- Hydrogeochemical Engineering and Remediation of Soils Laboratory, School of Chemical and Environmental Engineering, Technical University of Crete, Chania, Greece
The water ecosystem food (WEF) nexus duly acknowledges the complex interdependencies among water, ecosystems, and food production, underscoring nature based solutions (NBS) as an efficacious strategy for navigating these interconnections. In this research, four different NBS (terraces, riparian forest, livestock management and agro ecological practices) were assessed in terms of their impact to WEF nexus. The Karst-SWAT and the one-dimensional integrated critical zone (1D-ICZ) models were used to simulate the impact of NBS on water quantity and quality as well as on soil ecosystem services of Koiliaris River Basin, which serves as an illustrative example of a basin that has experienced severe soil and biodiversity degradation. The Karst-SWAT model showed that a combination of NBS of terraces and riparian forest can reduce soil erosion and the sediment load by 97%. The 1D-ICZ model successfully simulated the soil-plant-water system and showed that agro ecological practices affect biomass production, carbon and nutrient sequestration, soil structure and geochemistry.
1 Introduction
The concept of integrated management of natural resources using a nexus approach has gathered scientific attention over the past years, especially when assessing the interactions across the water, energy and food domains (Hoff, 2011). Recently, the nexus concept has been expanding in other directions, such as land use, soil, waste, climate, economy, ecosystems, health, making the approach more interdisciplinary (Avellan et al., 2017; Laspidou et al., 2018). The water-ecosystem-food (WEF) nexus is a conceptual framework that highlights the interconnected relationships between water, ecosystems and food production. This approach recognizes the interdependencies among these three essential components and aims to address their management in a holistic and integrated manner (Walker et al., 2022), rather than treating them in isolation (Sánchez-Zarco and Ponce-Ortega, 2023). The WEF nexus acknowledges the importance of sustainable water management to ensure sufficient water availability for drinking water, irrigation, energy generation as well as ecosystem support. Ecosystems provide a range of services, such as water purification, pollination and soil fertility, which are vital for supporting agricultural productivity and human sustenance (FAO, 2014; Liu, 2016). The WEF nexus recognizes the intrinsic link between healthy ecosystems and sustainable food production. Agricultural production relies on water resources and plays a vital role in providing ecosystem services (McGrane et al., 2019; Sánchez-Zarco and Ponce-Ortega, 2023). The WEF nexus approach encompasses a comprehensive understanding of how food production is intertwined with water availability and ecosystem services. It promotes sustainable agricultural practices that consider the broader environmental context (Albrecht et al., 2018; Simpson and Jewitt, 2019; Purwanto et al., 2021) and ecosystem resilience to environmental changes (Chambers et al., 2019).
The overall aim of the WEF nexus is to improve the cooperation among the sectors by considering trade-offs (Pittock et al., 2015; Karnib, 2017) and enhancing synergies, to achieve sustainability (Wu et al., 2021; Sun et al., 2022). This emphasis on adaptable and integrated solutions is critical for navigating the complex interactions within the system, thereby contributing to the resilience of ecosystems in the face of environmental uncertainties (Fader et al., 2018). Decision-makers, can formulate strategies that address immediate challenges while fostering long-term sustainability (Ding et al., 2023) using bottom-up approaches to managing resources (Flammini et al., 2014). By promoting collaboration among diverse stakeholders, the WEF nexus encourages a collective comprehension of interdependencies (Mohtar and Daher, 2016; Hoolohan et al., 2018; Melloni et al., 2020). Canessa et al. (2022) presented a methodological framework that seeks to integrate the perspectives of experts, practitioners and local stakeholders on the nexus through the combined application of the Delphi and Focus Group methods using the municipality of Apokoronas in Crete, Greece as case study.
This WEF nexus approach is fundamental in addressing the complex challenges faced by ecosystems and promoting their capacity to withstand disturbances. Nature-based solutions (NBS) are widely recognized as sustainable strategies (Maes and Jacobs, 2015) for tackling environmental challenges, such as climate change, food and water insecurity, human health and well-being (Kolokotsa et al., 2020), biodiversity loss (Faivre et al., 2017; Somarakis et al., 2019; United Nations Environment Programme, 2022). NBS play a crucial role in the WEF nexus and have been identified as key concepts to defuse the expected tensions within the WEF nexus due to their multiple benefits (Carvalho et al., 2022).
Recent EU funded projects and initiatives (e.g., the PRIMA-LENSES, the Horizon 2020 Rexus, the RETOUCH NEXUS and the NEXOGENESIS projects) have identified the need of developing frameworks for optimizing the WEF nexus at local and regional scales. This optimization would require the use of hydrological and geochemical models to simulate the effect of NBS in resolving various issues raised by the WEF nexus at the basin scale. Models that have already been used in the literature to simulate the impact of NBS implementation include Karst-SWAT, HEC-RAS, QUESTOR, and the XBeach hydro-morphological model (Unguendoli et al., 2023; Hutchins et al., 2024; Liao et al., 2024). Lilli et al. (2020b) used a combination of Karst-SWAT and the HEC-RAS to design the restoration of a riparian forest and measures for flood protection for the Koilaris River Basin. In particular, the calibrated karst-SWAT model provided gap filled surface flow data for the past 45 years that were used to statistically determine the 50-year return flow for the design of sustainable, ecologically-friendly flood protection measures.
The objective of this work was to illustrate how hydrological and geochemical models can be used for assessing ecosystem services provided by NBS and then in turn to be used for the optimization of the WEF nexus at the watershed scale (i.e., the Koiliaris River Basin of Crete, Greece). Specifically, the Karst-SWAT (Nikolaidis et al., 2013) and the one-dimensional integrated critical zone (1D-ICZ) (Giannakis et al., 2017; Kotronakis et al., 2017) models were used to simulate the impact of NBS on water quantity and quality as well as on soil ecosystem services. The NBS (Somarakis et al., 2019) that will be assessed in this work are the creation of terraces and riparian forest, management of livestock for the improvement of water quality and agro ecological practices for the assessment of soil ecosystem services (biomass production, nutrient sequestration, water filtration and transformation, soil structure and fertility and below ground biodiversity).
2 Materials and methods
2.1 Site description and WEF related challenges
The Koiliaris River Basin is situated 15 km east of the city of Chania in Crete. The total watershed area covers 130 km2 with the primary water source originating from the White Mountains. Over the past two decades, the Koiliaris River watershed has undergone a comprehensive investigation (Kourgialas and Karatzas, 2011; Vozinaki et al., 2011; Sibetheros et al., 2013; Giannakis et al., 2014; Moraetis et al., 2015; Nerantzaki et al., 2015; Vozinaki et al., 2015; Morianou et al., 2017; Yu et al., 2019; Nerantzaki and Nikolaidis, 2020; Lilli et al., 2020a,b). The geological composition of the region, coupled with a significant fault running in a northeast–southwest direction, directs water movement toward the springs within the Koiliaris River Basin (Steiakakis, 2018; Steiakakis et al., 2023). The study area encompasses karst systems with a distinctive characteristic possessing unique hydraulic properties and transmissivities (Kourgialas et al., 2010). The karst area outside the river basin but feeding into it covers 80 km2 (Nerantzaki et al., 2015; Lilli et al., 2020a), while the total length of the river is 36 km.
