- Hydrogeochemical Engineering and Remediation of Soils Laboratory, School of Chemical and Environmental Engineering, Technical University of Crete, Chania, Greece
Climate change, food and water security and ecosystem sustainable management are tightly interlinked and require holistic approaches to achieve solutions that do not impact adversely one-another. The objective of this work was to conduct studies, collect data and assess the Water-Ecosystem-Food (WEF) nexus in avocado plantations in the Mediterranean region systematically to minimize the environmental footprint while maximizing the benefits for the farmer and the environment. The study includes two distinct experiments; the first addresses the impact of soil organic amendments addition to optimize the WEF nexus and the second monitors experimentally crop water needs and thus illustrates how irrigation practices aided by technology can reduce substantially water consumption. The results showed that organic amendments addition improves fertility, nutrient sequestration and structure but only had a weak effect on biodiversity by increasing the number of unique species. For the development of an efficient irrigation system it is necessary to determine the radius around the tree, the depth of the roots and the time required for the water to reach the active root zone to determine the amount and duration of irrigation. In this way sufficient water will be added to replenish the soil moisture deficit created due to the evapotranspiration. HYDRUS-1D model was used to simulate soil moisture and the hydrologic budget of an avocado tree located in Koiliaris river basin and confirm the percolation losses to groundwater. The results of this study showed that the actual irrigation needs of avocados in the Mediterranean is less than 2,000 m3/ha which is 75% less than what is recommended and could become the primary measure for the mitigation of climate change impacts especially in semi-arid regions such as the Mediterranean.
1 Introduction
United Nations have adopted “ending hunger, achieving food security, improving nutrition, and promoting sustainable agriculture” as one of the most critical Sustainable Development Goals for 2030 (United Nations, 2015) since food security has been a growing concern worldwide. Even though significant progress has been made in addressing the issue, food security remains a challenge, since global food demand is expected to increase up to 98% by 2050 (Elferink and Schierhorn, 2016). As a result, farmers worldwide will need to increase crop production and export high-value agricultural products in order to achieve the objective of global food security.
Furthermore, climate change effects on temperature and precipitation are expected to reduce global agricultural productivity by 4.0% (Springmann et al., 2016). Most of the agricultural products intended for European and Mediterranean markets are grown in arid or semi-arid climatic regions which are being affected by severe water stress (Correa-Cano et al., 2022). As a result, groundwater extraction is increasing, leading to increased energy consumption and carbon footprint (Ayala et al., 2016) as well as global cropland expansion to address the required increases in food production (Zabel et al., 2019).
Food security, efficient use of water resources and sustainable management of agro-ecosystems are tightly interlinked and require holistic, integrated approaches to achieve solutions that minimize the negative feedbacks among them. Within this scope, the nexus approach has been widely used over the past years, especially when identifying the strategies across the water, energy and food domains (Purwanto et al., 2021) and expanded also in other directions, such as land use, soil, waste, climate, economy, ecosystems, health, making this approach more multidimensional (Laspidou et al., 2018). The Water-Ecosystem-Food (WEF) nexus highlights the interlinkages, synergies and trade-offs among these three sectors with the aim of identifying appropriate adaptation and mitigation strategies to address climate change impacts. Implementation of nexus approaches is a complicated procedure, since a series of data and tools that can analyze the inter-dependences among nexus elements are needed (Kaddoura and El Khatib, 2017). For this reason, and in order to help policy makers and stakeholders understand the impacts of agriculture on water, food production and the environment, Correa-Cano et al. (2022) developed a nexus modeling toolkit to evaluate pathways to sustainable agricultural development, combining irrigation, economic and environmental assessment modeling approaches.
A good case in applying the WEF nexus is the sector of avocado production (Becker and Gondhalekar, 2022). Avocado is considered one of the most profitable tropical fruits on the global market, since exports rose by an estimated 11% in 2021, to 2.5 106 tons (FAO, 2022). Avocado production is considered nowadays at risk due to climate change and the high water requirements and footprint of this crop (Fereres, 2012). A series of studies have reported that avocados display higher water footprint than other crops (Novoa et al., 2019). The average annual irrigation needs for avocados extends between 67 cm in Israel (Kalmar and Lahav, 1977) and 89 cm in South Africa (Hoffman and Du Plessis, 1999). For new plantations cultivated in Mediterranean climates, the average daily irrigation needs during summer were adjusted at 4–8 liters/tree for the first year and 80–150 liters/ tree for the fourth year (Lahav and Whiley, 2002). Novoa et al. (2019) showed that avocados presented the highest water footprint (1,400 m3 t−1) among other crops, while Frankowska et al. (2019) reported also a high value of water footprint (824 m3 t−1), rating avocado as a crop with adverse environmental impacts. Furthermore, other studies have emphasized its negative impact on natural resources (Cho et al., 2021) and the associated impacts due to virtual water trading between producing and consuming countries (Caro et al., 2021).
Given the fact that the cultivation of avocados has expanded significantly in recent years, and considering the challenges related with irrigation demands, there is need for the development and adoption of new strategies rooted on the principles of sustainability such as alternative growing systems (Beyer et al., 2021) and application of locally adjusted “best management practices” in avocado cultivation (Beyá-Marshall et al., 2022; Erazo-Mesa et al., 2022). These approaches would have also positive impacts on environmental indicators such as water and nutrient use efficiency. Novel sustainable agricultural approaches and practices should be applied at all scales of agricultural production to address the challenge of long-term food security (Muhie, 2022).
The avocado production of Greece was 12,760 tons in 2021 with a 4.7% annual growth. About 90% of the cultivated area is in western Crete. Since avocado is a dynamic cultivation for the region and the reported environmental impacts of the cultivation could be significant, a WEF nexus study was necessary to identify sustainable agricultural practices. The objectives of this research are (i) to illustrate that agro ecological practices and carbon addition optimizes the WEF nexus of avocado plantations, and (ii) irrigation based on the needs of the plant can reduce substantially irrigation consumption contributing positively to the sustainability of water resources and thus, to the WEF nexus activation.
2 Materials and methods
The experimental design of this research consists of two unique experiments one to address the impacts of soil organic carbon addition to optimize the WEF nexus and the second to measure experimentally the irrigation needs of the plant and thus illustrate how irrigation practices aided by technology can reduce substantially water consumption.
2.1 Sampling campaign and experimental design
The study area is located in the valleys of Keritis and Koiliaris rivers in the northern part of the island of Crete, Greece near the city of Chania (Supplementary Figure S1), mainly cultivated with olive, avocado and citrus crops (Kritikakis et al., 2022). Within the framework of this research, eleven avocado fields owned and managed by distinct farmers were selected to conduct soil sampling. The sampling took place on November 18, 2021. Seven fields were cultivated conventionally (field numbers: 1, 2, 3, 7, 9, 10, 11) and four were cultivated biologically (field numbers: 4, 5, 6, 8). Fields with numbers 1–10 are located in the valley of Keritis and field with number 11 in the valley of Koiliaris river (Supplementary Figure S1). Three soil cores were taken from each field (Α, Β, C) and each core provided 3 to 4 different samples designating different depths (0–10 cm, 10–20 cm, 20–40 cm, 40–60 cm) to a total of 83 soil samples. Each soil sample underwent 39 analyses regarding total chemical concentrations and 37 for extracted elements to a total of 6,020 physico-chemical analyses.
