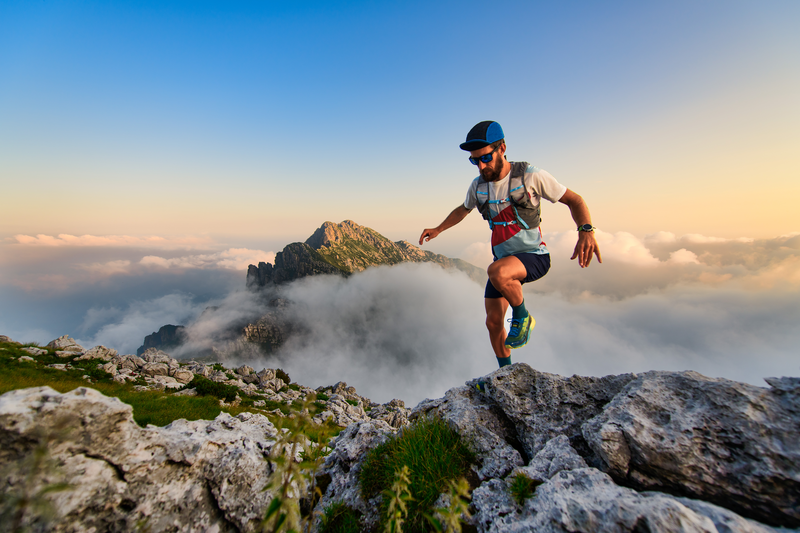
95% of researchers rate our articles as excellent or good
Learn more about the work of our research integrity team to safeguard the quality of each article we publish.
Find out more
REVIEW article
Front. Water , 07 February 2024
Sec. Water and Human Systems
Volume 6 - 2024 | https://doi.org/10.3389/frwa.2024.1301648
This article is part of the Research Topic Innovative Treatment Technologies for Sustainable Water and Wastewater Management View all 5 articles
The presence of arsenic in water bodies poses a significant risk to both human health and the environment. Arsenic (As) contamination in water sources is a global environmental concern caused by both natural processes and human activities. Due to its toxic and persistent nature, arsenic has detrimental effects on ecosystems and human wellbeing. This study aimed to elucidate the mechanisms behind arsenic accumulation in water bodies. In aquatic environments, arsenic concentrations in drinking water have been reported as high as 1,320 μg/L (Nicaragua), while groundwater levels exceeded 5,000 μg/L (Thailand), and wastewater contained up to 134,000 μg/L (landfill leachate in Brazil). Furthermore, bioaccumulation of arsenic (μg/g) in fish species ranges from 0.4 (catfish in the Paraná River Delta, Brazil) to 362 (Pteromylaeus bovinus, Northern Adriatic Sea). Recent research has predominantly focused on removing arsenic from aqueous solutions through adsorption methods. Notably, nanoparticle adsorbents and graphene-based adsorbents demonstrate a high capacity for arsenic removal from water bodies.
As the global demand for clean water rises, the importance of water resources is increasing. Factors such as population growth, pollution, and climate change are reducing the usability of limited water resources. Urbanization, agriculture, and industry have a significant impact on water quality. Pollutants, such as heavy metals, disrupt aquatic ecosystems, thereby undermining their sustainability (Sener et al., 2023). The presence of metal pollution, such as arsenic (As), has a harmful impact on the ecosystem because of its toxic, non-biodegradable, and persistent characteristics. Arsenic rapidly accumulates in the soil, water, and sediment, posing a threat to the environment (Prasad Ahirvar et al., 2023). The presence of As in the water is a result of weathering processes that occur in rocks and sulfidic minerals. One of these processes involves the oxidation of sulfidic minerals like arsenopyrites, which releases arsenic into water sources. Additionally, anthropogenic activities such as agriculture, mining, and industrial waste also contribute to the release of As into the environment (Irunde et al., 2023).
Arsenic, a harmful contaminant, is a toxic substance that is both colorless and odorless (Abedi and Mojiri, 2020). It can be found in elevated concentrations in water bodies across different countries worldwide. Exposure to high levels of arsenic can result in severe immediate and long-term health issues, including diarrhea, vomiting, diabetes, heart disease, cancer, miscarriage, childhood cancer, and even fatalities (Nguyen and Mulligan, 2023). To ensure safe drinking water and protect public health, the World Health Organization (WHO) recommends a maximum allowable concentration (MAC) of As in drinking water at 10 μg/L. This limit is legally defined in the European Union Drinking Water Directive. However, certain countries like Denmark and Ireland have set even lower limits (5 μg/L and 7.5 μg/L, respectively). Arsenic concentrations in groundwater and surface water have been found to range from 100 to 5,000 μg/L. In certain cases, particularly near hydrothermal systems close to shorelines, extremely high concentrations of 1,386 and 5,850 μg/L have been reported. This widespread contamination of As in aquatic systems is recognized as a significant global environmental issue, with impacts observed worldwide (Zhang et al., 2022).
Increasingly, more states are adopting stricter As standards based on the severity of As issues, population exposure, and the availability of improved removal technologies (Nikić et al., 2023). Adsorption is a widely used and promising technique for removing As from water (Fang et al., 2023). Adsorption has gained significant recognition as a cost-effective method for As removal. It offers several advantages, including high removal efficiency, lower cost, and simpler operation compared to alternative methods (Moradi et al., 2023). In this review manuscript, our study aims to study the fate of As in water bodies around the world as well as comprehensively evaluate the effectiveness of adsorption as a method for As removal from water.
Once released into the environment, arsenic becomes part of the biogeochemical cycle and cannot be degraded. In aquatic environments, arsenic undergoes complex chemical speciation, resulting in various inorganic and organic arsenic species. Inorganic forms include arsenite As(III) and arsenate As(V), while organic forms encompass methylarsonate (MMA), dimethylarsinate (DMA), tetramethylarsine (TMA), trimethylarsine oxide (TMAO), arsenocholine (AsC), arsenobetaine (AsB), thiolated arsenic, arsenosugars (As-Sug), and arsenolipids (Zhang et al., 2022).
Commonly, in water, As exists in various redox states, predominantly as inorganic species such as As(III) and As(V). However, the presence of organic species is more common when there is anthropogenic contamination (Fuoco et al., 2022). As(III) is widely recognized for its higher toxicity compared to As(V) (Wu et al., 2023). The fate and mobility of arsenic in water bodies are influenced by various processes: (1) redox reactions, (2) adsorption and desorption, (3) competitive adsorption (ion exchange), (4) solid-phase precipitation and dissolution, and (5) biological activity. These interconnected processes, along with factors such as redox potential (Eh), pH, chemical composition, and reaction kinetics, collectively determine the behavior of arsenic under specific conditions (Mohan and Pittman, 2007; Cheng et al., 2009). This redox sensitivity of arsenic is depicted in Figure 1, which illustrates the Eh-pH diagram for arsenic specie. In oxidizing conditions, arsenate (H2AsO4) is the predominant form at low pH levels (below approximately 6.9), while at higher pH levels, it exists in the form of HAsO. Conversely, under reducing conditions at pH levels below approximately 9.2, the dominant form is the uncharged arsenite species (H3AsO3) (Smedley and Kinniburgh, 2002).