The main WEF related challenges that need to be addressed focus on three geographic areas within the basin of Koiliaris (Area 1: the western part of the basin, Area 2: the southern part of the basin and Area 3: the northeastern part of the basin) (Figure 1). Area 1 presents intense soil degradation, particularly erosion due to cultivation of olive groves in steep slopes without the development of any terraces. Area 2 presents biodiversity degradation resulting from free-grazing livestock at the higher elevations of the basin and Area 3 presents land degradation due to unsustainable agricultural practices (soil tillage, no organic matter addition to soil, high pesticide and herbicide use). The challenges were extensively presented.
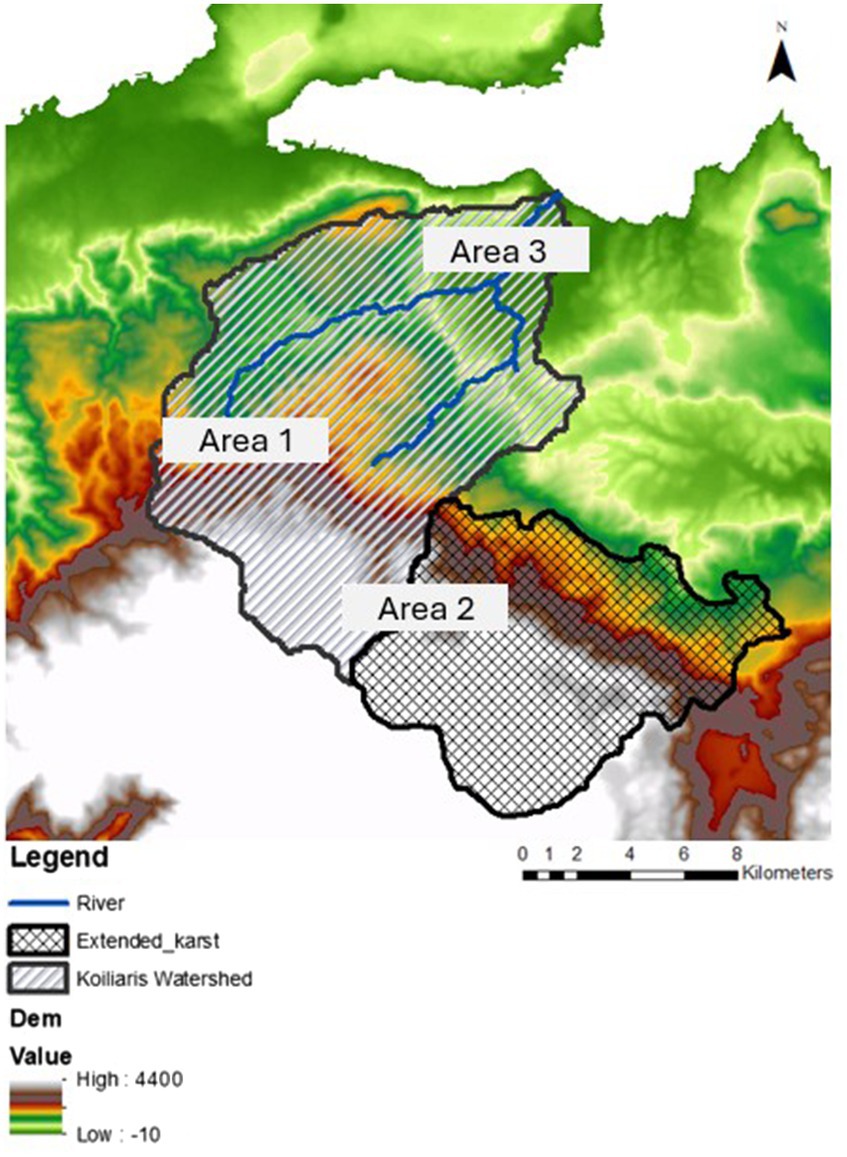
Figure 1. Approximate extent of the areas of the watershed related to the main challenges to be addressed at the Koiliaris River Basin.
The Koiliaris River watershed serves as an illustrative example of a basin that has experienced severe soil and biodiversity degradation (Sibetheros et al., 2013; Moraetis et al., 2015; Nerantzaki et al., 2015). As part of the Prima LENSES project,1 a methodological and practical approach (WEF nexus evaluation framework) was developed for the selection of a suite of solutions that use NBS that affect and improve the WEF nexus. This framework was modified into a user friendly module,2 to allow the selection of NBS and was built on available methodologies and information for selecting NBS (Somarakis et al., 2019; Dimitru and Wendling, 2021). This tool was used to identify NBS alternatives to address the WEF challenges of the Koiliaris River Basin.
2.2 Model description
The SWAT (Soil and Water Assessment Tool) model (Neitsch et al., 2011) is a widely utilized hydrological model designed to simulate and predict the impact of land management practices on water resources at the watershed scale. Developed by the United States Department of Agriculture (USDA), SWAT integrates various components, including hydrology, weather, soil, vegetation, and land use to simulate the complex interactions within a watershed. The model utilizes spatially distributed data on topography, soil properties, weather conditions, and land use to simulate processes such as water flow, sediment transport, nutrient cycling, etc. It’s important to note that the SWAT model cannot simulate karst formation (Nikolaidis et al., 2013). This limitation arises from the assumption that water surpassing the deep aquifer is lost from the system. In karstic formations, water from the deep aquifer contributes to the main river flow through a pothole. To address this, the karstic model was introduced (Nikolaidis et al., 2013), retrieving water from the deep aquifer and directing it into two reservoirs, subsequently feeding the surface flow again. In the aforementioned case study, specifically in the gorge of the watershed where karstic formations exist, the majority of the surface flow passes through a pothole and discharges downstream.
The one-dimensional integrated critical zone (1D-ICZ) model is a mechanistic mathematical model capable of simulating and quantifying key soil functions including food and biomass production, water flow and storage, carbon/nutrient sequestration and biodiversity (Giannakis et al., 2017; Kotronakis et al., 2017). The model couples soil formation (aggregation and disaggregation) and structure with soil hydrology, cycling of nutrients, plant productivity and weathering (Nikolaidis et al., 2014; Kotronakis et al., 2017). The 1D-ICZ model consists of four sub-modules: HYDRUS-1D, CAST, PROSUM and SAFE Weathering. HYDRUS-1D sub-module simulates water flow, heat and solute transport and the chemical weathering sub-module simulates the dissolution kinetics of minerals. PROSUM sub-module simulates the plant dynamics, i.e., biomass production, water and nutrient uptake and litter production of C and N (Nikolaidis et al., 2014; Giannakis et al., 2017; Kotronakis et al., 2017). The Carbon, Aggregation and Structure Turnover (CAST) sub-module is the core model that uses the RothC carbon pools and thus simulates the macro-aggregate formation (around POM) and disruption to form micro-aggregates and silt-clay sized micro-aggregates (Stamati et al., 2013; Giannakis et al., 2017). The CAST model has been used globally (Damma Glacier in Switzerland, Heilongjiang Mollisols in China, Koiliaris and Milia in Greece, Clear Creek in United States, Slavkov Forest in Czech Republic and Marchfeld in Austria) in order to simulate the soil structure, C/N/P dynamics and especially C sequestration (Panakoulia et al., 2017).
