- 1Department of Chemistry, College of Science, King Saud University, Riyadh, Saudi Arabia
- 2State Key Laboratory of High-Performance Precision Manufacturing, Dalian University of Technology, Dalian, China
- 3Chemistry Department, Faculty of Science, Alexandria University, Alexandria, Egypt
- 4Faculty of Advanced Basic Sciences, Alamein International University, New Alamein, Matrouh Governorate, Egypt
Introduction: Superhydrophobic materials are considered an ideal method for oil-water separation. However, existing oil-water separation methods have the problem of manufacturing complex and toxic chemical reagents. To address the limitation, we proposed a novel approach to sustainable and efficient oil-water separation using a superhydrophobic membrane based on the Bio Tin oxide nanoparticles (Bio-SnO2 NPs).
Methods: The study involves synthesizing Bio-SnO2 NPs from the sunflower leaf extract which was natural and non-toxic and modifying textile fabric with a superhydrophobic coating (S.T.F.). Characterization techniques including SEM, FTIR, and BET analysis are employed to assess the structural and textural properties of the modified membrane.
Results and Discussion: The textile fabric was modified with a superhydrophobic coating (S.T.F.), demonstrating enhanced wettability, oil absorption capacity, and oil-water separation performance. The Bio-SnO2 NPs exhibited crystalline structures with a length of 90 nm and a diameter of 20 nm, as confirmed by SEM analysis. FTIR results revealed characteristic peaks at 3410 cm-1 and 642 cm-1, indicating the presence of hydroxyl group and Sn-O bonds confirming the successful synthesis of Bio-SnO2 NPs. BET analysis showed a substantial specific surface area of 413 m2/g and a pore volume of 0.19 cm3/g, emphasizing the textural properties. The FTIR and SEM techniques were used to study the characteristics of the textile fabric before and after modification with the superhydrophobic coat. The S.T.F. exhibited remarkable superhydrophobicity with a water contact angle of 152° and a water sliding angle of 4°. Absorption capacities for coconut oil, diesel, and hexane were found to be 70.4 g/g, 63.5 g/g, and 49.6 g/g, respectively, with excellent cyclic stability. Separation efficiency for hexane, diesel, and coconut oil was found to be 99.5, 97.1%, and 96.3%, respectively, with excellent cyclic stability. Mechanical stability test revealed superhydrophobicity retention even after an abrasion length of 200 mm. The chemical stability test indicated that the superhydrophobicity was maintained in the pH range of 3-11. Moreover, the flux for hexane, diesel, and coconut oil was 9400 L m−2 h−1, 8800 L m−2 h−1, and 8100 L m−2 h−1, respectively, highlighting the membrane’s efficient oil-water separation capabilities. These results collectively position the developed S.T.F. as a promising and sustainable solution for diverse oil-water separation applications.
1 Introduction
The global challenge of separating oil and water has intensified due to the increasing prevalence of oily wastewater generated by industrial processes and oil spills (Jones et al., 2008; Dong et al., 2022; Wu et al., 2024). Discharging oily wastewater into the environment can have severe ecological consequences, including water contamination, aquatic habitat destruction, oxygen depletion, and even harm to aquatic organisms (Neale et al., 2023; Yamini et al., 2023). Addressing this issue is crucial from both the environmental and economic perspectives, necessitating the development of functional materials capable of efficiently separating the oil and the water. Although various techniques, such as centrifugation separation, demulsifier, functional material absorption, and gravity separation, have been explored for oil-water (O-W) separation, these methods have some limitations of the high cost and the low separation efficiency (Xiao et al., 2009; Khan et al., 2019; Saleh et al., 2022; Sousa et al., 2022). Hence, there is an urgent need for advanced and effective techniques that offer superior performance in this field.
In recent years, biomimetic design of advanced and multifunctional materials has been at the forefront of research work. Superhydrophobic (SH) membranes which are characterized by a water contact angle (WCA) larger than 150° and water sliding angle (WSA) less than 10°, possess the unique ability to repel water droplets while allowing the passage of oil, rendering them ideal for O-W separation (Bai et al., 2019; Kang et al., 2019; Ma et al., 2020). SH surface has demonstrated promising fog collection ability and fluid transportation ability, for example, Yan et al. (2023a) proposed the super-fast fog collector based on the extreme wettability surface was promising to overcome the water crisis; Yan et al. (2023b) developed a superhydrophilic serial cycloid-shaped pattern could enhance water transportation capacity (Mohamed and Abd-El-Nabey, 2022; Mohamed et al., 2023; Yan et al., 2023a,b). Exploring biomimetic designs for extreme wettability materials has unveiled their immense potential in O-W separation, offering benefits such as high separation efficiency, the absence of additional reagents, minimal secondary pollution, low cost, and the smaller required surface areas. Therefore, numerous materials, including metal mesh, film, sponge, fabric, and so on, have been investigated for their superb O-W separation capacity (Zhao et al., 2022; Gupta et al., 2023).
Several methods have been employed for fabricating SH membranes, such as chemical vapor deposition, electrodeposition, spraying, and more (Abd-El-Nabey et al., 2022; Xue et al., 2022; Huang et al., 2023). However, these methods had some problems, such as the high cost, the lengthy processing time, and the requirement for specialized equipment. In this manuscript, we proposed a simple and scalable method for fabricating SH membranes via dip coating. Dip coating was a promising approach for creating SH surfaces because it enabled convenient manipulation of surface composition and structure to achieve desired wetting properties (Zhao et al., 2022; Gupta et al., 2023). Additionally, it facilitated the production of functional coating on a wide range of substrates on a large scale, making it used for the O-W separation.