Figure 1. Redox potential (Eh)–pH diagram for aqueous arsenic species in the AsO2-H2O system at 25°C and 1 bar total pressure (Akter et al., 2005; copyright permission received from Elsevier).
The problem of arsenic contamination in aquatic environments is a worldwide concern (Singh et al., 2020). In the area near Lomé (in Togo), surface water samples exhibited elevated concentrations of arsenic, reaching as high as 6,460 μg/L. Similarly, in Prestea, Ghana, a wide range of arsenic concentrations ranging from 150 to 8,250 μg/L were reported in surface waters (Ahoulé et al., 2015). Table 1 presents the concentration of As reported in aquatic environments worldwide. According to the data presented in Table 1, the highest recorded arsenic concentration (μg/L) in drinking water was 1320 in Nicaragua. In Taiwan and Thailand, the maximum arsenic concentrations (μg/L) in groundwater were 5,000 and 1,820, respectively. Furthermore, the highest arsenic concentration (μg/L) in surface water was 2,650 in Nicaragua. In Brazil, the maximum arsenic concentration (μg/L) in wastewater was 134,000, observed in landfill leachate. Additionally, a notable concentration of arsenic (14.8 μg/L) was reported in seawater near Tarut Island in the Persian Gulf.
When aquatic organisms come into contact with arsenic through their diet and various sources like water, they have the ability to accumulate, retain, and convert different forms of arsenic within their bodies (Azizur Rahman et al., 2012). The primary bioaccumulation processes encompass the uptake, assimilation, biotransformation, and elimination of arsenic (Figure 2). Once absorbed by an organism, arsenic has the potential to undergo biotransformation, converting into a less toxic form. Notably, AsB is recognized for its low toxicity among aquatic organisms across different trophic levels (Zhang et al., 2022).
Figure 2. Processes of arsenic bioaccumulation in aquatic organisms (Zhang et al., 2022; open access paper under the terms of the Creative Commons CC-BY license).
The toxicity of arsenic varies among living organisms depending on their resistance capabilities and detoxification mechanisms. In terms of toxicity, inorganic arsenic (iAs) is more harmful than organoarsenic and has been classified as a proven carcinogen for humans. Arsenite (AsIII) is typically more toxic than arsenate (AsV), whereas dimethylarsinous acid (DMAAIII) and monomethylarsonous acid (MMAAIII) exhibit higher toxicity compared to their respective parent compounds (Azizur Rahman and Hasegawa, 2012). Azizur Rahman et al. (2012) stated that while it is commonly known that iAs is typically more toxic than organoarsenic species, the toxicity of iAs species toward aquatic organisms is still a topic of debate. Interestingly, there are exceptions to this generalization. Marine phytoplankton, for instance, often exhibit higher sensitivity to arsenite (AsIII), whereas freshwater phytoplankton are highly sensitive to arsenate (AsV). As an illustration, the marine phytoplankton species Dunaliella sp. and Polyphysa peniculus demonstrate greater sensitivity to AsV compared to AsIII. Table 2 shows the bioaccumulation As in aquatic organisms, which indicates that up to 362 μg/g of As reported in fish tissues.
Garai et al. (2021) stated that prolonged exposure of freshwater fish to low concentrations of arsenic leads to the accumulation of this toxic element primarily in the liver and kidney tissues. The exposure to arsenic causes histopathological changes in the gills and liver tissue of tilapia (Oreochromis mossambicus), a type of freshwater fish. The observed alterations in the gills include epithelial hyperplasia, lamellar fusion, epithelial lifting, edema, desquamation, and necrosis.
In recent decades, various treatment techniques have been used to remove heavy metals like As, including chemical precipitation, membrane filtration, extraction, and electrochemistry. However, these methods have drawbacks such as poor selectivity, high energy consumption, and high costs, limiting their application in engineering. As a result, adsorption has become a favored choice for heavy metal removal due to its broad applicability, low cost, and environmental friendliness (Di et al., 2023). On the other hand, the main advantages of the adsorption technique include its adaptability, user-friendly nature, cost-effectiveness, and its ability to accommodate a diverse range of adsorbents sourced from minerals, as well as biological and organic origins (Rajendran et al., 2022). Adsorption begins by transferring heavy metal ions from the aqueous solution to the surface of adsorbents. Subsequently, these ions bind to the surface through physical or chemical interactions. To facilitate this process, adsorbents must possess a large accessible surface area, which ensures the presence of numerous exposed active sites for effective and selective binding with heavy metal ions (Fei and Hu, 2023). A wide range of adsorbents have been employed for the adsorption of heavy metals (such as As) from wastewater and natural water (Chakraborty et al., 2022). These include engineered adsorbents like nano-adsorbents and metals coated adsorbents, as well as low-cost options. Among low-cost adsorbents, natural materials such as zeolites, and clay are commonly used. Additionally, agricultural waste materials and biochar serve as prominent sources of bio-adsorbents (Abdollahi et al., 2022; Oladoye, 2022). According to Ariffin et al. (2017), the most advantageous aspects of adsorption methods include their simplicity, flexibility in design, ease of operation, and resistance to toxic contaminants. However, a notable disadvantage of this method is the need for regeneration processes. The adsorption mechanism can involve physical entrapment (absorption) or chemical binding through weak Van der Waals forces, dipole-dipole and ion-dipole interactions, cation exchange, or strong covalent bonding (Mojiri et al., 2020). Physisorption occurs when the absorbent and adsorbate are united by van der Waals forces. Chemisorption takes place when the adsorbate forms chemical bonds with the surface of the adsorbent. Electrostatic attraction occurs when the adsorbent surface carries negative or positive charges. Ion exchange happens between divalent metal cations and oxygen-containing functional groups, taking advantage of the cation exchange capacity. Surface complexation occurs when heavy metals exceed the available sites on the adsorbent surface, and this involves the formation of multiatom assemblies during the reaction activities (Mahesh et al., 2022). The removal of As through adsorption methods is presented in Table 3. According to Table 3, the maximum adsorption capacity of engineered adsorbents is significantly higher than that of single adsorbents. For example, engineered nanoparticles can exhibit adsorption capacities exceeding 100 mg/g.
Carbon-based adsorbents possess significant potential as adsorbent materials due to their large specific surface area, high porosity, substantial pore volume, and adjustable morphological and functional group properties. These characteristics make them suitable for various applications, including water purification. The adsorption efficiency of carbonaceous materials is influenced by factors such as the raw material, production technique, and environmental variables (Mahesh et al., 2022). The main mechanisms of As removal with carbon-based adsorbents are shown in Figure 3. Sabzehmeidani et al. (2021) reported that activated carbon, biochar, and graphene-based adsorbents are commonly utilized for the removal of arsenic (As) from water sources.
