- 1Aquariparia-Limnology Lab, Department of Ecology, Institute of Biological Sciences, University of Brasília (UnB), Brasilia, Brazil
- 2ADASA—Agência Reguladora de Águas, Energia e Saneamento Básico do Distrito Federal, Brasília, Brazil
- 3TWRA—Tropical Water Research Alliance, Brasília, Brazil
Diverse anthropogenic activities have threatened the valuable tropical biodiversity and freshwater reserves. Despite that, there is little concern in the Brazilian legislation about the ecological integrity of aquatic ecosystems. We proposed a framework based on two joint indices, allowing the creation of a management tool. The first is the Tropical Water Healthy Index (TWHI), a river index that integrates pressures on aquatic ecosystems, their conditions, and societal/governmental response. The second is the Suitability of the Class of Use (SCU), which measures whether rivers’ conditions are in accordance with their classes of use proposed for the legal Brazilian water framework. Our results showed that most sites had good river health conditions, with the worst conditions occurring in areas with high urban occupations followed by agricultural areas. The second index indicated that few streams were poorly or incompatible with their classes of use, suggesting their classifications were based on current conditions but not future intentions. The force of these joint analyses was shown in the Melchior River stretch, which was classified as compatible with SCU, but the TWHI was critical. This is good to show that we simultaneously have a problem with the legislation and to indicate something that needs to be done quickly for managers. Therefore, we built a management model that can assess the real situation of aquatic ecosystems and define efficient management strategies, producing alerts for the decision-maker.
1 Introduction
Water bodies, such as rivers, lakes, and wetlands, are essential to Earth’s ecosystems, providing numerous benefits to humans and the environment. However, human activities and environmental changes have increasingly threatened these vital resources in recent decades. Pollution from agricultural and industrial activities, urbanization, deforestation, and climate change are just a few examples of the factors contributing to these aquatic ecosystems’ degradation (Crouzeilles et al., 2017; Strassburg et al., 2017; Wen et al., 2017). As a result, the quality and availability of freshwater resources are at risk, and these water bodies’ biodiversity and ecosystem services are under threat.
Brazil, which holds approximately 12% of the world’s freshwater supply, has classified water bodies based on a series of water quality parameters following the Water Bodies Framework by Class of Use (WFCU) since 2005. The Framework assigns water bodies to one of five different classes to ensure that water quality is compatible with the designated best uses for each class (Table 1). This allows management actions to be directed toward restoring and maintaining places where better conditions are expected. However, although the best water quality classes aim to preserve aquatic assemblages and ecosystems, only a few biological parameters, such as the thermotolerant coliforms and cyanobacteria density, are referenced in the legislation (Brasil, 2005). In contrast, India uses a similar approach but includes biological indicators, such as fish and insects, as part of the assessment criteria for their five-class classification system (Singh and Saxena, 2018). This reflects an intention in India to describe the quality of a freshwater ecosystem comprehensively. At the same time, in Brazil, only a few States have carried out some biomonitoring, such as São Paulo (CETESB, 2012).
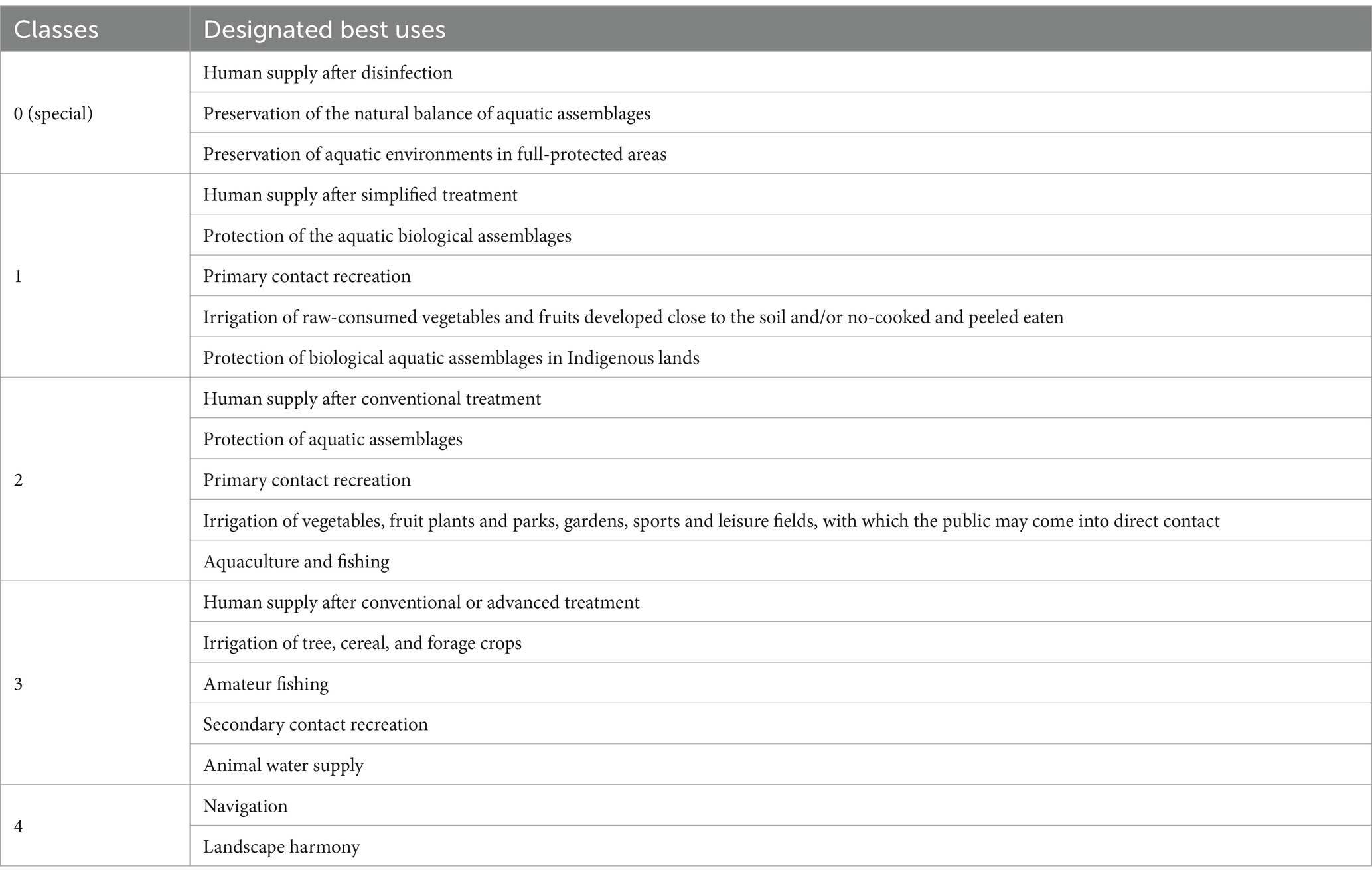
Table 1. Current water bodies framework by class of use, which presents five classes (0–4) and the respective uses foreseen for each one of them.
From an ecological perspective, a healthy river is characterized by “ecological integrity” (Boulton, 1999), which refers to its ability to sustain and maintain a balanced, integrated, and adaptive community of organisms with a species composition, diversity, and functional organization comparable to that of natural habitats in the region (Karr, 1999). By using biological assemblages and ecosystem processes, it may be possible to quantify the impact tools to measure the effects of the complex mechanisms of environmental degradation mechanisms and their interactions (Karr, 1999; Ruaro and Gubiani, 2013). Thus, shifting from a water quality approach to an ecosystem integrity approach is vital in dealing with the key changes facing the pressures and challenges aquatic ecosystems have been suffering and are currently facing.
The concept of ecological integrity assessment was extended to include political, social, and economic aspects that cannot be separated from the notion of river health (Fairweather, 1999). The Organization for Economic Co-Operation and Development (OECD) has proposed a model based on three indicators: pressure, condition, and response (P-C-R; OECD, 2003). Pressure indicators (P) measure human-induced environmental stressors such as vegetation removal, pollution release, and physical alteration of waterways. Condition (C) indicators assess environmental performance. Response” indicators (R) track society’s reactions to trends in other indicators to improve or rectify problems and may involve developing public policies, social engagement, and management actions.
Ecological integrity assessments considering human pressures on ecosystems and the interaction between society and water bodies are gaining popularity. Recently, Luo et al. (2018) proposed a novel framework to evaluate the health status of rivers based on the harmony theory, which considers the balance between river ecosystem integrity and human demand for services. In Australia, the widely used Australian River Assessment System (AUSRIVAS; Simpson and Norris, 2000) has come under scrutiny because it does not consider the anthropogenic pressures on ecosystems. A recent study recommends using diagnostic methods to identify stressors that cause ecological impact rather than simply inferring the intensity of the effect and assigning quality ratings to assessment sites (Chessman, 2021).