2.3 Modeling strategy of NBS impacts
2.3.1 Modeling NBS in Area 1 and 2
In order to mitigate soil erosion and enhance water quality in Area 1, two different NBS, enclosed the establishment of terracing and riparian forest were implemented and assessed through modeling. The expertise of the scientific team working on the Koiliaris River Basin in collaboration with the local stakeholders (Lilli et al., 2020b), has driven to the selection of the proposed NBS, according to the specific challenges. The SWAT model has been previous calibrated (Sibetheros et al., 2013; Nerantzaki et al., 2015; Nerantzaki and Nikolaidis, 2020) for the Koiliaris River Basin regarding the hydrology, sediment transport, and nutrient concentrations. In the context of this study, the simulation was extended until 2020 and the results are presented in the Supplementary Figures S1, S2.
Terraces were introduced into the model by defining the USLE practice factor, which depends on the slope of the selected terrace, the average slope length (TERR_SL), which relates to soil morphology, and the curve number (TERR_CN), which depends on the slope range (Neitsch et al., 2011). These modifications were applied for each hydrologic response unit (HRU) contained in subbasins of the model that comprise Area 1 (9 and 15), corresponding to the Keramianos tributary. The riparian forest was emulated in the SWAT model as filter strips at the HRU level on both sides of the river. The filter strip module was applied to subbasin 9 and 15 which are comprised of agricultural land (AGRL), pasture (PAST) and olive groves (OLIV) land uses. The filter strip related model parameters included the ratio of field area to filter strip area (VFSRATIO), the fraction of the HRU that drains to the most concentrated 10% of the filter strip area (VFSCON), and the fraction of flow within the most concentrated 10% of the filter strip that is fully channelized (VFSCH). In subbasin 9, for the AGRL land use, the average ratio of field area to filter strip area was 2% and for the PAST land use, it was 1%. In subbasin 15, for the OLIV land use and for the PAST land use, the ratio of field area to filter strip area was 2.5 and 0.5, respectively.
To mitigate soil erosion in this area (Area 1), three distinct scenarios were examined. The first scenario entailed the implementation of terraces in the Keramianos tributary that has been identified through sampling surveys as the source of erosion. The second scenario involves the establishment of riparian forests in these subbasins, and the third scenario is the combination of the two approaches.
In the Area 2, the strategy involved discontinuing the free grazing of livestock at high elevations and transitioning to organized caged livestock systems in lower elevations. This strategic shift aimed to alleviate the environmental pressures from livestock grazing in the highlands, allowing in this way the gradual restoration of biodiversity and facilitate the recycling of manure and reuse for agriculture. To model this NBS within the calibrated SWAT, all model operations associated with manure fertilization from sheep and goats in designated area were eliminated.
2.3.2 Modeling NBS in Area 3
The assessment of agroecological practices and the resulting impact on soil ecosystem functions and services was conducted using the 1D-ICZ model for an avocado plantation located (Latitude: 35.43717, Longitude: 24.1427, Elevation: 15 m) in Koiliaris River Basin. Agro ecological practices which are considered NBS, used in the plantation included manure addition, mulching and grass incorporation in the soil, sustainable irrigation practices etc. they have been applied to the field since 2010. The avocado plantation consists of 25 large trees (6-year-old) and 40 smaller ones (4-year-old) irrigated through drip irrigation with a piping system of 25 and 15 drips, respectively. Moreover, the avocado trees were fertilized and each December 10 kg/tree of manure was added to the soil. The model was calibrated to simulate the plant biomass production, carbon/nutrient sequestration, soil formation (aggregation and disaggregation) and soil nutrient concentrations for the period of time 2016–2023. As boundary conditions, monthly time series of air temperature (T, °C), evapotranspiration (ET), precipitation (PCP), irrigation (in m), average daytime photosynthetic active radiation (PAR, μmol/m2/s), fertilization (NO3, NH4, PO4, K in t/ha), manure and organic matter addition (tC/ha) were used. More specifically, the available daily data (T, PCP, PAR) were gap filled and then converted into monthly time series. The input time series of ET were calculated using the Penman–Monteith equation for the period of available data (2019–2022) and then gap filled to complete the 2016–2023 time series. To simulate soil structure dynamics, water stable aggregate (WSA) fractionation data for the years 2016, 2019 and 2023 were used. For the years 2016 and 2019, two soil samples (0–5 and 15–20 cm) were collected and analyzed in duplicates and aggregated to determine the WSA Fractionation for these years. For the year 2023, triplicate soil samples (0–20 cm) were collected from the avocado plantation and analyzed. The method used to separate the soil is analytically described by Elliott (1986) and Lichter et al. (2008). The available nutrient concentrations measured at the well located within the field were compared to the simulated nutrient concentrations of the fourth soil layer (30–40 cm) as the soil profile was defined to be at 40 cm, discretized in five nodes and four layers. The groundwater in the area is shallow and the water depth varies between 1–2 m below ground. Once the model is calibrated, then the impact of agroecological practices on soil functions and nutrient emissions can be assessed.
3 Results
3.1 Terrace and riparian forest simulation
To fully understand how the SWAT model simulates terraces, a sensitivity analysis was conducted on key model parameters (TERR_CN, TERR_SL, USLE practice factor). The tested range for the TERR_CN was between 40 and 45. The upper value of 45 was obtained from the hydrologic calibration which depicts the current unprotected slope conditions and the lower value from the scientific literature. The values of average slope length (TERR_SL) chosen to simulate were 3, 4, 5, 6, 10, and 15 meters while five categories of slopes were chosen: 0–2%, 2–8%, 12–16%, 16–20%, and 20–25%. Supplementary Figure S3 presents the results of the calculated SYLD from the model, varying the slope length and USLE practice factor in subbasins 9 and 15 where terracing was applied. The values defined for the implementation of the filter strip in specific HRUs were calculated under the assumption that the width of the riparian forest on both sides of the channel is 40 m. Table 1 shows the values of selected parameters used for the simulation of the terraces and the filter strip.
Table 2 presents the average sediment load, the range of sediment load and the percentage reduction for each scenario. In subbasin 9, the average sediment load was 0.175, 0.012 and 0.011 t/ha for the first, second and third scenario respectively, while the average sediment load was 0.176 t/ha for the case of non-implementing NBS. The percentage sediment reduction was calculated to 1, 93 and 94% for the first, second and third scenario, respectively, (Table 2). The results suggest that the most efficient individual NBS in subbasin 9 is the implementation of riparian forest. In subbasin 15, the average sediment load was 0.270, 3.147 and 0.168 t/ha for the first, second and third scenario respectively, while the average sediment load was 5.337 t/ha for the case of non-implementing NBS. The percentage sediment reduction was calculated to 95, 41 and 97% for the first, second and third scenario, respectively, (Table 2). The results suggest that the most efficient individual NBS in subbasin 15 is the implementation of terraces. The third scenario, combining the individual NBS, demonstrates the highest percentage of sediment reduction in both subbasins (Table 2). The results suggest that a combination of terraces and the creation of a riparian forest can reduce significantly (up to 97% reduction) the sediment loads exported from the basin.