Figure 3. Schematic representation of heavy metal adsorption mechanisms on carbon adsorbents (Yang et al., 2019; copyright permission received from Elsevier).
Activated carbon (AC) is a versatile and widely available carbon material used for adsorption. It comes in different forms, such as granular, powder, pellet, and spheres. These materials exhibit efficient and selective adsorption of toxins and heavy metals. Compared to other adsorbents, activated carbon-based materials are cost-effective, highly effective, and easy to use (Lan et al., 2023). AC has a structure that includes well-defined micro, meso, and macropores, as well as diverse surface functional groups. The surface area of activated carbon ranges from 500 to 3,000 m2/g (Sultana et al., 2022). In a study, Gao et al. (2023) utilized modified AC to effectively remove both As(III) and As(V). The maximum adsorption capacities were found to be 10.9 mg/g for As(III) and 16.0 mg/g for As(V). The maximum adsorption capacity (mg/g) observed for As(V) removal using As was 1.2 (Yürüm et al., 2014).
Graphene is a 2D lattice of carbon atoms with high surface area, thermal conductivity, Young's modulus, and charge carrier mobility. It exhibits the quantum Hall effect due to electron confinement in 2D materials (Sabzehmeidani et al., 2021). Graphene oxide (GO), a derivative of graphene, is a single sheet of graphite with a 2D honeycomb crystal plane structure. It exhibits a high specific surface area of up to 2,620 m2/g (Bian et al., 2015). GO is characterized by diverse functional groups on its surface, including epoxy, lactol, carboxyl, phenol, and hydroxyl groups, along with large π-stacking. These features enable GO to possess a high sorption capacity through strong interactions such as hydrogen bonding, electrostatic forces, and π-π interactions (Gabris et al., 2022). In the study (Bian et al., 2015), GO was synthesized using a modified Hummers method. A solution containing 1.0 g of NaNO3 dissolved in 50 ml of concentrated H2SO4 was prepared, and then 1.0 g of natural graphite was slowly added to the solution while stirring in an ice-water bath. After complete dissolution, 18.0 g of KMnO4 was gradually added over a period of 30 min. The mixture was stirred for 2 h at 309 ± 1 K and then heated in a water bath at 368 K for 30 min. Following this, 180 ml of deionized water was added, and a suspension of 20 ml of H2O2 (30 wt%) was slowly introduced. The desired products were washed with 10% v/v HCl and ultrapure water, undergoing centrifugation and ultrasonication until a constant pH value was reached. The resulting GO was dried at 333 K for 24 h. Chandra et al. (2010) removed As(III) and As(V) using modified GO (magnetic GO). The maximum adsorption capacity (mg/g) based on the Langmuir isotherm was found to be 10.2 for As(III) and 5.2 for As(V), respectively. Their study revealed that the adsorption process of As using magnetic-GO primarily followed surface complexation. In a study conducted by Wu et al. (2018b) GO-based adsorbent (GO/CuFe2O4) was used to remove both As(III) and As(V). The maximum adsorption capacities (mg/g) achieved were 51.64 for As(III) and 124.69 for As(V) removal. Their study demonstrated that both As(III) and As(V) adsorptions on the GO-CuFe2O4 adsorbent followed an inner-sphere complex mechanism. In another study conducted by Su et al. (2017), a modified GO called iron oxide-graphene oxide was used to successfully remove 99.9% of both As(III) and As(V). The maximum adsorption capacities were 147 mg/g for As(III) and 113 mg/g for As(V). The results of their study showed that the adsorption of As onto modified-GO takes place through a mechanism known as surface complexation.
Biochar is produced through the thermal treatment of natural organic feedstocks in an oxygen-limited environment. Huang et al. (2019) emphasized that biochar offers numerous advantages, such as its ion exchange capacity, expansive surface area, high porosity, and presence of surface functional groups, which make it promising for wastewater treatment. Biochar is composed of various elements, including carbon, sulfur, hydrogen, oxygen, nitrogen, and minerals found in the ash fraction. Although biochar shares similar properties and structure with AC, AC has a considerably larger surface area. The primary surface functional groups found in biochar include carboxyl, hydroxyl, phenolic hydroxyl, and carbonyl groups (Mojiri and Zhou, 2023). In a research, Wang et al. (2015) used a modified version of biochar called manganese oxide-modified biochars to successfully remove As(V). They discovered that the biochar had a maximum adsorption capacity of 0.59 mg/g, with the main adsorption mechanism being the interaction between the biochar and As(V). Navarathna et al. (2019) employed a modified biochar known as magnetic Fe3O4/Douglas fir biochar composites to effectively remove over 68% of As(III). The maximum adsorption capacity of the modified biochar was determined to be 6.9 mg/g. Their study suggested that the adsorption process was primarily governed by a chemisorption mechanism. In a research study, wood biochar exhibited remarkable efficiency in removing 92–100% of As. The maximum adsorption capacity for As(III) removal was measured at 3.1 mg/g, while for As(V) removal, it was 3.8 mg/g. The main identified adsorption mechanisms were chemisorption and physicosorption (which involve the filling of pores in the biochar) (Niazi et al., 2018).
One of the methods to analyze the structural properties and identify functional groups on biochar and other sorbents is FTIR spectroscopy. It enables the differentiation of functional groups between the original feedstock material and the resulting biochar, as well as the assessment of any changes in functional groups before and after As sorption (Amen et al., 2020). The FTIR spectrum of Oak wood biochar (OW-BC) before and after As removal is shown in Figure 4. In OW-BC, spectral bands indicated the presence of -OH and non-ionic carboxyl groups, as well as aliphatic methylene/methyl groups and C-O/C-O-C surface functional groups. After As sorption, slight shifts and intensity changes were observed. Notably, in As(III)-loaded OW-BC, peak shifts and transformations were observed at specific wavelengths. These changes were relatively smaller in As(V)-loaded OW-BC (Niazi et al., 2018).
Figure 4. FTIR absorbance spectra of Japanese oak wood-derived biochar (OW-BC) prepared at 500°C: (A) OW-BC_As-Unloaded (solid black line), (B) OW-BC_As(V)-Loaded (solid pink line), and (C) OW-BC_As(III)-Loaded (solid green line); (Niazi et al., 2018; copyright permission received from Elsevier).
