity influences CZ processes, we present three that we believe are especially pressing given the current environmental challenges in the Western U.S.
Beavers exert strong controls on hydrologic connectivity and water storage that can buffer ecosystems against stress and expedite ecosystem recovery after large disturbances such as floods, droughts, and wildfires. In the Western U.S., severe, large disturbances are occurring more frequently in parallel with dwindling water resources (Anderson and Woosley, 2005; Siirila-Woodburn et al., 2021), highlighting the need to shift land management strategies to enhance resiliency. Investigating the potential role of beaver activity in light of these objectives will provide insight into beneficial land management practices and expand our theoretical understanding of CZ science.
Large wildfires have increased in frequency and intensity in the Western U.S. (Iglesias et al., 2022), a trend that will likely continue due to climate change and past land management (Dennison et al., 2014). Severe wildfires modify water quality and surface processes by increasing erosion (McGuire et al., 2024; Moody and Martin, 2009), decreasing carbon storage, and elevating nitrogen availability, turbidity, and black carbon in surface waters (Hohner et al., 2019). Beaver ponds can reduce the load of toxic compounds released into surface water because of sedimentation and chemical transformations (Roth et al., 2022). Significant state and federal funding is allocated to help prevent and fight wildfires, as well as rehabilitating fire impacted landscapes across the Western U.S. (Bernhardt et al., 2005; Wohl et al., 2022). More research is needed to investigate how beaver-dominated river corridors might provide refugia during wildfires, contribute to ecosystem recovery, and serve as sites for the retention and transformation of fire-impacted fluxes (Fairfax and Whittle, 2020; Wohl et al., 2022). Current research suggests that beavers could offer an effective method for achieving these goals (Norman et al., 2022).
Extreme precipitation events are expected to become more intense under a warming climate. When combined with growing human populations and increased development, they are causing higher costs associated with flood damage (Corringham et al., 2019; Prein and Mearns, 2021). Beaver ponds can reduce the magnitude of flow released and attenuate the flood hydrograph (Brazier et al., 2021; Larsen et al., 2021). Increased shrubby vegetation near beaver ponds also helps attenuate stream flow by creating areas of greater hydraulic roughness (Thomas and Nisbet, 2007). Flow attenuation can mitigate the impacts of flooding downstream. However, the potential for flood mitigation due to beaver is not well constrained. Neumayer et al. (2020) found that flood attenuation and the delay of peak timing were only significant for smaller discharge events and had a greater effect in low slope, high floodplain connectivity sites. Others have found that flow attenuation was greatest during large flooding events (Nyssen et al., 2011; Puttock et al., 2017). Often, water overtops the dam but it does not fully collapse (Westbrook et al., 2020). This research suggests that beaver dams have the potential to enhance flood mitigation, but the specific outcomes of different flow conditions remain uncertain. There is a need for more accurate assessments of the capacity of beaver damming to change the range of catchment flood magnitudes, partially motivated by the desire to re-introduce beavers for the goal of flood management (Larsen et al., 2021).
Increased water residence time in beaver ponds enhances the storage of sediment, water, solutes, and particulates. The temporal scale of storage varies significantly depending on the spatial extent of beaver ponds, the permanence of the dams, and the frequency and severity of disturbance events like floods and fires (Larsen et al., 2021). Harnessing beaver activity to achieve river corridor restoration goals is becoming more common, yet more research is needed to quantify the spatial and temporal scale of storage – of water, sediment, solutes, and particulates and the constituents entrained within such as mercury and carbon. Long term carbon sequestration due to beaver activities promoting sedimentation is of particular interest due to elevated atmospheric carbon concentrations and the growing interest in understanding whether river restoration can increase carbon storage in the river corridor (Lininger and Lave, 2024). River corridors can have high concentrations of organic carbon compared to upland soils, which may be further enhanced by beaver activity (Knox et al., 2022; Sutfin et al., 2016). Beavers create spatially complex river corridors that are dynamic over time, which creates challenges when accounting for spatial variability in carbon storage and fluxes (e.g., sequestration rates, efflux, export) (Lininger and Lave, 2024).
Future work should assess the net impact of beaver activity over larger spatial extents and timescales. Research on carbon dynamics in beaver ponds indicates that beaver activity generally increase carbon storage in sediment and potentially riparian vegetation (Johnston, 2014; Laurel and Wohl, 2018; Lazar et al., 2015; Naiman et al., 1986; Nummi et al., 2018; Rees et al., 2024; Weyhenmeyer, 1999; Wohl, 2013a). Novel beaver activity can potentially create initial reductions in carbon storage (Naiman et al., 1994) and increases in gaseous emissions (Nummi et al., 2018). To date, we are not aware of studies that complete a comprehensive carbon mass balance accounting for changes in all pools and fluxes influenced by beaver activity, especially over long enough timescales to assess the net impact (Larsen et al., 2021; Nummi et al., 2018; Wohl, 2013a). Carbon is a well-studied element in the CZ community (Wymore et al., 2023), yet studies on carbon dynamics rarely incorporate beavers. Two key components must be investigated to better quantify the carbon storage potential of beaver activity: (1) does the increased storage of carbon via sedimentation outweigh gaseous emissions? and, if so, (2) over what time scale does carbon storage occur and for how long? The significant impact that beavers have on carbon, their growing populations in the Western U.S., and the increased interest in their use in river corridor restoration suggest that beavers should be incorporated into river carbon models.