Each soil sample was analyzed for pH, electrical conductivity (EC) (EPA Method 9045D/ ASTM D4972-19), bulk density, water stable aggregates (WSA) (Cambardella and Elliott, 1993), total metals and micronutrients (EPA Method 3051a, EPA Method 6010b), total organic carbon (TOC) (ASTM D6316) and total nitrogen (TN) (multi N/C 2100S, Analytik Jena). In addition, the soil samples underwent the EPA Method 1,312 extraction procedure to determine available concentrations of chloride (Cl), sulfate (SO4), ammonia (N-NH3), nitrate (N-NO3) and phosphate using Ion Chromatography (Dionex IonPacTM AS22 column (4 × 250 mm) & CS12A column (4 × 250 mm) with Suppressed Conductivity detection by Thermo Scientific). Finally, the Synthetic Precipitation Leaching Procedure (SPLP) (EPA Method 1312) was used to determine the leachable metal concentrations.
Soil genomic DNA was extracted from 0.3 g of dry soil using the DNeasy Power Soil kit (Qiagen). The bulk soil was thoroughly homogenized in a mortar with liquid nitrogen before DNA extraction. The quality of DNA was checked by running 2 μL in 2% agarose gel and in a second step its concentration was quantified in a QFX fluorometer using the Qubit dsDNA HS kit. The V4 region of 16S rRNA gene was amplified using the primer pair 515F and 806R. The ITS2 was used for the amplification of fungal communities using the FITS7, ITS4 primer pair. Libraries construction and sequencing, at a minimum depth of 50,000 sequences per sample, was performed by the NOVOGENE UK using the NovaSeq 6,000 platform (Illumina) to get 2 × 250 bp paired-end reads. Primers, adapters and barcodes were removed from raw reads and the remaining paired-end reads were joined into amplicon sequence variants (ASVs) using the DADA2 (v. 1.22) pipeline (Callahan et al., 2016) in R (v. 4.2.1) (R Core Team, 2017). Taxonomy was assigned to the reads by training the naive Bayesian classifier (Wang et al., 2007) against the SILVA database (v. 138.1) (Quast et al., 2013) for bacterial communities and UNITE database for fungal communities and implemented within the DADA2 pipeline. Raw sequences have been deposited in the NCBI database with accession number PRJNA1104275. Alpha diversity of microbial communities was estimated by the Shannon index with the microeco package (Liu et al., 2021). Beta diversity was assessed by Bray–Curtis dissimilarity. PERMANOVA, with 999 randomizations, was performed to assess the statistical significance.
2.2 Estimation of avocado irrigation requirements
The objective of this experiment was to determine the actual irrigation needs of the avocados by specifically focusing on individual avocado trees. An avocado plantation in the Koiliaris river basin was instrumented for this purpose (Field 11 of the previous study). The field instrumentation consisted of NDVI and PRI cameras (for the determination of productivity), a meteorological station (to calculate ET), soil moisture probes located at different soil depths (to estimate soil water storage and percolation to ground water) and flow meters to determine the amount of irrigation (Figure 1). The infrastructure comprises of wireless, autonomous monitoring stations which transmit data from their attached sensors, to a remote database server for storage and analysis. The study was initiated in 2019 and data were collected for the irrigation periods 2020–2023.
A drip irrigation system was installed with the piping surrounding the trunk of the tree at 1 m distance and equipped with 25 drippers (2 L/h capacity). In the first two years the irrigation height was determined empirically by applying common practices based on the knowledge of the farmer. In the third year, to determine more precisely the timing and amount of irrigation, the following rules were adopted:
• Irrigation was applied at such volumes to replenish the moisture deficit of the root zone,
• Water depth was limited to the active root zone since additional water will become practically unavailable to the tree
• Comprehend that the plant roots are very effective instruments in transporting moisture to the plant.
So, the objective of an effective irrigation system was to determine the radius around the tree that the roots extend, use the rate of infiltration of the water to determine the duration of irrigation so the water does not reach below the root level and add sufficient water to replenish the soil moisture deficit caused by ET.
The water budget was estimated as follows:
where is the change of soil water volume over time, is the rate of irrigation, is the amount of precipitation, is the amount of evapotranspiration and is the amount of water percolated below the root zone.
The change in the soil moisture volume was measured from the changes in soil moisture content over time. The volume of irrigation was measured with flowmeters. The amount of evapotranspiration was determined using the Pennman-Montheith equation and the amount of percolated water below the root zone was determined by balancing the hydrologic budget. To confirm the amount of percolation, the HYDRUS-1D model was used to simulate the amount of percolation between irrigation periods. In addition, soil infiltration rate measurements were conducted to estimate the time required by the water to reach below the root zone which for young avocado trees was estimated to be about 50 cm depth.
The HYDRUS-1D model simulates three main processes: water flow, heat and solute transport in unsaturated, partially saturated or completely saturated porous media (Šimůnek et al., 2009). The model numerically solves Richards’ equation to predict water flow and, advection-dispersion and diffusion equations to simulate solute transport in the liquid and gaseous phase, respectively, (Šimůnek et al., 2009; Kanzari et al., 2018). The water flow equation can be considered of single porosity (such as van Genuchten-Mualem), dual-porosity or dual-permeability type flow, with or without hysteresis. The transport equations may include nonlinear nonequilibrium or linear equilibrium reactions which are solved by Galerkin finite element scheme (Šimůnek et al., 2009). The model is driven by meteorological data, soil hydraulic parameters and geometry information in a selected timestep. Meteorological data include time series of precipitation, ET and air temperature, soil hydraulic parameters include the van Genuchten-Mualem parameters (θr, θs, a, n, Ks, and l) and geometry information include length units, soil depth and a number of soil materials and layers (Šimůnek et al., 2009; Saeidi et al., 2023).
3 Results and discussion
3.1 Physico-chemical analysis of soils
A total of 83 soil samples were analyzed for 39 physico-chemical parameters of the bulk soil total concentrations and 37 extractable chemical concentrations. The results are presented in the Supplementary material.
The average concentration of the physico-chemical parameters of the eleven sampled fields are summarized in Table 1. The average values were obtained from all soil depths (0–60 cm) of the samples of each field. The pH was acidic to slightly alkaline and ranged between 4.95 and 7.95 over the sampled fields (Table 1). The EC and the Mn concentrations ranged from 385 to 1,854 μS/cm and from 177 to 476 mg/kg respectively, establishing a significant positive linear correlation (R2 = 0.53). Field 1 showed the highest values of EC (1,854 μS/cm), Cl (155 mg/kg), and SO4 (1,958 mg/kg), used as indicators of salinity, which is one of the factors affecting growth and development of the Avocado crop fruit. On the other hand, Field 11 (located in Koiliaris river basin) presented the lowest average values of EC (385 μS/cm), Cl (19 mg/kg), and SO4 (17 mg/kg). In general, the average Cl and SO4 concentrations ranged between 22–68 mg/kg and 218–898 mg/kg, respectively, in the other fields of Keritis basin.