The key requirements for preparing SH surfaces are twofold: fabricating surface roughness by depositing micro-nano structures and modifying the rough surface with low surface energy materials (Ragheb et al., 2022). Researchers have explored various nanomaterials to enhance surface roughness. However, conventional methods of nanoparticle synthesis involving hazardous chemicals pose environmental risks, necessitating the development of alternative, eco-friendly approaches (Kumar et al., 2014; Daniel et al., 2023). Biogenic synthesis has emerged as an efficient, clean, and environmentally friendly method. Bio tin is a sustainable and versatile material with a wide range of applications. Its unique characteristics, including chemical stability, low toxicity, and moderate electrical conductivity, make it suitable for diverse fields such as energy storage, catalysis, sensing, biomedical, SH coating, and sustainable packaging (Yang et al., 2020; Mishra and Ahmaruzzaman, 2022). The environmentally friendly synthesis methods employed in bio tin production further contribute to its sustainable nature. As research and development in this field continue, bio tin is expected to play a significant role in addressing environmental challenges and promoting sustainable development across various industries. Biogenic synthesis of bio tin NPS utilizes molecules derived from living organisms and biomass waste as substitutes for the reducing agents and stabilizers typically employed in chemical methods. While previous studies have indeed explored the biogenic synthesis of tin oxide nanoparticles for various applications such as antibacterial, antioxidant, and photocatalytic activities, we would like to emphasize the distinctiveness of our methodology and its application in our research. Various studies have explored the biogenic synthesis of tin oxide nanoparticles for a range of applications. Vidhu and Philip (2015) focused on the antibacterial and antioxidant activities of SnO2 nanoparticles synthesized using Saraca indica flower. Meena Kumari and Philip (2015) investigated the synthesis of biogenic SnO2 nanoparticles from ripe pomegranate seed, studying their thermal, rheological, antibacterial, and antioxidant properties. Dong et al. (2007) explored the biogenic synthesis of tubular SnO2 with hierarchical intertextures using glycoprotein which has a potential application in gas sensors, dye-sensitized solar cells, and photocatalysts. Ma et al. (2020) examined the potential of L. acidissima-mediated tin oxide nanoparticles for cervical carcinoma treatment. Lastly, Mohanta and Ahmaruzzaman (2020) investigated the synthesis of SnO2 quantum dots using Allamanda cathartica leaf extract for the removal of bisphenol from aqueous solutions, showcasing their photocatalytic adsorbent properties.
Moreover, fluoro silanes or fluorocarbon molecules have traditionally served as low surface energy materials due to their low surface energy. However, the using of fluorocarbons with long chains poses concerns related to environmental persistence, bioaccumulation, and potential harm to ecosystems (Mohamed et al., 2023). Therefore, designing SH surfaces is a challenge, necessitating the development of inexpensive and eco-friendly methods and materials for fabricating surfaces with low surface energy. Stearic acid is a cheap and environmentally friendly low surface energy material; therefore, it is used as a low surface energy material in our research.
Here, to address the limitation of complex, high-cost, and toxic fabricating oil-water separation method, we proposed a new fabrication method based on the bio tin oxide NPs superhydrophobic coating on textile fabric (T.F.) for efficient O-W separation. Bio tin NPs were synthesized from biomass waste materials (sunflower leaves extract), representing a green and sustainable approach that used natural materials without toxicity. Stearic acid, an inexpensive and environmentally benign substance, was employed as a low surface energy material. The chemical composition, size, and morphology of the prepared bio tin NPS will be thoroughly investigated. Moreover, the wettability, oil absorption capacity, O-W separation performance, mechanical stability, chemical stability, and flux of the prepared superhydrophobic textile fabric will be studied.
2 Experimental
2.1 Materials
The following substances were used in the experiment: n-hexane (Hex.) (98%), sulphuric acid (99%), bromothymol blue (95%), tin chloride dihydrate (98%), sodium hydroxide (97%), and stearic acid (SA) (98.5%) were bought from Chematek company. A commercial diesel (Die.) and coconut oil (Coc.) were used. The spotless TF was bought from the Arabo filter company (Egypt).
2.2 Preparation method
2.2.1 Synthesis of bio-SnO2 NPS
Local residents picked sunflower leaves, dried them in the shade, and then pounded them into a fine powder in a mortar. One hundred milliliters of deionized water and 5 g of powdered sunflower leaves were refluxed at 100°C for 15 min. Filtered extract was used in subsequent studies.
By adding 10 mL of extract dropwise to 30 mL of 0.5 M tin chloride solution at room temperature and rapidly stirring for 10 min, tin oxide nanoparticles were created. The emergence of a light brown precipitate indicates the presence of tin oxide nanoparticles. The samples were centrifuged and submersed in deionized water before being thoroughly cleaned. The sample was annealed for a whole hour at 400°C.
2.2.2 Construction of superhydrophobic textile fabric
For 1 h, a circular T.F. with a 10.5 mm diameter was immersed in a bio-SnO2 NPs solution. The TF was next dried for 2 h at 30°C and then for 1 h at 135°C. The TF is then immersed for 30 min in an ethanolic solution that contains 0.01 M stearic acid. The S.T.F., T.F.@bio-SnO2 @SA, was then dried for 2 h at 30°C and 1 h at 60°C.
2.3 Characterization techniques
A scanning electron microscope (SEM) was employed to analyze the morphology of the bio-SnO2 NPs that were prepared. The Brunauer–Emmett–Teller method was used to analyze the bio SnO2 NPs’ textural properties (BET-Beckman Coulter, SA3100). The composition of the synthesized bio-SnO2 NPs was studied via a Bruker Tensor 37 FTIR Fourier transform infrared spectrophotometer.
2.4 Wettability, absorption capacity, chemical and mechanical stability of the S.T.F.
The wettability of the pristine textile fabric and S.T.F. was measured via measuring the WCA and WSA. The WCA and WSA were determined using a Rame-hart CA instrument (model 190-F2) and 5 μL water droplet, and the provided WCA and WSA results are the average of three tests carried out at various positions on the S.T.F. Using sulfuric acid and sodium hydroxide, the pH of the water droplets was changed. The absorption capacity measurements were conducted following the methodology described in previous research conducted by our group (Beagan et al., 2024).
A mechanical stability assessment was conducted on the S.T.F. through an abrasion test. This involved dragging the prepared S.T.F. across 800-mesh sandpaper at 5 kPa pressure, with measurements of WCA and WSA taken every 5 cm. Additionally, to evaluate its chemical stability, multiple samples were soaked in solutions ranging from pH 1 to 13 for 2 h. The impact of this chemical exposure on WSA and WCA values was then analyzed. The absorption capacity, mechanical, and chemical stability data presented are averages derived from the results of these three tests (Lv et al., 2020).
2.5 Separation performance measurements
Using an oil-water separation assembly and a variety of model oils, including Hex, Die., and Coc., the ability of the developed S.T.F. to separate oil from water was studied. A mixture of water and oil (20 mL each) was poured over the modified membrane while the O-W separation assembly was tilted at an angle of 30°. Bromothymol blue was used to color the water to make observations much clearer. The reported separation efficiency results are the average of three tests on different S.T.F. membranes. The separation efficiency and flux rate were estimated using previously published methodologies (Mohamed and Abd-El-Nabey, 2022).
