- 1Department of Water Management, Faculty of Civil Engineering and Geosciences, Delft University of Technology, Delft, Netherlands
- 2Department of Environmental Science and Management, Portland State University, Portland, OR, United States
- 3Environmental Engineering Research Center (EERC), Department of Civil and Environmental Engineering, Faculty of Engineering, Universidad de Los Andes, Bogotá, Colombia
Introduction: Water scarcity is a significant global challenge that frequently manifests as inadequate water supply for domestic purposes. However, domestic water insecurity can occur even in regions where water is naturally abundant. Despite Colombia’s plentiful surface water resources, rural and peri-urban communities often experience limited access to water. Existing water supply systems are frequently susceptible to poor maintenance, particularly in remote areas where much of the infrastructure remains outdated. Consequently, water is often lost through leaks or unintentional non-domestic use. Although a regulatory framework for water usage exists, it does not consistently translate into effective implementation.
Methodology: Based on an extensive survey of approximately 1000 households in four rural and four peri-urban communities in the Valle del Cauca Department, Colombia, we identified the factors underlying inefficient water supply and use. Perceived water use at the household level, based on self-reported time spent on various use types, such as bathing, and water supplied at the system level, was estimated.
Results and discussion: Household size, education level, age and occupation were found to be critical factors influencing end water use and water supply. This not only elucidates why water is supplied and used inefficiently in rural systems (e.g., due to non-domestic use), but also accounts for the variability of perceived water use within peri-urban systems. The water use perceived by households in the rural systems was statistically similar across the rural systems studied and was significantly lower than that in the peri-urban systems. Most rural systems exhibited very low ratios of perceived water use to water supplied, indicating that either water is lost in conveyance or that water is used for non-domestic purposes. Peri-urban users, who perceived to use more water than users in rural areas, were associated with younger and more educated households. Higher education levels were also associated with better financial capacity and technical ability to manage water systems; therefore, peri-urban systems were better managed.
1 Introduction
It is widely known that most people use more water than is strictly necessary for health and well-being (Chukwuma, 2017; Crouch et al., 2021; Hou et al., 2019). Excessive water use puts pressure on natural water sources, water supply systems (WSSs), and wastewater systems (Keshavarzi et al., 2006; Srinivasan et al., 2012), while the consequences could be that rural water suppliers cannot fully meet everyone’s basic water needs. In many countries, particularly those experiencing exacerbated water stress due to extreme weather conditions and persistent socio-economic inequalities, challenges abound (Gonzales and Ajami, 2017; Koop et al., 2019; Rahayu and Rini, 2019; Sant'Ana and Mazzega, 2018). These challenges, notably prevalent in low and middle-income countries, limit access to essential resources such water for domestic purposes and food security.
Water demand and overuse have posed significant challenges to water conservation for decades owing to population growth and increased welfare, increasing the demand for goods and services, and. Climate change exacerbates these challenges. The Intergovernmental Panel on Climate Change (IPCC) emphasizes the importance of demand management to address the increasing vulnerability of freshwater resources (Russell and Fielding, 2010). Climate change, combined with factors such as population growth and shifts in land use, exerts immense pressure on existing water sources worldwide, raising doubts about their capacity to meet the growing water demand. Water authorities are confronted with the daunting task of ensuring adequate water availability amidst droughts, population surges, and anticipated reductions in water supply due to climate change. Consequently, reducing water usage and enhancing water use efficiency have become pressing concerns in numerous regions (Fu and Wu, 2014; Jorgensen et al., 2009).
Ensuring sustainable water management is imperative, with a central focus on reducing water losses from supply systems. This becomes increasingly critical as water scarcity intensifies annually, and water utilities can no longer afford inefficiencies in their supply systems. Addressing water losses poses a myriad of challenges, encompassing various options with their inherent complexities, diverse evaluation criteria, uncertainties, and conflicting objectives and interests among stakeholders from different sectors (Zyoud et al., 2016). For example, in many low- and middle-income countries, most water utilities must deal with leakage and losses (Chawira et al., 2022; Liemberger and Wyatt, 2019), resulting in high levels of so-called Non-Revenue Water (NRW), which is “the difference between the system input volume and billed authorized consumption” (Lambert and Hirner, 2000). Therefore, NRW is often used as an indicator for efficiency within WSSs (Charalambous and Laspidou, 2017; Chawira et al., 2022; Makaya and Hensel, 2014). The NRW in urban areas can have levels up to 50%, while this information is not available for rural areas (Chawira et al., 2022; Liemberger and Wyatt, 2019; Makaya and Hensel, 2014). The NRW indicator for both urban and rural Latin America ranges from 18 to 62%, whereas in urban Colombia, the NRW ranges from 40 to 48% (Liemberger and Wyatt, 2019; SSPD); however, this information is not available for rural areas (Chawira et al., 2022; Liemberger and Wyatt, 2019; Makaya and Hensel, 2014). These percentages might be rooted in various factors such as the location of the WSSs and organizational issues that influence financial sustainability, operation and maintenance.
The peri-urban zone is a transitional area where rural and urban zones converge. Characterized by growing population, expansion in size, and a predominantly non-agricultural labor force (Mittal, 2019), this region signifies a dynamic interface between rural and urban zones. In the peri-urban and rural zones from low- and middle-income countries, water suppliers are frequently organized as water users’ associations (WUA) (Delgado-Serrano et al., 2017), which are mainly volunteer-led community management organizations, including hired staff who may be professional or non-professional. However, WUAs are often unable to afford regular maintenance of the WSSs to ensure efficient performance (Muniz et al., 2020). Sometimes there is even insufficient professional operational and executive personnel serving the WSSs. This situation is marked by weak knowledge and the capacity to apply this knowledge to decisions, which has been defined as water literacy (Dean et al., 2016a; Dean et al., 2016b; McCarroll and Hamann, 2020). This is exacerbated by the fact that most of the employees of WSSs receive low wages or even work without salaries. The distance from urban areas is another challenge which impacts communities and the functionality of the system by WUAs (Muniz et al., 2020), because it hinders providers from operating and maintaining systems. Low-income residents cannot afford the high costs of maintaining the quality services and logistics needed to support the system (Muniz et al., 2020). It could be that the proximity to the city gives the urban managers better access to information and technical support from the institutions compared to rural managers (Barde, 2017; Muniz et al., 2020).
Water system inefficiencies not only occur due to poor infrastructure (leakages and losses), but also how efficiently the water is used by individuals. Socio economic factors, such as income, household size, age, gender, education and occupation are known as contextual factors that influence the behavior of individuals (Mosler and Contzen, 2016; Singha et al., 2022; Steg and Vlek, 2009; Stern, 2000), and, thus, water use within households (Dean et al., 2016a; Dean et al., 2016b; Jorgensen et al., 2009; Millock and Nauges, 2010; Onyenankeya et al., 2021; Russell and Fielding, 2010; Russell and Knoeri, 2020).
Various studies, which link end water use to contextual factors in WSSs have considered individual, household, and WSSs levels in urban, peri-urban, and rural areas. These studies have been carried out both in developed (Abu-Bakar et al., 2021; Benito et al., 2019; Cominola et al., 2023; Mazzoni et al., 2023; Charlotte et al., 2016) and in low- and middle-income countries (Al-Amin et al., 2011; Arouna and Dabbert, 2010; Chukwuma, 2017; Charlotte et al., 2016; Keshavarzi et al., 2006; Polania and Vanessa, 2019; Onyenankeya et al., 2021; Rahayu and Rini, 2019; García and Brown, 2009; Strauch et al., 2021). Abstracting conceptual models of coupled human-urban water systems based on such studies have revealed the feedback between water use at system and household levels, performance of the systems, and the quality of WUA management. For example, the model developed by Muneepeerakul and Anderies (2017) integrated social, economic and environmental factors and proposed a link between the income generated from the use of water, and the extent to which the maintenance cost of water infrastructures could be sustained (Muneepeerakul and Anderies, 2017; Pande and Sivapalan, 2016).
Addressing challenges in rural water supply requires more than just technical solutions or community involvement alone, because water supply systems are shaped by human activities and, in turn, impact human well-being. This necessitates a comprehensive understanding of how water supply systems are integrated into households and communities, as well as how individuals within specific socioeconomic contexts utilize water (Stern, 2014; Workman et al., 2021). However, studies on factors affecting water use usually focus on one level but not on both levels together. Studying water use at both the household and supply system levels is necessary to understand the factors affecting water use and two-way feedback between households and the system (Roobavannan et al., 2018). It is important to consider contextual factors at the household level, and technical approaches at the water system level.
Single disciplines often fail to offer the comprehensive insights needed to fully understand or influence individual and household interactions with water systems. In studies on water demand reduction, research within each discipline has generally approached the topic from its own perspectives. This has resulted in a lack of interdisciplinary, problem-oriented collaboration aimed at developing an integrative understanding of water use at household and the system level (Workman et al., 2021).
Therefore, to fill this gap, this study aims to identify contextual factors that influence water use by employing a multilevel framework household and water supply levels. The case study comprised four rural WSSs and four peri-urban WSSs in Valle del Cauca Department of Colombia. In this study, we used a socio-technical approach that might provide insights into water use at the system level as well. This approach is useful for addressing water use interventions and improving water demand management at both household and system levels.
1.1 Water supply in peri-urban and rural areas: the Colombian context and management institutions
Approximately 23% of Colombia’s population, totaling nearly 12 million inhabitants, reside in rural areas, encompassing both remote rural and peri-urban zones. There are an estimated 11,000 water systems and 9,000 providers, despite it is not representative for all systems it reflects what is the current state of rural water supply systems in the entire country (SIASAR, 2022). Despite these efforts, persistent water shortages often result in demand exceeding supply (Barbier, 2019).
Regardless of the abundance of surface water, access to water for rural communities in Colombia remains limited, with only 40% of the rural population having access to piped water in 2017 (SSPD, 2019; United Nations, 2022). The SDG 6.4.1 indicator is calculated as the change in the ratio of gross economic value added by the water supply sector and the annual withdrawal of water from the public distribution network, expressed in USD/m3 (Hellegers and van Halsema, 2021; United Nations, 2022). A higher value of the SDG indicator signifies greater water supply efficiency. As of 2019, the SDG 6.4.1 value for the entire urban services sector in Colombia was 54.27 USD/m3, approximately 48% below the global average of 112 USD/m3 (United Nations, 2022). Unfortunately, no information regarding water use efficiency in rural areas could be obtained. In contrast, non-revenue water (NRW) estimates for urban areas vary widely, ranging from 7 to 90%. These estimates are measured across distribution networks, from the source to household connections (Liemberger and Wyatt, 2019; SSPD, 2020). In rural settings, the measurement of NRW is often challenging due to the absence of water metering fixtures or these fixtures do not work properly.
The Colombian water management and supply framework operates across various administrative scales, involving numerous institutions such as ministries and municipalities. This complex institutional structure encompasses a range of regulations and programs dedicated to water supply and management. Water User Associations (WUAs) play a crucial role, particularly in peri-urban and rural areas, and are subject to regulation by entities at the municipal, regional, and national scales. In rural contexts, WUAs often organize under regional water users’ associations, exemplified by entities like Fecoser and Aquacol in the Valle del Cauca department.