3.2 Discontinuation of livestock free grazing impact
The exclusion of livestock from the upland pasture areas was simulated by discontinuing the input of manure in these HRUs. Table 3 presents the annual average nitrate export from the Koiliaris River Basin, comparing scenarios with and without livestock activity. According to the calculations performed, the mean annual nitrate export per hectare associated with livestock activity, amounted to 9.8 kg/ha/year, whereas in the absence of livestock activity, the corresponding figure was 7.9 kg/ha/year. The observed reduction in nitrate levels, as depicted in Table 3, is approximately 19%. The results illustrate the impact of livestock activities on water quality.
3.3 Agroecological practices assessment
Figures 2–4 and Supplementary Figure S4 present the results of the simulation of the 1D-ICZ regarding biomass production, carbon/nutrient sequestration, soil structure and geochemistry. Figure 2 shows the evolution of the limiting factors of avocado growth. It is evident that temperature affects plant growth the most. This is consistent with other studies which suggest that temperature affects growth and concentration of dry matter in avocados (Lahav and Trochoulias, 1982). Avocados’ optimal temperature for growth is between 20–25°C. More specifically, the optimal air temperature during nighttime is greater than 10°C and the optimal range during daytime fluctuates from 20 to 30°C (Bhore et al., 2021). At high temperatures (above 30°C) root growth and dry matter production decreases and at low temperatures enzymatic activity and metabolic processes decline (Lahav and Trochoulias, 1982; Tzatzani et al., 2023). The reduction of dry matter results in low nutrition worthy avocados and the deceleration of enzymatic activity slows down maturation (Tzatzani et al., 2023).
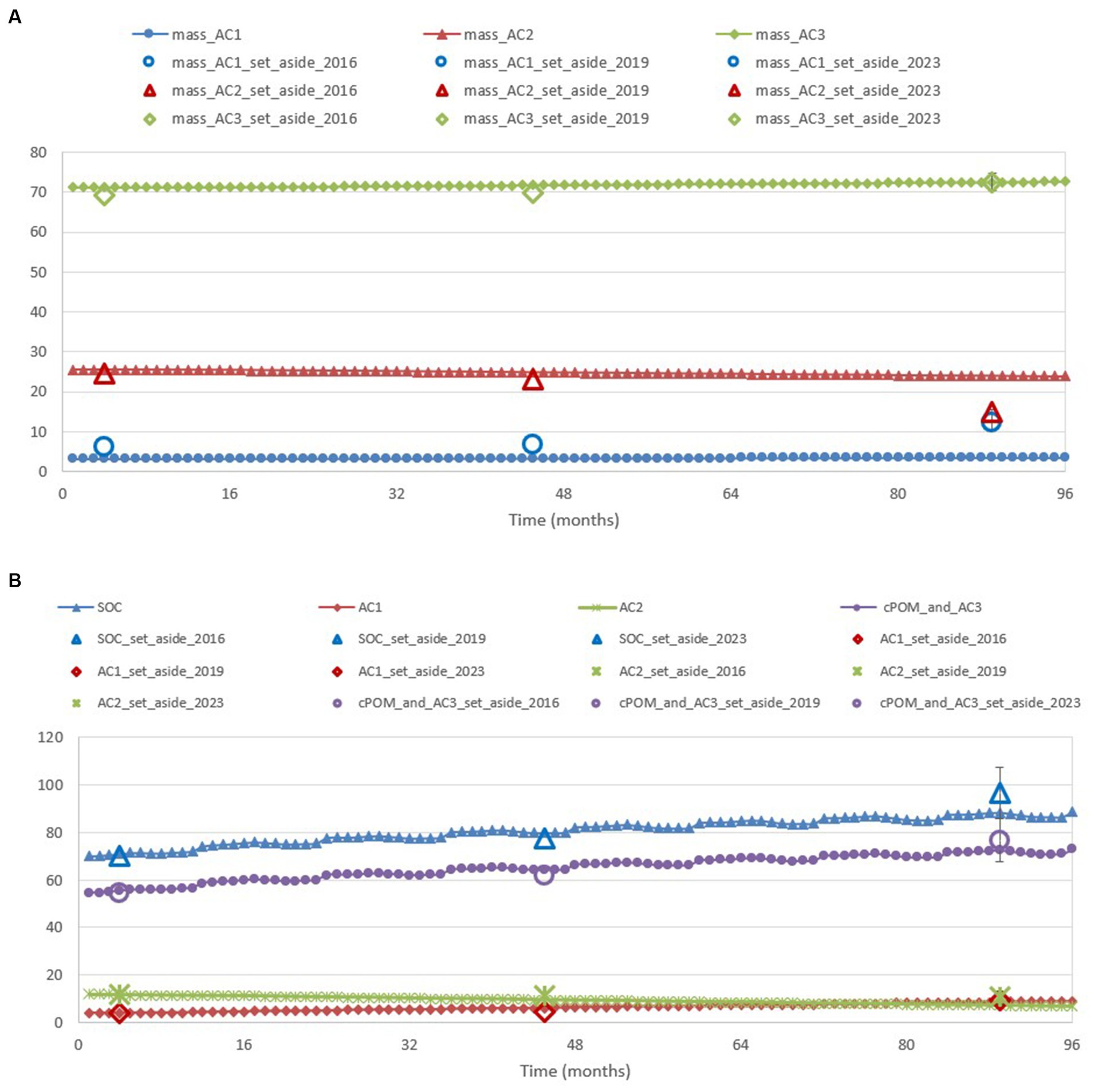
Figure 4. Comparison of simulated and measured (A) WSA (%) and (B) SOC and OC in AC1, AC2, cPOM and AC3 (tC/ha).
Figure 3 illustrates the simulated annual gross primary production (GPP) compared to the field measurement of the year 2023. To simulate GPP, the avocado tree is considered to be at steady state regarding its biomass production. The GPP remains stable over the years with the average annual GPP to be 1474.6 gC/m2 (Figure 3). Figure 4A presents the comparison of the simulated and measured WSA mass contained in silt-clay sized micro-aggregates (AC1), micro-aggregates (AC2) and macro-aggregates (AC3). One can observe that the majority of WSA mass (71.9%) is contained in the macro-aggregates (>250 μm). The WSA mass contained in the micro-aggregates (53–250 μm) is 24.7% and the WSA mass contained in the silt-clay sized micro-aggregates (<53 μm) is 3.4%. Figure 4B shows the comparison of SOC and the organic carbon (OC) contained in AC1, AC2, cPOM (coarse particulate organic matter) and AC3 between the model and the field (set aside). SOC increases from 70.1 to 88.6 tC/ha during the period 2016–2023. Most of the OC is contained in cPOM and AC3 and the least amount of OC is contained in AC1. The OC contained in AC1 increases from 4.0 to 9.0 tC/ha, in AC2 decreases from 11.6 to 6.7 tC/ha and in cPOM and AC3 increases from 54.5 to 72.9 tC/ha. Supplementary Figure S4 shows the comparison of TOC (Total OC), IC (Inorganic carbon), TN (Total N), DIN (Dissolved Inorganic N), NH4 − N, PO4 − P, , , , , , and well measurements with the daily simulated nutrients concentrations for the fourth soil layer (30–40 cm) in mol/L. The results suggest that the 1D-ICZ model is capable in simulating the soil geochemical conditions as well as the whole soil-plant-water system.