In addition to climate considerations, the expansion of beaver populations has implications for the impacts of mercury biogeochemistry on water quality and ecosystem health, which are poorly characterized. For instance, despite beaver activity usually increasing MeHg concentrations in water and sediment (Čiuldienė et al., 2020; Driscoll et al., 1998; Roy et al., 2009b), to our knowledge, demethylation (Levanoni et al., 2015) has not been measured in beaver ponds. Additionally, MeHg production can vary significantly depending on ecosystem characteristics. For instance, Roy et al. (2009a) found clear differences in MeHg concentrations in beaver ponds within coniferous versus mixed forests. The toxicity of MeHg and its poorly quantified biogeochemistry in beaver ponds requires further research, especially as beavers are increasingly used in riparian restoration. We need to understand the potential negative effects that beavers might have on water quality and ecosystem health. However, if there are potential negative effects, we need to quantify those effects to compare beaver impacts on MeHg production with human-caused MeHg production (e.g., through creating reservoirs and increasing atmospheric deposition) given the important ecosystem services that beavers provide. To further quantify mercury cycling in beaver-dominated river corridors we need to quantify: (1) spatial heterogeneity of MeHg storage, (2) the rates of methylation in different areas within the river corridor, and (3) rates of demethylation in water and sediment.
Beavers have the potential to increase surface and subsurface water storage, a particularly relevant impact as water demands increase across the Western U.S. (Leeper et al., 2022; Rippey, 2015). One of the factors exacerbating drought conditions in the Western U.S is the loss of wetland environments and a conversion of wetlands to managed ecosystems (Eagles-Smith et al., 2016; UEPA, 2016; Fairfax and Whittle, 2020). Wetlands are vital for managing landscapes’ water resources, including water quality improvement and flood abatement (UEPA, 2016). Beavers create wetlands (Larsen et al., 2021) and may provide a means of repairing degraded river ecosystems and increasing water storage, with implications for downstream water availability. To further understand of how beaver impact water dynamics, research efforts could investigate: (1) the degree to which beaver activity increases connectivity among surface water, groundwater, and the floodplain, (2) the magnitude of water storage in ponds and groundwater from raised water tables, and (3) water budgets to assess comprehensively the impact of beaver on water flows in the landscape. Additionally, water storage becomes increasingly unclear as we account for different environmental conditions across regions and expand our scope to greater spatial and temporal scales.
We can expand our perspective from only examining how individual animals impact CZ processes to the effects of animals in the context of trophic cascades, which can start due to human intervention. For instance, Hebblewhite et al. (2005) describe how the exclusion of wolves from one area due to human use in Banff National Park resulted in elevated elk populations, leading to decreased aspen recruitment and willow production due to elk browsing. Declines in riparian vegetation caused a decline in beaver lodge density due to a lack of resources for beaver persistence. Reduced beaver populations, and declines in diverse riparian habitat, led to a decrease in riparian animals. Thus, the loss of beavers on the landscape can have further profound and wide-ranging effects on larger ecosystem function (Larsen et al., 2021). In the Banff National Park example, the prioritization of human activity, leading to the exclusion of wolves, and the cascading effects on the ecosystem had impacts on landscape dynamics. It is important to note that the impact of wolves on trophic cascades is debated, and others have found that restoring apex predators to ecosystems failed to achieve specified goals (Hobbs et al., 2023). A CZ approach to research could aid in quantifying energy, water, and nutrient fluxes and provide land managers with evidence to inform decision-making.
The interaction between humans and beavers itself is an important consideration in CZ studies. Negative perceptions of beavers can impede efforts to expand their populations in regions where they could co-exist with humans. Beaver activity has historically been accompanied by social conflict (Pilliod et al., 2018) due to the increased likelihood of road flooding, property damage, and disease (Siemer et al., 2013). Because of humans’ challenges to coexist with beavers, researchers have found a correlation between personal experience with beaver-related problems and greater acceptability of lethal management (Siemer et al., 2013). Further, those who receive the benefits of beaver activity will have dramatically different perceptions of beaver activity than those who experience the cons (Brazier et al., 2020, 2021; Gaywood, 2018). Research is needed to improve understanding of the challenges associated with human-beaver co-existence. Specifically, we suggest: (1) assessing how beaver restoration is perceived by human communities; and (2) improving educational outreach about how to co-exist with beavers, similar to prior calls (Pilliod et al., 2018). Human-beaver co-existence is a key component not only of beaver reintroduction, but also CZ research. Human perception of beavers will dictate their abundance, and, in turn, the magnitude of effects that they have on the CZ.
In this article, we demonstrate that CZ science would benefit from greater consideration of the role that animals play in the evolution of landscapes, a perspective that is relatively absent from the CZ science framework. To understand how ecosystems will evolve under increasing human pressures, and to provide robust information to inform land managers, it is important to determine the short-and long-term eco-geomorphic effects of CZ engineers. We use beavers and their profound impacts on the river corridor—a key component of the CZ—as an example. We explore how beavers can create a landscape more resilient to the disturbances of wildfire, floods, and droughts; that has enhanced carbon sequestration and storage, retains sediment and nutrients; and supports a diversity of plants, animals, and landscape units. These beaver-induced changes contribute to a CZ that continues to provide essential ecosystem services in a changing world.
We highlight research opportunities that pave a way forward to address the unknowns of how beavers alter CZ structure and function through space and time. Resolving these questions will increase understanding of the impact that beavers have on CZ processes and evolution and advance basic CZ science. With continued efforts to restore ecosystems that have been degraded by human activity and prepare landscapes for continued climatic change, there is a need to animate the CZ by including animals in models and conceptualizations of CZ processes. The gap in understanding of how the present-day increase in beaver populations will impact the landscape requires multi-scale, interdisciplinary research. The results will inform land managers and restoration practitioners and advance theoretical CZ science.
The original contributions presented in the study are included in the article/supplementary material, further inquiries can be directed to the corresponding author.