In monitoring programs for aquatic ecosystems, applying a guiding framework becomes complex and challenging due to the diverse realities regarding human stressors, natural characteristics, management capacity and data availability (Pinto and Maheshwari, 2014). The need for such a framework is even more significant in countries with large territories, like Brazil, to facilitate understanding results at the managerial level (Anwar Sadat et al., 2020). Furthermore, a well-defined framework enables the standardization of a process that can be implemented on a larger scale with necessary adaptations.
Our goal was to take advantage of a comprehensive database on land use, hydrology, water quality and ecological attributes of different biological communities for 50 stream stretches draining three major river basins in the Cerrado biome, leading to two aims to (1) develop a comprehensive methodology for evaluating the health status of streams and rivers and assessing their suitability for their intended best uses (i.e., class of use); (2) to propose a river health framework that can assist in making management decisions related to watersheds.
2 Materials and methods
2.1 Study area and data sampling
The Brazilian central plateau over the federal capital (Distrito Federal) was studied. The region, entirely located in the Cerrado biome (Brazilian savannah), hosts headwaters of the three major Brazilian river basins: Tocantins-Araguaia, Paraná, and São Francisco (Figure 1). The predominant land uses are natural vegetation (Cerrado), agriculture and urban occupation (approx. 3 million inhabitants). The climate is characterized by two well-defined seasons, dry (April to September) and wet (October to March), with average temperature and annual precipitation around 20°C and 1,700 mm, respectively and altitude ranging from 750 to 1,350 m.
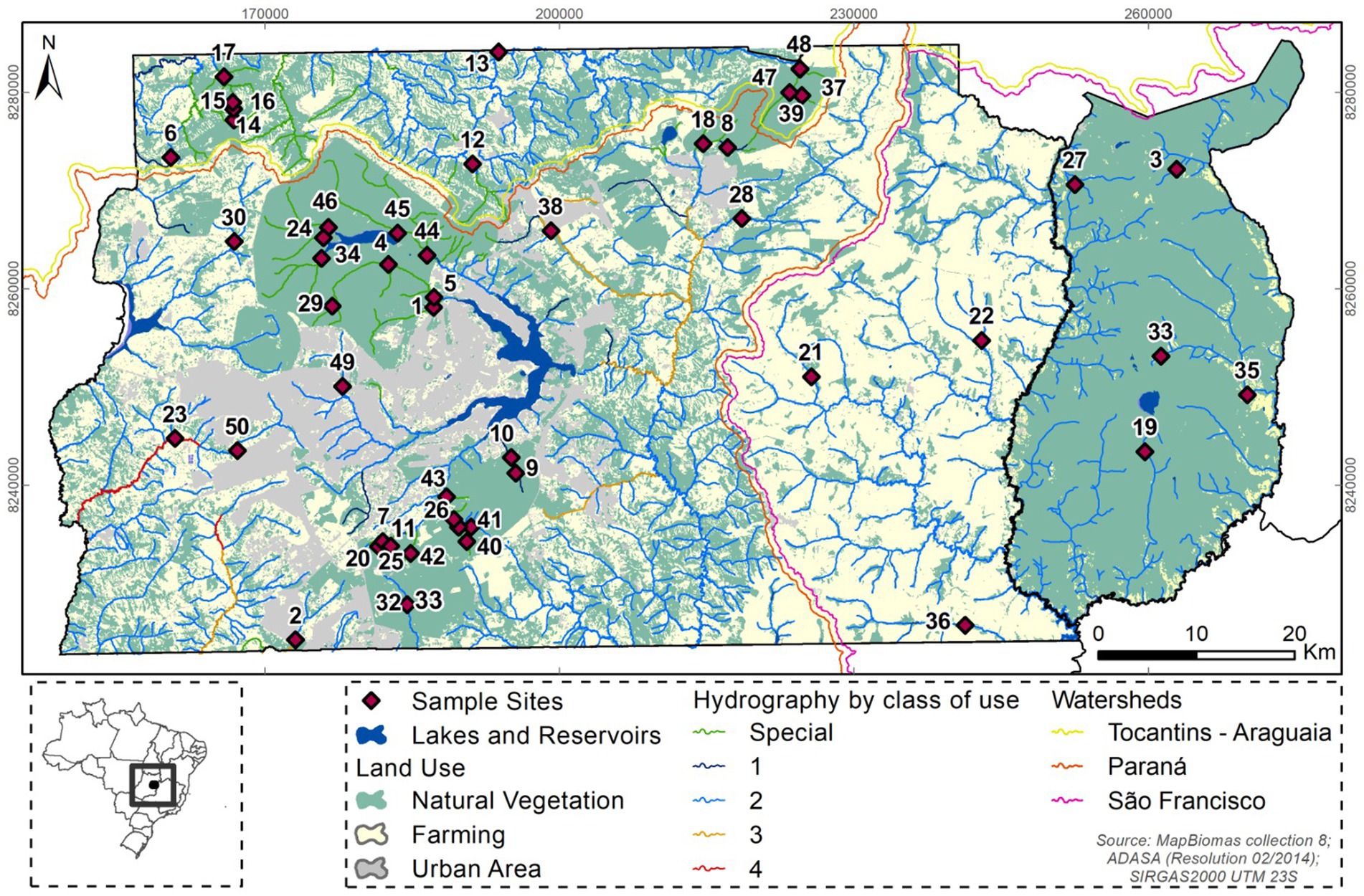
Figure 1. Study area, sample sites, and the hydrography colored according to the class of use determined by the Waterbodies Framework by Class of Use.
Abiotic and biotic data were collected in 50 study sites and in both seasons, however, previous data analyses indicated much more consistent results in the dry season due to the frequent regime of disturbance experienced by biological communities during rainy season. Thus, all further analyses were conducted with data collected in the dry season (August/September) of 2018 (COUTO, CAMILA AIDA CAMPOS, 2021).
2.2 Tropical waters health index and suitability to the class of use index
The methodology used to develop the Tropical Waters Health Index (TWHI) and the Suitability to the Class of Use (SCU) index was based on the Pressure-Condition-Response (P-C-R) approach proposed by OECD (2003) and the Harmony Degree method suggested by Luo et al. (2018). The steps taken to generate these indexes are described below and summarized in Figure 2.
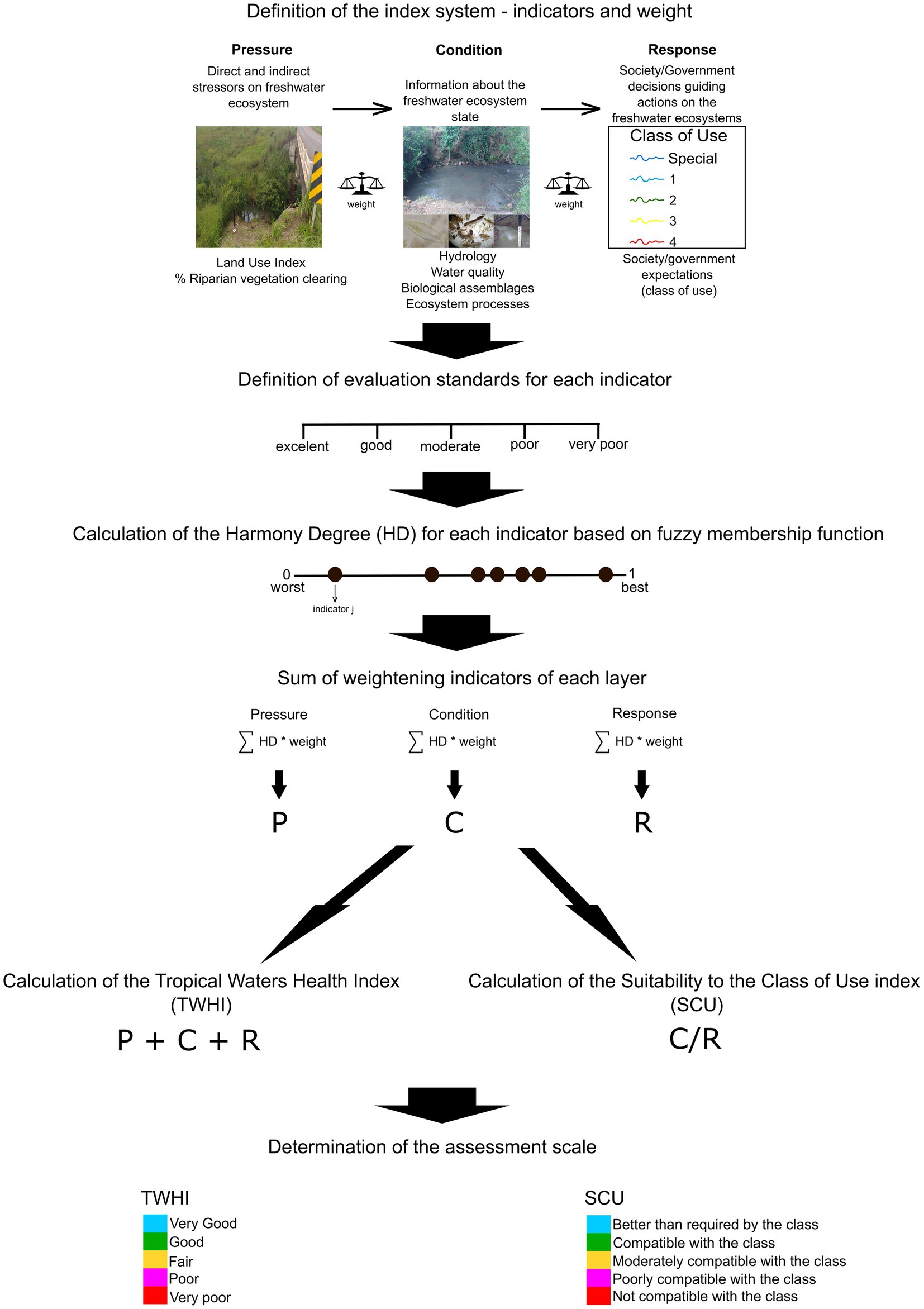
Figure 2. Scheme of the calculation of the Tropical Waters Health Index (TWHI) and the Suitability to the Class of Use Index (SCU) based on the P-C-R approach and the Harmony Degree.
