- 1Faculty of Science, National Autonomous University of Mexico (UNAM), Ciudad de México, México
- 2Regional Center for Multidisciplinary Studies, National Autonomous University of Mexico (CRIM-UNAM), Cuernavaca, México
The research investigates integrated water management with complex socio-environmental interactions in worsening climate change scenarios. The proposed methodology involves the integration of Atmosphere–Ocean General Circulation Models (AOGCM) with Water Evaluation and Planning models (WEAP) to ensure water availability in the future. Two Shared Socioeconomic Pathways (SSP) integrate environmental, socioeconomic, and political elements under climate change scenarios with greater rainfall variability, extreme droughts, and floods in the metropolitan area of Morelos, located in the Center of México. Water availability in this region, along with population growth, industrialization, service activities, and agriculture, depends on forest conservation in the Ravines System of Northwest Morelos (RSNM). Public policy lacks interdisciplinary socio-environmental development, which prioritizes unsustainable economic growth overexploiting aquifers and polluting rivers. Official data from national and state governments do not reflect water conditions, and aquifer statistics date back decades. The majority of the analyzed models predict a delay in the monsoon, higher temperatures, extreme climate events, depletion of groundwater, and severe water scarcity during the hot months, rendering them unable to meet the increasing demand. This research provides valuable insights into the complex socioeconomic dynamics of a region with future water scarcity, which could be useful for similar conditions in the Global South.
1 Introduction
Mexico is experiencing a water crisis and pollution in nearly two-thirds of its municipalities (Healing Waters International, 2023). The Global Risk Report 2024 indicates that Mexico is the country with the fourth highest risk of climate change and the second in terms of vulnerability (World Economic Forum, 2024). The interaction of socio-environmental factors in rapidly growing cities has limited clean water supply in the Global South (Watch, 2022). About 36% of the world’s population (2.4 billion people) live in water-scarce regions, and half of them will experience severe water shortages (UN Habitat, 2022). Climate change with higher temperatures and alteration of the precipitation cycle has increased water stress due to dangerous extreme events (droughts and floods; IPCC, 2014; UN-WATER, 2019; Kikstra et al., 2022). National water policies have focused on solving problems related to supply and demand through a centralized, mercantile, and infrastructure approach. Inefficient planning, management, unfair appropriation of rents for private concessions, high pollution, and corruption hindered the human right to water, sanitation, and a healthy environment. Multiple policies tried to correct water management; however, most of them aligned with power struggles, gaps in the application of norms, surveillance, and sanction, and a lack of professional training (García Barrios and Mozka Estrada, 2023).
Climate change is closely associated with the hydrological cycle, rainfall variability, extreme events, and the predictability of water availability, aquifer recharge, economic activities, and livelihoods. The alteration of quantity and quality of rain related to predominant trade winds, including the jet stream (Kara and Sahin, 2023) affects biodiversity and water availability (UN-WATER, 2019). The future hydrological demand for the Global South is not reflective of local realities. Changes in rainfall patterns have an impact on crops and productive conversions. In regions with lower rainfall, the temperature rises, irregular monsoon water evaporates, droughts increase, and a lack of water affects the growing demand, especially in metropolitan areas (Gao et al., 2022; Sarker, 2022).
According to the Drought Monitor in Mexico, on 31 May 2024, 57.88% of the country suffered from lack of water (CONAGUA, 2023). The study area of the metropolitan area of Cuernavaca, in the center of México, was greatly impacted by chaotic urbanization, economic activities, and deforestation. Later monsoons, poor water infrastructure, and insufficient sanitation put the government under increasing water stress availability and safe sanitation (Montero-Martínez et al., 2010; IMTA, 2011; Martínez-Austria and Patiño-Gómez, 2012; IPCC, 2024; INECC-SEMARNAT, 2018; UN-WATER, 2019; CONAGUA, 2021; INECC, 2021). The available data do not include an interdisciplinary assessment of water resources from local stakeholders and public administrators (Höllermann et al., 2009). The current national and regional public policy lacks an interdisciplinary socio-environmental development by prioritizing economic growth at the cost of chaotic urbanization (Delgado Ramos, 2019), ecosystem deterioration (Jaramillo et al., 2018), overexploitation of aquifers (Oswald, 2023), pollution of rivers, and irrigation channels (Valladares-Cisneros et al., 2021).
Confronted with these challenges, the present study addresses integrated water management, including complex socio-environmental interactions in worsening climate change scenarios in the center of Mexico. The research simulates with various climate models the water availability for different users in a drought-stressed region in the metropolitan area of Cuernavaca in the Center of Mexico, where complex socio-ecological activities affect the existing supply, demand, and hydrological balance. This study represents similar conditions in other countries in the Global South that are affected by climate change, population growth, chaotic urbanization, unsustainable ecosystems, and a lack of water management. The present research explores different climate change projections for rainfall variability with extreme droughts and floods in the study region.
Water availability in this metropolitan region depends on forest conservation in the Ravines System of Northwest Morelos (RSNM), which provides water for population, industrialization, service activities, and agriculture. Morelos, situated at a lower elevation near the coast, observed the region’s highest monthly mean temperature in May (2024; 24.87°C) and the driest monthly mean precipitation in February (2.07 mm). The Projected Anomalies by State for the period 2040–2059 (Ref. Period 1995–2014) under SSP3-7.0 (estimate) an increase in maximum annual temperature of 2.01°C, 28.5% of more summer days. There are 57.5% of tropical nights above 20°C. It has a steep slope of 3,000 meters that generates torrential downpours and drags sediments (World Bank Group, 2024).
The study investigates the development of a Water Evaluation and Planning (WEAP) model for precipitation, temperature rise, and annual aquifer recharge, incorporating socioeconomic factors and population growth. Interviews with key political and social authors examined the hydrological balance of water distribution among users, ecosystem requirements, and potential climate change impacts. The methodology selected the three best and most optimal Atmosphere–Ocean General Circulation Models (AOGCM) for the distribution of precipitation based on climate data (1970–2000). It established projections for AOGCM CNRM-ESM2, CNRM-CM6, and MIROC6, taking into account the long-term (2081–2100) simulation. The same database (WorldClim, 2024) processed SSP 370 and 585 for climate change scenarios. This study explores the future water balance of a regional dryland with potential climate change impacts, population growth, lack of clean water, and conflicts. The research examines an Integrated Water Resources Management (IWRM) approach in the RSNM, an exceptional biodiverse ecosystem (Jaramillo et al., 2018) with highly permeability of the basaltic rocks as the principal recharge of the aquifer in the growing metropolitan area of Cuernavaca for human and productive activities. The conclusion indicates that the current policy of water management is inadequate, affecting human water needs, industrial activities, services, agriculture, and biodiversity.
The legal framework, political priorities, environmental management, and climate change uncertainty (Taylor et al., 2008) establish the conditions for an analysis of the available water resources, the user demands, and the socio-environmental conditions. The predictive study contributes to informed decision-making, public planning, adaptation, and resilience-building to climate change impacts in a complex regional socioecological system for the short, medium, and long term.
1.1 Case study in the metropolitan area of Cuernavaca, Morelos
Mexico is highly exposed to multiple risks and vulnerability (World Economic Forum, 2024) because of two warming oceans, two mountain chains along the seas (Sierra Madre), and a transversal volcanic chain with semiarid and arid ecosystems that are increasingly impacted by drought (northwest, Pacific coast), floods in the southeast, and extreme weather events on both coasts. The projected scenarios for the 21st century predict an annual average temperature rise of 3.5°C by 2061–2090, roughly in the range of 1961–1990. The precipitation will decrease by at least 15% (Montero-Martínez et al., 2010; Gobierno del Estado de Morelos, 2014) related to changing trade winds. The RIOCCADAPT Report (Moreno et al., 2020) states that Mexico is experiencing the highest risks in Latin America with temperature increase, precipitation decrease, and extreme river flows. The costs for damages and losses are estimated to be US$ 3,754 million. Oswald (2023) has warned for more than 20 years that climate change represents serious threats to Mexico, not only to more frequent and stronger hurricanes in coastal areas but also because of temperature rise and the alteration of the monsoon pattern with severe droughts and floods in the country (Oswald, 2016, 2023).
The UNAM Climate Change Research Program (PINCC) states that the average temperature rise in Mexico is about 1.69°C to 2°C, compared to the beginning of the 20th century (PINCC, 2024). This is higher than the global average (1.23°C). In 2023, the disastrous hurricane Otis in the Acapulco tourist resort generated the fourth highest annual cost of 16 billion dollars due to a 30°C higher sea water temperature (SwissRe, 2023). In 2024, Hurricane John produced in 5 days the annual precipitation affecting again Acapulco and 214 more municipalities. Climate change models also indicate precipitation changes, later monsoons, and higher temperatures. Rainfall distribution is extreme, with longer periods of drought and destructive floods (Estrada et al., 2023). CONAGUA forecasted five periods of extreme temperature in 2024 (CONAGUA, 2024). Climate change scenarios necessitate Mexico to improve its models for extreme events, thereby allowing preventive adaptation in the most vulnerable regions. Hotter climate scenarios include planning, learning-based approaches, and flexible solutions grounded on local data for building resilience to the predicted hydrological and climate changes (Pahl -Wostl, C., 2008).
The increasing impact of climate change creates uncertainty in humans and ecosystems (Jaramillo et al., 2018). High altitude and permeability in the RSNM on the northern Chichinautzin mountain chain recharges the aquifer, called the “Water Forest,” by dominant groundwater flows north–south and north-southwest (Pohle, 2006). As per official sources, the groundwater level in the aquifer is in equilibrium with some degree of overexploitation whenever multiple differences exist in the official data (Table 1)1. The forest in the Ravines System of Northwest Morelos (RSNM) (Figure 1) is critical for biodiversity, surface water, and aquifer recharge for Cuernavaca’s metropolitan areas. The preservation of this RSNM forest is vulnerable to changes in land use, deforestation, bushfires, and population growth coupled with social marginalization. This region also includes small endorheic micro-watersheds characterized by their biotic, edaphologic, and geological characteristics (Jaramillo et al., 2018; Oswald, 2023).