The impact of agroecological practices on the plant-water-soil ecosystem is presented in Table 4 which is a summary of the ecosystem services derived from such management practices. The majority of WSA were found in macro-aggregates (71.9%) while the WSA in micro-aggregates (AC2) and silt-clay sized micro-aggregates (AC1) account for 24.7 and 3.4%, respectively. The soil is sandy (75.9% sand) and the C to N ratio is 13. The biomass production is 14.7 tC/ha/year and the C sequestration is 80.7 tC/ha (with the cPOM accounting for the 80.5% of the below ground C content). The N sequestration estimated at 6.2 tN/ha and the CO2 emissions at 8.3 tC/ha/year. The leaching of the chemicals TOC, TN, PO4-P and K to groundwater calculated to be 1.3, 14.6 2.2, 7.1 g/m2, respectively (see Figure 4).
4 Discussion
Different approaches have been suggested to integrate and mainstream the ecosystem dimension within the WEF nexus frameworks. Among them, strong emphasis has been given to NBS defined as “actions inspired by, supported by, or copied from nature, that deploy various natural features and processes, are efficient resources and adapted to systems in diverse spatial areas, facing social, environmental, and economic challenges.” In this research, the use of two models were illustrated for assessing ecosystem services provided by NBS which in turn can be used for the optimization of the WEF nexus at the watershed scale, the examination of different alternatives and the evaluation of their trade-offs. These tools are necessary in order to simulate the impact of NBS, quantify key performance indicators (KPIs) that relate to the effectiveness of NBS as well as the services provided by them.
We focused on four different types of NBS that can be widely used to improve the WEF nexus at the basin scale.
Terraces. The terraces are widespread in hilly-mountainous areas, representing an ancient anthropogenic landscape modification for agricultural purposes. Terracing technology is often developed to enable and to prevent land degradation and erosion simultaneously. Terraces belong to the soil and water conservation measures as they impact on erosion reduction, slope stabilization improvement and water levels management; as a result, they fit perfectly into the NBS definition and scope (Paliaga et al., 2021).
Livestock management. Land use practices (especially livestock grazing) have shown that impact adversely surface and ground water quality (Nikolaidis et al., 2013) and levels of erosion (Panagos et al., 2014). Proper management of free livestock grazing is a water management action, that considers NBS and according to the degree of intervention and the level of engineering is classified under the second type for sustainability and multifunctionality of managed ecosystems (Somarakis et al., 2019).
Riparian forests. Restoring ecosystems and their functions constitutes a primary goal of NBS, since degradation affects the delivery of ecosystem services, thus affecting human livelihoods. For this reason, the restoration of forests (and riparian forests) is defined as the ongoing process of regaining ecological functionality and enhancing human well-being across deforested or degraded forest landscapes (IUCN and WRI, 2014; Bhattacharjee, 2020). Lilli et al. (2020b) highlighted a series of co-benefits in a case study of a Mediterranean riparian forest restoration, proving that it was an exemplary example of a functional ecosystem restoration that can be used for flood and erosion protection in many parts of the world.
Land management. Finally, agroecological practices contribute to improving the sustainability of agroecosystems while being based on various ecosystem services such as nutrient cycling, biological nitrogen fixation, natural regulation of pests, soil and water conservation, farming system resilience, biodiversity conservation, land degradation and carbon sequestration (Wezel et al., 2014). Common agroecological practices include reduced tillage, elimination of chemical synthesized fertilizers and pesticides, and use of biofertilizers, organic fertilization, drip irrigation and carbon addition to soil and constitute examples of activities associated with NBS in agricultural landscapes that address agricultural production (Wilhelm, 2021; Zeng et al., 2023).
The agroecological practices, used in the plantation of the experimental field of this research included manure addition, mulching and grass incorporation in the soil and sustainable irrigation practices, and they have been applied to the field since 2010. The experimental field was a former orange tree plantation which had been abandoned for approximately 25 years. After this and within the framework of the EU FP7-SoilTrEC project, a 4-year (2011–2014) field-scale horticulture experiment was conducted in this field, where tomato plants were grown using different treatments of commercial mineral fertilizers, compost, manure, and a 30% manure—70% compost amendment (Kotronakis et al., 2017). For the past 7 years, the experimental field has been planted with avocado and the farmer follows the aforementioned agroecological practices. The Technical University of Crete is conducting studies for the quantification of ecosystem services to assess the impact of agroecological practices and using modeling to quantify the soil threats and biomass growth.
5 Conclusion
Sustainable land management requires the maximization of the efficacy of soil ecosystem functions (and the related services) as well as the minimization of soil threats. Soil ecosystem functions include biomass production, carbon and nutrient sequestration, water filtration and transformation and biodiversity. Whereas soil threats include loss of soil carbon and nutrients, loss of biodiversity, erosion and soil compaction (Nikolaidis, 2011). In addition, sustainable land management has to be considered in terms of optimizing the WEF nexus necessitating the use of hydrologic and geochemical models that assess not only the WEF nexus, but also soil ecosystem functions and threats.
In this research, four different NBS (terraces, riparian forest, livestock management and agro ecological practices) were assessed in terms of their impact to WEF nexus. All four NBS can directly or indirectly improve soil ecosystem functions and reduce soil threats. The NBS of terraces and riparian forest affect soil erosion. Specifically, terraces can reduce the sediment load up to 95%, riparian forest implementation can reduce this load up to 93%, while a combination of these NBS can reduce it up to 97%. Livestock management has impact on soil and water quality by reducing the nitrate levels at about 19%. The NBS of agro ecological practices impact biomass production, carbon and nutrient sequestration, soil structure and geochemistry. The impact of agroecological practices on the plant-water-soil ecosystem and the resulting ecosystem services derived from such management practices were assessed with the 1D-ICZ model. Agroecological practices were shown to increase the organic carbon sequestered in the soil, increase the WSA which are linked directly to soil health and fertility while maintaining a healthy biomass production. The below ground C sequestration is almost 6 time higher than the above ground plant production indicating the importance of soil carbon amendments in mitigating the impacts of climate change. In addition, the results of soil fractionation suggest that this carbon is fairly stable with a very long turnover time since more than 80% of it is in the particulate form.
Finally, the leaching of the chemicals TOC, TN, PO4-P and K to groundwater calculated to be 1.3, 14.6 2.2, 7.1 g/m2, respectively, which is only a small fraction of the total loads to the system.
The hydrologic and ecosystem models used in this work were able to quantify the direct impact of NBS and assess their effectiveness. Both the Karst-SWAT and the 1D-ICZ model were shown to be capable of simulating successfully the ecosystem services derived from the NBS application. This work showed that modeling tools as such as those used in this study can be used for the optimization of the WEF nexus and thus for the evaluation of the effectiveness of different NBS scenarios.