3 Results and discussion
3.1 Characterization of the bio-SnO2 nanoparticles, T.F. and S.T.F.
3.1.1 Characterization of the bio-SnO2 nanoparticles
Figure 1 illustrates the outcomes of the bio-SnO2 nanoparticles’ characterization through SEM, FTIR, and BET analyses. As shown in Figure 1A, the SEM image revealed that the synthesized Bio-SnO2 exhibited nano-scale dimensions. The average length of the nanoparticles was measured to be 80 nm, indicating their elongated morphology. The diameter of the nanoparticles was about 20 nm, signifying their nanoscale dimensions. As shown in Figure 1B, FTIR analysis shows two prominent peaks, providing insights into the functional groups present on the surface of the bio-SnO2 nanoparticles. The peak at 3,410 cm−1 corresponds to the stretching vibration of hydroxyl groups, suggesting the presence of –OH groups, possibly originating from the bio-reducing agent. The peak at 642 cm−1 may be associated with the characteristic vibrations of Sn–O bonds, indicating the formation of tin oxide. As shown in Figure 1C, BET analysis is employed to determine the textural properties of the bio-SnO2 NPs. The BET-specific surface area was found to be a substantial 413 m2/g, indicating a high surface area available for potential interactions. The significant pore volume of 0.19 cm3/g suggests the presence of pores, which can contribute to enhanced surface roughness.
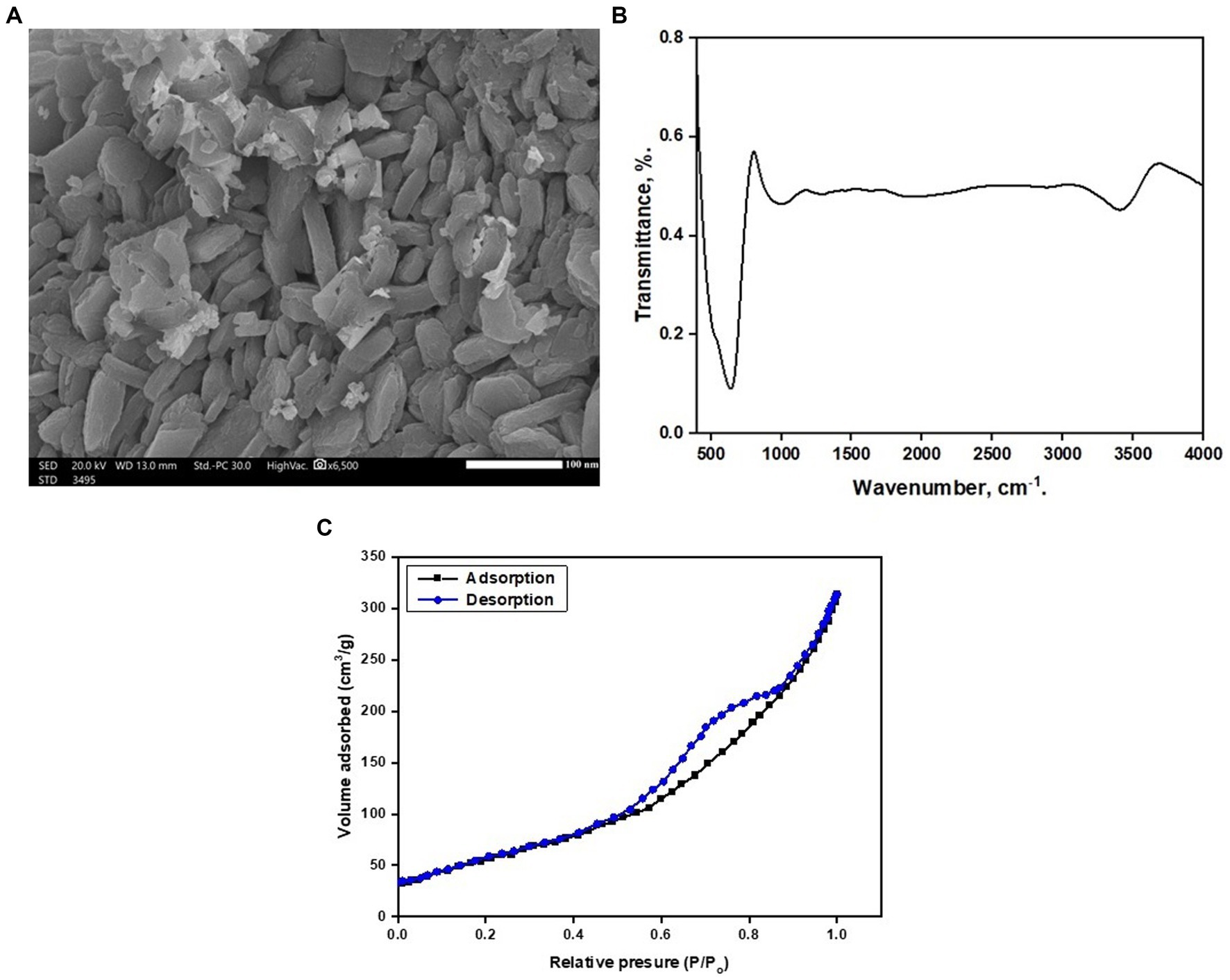
Figure 1. (A) SEM micrograph, (B) FTIR micrograph, and (C) BET isotherm of the prepared bio-SnO2 NPs.
3.1.2 Characterization of the T.F. and S.T.F.
The superhydrophobic property is estimated via both chemical composition and surface morphology. In Figure 2, the FTIR spectra of the T.F. and the S.T.F. are illustrated. The FTIR spectrum of T.F. exhibits several peaks corresponding to various functional groups. Specifically, the peak at 3,431 cm−1 is due to the N–H groups stretching, while peaks at 2,901 cm−1 and 2,952 cm−1 arise from the –CH2-group asymmetric and symmetric stretching, respectively (Abdel-Gaber et al., 2022). The peak at 1,726 cm−1 corresponds to the C=O stretching, and the peak at 1,408 cm−1 is attributed to the bending of C–H. Additionally, peaks at 1,236 cm−1 and 1,008 cm−1 arise from the C–N stretching, and the peak at 864 cm−1 corresponds to the out-of-plane bending of N–H. The spectrum of S.T.F. exhibits the same peaks as T.F., with the addition of a broad peak corresponding to OH stretching at 3309 cm−1 of the low surface energy stearic acid and a peak at 699 cm−1 due to the Sn–O bonds of the synthesized bio-SnO2. The FTIR results confirm the grafting of the T.F. with the prepared bio-SnO2 and the low surface energy material stearic acid.