Within the institutional framework, national regulations such as Law 142 of 1994 (Ministry of Housing, City and Territory - MVCT) and Decree 1076 of 2015 (Ministry of Environment and Sustainable Development- MADS), stipulate that the provision of rural water services is the responsibility of Water User Associations (WUAs). Furthermore, the legal framework mandates that water suppliers ensure the provision of safe water, obtain permits for water intake, and implement a Water Use Efficiency Programme (WUEP). This program comprises activities and objectives proposed by water suppliers to reduce and optimize the utilization of existing water infrastructure.
In Colombia and other low- and middle-income countries, many existing institutional structures, especially national organizations, often fail to adequately address the needs of peri-urban and rural communities. As a result, policies instituted at the national and regional scale frequently lack alignment with practices at the rural scale (Barde, 2017; Molinos-Senante et al., 2019; Muniz et al., 2020).
Three main institutional bottlenecks challenging water supply in rural and peri-urban areas exist. First, the process of decision-making and reaching agreements is complex because of conflicting objectives and interests among stakeholders from different sectors, as well as across various administrative scales and institutions. Second, weaknesses in water literacy persist, particularly due to the low wages or absence of salaries for many employees of WSSs, as well as limited access to information and technical support from institutions. Third, policies implemented at the national and regional levels often lack alignment with rural practices. The design and implementation of policies and projects have exhibited a top-down approach (Barde, 2017; Dean et al., 2016a; Dean et al., 2016b; McCarroll and Hamann, 2020).
Concerns about reducing high water demand, wastage, and overuse have resulted in the need for water use efficiency and water conservation. Exploring, understanding, and predicting water concerns and solutions is a growing area in water management. In the drinking water supply sector, the assessment usually includes either individual or water supply system levels. Sociohydrology integrates these levels and focus on the interactions between water systems and people (Blair and Buytaert, 2016; Roobavannan et al., 2018; Sanderson et al., 2017).
2 Materials and methods
In this study, we assessed the drinking water supply system using such a socio-hydrology approach to determine which factors influence water use at individual and system levels simultaneously and their impact on water efficiency.
To understand how, and how much, water is used, it can be estimated at three levels (Table 1). A previous study in the Valle del Cauca department considered water use at household and catchment levels (García and Brown, 2009). In the present study we examined both water users and water supply systems to determine the efficiency of water use as perceived by the end users. We used the household and water supply levels. People can increase their water use efficiency through “curtailment” habits that reduce water use such as taking shorter showers, and “efficiency” measures by purchasing saving fixtures and storage appliances (Attari, 2014; Russell and Fielding, 2010). At the system level, we considered two sub-levels to define water system efficiency. First, we used the percentage of water losses between the intake and the storage tank as a measure of efficiency at supply system level (see Figure 1). We used the water supplied (measured at the storage tank) and water used at the household level in liters per person per day (lpcd) to determine household level system efficiency.
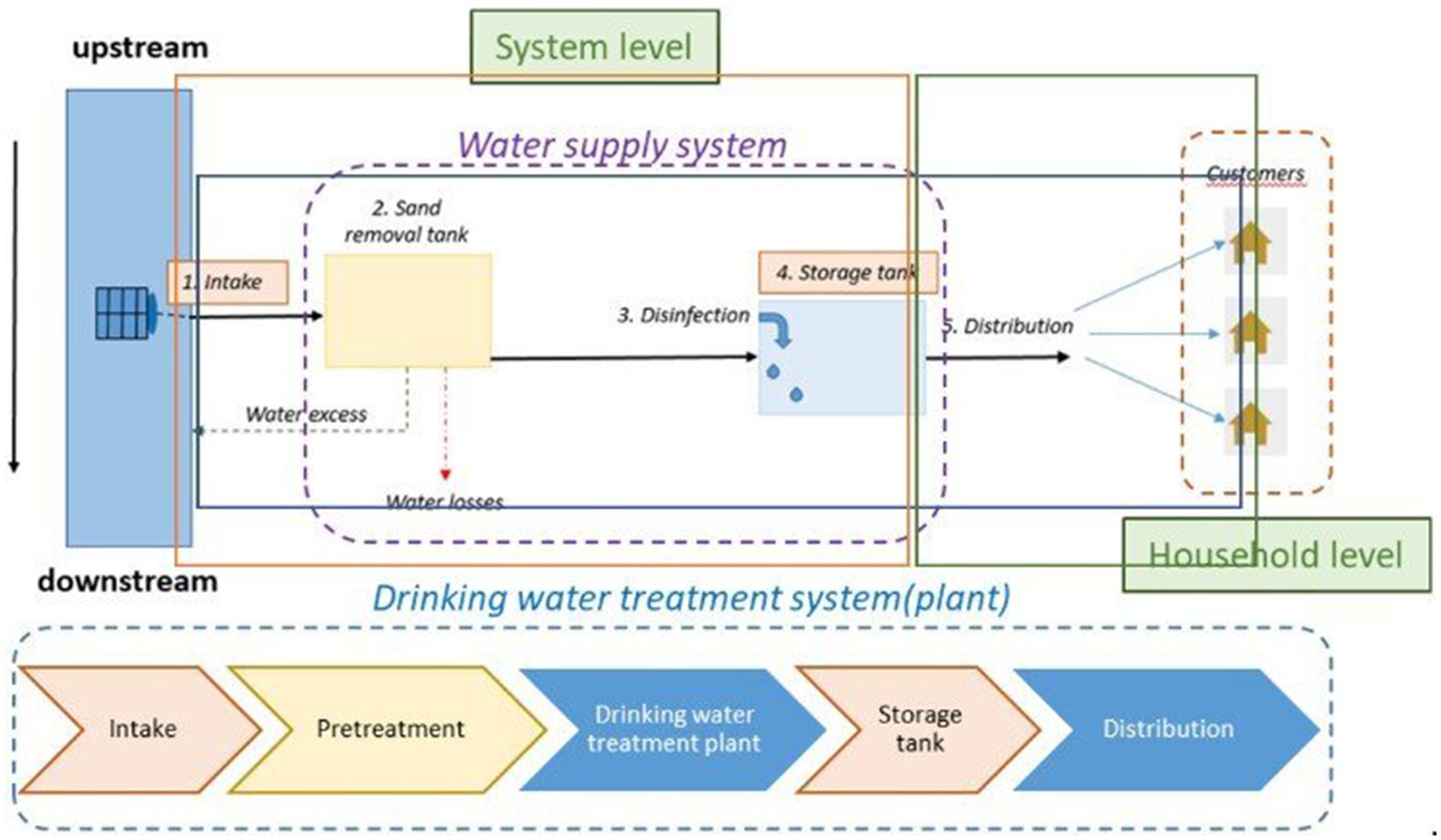
Figure 1. Schematic of a typical water supply and drinking water system. Water use efficiency is defined at two levels: distribution to household level (green) and supply system level (orange).
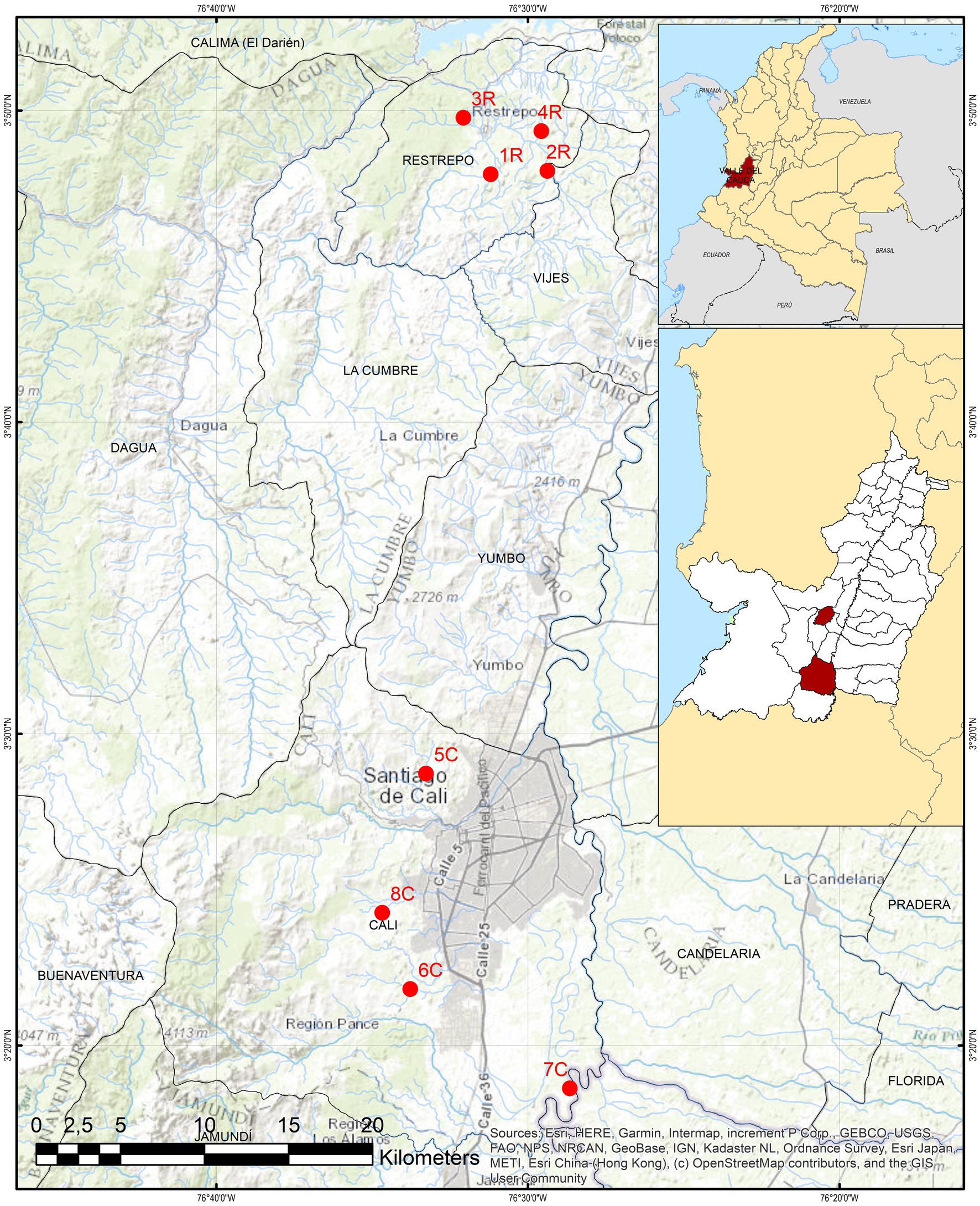
Figure 2. Location of Valle del Cauca department in Colombia, municipalities, and area of case study. The right panel shows the locations of the WSSs.