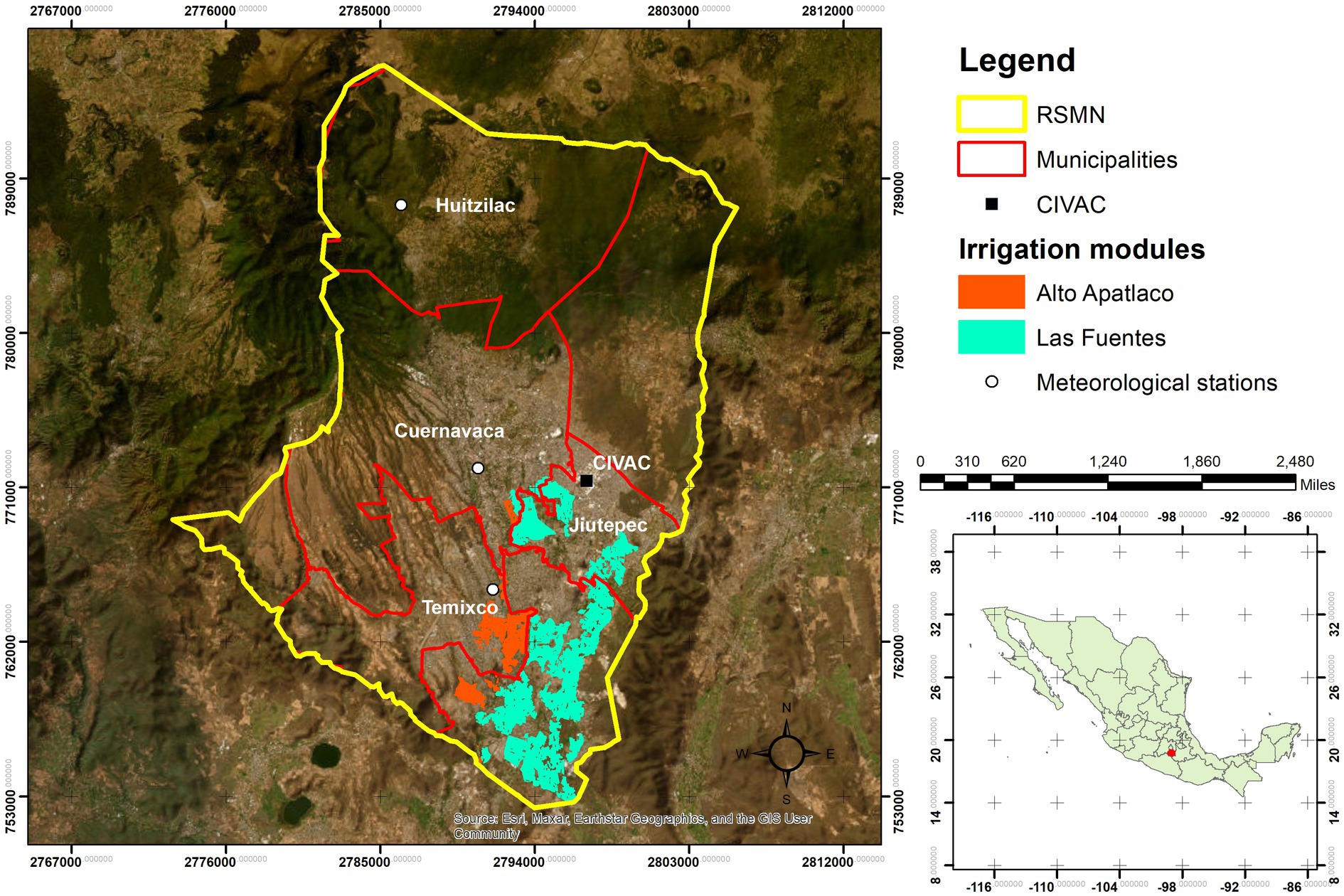
Figure 1. Study region. Own elaboration based on data from INEGI (2021).
Multiple sources of domestic or industrial wastewater, agriculture, livestock activities, Urban Solid Waste (USW), and soil erosion can contaminate water before it can serve the population. Bendig (1985) analyzed the highly fractured basalt rocks using isotopic studies and the DRASTIC methods, establishing a high vulnerability to contamination in the Cuernavaca aquifer (Bendig, 1985). Her isotopic studies dermonstrate that the main recharge comes from the north, corresponding to the Chichinautzin. Tritium concentrations suggest that groundwater age is young and does not exceed 42 years. The extraction by wells is concentrated in the megalopolis, generating some local sinking and altering the local flows toward the south. Since 2010, the average annual precipitation has been above 980 mm, and the study region is privileged with climate and water resources due to higher rainfall in this northern zone. During the dry season, the surface flows are reduced by 20–50% (CONAGUA, 2020). Climate change projections for the region simulate temperature rise and rainfall variability with extreme events (floods and droughts) that affect water quantity, exacerbate pollution, and deteriorate ecosystems (Gobierno del Estado de Morelos, 2014).
2 Methods
The three adequate AOGCM for the study region with the information from eight AOGCM of precipitation for SSP 370 and 585 were downloaded from the WorldClim portal (https://www.worldclim.org) between 1970 and 2000 and for the future of 2081–2100 to analyze climate change scenarios and future water demand for the study region. A system perspective integrates climate change uncertainty into simulations, and the simulations examine regional differences, anthropogenic processes, and potential conflicts among water users.
A possible modification of climate patterns could affect biodiversity and several crops, leading to a productive reconversion, especially sugar cane production. In regions that lack rainfall and experience rising temperatures, droughts may occur, leading to water evaporation (Gao et al., 2022; Sarker, 2022). Climate change scenarios identify future impacts of climate on local water resources. The IPCC (2024) presents reduced future climate projections from CMIP6 (Coupled Model Intercomparison Project), which, unlike CMIP5, integrates land use change and other socioeconomic data in addition to trajectories and forcing processes (O'Neill et al., 2016; WCRP, 2021). WorldClim processed 10 General Circulation Models (GCMs) for CMIP6: BCC-CSM2-MR, CNRM-CM6-1, CNRM-ESM2-1, CanESM5, GFDL-ESM4, IPSL-CM6A-LR, MIROC-ES2L, MIROC6, MRI-ESM2, and for four SSPs2: 126, 245, 370, and 585 (Kikstra et al., 2022). Additionally, the Water Evaluation and Planning (WEAP) model, developed by the Stockholm Environment Institute (SEI), is a worldwide reference tool for decision-making based on Integrated Water Resources Management (IWRM). It operates under the principle of hydrological balance (Díaz and López, 2017). Salinas Villafañe and Paz Rada (2011) explains that this model provides a comprehensive range of factors that influence the management of water resources, including anthropogenic processes. Giraldo (2014) elucidates that the WEAP approach investigates climate change impact on water distribution among diverse users, including the ecosystem.
According to NOM-011-CONAGUA-2015, in Mexico, the difference between the total sum of inflows (recharge) and the total sum of outflows (discharge) represents the volume of water lost or for aquifer storage over an established period (CONAGUA, 2020). The general balance equation, as per the law of conservation of mass, is as follows:
Applying this equation to the aquifer study, the inputs are represented by the total recharge, the outputs by the total discharge, and the change in mass by the water storage:
Different complex socioecological systems (Binder et al., 2013) were framed within the Social-Ecological System (SES), including the interactions between social users and resource governance, based on agreed rules and limited by the natural system, where the existing resources and their units determine the resource availability (Ostrom, 2019).
Assessing the regional risk of extreme events (droughts, forest fires, cyclones, tropical storms, tidal surges, landslides, typhoons, sea level rise, etc.) and projected changes in baseline climate parameters (terrestrial temperature, atmospheric precipitation, and specific humidity) do not exist whenever they identify potential patterns of changes (CAF, 2014). Climate models are “a numerical representation of the climate system that is based on physical, chemical, and biological properties, including its components, their interactions, and feedback processes, which explain some of its known properties.” Atmosphere–Ocean General Circulation Model (AOGCM) represents climate systems near the most complete end of the spectrum currently available, toward more complex models with interacting chemistry, social, and biological processes (Kikstra et al., 2022).
In the study region, climate change affects the biosphere and social systems with complex interactions (Calderón-Contreras and White, 2020), affecting multiple actors in economic and political activities (Sánchez-Santillán et al., 2015). Rathe (2017) suggests an interdisciplinary system methodology with complex, unpredictable, and nonlinear interrelated processes. This study examines a complex socio-ecological system analysis with interactions, dynamics, threats, and pressures at different levels (Collins et al., 2011). A hierarchical and multiscale approach promotes self-organization, which reduces vulnerability and increases resilience (Levin, 1988). The study also considers potential positive and negative impacts, including the global, national, and regional climate change impacts synthesized by the Intergovernmental Panel on Climate Change (Kikstra et al., 2022; IPCC, 2024).
The performance evaluation yielded the three best AOGCMs for the RSNM with estimated precipitation and temperature anomalies for the future climate, based on their effectiveness in modeling regional precipitation patterns. The performance of numerical variables is based on climate metrics and establishes the scope and limitations of the climate simulation in the study region by AOGCM scenarios. Cavazos et al. (2013) identified five validation climatic metrics to evaluate the performance of the AOGCM: the root mean square error (RMSE), mean absolute error (MAE), standard deviation (SD), and correlation coefficient (r). The sum of these variables gives an overall value, which should be close to 1 in order to get a good performance (see Supplementary Annexure 1).