The texture of the surface soils in Keritis basin was sandy with the sand content ranging between 56.4–69.9%, while the texture of the soil in the field of Koiliaris was silty-clay (39.3% sand). For all samples (except for Field 4), the majority of WSA mass (41.6–82.4%) was contained in the macro-aggregates (>250 μm). The WSA mass contained in the micro-aggregates (53–250 μm) was between 5.1 and 30% and the WSA mass contained in the silt-clay sized micro-aggregates (<53 μm) was between 12.5–33.4%. In the case of Field 4, the majority of WSA mass was distributed in the AC2 (40.7%) and AC1 (39.6%) (Table 1).
All the sampled fields were characterized by low values of N-NH3 (0.18–4.54 mg/kg) except for the field with number 9 which presented the higher value of N-NH3 (70.27 mg/kg). Similar results were also presented for the concentrations of N-NO3 and PO4, with the highest value (at about 170 mg/kg and 59 mg/kg respectively) to be presented in the same field (with number 9) (Table 1).
Average values of TOC and TN ranged between 15 to 29 g/kg and 1.4 to 2.9 g/kg, respectively except for Field 8 which showed the highest values (44 g/kg and 3.4 g/kg), respectively. In this field organic farming was applied. The applied agronomical practices varied between fields and the nutrient contents appeared independent of the cropping system (conventional or organic). Several farmers commonly apply organic amendments either in the form of manure, compost or mulching the undergrowth, however, reliable estimates of organic-C additions could not be established and the interpretation of results were performed based on the TOC of the (average value with depth) fields.
Data were integrated over the whole sampling depth. Similarly, the extractable nutrients were also used to develop similar relationships between availability and soil structure, fertility and biodiversity. The information ascertained the interrelationships of the WEF nexus and how agro-ecological practices can enhance it.
3.2 Microbial communities
From soil variables monitored only EC and pH showed significant correlations with α-diversity of bacteria. Particularly α-diversity decreased with increasing pH from 6 to approximately 8 (Figure 2A). In addition, our findings reveal a peak of α-diversity at pH values close to 6.0, while α-diversity also decreased at lower pH values (Figure 2A). A decline in α-diversity with increasing pH was also observed for fungal communities (Figure 2B). These findings are in line with earlier studies indicating soil pH is one of the most important factors shaping soil microbial communities (Wang et al., 2015; Tan et al., 2020). α-diversity of bacterial communities also showed a slight decreasing trend in response to increasing values of EC. By contrast no significant relationships between α-diversity of bacteria or fungi with SOC, P, TN, N forms or cropping systems were established (Supplementary Figures S2A,B).
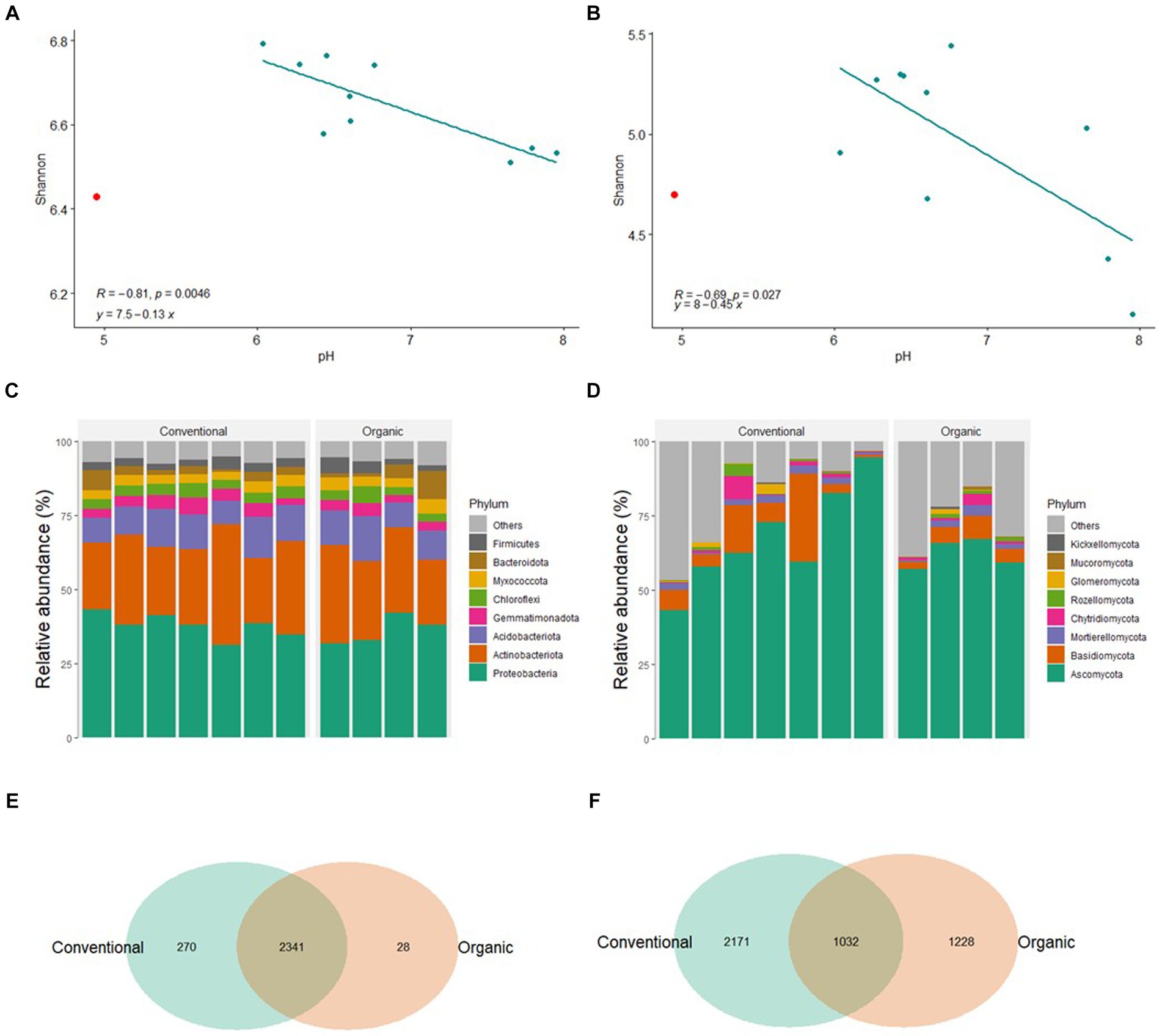
Figure 2. Microbial communities in avocado fields. (A,B) Relationships of soil pH with a diversity (Shannon index) bacterial and fungal communities, respectively. (C,D) Composition of bacterial and fungal communities in different fields aggregated according to the cropping system (conventional vs. organic). (E,F) Unique taxa in bacterial and fungal communities present in the different cropping systems.
Principal Coordinates Analysis (PCoA) of β-diversity (bray-curtis) distances showed that any of the monitored variables (cropping system or SOC content, texture aggregates all separated as low and high) could explain the observed variance (Supplementary Figures S2C,D) implying that explained variance was the cumulative effect of the monitored parameters and/or additional variables not assessed in the present study contributed to that.