Researchers have been increasingly drawn to natural mineral-based adsorbents as an alternative source. These adsorbents have garnered significant attention due to their low cost, abundance, easy retrievability, and exceptional adsorption capabilities. Among the numerous natural minerals found on Earth, zeolite and clays, particularly bentonite, have been extensively studied for their effectiveness in removing heavy metals (Zaimee et al., 2021). Clay minerals are phyllosilicates composed of T (tetrahedral) and O (octahedral) sheets in a 1:1 or 2:1 ratio. Due to isomorphous substitution, the layers of certain 2:1 clay minerals (such as smectite and vermiculite) carry a negative charge, which is balanced by cationic counterions in the interlayer space. These counterions can be exchanged, making clay minerals efficient adsorbents for cationic contaminants (Zhu et al., 2016). Bentonite, a clay with a high content of montmorillonite, is widely utilized for ion removal from water due to its bi-dimensional tetrahedral and octahedral sheets and isomorphic substitutions. It is both cheap and abundant, making it an ideal choice for synthesizing functionalized clay (Barakan and Aghazadeh, 2019). Arsenic was effectively removed using modified bentonite, achieving a maximum adsorption capacity of 9.14–9.99 mg/g. The removal mechanisms involved a combination of electrostatic attraction and inner-sphere complexation, indicating a mixed removal mechanism (Hua, 2018). A modified bentonite [Fe(III)-modified bentonite] successfully removed As(III), exhibiting a maximum adsorption capacity of 0.3 mg/g (Baigorria et al., 2022). A significant removal efficiency of 84.5% was achieved for As(V) using modified bentonite (lanthanum-modified bentonite), with a maximum adsorption capacity of 3.8 mg/g (Cui et al., 2021).
Zeolites, whether naturally occurring or artificially synthesized, are hydrated aluminosilicate minerals. Their porous and cage-like structure enables them to provide significant internal and external surface areas for efficient ion exchange. This characteristic makes zeolites highly effective in adsorbing arsenic from water, highlighting their potential as a valuable tool for eliminating this toxic substance (Salem Attia et al., 2014; Velarde et al., 2023). The application of a modified zeolite, the Copper Exchange Zeolite, resulted in the removal of over 98% of both As(III) and As(V). The maximum adsorption capacity for As(III) was measured at 1.3 mg/g, while for As(V) it was 1.4 mg/g (Pillewan et al., 2014). Electrostatic Interaction and ion substitution (Figure 5) are key mechanisms involved in the removal of arsenic (As) using zeolite (Shevade and Ford, 2004; Liu et al., 2015). The maximum adsorption capacity (mg/g) was 0.5, at pH=6, in As(V) removal with a modified zeolite (Macedo-Miranda and Olguín, 2007).
Figure 5. Principal Mechanisms of Heavy Metal Adsorption with Natural Zeolites: (A) Ion Exchange, (B) Electrostatic Interaction (Velarde et al., 2023; open access paper under the terms of the Creative Commons CC-BY license).
Nanoparticles are tiny particles ranging in size from 1 to 100 nanometers. They exist in the transitional zone between individual molecules and larger materials. Due to their small size, nanoparticles possess unique physicochemical properties, including a high specific surface area, significant energy, and the confinement of quantum effects (Jjagwe et al., 2023). Metal oxide nanoparticles (NPs) as adsorbents offer numerous benefits, including high specificity and capacity attributed to the quantum size effect and their large surface area. Moreover, these NPs possess unique structures, diverse pore sizes, low solubility, and can be easily synthesized using cost-effective techniques. Their low solubility, strong mechanical properties, and remarkable stability against organic dyes make metal oxides highly effective adsorbents (Hosny et al., 2023). Zinc oxide (ZnO) nanoparticles, silver oxide (Ag2O) nanoparticles, copper oxide (CuO) nanoparticles, titanium dioxide (TiO2) nanoparticles, and iron oxide nanoparticles are widely used metal oxide nanoparticles (Naseem and Durrani, 2021). Numerous iron-based materials and processes have been developed to address the issue of arsenic removal from drinking water. Iron oxides, oxyhydroxides, and hydroxides, such as amorphous hydrous ferric oxide (Fe(O)OH), goethite (FeO–OH), and hematite (Fe2O3), have demonstrated their efficacy in removing arsenic from aqueous solutions (Dhanasekaran and Sahu, 2021). Besides, in a study (Siddiqui and Chaudhry, 2017), a Fe2O3 nano-composite adsorbent has been functionalized to enhance arsenic (As) removal. As illustrated in Figure 6, Fe2O3 nano-composites have proven to be efficient adsorbents for arsenic cleanup from water. This efficacy is attributed to the presence of a large number of hydroxyl and other functional groups on the surface, enabling a higher capacity for the oxidation of As(III) to As(V). The functional groups attached to the composite material's surface provide strong trapping sites for arsenic across various pH levels.
Figure 6. Illustration of As(III) to As(V) oxidation and adsorption of As(III) and As(V) species on the modified iron oxide surface (Siddiqui and Chaudhry, 2017; copyright permission received from Elsevier).
In a study (Pham et al., 2020), As(V) was removed by a ferric hydroxide-based adsorbent, the maximum removal capacity was 2.9 (mg/g). In a separate study (Asadi Haris et al., 2023), superparamagnetic iron oxide nanoparticles (SPIONs) and alginate-encapsulated SPIONs (SPIONs-Alg) were synthesized for arsenic (As) removal from water. Figure 7 illustrates key bands: 3,400 cm−1 (O–H symmetric vibration), 2,936 cm−1 (aliphatic C–H stretching), 600 cm−1 ([Fe–O] intrinsic stretching), and 400 cm−1 (octahedral [Fe–O] vibration), confirming ferrite formation in spinel form. Notably, SPIONs-Alg exhibited a distinct 585 cm−1 band, indicating the presence of nanoferrite.
Figure 7. Illustration of As(III) to As(V) oxidation and adsorption of As(III) and As(V) species on the modified iron oxide surface. (Asadi Haris et al., 2023; copyright permission received from Elsevier).