Root mean square error (RMSE):
Where Dsim and D obs represent the simulated and observed data, respectively, and n is the number of observations at each grid point. The root mean square error frequently measures the difference between the values (sample or population values) predicted and observed in an estimated model. It magnifies prediction errors in a single measure by comparing different models for particular data sets.
Mean absolute error (MAE):
=
The Mean Absolute Error (MAE) measures the disparity between two continuous variables, taking into account two sets of data (some calculated, some observed) relating to the same phenomenon. It is used to quantify the accuracy of the prediction techniques by comparing predicted versus observed values.
Standard deviation (SD):
Where X and Y represent the value of a variable at a given time and X is the climatological average value.
Correlation coefficient:
Y represents the value of a variable at a given time, and X is the climatological average value from the WorldClim portal and analyzed for the eight AOGCM. GeoTiff files were processed by generating a raster cut that correspond to the study area. The correlation coefficient is a statistical measure that quantifies the strength and direction of a linear relationship between two variables. All of these statistical metrics underscore the choice of the selected AOGCM for the study area. The performance analysis selected from the eight AOGCM was the SSP 370 and 585 scenarios for the base climate (1970–2000) and the perspective (2081–2100) in RSNM. In addition, a formula was devised to attain the identical weighted average of these five standards (Table 2). The ranked analysis for precipitation and the eight AOGCMs evaluated for RSNM in Table 2 have values close to 1. The three AOGCMs used were CNRM-ESM2 (France), CNRM-CM6 (France), and MIROC6 (Japan) because they were the best evaluated for the region. The last column indicates the weighted average value of the five metrics applied to each AOGCM analyzed for precipitation (Supplementary Annexure 1 includes the calculations of the two performance analyses of the eight AOGCM).
This research analyzes SSP 3 (fragmentation) and SSP 5 (fossil fuel-based development), both dominant socioeconomic trends in Mexico and the study region. SSP3 with forcing level 70 represents substantial land use changes (especially global forest cover) and high greenhouse gas (GHG) emissions. It combines societal vulnerability (SSP3) with comparatively high forces. An SSP 5 with a high forcing level is the only SSP emission scenario for a radiative forcing of 8.5 Watts/m2 in 2100. The selection of models, scenarios, and horizons for this analysis visualized scenarios reflecting a fossil fuel-driven development with low adaptation and a high level of GHG emissions, without any additional efforts to limit CO2-equivalent emissions exceeding 1,000 ppm by the year 2100. This radiative forcing may increase the global surface temperature from 2.6°C to 4.8°C (Kikstra et al., 2022). These two SSPs were chosen to visualize the most adverse scenarios linked to the current national policy (O'Neill et al., 2016).
The precipitation anomalies scenarios analyzed using the WEAP model and WorldClim Coupled Model Intercomparison Project 6 (CMIP6) databases incorporate greenhouse gas emissions scenarios (SSP) to establish the performance analysis. The WorldClim version 2.1 (WorldClim, 2024) and the reduced climate projections from CMIP6 at a spatial resolution (expressed in min of a degree of longitude and latitude) of 2.5 min could result in some errors in capturing local variability, especially in the rocky study region. CONAGUA conducted the meteorological stations 17047 (Huitzilac), 17004 (Cuernavaca-DGE), and 17014 (Temixco) as the most representative for the upper, middle, and lower parts of the RSNM.
Figure 2 shows the methodology outline. The precipitation anomalies were obtained by subtracting the historical climate (1970–2000) from the data projected in the scenarios. The climate change scenarios provide the precipitation anomalies that feed the WEAP model. These climate change scenarios simulate precipitation anomalies, which feed the WEAP model with a Geographic Information System based on a graphical interface for water resources planning and hydrological balances for participatory planning. It incorporates an IWRM approach and generates impact models for climate change scenario anomalies. By linking supply and demand, WEAP integrates hydrological processes at the basin scale and allows regional water balances. Scenarios encompass demand, supply, precipitation, elevation, hydraulic sections, runoff, vegetation cover, infiltration, evapotranspiration, interaction between surface and groundwater, water quality, and projections of climate change scenarios with socioeconomic aspects (Sánchez Torres et al., 2006). Hydrologic balance is based on the law of conservation of mass, in which the total input of water (referring to precipitation and inflows) must be equal to the total output (evapotranspiration, withdrawals, and runoff) plus the net variation in accumulated storage, territory data (type of soils, land use, data from hydrometric stations, kc, ecological flow, etc.), and socioeconomic conditions (infrastructure, population growth, monthly variations of waste, users, reuse, demand, and deforestation) (Arreguín-Cortés et al., 2015).The WEAP model for the RSNM was developed by a GIS map defining the main watercourses, demand sites, water flows, wastewater treatment plants, ecological flow, the aquifer, and the general study area dynamics. Information was complemented by maps, documents, governmental studies, and scientific articles (Table 3 and Supplementary Annexure 2).
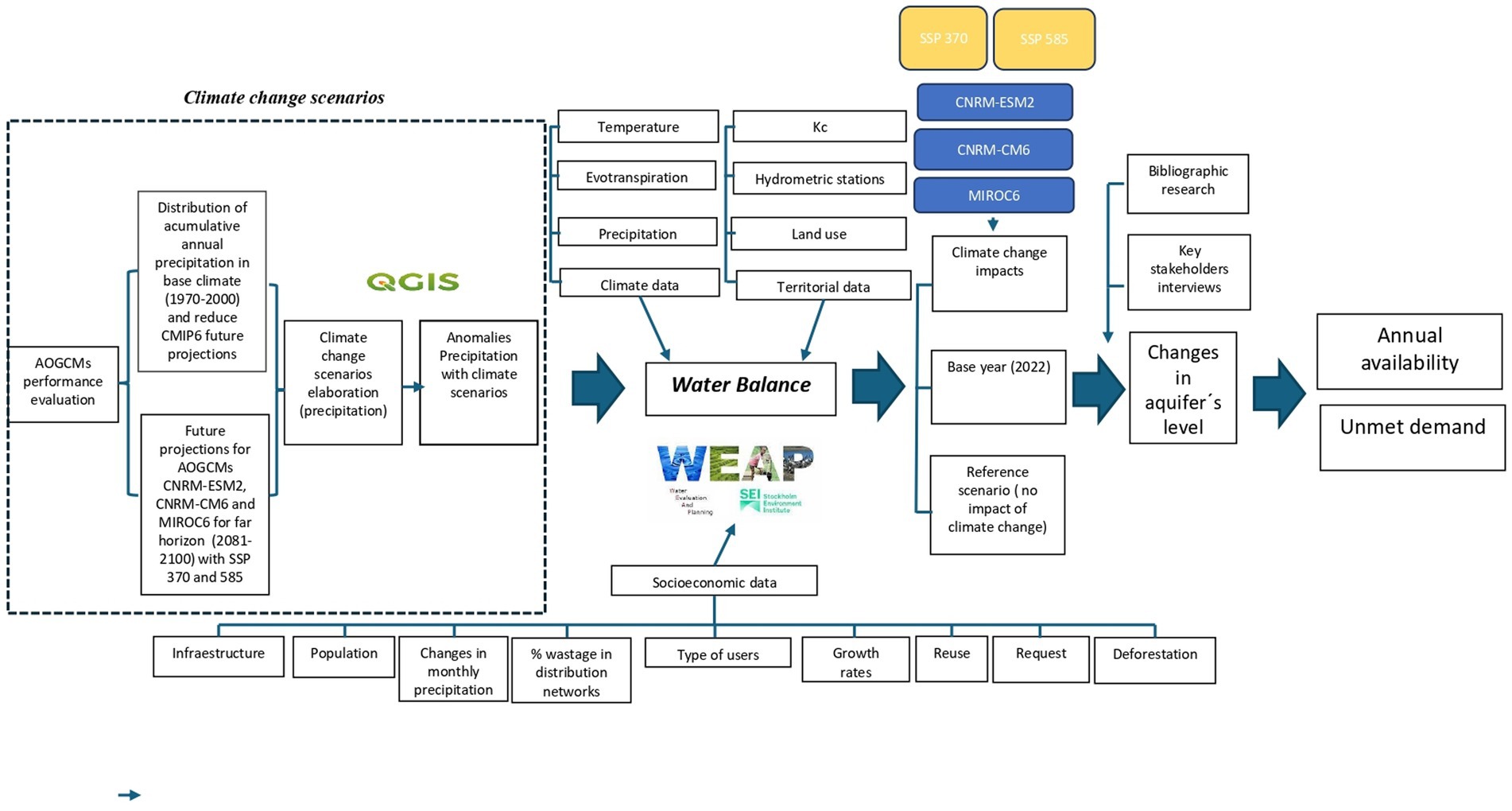
Figure 2. Modeling water availability (supply/demand) under a climate change scenario scheme. A systemic approach in the RSNM (own elaboration).