The TWHI consisted of three layers: pressure, condition, and response. To represent the relevant aspects of the study region, attributes and indicators were selected for each layer (Table 2).
Changes in land use can encompass multiple stressors on aquatic ecosystems (Allan, 2004). To assess these pressures, the Land Use Index (LUI, adapted from Rawer-Jost et al., 2004) was applied to the upstream catchment, and the percentage of riparian vegetation clearing (30 m each bank along upstream) was selected as an indicator.
The LUI was calculated using the following equation: LUI = (4 × CAT_urb) + (2 × CAT_agr) + (1 × CAT_mod) [COUTO, CAMILA AIDA CAMPOS, 2021; adapted from the Land Use Index (LUI) proposed by Rawer-Jost et al. (2004)], where CAT_urb, CAT_agr, and CAT_mod represent the percentage of urban, agricultural, and other land uses (such as allotment, exposed soil, and eucalyptus plantations) in the upstream catchment. The condition indicators were represented by hydrological, water quality, and ecological attributes (Table 2).
Hydrological indicators are crucial, particularly for headwater streams where stream flows are naturally low, as in the study area. The hydrological indicator was derived from the deviation of the expected natural streamflows values (minimum, maximum and average historical values for August/September) [Governo do Distrito Federal (GDF), 2012]. We considered values below and above expected since, despite the region’s major problem being drought, there are also large rainfall events that increase the flow to non-natural levels.
Water quality indicators were chosen based on their importance in the study region, where they are typically associated with human impacts, particularly urbanization (Silva et al., 2011; Fonseca et al., 2014; COUTO, CAMILA AIDA CAMPOS, 2021). These included conductivity and dissolved phosphorus. Ecological indicators selected were periphytic diatoms (percentage of Eunotia and Trophic Diatom Index according to Kelly, 1998); macroinvertebrate assemblages (percentage of Oligochaeta and Hirudinea, percentage of Ephemeroptera, Plecoptera, and Trichoptera, and macroinvertebrates abundance) and algal biomass production (chlorophyll a production). These indicators were chosen because they have been shown to respond to human disturbances in the study region (COUTO, CAMILA AIDA CAMPOS, 2021).
The official Water Bodies Framework by Class of Use (WFCU) determined the class of use for each watercourse and was considered the response indicator. The type of use is related to the designated best services intended by the stakeholders and does not necessarily constitute the current quality of the river but the expected quality. The class of service reflects society’s and government’s expectations about the water bodies, and technical, economic, social, and political aspects are considered in its determination.
2.3 Calculation weight of each indicator
We argue that pressure, condition and response together may represent the healthiness of an aquatic ecosystem, so we distributed equal weight among the three layers (~0.33) and into each layer, the weight was divided equally among the indicators (Table 2). We could have assigned different weights to the indicators, using the entropy method suggested by Luo et al. (2018). However, the index worked much better with equity among the indicators, and this is very much in line with the principle of equal importance among the three layers. Furthermore, the results were highly consistent with what we know about each river in the region, considering the authors’ great know-how in monitoring the rivers in this region.
2.4 River health assessment criteria
2.4.1 Determination of the evaluation standards
To determine the classification criteria of river health, it is usually necessary to carry out threshold research and analyze the values of the indicators among different rivers (Anwar Sadat et al., 2020). We considered land use and water ecological attributes as thresholds values identified in specific models developed in previous studies carried out at the same sites (COUTO, CAMILA AIDA CAMPOS, 2021). Water quality references were based on national guidelines (Brasil, 2005) and previous studies (COUTO, CAMILA AIDA CAMPOS, 2021). The reference stream flows were extracted from the Integrated Water Resources Management Plan for Distrito Federal [Governo do Distrito Federal (GDF), 2012], considering as reference the historical monthly average, maximum and minimum stream flows of August and September (Table 2). For the “Response,” the use classes were considered the standard values. Following the methodology of Luo et al. (2018), we selected five ranges for each indicator. The evaluation standard nodes were classified as Excellent, Good, Moderate, Poor, and Very poor (Table 2).
2.4.2 Harmony degree
The harmony degree was calculated considering the five nodes of the evaluation criteria (Table 2) and the fuzzy membership function, following the steps suggested by Luo et al. (2018). Efficiency indicators are those for which higher values are better for the environment (e.g., % of EPT)—also referred positive indicators–, while cost indicators are those for which higher values are worse for the environment (e.g., Land Use Index)—also referred as negative indicators.
We used formulas described by Anwar Sadat et al. (2020) to calculate the harmony degree of the cost and efficiency indicators so that the harmony degree is standardized with values ranging from 0 (indicative of the worst condition) to 1 (indicative of the best condition). The “cost indicator” formula was applied to natural streamflow deviation (S_dev) values above optimal conditions, while the “efficiency indicator” formula was applied to values below optimal conditions. This was done because, in this scenario, higher and lower values are both undesirable.
To the response layer, as the classes of use are discrete and non-continuous values, it was not necessary to apply the fuzzy membership function, and the harmony degree was determined just using intervals from 0.2 to 1. Class 0 (special), 1, 2, 3, and 4 have harmony degrees equal to 1, 0.8, 0.6, 0.4, and 0.2, respectively. We did not assign a value of zero to Class 4 because the suitability to the class of use index calculation uses the response layer as the denominator, and this cannot have a value of zero.
2.4.3 Determination of the river health index
The Tropical Waters Health Index (TWHI) is the weighted average of the harmony degree of collected single indexes calculated above. TWHI is now obtained by Equation 1:
Where TWHI(t) is the tropical waters health index at time t, TWHI(t) ∈ [0,1]. wj is the weight of the jth indicator, m is the river health indicators. The closer the TWHI value is to 1, the healthier the aquatic ecosystem.
A five-grade assessment scale from 0 to 1 with 0.2 increments was established to describe the river health status as Critical, Poor, Fair, Good, and Very Good (Table 3).
2.5 Determination of the suitability to the class of use index
To check if the water ecosystem conditions are coherent with the expected designated best uses (class of use), we applied the ratio between the weighted harmony degree of the “condition” layer by the “response” layer (Equation 2). If the “condition” value is higher than the “response” value, the classification is considering a worse condition than the freshwater ecosystem really has. In contrast, if the “condition” value is lower than “response” the stream/river classification is better than the freshwater ecosystem presents. This is a simplified way to verify the suitability of the ecosystem condition to the current class of use. A five-grade assessment scale from 0 to 1 was established to describe the SCU (Table 4), with intervals of 0.2. The first band was extended to zero for didactic purposes only, since, in fact, the lowest value would be 0.2 (minimum value for “response” indicator), and the first band from 0.2 to 0.4.

Table 4. Suitability of the ecosystem health conditions to the class of use (SCU) based on the ratio Condition/Response.
Where,
c = condition indicators, wj is the weight of the jth indicator
and
wj is the weight of the response indicator.
2.6 Framework for stream ecosystem health assessment
This study proposes the evaluation of water bodies under two aspects; the first is that the demands of society are considered part of the health index assessment, understanding that the classes of use guide management actions and contribute so much to the current condition of water bodies; the second in which the condition of the river is confronted with the respective class of use.
The core of the method remains on the elaboration of the index system, determination of the evaluation standards, the harmony degree calculation, the weighting of the indicators, the calculation of the health index (TWHI), and the suitability to the class of use index (SCU). However, after the index calculations, it is necessary to determine the acceptable limits for each index. In this study, following Anwar Sadat et al. (2020), the 0.4 (Table 3) limit for TWHI was considered the minimum acceptable condition. Below this value, it is necessary to define steps to improve the condition of the aquatic ecosystem. Between 0.6 and 0.4, the situation is acceptable but requires attention, and above 0.6, the stream is in good condition of ecological integrity.