Although shifts in the composition of bacterial and fungal communities were observed among avocado fields (Supplementary Figures S2C,D), no significant links were established with environmental variables monitored. In bacterial communities at the level of Phylum, Proteobacteria, Actinobacteriota, and Acidobacteriota accounted up to 75% on average of the relative abundance of bacteria, followed by Gemmatimonadota, Choroflexi, Myxocota, and Bacteroidota which accounted for an additional 15% (Figure 2C). Venn analysis revealed that a small number of taxa was unique in soils separated at low and high TOC content (Supplementary Figures S2E,F). Applying the same analysis to the different cropping systems (organic vs. conventional) showed that fields under conventional practices were characterized by a higher proportion of unique taxa (Figures 2E, F. Compared to a previous study in soils with similar pedo-climatic conditions in bacterial communities but cultivated with tomatoes significant compositional shifts were found in avocado fields (Lilli et al., 2023). Specifically, soils planted with avocado were enriched in Acidobacteriota and Gemmatimonadota and depleted in Firmicutes indicating a potential effect of plant species on soil microbial communities (Burrill et al., 2023). They were dominated by Ascomycota followed by Basidiomycota na Mortierellomycota the fungal communities, (Figure 2D). By contrast in the case of fungi a significant proportion of taxa was unique among sites characterized with low or high SOC (Supplementary Figure S1E).
3.3 Impact of the addition of organic matter on nutrient sequestration, soil structure, soil fertility, and biodiversity
Any management practice that stimulates organic-C return to the soil, increases stabilization of soil carbon, or reduces carbon losses may lead to SOM sequestration. Greater C inputs can occur by enhancing biomass production using fertilizers and/or irrigation, stubble retention, crop rotation, minimum tillage, and by improving soil properties such as pH, cation exchange capacity etc. (Chowdhury et al., 2021).
Figure 3 shows the relationship of the average TOC content of each field with the respective average TN content. Increasing TOC in the soil increases the overall nutrient sequestration of the soil. The slope of the relationship TOC/TN is about 12 which indicates fungal predominance in the soil. High C/N ratio in the soil indicates fungal predominance in microbial community, while low C/N ratio indicates bacterial dominance. A similar relationship in Avocado fields has been also reported by Paranychianakis et al. (2021).
Figure 4 presents the impact of organic-C addition to soil structure. Soil TOC improves soil structure by increasing soil mass distribution into macro-aggregates. Predictably, soil bulk density was decreased while porosity increased. That means increases in soil water retention capacity increase drainage, and in general the activation of microorganisms that create WSA and build the structure of the soil can be observed (Banwart et al., 2013).
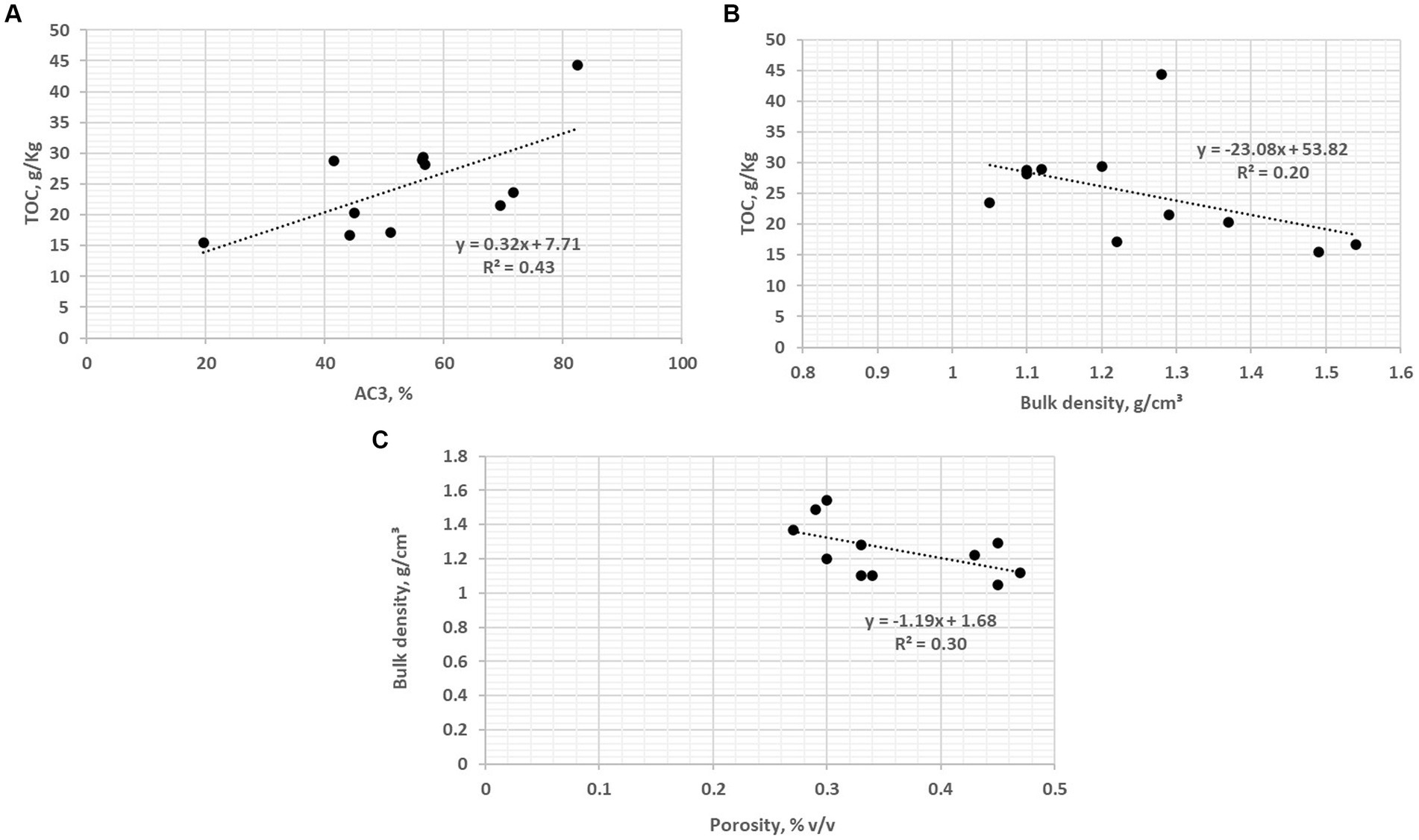
Figure 4. Relationships between soil organic matter and soil structure. (A) Average (in space and depth) TOC versus macro aggregate (>250 μm). (B) TOC and bulk density and (C) bulk density and porosity, relationships for the 11 fields.
Organic matter increases also reflect increases in soil fertility. The indicator used to assess soil fertility was proposed by Quiroga et al. (2006) and is the SOM/(silt-clay) ratio. Quiroga et al. (2006) proposed the threshold of 4.4 for mostly sandy soils suggesting that below this value the functioning of soils decreases. Giannakis et al. (2014) adjusted this value to 5.5 for soils with higher silt-clay and this value has also been adopted in the present study. Figure 5 presents the relationships between SOC and SOC/Silt-clay fertility index and micro and macro-aggregates and bacterial and fungal diversity (Shannon Index) for the 11 fields. Soil fertility increases with increasing organic carbon as does the biodiversity with the improvement of soil structure.