Data availability statement
The original contributions presented in the study are included in the article/Supplementary material, further inquiries can be directed to the corresponding author.
Author contributions
AM: Data curation, Resources, Visualization, Writing – original draft. EK: Data curation, Resources, Visualization, Writing – review & editing. ML: Conceptualization, Methodology, Resources, Writing – review & editing. DE: Data curation, Writing – review & editing. NN: Conceptualization, Funding acquisition, Methodology, Project administration, Supervision, Writing – review & editing.
Funding
The author(s) declare that financial support was received for the research, authorship, and/or publication of this article. This research was conducted in the framework of the project LENSES- “LEarning and action alliances for NexuS EnvironmentS in an uncertain future,” funded by the European Union’s PRIMA Programme, under Grant Agreement No. 2041.
Conflict of interest
The authors declare that the research was conducted in the absence of any commercial or financial relationships that could be construed as a potential conflict of interest.
Publisher’s note
All claims expressed in this article are solely those of the authors and do not necessarily represent those of their affiliated organizations, or those of the publisher, the editors and the reviewers. Any product that may be evaluated in this article, or claim that may be made by its manufacturer, is not guaranteed or endorsed by the publisher.
Supplementary material
The Supplementary material for this article can be found online at: https://www.frontiersin.org/articles/10.3389/frwa.2024.1386925/full#supplementary-material
Footnotes
References
Albrecht, T. R., Crootof, A., and Scott, C. A. (2018). The water-energy-food nexus: a systematic review of methods for nexus assessment. Environ. Res. Lett. 13, 1–26. doi: 10.1088/1748-9326/aaa9c6
Avellan, T., Roidt, M., Emmer, A., von Koerber, J., Schneider, P., and Raber, W. (2017). Making the water-soil-waste nexus work: framing the boundaries of resource flows. Sustainability 9:1881. doi: 10.3390/su9101881
Bhattacharjee, A. (2020). “Forest landscape restoration as a NBS strategy for achieving Bonn challenge pledge: lessons from India’s restoration efforts” in Nature-based solutions for resilient ecosystems and societies. Disaster resilience and green growth. eds. S. Dhyani, A. Gupta, and M. Karki (Singapore: Springer)
Bhore, S. J., Ochoa, D. S., Houssari, A. A, Zelaya, A. L., Yang, R., Chen, Z., et al. (2021). The avocado (Persea americana Mill.): a review and sustainability perspectives: Preprints. 2021120523. Available at:. doi: 10.20944/preprints202112.0523.v1
Canessa, C., Vavvos, A., Triliva, S., Kafkalas, I., Vrachioli, M., and Sauer, J. (2022). Implementing a combined Delphi and Focus Group qualitative methodology in nexus research designs—the case of the WEFE nexus in Apokoronas, Crete. PLoS One 17:e0271443. doi: 10.1371/journal.pone.0271443
Carvalho, P. N., Finger, D. F., Masi, F., Cipolletta, G., Oral, H. V., Tóth, A., et al. (2022). Nature-based solutions addressing the water-energy-food nexus: review of theoretical concepts and urban case studies. J. Clean. Prod. 338:130652. doi: 10.1016/j.jclepro.2022.130652
Chambers, J. C., Allen, C. R., and Cushman, S. A. (2019). Operationalizing ecological resilience concepts for managing species and ecosystems at risk. Front. Ecol. Evol. 7:241. doi: 10.3389/fevo.2019.00241
Dimitru, A., and Wendling, L. (Eds.) (2021). Evaluating the impact of nature-based solutions: a handbook for practitioners European Commission Directorate-General for Research and Innovation.
Ding, T., Fang, L., Chen, J., Ji, J., and Fang, Z. (2023). Exploring the relationship between water-energy-food nexus sustainability and multiple ecosystem services at the urban agglomeration scale. Sustain. Prod. Consum. 35, 184–200. doi: 10.1016/j.spc.2022.10.028
Elliott, E. T. (1986). Aggregate structure and carbon, nitrogen, and phosphorus in native and cultivated soils. Soil Sci. Soc. Am. J. 50, 627–633. doi: 10.2136/sssaj1986.03615995005000030017x
Fader, M., Cranmer, C., Lawford, R., and Engel-Cox, J. (2018). Toward an understanding of synergies and trade-offs between water, energy, and food SDG targets. Front. Environ. Sci. 6:112. doi: 10.3389/fenvs.2018.00112
Faivre, N., Fritz, M., Freitas, T., Boissezon, B., and Vandewoestijne, S. (2017). Nature-based solutions in the EU: innovating with nature to address social, economic and environmental challenges. Environ. Res. 159, 509–518. doi: 10.1016/j.envres.2017.08.032
FAO (2014). The water-energy-food nexus a new approach in support of food security and sustainable agriculture. Food and Agriculture Organization of the United Nations (FAO): Rome.
Flammini, A., Puri, M., Pluschke, L., and Dubois, O. (2014). “Walking the nexus talk: assessing the water-energy-food nexus in the context of sustainable energy for all initiative” in Environment and natural resources management working paper No. 58 (Food and Agriculture Organization of the United Nations (FAO)) Available at: https://www.fao.org/3/i3959e/i3959e.pdf
Giannakis, G. V., Nikolaidis, N. P., Valstar, J., Rowe, E. C., Moirogiorgou, K., Kotronakis, E., et al. (2017). “Integrated critical zone model (1D-ICZ): a tool for dynamic simulation of soil functions and soil structure” in Quantifying and managing soil functions in earth’s critical zone: combining experimentation and mathematical modelling, advances in agronomy (Academic Press), 277–314.
Giannakis, G. V., Panakoulia, S. K., Nikolaidis, N. P., and Paranychianakis, N. V. (2014). Simulating soil fertility restoration using CAST model. Procedia Earth Planet. Sci. 10:325. doi: 10.1016/j.proeps.2014.08.027
Hoff, H. (2011). Understanding the nexus. Background Paper for the Bonn 2011 Nexus Conference: The water, Energy and Food Security Nexus. Stockholm: Stockholm Environment Institute, Stockholm.
Hoolohan, C., Larkin, A., McLachlan, C., Falconer, R., Soutar, I., Suckling, J., et al. (2018). Engaging stakeholders in research to address water–energy–food (WEF) nexus challenges. Sustain. Sci. 13, 1415–1426. doi: 10.1007/s11625-018-0552-7
Hutchins, M., Qu, Y., Seifert-Dahnn, I., and Levin, G. (2024). Comparing likely effectiveness of urban nature-based solutions worldwide: the example of riparian tree planting and water quality. J. Environ. Manag. 351:119950. doi: 10.1016/j.jenvman.2023.119950
IUCN and WRI (2014). A guide to the restoration opportunities assessment methodology (ROAM): assessing forest landscape restoration opportunities at the national or sub-national level. Eds. Stewart Maginnis, Lars Laestadius, Michael Verdone, Sean DeWitt, Carole Saint-Laurent, Jennifer Rietbergen-McCracken, Daniel M. P. Shaw Gland, Switzerland. Available at: https://portals.iucn.org/library/sites/library/files/documents/2014-030.pdf
Karnib, A. (2017). A quantitative assessment framework for water, energy and food nexus. Comput. Water Energy Environ. Eng. 6, 11–23. doi: 10.4236/cweee.2017.61002
Kolokotsa, D., Lilli, A. A., Lilli, M. A., and Nikolaidis, N. P. (2020). On the impact of nature-based solutions on citizens’ health & well being. Energy Build. 229:110527. doi: 10.1016/j.enbuild.2020.110527
Kotronakis, E., Giannakis, G. V., Nikolaidis, N. P., Rowe, E. C., Valstar, J., Paranychianakis, N. V., et al. (2017). “Modeling the impact of carbon amendments on soil ecosystem functions using the 1D-ICZ model” in Quantifying and managing soil functions in earth’s critical zone: combining experimentation and mathematical modelling, advances in agronomy (Academic Press), 315–352.