Due to the formation of stable bidentate binuclear surface complexes on its surface, TiO2 shows great promise as a material for the removal of As from industrial wastewater (Qiu et al., 2019). In a study (Deng et al., 2021), the maximum adsorption capacities (mg/g) for the removal of As(III) and As(V) using a TiO2-based adsorbent were 7.7 and 18.2, respectively. A study utilized a modified metal oxide nanoparticle (Ce0.8Ti0.2O2−y) to remove arsenic. The adsorbent demonstrated a maximum adsorption capacity of 2×105 mg/g (Mishra and Rai, 2019). Their study revealed that the removal procedure was primarily governed by two mechanisms. Firstly, the redox reaction involving ceria and titania metals facilitates the partial oxidation of the more toxic As(III) to the less toxic As(V). The resulting As(V) can readily form monodentate and bidentate complexes. Secondly, the adsorption of As(III) occurs through the interaction between the surface hydroxyl groups of the synthesized adsorbent and the As(III) species. In the study (Mishra and Rai, 2019), Titania-doped cerium oxide nanoparticles (Ce1−xTixO2−y) were synthesized using a single-pot aerogel process. Cerium nitrate hexahydrate (40 mM) and stoichiometric oxalic acid were dissolved in a mixture of ethanol and toluene. Titanium isopropoxide (10% and 20% w/w of cerium nitrate hexahydrate) in 50 mL of ethanol, along with distilled water, were gradually added to the precursor mixture. Vigorous stirring at 300 rpm resulted in a light yellowish gel. The gel was transferred to a Parr reactor and heated at a rate of 1°C per minute. The solvents were supercritically dried under elevated temperature and high pressure, yielding a light yellow, low-density powder of Ce1−xTixO2−y oxide nanoparticles. These nanoparticles were then used as adsorbents for arsenic removal. The use of various metal oxide nanoparticles resulted in the removal of over 92% of As(V), with an impressive adsorption capacity of up to 305 mg/g (Hocaoglu et al., 2019). They mentioned that the primary adsorption mechanism of metal oxides relies on the formation of strong bonds between the surface –OH groups of the metal oxides and the –OH group of the arsenic(V) species. In another study (Sunil et al., 2018), more than 96% of As was removed with Al-Ti2O6 nanoparticles. In another study (Powell et al., 2020), carbon-coated iron carbide (Fe3C@C) was used to remove arsenic (As) from groundwater. The maximum adsorption capacity was 168 micrograms per gram (μg/g). Figure 8 illustrates the interactions between arsenic and metal oxide-based nanoadsorbents under neutral pH conditions. Table 4 shows the removal of As using various nanoparticle adsorbents.
Figure 8. Arsenic's interactions with metal oxide-based nanoadsorbents at neutral pH (Ersan et al., 2023; copyright permission received on June 12, 2023, from Elsevier).
The presence of arsenic in water bodies poses a significant risk to both human health and the environment. Arsenic contamination in water sources is a global environmental concern caused by both natural processes and human activities. The high concentrations of arsenic found in drinking water, groundwater, wastewater, and aquatic organisms highlight the urgent need for effective removal methods. This review manuscript critically evaluated the effectiveness and limitations of adsorption methods for arsenic removal from water bodies. Adsorption emerged as a promising technique due to its cost-effectiveness, high removal efficiency, and simplicity of operation. Various adsorbents, including nanoparticle adsorbents and graphene-based adsorbents, demonstrated a high capacity for arsenic removal. Additionally, low-cost adsorbents such as zeolites, clay, and chitosan, as well as agricultural waste materials and biochar, have shown potential for arsenic adsorption. Future research should focus on optimizing adsorbent materials, understanding the mechanisms of arsenic adsorption, and developing sustainable and efficient regeneration techniques.
AM: Conceptualization, Investigation, Resources, Supervision, Writing—original draft, Writing—review & editing. ER: Conceptualization, Validation, Writing—review & editing. BK: Conceptualization, Validation, Writing—original draft, Writing—review & editing. SR: Writing—review & editing. NK: Funding acquisition, Writing—review & editing. MV: Writing—review & editing. HF: Writing—review & editing.
The author(s) declare that no financial support was received for the research, authorship, and/or publication of this article.
ER and HF were employed by Envirowise Consultant Limited.
The remaining authors declare that the research was conducted in the absence of any commercial or financial relationships that could be construed as a potential conflict of interest.
The author(s) declared that they were an editorial board member of Frontiers, at the time of submission. This had no impact on the peer review process and the final decision.
All claims expressed in this article are solely those of the authors and do not necessarily represent those of their affiliated organizations, or those of the publisher, the editors and the reviewers. Any product that may be evaluated in this article, or claim that may be made by its manufacturer, is not guaranteed or endorsed by the publisher.
Abadi, M., Zamani, A., Parizanganeh, A., Khosravi, Y., and Badiee, H. (2018). Heavy metals and arsenic content in water along the southern Caspian coasts in Iran. Environ. Sci. Pollut. Res. 25, 23725–23735. doi: 10.1007/s11356-018-2455-7
Abdollahi, N., Moussavi, G., and Giannakis, S. (2022). A review of heavy metals' removal from aqueous matrices by Metal-Organic Frameworks (MOFs): State-of-the art and recent advances. J Environ Chem Eng 10, 107394. doi: 10.1016/j.jece.2022.107394
Abedi, T., and Mojiri, A. (2020). Arsenic uptake and accumulation mechanisms in rice species. Plants 9, 129. doi: 10.3390/plants9020129
Abiye, T. A., and Bhattacharya, P. (2019). Arsenic concentration in groundwater: archetypal study from South Africa. Groundw. Sustain. Dev. 9, 100246. doi: 10.1016/j.gsd.2019.100246