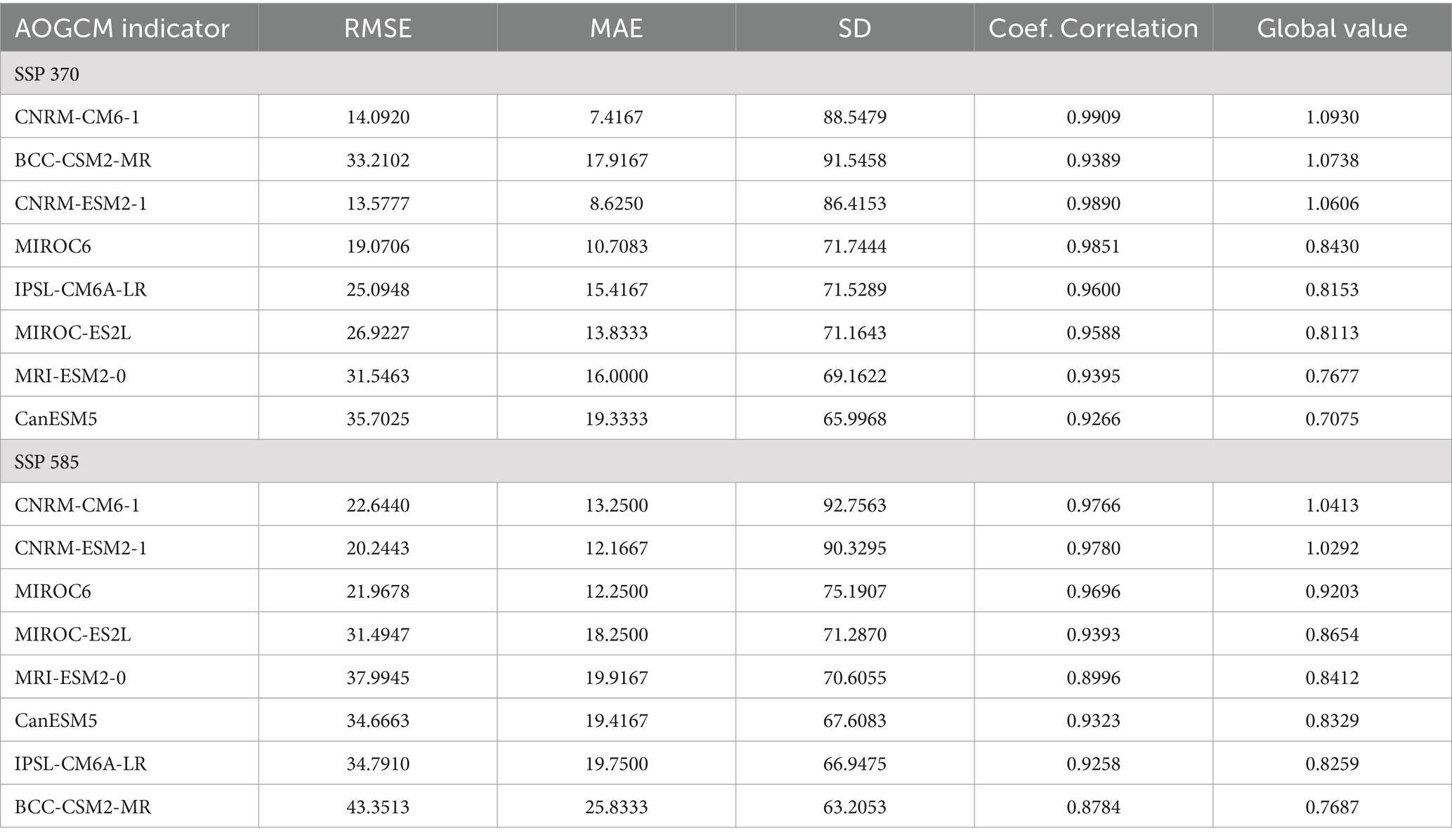
Table 3. Performance evaluation of eight AOGCM for SSP 370 and SSP 570 for precipitation in 2081–2100 (own elaboration).
The study examined water use demands for the base year, population, water consumption, water reuse, monthly variations in consumption, losses in the distribution system, and other data were included. The RSNM water system was mapped for the components of the water table depth, hydrological parameters, land use, topographic and geomorphic features, crop data, soil properties, metrology/climatology, irrigation, yields, water demand, demographic details, water transmission and availability of both surface and groundwater, abstraction nodes, supplies, outflows, demand sites, infiltration/runoff links, together with other data from the WEAP model for the study area (see Supplementary Annexure 2).
The perimeter is the boundary of the Cuernavaca aquifer, considering the natural boundaries and its hydrological position. The total outflow comprises an update based on the groundwater REPDA (Registro Público de Derechos de Agua-CONAGUA) of pumping extractions (REPDA-CONAGUA, 2019). Horizontal outflows consist of discharges from the aquifer to a surface stream, which contribute to ecosystem conservation. Flows calculated rainfall and runoff for the Soil Conservation Service (SCS), developed by the U.S. government agency (Sánchez Torres, 2016) (see Supplementary Annexure 3). The available water quality data (BOD5, COD, and TSS) were recorded in the return flows of the demand centers, wastewater treatment plants, and rivers. The main wastewater treatment plants for the study area were included with their percentage of removal of pollutants and efficiency. The data corresponding to the Cuernavaca aquifer was also verified for the storage capacity, recharge, interaction with other aquifers, ecological flows, and infiltration zones for the scenarios from 2023 to 2100.
The WEAP model structure consisted of the development of (1) the base year (2022), (2) the reference scenario with no climate change impact for the period 2023–2100, (3) the SSP 370 climate change scenarios for the three AOGCMs chosen in the performance analysis for the period 2081–2100, and (4) the SSP 585 climate change scenarios for the three chosen AOGCMs in the performance analysis for 2081–2100. This WEAP model calculates the water balance for each node by linking distinct time scenarios. A node represents a physical component, such as a demand site, treatment plant, aquifer, or location along the Apatlaco River (the main tributary in the RSNM). The nodes were linked by lines representing natural or human-made processes, such as rivers, diversions, and return flows. All water entering the system at any given time is stored in the soil, aquifer, river, tributary, reservoir, or exits the system (Supplementary Annexure 4). Finally, the constructed WEAP model was calibrated by calculating the values of the indicators Nash-Sutcliuffe Efficiency NSE, Percent Bias, PBIAS, Root Mean Squared Error to the Standard Deviation, RSR, and Ratio Simulated vs. Observed Flow Standard Deviation, SDR, utilizing the simulated and real flow values for the Apatlaco River, provided by the hydrometric station B18271 Temixco of CONAGUA (Sánchez Torres, 2016) (Supplementary Annexure 5). Thirty interviews with key actors in the study area provided qualitative data for the interpretation of climate change impacts for the study area, mentioning existing conflicts and trends of the ongoing anthropogenic processes.
3 Results
The three meteorological stations (17047-Huitzilac, 17004-Cuernavaca DGE, and 17014-Temixco) operated by CONAGUA for the metropolitan area indicate the most significant rainfall during June and September (Figure 3) in 2022. In 2024, the delay in the monsoon was until July (CONAGUA, 2024).
Figure 4 shows the distribution of cumulative annual precipitation and its anomalies in the climate base 2022 and the total precipitation projections for the three selected AOGCM in 2081–2100 by SSP 370 (fragmentation). These scenarios indicate a significant decrease in precipitation, especially for AOGCM MIROC6, with a loss of up to 46.5 mm during August. There are also summers with less rainfall, atypical rainfall, and severe drought periods. The monsoon season commences later in all of the simulation models. Traditionally, in August, there is an interstival drought, which is necessary for the development of the corncob, the basic food in the region (Ibarra Sánchez et al., 2020). The lack of water and delay in the monsoon affect the entire crop cycle (Estrada et al., 2023), threatening especially the poor farmers in rainfed agriculture, forcing them to migrate (Oswald, 2023).
Figure 5 shows the cumulative annual precipitation, its anomalies, and the AOGCMs for 2081–2100 and SSP 585 (fossil fuel-based development) precipitation projections. The AOGCM model for SSP 585 predicts a significant decrease in precipitation from June to September, atypical rainfall in June, and prolonged periods of drought with higher temperatures. Similar data was observed in the case of SSP 370. MIROC6 indicates the highest decrease of 59 mm in July, specifying a shift of the rainy months toward September and October. MIROC6 is composed of three sub-models: atmosphere, land, and sea ice-ocean. It has improved accuracy in simulating natural climate variability and a better response to climate change representation of ocean–atmosphere interaction. It has also improved fidelity in simulating extreme events in extreme pathways for catastrophic scenarios (Tatebe et al., 2019).
Figure 6 shows the precipitation anomalies for scenarios with SSP 370 and 585 in the study area. The SSP 370 model projects higher anomalies, whereas the AOGCM MIROC6 indicates the highest decrease in precipitation related to massive fossil use. Both SSP projects simulate a displacement and a later start to the monsoon season with atypical rainfall up to October. The transition from fossil energy to renewable energy and water forest recovery could improve water availability and improve regional climate conditions with lower temperatures. SSP 585 (intensive hydrocarbon use) simulates a longer drought period with less rainfall, whereas SSP 370 indicates greater rainfall.