CA: Conceptualization, Writing – original draft, Writing – review & editing, Visualization. KL: Conceptualization, Writing – original draft, Writing – review & editing. E-LH: Conceptualization, Writing – original draft, Writing – review & editing.
The author(s) declare that financial support was received for the research, authorship, and/or publication of this article. This work was supported by NSF Critical Zone Collaborative Network EAR-2012669.
The authors declare that the research was conducted in the absence of any commercial or financial relationships that could be construed as a potential conflict of interest.
The author(s) declare that no Gen AI was used in the creation of this manuscript.
All claims expressed in this article are solely those of the authors and do not necessarily represent those of their affiliated organizations, or those of the publisher, the editors and the reviewers. Any product that may be evaluated in this article, or claim that may be made by its manufacturer, is not guaranteed or endorsed by the publisher.
Anderson, M. T., and Woosley, L. H. W. (2005). Water availability for the Western United States—key scientific challenges. New York, NY: U. S. Geological Survey.
Arismendi, I., Penaluna, B. E., and Jara, C. G. (2020). Introduced beaver improve growth of non-native trout in Tierra del Fuego, South America. Ecol. Evol. 10, 9454–9465. doi: 10.1002/ece3.6636
Battin, T. J., Besemer, K., Bengtsson, M. M., Romani, A. M., and Packmann, A. I. (2016). The ecology and biogeochemistry of stream biofilms. Nat. Rev. Microbiol. 14, 251–263. doi: 10.1038/nrmicro.2016.15
Bernhardt, E. S., Palmer, M. A., Allan, J. D., Alexander, G., Barnas, K., Brooks, S., et al. (2005). Synthesizing U.S. river restoration efforts. Science 308, 636–637. doi: 10.1126/science.1109769
Brantley, S. L., Goldhaber, M. B., and Vala Ragnarsdottir, K. (2007). Crossing disciplines and scales to understand the critical zone. Elements 3, 307–314. doi: 10.2113/gselements.3.5.307
Brazier, R. E., Elliot, M., Andison, E., Auster, R. E., Bridgewater, S., Burgess, P., et al. (2020). River otter beaver trial: Science and evidence report. England: University of Exeter.
Brazier, R. E., Puttock, A., Graham, H. A., Auster, R. E., Davies, K. H., and Brown, C. M. L. (2021). Beaver: Nature’s ecosystem engineers. Wiley Interdiscip. Rev. Water 8:e1494. doi: 10.1002/wat2.1494
Briggs, M. A., Lautz, L. K., Hare, D. K., and González-Pinzón, R. (2013). Relating hyporheic fluxes, residence times, and redox-sensitive biogeochemical processes upstream of beaver dams. Freshwater Sci. 32, 622–641. doi: 10.1899/12-110.1
Briggs, M. A., Lautz, L. K., McKenzie, J. M., Gordon, R. P., and Hare, D. K. (2012). Using high-resolution distributed temperature sensing to quantify spatial and temporal variability in vertical hyporheic flux. Water Resour. Res. 48:227. doi: 10.1029/2011WR011227
Briggs, M. A., Wang, C., Day-Lewis, F. D., Williams, K. H., Dong, W., and Lane, J. W. (2019). Return flows from beaver ponds enhance floodplain-to-river metals exchange in alluvial mountain catchments. Sci. Total Environ. 685, 357–369. doi: 10.1016/J.SCITOTENV.2019.05.371
Bubier, J. L., Moore, T. R., and Roulet, N. T. (1993). Methane emissions from wetlands in the Midboreal region of northern Ontario, Canada. Ecology 74, 2240–2254. doi: 10.2307/1939577
Butler, D. R. (2012). Characteristics of beaver ponds on deltas in a mountain environment. Earth Surf. Process. Landf. 37, 876–882. doi: 10.1002/esp.3218
Cazzolla Gatti, R., Callaghan, T. V., Rozhkova-Timina, I., Dudko, A., Lim, A., Vorobyev, S. N., et al. (2018). The role of Eurasian beaver (Castor fiber) in the storage, emission and deposition of carbon in lakes and rivers of the river Ob flood plain, western Siberia. Sci. Total Environ. 644, 1371–1379. doi: 10.1016/J.SCITOTENV.2018.07.042
Cirmo, C. P., and Driscoll, C. T. (1993). Beaver pond biogeochemistry: acid neutralizing capacity generation in a headwater wetland. Wetlands 13, 277–292. doi: 10.1007/BF03161294
Cirmo, C. P., and Driscoll, C. T. (1996). The impacts of a watershed CaCO3 treatment on stream and wetland biogeochemistry in the Adirondack Mountains. Biogeochemistry 32, 265–297. doi: 10.1007/BF02187142
Čiuldienė, D., Vigricas, E., Belova, O., Aleinikovas, M., and Armolaitis, K. (2020). The effect of beaver dams on organic carbon, nutrients and methyl mercury distribution in impounded waterbodies. Wildl. Biol. 2020, 1–8. doi: 10.1111/wlb.00678
Cluer, B., and Thorne, C. (2014). A stream evolution model integrating habitat and ecosystem benefits. River Res. Appl. 30, 135–154. doi: 10.1002/RRA.2631
Correll, D. L., Jordan, T. E., and Weller, D. E. (2000). Beaver pond biogeochemical effects in the Maryland coastal plain. Biogeochemistry 2000 49, 217–239. doi: 10.1023/A:1006330501887
Corringham, T. W., Ralph, F. M., Gershunov, A., Cayan, D. R., and Talbot, C. A. (2019). Atmospheric rivers drive flood damages in the western United States. Science. Advances 5:eaax4631. doi: 10.1126/sciadv.aax4631
Curran, J. C., and Cannatelli, K. M. (2014). The impact of beaver dams on the morphology of a river in the eastern United States with implications for river restoration. Earth Surf. Process. Landf. 39, 1236–1244. doi: 10.1002/ESP.3576
Dennison, P. E., Brewer, S. C., Arnold, J. D., and Moritz, M. A. (2014). Large wildfire trends in the western United States, 1984–2011. Geophys. Res. Lett. 41, 2928–2933. doi: 10.1002/2014GL059576