Regarding the suitability of the class of use (SCU), we determined three ranges of values. Values above 1 indicate that the river’s condition is better than the proposed class. Between 1 and 0.6, there is some degree of compatibility between condition and class of use. Less than 0.6 indicates that actions are necessary to achieve the proposed uses.
The framework indicates each index’s required and general actions (TWHI and SCU). However, we proposed more specific management actions for each combination of results from the two indices.
We did not perform an exclusive model validation step for a few reasons. First, the main objective of this framework was to compare a stretch of stream with its best expectation and with its class of use. Thus, the TWHI is not assumed to be (or be used) as a predictive model. Second, a classic validation of any comprehensive model (such as ours) will involve a huge amount of collected data, which was not feasible our case, considering the high number of parameters, sampling and, time and cost of analysis. However, validation of data analyses was indeed applied in several steps of index construction. For example, residuals of exploratory and BRT analyses were a first step of validation; BRT analyses also used cross-validation to reduce data variability and to prevent overfitting (details of preliminary and additional data exploration can be found at Campos et al., 2021a). Thus, the validation was done indirectly through previous data analyses, while validation of the proposed index requires the application of the index in future initiatives such as those related to a continuous monitoring effort.
3 Results
3.1 Harmony degree of indicators
Considering all the study sites, the lower harmony degree average values were observed for the following indicators: natural streamflow deviance (S_dev; 0.502), the percentage of Eunotia (Eunotia; 0.657), and the percentage of the EPT group (EPT; 0.418). On the other hand, those that presented higher values were the Trophic Diatom Index (TDI; 0.899), percentage of Oligochaeta and Hirudinea (Oli_Hir; 0.922), and Algal biomass (Chl; 0.922) (Figure 3; Supplementary material).
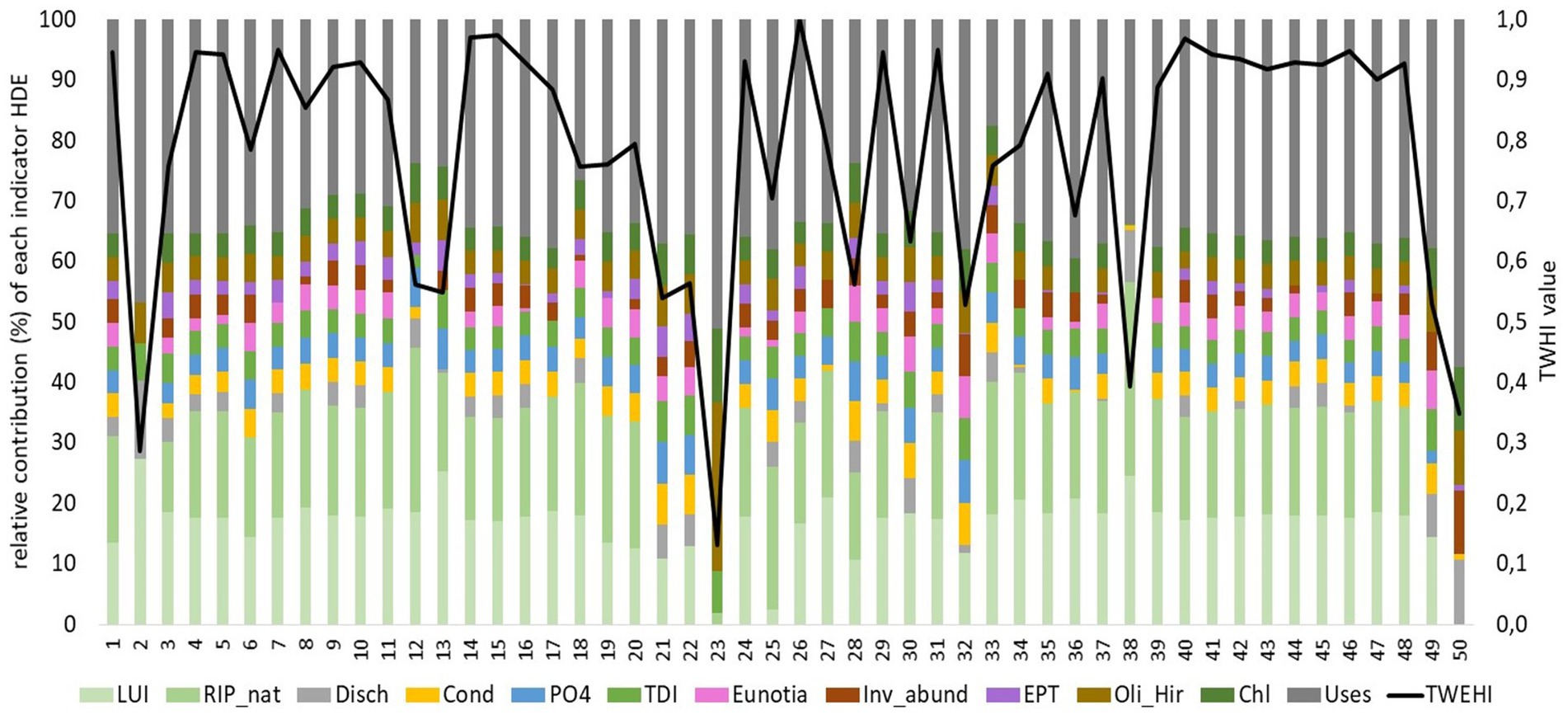
Figure 3. Weightening harmony degree (HD) of each indicator and the Tropical Waters Health Index (TWHI, black point). Indicators are grouped by layer (pressure, condition, and response) on the bottom of the image.
The absence of any indicator means its contribution was zero, i.e., it contributed to reducing the value of TWHI. Sites that presented the lowest TWHI values (below 0.4) were 2, 23, and 50 characterized by low contributions of the pressure layer indicators, i.e., they are more subject to the action of land uses in the catchment and/or riparian corridor. Also, they presented low contribution for some of the condition indicators. Site 38 (below 0.4), despite showing relatively good contribution from pressure indicators, it fails to present harmony degree zero or very close to zero for almost all condition indicators. In sites 12 and 13, despite presenting a balance between the three layers, all have moderated values, dropping the value of the index. Sites 21, 22, and 32 are negatively influenced by pressure, but still have good condition and moderated response. In site 28, despite the low contributions of the pressure and response layers, the condition layer had a good contribution of all indicators, which ensured a still reasonable TWHI value (0.6).
3.2 Tropical waters health index and suitability to the class of use index
Among the study sites, most of them (31) presented TWHI status “Very Good,” 10 were classified as “Good,” 5 were “Fair,” 3 were “Poor,” and 1 site was “Critical” (Figure 4). Sites classified as “Good” and “Very Good” were located in areas with a predominance of natural vegetation, whereas sites with “Fair” status were predominant in agricultural areas. “Poor” and “Critical” status sites were related to urban areas (Figure 4).
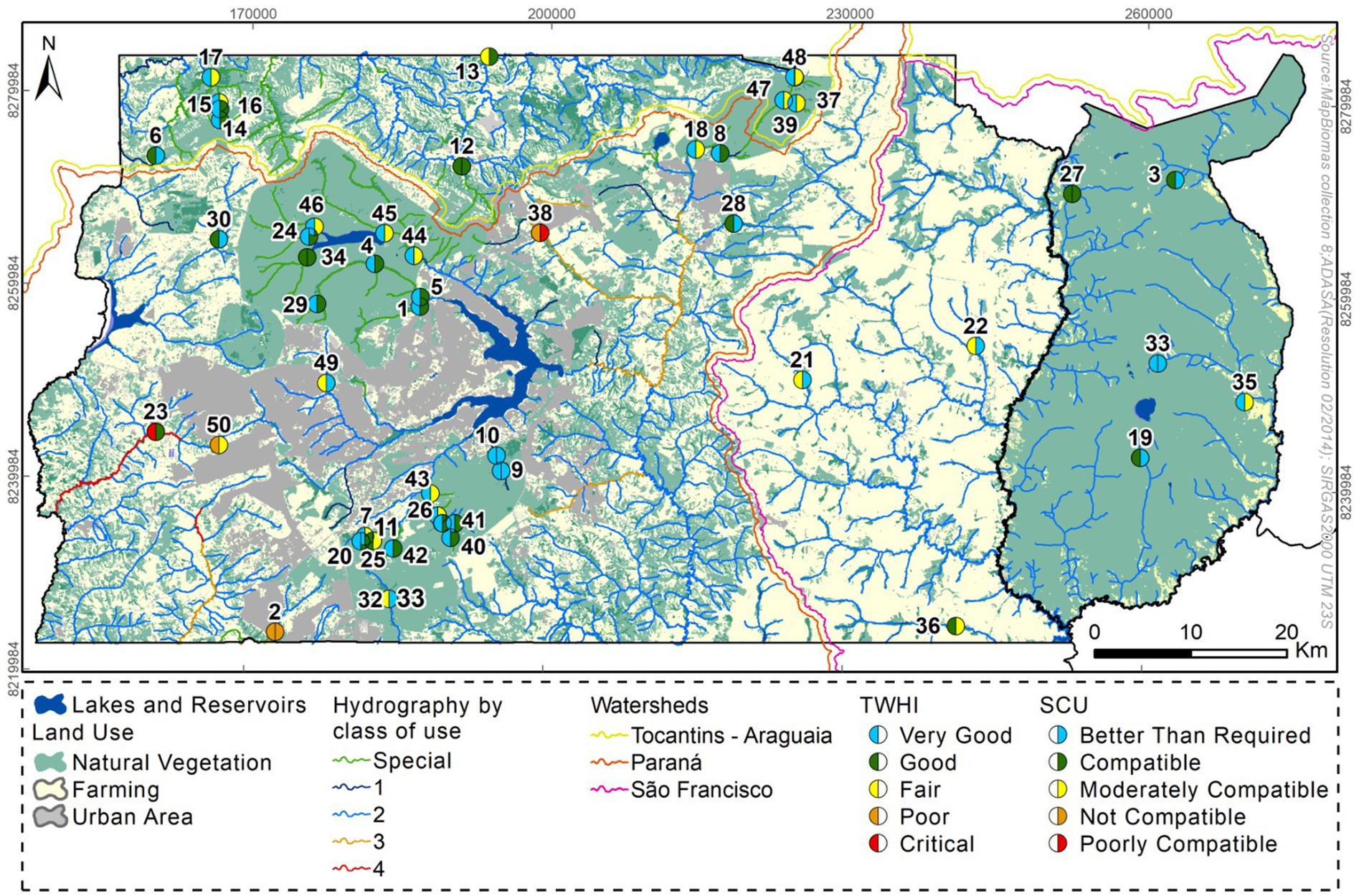
Figure 4. Spatial distribution of Tropical Waters Health Index (TWHI) and Suitability to the Class of Use (SCU). Number of sites 1–50 (see TWHI and SCU values for each sample site in Supplementary material).