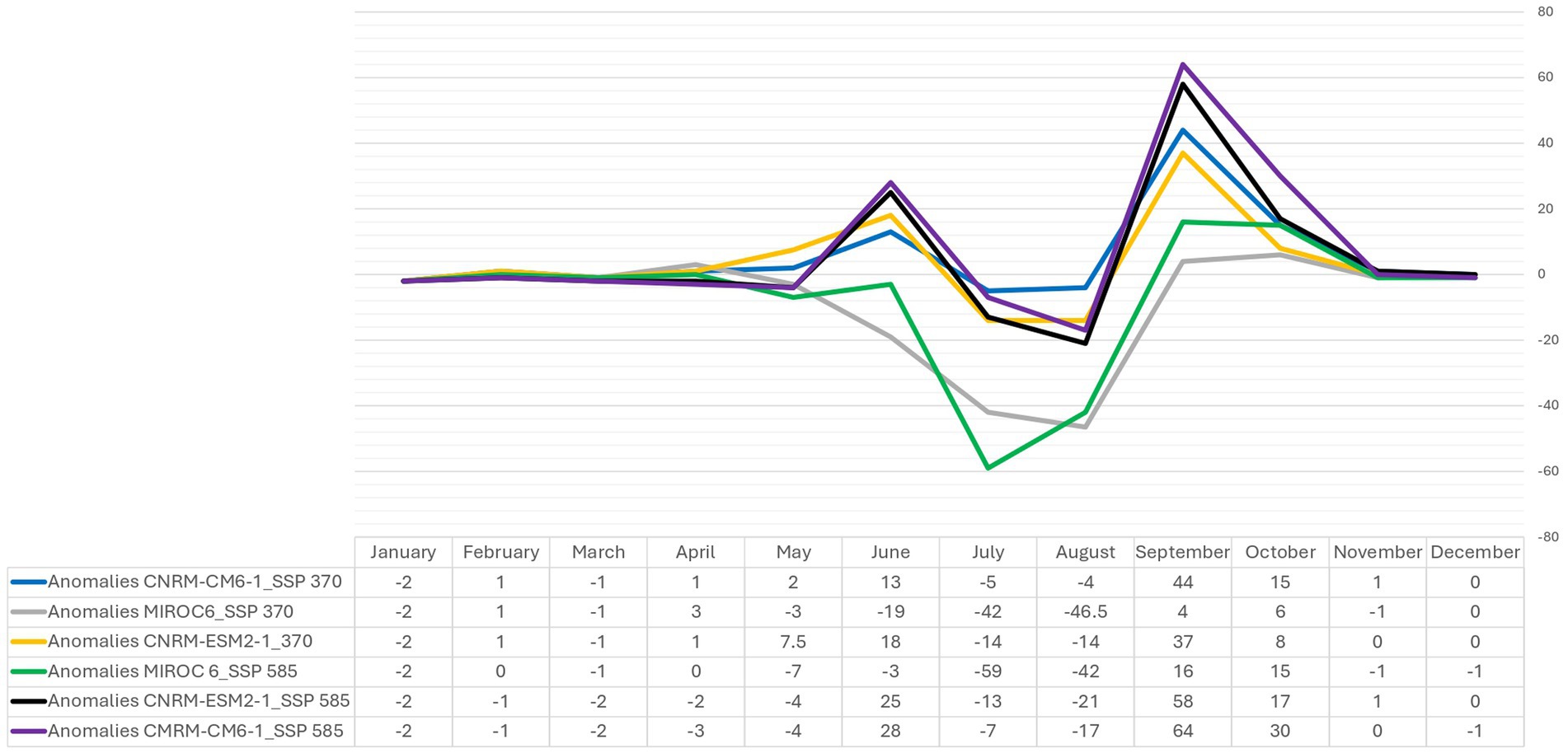
Figure 6. Precipitation anomalies comparative (mm) for RSNM-SSP 370 and SSP 585 between 2081 to 2100 (own elaboration).
The reference data on recharge and extraction published by CONAGUA, 2020 identifies a total annual recharge of 344 Mm3 for the Cuernavaca aquifer (CONAGUA, 2020). The highest recharge occurs during the rainy months (June–September). The reference scenario (2023–2100) and the comparison of natural recharge indicate a tendency to decrease in most of the models, from 344 Mm3 (reference scenario) to 323 Mm3 for SSP 370 and AOGCM MIROC6 (Figure 7).
The estimated water demand of 197 Mm3 in the study area is mainly extracted from the aquifer, where the cities of Cuernavaca and Jiutepec have the highest water requirement (Figure 8). The irrigation module Las Fuentes derives the majority of its water from the aquifer, in contrast to the Alto Apatlaco irrigation module, which relies on surface water. Furthermore, there are water leaks in the distribution networks. In the base year (2022), the aquifer supply requirement for the City of Cuernavaca was estimated at 120 Mm3, and it is projected to exceed 200 Mm3 by 2100. The Las Fuentes irrigation module also has a significant aquifer requirement of more than 60 Mm3 compared to the Alto Apatlaco irrigation module, predominantly supplied by surface water.
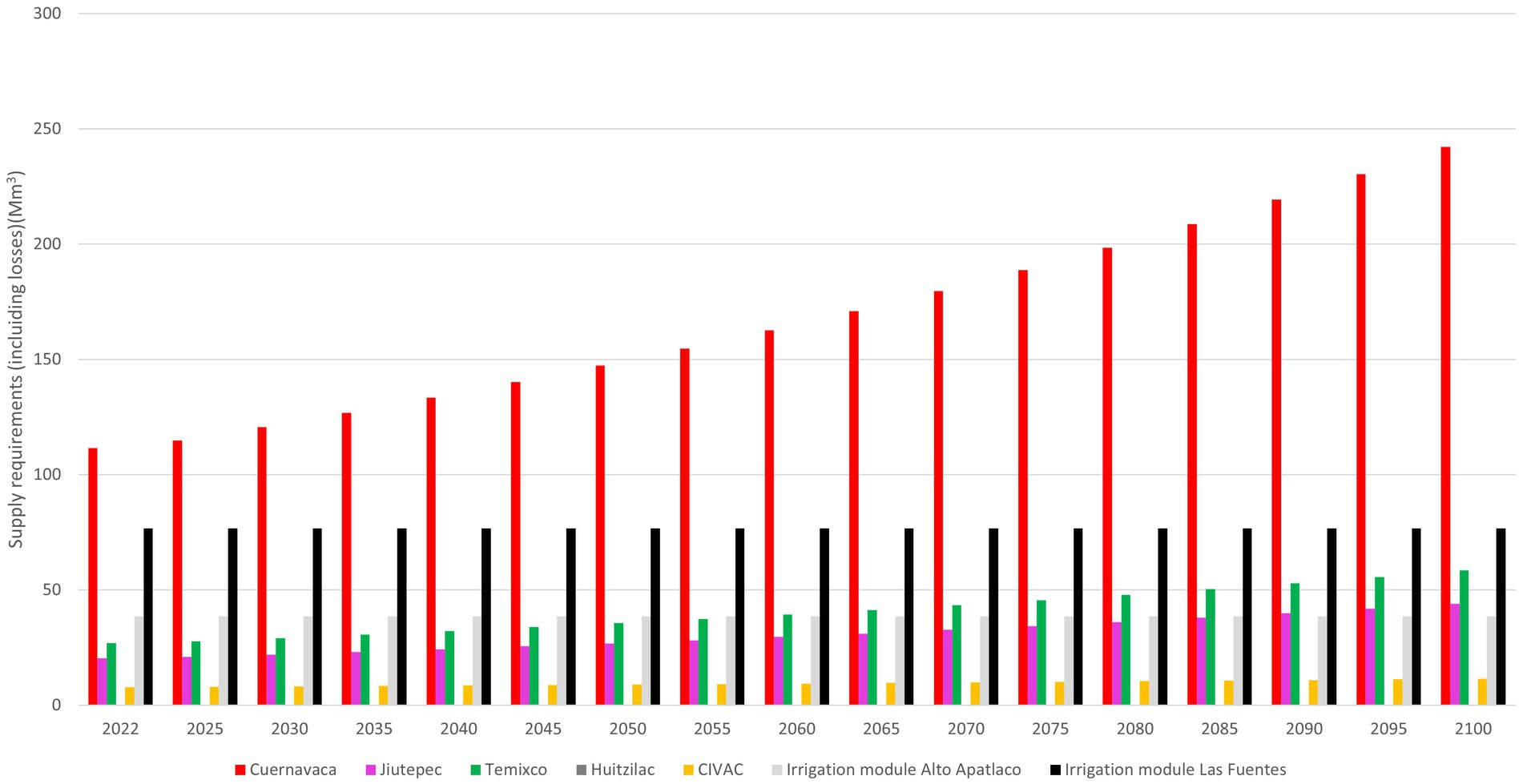
Figure 8. Comparative supply requirements (including losses) (own elaboration). Reference scenario (2023–2100) and scenarios impacted by climate change.
Figure 9 shows a tendency to increase supply requirements under change scenarios conditions to more than 500 Mm3 in 2090 in the study region.
Figure 10 shows the hydrological balance and annual aquifer availability with an approximate yearly average availability of 20 Mm3 for 2022 with a decreasing equilibrium from 2032. In 2024, the population did multiple protest marches of lack of water because the official data of piezometry date at least two decades ago.
Figure 11 shows SSPs and AOGCM indicating a significant decrease in the average annual availability of the aquifer of 20 Mm3 for 2022 and a decreasing tendency for almost all scenarios. The worst scenario was MIROC6, with SSP 585 missing 1,500 Mm3 in 2099.
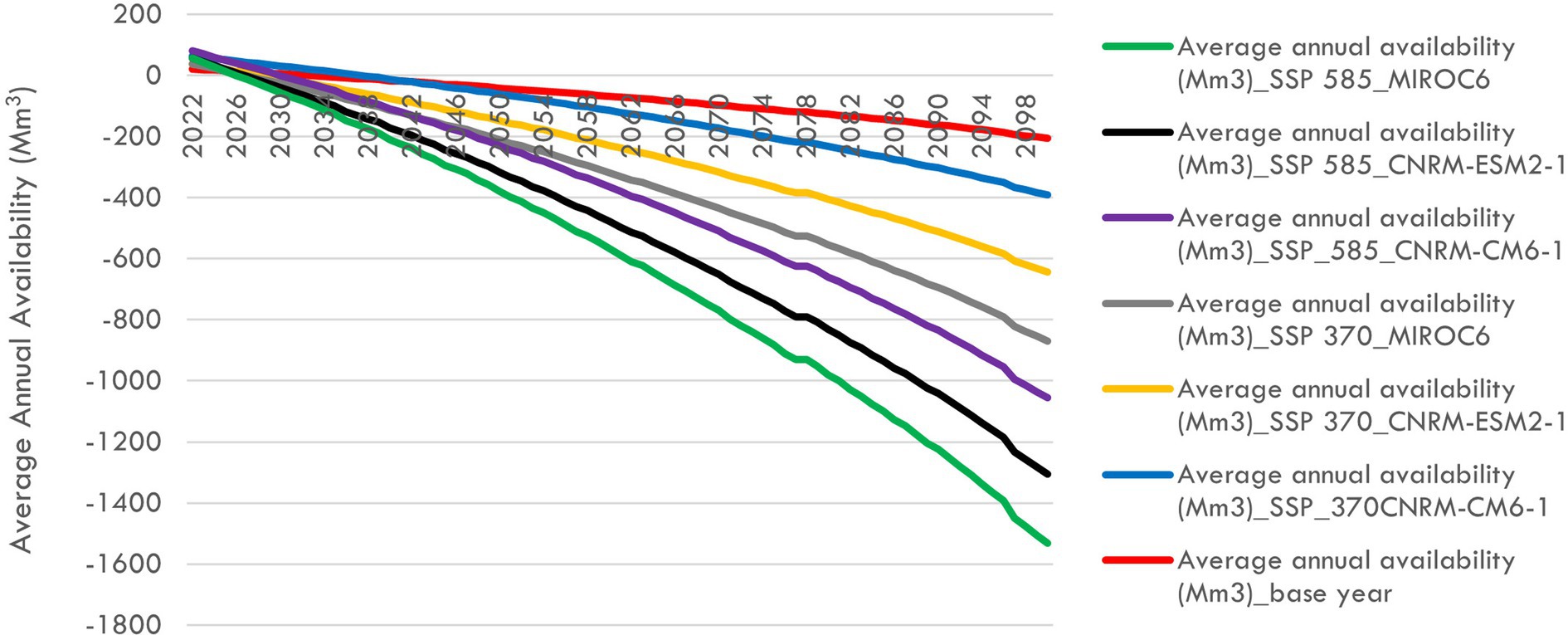
Figure 11. Comparison of average annual availability in impacted scenarios and reference scenarios (own elaboration).