The morphology of T.F. and S.T.F. was investigated using SEM, as shown in Figure 3. The surface of T.F. appears smooth in the image, while the surface of S.T.F. exhibits significantly enhanced roughness due to the presence of extensive micro/nanostructures of bio-SnO2.
3.2 Wettability and absorption capacity measurements
The wettability results showed that the WCA of TF is close to 0°, which indicated superhydrophilic behavior, where water droplets adhere to the surface, and sliding off is difficult even when the fabric is tilted. The superhydrophilic nature had a strong affinity for water, which might not be desirable for applications involving O-W separation. The S.T.F. demonstrates remarkable SH properties. The measured WCA of 152° ± 0.4° indicated a significant transformation from superhydrophilicity to superhydrophobicity. The water sliding off the surface demonstrated the effectiveness of the Bio-SnO2-based superhydrophobic coating. The low WSA value of 4° ± 0.4° showed the ability of the S.T.F. to repel water efficiently. This low WSA indicated that even a small tilt of the fabric results in water droplets rolling off, emphasizing the robust SH nature.
The absorption capacity of the S.T.F. was assessed for three different oils: Coc., Die., and Hex., as shown in Figure 4. The absorption capacity values of 70.4 g/g for Coc., 63.5 g/g for Die., and 49.6 g/g for Hex. showcase the versatile oil absorption capability of the S.T.F. Earlier research had established a correlation between heightened viscosity and increased oil density, showing a positive impact on oil absorption capacity (Xu et al., 2015; Lv et al., 2018; Shi et al., 2020; Parsaie et al., 2021; Shang et al., 2021). This phenomenon was explained by the delayed release of oils with elevated viscosities and densities from the SH membrane. Consequently, more oil was retained in the porous structure, augmenting the membrane’s absorption capacity. The weightier nature of oils with higher viscosity and density within the identical number of pores in the SH membrane, in comparison to lighter oils, contributed to a superior absorption capacity.
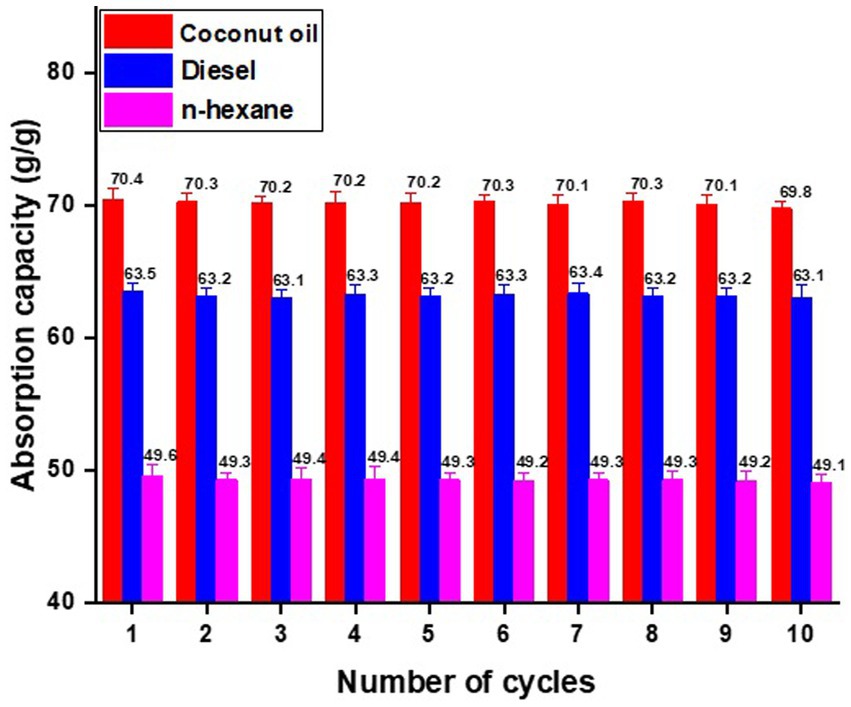
Figure 4. The absorption capacity of the S.T.F. for different O-W combinations with the cycle number.
The absorption capacity of the S.T.F. was evaluated over 10 cycles for each oil. The S.T.F. membrane was sequenced between cycles to desorb the absorbed oil. Remarkably, the absorption capacity showed only a minimal reduction with increasing cycle number, highlighting the mechanical and chemical stability of the S.T.F. This cyclic stability was crucial for real-world applications, which ensured the prolonged effectiveness in the O-W separation. The impressive oil absorption capacities, combined with the stability over multiple cycles, emphasize the practical utility and longevity of the S.T.F. for efficient oil absorption and separation. The S.T.F. exhibited superior absorption capacities compared with previously identified O-W separation materials (Zhou et al., 2017; Xu et al., 2020).
3.3 O-W separation efficiency
Figure 5 depicts the O-W separation efficiency of the developed S.T.F. over 10 cycles of use for three distinct oils: Hex., Die., and Coc. Among these oils tested, Hex. demonstrated the highest separation efficiency at 99.5%, showing the excellent capability of the S.T.F. in efficiently separating Hex. from water. Die. follows with a separation efficiency of 97.1%, and Coc. with 96.3%, indicating robust performance in the different oil types. Notably, the separation efficiency of the S.T.F. exhibited a gradual minimal decrease with an increase in the number of cycles. The sustained high separation efficiency over multiple cycles highlights the coating’s durability and stability, which was critical for the practical applications. The overall high separation efficiencies across multiple cycles confirm the effectiveness of the developed S.T.F. in the O-W separation. The varying separation efficiencies among different oils showed that the S.T.F. could effectively handle oils with distinct properties, making it a versatile solution for diverse O-W mixtures. Table 1 summarizes a comparison of the separation efficiency of the prepared S.T.F. with that of the recent literature studies.