Kourgialas, N. N., and Karatzas, G. P. (2011). Flood management and a GIS modelling method to assess flood-hazard areas—a case study. Hydrol. Sci. J. 56, 212–225. doi: 10.1080/02626667.2011.555836
Kourgialas, N. N., Karatzas, G. P., and Nikolaidis, N. P. (2010). An integrated framework for the hydrologic simulation of a complex geomorphological river basin. J. Hydrol. 381, 308–321. doi: 10.1016/j.jhydrol.2009.12.003
Lahav, E., and Trochoulias, T. (1982). The effect of temperature on growth and dry matter production of avocado plants. Aust. J. Agric. Res. 33, 549–558. doi: 10.1071/AR9820549
Laspidou, C. S., Kofinas, D. T., Mellios, N. K., and Witmer, M. (2018). Modelling the water-energy-food-land use-climate nexus: the nexus tree approach. Proceedings 2:617. doi: 10.3390/proceedings2110617
Liao, Κ. W., Thedy, J., and Tai, C. C. (2024). Fluvial flood adaptation using nature-based solutions: a comprehensive and effective assessment of hydro-meteorological risks. Sci. Total Environ. 912:169329. doi: 10.1016/j.scitotenv.2023.169329
Lichter, K., Govaerts, B., Six, J., Sayre, K. D., Deckers, J., and Dendooven, L. (2008). Aggregation and C and N contents of soil organic matter fractions in a permanent raised-bed planting system in the highlands of central Mexico. Plant Soil 305, 237–252. doi: 10.1007/s11104-008-9557-9
Lilli, M. A., Efstathiou, D., Moraetis, D., Schuite, J., Nerantzaki, S. D., and Nikolaidis, N. P. (2020a). A multi-disciplinary approach to understand hydrologic and geochemical processes at Koiliaris critical zone observatory. Water 12:2474. doi: 10.3390/w12092474
Lilli, M. A., Nerantzaki, S. D., Riziotis, C., Kotronakis, M., Efstathiou, D., Kontakos, D., et al. (2020b). Vision-based decision-making methodology for riparian forest restoration and flood protection using nature-based solutions. Sustainability 12:3305. doi: 10.3390/su12083305
Liu, Q. (2016). Interlinking climate change with water-energy-food nexus and related ecosystem processes in California case studies. Ecol. Process. 5:14. doi: 10.1186/s13717-016-0058-0
Maes, J., and Jacobs, S. (2015). Nature-based solutions for Europe’s sustainable development. Conserv. Lett. 10, 121–124. doi: 10.1111/conl.12216
McGrane, S. J., Acuto, M., Artioli, F., Chen, P.-Y., Comber, R., Cottee, J., et al. (2019). Scaling the nexus: towards integrated frameworks for analysing water, energy and food. Geogr. J. 185, 419–431. doi: 10.1111/geoj.12256
Melloni, G., Turetta, A. P. D., Bonatti, M., and Sieber, S. A. (2020). Stakeholder analysis for a water-energy-food nexus evaluation in an Atlantic forest area: implications for an integrated assessment and a participatory approach. Water 12:1977. doi: 10.3390/w12071977
Mohtar, R. H., and Daher, B. (2016). Water-energy-food nexus framework for facilitating multi-stakeholder dialogue. Water Int. 41, 655–661. doi: 10.1080/02508060.2016.1149759
Moraetis, D., Paranychiannakis, N. V., Nikolaidis, N. P., Banwart, S. A., Rousseva, S., Kercheva, M., et al. (2015). Sediment provenance, soil development, and carbon content in fluvial and manmade terraces at Koiliaris River critical zone observatory. J. Soils Sediments 15, 347–364. doi: 10.1007/s11368-014-1030-1
Morianou, G., Kourgialas, N. N., Karatzas, G. P., and Nikolaidis, N. P. (2017). Assessing hydro-morphological changes in Mediterranean stream using curvilinear grid modeling approach—climate change impacts. Earth Sci. Inform. 11, 205–216. doi: 10.1007/s12145-017-0326-2
Neitsch, S. L., Arnold, J. G., Kiniry, J. R., and Williams, J. R. (2011). Soil and water assessment tool theoretical documentation version 2009. Texas Water Resources Institute, College Station, Texas.
Nerantzaki, S. D., Giannakis, G. V., Efstathiou, D., Nikolaidis, N. P., Sibetheros, I. A., Karatzas, G. P., et al. (2015). Modeling suspended sediment transport and assessing the impacts of climate change in a Karstic Mediterranean watershed. Sci. Total Environ. 538, 288–297. doi: 10.1016/j.scitotenv.2015.07.092
Nerantzaki, S. D., and Nikolaidis, N. P. (2020). The response of three Mediterranean karst springs to drought and the impact of climate change. J. Hydrol. 591, 125296–125217. doi: 10.1016/j.jhydrol.2020.125296
Nikolaidis, N. (2011). Human impacts on soils: tipping points and knowledge gaps. Appl. Geochem. 26, S230–S233. doi: 10.1016/j.apgeochem.2011.03.111
Nikolaidis, N. P., Bouraoui, F., and Bidoglio, G. (2013). Hydrologic and geochemical modeling of a karstic Mediterranean watershed. J. Hydrol. 477, 129–138. doi: 10.1016/j.jhydrol.2012.11.018
Nikolaidis, N. P., Valstar, J., Rowe, E. C., Moirogiorgou, K., Kotronakis, E., Giannakis, G. V., et al. (2014). Integrated critical zone model (1D-ICZ model)—a tool for dynamic simulation of soil functions and soil structure: technical manual. School of Environmental Engineering Technical University of Crete, Chania, Greece. Available at: http://www.herslab.tuc.gr/downloads/1D-ICZ-model
Paliaga, G., Faccini, F., Luino, F., Turconi, L., and Vojinovic, Z., (2021). The role of man-made terraces as NBS measure for geo-hydrological risk reduction in the Portofino Park (Italy) - H2020 RECONECT project. vEGU21, the 23rd EGU General Assembly, April 19–30, 2021. EGU21-3977
Panagos, P., Christos, K., Cristiano, B., and Ioannis, G. (2014). Seasonal monitoring of soil erosion at regional scale: an application of the G2 model in Crete focusing on agricultural land uses. Int. J. Appl. Earth Obs. Geoinf. 27, 147–155. doi: 10.1016/j.jag.2013.09.012
Panakoulia, S. K., Nikolaidis, N. P., Paranychianakis, N. V., Menon, M., Schiefer, J., Lair, G. J., et al. (2017). “Factors controlling soil structure dynamics and carbon sequestration across different climatic and lithological conditions” in Quantifying and managing soil functions in earth’s critical zone: combining experimentation and mathematical modelling, advances in agronomy (Academic Press), 241–276.