Suitability of the Class of Use-wise, 12 sites presented better conditions than expected for their class of use, 19 sites presented condition compatible with the class of use, 17 were moderately compatible, one poorly compatible and one not compatible (Figure 4).
3.3 River health and suitability to the class of use assessment framework
Our framework proposal was built up on the basic concepts of P-C-R and Harmony Degree approaches. The activities flow involved steps from data collection/selection to management actions for the different range values of TWHI and SCU (Figure 5). The framework predicted the interaction between the two main indices and feedback vectors since management decisions should impact new pressure/condition/response profiles.
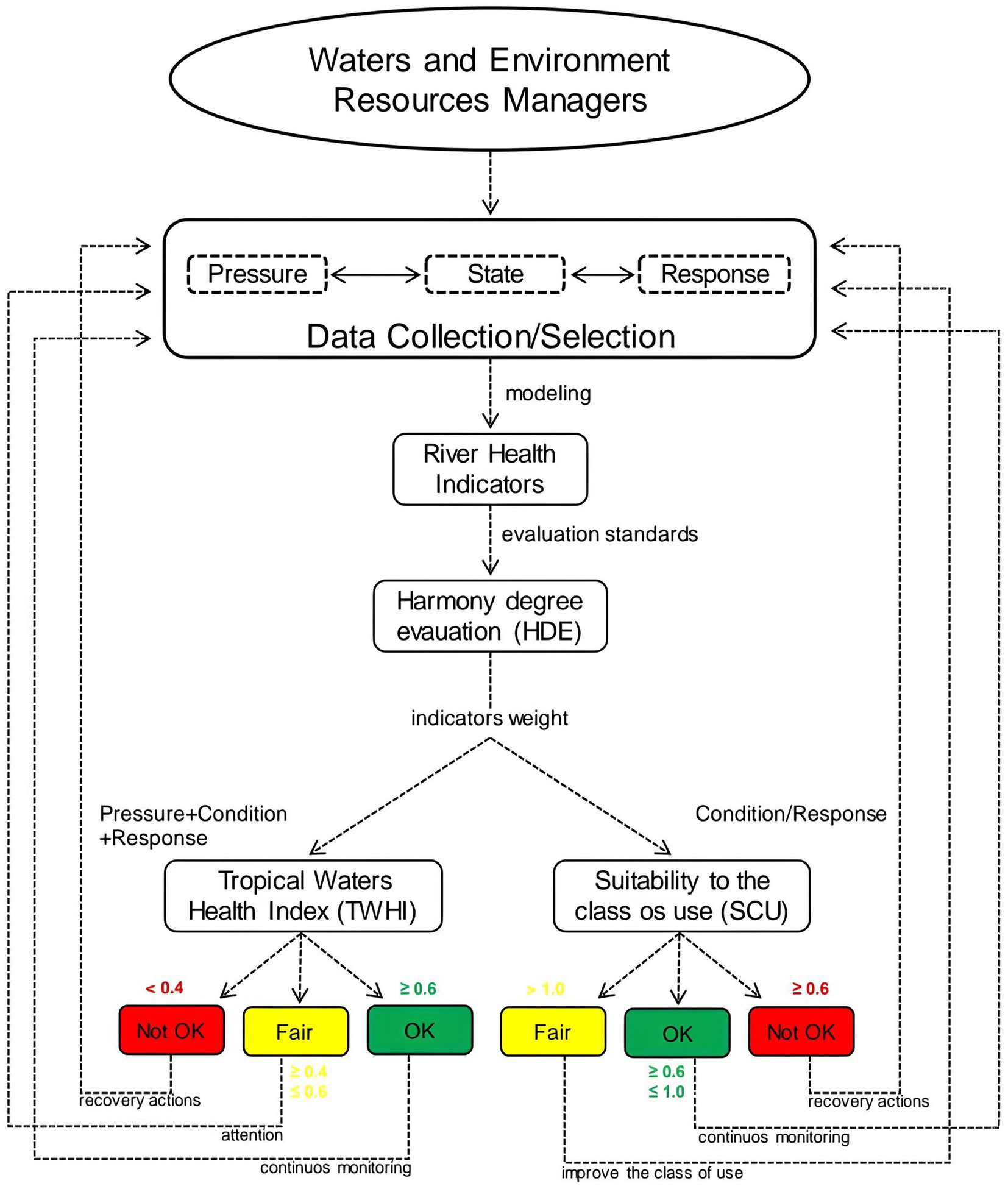
Figure 5. Framework for river health assessment and suitability to the class of use to support water and watershed management.
We proposed specific management actions for each combination TWHI × SCU (Table 5). The most common result (31 sample sites) suggested continuing monitoring since the health status was equal or higher than “Good” (TWHI >0.6) and the conditions were at least moderately compatible with the class of use but not better than expected (0.6 < SCU ≤ 1).
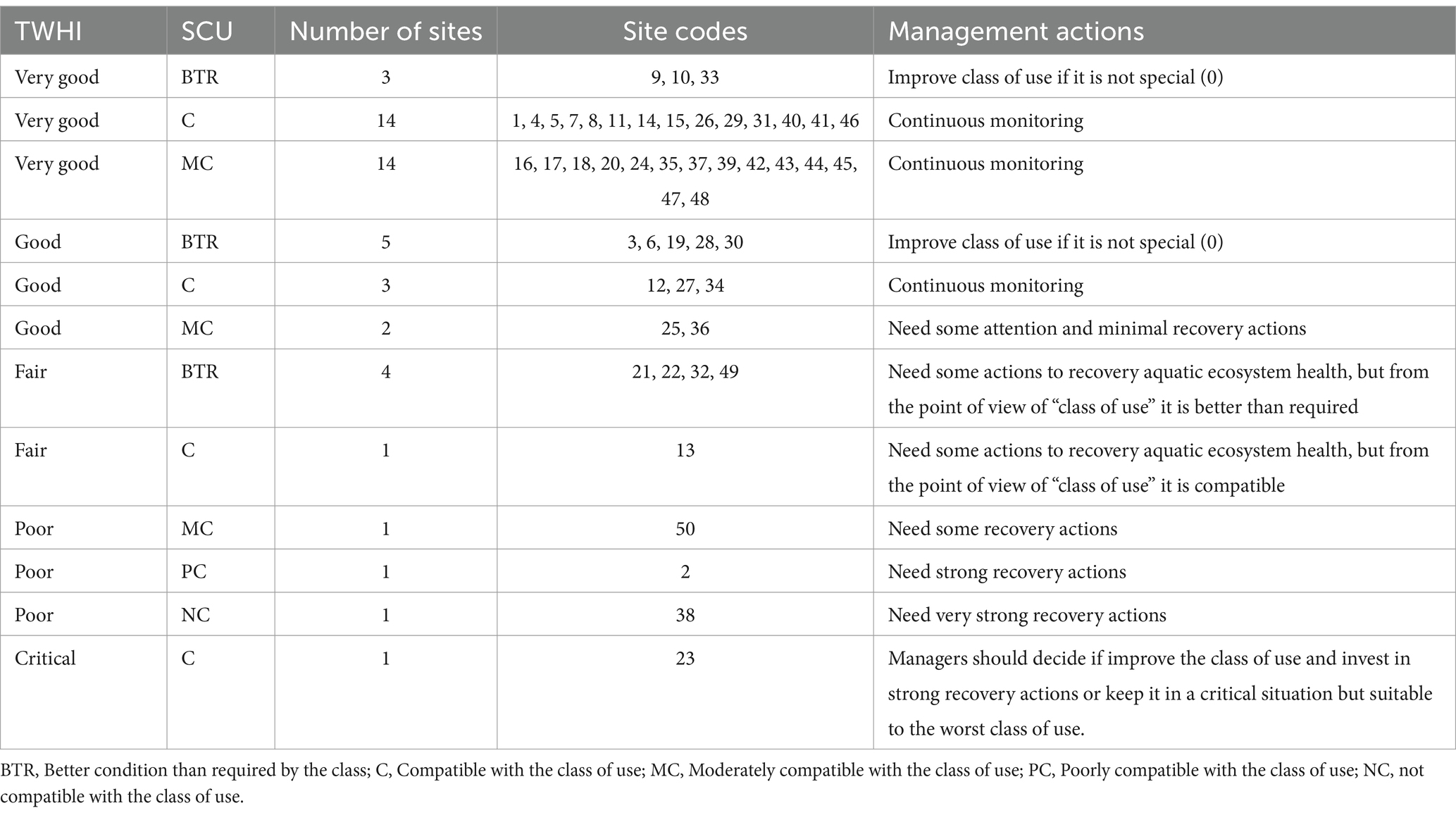
Table 5. Detected combinations of TWHI and SCU in the study sites, number of sites in each situation, site codes and management actions proposed.