Dittbrenner, B. J., Schilling, J. W., Torgersen, C. E., and Lawler, J. J. (2022). Relocated beaver can increase water storage and decrease stream temperature in headwater streams. Ecosphere 13:e4168. doi: 10.1002/ecs2.4168
Driscoll, C. T., Holsapple, J., Schofield, C. L., and Munson, R. (1998). The chemistry and transport of mercury in a small wetland in the Adirondack region of New York, USA. Biogeochemistry 40, 137–146. doi: 10.1023/A:1005989229089
Driscoll, C. T., Mason, R. P., Chan, H. M., Jacob, D. J., and Pirrone, N. (2013). Mercury as a global pollutant: sources, pathways, and effect. Environ. Sci. Technol. 47, 4967–4983. doi: 10.1021/es305071v
Eagles-Smith, C. A., Wiener, J. G., Eckley, C. S., Willacker, J. J., Evers, D. C., Marvin-DiPasquale, M., et al. (2016). Mercury in western North America: a synthesis of environmental contamination, fluxes, bioaccumulation, and risk to fish and wildlife. Sci. Total Environ. 568, 1213–1226. doi: 10.1016/j.scitotenv.2016.05.094
Fairfax, E., and Small, E. E. (2018). Using remote sensing to assess the impact of beaver damming on riparian evapotranspiration in an arid landscape. Ecohydrology 11:e1993. doi: 10.1002/ECO.1993
Fairfax, E., and Whittle, A. (2020). Smokey the beaver: beaver-dammed riparian corridors stay green during wildfire throughout the western United States. Ecol. Appl. 30:e02225. doi: 10.1002/EAP.2225
Ford, T. E., and Naiman, R. J. (1988). Alteration of carbon cycling by beaver: methane evasion rates from boreal forest streams and rivers. Can. J. Zool. 66, 529–533. doi: 10.1139/z88-076
Fryirs, K., and Brierley, G. (2022). Assemblages of geomorphic units: a building block approach to analysis and interpretation of river character, behaviour, condition and recovery. Earth Surf. Process. Landf. 47, 92–108. doi: 10.1002/esp.5264
Gabet, E. J., Perron, J. T., and Johnson, D. L. (2014). Biotic origin for Mima mounds supported by numerical modeling. Geomorphology 206, 58–66. doi: 10.1016/j.geomorph.2013.09.018
Gaywood, M. J. (2018). Reintroducing the Eurasian beaver Castor fiber to Scotland. Mammal Rev. 48, 48–61. doi: 10.1111/mam.12113
Grudzinski, B. P., Cummins, H., and Vang, T. K. (2019). Beaver canals and their environmental effects. Prog. Phys. Geogr. 44, 189–211. doi: 10.1177/0309133319873116
Gurnell, A. M. (2016). The hydrogeomorphological effects of beaver dam-building activity. Prog. Phys. Geogr. 22, 167–189. doi: 10.1177/030913339802200202
Halley, D. J., Saveljev, A. P., and Rosell, F. (2021). Population and distribution of beavers Castor fiber and Castor canadensis in Eurasia. Mammal Rev. 51, 1–24. doi: 10.1111/MAM.12216
Harvey, J., and Gooseff, M. (2015). River corridor science: hydrologic exchange and ecological consequences from bedforms to basins. Water Resour. Res. 51, 6893–6922. doi: 10.1002/2015WR017617
Haynes, G. (2012). Elephants (and extinct relatives) as earth-movers and ecosystem engineers. Geomorphology 157-158, 99–107. doi: 10.1016/j.geomorph.2011.04.045
Hebblewhite, M., White, C. A., Nietvelt, C. G., McKenzie, J. A., Hurd, T. E., Fryxell, J. M., et al. (2005). Human activity mediates a trophic Cascade caused by wolves. Ecology 86, 2135–2144. doi: 10.1890/04-1269
Hobbs, N. T., Johnston, D. B., Marshall, K. N., Wolf, E. C., and Cooper, D. J. (2023). Does restoring apex predators to food webs restore ecosystems? Large carnivores in Yellowstone as a model system. Ecol. Monogr. 94:e1598. doi: 10.1002/ecm.1598
Hohner, A. K., Rhoades, C. C., Wilkerson, P., and Rosario-Ortiz, F. L. (2019). Wildfires Alter Forest watersheds and threaten drinking water quality. Acc. Chem. Res. 52, 1234–1244. doi: 10.1021/acs.accounts.8b00670
Hood, G. A., and Bayley, S. E. (2008). Beaver (Castor canadensis) mitigate the effects of climate on the area of open water in boreal wetlands in western Canada. Biol. Conserv. 141, 556–567. doi: 10.1016/J.BIOCON.2007.12.003
Hood, G. A., and Larson, D. G. (2015). Ecological engineering and aquatic connectivity: a new perspective from beaver-modified wetlands. Freshw. Biol. 60, 198–208. doi: 10.1111/FWB.12487
Iglesias, V., Balch, J. K., and Travis, W. R. (2022). U.S. fires became larger, more frequent, and more widespread in the 2000s. Science. Advances 8:eabc0020. doi: 10.1126/sciadv.abc0020
Janzen, K., and Westbrook, C. J. (2013). Hyporheic flows along a Channelled peatland: influence of beaver dams. Canadian Water Res. J. 36, 331–347. doi: 10.4296/CWRJ3604846
Johnson-Bice, S. M., Gable, T. D., Windels, S. K., and Host, G. E. (2022). Relics of beavers past: time and population density drive scale-dependent patterns of ecosystem engineering. Ecography 2022:5814. doi: 10.1111/ECOG.05814
Johnson-Bice, S. M., Renik, K. M., Windels, S. K., and Hafs, A. W. (2018). A review of beaver–salmonid relationships and history of management actions in the Western Great Lakes (USA) region. N. Am. J. Fish Manag. 38, 1203–1225. doi: 10.1002/nafm.10223
Johnston, C. A. (2014). Beaver pond effects on carbon storage in soils. Geoderma 213, 371–378. doi: 10.1016/j.geoderma.2013.08.025
Johnston, C. A., and Naiman, R. J. (1990). Aquatic patch creation in relation to beaver population trends. Source. Ecology 71, 1617–1621. doi: 10.2307/1938297
Jones, C. G., Lawton, J. H., and Shachak, M. (1996). “Organisms as ecosystem engineers” in Ecosystem management: Selected readings. eds. F. B. Samson and F. L. Knopf (Cham: Springer), 130–147.