2.1 Case study
2.1.1 Criteria for selection case study
For the present study, eight (8) locations in Colombia at peri-urban and rural scale were selected based on WSSs heterogeneity, different SEC characteristics, climatic conditions, presence of formal WUAs and sizes of WSSs. In addition, the selection criteria included: (1) interviewer security; (2) the willingness of stakeholders to participate; (3) accessibility to the location (e.g., distance from main cities, rural road conditions); and (4) whether the WSSs were in operation. The locations that were selected were eight WSSs in the rural zone of Restrepo and the peri-urban zone of Cali, located in the Valle del Cauca Department, close to the Pacific Ocean in the south-eastern region of Colombia (Figure 2) (Metabolism of Cities - Data Hub, 2023; NASA Shuttle Radar Topography Mission (SRTM), 2013).
The institutional structure regarding WSSs consists of seven main institutions at the department, municipal, and rural scales (Figure 3). All WUA belong either to Fecoser (Community Aqueducts Federation of Valle del Cauca) or Aquacol (Association of Community Organizations Providing Water and Sanitation Services in Colombia) that are active in Restrepo and the peri-urban zone of Cali, respectively.
In Restrepo, most of the WUA emerged in the 1980s when coffee production was one of the most important economic sectors and the inhabitants were mainly coffee farmers. According to Fecoser,1 the National Federation of Coffee Growers of Colombia (NFCG) constructed the water supply infrastructure, consisting of an intake and a sand removal pre-treatment tank (see Figure 1). Since then, water infrastructure has been managed and operated by WUAs, without or with the Government’s economic or technical support or planning. Over time, the growing population has increased domestic water demand, and the use of water for irrigation, cattle, and pig farming has constrained the water supply and has led to unequal access between domestic and other uses.
In peri-urban zones, WUAs originated during the 1960s and 1990s, and WSSs were constructed, and designed by the environmental, and health authorities, a local university and municipalities. Since then, these areas have expanded. The growing population in Cali, partly because of violence that has displaced people from remote areas and from other departments (Urrea and Canelo, 2017; Vergara et al., 2020), has increased the migration from urban to peri-urban zones.
The systems tap either surface or groundwater sources (Table 2) and type of treatment for drinking water varies depending on the water source. Additionally, seven of these systems are divided into sectors in which the systems intermittently distribute water to users (Charalambous and Laspidou, 2017; Sione, 2021).
2.2 Research approach
2.2.1 Field campaign
A field campaign was conducted to obtain self-reported patterns of water use and characteristics of fixtures and appliances used by households, and data of water flows at the system level to estimate end water use and water supply in the WSSs. These WSSs were further classified as peri-urban and rural based on its distances from the capital city of the department, Cali.
The field campaign involved two methodologies, social research and technical measurements (Figure 4). To collect information about the SEC, we used online and face-to-face fieldwork and social mapping methods. As a starting point, meetings and workshops with local WUAs and staff from WSSs were carried out online (due to the COVID-19 pandemic), assisted by a person from Restrepo, who collaborated on the project at the location. We contacted water leaders, using a smartphone or laptop and online meeting tools, such as WhatsApp (Meta Platforms, Inc., 2021) Zoom (Zoom Video Communications, Inc., 2021) and Teams (Microsoft, 2021), depending on the internet connection. Online invitations were sent through email in advance to the participants, including the aim and program of the meeting and the request to confirm their presence. Participants signed an “informed consent” before the meeting. The groups per meeting were between three and ten people, facilitating the participation of all attendees, and stimulating them to pose questions and make suggestions.
2.2.2 Data collection
2.2.2.1 Criteria for household selection
Data collection involved an inventory of water users and utilities, the number of dwellings and their location, and, to understand the water-use habits of domestic users, questionnaires were designed. Households were randomly selected covering all sectors at each site. A sector is a subarea that belongs to the total area where the water supply system is located. One adult per family was interviewed, and only adults (above the age of 18) were considered to conduct the interviews. The households were informed in advance by the manager of each water users association. Data were acquired from a questionnaire survey focusing on SEC and water use. The water bill number of the household’s owners was used to randomly select interviewees. For the WSSs that do not bill, a list of customers was used. For “self-reporting” we did not ask participants to keep records, we asked for their perceptions by responding the questionnaire items.
Data were collected between November 2020 and December 2021. During the first stage (November 2020 – April 2021) interviews and workshops were developed online with the local village leaders from the WUAs, to collect information on the SEC context and features of the WSS of each village. During the interviews and workshops, the purpose of the study was explained and information about the number of water users per system, the type of water treatment plant, the main water uses and economic sectors in the villages, and how WSSs were established was collected.
We employed a cross-sectional survey, which is a widely recognized approach for collecting self-reported information regarding perceptions, attitudes, beliefs, and behaviors related to water usage (Gifford, 2016; Haslam and McGarty, 2014). Therefore, we asked the participants directly without making records during longer period of time. A total of 965 rural and peri-urban households were sampled, and the rate of response was 96%. The sample size represented approximately 20% of domestic water users from the each of WSSs located in the peri-urban zone of Cali and rural Restrepo. The sample size was determined using the “G*power” software, which computes the sample size and statistical power. In this study, we specified a small effect size (f2 = 0.07), significance level (α) of 0.05, and statistical power of 0.9 (Erdfelder et al., 2009). Following the removal of missing and erroneous data, the final sample size was 965 households. For data management and analysis, the questionnaire was translated from Spanish to English, questions were renamed, and reliable coding was established.
The second step involved (physically) visiting eight WSSs to attain official records, observing existing water supply infrastructure, and interviewing water suppliers. Teams were organized to measure water flows in the facilities of the WSSs and to conduct household surveys (Table 2). During the rainy season (May–December 2021), face-to-face surveys were conducted with adult members of the households and primary data were gathered that included the SEC, self-reported water use habits and end water uses of the individual participants. The online survey software Qualtrics XM was used to conduct the surveys (Qualtrics, 2021).
Volumetric methods were used to measure water flows at the utilities. Water flow measurements at the water source and intake were measured assuming a constant flow; the outflow measurements were taken in the storage tank–hourly for 24 h, except for the “2R” WSS (Table 2), where it was not possible to take water flow measurements because there was no “valve” to partially interrupt the flow of water and this would imply cutting off the water supply for users.
2.2.3 Estimation of end water use perceived at the household level based on self-reports
To estimate water used by domestic appliances and fixtures several alternative methods exist (Abu-Bakar et al., 2021; Charlotte et al., 2016), such as water metering (Polania and Vanessa, 2019), data loggers and sensors (Abu-Bakar et al., 2021), using water bills or census (Attari, 2014; U.S. Geological Survey, 2005), and asking water users about their water use habits and type of appliances and fixtures at home. In the latter method participants report their perceptions of end water use (Abu-Bakar et al., 2021; Attari, 2014; Chacón et al., 2011; Mourad et al., 2011). Not all WSSs had water meter fixtures, and few had metering at the household level. Therefore, questionnaires and water flow standards for conventional and efficient fixtures and appliances from literature were used to estimate ‘perceived’ end water use. We assumed individuals taking traditional showers using a bucket when they do not have a showerhead. We determined the end water use of domestic water use by considering only indoor uses and not considering leakages.
The questionnaire comprised closed-ended questions pertaining to socio-economic factors, types of appliances, and water habits. Detailed information regarding these questions can be found in the codebook (see Appendix 1), which was adapted from previous studies (Attari, 2014; Dean et al., 2016a; Dean et al., 2016b; Jorgensen et al., 2009; Manouseli et al., 2018; Newton and Meyer, 2012), and the frequency per day or week for each water use. A Likert scale (1–5) was used to collect the responses for categorical variables (Edmondson, 2005). Water flow values for appliances and fixtures were selected, and water use per person per day for specific uses, such as cooking and cleaning the house, and with and without water-saving appliances and fixtures were collected based on literature (Attari, 2014; Crouch et al., 2021; García and Brown, 2009; Jorge et al., 2015; Kunitsuka, 2014; Restrepo Tarquino, 2010; Singh and Turkiya, 2013; USGS as cited in BID, 2014).
Estimation of perceived end water use per user was then calculated based on the equations 1–8. Similar surveys and equations have been used earlier to calculate water use (Mourad et al., 2011). For example, the daily amount of water used for a shower per person was calculated by multiplying the number of self-reported showers of the respondent by the estimated water flow from the shower and the length of the shower. It was assumed that the shower was used even when the service water was not continuous. It is then multiplied this number by the number of people in the household, assuming that household members tend to behave similarly. Most respondents did not respond to the question about the frequency of flushing toilets, because they considered it to be private information. Estimating other uses also proved to be difficult. Often, there were older or non-standard fixtures and appliances within the house. Therefore, we assumed that toilets were flushed three times per day per person (Sant'Ana and Mazzega, 2018), and the following lengths of tap opening were considered: one minute for handwashing and teeth-brushing, and three minutes for dishwashing. The frequency of each use was collected through the survey.
2.2.4 Water supply at system level
The WSSs in our case study provide water through small dams and settling ponds, connected to community water tanks, located upstream close to the intake (see Figure 1). Seven of these WSSs use gravity to transport the (treated) surface water to the households, while only the “7C” supply relies on pumping, being dependent on groundwater. At least in three of the systems (“2R,” “6C,” “7C”), pipes and storage tanks between the intake and the system, and water flux meters have fallen into disrepair.
The water supply flows were estimated based on the inflow and water level measurements of the storage tanks. The inflow of the storage tank (Qiin) and water levels (Ii) were measured every hour for 24 h. The changes in storages (∆Si) were obtained from Equation 5 and the hourly outflows (Qout) were calculated using the water balance (Equation 6). Finally, total outflows, Qtotal, for 24 h were obtained as the sum of all hourly outflows. Water supplied per person (W) in each WSSs was then calculated by dividing the total outflow Qtotal by the people (N) supplied per day (Equation 7).
The water losses were considered leakages during the transmission and distribution of water, and leakages and spills at utility storage tanks (Charalambous and Laspidou, 2017; Lambert and Hirner, 2000). The percentage of water losses was estimated by comparing intake and outflow (see Figure 1, Equation 8). The information of leakages was not available for whole systems due to barriers of access to the facilities, and other difficulties such as with the “2R.” Water loss was estimated for each water supply system, not per household.
2.2.5 Analysis method
To explore the associations among end water use, water supply and SEC factors, we used descriptive statistics. Firstly, we examined the dispersion of estimated end water use, using the coefficient of variation (CV) (Doane and Seward, 2013; Soetewey, 2020). As part of the descriptive characteristics, the correlogram to study the relationships between these variables was used (Soetewey, 2020). Analysis of variance (one – way ANOVA) was conducted to compare the means of end water use perceived by users between peri- urban and rural zones and among the WSSs. Further, it was tested whether the means of end water use were significantly different in the various WSSs. To identify which groups were responsible for the differences, a post hoc comparison was used (Creswell, 2014; Doane and Seward, 2013; Patil, 2021; Soetewey, 2020) and for the statistical analysis, R software version 4.2.3 was used (R Core Team, 2021) and the statistical packages (Patil, 2021; Revelle, 2022).
2.2.6 Limitations
We gathered data through a survey administered during interviews. We did not furnish households with a form to be completed on a weekly basis. Water usage for non-domestic purposes was not recorded in the questionnaires during field data collection.