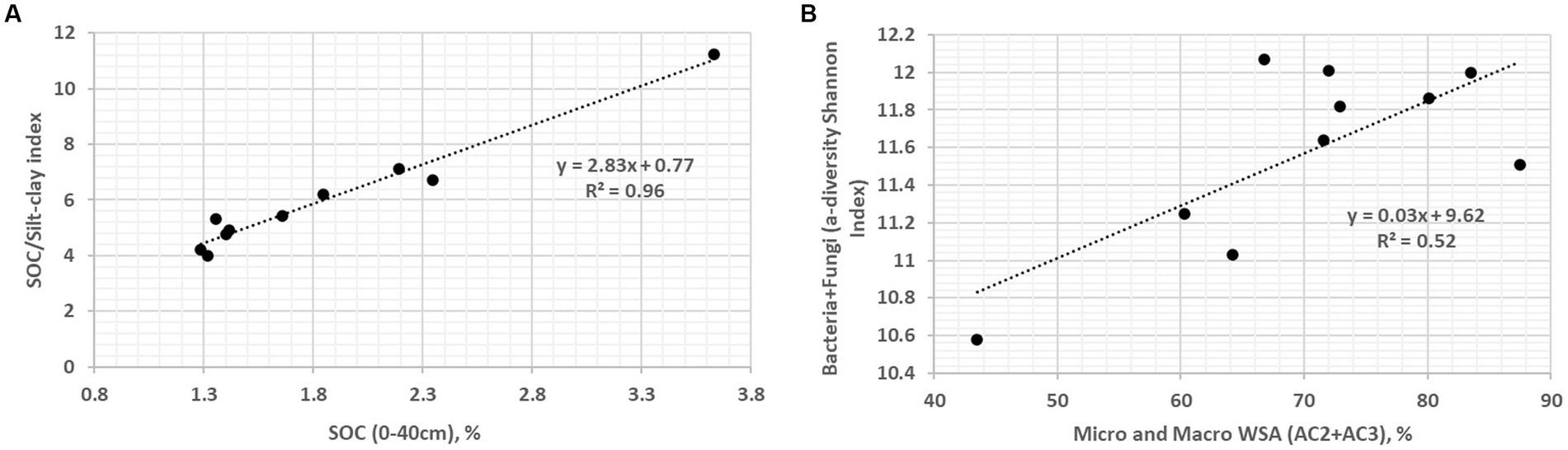
Figure 5. Relationships between (A) SOC and SOC/Silt-clay fertility index, (B) micro and macro aggregates and bacteria and fungi (a-diversity) Shannon Index for the 11 fields.
These results illustrate clearly that agro-ecological practices and particularly those related to organic-C addition stimulate nutrient sequestration into soil, improve soil structure, improve fertility and support higher microbial biodiversity. Improvement in soil structure increases the soil water holding capacity, improving in this way the resilience of agroecosystems to droughts (Rousseva et al., 2017). Agro-ecological practices can address these aspects of the WEF nexus by improving all aspects of the nexus as well as establishing a sustainable environment that can cope with external drivers such as climate change impacts.
3.4 Irrigation study results
The irrigation study was conducted between 2020 and 2023 and the data are presented in Table 2. All years, the farmer was irrigating with drip irrigation with a pipe located 1 m radius from the tree trunk. Table 2 presents the dates of the irrigation season, the number of irrigation events as well as the amount and duration of irrigation. In the first two years, the farmer was irrigating based on his empirical knowledge without on-line access to soil moisture measurements while in the last two years the farmer irrigated with real time on-line access to the soil moisture data as well as the instructions regarding the duration of irrigation based on soil infiltration experiments and hydraulic conductivity measurements. The first two years were considered as baseline data that were compared with the last two years that were based on the actual evapotranspiration needs of the plant. The number of irrigation events ranged from 25 to 34 based on the extent of the irrigation season as well as the summer temperatures that determined the amount of water needed by the plant.
The average duration of irrigation was 8 h for 2020 and 9.2 for 2021 and the average irrigation volume was 11.1 and 8.7 m3/tree/season, respectively. The irrigation volume for 2020 was significantly higher than 2021 because on the 12th of August and 12th of September 2020, the field was over irrigated with a total water volume of 16.1 m3 (0.64 m3/tree) and 46 m3 (1.84 m3/tree) while the typical irrigation volume was 0.25 m3/tree. If we adjust the irrigation volume 2020 by excluding the amount of over irrigation (9.2 m3/tree/season), then the amount of irrigation in 2020 is consistent with the amount of 2021. The irrigation amount per hectare was calculated assuming 24 to 30 trees in a hectare and ranges between 2,000 and 2,800 m3/ha for the years 2020 and 2021.
Before the onset of the 2022 irrigation season, infiltration experiments were conducted and the infiltration rate was determined to be 12.5 cm/h. At the same time, saturated hydraulic conductivity measurements (5–10 and 15–20 cm depth) were 12.7 cm/h. Based on these estimates and assuming the root zone depth of the avocado to be 50 cm, we determined the duration of irrigation to be 3 h.
The average duration of irrigation for 2022 and 2023 was 3 h and the average irrigation volume was 4.4 and 6.2 m3/tree/season, respectively. The typical irrigation volume was 0.18 m3/tree per irrigation event for both years. Assuming 24 to 30 trees in a hectare, the irrigation amount per hectare ranges between 1,000 and 1,900 m3/ha for the years 2022 and 2023.
Table 3 presents the hydrological balance for the years 2020 to 2023. The start of the hydrological budgets for each year was determined to be the 1st of May (start of irrigation on the year 2020) and the end was determined from the last irrigation event. In 2020, each tree was irrigated with 10.39 m3, the amount of precipitation and evapotranspiration was 0.23 and 5.10m3, respectively, and the amount of water percolated below the root zone was calculated by balancing the budget to be 5.53 m3 (53% of the water irrigated). In 2021 the amount of water irrigated per tree was 8.66 m3, the amount of precipitation was 0.14 m3, the amount of evapotranspiration was 4.88m3 and the amount of water percolated below the root zone was estimated to be 4.04 m3 (47% of the water irrigated). Under the reduced irrigation regime in 2022, the volume of irrigation was 4.39 m3, the amount of precipitation and evapotranspiration was 2.31 and 5.37m3, respectively, and the amount of water percolated below the root zone was calculated to be 1.35m3 (31% of the water irrigated). Finally, for 2023, the volume of irrigation was 6.18 m3, the amount of precipitation was 0.94 m3, the amount of evapotranspiration was 5.37 m3 and the amount of percolation 1.99 m3 (32% of irrigation).
It is important to note that the onset of irrigation season changed from 1–19 of May in the 2020 and 2021 seasons to 20–21 of June in the 2022 and 2023 when the farmer was using the soil moisture measurements to determine the need of the plants for irrigation (while most of the farmers in the area start irrigating at the beginning of April). The reason for such a delay regarding the onset of irrigation is that the particular avocado field has a significantly high organic matter (2.5% OC to a depth of 50 cm) and thus has higher soil water retention capacity. These results confirm that agro-ecological practices together can sift the onset of irrigation season by one to two months if combined with soil moisture measurements and appropriate irrigation practices.