Figure 12 shows the unmet demand resulting from the WEAP model for the study area. A similar shortage is observed in the first place for the irrigation module. Las Fuentes is the most vulnerable, as it depends on the recharge of springs. Similarly, the industrial city of CIVAC is committed from 2045 and the City of Cuernavaca by 2070.
4 Discussion
Observing the distribution of accumulated annual precipitation in 2022 and the accumulated precipitation projections in the three AOGCM obtained for both SSPs indicates that the regional water demand is increasing as a result of population growth, economic activities, food demands, higher temperatures, less rainfall, delays in the monsoon, and extreme weather events. In 2022, the region demanded about 197 Mm3, mainly extracted from the metropolitan aquifer. The irrigation module in Las Fuentes experiences the highest demand from the regional aquifer and is the first affected by a deficiency in groundwater, to produce ornamental plants and party gardens. The cities of Cuernavaca, Jiutepec, and the entire metropolitan area are threatened to run out of drinking water supplies without an alternative management of the water forest and an energy transition. The irrigation module of the Alto Apatlaco is mainly supplied with surface water from the mountain. Therefore, a collective effort of peasants and local and state authorities is required to protect the natural protected area, conduct systematic reforestation with local plants, combat bushfires, and establish a technical irrigation system to provide subsistence food from corn, beans, and vegetables.
According to the WEAP model, the demand for water increases during the hot months of March to May, later June or July with higher temperatures, evapotranspiration, and less rainfall. Furthermore, the regional water supply in the metropolitan area is over 40 years old, has been broken by earthquakes, and nearly half of the water has been lost in the distribution networks. A projected increase in water demand of 242 Mm3 for Cuernavaca, coupled with a high population growth rate, is projected by the year 2100. The metropolitan area of Cuernavaca may expand to the Yautepec-Cuautla metropolis and the different aquifer, indicating a significant population growth from 378, 476 inhabitants in 2020 to 445, 848 inhabitants in 2032 (INEGI, 2020; Gobierno de Morelos-Hacienda, 2023). An adverse climate change scenario may increase the monthly demand by more than 20 Mm3 during the dry season and later monsoon.
In the historical climate scenario, the monsoon commenced from June to September with about 980 mm of annual rainfall. All of the AOGCMs indicate a significant decrease in precipitation (less severe with SSP 370), atypical rain, and longer periods of drought in SSP 585 (referring to conventional fossil fuel-based development with major mitigation challenges). MIROC6 simulates a severe drop of −59 mm for July in 2098, while the monsoon moves to September and October with much higher temperatures during the dry months. CNRM-CM6_SSP 585 also illustrates a decrease in rainfall with anomalies of −17 mm in August, 64 mm in September, and 30 mm in October in the long-term scenario. The largest lack of precipitation occurred in MIROC6 SSP 370 (assuming high population growth and low economic development with low levels of education in the local society) with anomalies of up to −59 mm in July. Both of these SSP models assume less rainfall, atypical rainfall, extreme events, and longer severe drought periods. The WEAP model for the study region demonstrates a negative trend in the metropolitan aquifer’s natural recharge, with significantly higher demand and unmet demands between −200 and – 1,500 Mm3.
These climate impacts also impact the historical flow of the Apatlaco River and the existing springs in the mountain, and the water quality in terms of BOD5, COD, and TSS deteriorate with lack of water and higher temperatures, worse during the dry season. The WEAP model coincides with official sources of the hydrological balance with an annual availability of the aquifer of approximately 20 Mm3 per year in 2022 (CONAGUA, 2020). In 2020, the same source published data for recharge and extraction with an annual natural recharge of 344 Mm3 in the metropolitan aquifer, where the rainy season contributed from June to September. The AOGCM, MIROC 6_ SSP 370, observes the greatest tendency to decrease the natural aquifer recharge, with a decrease from the reference year of 344 Mm3 to 323 Mm3 in MIROC6_SSP 370. The hydrological balance also expresses the unmet demand for the irrigation module Las Fuentes in 2024, as well as the city of Cuernavaca, which is highly affected by climate change scenarios. Water shortages occurred in 2022 between April and May in Cuernavaca and during the same months in 2024. By 2061, significant water shortages are projected for Cuernavaca, reaching approximately 18 Mm3 by 2086.
A trend toward overexploitation of the Cuernavaca aquifer exists today with a discrepancy of official data. If current extraction rates and trends continue, it would not be feasible to satisfy the demands to satisfy the needs of the region with the unmet demand in the WEAP model for the study area. The modification of climate patterns affects several crops, leading to a productive reconversion from corn, sugar cane, and sorghum, reducing the local subsistence food. The most vulnerable areas are the southern and southeastern regions of Morelos. These trends confirm the climate change models developed by Estrada et al. (2023), which predict that climate change has altered and delayed the distribution of precipitation during the annual rainy seasons.
Morelos in the Face of Climate Change, Ortíz et al. (2013) analyze the climate series of the state with a trend toward an increasing air temperature, more hot days (Tx >35°C), and maximum temperatures above the 90th percentile. This increase is noticeable from 1990 on in all climate zones of the state, which is highly evident in the semi-hot and hot zones in the center and south of Morelos, where the temperature increased in May 2024 up to 47°C in Tlaquiltenango, Morelos (CONAGUA, 2024). Regarding precipitation, Bolongaro-Crevenna et al. (2013) demonstrate the variability of rainfall regimes in Morelos with a delay at the beginning of the monsoon. These authors analyze the water availability in the Cuernavaca aquifer with the recorded trends of groundwater extraction, producing overexploitation of the aquifer in 4.7 years starting in 2011. They use a Spatial Distributed Analysis (SDA) to perform a pixel-by-pixel water balance model, considering temperature, precipitation, EVT, vegetation, land use, edaphology, and geology. Precipitation reached 4,781 Mm3; 64% was lost by EVT, 24% generated virgin runoff, and 12% recharged the aquifer. This analysis reported a deficit in water availability in the Cuernavaca aquifer of −133.34 Mm3 since 2013. Thus, studies on groundwater dynamics are necessary, such as the simulations with SWAT (Soil and Water Assessment Tool) and MODFLOW (distributed hydrogeological model) software (Arnold et al., 1993) or piezometric or isotopic studies (Bendig, 1985).
Specific risks for the water supply in Morelos are related to the changes of 73% in biodiversity and 97% in forest cover, agriculture, and livestock, according to the Study of Vulnerability and Adaptation to the Effects of Climate Change prepared by the National Autonomous University of Mexico in its Climate Atlas (UNAM, 2022). Despite this knowledge being public, the actions of the state and municipal governments are limited and poorly articulated. The existing bias in official water data represents interests and power relations with public information manipulation, where bottled water suppliers, irrigated agriculture, real estate, ornamental fisheries, industries, and growing cities are pressuring for more water. At the state level, alternative sources and greater efficiency in the use and reuse of treated water should complete the missing and disperse water supply data by the irrigation module Las Fuentes, the industrial zone CIVAC, and the metropolitan area of Cuernavaca and Jiutepec. Greater adaptation to growing water shortage implies searching for efficiency in agricultural and industrial activities, policies to recover springs, aquifer recharge with treated water, conservation of natural areas, capture of rainfall water, energy transition technologies, and reforestation of the water forest. Sufficient water in quantity and quality allows economic development in the region, grants people living standards, and the SDG 6 to water and safe sanitation.
Processing hydrological data and forecasting climate change obliges a system approach to understanding the multiple interrelated factors affecting water supply, use, and pollution. Methodologically, evaluating water availability in the region with climate change scenarios should promote better data collection. Further, multiple extreme events oblige the development at the municipal level of the Atlas of Risk and train vulnerable people with increasing adaptation and resilience to upcoming extreme events. The Water Forest in the RSNM represents great potential to supply the water necessary for the development of Morelos. Its conservation in the upper watershed represents the hydric factory (Yu et al., 2022) for the whole region (Jaramillo et al., 2018). The surrounding mountains of the metropolitan area with greater precipitation and high permeability of basaltic rocks can recharge the aquifer. The semi-dry conditions of Morelos, changes in the monsoon, higher temperature, extreme climatic events, and less water availability represent similar conditions in multiple countries of the Global South and the reflections can serve as a case study for these regions.