We faced technical and logistic limitations in estimating the water balance due to the remote locations of some of the WSSs. Some systems still use handmade designs and the usual standards are often not applied. The validity of the results is also limited by insufficient historical measurements at the system level, such as intake inflow, leakage and losses. Metering fixtures were not installed at the household level. Therefore, estimated perceived water use serves as an approximation of the water used at the household level in the studied systems.
In the case of 6C, the water system operated intermittently, meaning that users did not have continuous access to water; instead, water was supplied in shifts. Additionally, in the case of 7C, the physical configuration of the system introduced limitations accurately measuring the volume of water. The pumping system operates intermittently, and there is no macro flow meter. Consequently, measurements were taken only when the pump was operational.
We exclusively assessed the water loss from the intake to the water storage tank. However, we did not assess losses storage tanks to households. In addition, we did not account for other commercial losses, including meter inaccuracies and unauthorized consumption.
3 Results
Table 2 presents the characteristics of the selected WSSs, number of households and sectors, and the heterogeneity of these systems, such as the type of water source, and type of treatment.
In three out of the eight case studies, technical and financial capacities were strong, whereas in the remaining five, these aspects were weak. According to our interviews, water managers identified challenges in water supply arising from conflicts among various water uses, including domestic, agricultural, livestock, and tourism. Additionally, low water prices pose an obstacle to the sustainability of the system, and water sources are depleting because of urbanization.
3.1 Perceived water use at household level
Based on the self-reported habits and water use estimates, the overall average water use for domestic indoor use at the household level was calculated to be approximately 253 L per person per day (lpcd) (Figure 5A). The range of values varied from a minimum of 39 lpcd to a maximum of 1016 lpcd, with 75% of water users consuming less than 303 lpcd. The coefficient of variation (CV) for water use was 0.4, indicating relatively homogeneous estimates of end water use.
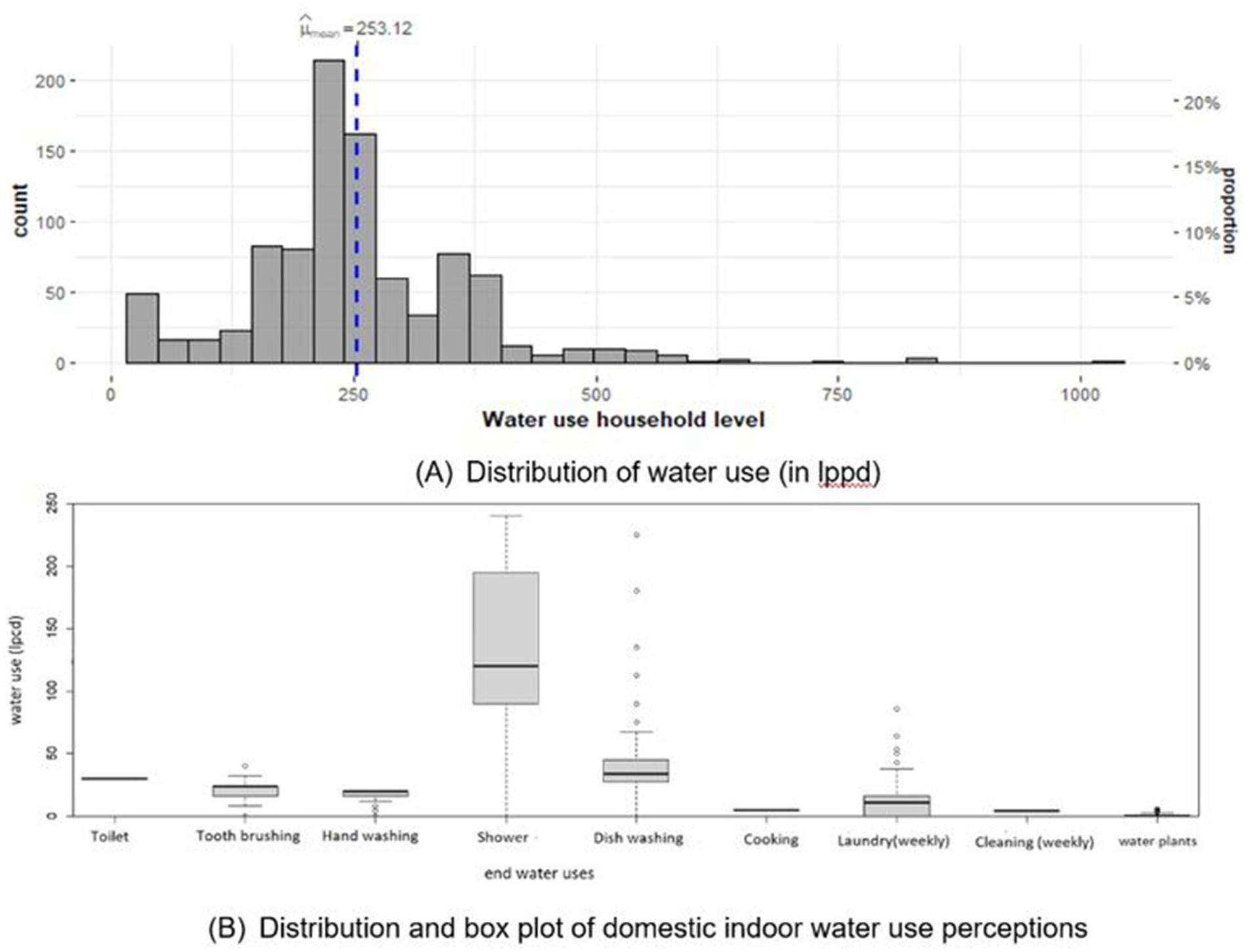
Figure 5. Distribution of water use (in lpcd). (A) Distribution of water use and (B) Box plot illustrating perceived domestic indoor water use.
Personal hygiene, including hand washing, teeth brushing and showering, accounted for an estimated 70% of total water use. Among these showering represented the highest proportion at (54.8%). Participants reported showering once (64%), twice (22.79%), or even three times (2.27%) per day, with shower durations ranging from 3 to 15 min Notably, 60% of the participants reported an average shower duration of 8 min. Comparable findings were observed in the Netherlands in 2013, where showering was the primary water use activity (41%), with an average shower duration of 8.9 min. Similarly, in the eight administrative regions of the Federal District located in the centre– west of Brazil, shower durations ranged between 6.3 and 9.2 min. Across 18 countries worldwide, the average reported shower time was 8.1 min (Mazzoni et al., 2023; Sant'Ana and Mazzega, 2018; Vewin – Association of Dutch water companies, 2017). Other studies have also indicated that showers are a key component of indoor water use, accounting for about one-third of indoor use (Makki et al., 2013). The volume of water used for showering depends on the implementation of efficient appliances and the duration of the shower. Even with the use of efficient fixtures, increasing shower duration by a minute can lead to approximately double the volume of water usage (Skipton and Dvorak, 2023). Our results indicate that participants allocated water usage as follows: 0.3% on watering plants, 1.6% on housecleaning, 3% on cooking, 4.6% on laundry, 11.9% on flushing the toilet, and on 17.5% dishwashing, respectively (Figure 5B).
Self-reported water use varied by geographical region. In peri-urban areas, nearly 50% of the users perceived using less than 250 lpcd, compared to 75% by the users of the WSSs in the rural areas. Overall, peri-urban zones exhibited higher mean values for self-reported water use compared to estimations in rural zones. The average estimated end water use in peri-urban zone’s was 263 lpcd, which was 27% higher than in rural zones. The ANOVA test reveals a significant difference in perceived water use between the two zones (p-value <0.05), indicating that household level perceptions of water use vary depending on location. Specifically, perceived water use per capita in peri-urban systems is significantly higher than in rural systems.
The reduction in water use for various household activities involves two actions: curtailment and efficient use (Attari, 2014). Curtailment involves reducing the duration of activities, such as showering, while efficient use entails installing or switching to water-efficient appliances and fixtures (Attari, 2014). From the surveyed households, results showed that, on average, the perception of the shower time is lower in rural zones (3.56 min) compared to peri-urban zones (7.49 min). This difference may be attributed to higher access to showers in the peri-urban zones (98%) compared to the rural zones (85). Therefore, it was assumed that more rural households may use buckets for bathing due to the lack shower heads, resulting in shorter showers compared to inhabitants of peri-urban zones. For instance, Ramsey et al. (2017) indicated that traditional bucket bathing proves to be more water-efficient as individuals only use on average 20 L of water per bath.
Furthermore, participants were asked about the type and use of basin, toilet, faucet, washing machine, and garden hose (Appendix 1). Most households either had conventional appliances and fixtures or incomplete plumbing (Meehan et al., 2021). Among the total interviewees, 79% of had conventional showers. However, when considering the type of showerheads and shower time, the estimated water use for reported showering was 79.6% higher in peri-urban zones compared to rural zones, with values of 150 lpcd and 83.3 lpcd, respectively. Additionally, households in peri-urban areas (88%) had more conventional faucets than rural households (68%). However, in the peri-urban areas, 61% of households had water-saving flushing toilets compared to 38% with conventional toilets.
A comparison of household water use, estimated by self-reported behavior, with absolute basic consumption (ABC) [52–100 lpcd], and realistic everyday acceptable limited needs consumption (REAL) [92–175] (Crouch et al., 2021) indicates that the mean water use (262 lpcd; 206 lpcd) exceeded the ABC and REAL references in peri-urban and rural zones, respectively. When referring to the water supplied by the system in liters per capita per day, as presented in Table 3 “Estimated Water Supplied and Perceived Water Used,” it noteworthy can be observed that only the 8C water system, with an estimated supplied volume of 136 lpcd, fell within the REAL range. This is likely due to several factors: water systems 8C had implemented a water use efficiency plan, installed a water flow meter, and assigned two plumbers to oversee the operation and maintenance of water infrastructure. Installing a water flow meter and retaining a plumber for operation and maintenance (O&M) exemplifies system-level measures. These actions directly impact the volume of water supplied by the system by aiding in the control of leakages and spills. Installing a water flow meter contributes better control of the volume of water distributed by the system to the users. Consequently, instances of overuse and waste can be minimized. In contrast, systems 1R and 7C systems, which are similar to 8C in terms of the number of users, lacked a water use efficiency plan. Additionally, 1R had only one plumber, and 7C did not have a water flow meter (see footnote 1).
In addition, the estimated, self-reported, water use was found to be between 116 and 204% higher than in the rural areas of Bangladesh (83.2 lpcd) (Al-Amin et al., 2011), India (117 lpcd) (Singh and Turkiya, 2013), Nigeria (95.8 lpcd) (Chukwuma, 2017), urban Australia (146 lpcd) (Beal et al., 2013), and Syria (130 lpcd) (Mourad et al., 2011). Conversely, in Malaysia (226 lpcd) (Hasan et al., 2021), Mexico (192 lpcd) (Ojeda de la Cruz et al., 2017) and various areas in Valle del Cauca in Colombia (160, 200, 204 lpcd respectively) (García and Brown, 2009; Restrepo Tarquino, 2010; SSPD, 2022), the self-reported range was between 12 and 58%. In urban Brazil, end water use values were 117 lpcd, 145 lpcd, 221 lpcd, and 226 lpcd, representing different income levels from low to high (Sant'Ana and Mazzega, 2018).