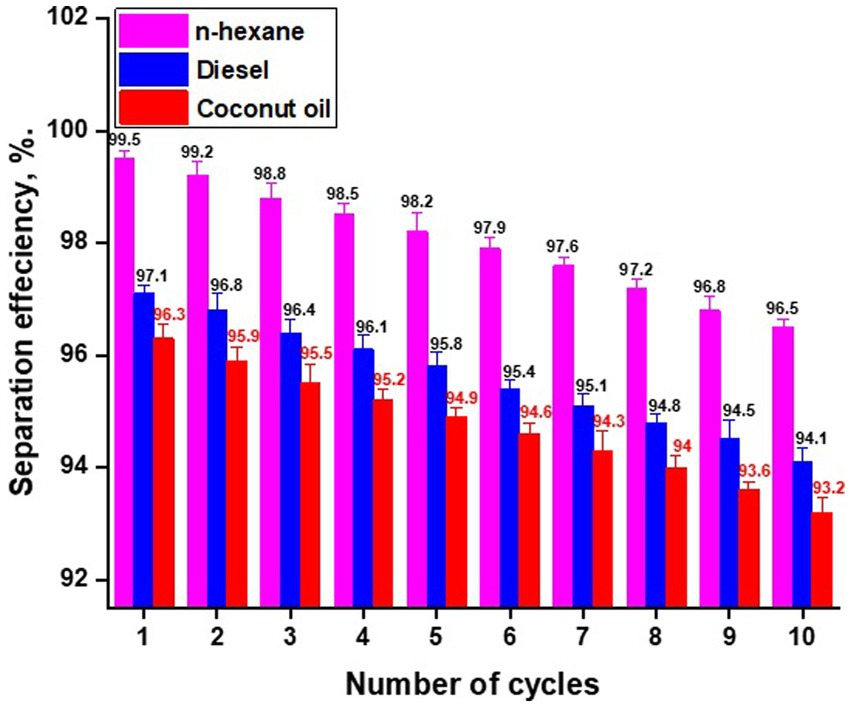
Figure 5. The separation efficiency of the S.T.F. for O-W mixture with the separation cycles number.
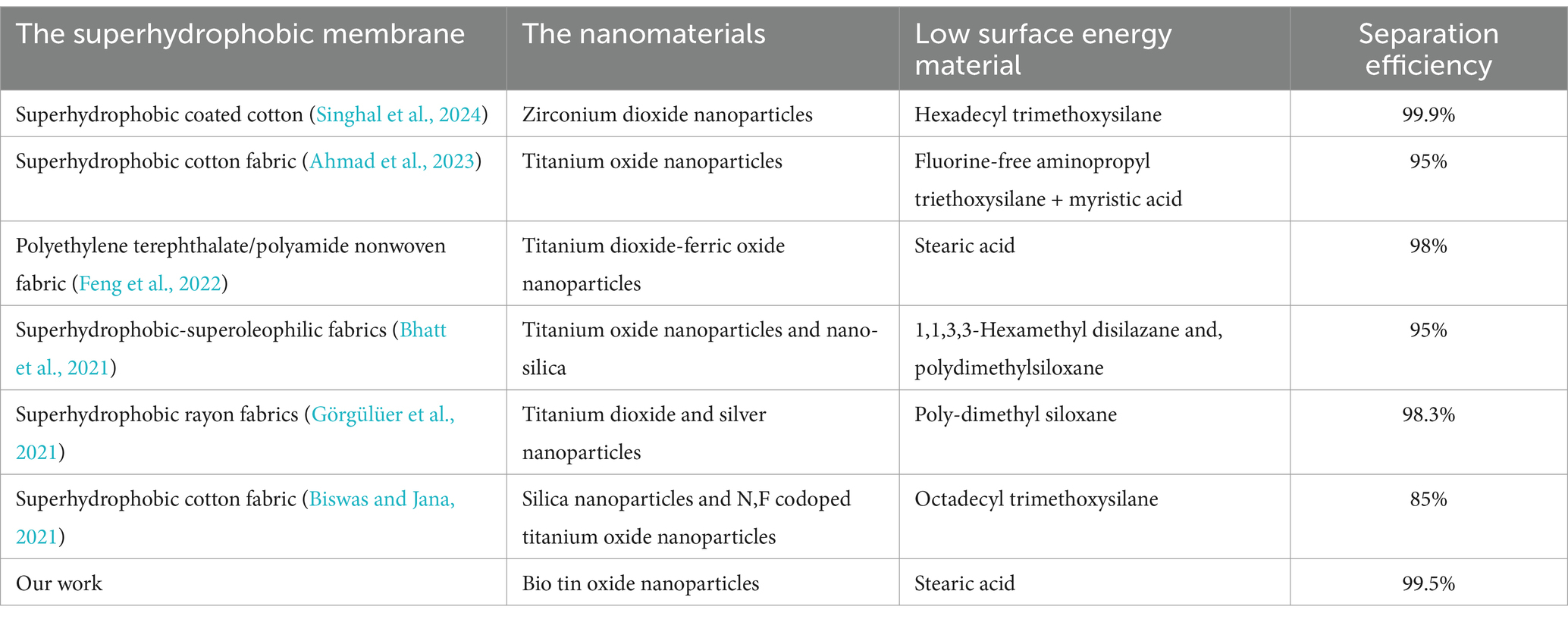
Table 1. A comparison of the separation efficiency of the prepared S.T.F. in our study with that of the recent literature studies.
3.4 Mechanical stability
The study of the mechanical stability of the S.T.F. involved examining the impact of abrasion length on both the WCA and WSA of the membrane, as depicted in Figure 6. The investigation focused on understanding how varying abrasion lengths affect the crucial wetting parameters of the membrane. Figure 6 shows that the S.T.F. exhibits superior mechanical stability as it maintains superhydrophobicity up to an abrasion length of 200 mm. The resistance to changes in wetting angles under abrasion implied practical resilience, making the S.T.F. suitable for applications where mechanical stress was common. The enhanced mechanical stability broadened the potential applications of the S.T.F., making it a promising candidate for use in various industries and environments. This enhanced mechanical stability surpasses values reported in previous studies, highlighting the durability of the developed SH coating (Zhou et al., 2017; Chen and Guo, 2018).