Data availability statement
The datasets presented in this study can be found in online repositories. The names of the repository/repositories and accession number(s) can be found in the article/Supplementary material.
Author contributions
JG-Z: Writing – original draft, Writing – review & editing. ÚO-S: Conceptualization, Methodology, Supervision, Validation, Writing – review & editing.
Funding
The author(s) declare that no financial support was received for the research, authorship, and/or publication of this article.
Acknowledgments
The authors would like to thank Anita Breuer, PhD, from the German Institute of Development and Sustainability, Gerardo Sánchez Torres Esqueda, PhD, from Faculty of Engineering of Autonomous University of Tamaulipas and Leticia Merino Pérez, PhD, from the Social Research Institute from Autonomous University of Mexico for their valuable reviews and inputs. The first author gratefully acknowledges the Program in Sustainability Sciences, UNAM (Posgrado en Ciencias de la Sostenibilidad, Universidad Nacional Autónoma de México) and the Consejo Nacional de Ciencia y Tecnologia (CONACYT) for the scholarship (No. 226325).
Conflict of interest
The authors declare that the research was conducted in the absence of any commercial or financial relationships that could be construed as a potential conflict of interest.
Publisher’s note
All claims expressed in this article are solely those of the authors and do not necessarily represent those of their affiliated organizations, or those of the publisher, the editors and the reviewers. Any product that may be evaluated in this article, or claim that may be made by its manufacturer, is not guaranteed or endorsed by the publisher.
Supplementary material
The Supplementary material for this article can be found online at: https://www.frontiersin.org/articles/10.3389/frwa.2024.1466380/full#supplementary-material
Footnotes
1. ^The present research indicates a tendency toward an equilibrium with slight aquifer overexploitation. The official data indicate availability of 33 Mm3 (DOF, 2003); on August 28, 2009, 21.31 Mm3; in 2013, 7.65 Mm3. This discrepancy does not reflect the real availability of water in the Cuernavaca aquifer; reality could be worse than expected. No available data on aquifer extraction exists.
2. ^The SSP (Shared Socioeconomic Pathways) are scenarios of global socioeconomic change projected to 2100. They define different reference policies that occur with concerted international efforts addressing climate change beyond the already adopted measures by countries committed to the Paris Agreement. They also include multiple reference models related to underlying factors, such as population, technological, and economic growth, which could lead to highly different future emissions and temperature rises, even without any climate mitigation policy (Hausfather and Drake, 2018).
References
Arnold, J. G., Allen, P. M., and Bernhardt, G. (1993). A comprehensive surface-groundwater flow model. J. Hydrol. 142, 47–69. doi: 10.1016/0022-1694(93)90004-S
Arreguín-Cortés, F., Rodríguez, O., and Montero, M. J. (2015). Atlas de vulnerabilidad hídrica en México ante el cambio climático. Morelos, México: Instituto Mexicano de Tecnología del Agua (IMTA).
Bendig, G. (1985). “Susceptibilidad Regional de la Contaminación y Estrategias de su Protección en el Valle de Cuernavaca,” en eds. U. Oswald, El recurso agua en el Alto Balsas, CRIM-UNAM / El Colegio de Tlaxcala / Instituto de Geofísica-UNAM / Fundación Heinrich Böll / Coordinación General de Ecología de Tlaxcala, México, 2003.
Binder, H., Bots, P. W. G., and Pahl -Wostl, C. (2013). Comparison of frameworks for analyzing social-ecological. Syst. Ecol. Soc. 18:28. doi: 10.5751/ES-05551-180426
Bolongaro-Crevenna, R. A., Torres, R. V., Pohle, O., Chavarría, H. J., and García, V. F. (2013). “Escenarios de cambio climático en el Estado de Morelos” in Cambio climático: Vulnerabilidad de sectores clave en el Estado de Morelos. eds. M. L. Ortiz-Hernández and E. Sánchez-Salinas (Cuernavaca, Morelos, México: Universidad Autónoma del Estado de Morelos).
CAF (2014). Indice de vulnerabilidad y adaptación al cambio climático en la región de América Latina y el Caribe. Caracas: CAF- Banco de desarrollo de América Latina. Available at: https://scioteca.caf.com/handle/123456789/517
Calderón-Contreras, R., and White, C. S. (2020). Access as the means for understanding social-ecological resilience: bridging analytical frameworks. Sociedad y recursos naturales 33, 205–223. doi: 10.1080/08941920.2019.1597233
Cavazos, T., Salinas, J. A., Martínez, B., Colorado, G., de Grau, P., Prieto-González, P., et al. (2013). Actualización de escenarios de cambio climático para México como parte de los productos de la Quinta Comunicación Nacional. INECC. Instituto Nacional de Ecología y Cambio Climático (INECC). 151 pp.
Collins, S. L., Carpenter, S. R., Swinton, S. M., Orenstein, D. E., Childers, D. L., Gragson, T. L., et al. (2011). An integrated conceptual framework for long-term social-ecological research. Front. Ecol. 9, 351–357. doi: 10.1890/100068
CONAGUA (2020). Actualización de la disponibilidad media anual de agua en el acuífero Cuernavaca (1701). Estado de: Morelos.
CONAGUA (2021). Sistema Nacional de Información del Agua. Available at: http://sina.conagua.gob.mx/sina/index.php?p=7 (Accessed March, 2021).
CONAGUA (2023). Monitor de Sequía de México. Available at: https://smn.conagua.gob.mx/tools/DATA/Climatolog%C3%ADa/Sequ%C3%ADa/Monitor%20de%20sequ%C3%ADa%20en%20M%C3%A9xico/Seguimiento%20de%20Sequ%C3%ADa/MSM20240531.pdf. (Accessed May 31, 2024).
CONAGUA (2024). Sistema Meteorologico Nacional. Available at: smn.conagua.gob.mx (Accessed June, 2024).
Delgado Ramos, G. C. (2019). Asentamientos urbanos sustentables y resilientes: retos y oportunidades para la transformación urbana en California y Baja California. Centro de Investigaciones Interdisciplinarias en Ciencias y Humanidades, UNAM. https://ru.ceiich.unam.mx/handle/123456789/3806
Díaz, H., and López, T. (2017). Calibración del modelo WEAP para la planificación hidrológica de la cuenca San Diego, Tesis de diploma presentada en opción al título de Ingeniería Hidráulica. Facultad de Ingeniería Civil: Centro de Investigaciones Hidráulicas, Universidad Tecnológica de La Habana, Cuba.
DOF (2003). ACUERDO por el que se dan a conocer los límites de 188 acuíferos de los Estados Unidos Mexicanos, los resultados de los estudios realizados para determinar su disponibilidad media anual de agua y sus planos de localización. Diario Oficial de la Federación, 31 de enero de 2003. Available at: https://dof.gob.mx/nota_detalle.php?codigo=706659&fecha=31/01/2003#gsc.tab=0
Estrada, F., Zavala, J., Martínez, A., Raga, G., and Gay, C. (2023). Estado y perspectivas del cambio climático en México: un punto de partida. Programa de Investigación en Cambio Climático. (PINCC)-UNAM Available at: https://www.pincc.unam.mx
Gao, Y., Sarker, S., Sarker, T., and Leta, O. T. (2022). Analyzing the critical locations in response of constructed and planned dams on the Mekong River basin for environmental integrity. Environmental Research Communications 4, 4–16. doi: 10.1088/2515-7620/ac9459
Giraldo, N. (2014). Implementación del sistema de modelación WEAP como herramienta para la gestión integral del recurso hídrico en la vereda la bella. Tesis de maestría, Universidad Nacional de Colombia.
Gobierno de Morelos-Hacienda (2023). Síntesis Estadística Municipal 2021. Cuernavaca: Gobierno del Estado de Morelos. http://marcojuridico.morelos.gob.mx/archivos/decretos_ejecutivo/pdf/DPEACCMOR.pdf
Gobierno del Estado de Morelos (2014). Decreto por el que se expide el Programa Estatal de Acción ate el Cambio Climático de Morelos “PEACCMOR” : Consejeria Juridica del Poder Ejecutivo del Estado de Morelos.
Hausfather, Z., and Drake, H. (2018). Evaluating the performance of past climate model projections. Wiley 47, 2–10. doi: 10.1029/2019GL085378
Healing Waters International (2023). The Current Water Crisis in Mexico. Available at: https://healingwaters.org/the-current-water-crisis-in-mexico/
Höllermann, B., Giertz, S., and Diekkrüger, B. (2009). Benin 2025- balancing future water availability and demand using the WEAP ‘water evaluation and planning’ system. Water Resour. Manag. 24, 2999–3012. doi: 10.1007/s11269-010-9622-z
Ibarra Sánchez, E., Castillo Gutiérrez, A., Núñez Valdéz, M. E., Suárez Rogríguez, R., Andrade Rogríguez, M., and Perdino Roldrán, F. (2020). Caracterización de la respuesta a la sequía de líneas segregantes de maíz. Revista mexicana de ciencias agrícolas 11, 1511–1524. doi: 10.29312/remexca.v11i7.2196
IMTA (2011). Impacto del Cambio Climático en la Calidad del Agua en México. TC-0872.4. Informe final. Coordinación de tratamiento y calidad del agua. Instituto Mexicano de Tecnología del Agua. Available at: http://hdl.handle.net/20.500.12013/1427
INECC (2021). México, entre los países más vulnerables ante el cambio climático. Available at: https://www.gob.mx/inecc/prensa/mexico-entre-los-paises-mas-vulnerables-ante-cambio-climatico?idiom=es (Accessed, March, 2021).
INECC-SEMARNAT (2018). Inventario Nacional de Emisiones de Gases y Compuestos de Efecto Invernadero 1990–2015. Instituto Nacional de Ecología y Cambio Climático. Available at: http://cambioclimatico.gob.mx:8080/xmlui/handle/publicaciones/226
INEGI (2020). Censo INEGI 2020. Available at: https://www.inegi.org.mx/ (Accessed March, 2020).