Figure 6 presents the ANOVA results assessing whether the mean estimates of perceived water use per capita were similar across the eight distinct WSSs at 5% significance level (Patil, 2021). It can be observed that all the rural systems exhibited similar mean estimates, but these were significantly lower than one or more peri-urban systems. Furthermore, there are significant differences among peri-urban systems.
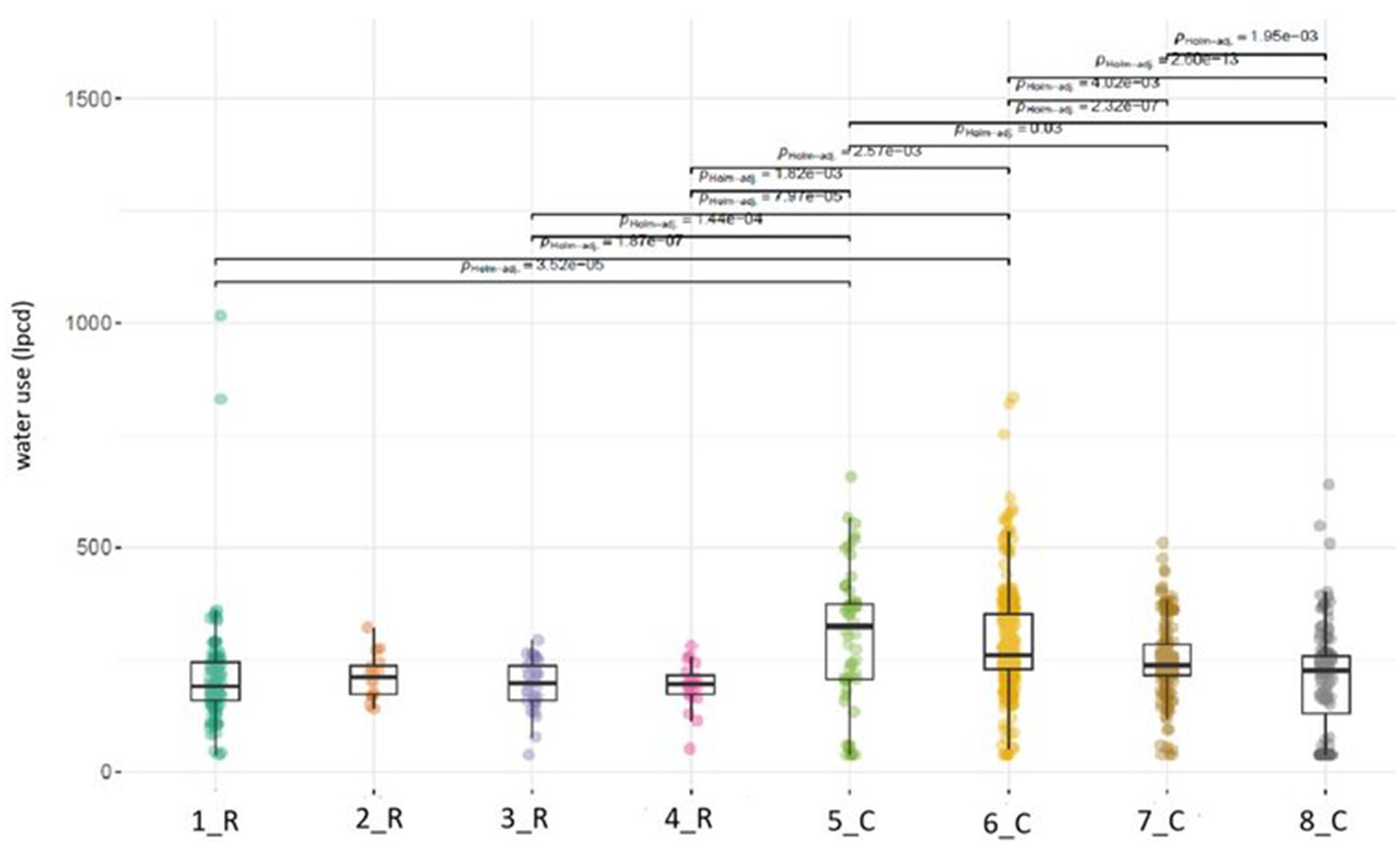
Figure 6. ANOVA by WSSs. On average, he perceived water use in rural (R) systems significantly differs from peri-urban (C) systems. Significant differences exist within peri-urban systems. The horizontal bars in the graph indicate pairs of WSSs with significantly different means, with corresponding -values shown.
Figure 7 illustrates the correlations between SEC factors and perceived water use. In the Figure, dark blue boxes represents a strong positive correlation, while dark red boxes indicate a strong negative correlation. Crosses displayed on the correlation coefficients signify non-significant correlations at 5% significance level, with the Holm adjustment method (Friendly, 2012; Soetewey, 2020). It can be observed that end water usage per capita decreased as the number of individuals in a household increased, i.e., as more people dwell in a house. Additionally, perceived water use increases with education level, which was assumed as a proxy for household income. This may be explained by the fact that individuals with high income levels typically have greater access to additional appliances and devices and an increased number of toilets and showerheads per household. Additionally, in some cases, high-income households may have amenities such as swimming pools and large gardens (Baker, 2014). Whereas water use tends to decreased as the average age of household members increased. The variable “income” was removed from the analysis due to approximately half of the participants did not respond to it.
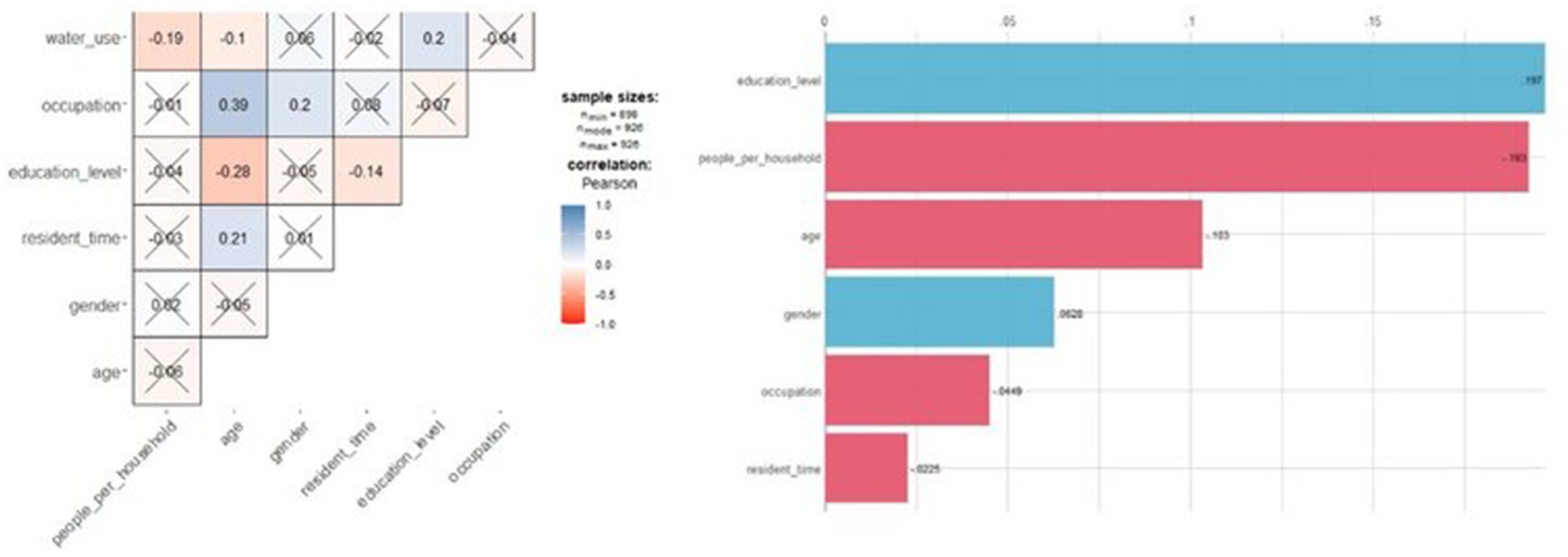
Figure 7. Correlogram and ranking of correlation test for socio-economic factors and water use at the household level.
Further examination of socio-economic factors in each zone revealed similarities in household sizes within each zone. Additionally, it was observed that individuals with low levels of education tend to reside in rural areas. For instance, in the peri-urban zones, 8.4 and 1.2% of people have earned bachelor’s and postgraduate degrees, respectively, compared to 2.5 and 0%, respectively, in the rural zones. In addition, on average, the peri-urban zones had younger individuals compared to the rural zones.
The mentioned SEC factors were used as independent variables, along with zone type as a binary variable (i.e., whether a system is rural or peri-urban), to study whether these factors explain the variance in perceived water use within peri-urban and rural supply systems. Appendix 2 shows that number of people per household, education level and zone are significant. A higher number of people per household was associated with lower perceived water use, while water use was perceived to increase with higher education levels residence in peri – urban areas. This trend may be attributed to the fact that higher education often correlates with higher income levels (Baker, 2014).
SEC factors accounted for only 10% of the variance, a result consistent with previous studies (Abu-Bakar et al., 2021; Cominola et al., 2023; Fielding et al., 2012; Jorgensen et al., 2009; Piedra-Muñoz et al., 2018; Russell and Knoeri, 2020). These studies have highlighted the importance of education level, age, and income as crucial variables in understanding water use or water conservation. Usually, there is a relationship between educational level and water use, which can be either positive or negative.
Our results indicate a positive relationship, meaning that water use increases with education level. This finding aligns with that of previous studies. This suggests that, on one hand, individuals with higher levels of education tend to use more water. However, they also have more financial resources, which allow them to invest in water-saving devices. However, they still used more water because they had more appliances and devices. It is possible that by including additional factor groups, such as technical and behavioral factors, the amount of explained variance might increase.
3.2 Water supplied at the system level
In this context, water supply on a system level refers to the water supplied from storage tanks through the distribution network to households (see Figure 1). This estimation was based on the water balance method described in Section 2, resulting in a range of percentages of supplied water and losses (Table 3) (Karamountzos, 2022). However, due to missing data for Q_intake (intake flows) for several systems, information on system water losses from intake to water treatment tanks was only available for 1R, 3R, 4R, 6C1, 6C2 and 8C. Interestingly, the losses due to leakage were found to be similar for both peri urban and rural WSSs. A comparison between perceived end water use per capita (“perception of water used”) and water supplied per capita (W) reveals a huge difference (Table 3). This disparity underscores the fate of water distributed from storage tanks to households and highlights the existing water losses between the distribution point and households.
Table 3 also shows the ratio of perceived end water use at the household level and water supplied from storage tank to households via the distributions of WSSs. A value greater than one indicates that perceived water use exceeds the system supply, while a value less than one could indicate inefficient water supply, e.g., due to losses or use for non-domestic purposes (García and Brown, 2009). Both scenarios are indicative of less-than-optimal governance of water systems.