In 12 sites, the SCU presented “Better condition than required by the class” (BTR) status. In those cases, different strategies have been suggested for each corresponding TWHI. If the TWHI was “Very Good” or “Good,” the recommendation was to improve the class of use that have been adopted, unless the current class is already 0 (special). In case of TWHI equal to “Fair,” actions are necessary to improve the river health conditions, although it is suitable to the uses for which it was intended according to the current classification.
Two sites presented “Poor” TWHI status and SCU poor compatible or not compatible. One site presented “Poor” TWHI status and SCU moderately compatible. Although our framework suggested attention for SCU moderately compatible, the poor health condition induces the recommendation of strong recovery actions in the three sites.
In two sites the health condition was “Good,” but the SCU was moderately or poor compatible. In these cases, we suggested some attention and minimal or moderated recovery measures, respectively.
Finally, one site presented critical health status but compatible conditions to its class of use. From the point of view of ecosystem health, it needs strong recovery actions, but in terms of suitability to the class of use, it is suitable since it is not intended for uses that demand good water quality (class 4).
4 Discussion
The results showed that the water bodies in the study region were, in general, in good ecosystem health status. Sites in poor or critical situations were in urbanized regions, while those with status equal to “Fair” or equal/higher than “Good” were predominantly in agricultural and preserved areas, respectively. It was expected and not surprising since the pressures from urbanization are proven to be harmful to aquatic and terrestrial environments across the planet (Murray et al., 2019). Agricultural occupation, although to a lesser extent, also causes damage to aquatic ecosystems, especially in small streams (Szöcs et al., 2017). This occurs due removing of natural vegetation from the upstream drainage area and riparian forest or the chemical pollutant’s diffuse inputs (e.g., fertilizers and pesticides; Clapcott et al., 2012; Szöcs et al., 2017).
The conditions of an ecosystem are the result of the natural characteristics and pressures that it suffers. This, in turn, leads to human management actions and decisions that also end up corroborating the current condition. The imbalance of the three layers with lower TWHI values shows us potential problems and enables directing solutions. Removing of riparian vegetation and changes in land use in the upstream catchment can lead to changes in the aquatic ecosystem (Allan, 2004; Campos et al., 2021b). Even though there is still a good condition in terms of water quality, biology, and hydrology, it is expected that at some point, they will respond to pressures. Perhaps such changes have not yet been perceived due to the resistance and resilience of ecosystems (Sarremejane et al., 2020). However, it is also clear in some places that the high pressure has already caused changes in several aspects of the condition. Therefore, the level of intervention must be even higher to restore an environmental balance. A response layer with a low contribution to the index can also be interpreted as an alert because if we have expected little of a given water body in terms of quality, then little will be done for its preservation. In the long run, this can lead to increased pressures and worsening condition indicators.
Among the condition indicators, the deviation from natural streamflows was the indicator that presented lower harmony degree values. This indicates a widespread water pressure in the study area. It may be related to the more significantly water crisis (drought) recorded in the region between 2016 and 2017, with the river flows and accumulated precipitation still recovering in 2018 (Lima et al., 2018). Even preserved sites have suffered this impact, clearly demonstrating a response to a regional-scale phenomenon. In biological terms, specific indicators of diatoms, macroinvertebrates and algal biomass showed high or low contributions depending on each location. The diversity of responses for these indicators was important from an ecological point of view since this may indicate the influence of different stressors, opening possibilities for targeted recovery actions (Waite et al., 2019; de Castro-Català et al., 2020).
Although the proposed health index is linked to a specific collection site and its projection downstream depends on many factors, such as the maintenance of the river’s physical conditions, land use characteristics in the surrounding area, and the entry of specific pollutants, the study allowed for the evaluation of some rivers where collections were made at more than one point. In well-preserved areas, such as points 14, 15, 16, and 17, located on the same stream, all sites presented a “very good” TWHI. On the other hand, sites 23 and 50, also located on the same stream, showed quite different conditions, as this river suffers from a series of urban impacts, such as sewage discharge, leachate collection, and impermeable areas. This suggests that critical conditions observed at one site can propagate to other stretches of the river, especially in areas affected by urban impacts. The extent of these downstream impacts can vary based on the intensity and nature of the stressors, as well as the natural characteristics and management actions in each stretch of the river. Therefore, it is crucial to consider this propagation and extent when formulating management and restoration strategies. In other words, conditions found in critical sites may serve as indicators for assessing the health of other parts of the river and guiding appropriate mitigation and recovery actions.
Most of the study sites presented conditions at least moderately compatible with the class of use and, in some cases, even better than required by the class, which indicates a tendency to classify the rivers according to their current uses and water quality and with no prospect of improving or creating more daring goals. Most countries and regions have adopted the practice of having river health status targets as similar as possible to reference sites (e.g., European Water Framework Directive, US Clean Water Act, Freshwater Environmental Health Monitoring Program—Queensland, Australia), i.e., pristine sites (or as close as possible to their natural conditions; Marshall and Negus, 2019). From an environmental point of view, this practice makes more sense since, even if the current conditions are not the best, the goal is always to get closer to ecological integrity.
Results indicating “Better conditions than required by the class” can be understood as a “permission” to the impact. Even if its quality deteriorates, the water body will still be suit its class, and no preservation measure will be applied. Conditions that are compatible or moderately compatible with the class may indicate that rivers that should have good conditions do have them but rivers that may have bad conditions also have them. In the latter case, low condition requirements can also be understood as the “acceptance” of the impact. This government strategy moves current management away from sustainable development goals (SDGs), such as maintaining healthy and productive ecosystems and the sustaining biodiversity and ecosystem services through better management, valuation, measurement, conservation, and restoration (Griggs et al., 2013).
Only two sites presented inferior conditions (“poor” or “not compatible”) to their respective classes. Both are located downstream of the point source of treated sewage releases (2 and 38; Caesb, 2021) and are inserted in urban areas. Although low demand for water quality class (class 3) was defined for these rivers [Governo do Distrito Federal (GDF), 2014], the environmental conditions are even worse. According to the current approach, these two rivers showed results “adequate” and “in accordance with” the class of use in 2018 (ADASA, 2021), the same year we carried out the fieldwork of this study. The instability of water quality variables and the restricted response to only a few stressors can lead to misinterpretations from an environmental point of view (Gatti, 2016). The inclusion of biological and hydrological indicators in the assessment of the suitability to the class of use allowed better visualization of the river conditions concerning their classes (Anwar Sadat et al., 2020), even though the classification by classes of use is, in itself, an outdated tool.
In terms of functionality, the beauty of these indexes lies in the combination of the precision of its calculations with the flexibility in choosing the indicators and thresholds. If other variables are more relevant in another region, they can be included in the model without compromising the scale, since the degree of harmony will even out this difference. This allows the methodology to be adapted to different realities, allowing a wide diffusion of its use and, simultaneously, comparability between the results. In a country with environmental, social and political characteristics that are so different in its territory (Veiga and Magrini, 2013), this flexibility and ease of communication of results (guaranteed by color scales and value ranges) are essential for the model to be applied in large scale (Flint et al., 2017). At the same time, the composition of the indicators allows a thorough assessment of the managers about which problems must be tackled. Meeting the expectations of the various stakeholders in a cooperative approach, based on scientific evidence and effective communication, is a fundamental part of the success of a water resources management framework (Bunn et al., 2010).