The recommended irrigation volume for avocado in Greece ranges between 6,080 to 7,270 m3/ha (Ministry of Agricultural Development, Government Gazette Φ16/6631/1989) and the recommendations of the Region of Crete is 4,410 m3/ha which is significantly higher of the amounts the farmer was using during the years 2020 and 2021 when even though he was irrigating empirically about 9 h per event, the fact that irrigated around the tree, significantly reduced the total volume of irrigation. During the years 2022 and 2023, by reducing the duration of irrigation and determining the frequency based on the soil moisture measurement, the farmer reduced further the total volume of irrigation to 1,000 to 2,000 m3/ha. These values can be further reduced since we are still losing about 32% of the irrigation volume to percolation. The reduced irrigation needs are not only applicable to avocado. Greenhouse and field tomato experiments also showed that the irrigation needs of tomatoes are less than 2,000 m3/ha, which is consistent with the results of this study (Lilli et al., 2023). To confirm that the percolation estimates based on the mass balance budget were correct, we used modeling to simulate the hydrologic budget and evaluate the consistency of the results.
HYDRUS-1D model was used to simulate soil moisture and the hydrologic budget of an avocado tree for July 2022 using an hourly timestep. The soil depth was selected to be 40 cm with the soil profile to be discretized into five nodes and four layers. The soil hydraulic model was selected as a single porosity model (van Genuchten-Mualem) with no hysteresis. The model requires hourly time series of temperature (°C), evapotranspiration and irrigation (in meters). The daily time series of ET was calculated using the Pennman-Monteith equation. The hourly time series of ET were determined by dividing each daily ET by 12, which is the number of hours per day (from 8:00 AM to 8:00 PM) that ET occurs. For the remaining hours, ET was considered to be zero. During the simulation period, irrigation took place on July 1, 7, 13, 17, 21, 25, and 29, 2022. Each day, the avocado trees were irrigated from 1:00 PM to 4:00 PM (3 h) with approximately 192 L per tree. Other parameters required as inputs are bulk density (1.22 g/cm3) and hydraulic conductivity (12.7 cm/h) which were obtained from field measurements.
To simulate the soil moisture and balance the hydrologic budget, the initial water content (theta) and the van Genuchten-Mualem parameters θr, θs and n were calibrated. The simulated soil moisture was compared to the soil moisture probes (Avocado Irrigated 001 and 002) measurements in a depth of 30 cm (Figure 6). Avocado Irrigated 001 and 002 measurements present different variability with the 001 probe’s measurements to be relatively stable (32.2 to 36.8%) and the 002 probe’s measurements to fluctuate from 23.4 to 35.1%. At this point we should note that the amplitude of the soil moisture values of the Avocado Irrigated 001 were adjusted to reflect the amplitude of Avocado Irrigated 002 which are more representative of the soil moisture variability of the field. The HYDRUS-1D model was capable of simulating the soil moisture compared to the Avocado Irrigated 002 measurements. Regarding the water budget derived from the model, in July 2022 the amount of water irrigated was 16.96 cm, the amount of evapotranspiration was 14.49 cm and the amount of water percolated below the root zone and thus lost, was estimated to be 2.47 cm. The percolated water that becomes unavailable for the tree accounts for 15% of the water irrigated initially.
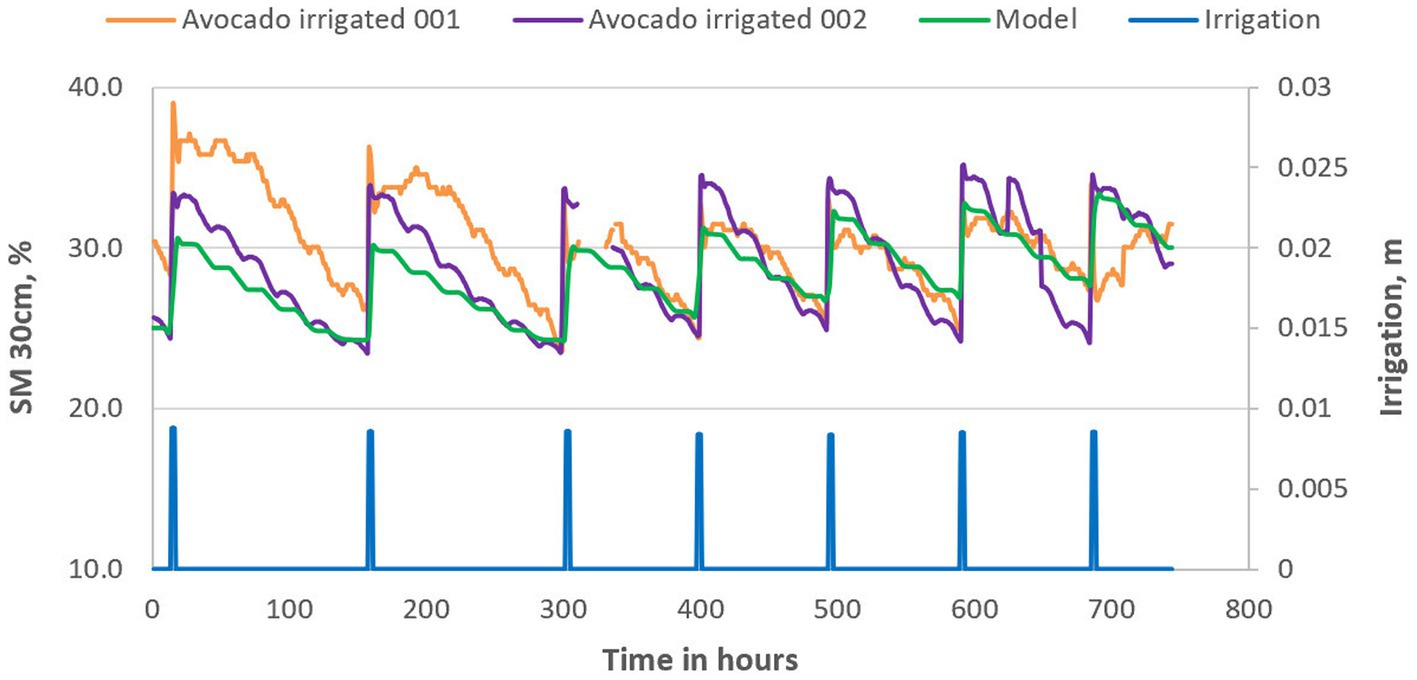
Figure 6. Irrigation events and comparison of field measurements with simulated soil moisture at 30 cm (July 2022).
The modeling results are consistent with the mass balance percolation estimates suggesting that even the 3-h duration of irrigation can be reduced which will reduce the total amount of irrigation water further.
4 Conclusion
Humanity today is faced with multiple environmental challenges including climate change, food and water security and natural disasters that require immediate attention globally and should be addressed simultaneously. To these challenges, if we add the regional environmental problems of the Mediterranean region that derive from the imminent threat of desertification due to land degradation, the need for sustainable management of water and land resources becomes an absolute necessity.
The objective of this work was to conduct studies, collect data and assess the WEF nexus in avocado plantations in systematic way to minimize the environmental footprint and impact of the operation while maximizing the benefits for the farmer and the environment. This study highlighted the following results:
• Agro-ecological practices can improve all aspects of the WEF nexus and establishing a sustainable environment that can cope to external drivers such as climate change impacts. Organic-C additions can stimulate nutrient sequestration, improve soil structure, improve fertility and support higher microbial biodiversity. Improvement in soil structure increases the soil water holding capacity, improving in this way the resilience of agroecosystems to droughts.
• pH value was identified as the most important factor shaping microbial communities in avocado fields while avocado fields appear to be characterized by distinct composition. The functional potential of these communities has to be evaluated.