Non-Revenue Water (NRW) comprises physical losses and commercial losses (Lambert and Hirner, 2000). The average national NRW rate is approximately 40% (SSPD, 2020). An estimation of NRW in urban supply systems worldwide suggests that in Global South countries, 60% of these losses are physical, while 40% are commercial (Kingdom et al., 2006). Water losses are only available for 6_C and 8_C because of the availability of data for Q_intake. Our results indicate that the estimated physical losses range from 42 to 89%, suggesting that upper limit of this range is higher than the global average for physical losses. However, despite this, the ratio of perceived water use to water supplied through the network for these two systems is around 1. This implies that the water is being used efficiently for domestic purposes.
Most of the rural systems exhibit very low ratios of perceived water use to water supplied. This discrepancy suggest that water may be lost during conveyance or used for non-domestic purposes. Studies have indicated that households in rural areas often use domestic water for non-domestic activities such as for cattle and coffee processing, which can account for up to twice the amount of water needed for domestic purposes.
Organizational issues also arise in rural systems. Rural systems typically have limited staffing, as shown in Table 3. Staffing mainly comprises people who participate in the community. Staffing and NRW fall within the category of indicators used to assess the quality and provision of rural drinking water supply services (Molinos-Senante et al., 2019). The engagement of communities in decision-making processes, technical determinations to decrease water use, and their understanding of water use indicators, such as NRW, are crucial for implementing measures to reduce water usage (Flórez et al., 2019).
An exception is system 1R, which distributes water to a larger area for a larger population across different sectors of the water supply system. The water users’ association in this system comprises several people on the board, although the majority serve voluntarily. While the plumber is primarily responsible for day-to-day operations and maintenance, the community comes together to address major damages or conflicts over water. In the event of conflicts, complaints are reported to the local or regional government. These unique attributes of system 1_R distinguish it from other rural systems that likely contribute to its better governance of the water system.
In contrast to rural systems, peri-urban systems typically supply water that meets the demand of the households (as indicated by perceived use). These systems, often larger and closer to urban centers, experience minimal or no non-domestic use. Some of these systems have WUEP in place, indicating greater attention to efficient water use compared to rural areas. The served population in peri-urban areas tends to be more educated and more specialized in their occupations (unlike mostly farmers in rural areas). Although, this demographic was associated with higher perceived water use per capita on average, peri-urban systems are more professionally managed, resulting in better overall system management.
In system 5_C, we can be observed an exception with a low ratio between perceived water use and water supplied through the network, approximately 0.5. Unlikely other peri-urban water systems, the community and area served by 5_C is smaller, although the population density comparable to that of 1R. Water in this system was mainly used for domestic purposes, but there were also commercial activities. Similar to 1R, operation and maintenance activities were managed by a single person, the plumber. However, the size of the WSSs in 5C is smaller than that of 1R, and the community do not provide the same level of support as in 1R. These differences may explain why the ratio value in 5_C is smaller than in 1R.
The availability of better quality employment opportunities in peri-urban areas, coupled with their proximity to urban areas, nables a better capacity for professionally management of water supply systems. In peri-urban zones, these systems often function more akin to a company, with sufficient financial resources and minimal reliance on subsidies for operation and maintenance (O&M). Consequently, revenue from water bills contributes to covering O&M costs. Conversely, in rural zones, water systems are not self-financed and typically rely on public or private assistance, subsidies, or community support for their O&M.
The infrastructure of utilities’ water supply systems is heavily influenced by the socio-economic context, specially, in low and middle-income countries where the infrastructure is often old and deteriorating. In this study, the average age of infrastructure of water supply systems exceeds 10 years, with only three systems having recent renewal. Moreover, aspects such as poor maintenance, inadequate operation, low water prices, population growth, and limited skills in water administration further contribute to performance issues.
Regarding indoor infrastructure, the presence or absence of water reservoirs in households may have influenced user’ perceptions. We did not inquire about this type of infrastructure in the questionnaire. However, during interviews with water managers, it was mentioned that some users have storage tanks to store water during the two-to-three-day gaps in supply, as the water distribution is not continuous. These tanks are transparent, allowing users to monitor the water levels at any time while using water. This practice likely fosters greater awareness of water consumption among users.
4 Discussion
Water scarcity is a major global challenge and often leads to inadequate water supplies for domestic use. Existing water systems tend to be poorly maintained, loosely regulated, and often lose water through leaks and unintended use outside the home. In this study, we identified that household size, education level, age and occupation are the key socio – economic factors link to water use perception. With higher education level, people use more water, there is a positive relationship. In peri-urban areas people use water more efficiency, but they use more water per capita because they have more appliances. Contextual factors such as zone and characteristics of water supply system are related with water use at system level.
The eight systems considered in this study, shared similarities such as being established during the last four decades, having changed from hand water collection to piped systems, belonging to the same department of Colombia, and being affiliated with similar water associations. However, the water infrastructure, water management (water bill; water quality criteria; water use permission), self-reported water use at the household level and water supply were different.
Our study showed that water supply was higher in rural areas when compared to peri-urban, even though perceived end water use was higher in peri-urban areas (Table 3). This apparent contradiction can be explained by SEC factors and distance from the capital cities. For example, peri-urban systems supply water to more people than rural systems. The urban water supply systems are better organized as drinking water supply companies even though these are WUAs.
Our workshops, interviews and results revealed that most WUA members in isolated rural areas were part-time volunteers, and in general these are low in number. Additionally, given that this region had a low prevalence of technical and professional education, water managers in rural areas lacked the time, money, and water literacy necessary to support full-time water management.
Household size, education level, age and occupation were found to be critical factors that influence end water use and water supply. These not only account for why water was used relatively efficiently and supplied inefficiently in rural systems (e.g., due to non-domestic use based on qualitative information obtained from water managers) and vice versa in peri-urban areas, but it also accounted for variability within the peri-urban systems. While the peri-urban users were perceived to use more water associated with younger and more educated households, these were also the factors that explain why systems were better managed.
In addition to SEC considerations, distance from major cities was another contextual factor that had an impact on water supply at the system and perceived use at household levels. Most peri-urban areas are well served by water services compared to rural areas. The rural systems had very low ratios of perceived water use to water supplied indicating that either water is lost in conveyance or water is used for non-domestic purposes. An overestimation of lpcd at the household and system level may result from a difference between the value of household size provided by respondents and that provided by the water suppliers.
Most of these studies have emphasized the role of socio-economic (SEC) factors on (in)efficiency of the end water use and water supply systems with little consideration of the distances of water supply systems from urban areas that limit income opportunities, technical outreach and its effect on water use at system level as perceived by the water users. Our results indicate differences in SEC and water usage between peri-urban and rural systems. When referring to the SEC, we aim to emphasize the contextual factors relevant to assessing water usage at the household level. This focus is important because decision makers often highlight the need to considering the diversity of socio-economic conditions. Indeed, numerous studies have demonstrated a correlation between higher income levels and increased water consumption.
Data availability statement
The original contributions presented in the study are included in the article/Supplementary material, further inquiries can be directed to the corresponding author.
Ethics statement
The studies involving humans were approved by Dr. Cath Cotton, Policy Advisor Academic Integrity/TUDelft. The studies were conducted in accordance with the local legislation and institutional requirements. The participants provided their written informed consent to participate in this study.
Author contributions
DC: Conceptualization, Data curation, Formal analysis, Funding acquisition, Investigation, Methodology, Project administration, Resources, Software, Supervision, Validation, Visualization, Writing – original draft, Writing – review & editing. SP: Conceptualization, Formal analysis, Supervision, Writing – review & editing. MH: Conceptualization, Writing – review & editing. JR: Conceptualization, Supervision, Writing – review & editing. LR: Conceptualization, Formal analysis, Supervision, Writing – review & editing.
Funding
The author(s) declare that financial support was received for the research, authorship, and/or publication of this article. This work was supported by Pasaporte a la Ciencia programme of Instituto Colombiano de Crédito Educativo y Estudios Técnicos en el Exterior-ICETEX (grant no. 3787907), Colfuturo (grant no. PCB-2018) and Fondo Fundación WWB Colombia para la Investigación (grant no. 096-2021).
Acknowledgments
This study is part of the first author’s PhD Program from Delft University of Technology. The author expressed her gratitude for useful meetings to organize teamwork, review the questionnaire and flux measurements with a lot of good inputs from water managers and staff of each of WSSs, Fecoser and Aquacol water associations, Luis Dario Sanchez, and Carolina Blanco, Universidad del Valle; D. Daniel, Gadjah Mada, University; Marlen Ramírez for their collaboration and support during the whole process of data collection; Julián Robles, Ministry of Environment and Sustainable Development of Colombia; Aansha Mittal, David Sehouevi, Linnaea Cahill, Job van der Werf and Pierre Karamountzos from CEG -TUDelft, Mohammad Alam from cigar.org, and Ignacio Hernández from TPM—TUDelft, and Henry Amorocho, and Jorge Díaz from IHE—Delft, for their insights to data management and analysis the data.
Conflict of interest
The authors declare that the research was conducted in the absence of any commercial or financial relationships that could be construed as a potential conflict of interest.
The author(s) declared that they were an editorial board member of Frontiers, at the time of submission. This had no impact on the peer review process and the final decision.
Publisher’s note
All claims expressed in this article are solely those of the authors and do not necessarily represent those of their affiliated organizations, or those of the publisher, the editors and the reviewers. Any product that may be evaluated in this article, or claim that may be made by its manufacturer, is not guaranteed or endorsed by the publisher.
Supplementary material
The Supplementary material for this article can be found online at: https://www.frontiersin.org/articles/10.3389/frwa.2024.1389648/full#supplementary-material
Footnotes
1. ^personal communication – Zoom-meting, October 11, 2021
References
Abu-Bakar, H., Williams, L., and Stephen, H. H. (2021). A review of household water demand management and consumption measurement. J. Clean. Prod. 292:125872. doi: 10.1016/j.jclepro.2021.125872
Al-Amin, M., and Mahmud, K., Hosen, S ., & Islam, M. A . (2011). Domestic water consumption patterns in a village in Bangladesh. In Proceeding of 4th annual paper meet and 1st civil engineering congress, 4, 83–85.
Arouna, A., and Dabbert, S. (2010). Determinants of domestic water use by rural households without access to private improved water sources in Benin: A seemingly unrelated Tobit approach. Water Resour. Manag. 24, 1381–1398. doi: 10.1007/s11269-009-9504-4
Attari, S. Z. (2014). Perceptions of water use. Proc. Natl. Acad. Sci. USA 111, 5129–5134. doi: 10.1073/pnas.1316402111
Baker, E. (2014). “Socioeconomic status, definition” in Wiley Blackwell encyclopedia of health, illness, behavior, and society. eds. W. C. Cockerham, R. Dingwall, and S. R. Quah, The Wiley Blackwell Encyclopedia of Health, Illness, Behavior, and Society. Chichester, UK: John Wiley & Sons, Ltd, 2010–2214.
Barbier, E. B. (2019). The water paradox: Overcoming the global crisis in water management USA; London, UK: Yale University Press: New Haven, CT.