Jordan, C. E., and Fairfax, E. (2022). Beaver: the north American freshwater climate action plan. Wiley Interdiscip. Rev. Water 9:e1592. doi: 10.1002/wat2.1592
Karran, D. J., Westbrook, C. J., and Bedard-Haughn, A. (2018). Beaver-mediated water table dynamics in a Rocky Mountain fen. Ecohydrology 11:e1923. doi: 10.1002/ECO.1923
Knox, R. L., Morrison, R. R., and Wohl, E. E. (2022). A river ran through it: floodplains as America’s newest relict landform. Science. Advances 8:1082. doi: 10.1126/SCIADV.ABO1082
Larsen, A., Larsen, J. R., and Lane, S. N. (2021). Dam builders and their works: beaver influences on the structure and function of river corridor hydrology, geomorphology, biogeochemistry and ecosystems. Earth Sci. Rev. 218:103623. doi: 10.1016/J.EARSCIREV.2021.103623
Laurel, D., and Wohl, E. (2018). The persistence of beaver-induced geomorphic heterogeneity and organic carbon stock in river corridors. Earth Surf. Proc. Landforms 44, 342–353. doi: 10.1002/esp.4486
Lautz, L. K., Siegel, D. I., and Bauer, R. L. (2006). Impact of debris dams on hyporheic interaction along a semi-arid stream. PRO 20, 183–196. doi: 10.1002/hyp.5910
Law, A., Gaywood, M. J., Jones, K. C., Ramsay, P., and Willby, N. J. (2017). Using ecosystem engineers as tools in habitat restoration and rewilding: beaver and wetlands. Sci. Total Environ. 605-606, 1021–1030. doi: 10.1016/J.SCITOTENV.2017.06.173
Law, A., Mclean, F., and Willby, N. J. (2016). Habitat engineering by beaver benefits aquatic biodiversity and ecosystem processes in agricultural streams. Freshw. Biol. 61, 486–499. doi: 10.1111/FWB.12721
Lazar, J. G., Addy, K., Gold, A. J., Groffman, P. M., McKinney, R. A., and Kellogg, D. Q. (2015). Beaver ponds: resurgent nitrogen sinks for rural watersheds in the northeastern United States. J. Environ. Qual. 44, 1684–1693. doi: 10.2134/JEQ2014.12.0540
Leeper, R. D., Bilotta, R., Petersen, B., Stiles, C. J., Heim, R., Fuchs, B., et al. (2022). Characterizing U.S. drought over the past 20 years using the U.S. drought monitor. Int. J. Climatol. 42, 6616–6630. doi: 10.1002/joc.7653
Levanoni, O., Bishop, K., Mckie, B. G., Hartman, G., Eklöf, K., and Ecke, F. (2015). Impact of beaver pond colonization history on methylmercury concentrations in surface water. Environ. Sci. Technol. 49, 12679–12687. doi: 10.1021/acs.est.5b03146
Levine, R., and Meyer, G. A. (2014). Beaver dams and channel sediment dynamics on Odell Creek, Centennial Valley, Montana, USA. Geomorphology 205, 51–64. doi: 10.1016/J.GEOMORPH.2013.04.035
Lininger, K., and Lave, R. (2024). River restoration can increase carbon storage but is not yet a suitable basis for carbon credits. Bio Sci. 74, 717–724. doi: 10.1093/biosci/biae083
Macfarlane, W. W., Wheaton, J. M., Bouwes, N., Jensen, M. L., Gilbert, J. T., Hough-Snee, N., et al. (2017). Modeling the capacity of riverscapes to support beaver dams. Geomorphology 277, 72–99. doi: 10.1016/J.GEOMORPH.2015.11.019
Mauri, L., Sallustio, L., and Tarolli, P. (2019). The geomorphologic forcing of wild boars. Earth Surf. Process. Landf. 44, 2085–2094. doi: 10.1002/esp.4623
McClain, M. E., Boyer, E. W., Dent, C. L., Gergel, S. E., Grimm, N. B., Groffman, P. M., et al. (2003). Biogeochemical hot spots and hot moments at the Interface of terrestrial and aquatic ecosystems. Ecosystems 6, 301–312. doi: 10.1007/s10021-003-0161-9
McCreesh, R. K., Fox-Dobbs, K., Wimberger, P., Woodruff, K., Holtgrieve, G., and Pool, T. K. (2019). Reintroduced beavers rapidly influence the storage and biogeochemistry of sediments in headwater streams (Methow River, Washington). Oecologia 93, 112–121. doi: 10.3955/046.093.0203
McDowell, D. M., and Naiman, R. J. (1986). Structure and function of a benthic invertebrate stream community as influenced by beaver (Castor canadensis). Oecologia 68, 481–489. doi: 10.1007/BF00378759
McGuire, L. A., Ebel, B. A., Rengers, F. K., Vieira, D. C. S., and Nyman, P. (2024). Fire effects on geomorphic processes. Nat. Rev. Earth Environ. 5, 486–503. doi: 10.1038/s43017-024-00557-7