• Irrigation based on the needs of the plant can reduce significantly the amount of irrigation required for Avocado. The results showed that the actual irrigation needs of avocados in the Mediterranean are less than 2,000 m3/ha which is 75% less than what is recommended and typically used for the water footprint and virtual water trading calculations (Cho et al., 2021).
• The irrigation volume can be further reduced since about 32% of it is still lost to groundwater. These results are not unique to avocado. Lilli et al. (2023) has also shown that the irrigation needs of both greenhouse and field tomatoes are significantly reduced compared with recommended values and are close to this study.
• Soil moisture-based irrigation has been shown to be necessary if we are to reduce significantly water use especially in arid and semi-arid climates. It is important to conduct more studies similar to the one presented in this work and expand them to more cultivations.
Agro-ecological practices and irrigation based on the needs of the plant are effective practices that address all aspects of the WEF nexus and are suggested to be adapted as the primary measure for the mitigation of climate change impacts especially in semi-arid regions such as the Mediterranean.
Data availability statement
The datasets presented in this study can be found in online repositories. The names of the repository/repositories and accession number(s) can be found in the article/Supplementary material.
Author contributions
ML: Conceptualization, Data curation, Formal analysis, Methodology, Resources, Visualization, Writing – original draft. DE: Data curation, Writing – review & editing. EK: Data curation, Formal analysis, Investigation, Writing – review & editing. NP: Data curation, Formal analysis, Investigation, Software, Visualization, Writing – review & editing. NN: Conceptualization, Formal analysis, Funding acquisition, Methodology, Project administration, Supervision, Validation, Writing – review & editing.
Funding
The author(s) declare that financial support was received for the research, authorship, and/or publication of this article. This research was conducted in the framework of the project LENSES- “LEarning and action alliances for NexuS EnvironmentS in an uncertain future,” funded by the European Union’s PRIMA Programme, under grant agreement no 2041.
Conflict of interest
The authors declare that the research was conducted in the absence of any commercial or financial relationships that could be construed as a potential conflict of interest.
Publisher’s note
All claims expressed in this article are solely those of the authors and do not necessarily represent those of their affiliated organizations, or those of the publisher, the editors and the reviewers. Any product that may be evaluated in this article, or claim that may be made by its manufacturer, is not guaranteed or endorsed by the publisher.
Supplementary material
The Supplementary material for this article can be found online at: https://www.frontiersin.org/articles/10.3389/frwa.2024.1412146/full#supplementary-material
References
Ayala, L. M., van Eupen, M., Zhang, G., Perez-Soba, M., Martorano, L. G., Lisboa, L. S., et al. (2016). Impact of agricultural expansion on water footprint in the Amazon under climate change scenarios. Sci. Total Environ. 569-570, 1159–1173. doi: 10.1016/j.scitotenv.2016.06.191
Banwart, S. A., Chorover, J., Gaillardet, J., Sparks, D., White, T., Anderson, S., et al. (2013). Sustaining Earth's critical zone. Basic science and interdisciplinary solutions for global challenges. United Kingdom: The University of Sheffield.
Becker, L., and Gondhalekar, D. (2022). Estimating the water and carbon footprints of growing avocados in the Munich metropolitan region using waste heat as a water-energy-food nexus potential. Front. Sustain. Food Syst. 6:857650. doi: 10.3389/fsufs.2022.857650
Beyá-Marshall, V., Arcos, E., Seguel, O., Galleguillos, M., and Kremer, C. (2022). Optimal irrigation management for avocado (cv. 'Hass') trees by monitoring soil water content and plant water status. Agric. Water Manag. 271:107794. doi: 10.1016/j.agwat.2022.107794
Beyer, C. P., Cuneo, I. F., Alvaro, J. E., and Pedreschi, R. (2021). Evaluation of aerial and root plant growth behavior, water and nutrient use efficiency and carbohydrate dynamics for Hass avocado grown in a soilless and protected growing system. Sci. Hortic. 277:109830. doi: 10.1016/j.scienta.2020.109830
Burrill, H. M., Wang, G., and Bever, J. D. (2023). Rapid differentiation of soil and root microbiomes in response to plant composition and biodiversity in the field. ISME Commun. 3:31. doi: 10.1038/s43705-023-00237-5
Caro, D., Alessandrini, A., Sporchia, F., and Borghesi, S. (2021). Global virtual water trade of avocado. J. Clean. Prod. 285:124917. doi: 10.1016/j.jclepro.2020.124917
Callahan, B., McMurdie, P., Rosen, M., Han, A. W., Johnson, A. J. A., and Holmes, S. P. (2016). DADA2: High-resolution sample inference from Illumina amplicon data. Nat Methods 13, 581–583. doi: 10.1038/nmeth.3869
Cambardella, C. A., and Elliott, E. T., (1993). Carbon and Nitrogen Distribution in Aggregates from Cultivated and Native Grassland Soils. Soil Science Society of America Journal, 57:1071–1076. doi: 10.2136/sssaj1993.03615995005700040032x
Cho, K., Goldstein, B., Gounaridis, D., and Newell, J. P. (2021). Where does your guacamole come from? Detecting deforestation associated with the exports of avocados from Mexico to the United States. J. Environ. Manag. 278:111482. doi: 10.1016/j.jenvman.2020.111482
Chowdhury, S., Bolan, N., Farrell, M., Sarkar, B., Sarker, J. R., Kirkham, M. B., et al. (2021). Chapter two – role of cultural and nutrient management practices in carbon sequestration in agricultural soil. Adv. Agron. 166, 131–196. doi: 10.1016/bs.agron.2020.10.001
Correa-Cano, M. E., Salmoral, S., Rey, D., Knox, J. W., Graves, A., Melo, O., et al. (2022). A novel modelling toolkit for unpacking the water-energy-food-environment (WEFE) nexus of agricultural development. Renew. Sust. Energ. Rev. 159:112182. doi: 10.1016/j.rser.2022.112182
Elferink, M., and Schierhorn, F. (2016). Global demand for food is rising. Can we meet it? Harvard business review. Open Access Library Journal, Vol.11 No.3.
Erazo-Mesa, E., Gómez, E. H., and Sánchez, A. E. (2022). Surface soil water content as an indicator of Hass avocado irrigation scheduling. Agric. Water Manag. 273:107864. doi: 10.1016/j.agwat.2022.107864
FAO . (2022). Major tropical fruits: Preliminary results 2021. Rome. Available at: https://www.fao.org/3/cb9412en/cb9412en.pdf
Fereres, E. (2012). “Fruit trees and vines” in Crop yield response to water. FAO irrigation and drainage paper 66, Rome, Italy. eds. P. Steduto, T. C. Hsiao, E. Fereres, and D. Raes, vol. 10, 300–497.
Frankowska, A., Jeswani, H. K., and Azapagic, A. (2019). Life cycle environmental impacts of fruits consumption in the UK. J. Environ. Manag. 248:109111. doi: 10.1016/j.jenvman.2019.06.012
Giannakis, G. V., Panakoulia, S. K., Nikolaidis, N. P., and Paranychianakis, N. V. (2014). Simulating soil fertility restoration using the CAST model. Procedia Earth Planet. Sci. 10, 325–329. doi: 10.1016/j.proeps.2014.08.027
Hoffman, J. E., and Du Plessis, S. F. (1999). Seasonal water requirements of avocado trees grown under subtropical conditions. Rev. Chapingo Ser. Hortic. 5, 191–194.