Brazil has an internationally prominent role in terms of biodiversity and water resources (Agostinho et al., 2005) and, in addition, has many studies on freshwater bioindicators and environmental pressure thresholds in its various biomes (e.g., Ligeiro et al., 2013; Firmiano et al., 2017; Brito et al., 2020; Dala-Corte et al., 2020). However, the restoration of freshwater ecosystems has barely been discussed in the country and is still a topic largely restricted to the academic community. Brazilian authorities need to rethink opportunities for de-pollution, following trends in several other countries (Ko et al., 2020; Azevedo-Santos et al., 2021). The proposed framework is a valuable tool for self-evaluating the current model adopted and identifying its discrepancies about an approach committed to environmental sustainability. Brazil has all the potential for leadership in sustainable management models for aquatic environments, and this work presented one more tool to facilitate this endeavor.
5 Conclusion
River health assessment is a management tool useful to restore the health status of rivers, protect their ecological and social service functions, and create an environment in which people are in harmony with nature (Zhao et al., 2019). The simplicity of determining and communicating the indexes makes its implementation effective and accessible to the managers and other stakeholders involved (Flint et al., 2017). The combination of indexes (TWHI and SCU) made it possible to visualize the rivers under two different aspects: health and suitability to the class of use. The thresholds and actions proposed in the framework tend to broaden the vision and help managers in decision-making. In the long term, it is suggested that the current approach focused on classes by demanded best uses be shifted to a holistic approach to river health. It is also recommended that any methodologies, including this one, be continually revised and improved, as has been done even in countries where there is already a consolidated assessment of the ecological integrity of streams (Chessman, 2021).
Data availability statement
The raw data supporting the conclusions of this article will be made available by the authors, without undue reservation.
Ethics statement
The manuscript presents research on animals that do not require ethical approval for their study.
Author contributions
CC: Conceptualization, Data curation, Formal analysis, Investigation, Methodology, Project administration, Validation, Visualization, Writing – original draft, Writing – review & editing. AT: Formal analysis, Methodology, Visualization, Writing – review & editing. GS: Investigation, Visualization, Writing – review & editing. JG: Conceptualization, Funding acquisition, Project administration, Resources, Supervision, Writing – review & editing.
Funding
The author(s) declare that financial support was received for the research, authorship, and/or publication of this article. This work was supported by the Fundação de Amparo à Pesquisa do Distrito Federal (FAP-DF), Aquariparia Project (edital 05/2016-Pró-Águas; Proc. no.: 193.000716/2016), that allowed the execution of fieldwork and laboratory analyses; the Conselho Nacional de Desenvolvimento Cientifico e Tecnológico (CNPq) through research fellowship to José Francisco Gonçalves Jr. (Proc. no.: 310641/2017-9); and the Agência Reguladora de Águas, Energia e Saneamento Básico do Distrito Federal (ADASA) that provided financial support to Campos C.A.
Conflict of interest
The authors declare that the research was conducted in the absence of any commercial or financial relationships that could be construed as a potential conflict of interest.
Publisher’s note
All claims expressed in this article are solely those of the authors and do not necessarily represent those of their affiliated organizations, or those of the publisher, the editors and the reviewers. Any product that may be evaluated in this article, or claim that may be made by its manufacturer, is not guaranteed or endorsed by the publisher.
Supplementary material
The Supplementary material for this article can be found online at: https://www.frontiersin.org/articles/10.3389/frwa.2024.1423458/full#supplementary-material
References
ADASA (2021). Agência Reguladora de Águas, Energia e Saneamento Básico do Distrito Federal. Sistema de informações sobre Recursos Hídricos—DF. Available online at: www.adasa.df.gov.br (Accessed August 2, 2022).
Agostinho, A. A., Thomaz, S. M., and Gomes, L. C. (2005). Conservation of the biodiversity of Brazil’s inland waters. Conserv. Biol. 19, 646–652. doi: 10.1111/j.1523-1739.2005.00701.x
Allan, J. D. (2004). Landscapes and riverscapes: the influence of land use on stream ecosystems. Annu. Rev. Ecol. Evol. Syst. 35, 257–284. doi: 10.1146/annurev.ecolsys.35.120202.110122
Anwar Sadat, M., Guan, Y., Zhang, D., Shao, G., Cheng, X., and Yang, Y. (2020). The associations between river health and water resources management lead to the assessment of river state. Ecol. Indic. 109:105814. doi: 10.1016/j.ecolind.2019.105814
Azevedo-Santos, V. M., Rodrigues-Filho, J. L., Fearnside, P. M., Lovejoy, T. E., and Brito, M. F. G. (2021). Conservation of Brazilian freshwater biodiversity: thinking about the next 10 years and beyond. Biodivers. Conserv. 30, 235–241. doi: 10.1007/s10531-020-02076-5
Boulton, A. J. (1999). An overview of river health assessment: philosophies, practice, problems and prognosis. Freshw. Biol. 41, 469–479. doi: 10.1046/j.1365-2427.1999.00443.x
Brasil (2005). Resolução CONAMA n°357, de 17 de março de 2005. Classificação de águas, doces, salobras e salinas do Território Nacional (in Portuguese).
Brito, J. G., Roque, F. O., Martins, R. T., Nessimian, J. L., Oliveira, V. C., Hughes, R. M., et al. (2020). Small forest losses degrade stream macroinvertebrate assemblages in the eastern Brazilian Amazon. Biol. Conserv. 241:108263. doi: 10.1016/j.biocon.2019.108263
Bunn, S. E., Abal, E. G., Smith, M. J., Choy, S. C., Fellows, C. S., Harch, B. D., et al. (2010). Integration of science and monitoring of river ecosystem health to guide investments in catchment protection and rehabilitation. Freshw. Biol. 55, 223–240. doi: 10.1111/j.1365-2427.2009.02375.x
Caesb,. (2021). Companhia de Saneamento Ambiental do Distrito Federal. Available at: https://www.caesb.df.gov.br/ (Accessed August, 2022).
Campos, C. A., Kennard, M. J., and Gonçalves Júnior, J. F. (2021a). Diatom and macroinvertebrate assemblages to inform management of Brazilian savanna’s watersheds. Ecol. Indic. 128:107834. doi: 10.1016/j.ecolind.2021.107834
Campos, C. A., Tonin, A. M., Kennard, M. J., and Gonçalves Júnior, J. F. (2021b). Setting thresholds of ecosystem structure and function to protect streams of the Brazilian savanna. Front. Environ. Sci. 10:867905. doi: 10.3389/fenvs.2022.867905
CETESB (2012). Protocolo para o biomonitoramento com as comunidades bentônicas de rios e reservatórios do estado de São Paulo [recurso eletrônico]/CETESB; Mônica Luisa Kuhlmann et al. Disponível em. Available online at: https://cetesb.sp.gov.br/aguas-interiores/wp-content/uploads/sites/12/2013/11/protocolo-biomonitoramento-2012.pdf (Acessado em 15 de março de 2021).
Chessman, B. C. (2021). What’s wrong with the Australian River assessment system (AUSRIVAS)? Mar. Freshw. Res. 72, 1110–1117. doi: 10.1071/MF20361
Clapcott, J. E., Collier, K. J., Death, R. G., Goodwin, E. O., Harding, J. S., Kelly, D., et al. (2012). Quantifying relationships between land-use gradients and structural and functional indicators of stream ecological integrity. Freshw. Biol. 57, 74–90. doi: 10.1111/j.1365-2427.2011.02696.x
COUTO, CAMILA AIDA CAMPOS . (2021). Desenvolvimento de um framework para avaliação da integridade ecológica de riachos. 171 f. Tese de Doutorado em Ecologia, Universidade de Brasília, Brasília (in Portuguese).