Agrafioti, E., Kalderis, D., and Diamadopoulos, E. (2014). Arsenic and chromium removal from water using biochars derived from rice husk, organic solid wastes and sewage sludge. J. Environ. Manage. 133, 309–314. doi: 10.1016/j.jenvman.2013.12.007
Ahmad, M., Usman, A. R. A., Hussain, Q., Al-Farraj, A. S. F., Tsang, Y. F., Bundschuh, J., et al. (2020). Fabrication and evaluation of silica embedded and zerovalent iron composited biochars for arsenate removal from water. Environm. Pollut. 266, 115256. doi: 10.1016/j.envpol.2020.115256
Ahmed Baig, J., Gul Kazi, T., Qadir Shah, A., Abbas Kandhro, G., Imran Afridi, H., Balal Arain, M., et al. (2010). Speciation and evaluation of Arsenic in surface water and groundwater samples: a multivariate case study. Ecotoxicol. Environ. Saf. 73, 914–923. doi: 10.1016/j.ecoenv.2010.01.002
Ahoulé, D. G., Lalanne, F., Mendret, J., Brosillon, S., and Maïga, A. H. (2015). Arsenic in african waters: a review. Water Air Soil Pollut. 226, 302. doi: 10.1007/s11270-015-2558-4
Akter, K. F., Owens, G., Davey, D. E., and Naidu, R. (2005). Arsenic speciation and toxicity in biological systems. Rev. Environ. Contam. Toxicol. 184, 97–149. doi: 10.1007/0-387-27565-7_3
Al-Absi, E., Manasrah, R., Abukashabeh, A., and Wahsha, M. (2019). Assessment of heavy metal pollutants at various sites along the Jordanian coastline of the Gulf of Aqaba, Red Sea. Int. J. Environ. Anal. Chem. 99, 726–740. doi: 10.1080/03067319.2019.1609459
Alarcón-Herrera, M. T., Martin-Alarcon, D. A., Gutiérrez, M., Reynoso-Cuevas, L., Martín-Domínguez, A., Olmos-Márquez, M. A., et al. (2020). Co-occurrence, possible origin, and health-risk assessment of arsenic and fluoride in drinking water sources in Mexico: geographical data visualization. Sci. Total Environm 698, 134168. doi: 10.1016/j.scitotenv.2019.134168
Alharbi, T., Alfaifi, H., and El-Sorogy, A. (2017). Metal pollution in Al-Khobar seawater, Arabian Gulf, Saudi Arabia. Mar. Pollut. Bull. 119, 407–415. doi: 10.1016/j.marpolbul.2017.03.011
Al-Taani, A. A., Batayneh, A., Nazzal, Y., Ghrefat, H., Elawadi, E., and Zaman, H. (2014). Status of trace metals in surface seawater of the Gulf of Aqaba, Saudi Arabia. Mar. Pollut. Bull. 86, 582–590. doi: 10.1016/j.marpolbul.2014.05.060
Alves, R. I. S., Sampaio, C. F., Nadal, M., Schuhmacher, M., Domingo, J. L., and Segura-Muñoz, S. I. (2014). Metal concentrations in surface water and sediments from Pardo River, Brazil: Human health risks. Environ. Res. 133, 149–155. doi: 10.1016/j.envres.2014.05.012
Amen, R., Bashir, H., Bibi, I., Shaheen, S. M., Niazi, N. K., Shahid, M., et al. (2020). A critical review on arsenic removal from water using biochar-based sorbents: The significance of modification and redox reactions. Chem. Eng. J. 396, 125195. doi: 10.1016/j.cej.2020.125195
Ariffin, N., Abdullah, M. M. A. B., Mohd Arif Zainol, M. R. R., Murshed, M. F., Zain, H., Faris, M. A., et al. (2017). Review on adsorption of heavy metal in wastewater by using geopolymer. MATEC Web Conf. 97, 01023. doi: 10.1051/matecconf/20179701023
Asadi Haris, S., Dabagh, S., Mollasalehi, H., and Ertas, Y. N. (2023). Alginate coated superparamagnetic iron oxide nanoparticles as nanocomposite adsorbents for arsenic removal from aqueous solutions. Sep. Purif. Technol. 310, 123193. doi: 10.1016/j.seppur.2023.123193
Avigliano, E., Schlotthauer, J., de Carvalho, B. M., Sigrist, M., and Volpedo, A. V. (2020). Inter-and intra-stock bioaccumulation of anionic arsenic species in an endangered catfish from South American estuaries: risk assessment through consumption. J. Food Compos. Analy. 87, 103404. doi: 10.1016/j.jfca.2019.103404
Azizur Rahman, M., and Hasegawa, H. (2012). Arsenic in freshwater systems: influence of eutrophication on occurrence, distribution, speciation, and bioaccumulation. Appl. Geochem. 27, 304–314. doi: 10.1016/j.apgeochem.2011.09.020
Azizur Rahman, M., Hasegawa, H., and Peter Lim, R. (2012). Bioaccumulation, biotransformation and trophic transfer of arsenic in the aquatic food chain. Environ. Res. 116, 118–135. doi: 10.1016/j.envres.2012.03.014
Baig, J. A., Kazi, T. G., Arain, M. B., Afridi, H. I., Kandhro, G. A., Sarfraz, R. A., et al. (2009). Evaluation of arsenic and other physico-chemical parameters of surface and ground water of Jamshoro, Pakistan. J. Hazard. Mater. 166, 662–669. doi: 10.1016/j.jhazmat.2008.11.069
Baigorria, E., Cano, L., Sapag, K., and Alvarez, V. (2022). Removal efficiency of As(III) from aqueous solutions using natural and Fe(III) modified bentonites. Environ. Technol. 43, 3728–3741. doi: 10.1080/09593330.2021.1934559
Barakan, S., and Aghazadeh, V. (2019). Structural modification of nano bentonite by aluminum, iron pillarization and 3D growth of silica mesoporous framework for arsenic removal from gold mine wastewater. J. Hazard. Mater. 378, 120779. doi: 10.1016/j.jhazmat.2019.120779
Bhattacharya, P., Sracek, O., Eldvall, B., Asklund, R., Barmen, G., Jacks, G., et al. (2012). Hydrogeochemical study on the contamination of water resources in a part of Tarkwa mining area, Western Ghana. J. Afric. Earth Sci. 66–67, 72–84. doi: 10.1016/j.jafrearsci.2012.03.005
Bi, X., Zeng, C., and Westerhoff, P. (2020). Adsorption of arsenic ions transforms surface reactivity of engineered cerium oxide nanoparticles. Environ. Sci. Technol. 54, 9437–9444. doi: 10.1021/acs.est.0c02781
Bian, Y., Bian, Z.-Y., Zhang, J.-X., Ding, A.-Z., Liu, S.-L., and Wang, H. (2015). Effect of the oxygen-containing functional group of graphene oxide on the aqueous cadmium ions removal. Appl. Surf. Sci. 329, 269–275. doi: 10.1016/j.apsusc.2014.12.090
Boonkaewwan, S., Sonthiphand, P., and Chotpantarat, S. (2021). Mechanisms of arsenic contamination associated with hydrochemical characteristics in coastal alluvial aquifers using multivariate statistical technique and hydrogeochemical modeling: a case study in Rayong province, eastern Thailand. Environ. Geochem. Health 43, 537–566. doi: 10.1007/s10653-020-00728-7
Budinova, T., Savova, D., Tsyntsarski, B., Ania, C. O., Cabal, B., Parra, J. B., et al. (2009). Biomass waste-derived activated carbon for the removal of arsenic and manganese ions from aqueous solutions. Appl. Surf. Sci. 255, 4650–4657. doi: 10.1016/j.apsusc.2008.12.013