Barde, A. (2017). What determines access to piped water in rural areas? Evidence from small-scale supply Systems in Rural Brazil. World Dev. 95, 88–110. doi: 10.1016/j.worlddev.2017.02.012
Beal, C. D., Stewart, R. A., and Fielding, K. (2013). A novel mixed method smart metering approach to reconciling differences between perceived and actual residential end-use water consumption. J. Clean. Prod. 60, 116–128. doi: 10.1016/j.jclepro.2011.09.007
Benito, B., Faura, U., Guillamón, M., and Ríos, A. (2019). Empirical evidence for efficiency in provision of drinking water. J. Water Resour. Plan. Manag. 145, 1–10. doi: 10.1061/(ASCE)WR.1943-5452.0001049
Blair, P., and Buytaert, W. (2016). Socio-hydrological modelling: A review asking “why, what and how?”. Hydrol. Earth Syst. Sci. 20, 443–478. doi: 10.5194/hess-20-443-2016
Chacón, M. G., Lizcano, I., and Asprilla, L. Y. (2011). Consumo básico de agua potable en Colombia. Tecnogestión: Una mirada al ambiente, 8 Available at: https://revistas.udistrital.edu.co/index.php/tecges/article/view/4379.
Charalambous, B., and Laspidou, C. (2017). Dealing with the complex interrelation of intermittent supply and water losses. In scientific and technical report (issue 25).
Charlotte, C. T., Bessias, S., Villada, A., Tulsiani, S. M., Ensink, H. J., Gurley, E., et al. (2016). Measuring domestic water use: a systematic review of methodologies that measure unmetered water use in low-income settings. Trop. Med. Int. Health 21, 1389–1402. doi: 10.1111/tmi.12769
Chawira, M., Hoko, Z., and Mhizha, A. (2022). Partitioning non-revenue water for Juru rural service Centre. Goromonzi District, Zimbabwe: Physics and Chemistry of the Earth.
Chukwuma, O. M. (2017). Patterns and problems of domestic water supply to rural communities in Enugu state, Nigeria. J. Agric. Extension Rural Develop 9, 172–184. doi: 10.5897/JAERD2016.0802
Cominola, A., Preiss, L., Thyer, M., et al. (2023). The determinants of household water consumption: A review and assessment framework for research and practice. NPJ Clean Water 6, 1–14. doi: 10.1038/s41545-022-00208-8
Creswell, J. (2014). Research design: qualitative, quantitative, and mixed method approaches. Los Angeles: Sage, 717.
Crouch, M. L., Jacobs, H. E., and Speight, V. L. (2021). Defining domestic water consumption based on personal water use activities. Aqua Water Infrastruct. Ecosyst. Soc. 70, 1002–1011. doi: 10.2166/aqua.2021.056
Dean, A. J., Fielding, K. S., and Newton, F. J. (2016a). Community knowledge about water: who has better knowledge and is this associated with water-related behaviors and support for water-related policies? PLoS One 11, 1–18. doi: 10.1371/journal.pone.0159063
Dean, A. J., Lindsay, J., Fielding, K. S., and Smith, L. D. G. (2016b). Fostering water sensitive citizenship - community profiles of engagement in water-related issues. Environ Sci Policy 55, 238–247. doi: 10.1016/j.envsci.2015.10.016
Delgado-Serrano, M., Ramos, P., Lasso Zapata, E., Ramos, P. A., and Zapata, E. (2017). Using Ostrom’s DPs as fuzzy sets to analyse how water policies challenge community-based. Water 9:535. doi: 10.3390/w9070535
Doane, D., and Seward, L. (2013). Applied statistics in business and economics. New York: McGraw-Hill/Irwin.
Edmondson, D. (2005). Likert scales: A history. In Proceedings of the conference on historical analysis and research in marketing, 127–133
Erdfelder, E., Faul, F., Buchner, A., and Lang, A. G. (2009). Statistical power analyses using G*Power 3.1: Tests for correlation and regression analyses. Behav. Res. Methods 41, 1149–1160. doi: 10.3758/BRM.41.4.1149
Fielding, K. S., Russell, S., Spinks, A., and Mankad, A. (2012). Determinants of household water conservation: the role of demographic, infrastructure, behavior, and psychosocial variables. Water Resour. Res. 48, 1–12. doi: 10.1029/2012WR012398
Flórez, M. P., Ramírez, M. C., Payán-Durán, L. F., Peralta, M., and Acero Lopez, A. E. (2019). A systemic methodology for the reduction of water consumption in rural areas. Kybernetes 48, 163–182. doi: 10.1108/K-10-2017-0406
Friendly, M. (2012). Correlograms: exploratory displays for correlation matrices. Am. Stat. 56, 316–324. doi: 10.1198/000313002533
Fu, Y., and Wu, W. (2014). Behaviour interventions for water end use: An integrated model. In Proceedings of the 20th International Conference on Automation and Computing: Future Automation, Computing and Manufacturing, 266–271
García, C. E. R., and Brown, S. (2009). Assessing water use and quality through youth participatory research in a rural Andean watershed. J. Environ. Manag. 90, 3040–3047. doi: 10.1016/j.jenvman.2009.04.014
Gonzales, P., and Ajami, N. (2017). Social and structural patterns of drought-related water conservation and rebound. Water Resour. Res. 53, 10619–10634. doi: 10.1002/2017WR021852
Hasan, H. H., Razali, S. F. M., and Razali, N. H. M. (2021). Does the household save water? Evidence from behavioral analysis. Sustainability 13, 1–20. doi: 10.3390/su13020641
Haslam, S., and McGarty, C. (2014). Research methods and statistics in psychology. 2nd Edn. London,UK: SAGE Publications Ltd.
Hellegers, P., and Halsema, G.Van. (2021). Science of the Total Environment SDG indicator 6. 4. 1 “change in water use efficiency over time”: Methodological fl aws and suggestions for improvement. 801. doi: 10.1016/j.scitotenv.2021.149431
Hussien, W. A., and Memon, F. A. (2016). Assessing and Modelling the Influence of Household Characteristics on Per Capita Water Consumption. Water Resour. Manag. 2931–2955. doi: 10.1007/s11269-016-1314-x
Hou, B. D., Yang, R. X., Wang, J. H., Xiao, W. H., Zhao, Y., and Wang, H. (2019). Conceptual framework of hierarchical water demand. IOP Conf. Series 344. doi: 10.1088/1755-1315/344/1/012074
Jorge, C., Vieira, P., Rebelo, M., and Covas, D. (2015). Assessment of water use efficiency in the household using cluster analysis. Procedia Engin. 119, 820–827. doi: 10.1016/j.proeng.2015.08.945
Jorgensen, B., Graymore, M., and O’Toole, K. (2009). Household water use behavior: an integrated model. J. Environ. Manag. 91, 227–236. doi: 10.1016/j.jenvman.2009.08.009
Karamountzos, P. (2022). Water use efficiency in water supply systems (Bachelor’s thesis). Delft University of Technology, Department of Water Management, Faculty of Civil Engineering and Geosciences, Delft.
Keshavarzi, A. R., Sharifzadeh, M., Kamgar Haghighi, A. A., Amin, S., Keshtkar, S., and Bamdad, A. (2006). Rural domestic water consumption behavior: A case study in Ramjerd area, Fars province, I.R Iran. Water Res. 40, 1173–1178. doi: 10.1016/j.watres.2006.01.021
Khair, S. M., Mushtaq, S., Reardon-Smith, K., and Ostini, J. (2019). Diverse drivers of unsustainable groundwater extraction behaviour operate in an unregulated water scarce region. J. Environ. Manage., 340–350. doi: 10.1016/j.jenvman.2018.12.077
Kingdom, B., Liemberger, R., and Philippe, M. (2006). The Challenge of Reducing Non-Revenue Water (NRW) in Developing Countries. Water Supply and Sanitation Sector Board Discussion Paper Series-Paper No.8. Opgeroepen op August 5, 2024, Available at: https://documents1.worldbank.org/curated/en/385761468330326484/pdf/394050Reducing1e0water0WSS81PUBLIC1.pdf (Accessed June 1, 2022).
Koop, S. H. A., Van Dorssen, A. J., and Brouwer, S. (2019). Enhancing domestic water conservation behaviour: A review of empirical studies on influencing tactics. J. Environ. Manag. 247, 867–876. doi: 10.1016/j.jenvman.2019.06.126
Kunitsuka, I. (2014). Water, sanitation and solid water. How much water do you actually consume every day? Available at: https://blogs.iadb.org/agua/en/much-water-actually-consume-every-day/ (Accessed May 26, 2022).
Lambert, A., and Hirner, W. (2000). Losses from water supply systems: standard terminology and recommended performance measures. London, UK: International Water Association (IWA), 1–13.
Liemberger, R., and Wyatt, A. (2019). Quantifying the global non-revenue water problem. Water Sci. Technol. Water Supply 19, 831–837. doi: 10.2166/ws.2018.129
Makaya, E., and Hensel, O. (2014). Water distribution systems efficiency assessment indicators – concepts and application. Int. J. Sci. Res. 3, 219–228.
Makki, A. A., Stewart, R. A., Panuwatwanich, K., and Beal, C. (2013). Revealing the determinants of shower water end use consumption: enabling better targeted urban water conservation strategies. J. Clean. Prod. 60, 129–146. doi: 10.1016/j.jclepro.2011.08.007
Manouseli, D., Anderson, B., and Nagarajan, M. (2018). Domestic water demand during droughts in temperate climates: synthesising evidence for an integrated framework. Water Resour. Manag. 32, 433–447. doi: 10.1007/s11269-017-1818-z
Mazzoni, F., Alvisi, S., Blokker, M., Buchberger, S. G., Castelletti, A., Cominola, A., et al. (2023). Investigating the characteristics of residential end uses of water: A worldwide review. Water Res. 230:119500. doi: 10.1016/j.watres.2022.119500
McCarroll, M., and Hamann, H. (2020). What we know about water: a water literacy review. Water 12, 1–28. doi: 10.3390/w12102803
Meehan, K., Jurjevich, J. R., Griswold, A., Chun, N. M., and Sherrill, J. (2021). Plumbing poverty in U.S. cities: A report on gaps and trends in household water access, 2000 to 2017. London, UK: King's College London
Metabolism of Cities - Data Hub (2023). Map - Division Politico Administrativa Departamental de Colombia 2018. Map - Division Politico Administrativa Departamental de Colombia 2018 Metabolism of Cities Data Hub
Millock, K., and Nauges, C. (2010). Household adoption of water-efficient equipment: the role of socio-economic factors, environmental attitudes and policy. Environ. Resour. Econ. 46, 539–565. doi: 10.1007/s10640-010-9360-y
Mittal, A. (2019). Diagnosing Community-based Management of Groundwater in Peri-urban areas. Master thesis, Delft University of Technology, Delft
Molinos-Senante, M., Muñoz, S., and Chamorro, A. (2019). Assessing the quality of service for drinking water supplies in rural settings: A synthetic index approach. J. Environ. Manag. 247, 613–623. doi: 10.1016/j.jenvman.2019.06.112
Mosler, H.-J., and Contzen, N. (2016). Systematic behavior change in water sanitation and hygiene A practical guide using the RANAS approach : Eawag Available at: https://www.ranasmosler.com/ranas. (Accessed 27 April, 2022).