Kaddoura, S., and El Khatib, S. (2017). Review of water-energy-food nexus tools to improve the nexus modelling approach for integrated policy making. Environ. Sci. Pol. 77, 114–121. doi: 10.1016/j.envsci.2017.07.007
Kalmar, D., and Lahav, E. (1977). Water requirements of avocado in Israel. I. Tree and soil parameters. Aust. J. Agric. Res. 28, 859–868. doi: 10.1071/AR9770859
Kanzari, S., Nouna, B. B., Mariem, S. B., and Rezig, M. (2018). Hydrus-1D model calibration and validation in various field conditions for simulating water flow and salts transport in a semi-arid region of Tunisia. Sustain. Environ. Res. 28, 350–356. doi: 10.1016/j.serj.2018.10.001
Kritikakis, G., Kokinou, E., Economou, N., Andronikidis, N., Brintakis, J., Daliakopoulos, I. N., et al. (2022). Estimating soil clay content using an Agrogeophysical and Agrogeological approach: a case study in Chania plain. Greece. Water 14:2625. doi: 10.3390/w14172625
Lahav, E., and Whiley, A. H. (2002). “Irrigation and mineral nutrition” in In the avocado: Botany, production and uses. eds. A. W. Whiley, B. Schaffer, and B. N. Wolstenholme (Wallingford, UK: CABI Publishing), 259–297.
Laspidou, C. S., Kofinas, D. T., Mellios, N. K., and Witmer, M. (2018). Modelling the water-energy-food-land use-climate nexus: the nexus tree approach. PRO 2:617. doi: 10.3390/proceedings2110617
Lilli, M. A., Paranychianakis, N. V., Lionoudakis, K., Kritikaki, A., Voutsadaki, S., Saru, M. L., et al. (2023). The impact of sewage-sludge- and olive-mill-waste-derived biochar amendments to tomato cultivation. Sustain. For. 15:3879. doi: 10.3390/su15053879
Liu, W., Ye, T., Jägermeyr, J., Müller, C., Chen, S., Liu, X., et al. (2021). Future climate change significantly alters interannual wheat yield variability over half of harvested areas. Environ. Res. Lett. 16:094045. doi: 10.1088/1748-9326/ac1fbb
Muhie, S. H. (2022). Novel approaches and practices to sustainable agriculture. J. Agric. Food Res. 10:100446. doi: 10.1016/j.jafr.2022.100446
Novoa, V., Ahumada-Rudolph, R., Rojas, O., S’aez, K., de la Barrera, F., and Arumí, J. L. (2019). Understanding agricultural water footprint variability to improve water management in Chile. Sci. Total Environ. 670, 188–199. doi: 10.1016/j.scitotenv.2019.03.127
Paranychianakis, N. V., Giannakis, G. V., Moraetis, D., Tzanakakis, V. A., and Nikolaidis, N. P. (2021). Crop litter has a strong effect on soil organic matter sequestration in semi-arid Environments. Sustainability 13:13278. doi: 10.3390/su132313278
Purwanto, A., Sušnik, J., Suryadi, F. X., and de Fraiture, C. (2021). Water-energy-food nexus: critical review, practical applications, and prospects for future research. Sustainability 13:1919. doi: 10.3390/su13041919
Quast, C., Pruesse, E., Yilmaz, P., Gerken, J., Schweer, T., Yarza, P., et al. (2013). The SILVA ribosomal RNA gene database project: improved data processing and web-based tools. Nucleic Acids Res. 41(Database issue):D590–596. doi: 10.1093/nar/gks1219
Quiroga, A., Funaro, D., Noellemeyer, E., and Peinemann, N. (2006). Barley yield response to soil organic matter and texture in the pampas of Argentina. Soil Tillage Res. 90, 63–68. doi: 10.1016/j.still.2005.08.019
R Core Team (2017). R: A Language and Environment for Statistical Computing. Available at: https://www.R-project.org/
Rousseva, S., Kercheva, M., Shishkov, T., Lair, G. J., Nikolaidis, N. P., Moraetis, D., et al. (2017). Chapter two – soil water characteristics of European SoilTrEC critical zone observatories. Adv Agron. 142, 29–72. doi: 10.1016/bs.agron.2016.10.004
Saeidi, T., Mosaddeghi, M. R., Afyuni, M., Ayoubi, S., and Sauer, D. (2023). Modeling the effect of slope aspect on temporal variation of soil water content and matric potential using different approaches by HYDRUS-1D. Geoderma Reg. 35, e00724–e00718. doi: 10.1016/j.geodrs.2023.e00724
Šimůnek, J., Šejna, M., Saito, H., Sakai, M., and van Genuchten, W. T. (2009). The HYDRUS-1D software package for simulating the one-dimensional movement of water, heat, and multiple solutes in variably-saturated media version 4.08. Riverside, CA: University of California Riverside, 1–296.
Springmann, M., Mason-D’Croz, D., Robinson, S., Garnett, T., Godfray, H. C. J., Gollin, D., et al. (2016). Global and regional health effects of future food production under climate change: a modelling study. Lancet 387, 1937–1946. doi: 10.1016/S0140-6736(15)01156-3
Tan, W., Wang, J., Bai, W., Qi, J., and Chen, W. (2020). Soil bacterial diversity correlates with precipitation and soil pH in long-term maize cropping systems. Sci. Rep. 10:6012. doi: 10.1038/s41598-020-62919-7
United Nations (2015). Transforming our world: The 2030 agenda for sustainable development. New York, NY: United Nations, 35.
Wang, J.-T., Zheng, Y.-M., Hu, H.-W., Zhang, L.-M., Li, J., and He, J.-Z. (2015). Soil pH determines the alpha diversity but not beta diversity of soil fungal community along altitude in a typical Tibetan forest ecosystem. J. Soils Sediments 15, 1224–1232. doi: 10.1007/s11368-015-1070-1
Wang, Q., Garrity, G. M., Tiedje, J. M., and Cole, J. R. (2007). Naive Bayesian classifier for rapid assignment of rRNA sequences into the new bacterial taxonomy. Appl Environ Microbiol 73.
Keywords: irrigation, agro ecological practices, soil fertility, nutrient sequestration, soil structure, biodiversity, modeling
Citation: Lilli MA, Efstathiou D, Koukianaki EA, Paranychianakis N and Nikolaidis NP (2024) Optimizing the water-ecosystem-food nexus of avocado plantations. Front. Water. 6:1412146. doi: 10.3389/frwa.2024.1412146
Edited by:
Barbara Anna Willaarts, International Institute for Applied Systems Analysis (IIASA), AustriaReviewed by:
Dimitrios Malamataris, Institute of Soil and Water Resources (ISWR), GreeceEvangelos Hatzigiannakis, Institute of Soil and Water Resources (ISWR), Greece
Copyright © 2024 Lilli, Efstathiou, Koukianaki, Paranychianakis and Nikolaidis. This is an open-access article distributed under the terms of the Creative Commons Attribution License (CC BY). The use, distribution or reproduction in other forums is permitted, provided the original author(s) and the copyright owner(s) are credited and that the original publication in this journal is cited, in accordance with accepted academic practice. No use, distribution or reproduction is permitted which does not comply with these terms.
*Correspondence: Maria A. Lilli, mlilli@tuc.gr