Meentemeyer, R. K., and Butler, D. R. (1999). Hydrogeomorphic effects of beaver dams in glacier national park, Montana. Phys. Geography 20, 436–446. doi: 10.1080/02723646.1999.10642688
Mitchell, S. C., and Cunjak, R. A. (2007). Stream flow, salmon and beaver dams: roles in the structuring of stream fish communities within an anadromous salmon dominated stream. J. Anim. Ecol. 76, 1062–1074. doi: 10.1111/J.1365-2656.2007.01286.X
Moody, J. A., and Martin, D. A. (2009). Synthesis of sediment yields after wildland fire in different rainfall regimes in the western United States. Int. J. Wildland Fire 18, 96–115. doi: 10.1071/WF07162
Morrison, R. R., Simonson, K., McManamay, R. A., and Carver, D. (2023). Degradation of floodplain integrity within the contiguous United States. Commun. Earth Environ. 4:215. doi: 10.1038/s43247-023-00877-4
Murray, D., Neilson, B. T., and Brahney, J. (2023). Beaver pond geomorphology influences pond nitrogen retention and denitrification. J. Geophys. Res. Biogeosci. 128:e2022JG007199. doi: 10.1029/2022JG007199
Naiman, R. J., Johnston, C. A., and Kelley, J. C. (1988). Alteration of north American streams by beaver. Bio Sci. 38, 753–762. doi: 10.2307/1310784
Naiman, R. J., and Melillo, J. M. (1984). Nitrogen budget of a subarctic stream altered by beaver (Castor canadensis). Oecologia 62, 150–155. doi: 10.1007/BF00379007
Naiman, R. J., Melillo, J. M., and Hobbie, J. E. (1986). Ecosystem alteration of boreal Forest streams by beaver (Castor Canadensis). Ecology 67, 1254–1269. doi: 10.2307/1938681
Naiman, R. J., Pinay, G., Johnston, C. A., and Pastor, J. (1994). Beaver influences on the long-term biogeochemical characteristics of boreal forest drainage networks. Ecology 75, 905–921. doi: 10.2307/1939415
Neumayer, M., Teschemacher, S., Schloemer, S., Zahner, V., and Rieger, W. (2020). Hydraulic modeling of beaver dams and evaluation of their impacts on flood events. Water 12:300. doi: 10.3390/W12010300
Norman, L. M., Lal, R., Wohl, E., Fairfax, E., Gellis, A. C., and Pollock, M. M. (2022). Natural infrastructure in dryland streams (NIDS) can establish regenerative wetland sinks that reverse desertification and strengthen climate resilience. Sci. Total Environ. 849:157738. doi: 10.1016/j.scitotenv.2022.157738
Nummi, P., Vehkaoja, M., Pumpanen, J., and Ojala, A. (2018). Beavers affect carbon biogeochemistry: both short-term and long-term processes are involved. Mammal Rev. 48, 298–311. doi: 10.1111/MAM.12134
Nyssen, J., Pontzeele, J., and Billi, P. (2011). Effect of beaver dams on the hydrology of small mountain streams: example from the Chevral in the Ourthe Orientale basin, Ardennes, Belgium. J. Hydrol. 402, 92–102. doi: 10.1016/j.jhydrol.2011.03.008
Painter, K. J., Westbrook, C. J., Hall, B. D., O’Driscoll, N. J., Jardine, T. D., and Peters, D. P. C. (2015). Effects of in-channel beaver impoundments on mercury bioaccumulation in Rocky Mountain stream food webs. Ecosphere 6, 1–17. doi: 10.1890/ES15-00167.1
Pilliod, D. S., Rohde, A. T., Charnley, S., Davee, R. R., Dunham, J. B., Gosnell, H., et al. (2018). Survey of beaver-related restoration practices in rangeland streams of the Western USA. Environ. Manag. 61, 58–68. doi: 10.1007/s00267-017-0957-6
Pollock, M. M., Beechie, T. J., and Jordan, C. E. (2007). Geomorphic changes upstream of beaver dams in Bridge Creek, an incised stream channel in the interior Columbia River basin, eastern Oregon. Earth Surf. Process. Landf. 32, 1174–1185. doi: 10.1002/esp.1553
Pollock, M. M., Beechie, T. J., Wheaton, J. M., Jordan, C. E., Bouwes, N., Weber, N., et al. (2014). Using beaver dams to restore incised stream ecosystems. Bio Sci. 64, 279–290. doi: 10.1093/biosci/biu036
Pollock, M. M., Heim, M., and Werner, D. (2003). Hydrologic and geomorphic effects of beaver dams and their influence on fishes. Am. Fish. Soc. Symp. 2003, 213–233.