INEGI (2021). Cuéntame, información por entidad. Instituto Nacional de Geografía y Estadísticas Available at: http://www.cuentame.inegi.org.mx/monografias/informacion/mor/territorio/clima.aspx?tema=me&e=17 (Accessed March, 2021).
IPCC (2014). Conclusiones de nivel superior del resumen para responsables de políticas de la construcción del grupo de trabajo II al Quinto Informe de Evaluación. Intergovernmental Panel on Climate Change (IPCC). Available at: https://www.ipcc.ch/
IPCC (2024). The Intergovernmental Panel on Climate Change. Available at: https://www.ipcc.ch/ (Accessed July, 2024).
Jaramillo, F., González, J., and Flores, V. H. (2018). Plan para el Manejo Integral del Sistema de Barrancas del Norponiente de Morelos. Secretaría de Medio Ambiente y Recursos Naturales (SEMARNAT).
Kara, T., and Sahin, A. D. (2023). Implications of climate change on wind energy potential. Sustain. For. 15:14822. doi: 10.3390/su152014822
Kikstra, J. S., Nicholls, Z. R., Smith, C. J., Lewis, J., Lamboll, R. D., and Byers, E. (2022). The IPCC sixth assessment report WGIII climate assessment of mitigation pathways: from emissions to global temperatures. Geosci. Model Dev. 15, 9075–9109. doi: 10.5194/gmd-15-9075-2022
Levin, S. A. (1988). Ecosystems and the biosphere as complex adaptative systems. Ecosistems 1, 431–436. doi: 10.1007/s100219900037
Martínez-Austria, P., and Patiño-Gómez, C. (2012). Efectos del cambio climático en la disponibilidad de agua en México. Tecnología y Ciencias del Agua. Instituto Mexicano de Tecnología del Agua III, 5–20.
Montero-Martínez, M., Martínez-Jiménez, J., Castillo-Pérez, N., and Espinoza-Tamarindo, B. (2010). Escenarios climáticos en México proyectados para el siglo XXI: precipitación y temperaturas máxima y mínima. En Atlas de Vulnerabilidad Hídrica en México ante el Cambio Climático. Instituto Mexicano de Tecnología del Agua, 39–63.
Moreno, J. M., Laguna, C., Calvo, E., Marengo, J. A., and Oswald, U. (2020). Adaptation to climate change risks in Ibero-American countries—RIOCCADAPT report. Madrid, España: McGraw-Hill.
O'Neill, B. C., Tebaldi, C., Van Vuuren, D. P., Eyring, V., Friedlingstein, P., Hurtt, G., et al. (2016). The scenario model Intercomparison project (scenario MIP) for CMIP6. Geosci. Model Dev. 9, 3461–3482. doi: 10.5194/gmd-9-3461-2016
Ortíz, L., Sánchez, E., Castrejón, M. L., Terrazas, H., Rodríguez, A., Quiroz, R. E., et al. (2013). Morelos frente al cambio climático: Análisis y perspectivas. Gobierno del Estado de Morelos, Secretaría de Desarrollo Sustentable de Morelos.
Ostrom, E. (2019). A general framework for analyzing sustainability of social-ecological systems. Science 325, 419–422. doi: 10.1126/science.1172133
Oswald, U. (2016). Cambio ambiental global, seguridad alimentaria y de agua en América Latina: un reto para la paz. MEC-EDUPAZ 1. Available at: http://www.mec-edupaz.unam.mx/index.php/mecedupaz/article/view/54805/0
Oswald, U. (2023). Migración climática y fronteras militarizadas: seguridad humana, de género y ambiental. Frontera norte 35, 1–28. doi: 10.33679/rfn.v1i1.2292
Pahl -Wostl, C. (2008). Requirements for adaptive water management. Adaptive and integrated water management: Coping with complexity and uncertainty : Springer, 1–22.
PINCC. (2024). Climate Change Research Program. Available at: https://www.pincc.unam.mx/ (Accessed January, 2024).
Pohle, O. (2006). Caracterización física del pueblo de San Antón y áreas Adyacentes. Tesis de maestría, Universidad Autónoma de Guerrero.
Rathe, L. (2017). La sustentabilidad en los sistemas socio-ecológicas. Utopía y Praxis Latinoamericana 22. https://produccioncientificaluz.org/index.php/utopia/article/view/22635
REPDA-CONAGUA (2019). Volumen concesionado por municipio. Available at: http://sina.conagua.gob.mx/sina/tema.php?tema=usosAgua
Salinas Villafañe, A. A., and Paz Rada, O. (2011). Aplicación del Modelo de Planificación Hídrica de Cuencas Weap al Proyecto: Aducción de Recursos Hídricos Mururata. Revista Tecnología, Investigación y Docencia, 6:27.
Sánchez Torres, G. R. (2016). Consultoría para la integración, análisis y propuesta de un esquema que permita aplicar y monitorear el balance hidráulico a nivel cuenca y que incluya escenarios de cambio climático disponibles en México. Proyecto: 00086487 “Plataforma de colaboración sobre cambio climático y crecimiento verde entre Canadá y México 2013-2016.” Reporte técnico. Programa de Naciones Unidas para el Desarrollo (PNUD)- Instituto Nacional de Ecología y Cambio Climático. (INECC).
Sánchez Torres, G. R., Vargas, R., Conde, A. C., and Echevarría, L. (2006). Modelación de la disponibilidad del agua en la cuenca del río Guayalejo-Tamesí, Tam. Reporte de avance del proyecto “Modelo WEAP”. Presentación en PowerPoint. Consejo de Cuenca del Río Pánuco. Reserva de la Biósfera de “El Cielo.” El Colegio de Tamaulipas.
Sánchez-Santillán, N., de la Lanza-Espino, G., Garduño, R., and Sánchez-Trejo, R. (2015). La influencia antropogénica en el Cambio Climático bajo la óptica de los Sistemas Complejos. Revista Iberoamericana de Ciencias. Available at: https://www.scielo.org.mx/scielo.php?script=sci_arttext&pid=S0188-46111996000100003
Sarker, S. (2022). Fundamentals of climatology for engineers: lecture note. Eng 3, 573–595. doi: 10.3390/eng3040040
SwissRe (2023). SwissRe sustainability report 2023. Retrieved from https://www.swissre.com/sustainability/stories/sustainability-report-2023.html
Tatebe, H., Ogura, T., and Nitta, T. (2019). Description and basic evaluation of simulated mean state, internal variability, and climate sensitivity in MIROC6. Geosci. Model Dev. 12, 2727–2765. doi: 10.5194/gmd-12-2727-2019
Taylor, P., Lidèn, R., Ndirangu, W., and Jin, L. (2008). Gestión integrada de los recursos hídricos para organizaciones de cuencas fluviales. Pretoria, Sudáfrica: International Network for Capacity Development in Sustainable Water Management (Cap-Net), United Nations Development Programme (undp). Available at: https://gsagua.com/wp-content/uploads/2010/10/2008-girh-para-organizaciones-de-cuencas-fluviales.pdf.
UN Habitat (2022). United nations 2023 water conference on the midterm comprehensive review of the implementation of the objectives of the international decade for action, “water for sustainable development.” Available at: https://sdgs.un.org/sites/default/files/2022-12/UN-Habitat%20new.pdf.
UNAM (2022). Atlas Climático Digital de México. Available at: https://atlasclimatico.unam.mx/acdm/visualizador (Accessed May, 2022).
UN-WATER (2019). Informe de políticas de ONU-AGUA sobre el Cambio Climático y el Agua. Recuperado de https://www.fsnnetwork.org/es/resource/climate-change-and-water-un-water-policy-brief
Valladares-Cisneros, M. G., Miranda, Z. L., and González, Z. V. (2021). Índice de Calidad del Agua del río Yautepec del Estado de Morelos, vol. 17, 1–7. doi: 10.30973/inventio/2021.17.41/7
Watch, W. R. (2022). Global historical emissions by Contry. Washington, DC: World Resources Institute. https://www.climatewatchdata.org/ghg-emissions (Accessed June, 2022).
WCRP (2021). Descripción general CMIP6 : Diseño y organización experimental Available at: https://www.wcrp-climate.org/wgcm-cmip/wgcm-cmip6 (Accessed July, 2021).
World Economic Forum (2024). The Global Risks Report 2024. 19th Edition. Insight Report. Available at: https://www.weforum.org/publications/global-risks-report-2024/ (Accessed January, 2024).
WorldClim (2024). World Clim database. Available at: https://www.worldclim.org/ (Accessed February, 2024).
Keywords: water models with climate change scenarios, Ravines System of Northwest Morelos, climate extremes, socioenvironmental impacts on water, groundwater availability, water scarcity, chaotic urbanization, global south
Citation: González-Zurita J and Oswald-Spring Ú (2024) Modeling water availability under climate change scenarios: a systemic approach in the metropolitan area in Morelos, México. Front. Water. 6:1466380. doi: 10.3389/frwa.2024.1466380
Edited by:
Christopher E. Ndehedehe, Griffith University, AustraliaReviewed by:
Shiblu Sarker, Virginia Department of Conservation and Recreation, United StatesPankaj Kaushik, WSP, Australia
Copyright © 2024 González-Zurita and Oswald-Spring. This is an open-access article distributed under the terms of the Creative Commons Attribution License (CC BY). The use, distribution or reproduction in other forums is permitted, provided the original author(s) and the copyright owner(s) are credited and that the original publication in this journal is cited, in accordance with accepted academic practice. No use, distribution or reproduction is permitted which does not comply with these terms.
*Correspondence: Jazmín González-Zurita, amF6bWluZ29uemFsZXp6dXJpdGFAZ21haWwuY29t