3.5 Chemical stability and flux rate
The chemical stability of the developed S.T.F. was systematically investigated under varying pH conditions. Figure 7 presents the outcomes of these investigations, illustrating the impact of solution pH on the chemical stability of the S.T.F. The study evaluated the S.T.F.’s response to the different pH values, examining how it retained S.P. properties in different pH solutions. Notably, the results showed that the developed S.T.F. maintains its S.P. characteristics within the pH range of 3–11. The well-synthesized SnO2 nanoparticles, characterized by their small size uniform morphology, and high surface area contribute to the high mechanical stability of the superhydrophobic textile fabric. This wide pH stability was significant for applications where the membrane might come into contact with solutions of varying acidity or alkalinity. The chemical stability of the S.T.F. with a wide pH range enhanced the S.T.F.’s versatility for applications in diverse environments, including those with fluctuating pH conditions.
The flux rate is a crucial parameter that influences the efficiency of the O-W separation process. The flux rate of the developed S.T.F. for these three distinct oils, Hex., Die., and Coc., was systematically studied. We measured the flux rates of the S.T.F. for each oil type, providing insights into its efficiency in facilitating the flow of oil through the membrane during the O-W separation process. The results revealed that the flux rate of the S.T.F. was highest for Hex., with a value of 9,400 L m−2 h−1. Diesel follows with a flux rate of 8,800 L m−2 h−1, and coconut oil exhibits a flux rate of 8,100 L m−2 h−1. The varying flux rates showed that the S.T.F. was particularly efficient in separating Hex., demonstrating the highest flux rate among the three oils. The high flux rates for Die. and Coc. further signified the membrane’s versatility in handling different types of oils. The observed flux rates were crucial for applications where the rapid and efficient separation of oil and water is essential, such as in industrial or environmental settings. The variations in flux rates among different oils showed the membrane’s adaptability and effectiveness across various oil types, making it a promising solution for applications requiring rapid and efficient O-W separation. The flux rates exhibited by the S.T.F. surpass those of numerous absorbents reported in the literature (Chen and Guo, 2018; Yan et al., 2018).
4 Conclusion
This work successfully proposed a superhydrophobic textile fabric (S.T.F.) via the bio tin oxide nanoparticles synthesized from sunflower leaf extract, which was low-cost and non-toxic. This S.T.F. demonstrated good characteristics, including high wettability, superior oil absorption capacity, and efficient oil-water separation performance. The scanning electron microscope, SEM, characterization confirmed the nano-scale dimensions of the prepared nanoparticles. Additionally, FTIR analysis was utilized to confirm the structure of the prepared nanoparticles, while BET analysis was employed to measure the surface area. FTIR and SEM techniques were employed to examine the characteristics of the textile fabric both before and after modification with the superhydrophobic coating. Mechanical stability test revealed remarkable durability, with superhydrophobicity retention even after 200 mm abrasion. The S.T.F. exhibited strong chemical stability with a broad pH range, ensuring sustained superhydrophobicity. Additionally, flux rate analyses demonstrated efficient oil flow during oil-water separation, further emphasizing the practicality of the developed membrane. These findings collectively position the S.T.F. as a promising, sustainable, and versatile solution for various oil-water separation applications, contributing to advancements in environmentally friendly technologies.
Data availability statement
The original contributions presented in the study are included in the article/Supplementary material, further inquiries can be directed to the corresponding author.
Author contributions
AB: Conceptualization, Resources, Validation, Writing – review & editing. JL: Writing – review & editing. YL: Writing – review & editing. MM: Conceptualization, Data curation, Formal analysis, Investigation, Methodology, Project administration, Resources, Software, Supervision, Validation, Visualization, Writing – original draft, Writing – review & editing.
Acknowledgments
The authors declare financial support was received for the research, authorship, and/or publication of this article. This research was supported by Researchers Supporting Project number (RSP 2024/R239), King Saud University, Riyadh, Saudi Arabia.
Conflict of interest
The authors declare that the research was conducted in the absence of any commercial or financial relationships that could be construed as a potential conflict of interest.
Publisher’s note
All claims expressed in this article are solely those of the authors and do not necessarily represent those of their affiliated organizations, or those of the publisher, the editors and the reviewers. Any product that may be evaluated in this article, or claim that may be made by its manufacturer, is not guaranteed or endorsed by the publisher.
Supplementary material
The Supplementary material for this article can be found online at: https://www.frontiersin.org/articles/10.3389/frwa.2024.1390739/full#supplementary-material
References
Abdel-Gaber, A. M., Ezzat, A., and Mohamed, M. E. (2022). Fenugreek seed and cape gooseberry leaf extracts as green corrosion inhibitors for steel in the phosphoric acid industry. Sci. Rep. 12:22251. doi: 10.1038/s41598-022-26757-z
Abd-El-Nabey, B. A., Ashour, M., Aly, A. M., and Mohamed, M. E. (2022). Fabrication of robust superhydrophobic nickel films on steel surface with high corrosion resistance, mechanical and chemical stability. J. Eng. Mater. Technol 144, 1–27. doi: 10.1115/1.4052768