Polvi, L. E., and Wohl, E. (2012). The beaver meadow complex revisited – the role of beavers in post-glacial floodplain development. Earth Surf. Process. Landf. 37, 332–346. doi: 10.1002/ESP.2261
Polvi, L. E., and Wohl, E. (2013). Biotic drivers of stream planform implications for understanding the past and restoring the future. Bio Sci. 63, 439–452. doi: 10.1525/BIO.2013.63.6.6
Prein, A. F., and Mearns, L. O. (2021). U.S. extreme precipitation weather types increased in frequency during the 20th century. J. Geophys. Res. Atmos. 126:e2020JD034287. doi: 10.1029/2020JD034287
Puttock, A., Graham, H. A., Ashe, J., Luscombe, D. J., and Brazier, R. E. (2021). Beaver dams attenuate flow: a multi-site study. Hydrol. Process. 35:e14017. doi: 10.1002/hyp.14017
Puttock, A., Graham, H. A., Cunliffe, A. M., Elliott, M., and Brazier, R. E. (2017). Eurasian beaver activity increases water storage, attenuates flow and mitigates diffuse pollution from intensively-managed grasslands. Sci. Total Environ. 576, 430–443. doi: 10.1016/J.SCITOTENV.2016.10.122
Rees, J. C., Lininger, K. B., Landis, J. D., and Briles, C. E. (2024). Assessing controls on sedimentation rates and sediment organic carbon accretion in beaver ponds. Sci. Total Environ. 949:174951. doi: 10.1016/j.scitotenv.2024.174951
Reuss, M. (2004). Designing the bayous: The control of water in the Atchafalaya Basin, 1800–1995. College Station, TX: Texas A&M University Press.
Rippey, B. R. (2015). The U.S. drought of 2012. Weather Clim. Extr. 10, 57–64. doi: 10.1016/j.wace.2015.10.004
Rosell, F., Bozsér, O., Collen, P., and Parker, H. (2005). Ecological impact of beavers Castor fiber and Castor canadensis and their ability to modify ecosystems. Mammal Rev. 35, 248–276. doi: 10.1111/J.1365-2907.2005.00067.X
Roth, H. K., Nelson, A. R., McKenna, A. M., Fegel, T. S., Young, R. B., Rhoades, C. C., et al. (2022). Impact of beaver ponds on biogeochemistry of organic carbon and nitrogen along a fire-impacted stream. Environ. Sci.: Processes Impacts 24, 1661–1677. doi: 10.1039/D2EM00184E
Roulet, N. T., Crill, P. M., Comer, N. T., Dove, A., and Boubonniere, R. A. (1997). CO2 and CH4 flux between a boreal beaver pond and the atmosphere. J. Geophys. Res. Atmos. 102, 29313–29319. doi: 10.1029/97JD01237
Roy, V., Amyot, M., and Carignan, R. (2009a). Beaver ponds increase methylmercury concentrations in Canadian shield streams along vegetation and pond-age gradients. Environ. Sci. Technol. 43, 5605–5611. doi: 10.1021/es901193x
Roy, V., Amyot, M., and Carignan, R. (2009b). Seasonal methylmercury dynamics in water draining three beaver impoundments of varying age. J. Geophys. Res. Biogeo. 114:763. doi: 10.1029/2008JG000763
Ruedemann, R., and Schoonmaker, W. J. (1938). Beaver-dams as geologic agents. Science 88, 523–525. doi: 10.1126/SCIENCE.88.2292.523
Scamardo, J. E., Marshall, S., Wohl, E., Julianne Scamardo, C. E., and Peters, C. (2022). Estimating widespread beaver dam loss: habitat decline and surface storage loss at a regional scale. Ecosphere 13:e3962. doi: 10.1002/ECS2.3962
Schmitz, O. J., Raymond, P. A., Estes, J. A., Kurz, W. A., Holtgrieve, G. W., Ritchie, M. E., et al. (2014). Animating the carbon cycle. Ecosystems 17, 344–359. doi: 10.1007/s10021-013-9715-7
Schoelynck, J., Subalusky, A. L., Struyf, E., Dutton, C. L., Unzué-Belmonte, D., Van de Vijver, B., et al. (2019). Hippos (Hippopotamus amphibius): the animal silicon pump. Science. Advances 5:eaav0395. doi: 10.1126/sciadv.aav0395
Siemer, W. F., Jonker, S. A., Decker, D. J., and Organ, J. F. (2013). Toward an understanding of beaver management as human and beaver densities increase. Hum. Wildlife Inter. 7, 114–131.
Siirila-Woodburn, E. R., Rhoades, A. M., Hatchett, B. J., Huning, L. S., Szinai, J., Tague, C., et al. (2021). A low-to-no snow future and its impacts on water resources in the western United States. Nat. Rev. Earth Environ. 2, 800–819. doi: 10.1038/s43017-021-00219-y
Skewes, O., Gonzalez, F., Olave, R., Ávila, A., Vargas, V., Paulsen, P., et al. (2006). Abundance and distribution of American beaver, Castor canadensis (Kuhl 1820), in Tierra del Fuego and Navarino islands, Chile. Eur. J. Wildlife Res. 52, 292–296. doi: 10.1007/s10344-006-0038-2
Streets, D. G., Horowitz, H. M., Lu, Z., Levin, L., Thackray, C. P., Sunderland, E. M., et al. (2019). Global and regional trends in mercury emissions and concentrations, 2010-2015. Atmos. Environ. 201, 417–427. doi: 10.1016/j.atmosenv.2018.12.031
Sutfin, N. A., Wohl, E. E., and Dwire, K. A. (2016). Banking carbon: a review of organic carbon storage and physical factors influencing retention in floodplains and riparian ecosystems. Earth Surf. Process. Landf. 41, 38–60. doi: 10.1002/esp.3857
Tape, K. D., Jones, B. M., Arp, C. D., Nitze, I., and Grosse, G. (2018). Tundra be dammed: beaver colonization of the Arctic. Glob. Chang. Biol. 24, 4478–4488. doi: 10.1111/gcb.14332
Thomas, H., and Nisbet, T. R. (2007). An assessment of the impact of floodplain woodland on flood flows. Water Environ. J. 21, 114–126. doi: 10.1111/j.1747-6593.2006.00056.x
Tonina, D., and Buffington, J. M. (2009). Hyporheic exchange in mountain Rivers I: mechanics and environmental effects. Compass 3, 1063–1086. doi: 10.1111/j.1749-8198.2009.00226.x
UEPA (2016). National wetland condition assessment: 2011 technical report. Washington, DC: US Environmental Protection Agency.