Bui, T. H., Kim, C., Hong, S. P., and Yoon, J. (2017). Effective adsorbent for arsenic removal: core/shell structural nano zero-valent iron/manganese oxide. Environ. Sci. Pollut. Res. 24, 24235–24242. doi: 10.1007/s11356-017-0036-9
Bundschuh, J., Armienta, M. A., Morales-Simfors, N., Alam, M. A., López, D. L., Delgado Quezada, V., et al. (2021). Arsenic in Latin America: New findings on source, mobilization and mobility in human environments in 20 countries based on decadal research 2010-2020. Crit. Rev. Environ. Sci. Technol. 51, 1727–1865. doi: 10.1080/10643389.2020.1770527
Camacho, L. M., Gutiérrez, M., Alarcón-Herrera, M. T., Villalba, M., de, L., and Deng, S. (2011). Occurrence and treatment of arsenic in groundwater and soil in northern Mexico and southwestern USA. Chemosphere 83, 211–225. doi: 10.1016/j.chemosphere.2010.12.067
Carraro, A., Fabbri, P., Giaretta, A., Peruzzo, L., Tateo, F., and Tellini, F. (2013). Arsenic anomalies in shallow Venetian Plain (Northeast Italy) groundwater. Environ. Earth Sci. 70, 3067–3084. doi: 10.1007/s12665-013-2367-2
Chakraborty, R., Asthana, A., Singh, A. K., Jain, B., and Susan, A. B. H. (2022). Adsorption of heavy metal ions by various low-cost adsorbents: a review. Int. J. Environ. Anal. Chem. 102, 342–379. doi: 10.1080/03067319.2020.1722811
Chandra, V., Park, J., Chun, Y., Lee, J. W., Hwang, I.-C., and Kim, K. S. (2010). Water-dispersible magnetite-reduced graphene oxide composites for arsenic removal. ACS Nano 4, 3979–3986. doi: 10.1021/nn1008897
Chen, H., Lv, K., Du, Y., Ye, H., and Du, D. (2016). Microwave-assisted rapid synthesis of Fe2O3/ACF hybrid for high efficient As(V) removal. J. Alloys Compd. 674, 399–405. doi: 10.1016/j.jallcom.2016.03.024
Chen, T., Su, Y., and Yuan, X. (2018). Influences of the petroleum-recovering activity on the arsenic level in groundwater of Kuitun, Xinjiang, China. Hum. Ecol. Risk Assessm. 24, 2195–2208. doi: 10.1080/10807039.2018.1442709
Chen, W., Parette, R., Zou, J., Cannon, F. S., and Dempsey, B. A. (2007). Arsenic removal by iron-modified activated carbon. Water Res. 41, 1851–1858. doi: 10.1016/j.watres.2007.01.052
Cheng, H., Hu, Y., Luo, J., Xu, B., and Zhao, J. (2009). Geochemical processes controlling fate and transport of arsenic in acid mine drainage (AMD) and natural systems. J. Hazard. Mater. 165, 13–26. doi: 10.1016/j.jhazmat.2008.10.070
Cheng, W., Zhang, W., Hu, L., Ding, W., Wu, F., and Li, J. (2016). Etching synthesis of iron oxide nanoparticles for adsorption of arsenic from water. RSC Adv. 6, 15900–15910. doi: 10.1039/C5RA26143K
Cui, J., Wang, D., Lin, J., Wang, Y., Ren, M., Yang, Y., et al. (2021). New application of lanthanum-modified bentonite (Phoslock®) for immobilization of arsenic in sediments. Environ. Sci. Pollut. Res. 28, 2052–2062. doi: 10.1007/s11356-020-10565-x
Delgado Quezada, V., Altamirano Espinoza, M., and Bundschuh, J. (2020). Arsenic in geoenvironments of Nicaragua: Exposure, health effects, mitigation and future needs. Sci. Total Environm. 716, 136527. doi: 10.1016/j.scitotenv.2020.136527
Deng, A., Ye, C., and Liu, W. (2018). Spatial and Seasonal Patterns of Nutrients and Heavy Metals in Twenty-Seven Rivers Draining into the South China Sea. Water (Basel) 10, 50. doi: 10.3390/w10010050
Deng, M., Chi, M., Wei, M., Zhu, A., Zhong, L., Zhang, Q., et al. (2021). A facile route of mesoporous TiO2 shell for enhanced arsenic removal. Colloids Surf. A Physicochem. Eng. Asp. 627, 127138. doi: 10.1016/j.colsurfa.2021.127138
Devic, G., Djordjevic, D., and Sakan, S. (2014). Natural and anthropogenic factors affecting the groundwater quality in Serbia. Sci. Total Environm. 468–469, 933–942. doi: 10.1016/j.scitotenv.2013.09.011
Dhanasekaran, P., and Sahu, O. (2021). Arsenate and fluoride removal from groundwater by sawdust impregnated ferric hydroxide and activated alumina (SFAA). Groundw. Sustain. Dev. 12, 100490. doi: 10.1016/j.gsd.2020.100490
Di, L., Chen, X., Lu, J., Zhou, Y., and Zhou, Y. (2023). Removal of heavy metals in water using nano zero-valent iron composites: a review. J. Water Proc. Eng. 53, 103913. doi: 10.1016/j.jwpe.2023.103913
dos Santos, Q. O., Silva Junior, M. M., Lemos, V. A., Ferreira, S. L. C., and de Andrade, J. B. (2018). An online preconcentration system for speciation analysis of arsenic in seawater by hydride generation flame atomic absorption spectrometry. Microchem. J. 143, 175–180. doi: 10.1016/j.microc.2018.08.004
Dsikowitzky, L., Mengesha, M., Dadebo, E., de Carvalho, C. E. V., and Sindern, S. (2013). Assessment of heavy metals in water samples and tissues of edible fish species from Awassa and Koka Rift Valley Lakes, Ethiopia. Environ. Monit. Assess. 185, 3117–3131. doi: 10.1007/s10661-012-2777-8
El-Aassar, M. R., Alezbaway, A. K., Althobaiti, I. O., El-Sayed, M. Y., Abu Salem, H. S., Hassan, H. M. A., et al. (2022). Fabrication of novel bentonite-anthracite@Zetag (BT-An@Zetag) composite for the removal of arsenic (V) from an aqueous solution. Molecules 27, 7635. doi: 10.3390/molecules27217635
El-Sorogy, A. S., and Attiah, A. (2015). Assessment of metal contamination in coastal sediments, seawaters and bivalves of the Mediterranean Sea coast, Egypt. Mar. Pollut. Bull. 101, 867–871. doi: 10.1016/j.marpolbul.2015.11.017
El-Sorogy, A. S., Youssef, M., Al-Kahtany, K., and Al-Otaiby, N. (2016). Assessment of arsenic in coastal sediments, seawaters and molluscs in the Tarut Island, Arabian Gulf, Saudi Arabia. Journal of African Earth Sciences 113, 65–72. doi: 10.1016/j.jafrearsci.2015.10.001
Ersan, G., Brienza, M., Mulchandani, A., Apul, O. G., and Garcia-Segura, S. (2023). Trends on arsenic species removal by metal-based nanoadsorbents. Curr. Opin. Environ. Sci. Health 34, 100478. doi: 10.1016/j.coesh.2023.100478
Ezeabasili, A., Anike, O. L., Okoro, B. U., and U-Dominie, G. M. (2014). Arsenic pollution of surface and subsurface water in Onitsha, Nigeria. Afr. J. Environ. Sci. Tech. 8, 491–497. doi: 10.5897/AJEST2014.1588
Famil Yusif, H., Majid Mirza, A., Valeh Saleh, B., and Bahruz Allahverdi, S. (2015). Trace metals in water samples taken from azerbaijan sector of Caspian Sea. J. Chem. Chem. Eng. 9. doi: 10.17265/1934-7375/2015.04.007