Ahmad, N., Rasheed, S., Ahmed, K., Musharraf, S. G., Najam-ul-Haq, M., and Hussain, D. (2023). Facile two-step functionalization of multifunctional superhydrophobic cotton fabric for UV-blocking, self cleaning, antibacterial, and oil-water separation. Sep. Purif. Technol. 306:122626. doi: 10.1016/j.seppur.2022.122626
Bai, X., Shen, Y., Tian, H., Yang, Y., Feng, H., and Li, J. (2019). Facile fabrication of superhydrophobic wood slice for effective water-in-oil emulsion separation. Sep. Purif. Technol. 210, 402–408. doi: 10.1016/j.seppur.2018.08.010
Beagan, A., Chen, C., and Mohamed, M. E. (2024). Bio-copper nanoparticle-based superhydrophobic membranes for sustainable oil/water separation. Water Sci. Technol. 89, 799–810. doi: 10.2166/wst.2024.021
Bhatt, N., Mishra, A., and Goswami, R. (2021). Hydrophobically modified cotton fabric assisted separation of oil-water mixture. Water Sci. Technol. 84, 2830–2841. doi: 10.2166/wst.2021.266
Biswas, A., and Jana, N. R. (2021). Cotton modified with silica nanoparticles, N,F codoped TiO2 nanoparticles, and octadecyltrimethoxysilane for textiles with self-cleaning and visible light-based cleaning properties. ACS Appl. Nano Mater. 4, 877–885. doi: 10.1021/acsanm.0c03282
Chen, L., and Guo, Z. (2018). A facile method to mussel-inspired superhydrophobic thiol-textiles@polydopamine for oil/water separation. Colloids Surf. A 554, 253–260. doi: 10.1016/j.colsurfa.2018.06.059
Daniel, A. I., Keyster, M., and Klein, A. (2023). Biogenic zinc oxide nanoparticles: a viable agricultural tool to control plant pathogenic fungi and its potential effects on soil and plants. Sci. Total Environ. 897:165483. doi: 10.1016/j.scitotenv.2023.165483
Dong, Y., Liu, Y., Hu, C., MacDonald, I. R., and Lu, Y. (2022). Chronic oiling in global oceans. Science 376, 1300–1304. doi: 10.1126/science.abm5940
Dong, Q., Su, H., Zhang, D., Cao, W., and Wang, N. (2007). Biogenic synthesis of tubular SnO2 with hierarchical intertextures by an aqueous technique involving glycoprotein. Langmuir 23, 8108–8113. doi: 10.1021/la062653k
Feng, L., Hou, Y., Hao, Q., Chen, M., Wang, S., Hu, X., et al. (2022). A multi-function textile with pH-induced switch wettability transition for controllable oil−water separation. Text. Res. J. 92, 1357–1368. doi: 10.1177/00405175211056981
Görgülüer, H., Çakıroğlu, B., and Özacar, M. (2021). Ag NPs deposited TiO2 coating material for superhydrophobic, antimicrobial and self-cleaning surface fabrication on fabric. J. Coatings Technol. Res. 18, 569–579. doi: 10.1007/s11998-020-00412-6
Gupta, R., Verma, R., Kango, S., Constantin, A., Kharia, P., Saini, R., et al. (2023). A critical review on recent progress, open challenges, and applications of corrosion-resistant superhydrophobic coating. Mater. Today Commun. 34:105201. doi: 10.1016/j.mtcomm.2022.105201
Huang, X., Sun, M., Shi, X., Shao, J., Jin, M., Liu, W., et al. (2023). Chemical vapor deposition of transparent superhydrophobic anti-icing coatings with tailored polymer nanoarray architecture. Chem. Eng. J. 454:139981:139981. doi: 10.1016/j.cej.2022.139981
Jones, D. M., Head, I. M., Gray, N. D., Adams, J. J., Rowan, A. K., Aitken, C. M., et al. (2008). Crude-oil biodegradation via methanogenesis in subsurface petroleum reservoirs. Nature 451, 176–180. doi: 10.1038/nature06484
Kang, H., Zhao, B., Li, L., and Zhang, J. (2019). Durable superhydrophobic glass wool@polydopamine@PDMS for highly efficient oil/water separation. J. Colloid Interface Sci. 544, 257–265. doi: 10.1016/j.jcis.2019.02.096
Khan, J. A., Al-Kayiem, H. H., Aleem, W., and Saad, A. B. (2019). Influence of alkali-surfactant-polymer flooding on the coalescence and sedimentation of oil/water emulsion in gravity separation. J. Pet. Sci. Eng. 173, 640–649. doi: 10.1016/j.petrol.2018.10.055
Kumar, B., Smita, K., Cumbal, L., and Debut, A. (2014). Biogenic synthesis of iron oxide nanoparticles for 2-arylbenzimidazole fabrication. J. Saudi Chem. Soc. 18, 364–369. doi: 10.1016/j.jscs.2014.01.003
Lv, N., Wang, X., Peng, S., Luo, L., and Zhou, R. (2018). Superhydrophobic/superoleophilic cotton-oil absorbent: preparation and its application in oil/water separation. RSC Adv. 8, 30257–30264. doi: 10.1039/C8RA05420G
Lv, Z., Yu, S., Song, K., Zhou, X., and Yin, X. (2020). Fabrication of a leaf-like superhydrophobic CuO coating on 6061Al with good self-cleaning, mechanical and chemical stability. Ceram. Int. 46, 14872–14883. doi: 10.1016/j.ceramint.2020.03.013
Ma, W., Ding, Y., Zhang, M., Gao, S., Li, Y., Huang, C., et al. (2020). Nature-inspired chemistry toward hierarchical superhydrophobic, antibacterial and biocompatible nanofibrous membranes for effective UV-shielding, self-cleaning and oil-water separation. J. Hazard. Mater. 384:121476. doi: 10.1016/j.jhazmat.2019.121476
Ma, J., Zhao, M., Zhang, C., Wu, X., and Yang, G. (2020). Synthesis of L. acidissima mediated tin oxide nanoparticles for cervical carcinoma treatment in nursing care. J. Drug Deliv Sci. Technol. 57:101745. doi: 10.1016/j.jddst.2020.101745
Meena Kumari, M., and Philip, D. (2015). Synthesis of biogenic SnO2 nanoparticles and evaluation of thermal, rheological, antibacterial and antioxidant activities. Powder Technol. 270, 312–319. doi: 10.1016/j.powtec.2014.10.034
Mishra, S. R., and Ahmaruzzaman, M. (2022). Tin oxide based nanostructured materials: synthesis and potential applications. Nanoscale 14, 1566–1605. doi: 10.1039/d1nr07040a
Mohamed, M. E., and Abd-El-Nabey, B. A. (2022). Fabrication of a biological metal-organic framework based superhydrophobic textile fabric for efficient oil/water separation. Sci. Rep. 12:15483. doi: 10.1038/s41598-022-19816-y
Mohamed, M. E., Adel, O., and Khamis, E. (2023). Fabrication of biochar-based superhydrophobic coating on steel substrate and its UV resistance, anti-scaling, and corrosion resistance performance. Sci. Rep. 13:9453. doi: 10.1038/s41598-023-36589-0