Weber, N., Bouwes, N., Pollock, M. M., Volk, C., Wheaton, J. M., Wathen, G., et al. (2017). Alteration of stream temperature by natural and artificial beaver dams. PLoS One 12:e0176313. doi: 10.1371/journal.pone.0176313
Wegener, P., Covino, T., and Wohl, E. (2017). Beaver-mediated lateral hydrologic connectivity, fluvial carbon and nutrient flux, and aquatic ecosystem metabolism. Water Resour. Res. 53, 4606–4623. doi: 10.1002/2016WR019790
Westbrook, C. J., Cooper, D. J., and Baker, B. W. (2006). Beaver dams and overbank floods influence groundwater–surface water interactions of a Rocky Mountain riparian area. Water Resour. Res. 42:6404. doi: 10.1029/2005WR004560
Westbrook, C. J., Cooper, D. J., and Baker, B. W. (2011). Beaver assisted river valley formation. River Res. Appl. 27, 247–256. doi: 10.1002/RRA.1359
Westbrook, C. J., Ronnquist, A., and Bedard-Haughn, A. (2020). Hydrological functioning of a beaver dam sequence and regional dam persistence during an extreme rainstorm. Hydrol. Process. 34, 3726–3737. doi: 10.1002/HYP.13828
Weyhenmeyer, C. E. (1999). Methane emissions from beaver ponds: rates, patterns, and transport mechanisms. Glob. Biogeochem. Cycles 13, 1079–1090. doi: 10.1029/1999GB900047
Whigham, D. F. (1999). Ecological issues related to wetland preservation, restoration, creation and assessment. Sci. Total Environ. 240, 31–40. doi: 10.1016/S0048-9697(99)00321-6
Whitfield, C. J., Chun, K. P., Baulch, H. M., and Westbrook, C. J. (2015). Beaver-mediated methane emission: The effects of population growth in Eurasia and the Americas. Ambio. 44, 7–15. doi: 10.1007/s13280-014-0575-y
Wohl, E. (2013a). Landscape-scale carbon storage associated with beaver dams. Geophys. Res. Lett. 40, 3631–3636. doi: 10.1002/grl.50710
Wohl, E. (2013b). Wilderness is dead: whither critical zone studies and geomorphology in the Anthropocene? Anthropocene 2, 4–15. doi: 10.1016/j.ancene.2013.03.001
Wohl, E., Lininger, K. B., and Baron, J. (2017). Land before water: the relative temporal sequence of human alteration of freshwater ecosystems in the conterminous United States. Anthropocene 18, 27–46. doi: 10.1016/J.ANCENE.2017.05.004
Wohl, E., Marshall, A. E., Scamardo, J., White, D., and Morrison, R. R. (2022). Biogeomorphic influences on river corridor resilience to wildfire disturbances in a mountain stream of the southern Rockies, USA. Sci. Total Environ. 820:153321. doi: 10.1016/j.scitotenv.2022.153321
Woo, M.-K., and Waddington, J. M. (1990). Effects of beaver dams on subarctic wetland hydrology. Arctic 43, 223–230. doi: 10.14430/arctic1615
Wróbel, M., and Krysztofiak-Kaniewska, A. (2020). Long-term dynamics of and potential management strategies for the beaver (Castor fiber) population in Poland. Eur. Zool. J. 87, 116–121. doi: 10.1080/24750263.2020.1727969
Wymore, A. S., Ward, A. S., Wohl, E., and Harvey, J. W. (2023). Viewing river corridors through the lens of critical zone science. Front. Water 5:1147561. doi: 10.3389/FRWA.2023.1147561
Keywords: biogeochemistry, fluvial geomorphology, Western United States, restoration, river corridor, beavers
Citation: Adamchak C, Lininger KB and Hinckley E-LS (2025) Animating the critical zone: beavers as critical zone engineers. Front. Water. 7:1547094. doi: 10.3389/frwa.2025.1547094
Received: 17 December 2024; Accepted: 28 January 2025;
Published: 12 February 2025.
Edited by:
Aleksandar Valjarević, University of Belgrade, SerbiaReviewed by:
Jelena Golijanin, University of East Sarajevo, Bosnia and HerzegovinaCopyright © 2025 Adamchak, Lininger and Hinckley. This is an open-access article distributed under the terms of the Creative Commons Attribution License (CC BY). The use, distribution or reproduction in other forums is permitted, provided the original author(s) and the copyright owner(s) are credited and that the original publication in this journal is cited, in accordance with accepted academic practice. No use, distribution or reproduction is permitted which does not comply with these terms.
*Correspondence: Clifford Adamchak, Y2xpZmZvcmQuYWRhbWNoYWtAY29sb3JhZG8uZWR1
Disclaimer: All claims expressed in this article are solely those of the authors and do not necessarily represent those of their affiliated organizations, or those of the publisher, the editors and the reviewers. Any product that may be evaluated in this article or claim that may be made by its manufacturer is not guaranteed or endorsed by the publisher.
Research integrity at Frontiers
Learn more about the work of our research integrity team to safeguard the quality of each article we publish.