Crouzeilles, R., Feltran-Barbieri, R., Ferreira, M. S., and Strassburg, B. B. N. (2017). Hard times for the Brazilian environment. Nat. Ecol. Evol. 1:1213. doi: 10.1038/s41559-017-0303-7
Dala-Corte, R. B., Melo, A. S., Siqueira, T., Bini, L. M., Martins, R. T., Cunico, A. M., et al. (2020). Thresholds of freshwater biodiversity in response to riparian vegetation loss in the Neotropical region. J. Appl. Ecol. 57, 1391–1402. doi: 10.1111/1365-2664.13657
de Castro-Català, N., Dolédec, S., Kalogianni, E., Skoulikidis, N. T., Paunovic, M., Vasiljević, B., et al. (2020). Unravelling the effects of multiple stressors on diatom and macroinvertebrate communities in European river basins using structural and functional approaches. Sci. Total Environ. 742:140543. doi: 10.1016/j.scitotenv.2020.140543
Fairweather, P. G. (1999). State of environment indicators of “river health”: exploring the metaphor. Freshw. Biol. 41, 211–220. doi: 10.1046/j.1365-2427.1999.00426.x
Firmiano, K. R., Ligeiro, R., Macedo, D. R., Juen, L., Hughes, R. M., and Callisto, M. (2017). Mayfly bioindicator thresholds for several anthropogenic disturbances in neotropical savanna streams. Ecol. Indic. 74, 276–284. doi: 10.1016/j.ecolind.2016.11.033
Flint, N., Rolfe, J., Jones, C. E., Sellens, C., Johnston, N. D., and Ukkola, L. (2017). An ecosystem health index for a large and variable river basin: methodology, challenges and continuous improvement in Queensland’s Fitzroy Basin. Ecol. Indic. 73, 626–636. doi: 10.1016/j.ecolind.2016.10.007
Fonseca, B. M., De Mendonça-Galvão, L., Padovesi-Fonseca, C., De Abreu, L. M., and Fernandes, A. C. M. (2014). Nutrient baselines of Cerrado low-order streams: Comparing natural and impacted sites in Central Brazil. Environ. Monit. Assess. 186, 19–33. doi: 10.1007/s10661-013-3351-8
Gatti, R. C. (2016). Freshwater biodiversity: a review of local and global threats. Int. J. Environ. Stud. 73, 887–904. doi: 10.1080/00207233.2016.1204133
Griggs, D., Stafford-Smith, M., Gaffney, O., Rockström, J., Öhman, M. C., Shyamsundar, P., et al. (2013). Sustainable development goals for people and planet. Nature 495, 305–307.
Governo do Distrito Federal (GDF) (2012). Plano de Gerenciamento Integrado de Recuros Hídricos do Distrito Federal. Available online at: http://www.adasa.df.gov.br/images/storage/programas/PIRHFinal/volume1-diagnostico_Completo.rar (Accessed June 06, 2018).
Governo do Distrito Federal (GDF) (2014). Resolução CRH n°02 de 17 de dezembro de 2014. Available online at: http://www.sema.df.gov.br/wp-conteudo/uploads/2017/09/Resolu%C3%A7%C3%A3o-CRH-n%C2%BA-02-de-2014.pdf. (Accessed March 03, 2018).
Karr, J. R. (1999). Defining and measuring river health. Freshw. Biol. 41, 221–234. doi: 10.1046/j.1365-2427.1999.00427.x
Kelly, M. G. (1998). Use of the trophic diatom index to monitor eutrophication in rivers. Water Res. 32, 236–242. doi: 10.1016/S0043-1354(97)00157-7
Ko, N. T., Suter, P., Conallin, J., Rutten, M., and Bogaard, T. (2020). The urgent need for river health biomonitoring tools for large tropical rivers in developing countries: preliminary development of a river health monitoring tool for Myanmar rivers. Water 12, 1–15. doi: 10.3390/w12051408
Ligeiro, R., Hughes, R. M., Kaufmann, P. R., Macedo, D. R., Firmiano, K. R., Ferreira, W. R., et al. (2013). Defining quantitative stream disturbance gradients and the additive role of habitat variation to explain macroinvertebrate taxa richness. Ecol. Indic. 25, 45–57. doi: 10.1016/j.ecolind.2012.09.004
Lima, J. E. F. W., Freitas, G. K., Pinto, M. A. T., and Salles, P. S. B. A. (2018) in Gestão da Crise Hídrica 2016–2018—Experiências do Distrito. ed. D. F. Emater (Brasília, DF: Adasa Caesb), 328.
Luo, Z., Zuo, Q., and Shao, Q. (2018). A new framework for assessing river ecosystem health with consideration of human service demand. Sci. Total Environ. 640-641, 442–453. doi: 10.1016/j.scitotenv.2018.05.361
Marshall, J. C., and Negus, P. M. (2019). ““Application of a Multi-Stressor Risk Framework to the Monitoring, Assessment, and Diagnosis of River Health.” n Chap. 15” in Multiple Stressors in River Ecosystems: Status, Impacts and Prospects for the Future. eds. S. Sabater, A. Elosegi, and R. Ludwig (Amsterdam: Elsevier), 255–280.
Murray, M. H., Sánchez, C. A., Becker, D. J., Byers, K. A., Worsley-Tonks, K. E. L., and Craft, M. E. (2019). City sicker? A meta-analysis of wildlife health and urbanization. Front. Ecol. Environ. 17, 575–583. doi: 10.1002/fee.2126
OECD (2003). Environmental indicators. Development, Measurement and Use. Reference Paper, OECD, Paris.
Pinto, U., and Maheshwari, B. (2014). A framework for assessing river health in peri-urban landscapes. Ecohydrol. Hydrobiol. 14, 121–131. doi: 10.1016/j.ecohyd.2014.04.001
Rawer-Jost, C., Zenker, A., and Böhmer, J. (2004). Reference conditions of German stream types analysed and revised with macroinvertebrates fauna. Limnologica 34, 390–397. doi: 10.1016/S0075-9511(04)80008-2
Ruaro, R., and Gubiani, É. A. (2013). A scientometric assessment of 30 years of the Index of Biotic Integrity in aquatic ecosystems: applications and main flaws. Ecol. Indic 29, 105–110.
Sarremejane, R., England, J., Sefton, C. E. M., Parry, S., Eastman, M., and Stubbington, R. (2020). Local and regional drivers influence how aquatic community diversity, resistance and resilience vary in response to drying. Oikos 129, 1877–1890. doi: 10.1111/oik.07645
Simpson, J. C., and Norris, R. H. (2000). “Biological assessment of river quality: development of AusRivAS models and outputs” in Assessing the Biological Quality of Freshwaters: RIVPACS and other techniques. Freshwater Biological Association. eds. J. F. Wright, D. W. Sutcliffe, and M. T. Furse (Ambleside), 125–142.
Silva, J. S. O., da Bustamante, M. M. C., Markewitz, D., Krusche, A. V., and Ferreira, L. G. (2011). Effects of land cover on chemical characteristics of streams in the Cerrado region of Brazil. Biogeochemistry 105, 75–88. doi: 10.1007/s10533-010-9557-8
Singh, P. K., and Saxena, S. (2018). Towards developing a river health index. Ecol. Indic. 85, 999–1011. doi: 10.1016/j.ecolind.2017.11.059
Strassburg, B. B. N., Brooks, T., Feltran-Barbieri, R., Iribarrem, A., Crouzeilles, R., Loyola, R., et al. (2017). Moment of truth for the Cerrado hotspot. Nat. Ecol. Evol. 1, 13–15. doi: 10.1038/s41559-017-0099
Szöcs, E., Brinke, M., Karaoglan, B., and Schäfer, R. B. (2017). Large scale risks from agricultural pesticides in small streams. Environ. Sci. Technol. 51, 7378–7385. doi: 10.1021/acs.est.7b00933
U.S. Environmental Protection Agency (USEPA) (2016). National rivers and streams assessment 2008–2009: A collaborative survey. Office of Water and Office of Research and Development, Washington, DC. Available online at: https://www.epa.gov/sites/production/files/2016-03/documents/nrsa_0809_march_2_final.pdf (Accessed August 2, 2022).
Veiga, L. B. E., and Magrini, A. (2013). The Brazilian water resources management policy: fifteen years of success and challenges. Water Resour. Manag. 27, 2287–2302. doi: 10.1007/s11269-013-0288-1
Waite, I. R., Munn, M. D., Moran, P. W., Konrad, C. P., Nowell, L. H., Meador, M. R., et al. (2019). Effects of urban multi-stressors on three stream biotic assemblages. Sci. Total Environ. 660, 1472–1485. doi: 10.1016/j.scitotenv.2018.12.240
Wen, Y., Schoups, G., and Van De Giesen, N. (2017). Organic pollution of rivers: combined threats of urbanization, livestock farming and global climate change. Sci. Rep. 7, 1–9. doi: 10.1038/srep43289
Keywords: ecological integrity, river health framework, freshwater management, river health index, water quality
Citation: Campos CA, Tonin AM, Sena G and Gonçalves Júnior JF (2024) Progressing a river health assessment framework to tropical waters. Front. Water. 6:1423458. doi: 10.3389/frwa.2024.1423458
Edited by:
Jose Fabrega Duque, Technological University of Panama, PanamaReviewed by:
Jacinto Elías Sedeño-Díaz, Instituto Politécnico Nacional (IPN), MexicoPamela A. Green, CUNY Advanced Science Research Center, United States
Copyright © 2024 Campos, Tonin, Sena and Gonçalves Júnior. This is an open-access article distributed under the terms of the Creative Commons Attribution License (CC BY). The use, distribution or reproduction in other forums is permitted, provided the original author(s) and the copyright owner(s) are credited and that the original publication in this journal is cited, in accordance with accepted academic practice. No use, distribution or reproduction is permitted which does not comply with these terms.
*Correspondence: Camila Aida Campos, camila.aida@gmail.com