Mohanta, D., and Ahmaruzzaman, M. (2020). Biogenic synthesis of SnO2 quantum dots encapsulated carbon nano flakes: an efficient integrated photocatalytic adsorbent for the removal of bisphenol a from aqueous solution. J. Alloys Compd. 828:154093. doi: 10.1016/j.jallcom.2020.154093
Neale, P. J., Williamson, C. E., Banaszak, A. T., Häder, D. P., Hylander, S., Ossola, R., et al. (2023). The response of aquatic ecosystems to the interactive effects of stratospheric ozone depletion, UV radiation, and climate change. Photochem. Photobiol. Sci. 22, 1093–1127. doi: 10.1007/s43630-023-00370-z
Parsaie, A., Tamsilian, Y., Pordanjani, M. R., Abadshapoori, A. K., and McKay, G. (2021). Novel approach for rapid oil/water separation through superhydrophobic/superoleophilic zinc stearate coated polyurethane sponges. Colloids Surf. A 618:126395. doi: 10.1016/j.colsurfa.2021.126395
Ragheb, D. M., Abdel-Gaber, A. M., Mahgoub, F. M., and Mohamed, M. E. (2022). Eco-friendly method for construction of superhydrophobic graphene-based coating on copper substrate and its corrosion resistance performance. Sci. Rep. 12:17929. doi: 10.1038/s41598-022-22915-5
Saleh, T. A., Satria, M., Moshawer, D. A., and Abdulgader, H. A. (2022). Synthesis of novel smart pH-sensitive modified silica nanoparticles for controllable oil-water separation. Surf. Interfaces 34:102346. doi: 10.1016/j.surfin.2022.102346
Shang, Q., Chen, J., Hu, Y., Yang, X., Hu, L., Liu, C., et al. (2021). Facile fabrication of superhydrophobic cross-linked nanocellulose aerogels for oil-water separation. Polymers 13, 1–14. doi: 10.3390/polym13040625
Shi, M., Huang, R., Qi, W., Su, R., and He, Z. (2020). Synthesis of superhydrophobic and high stable Zr-MOFs for oil-water separation. Colloids Surf. A 602:125102. doi: 10.1016/j.colsurfa.2020.125102
Singhal, H., Pandit, S. K., Kumari, P., and Kumar, A. (2024). In-situ assessment of the performance of oil-water separation by superhydrophobic coated cotton under extreme conditions. Mar. Pollut. Bull. 200:116062. doi: 10.1016/j.marpolbul.2024.116062
Sousa, A. M., Pereira, M. J., and Matos, H. A. (2022). Oil-in-water and water-in-oil emulsions formation and demulsification. J. Pet. Sci. Eng. 210:110041. doi: 10.1016/j.petrol.2021.110041
Vidhu, V. K., and Philip, D. (2015). Biogenic synthesis of SnO2 nanoparticles: evaluation of antibacterial and antioxidant activities. Spectrochim. Acta A 134, 372–379. doi: 10.1016/j.saa.2014.06.131
Wu, S., Yan, D., Chen, Y., and Song, J. (2024). Nano energy self-driven oil/water separator with super-high separation rate. Nano Energy 119:109066. doi: 10.1016/j.nanoen.2023.109066
Xiao, Y., Zhao, J. K., Xu, T., Liu, S. W., and Wang, H. Z. (2009). Review of the technology of electrostatic enhancement of coalescence of water droplets in oil. Petrochemical Equip. 38, 49–53,
Xu, L., Liu, Y., Yuan, X., Wan, J., Wang, L., Pan, H., et al. (2020). One-pot preparation of robust, ultraviolet-proof superhydrophobic cotton fabrics for self-cleaning and oil/water separation. Cellulose 27, 9005–9026. doi: 10.1007/s10570-020-03369-2
Xu, Z., Miyazaki, K., and Hori, T. (2015). Dopamine-induced superhydrophobic melamine foam for oil/water separation. Adv. Mater. Interfaces 2:1500255. doi: 10.1002/admi.201500255
Xue, F., Shi, X., Bai, W., Li, J., Li, Y., Zhu, S., et al. (2022). Enhanced durability and versatile superhydrophobic coatings via facile one-step spraying technique. Colloids Surf. A 640:128411. doi: 10.1016/j.colsurfa.2022.128411
Yamini, V., Shanmugam, V., Rameshpathy, M., Venkatraman, G., Ramanathan, G., al Garalleh, H., et al. (2023). Environmental effects and interaction of nanoparticles on beneficial soil and aquatic microorganisms. Environ. Res. 236:116776. doi: 10.1016/j.envres.2023.116776
Yan, D., Chen, Y., Liu, J., and Song, J. (2023a). Super‐fast fog collector based on self‐driven jet of mini fog droplets. Small 19:e2301745. doi: 10.1002/smll.202301745
Yan, T., Chen, X., Zhang, T., Yu, J., Jiang, X., Hu, W., et al. (2018). A magnetic pH-induced textile fabric with switchable wettability for intelligent oil/water separation. Chem. Eng. J. 347, 52–63. doi: 10.1016/j.cej.2018.04.021
Yan, D., Lu, Y., Liu, J., Chen, Y., Sun, J., and Song, J. (2023b). Enhanced water transportation on a superhydrophilic serial cycloid-shaped pattern. Nanoscale 15, 11473–11481. doi: 10.1039/D3NR02180G
Yang, W. F., Igbari, F., Lou, Y. H., Wang, Z. K., and Liao, L. S. (2020). Tin halide perovskites: progress and challenges. Adv. Energy Mater. 10:1902584. doi: 10.1002/aenm.201902584
Zhao, W., Wang, Y., Han, M., Xu, J., and Tam, K. C. (2022). Surface modification, topographic design and applications of superhydrophobic systems. Chemistry 28:e202202657. doi: 10.1002/chem.202202657
Keywords: oil-water separation, superhydrophobic membrane, biomimetic design, dip coating, biogenic synthesis, sustainable approach
Citation: Beagan A, Lin J, Lu Y and Mohamed ME (2024) Sustainable and efficient oil-water separation using bio tin oxide-based superhydrophobic membrane. Front. Water. 6:1390739. doi: 10.3389/frwa.2024.1390739
Edited by:
Andrea Maranzoni, University of Parma, ItalyReviewed by:
Cleophas Achisa Mecha, Moi University, KenyaShamsuddeen Haladu, Imam Abdulrahman Bin Faisal University, Saudi Arabia
Copyright © 2024 Beagan, Lin, Lu and Mohamed. This is an open-access article distributed under the terms of the Creative Commons Attribution License (CC BY). The use, distribution or reproduction in other forums is permitted, provided the original author(s) and the copyright owner(s) are credited and that the original publication in this journal is cited, in accordance with accepted academic practice. No use, distribution or reproduction is permitted which does not comply with these terms.
*Correspondence: M. E. Mohamed, ZWxzaGFoYXRjaGVtaXN0OTNAZ21haWwuY29t