Pittock, J., Orr, S., Stevens, L., Aheeyar, M., and Smith, M. (2015). Tackling trade-offs in the nexus of water, energy and food. Aquat. Proc. 5, 58–68. doi: 10.1016/j.aqpro.2015.10.008
Purwanto, A., Sušnik, J., Suryadi, F. X., and de Fraiture, C. (2021). Water-energy-food nexus: critical review, practical applications, and prospects for future research. Sustainability 13:1919. doi: 10.3390/su13041919
Sánchez-Zarco, X. G., and Ponce-Ortega, J. M. (2023). Water-energy-food-ecosystem nexus: an optimization approach incorporating life cycle, security and sustainability assessment. J. Clean. Prod. 414, 137534–137512. doi: 10.1016/j.jclepro.2023.137534
Sibetheros, I. A., Nerantzaki, S. D., Efstathiou, D., Giannakis, G., and Nikolaidis, N. P. (2013). Sediment transport in the Koiliaris river of Crete. Proc. Technol. 8, 315–323. doi: 10.1016/j.protcy.2013.11.042
Simpson, G. B., and Jewitt, G. P. (2019). The development of the water-energy-food nexus as a framework for achieving resource security: a review. Front. Environ. Sci. 7, 1–9. doi: 10.3389/fenvs.2019.00008
Somarakis, G., Stagakis, S., and Chrysoulakis, N. (2019). ThinkNature nature-based solutions handbook.
Stamati, F. E., Nikolaidis, Ν. P., Banwart, S., and Blum, W. E. H. (2013). A coupled carbon, aggregation, and structure turnover (CAST) model for topsoils. Geoderma 211-212, 51–64. doi: 10.1016/j.geoderma.2013.06.014
Steiakakis, E. (2018). Evaluation of exploitable groundwater reserves in karst terrain: a case study from Crete, Greece. Geosciences 8:19. doi: 10.3390/geosciences8010019
Steiakakis, E., Vavadakis, D., and Mourkakou, O. (2023). Groundwater vulnerability and delineation of protection zones in the discharge area of a karstic aquifer—application in Agyia’s Karst System (Crete, Greece). Water 15:231. doi: 10.3390/w15020231
Sun, L., Niu, D., Yu, M., Li, M., Yang, X., and Ji, Z. (2022). Integrated assessment of the sustainable water-energy food nexus in China: case studies on multi-regional sustainability and multi-sectoral synergy. J. Clean. Prod. 334, 130235–130220. doi: 10.1016/j.jclepro.2021.130235
Tzatzani, T. T., Morianou, G., Tül, S., and Kourgialas, N. N. (2023). Air temperature as a key indicator of avocado (Cvs. Fuerte, Zutano, Hass) maturation time in Mediterranean climate areas: the case of Western Crete in Greece. Agriculture 13:1342. doi: 10.3390/agriculture13071342
Unguendoli, S., Germano-Biolchi, L., Aguzzi, M., Ayyappan-Pillai, U. P., Alessandri, J., and Valentini, A. (2023). A modeling application of integrated nature based solutions (NBS) for coastal erosion and flooding mitigation in the Emilia-Romagna coastline (Northeast Italy). Sci. Total Environ. 867, 161357–21161357. doi: 10.1016/j.scitotenv.2022.161357
United Nations Environment Programme. (2022). Nature-based solutions: opportunities and challenges for scaling up. Nairobi Nature-based Solutions: Opportunities and Challenges for Scaling Up. Available at: https://wedocs.unep.org/20.500.11822/40783
Vozinaki, A., Karatzas, G. P., Sibetheros, I. A., and Varouchakis, E. A. (2015). An agricultural flash flood loss estimation methodology: the case study of the Koiliaris basin (Greece), February 2003 flood. Nat. Hazards 79, 899–920. doi: 10.1007/s11069-015-1882-8
Vozinaki, A. E., Kourgialas, N., and Karatzas, G. P. (2011). An Integrated Surface and Groundwater Modeling System for Flood Loss Estimation. A Field Application in the Koiliaris River Basin in Crete – Greece. Proceedings of the 12th International Conference on Environmental Science and Technology (CEST2011), Greece, September 8–10, 2011.
Walker, S., Jacobs-Mata, I., Fakudze, B., Phahlane, M. O., and Masekwana, N. (2022). “Applying the WEF nexus at a local level: a focus on catchmen level” in Water-energy-food nexus narratives and resource securities (Amsterdam: Elsevier), 111–144.
Wezel, A., Casagrande, M., Celette, F., Vian, J. F., Ferrer, A., and Peigné, J. (2014). Agroecological practices for sustainable agriculture. A review. Agron. Sustain. Dev. 34, 1–20. doi: 10.1007/s13593-013-0180-7
Wilhelm, F. M. (2021). Nature-based solutions in agriculture: sustainable management and conservation of land, water, and biodiversity FAO and The Nature Conservancy.
Wu, L., Elshorbagy, A., Pande, S., and Zhuo, L. (2021). Trade-offs and synergies in the water-energy food nexus: the case of Saskatchewan, Canada. Resour. Conserv. Recycl. 164, 105192–105114. doi: 10.1016/j.resconrec.2020.105192
Yu, X., Moraetis, D., Nikolaidis, N. P., Li, B., Duffy, C., and Liu, B. (2019). A coupled surface-subsurface hydrologic model to assess groundwater flood risk spatially and temporally. Environ. Model. Softw. 114, 129–139. doi: 10.1016/J.ENVSOFT.2019.01.008
Keywords: ecosystem services, hydrological modeling, geochemical modeling, land management, soil ecosystem functions, soil threats
Citation: Maragkaki A, Koukianaki EA, Lilli MA, Efstathiou D and Nikolaidis NP (2024) Optimizing the water-ecosystem-food nexus using nature-based solutions at the basin scale. Front. Water. 6:1386925. doi: 10.3389/frwa.2024.1386925
Edited by:
Jose Gonzalez-Piqueras, University of Castilla-La Mancha, SpainReviewed by:
Umberto Fratino, Politecnico di Bari, ItalyAntonio Lo Porto, National Research Council (CNR), Italy
Copyright © 2024 Maragkaki, Koukianaki, Lilli, Efstathiou and Nikolaidis. This is an open-access article distributed under the terms of the Creative Commons Attribution License (CC BY). The use, distribution or reproduction in other forums is permitted, provided the original author(s) and the copyright owner(s) are credited and that the original publication in this journal is cited, in accordance with accepted academic practice. No use, distribution or reproduction is permitted which does not comply with these terms.
*Correspondence: Maria A. Lilli, bWFyaWFsaWxsaTAyQGdtYWlsLmNvbQ==;bWxpbGxpQHR1Yy5ncg==