Mourad, K. A., Berndtsson, J. C., and Berndtsson, R. (2011). Potential fresh water saving using greywater in toilet flushing in Syria. J. Environ. Manag. 92, 2447–2453. doi: 10.1016/j.jenvman.2011.05.004
Muneepeerakul, R., and Anderies, J. M. (2017). Strategic behaviors and governance challenges in social-ecological systems. Earth Future. 5, 865–876. doi: 10.1002/2017EF000562
Muniz, M. A., Thurler, N. M., Carneiro, A. L., da Silveira, Q. N., da Costa, D. J., de Paiva, A. P., et al. (2020). Assessment of community- management water systems in rural areas of Espirito Santo, Brazil, using the SIASAR tool. Interdiscip. J. Appl. Sci. 15, 1–19. doi: 10.4136/ambi-agua.2517
NASA Shuttle Radar Topography Mission (2013). Shuttle radar topography Mission (SRTM) global. Distributed by Open Topography.
Newton, P., and Meyer, D. (2012). The determinants of urban resource consumption. Environ. Behav. 44, 107–135. doi: 10.1177/0013916510390494
Ojeda de la Cruz, A., Alvarez-Chavez, C. R., Ramos-Corella, M. A., and Soto-Hernandez, F. (2017). Determinants of domestic water consumption in Hermosillo, Sonora, Mexico. J. Clean. Prod. 142, 1901–1910. doi: 10.1016/j.jclepro.2016.11.094
Onyenankeya, K., Onyenankeya, O. M., and Osunkunle, O. (2021). Barriers to water use efficiency in rural and peri-urban areas of South Africa. Water Environ. J. 35, 1164–1173. doi: 10.1111/wej.12707
Pande, S., and Sivapalan, M. (2016). Progress in socio-hydrology: a meta-analysis of challenges and opportunities. WIREs Water 4. doi: 10.1002/wat2.1193
Patil, I. (2021). Visualizations with statistical details: the 'ggstatsplot' approach. J. Open Source Softw. 6:3167. doi: 10.21105/joss.03167
Piedra-Muñoz, L., Vega-López, L. L., Galdeano-Gómez, E., and Zepeda-Zepeda, J. A. (2018). Drivers for efficient water use in agriculture: an empirical analysis of family farms in Almería, Spain. Exp. Agric. 54, 31–44. doi: 10.1017/S0014479716000661
Polania, J., and Vanessa, A. (2019). Comparative water system analysis of urban quarters under consideration of different climate conditions. (Master’s thesis, Institute of Sanitary Engineering and Waste Management). Hannover: Leibniz Universitat Hannover.
Qualtrics . (2021). The Leading Experience management Software. Available at: https://www.qualtrics.com
R Core Team . (2021). R: A language and environment for statistical computing. R Foundation for Statistical Computing. https://www.R-project.org/ (Accessed August 30, 2021).
Rahayu, P., and Rini, E. F. (2019). Towards sustainable communities: socioeconomic determinants of domestic water consumption in Surakarta City, Indonesia. MATEC Web Conf. 280:05016. doi: 10.1051/matecconf/201928005016
Ramsey, E., Berglund, E. Z., and Goyal, R. (2017). The impact of demographic factors, beliefs, and social influences on residential water consumption and implications for non-price policies in urban India. Water 9:844. doi: 10.3390/w9110844
Restrepo Tarquino, I. (2010). Usos múltiples del agua como una estrategia para la reducción de la pobreza. Cali: Universidad del Valle.
Revelle, W . (2022). Procedures for psychology, psychometric, and personality research. Package "psych". Available at: https://personality-project.org/r/psych/ (Accessed March 20, 2023).
Roobavannan, M., Van Emmerik, T. H. M., Elshafei, Y., Kandasamy, J., Sanderson, M. R., Vigneswaran, S., et al. (2018). Norms and values in sociohydrological models. Hydrol. Earth Syst. Sci. 22, 1337–1349. doi: 10.5194/hess-22-1337-2018
Russell, S., and Fielding, K. (2010). Water demand management research: A psychological perspective. Water Resour. Res. 46, 1–12. doi: 10.1029/2009WR008408
Russell, S. V., and Knoeri, C. (2020). Exploring the psychosocial and behavioural determinants of household water conservation and intention. Int. J. Water Resources Develop. 36, 940–955. doi: 10.1080/07900627.2019.1638230
Sanderson, M. R., Bergtold, J. S., Heier Stamm, J. L., Caldas, M. M., and Ramsey, S. M. (2017). Bringing the “social” into sociohydrology: conservation policy support in the central Great Plains of Kansas, USA. Water Resour. Res. 53, 6725–6743. doi: 10.1002/2017WR020659
Sant'Ana, D., and Mazzega, P. (2018). Socioeconomic analysis of domestic water end-use consumption in the Federal District, Brazil. Sustain. Water Resour. Manag. 4, 921–936. doi: 10.1007/s40899-017-0186-4
SIASAR . (2022). Data by country – Colombia. Rural Water and Sanitation Information System (SIASAR). Available at: https://globalsiasar.org/en (Accessed July 10, 2022).
Singh, O., and Turkiya, S. (2013). A survey of household domestic water consumption patterns in rural semi-arid village, India. GeoJournal 78, 777–790. doi: 10.1007/s10708-012-9465-7
Singha, B., Eljamal, O., Chandra, S. K., Maamoun, I., and Sugihara, Y. (2022). Water conservation behavior: exploring the role of social, psychological, and behavioral determinants. J. Environ. Manag. 317:115484. doi: 10.1016/j.jenvman.2022.115484
Sione, L. (2021). Assisting stakeholders in data-scarce settings: Citizen science as a tool to assess intermittent water supply systems in developing countries. (Doctoral’s thesis, Imperial College, Civil and Environmental Engineering). Spiral - Repository. London
Skipton, S., and Dvorak, B. (2023). Water conservation in the shower. Available at: https://droughtresources.unl.edu/water-conservation-shower (Accessed October 10, 2023).
Soetewey, A . (2020). Descriptive statistics in R. Available at: https://statsandr.com/blog/descriptive-statistics-in-r/#coefficient-of-variation (Accessed November 21, 2021).
Srinivasan, V., Lambin, E. F., Gorelick, S. M., Thompson, B. H., and Rozelle, S. (2012). The nature and causes of the global water crisis: syndromes from a meta-analysis of coupled human-water studies. Water Resour. Res. 48, 1–16. doi: 10.1029/2011WR011087
SSPD . (2019). Informe nacional de coberturas de los servicios públicos de acueducto y alcantarillado. Available at: https://www.superservicios.gov.co/sites/default/files/2022-01/informe_nacional_de_coberturas_de_los_servicios_publicos_de_acueducto_y_alcantarillado-_2019_vfinal_1_0.pdf (Accessed September 10, 2020).
SSPD . (2020). Informe sectorial de los servicios públicos domiciliarios de acueducto y alcantarillado 2020. Available at: https://www.superservicios.gov.co/sites/default/files/inline-files/informe_sectorial_aa_30-12-21_vf%20%281%29.pdf (Accessed June 15, 2022).
SSPD . (2022). Informe Sectorial de los Servicios Públicos Domiciliarios de Acueducto y Alcantarillado 2022. Available at: https://www.superservicios.gov.co/sites/default/files/inline-files/Informe-Sectorial-de-los-Servicios-Publicos-Domiciliarios-de-Acueducto-y-Alcantarillado-Vigencia-2022.pdf
Steg, L., and Vlek, C. (2009). Encouraging pro-environmental behaviour: an integrative review and research agenda. J. Environ. Psychol. 29, 309–317. doi: 10.1016/j.jenvp.2008.10.004
Stern, P. C. (2000). New environmental theories: toward a coherent theory of environmentally significant behavior. J. Soc. Issues 56, 407–424. doi: 10.1111/0022-4537.00175
Stern, P. C. (2014). Individual and household interactions with energy systems: toward integrated understanding. Energy Res. Soc. Sci. 1, 41–48. doi: 10.1016/j.erss.2014.03.003
Strauch, A. M., Kalumbwa, E., and Almedom, A. M. (2021). Spatial analysis of domestic water use and rural livelihoods in a semi-arid African highland. J. Arid Environ. 194:104608. doi: 10.1016/j.jaridenv.2021.104608
Workman, C. L., Cairns, M. R., de Los Reyes, F. L. 3rd, and Verbyla, M. E. (2021). Global Water, Sanitation, and Hygiene Approaches: Anthropological Contributions and Future Directions for Engineering. Environ. Eng. Sci. 38, 402–417. doi: 10.1089/ees.2020.0321
United Nations . (2022). Indicator 6.4. - sustainable development goals (SGD). Available at: https://country-profiles.unstatshub.org/col#goal-6 (Accessed October 10, 2023).
Urrea, F., and Canelo, A. F. (2017). Cali, ciudad región ampliada: una aproximación desde la dimensión étnica-racial y los flujos poblacionales. Soc. Econ. 33, 145–174.
U.S. Geological Survey . (2005). Water Q&A: How much water do I use at home each day?. Available at: https://www.usgs.gov/special-topics/water-science-school/science/water-qa-how-much-water-do-i-use-home-each-day
Veldt, T., and Burger, R. (2015). Inventory of water treatment plants in rural area of Valle del Cauca. Civil Engineering and Geosciences Faculty, Water Management Department: University of Technology TU Delft.
Vergara, R., Arias, J. E., and Rodríguez, M. E. (2020). Congestión urbana en Santiago de Cali, un estudio de caso de política pública. Territorios 42, 1–29. doi: 10.12804/revistas.urosario.edu.co/territorios/a.7239
Vewin – Association of Dutch water companies . (2017). Dutch drinking water statistics 2017 from source to tap. The drinking water statistics series, The Hague.
Keywords: water use behavior, contextual factors, distance, household water use, water users’ associations
Citation: Callejas Moncaleano DC, Pande S, Haeffner M, Rodríguez Sánchez JP and Rietveld L (2024) Inefficiencies in water supply and perceptions of water use in peri-urban and rural water supply systems: case study in Cali and Restrepo, Colombia. Front. Water. 6:1389648. doi: 10.3389/frwa.2024.1389648
Edited by:
Ricard Gine-Garriga, Stockholm International Water Institute, SwedenReviewed by:
Leonardo Capeleto de Andrade, University of São Paulo, BrazilEdgar Quiroga, University of the Valley, Colombia
Copyright © 2024 Callejas Moncaleano, Pande, Haeffner, Rodríguez Sánchez and Rietveld. This is an open-access article distributed under the terms of the Creative Commons Attribution License (CC BY). The use, distribution or reproduction in other forums is permitted, provided the original author(s) and the copyright owner(s) are credited and that the original publication in this journal is cited, in accordance with accepted academic practice. No use, distribution or reproduction is permitted which does not comply with these terms.
*Correspondence: Diana Carolina Callejas Moncaleano, ZC5jLmNhbGxlamFzbW9uY2FsZWFuby0xQHR1ZGVsZnQubmw=