Fang, Z., Li, Y., Huang, C., and Liu, Q. (2023). Amine functionalization of iron-based metal-organic frameworks MIL-101 for removal of arsenic species: Enhanced adsorption and mechanisms. J. Environ. Chem. Eng. 11, 110155. doi: 10.1016/j.jece.2023.110155
Farooqi, A., Sultana, J., and Masood, N. (2017). Arsenic and fluoride co-contamination in shallow aquifers from agricultural suburbs and an industrial area of Punjab, Pakistan: Spatial trends, sources and human health implications. Toxicol. Ind. Health 33, 655–672. doi: 10.1177/0748233717706802
Fei, Y., and Hu, Y. H. (2023). Recent progress in removal of heavy metals from wastewater: A comprehensive review. Chemosphere 335, 139077. doi: 10.1016/j.chemosphere.2023.139077
Ferreira, N. S., Oliveira, L. H. B., Agrelli, V., de Oliveira, A. F., Nogueira, A. R. A., Oliveira, A., et al. (2019). Bioaccumulation and acute toxicity of As(III) and As(V) in Nile tilapia (Oreochromis niloticus). Chemosphere 217, 349–354. doi: 10.1016/j.chemosphere.2018.11.013
Fuoco, I., De Rosa, R., Barca, D., Figoli, A., Gabriele, B., and Apollaro, C. (2022). Arsenic polluted waters: application of geochemical modelling as a tool to understand the release and fate of the pollutant in crystalline aquifers. J. Environ. Manage. 301, 113796. doi: 10.1016/j.jenvman.2021.113796
Gabrielyan, A. V., Shahnazaryan, G. A., and Minasyan, S. H. (2018). Distribution and identification of sources of heavy metals in the voghji river basin impacted by mining activities (Armenia). J Chem 2018, 1–9. doi: 10.1155/2018/7172426
Gabris, M. A., Rezania, S., Rafieizonooz, M., Khankhaje, E., Devanesan, S., AlSalhi, M. S., et al. (2022). Chitosan magnetic graphene grafted polyaniline doped with cobalt oxide for removal of Arsenic(V) from water. Environ. Res. 207, 112209. doi: 10.1016/j.envres.2021.112209
Gao, Q., Feng, Z., He, Y., Hou, Y., Ren, H., Su, M., et al. (2023). Pyrolysis self-activation: An environmentally friendly method to transform biowaste into activated carbon for arsenic removal. Bioresour. Technol. 368, 128353. doi: 10.1016/j.biortech.2022.128353
Garai, P., Banerjee, P., Mondal, P., and Saha, N. C. (2021). Effect of Heavy Metals on Fishes: Toxicity and Bioaccumulation. J Clin Toxicol 11, 1–10.
Gbogbo, F., Otoo, S. D., Asomaning, O., and Huago, R. Q. (2017). Contamination status of arsenic in fish and shellfish from three river basins in Ghana. Environ. Monit. Assess. 189, 400. doi: 10.1007/s10661-017-6118-9
George, C. M., Sima, L., Arias, M. H. J., Mihalic, J., Cabrera, L. Z., Danz, D., et al. (2014). Arsenic exposure in drinking water: an unrecognized health threat in Peru. Bull. World Health Organ. 92, 565–572. doi: 10.2471/BLT.13.128496
Goswami, A., Raul, P. K., and Purkait, M. K. (2012). Arsenic adsorption using copper (II) oxide nanoparticles. Chem. Eng. Res. Design 90, 1387–1396. doi: 10.1016/j.cherd.2011.12.006
Hocaoglu, S. M., Wakui, Y., and Suzuki, T. M. (2019). Separation of arsenic(V) by composite adsorbents of metal oxide nanoparticles immobilized on silica flakes and use of adsorbent coated alumina tubes as an alternative method. J. Water Proc. Eng. 27, 134–142. doi: 10.1016/j.jwpe.2018.11.014
Hokkanen, S., Doshi, B., Srivastava, V., Puro, L., and Koivula, R. (2019). Arsenic (III) removal from water by hydroxyapatite-bentonite clay-nanocrystalline cellulose. Environ. Prog. Sustain. Energy 38, 13147. doi: 10.1002/ep.13147
Hosny, N. M., Gomaa, I., and Elmahgary, M. G. (2023). Adsorption of polluted dyes from water by transition metal oxides: a review. Appl. Surf. Sci. Adv. 15, 100395. doi: 10.1016/j.apsadv.2023.100395
Hua, J. (2015). Synthesis and characterization of bentonite based inorgano–organo-composites and their performances for removing arsenic from water. Appl. Clay Sci. 114, 239–246. doi: 10.1016/j.clay.2015.06.005
Hua, J. (2018). Adsorption of low-concentration arsenic from water by co-modified bentonite with manganese oxides and poly(dimethyldiallylammonium chloride). J. Environ. Chem. Eng. 6, 156–168. doi: 10.1016/j.jece.2017.11.062
Huang, Q., Song, S., Chen, Z., Hu, B., Chen, J., and Wang, X. (2019). Biochar-based materials and their applications in removal of organic contaminants from wastewater: state-of-the-art review. Biochar 1, 45–73. doi: 10.1007/s42773-019-00006-5
Ilgen, A. G., Rychagov, S. N., and Trainor, T. P. (2011). Arsenic speciation and transport associated with the release of spent geothermal fluids in Mutnovsky field (Kamchatka, Russia). Chem. Geol. 288, 115–132. doi: 10.1016/j.chemgeo.2011.07.010
Irunde, R., Ligate, F. J., Ijumulana, J., Ahmad, A., Maity, J. P., Hamis, R., et al. (2023). The natural magnesite efficacy on arsenic extraction from water and alkaline influence on metal release in water. Applied Geochemistry 105705. doi: 10.1016/j.apgeochem.2023.105705
Jia, Y., Wang, L., Li, S., Cao, J., and Yang, Z. (2018). Species-specific bioaccumulation and correlated health risk of arsenic compounds in freshwater fish from a typical mine-impacted river. Sci. Total Environm. 625, 600–607. doi: 10.1016/j.scitotenv.2017.12.328
Jin, H., Capareda, S., Chang, Z., Gao, J., Xu, Y., and Zhang, J. (2014). Biochar pyrolytically produced from municipal solid wastes for aqueous As(V) removal: adsorption property and its improvement with KOH activation. Bioresour. Technol. 169, 622–629. doi: 10.1016/j.biortech.2014.06.103
Jjagwe, J., Olupot, P. W., and Carrara, S. (2023). Iron oxide nanoparticles/nanocomposites derived from steel and iron wastes for water treatment: a review. J. Environ. Manage. 343, 118236. doi: 10.1016/j.jenvman.2023.118236
Jovanovic, D., Jakovljević, B., Rašić-Milutinović, Z., Paunović, K., Peković, G., and Knezević, T. (2011). Arsenic occurrence in drinking water supply systems in ten municipalities in Vojvodina Region, Serbia. Environ. Res. 111, 315–318. doi: 10.1016/j.envres.2010.11.014
Kaya, G., and Turkoglu, S. (2017). Bioaccumulation of heavy metals in various tissues of some fish species and green tiger shrimp (Penaeus semisulcatus) from Iskenderun Bay, Turkey, and Risk Assessment for Human Health. Biol. Trace Elem. Res. 180, 314–326. doi: 10.1007/s12011-017-0996-0
Koley, S. (2021). Contemporary practices in groundwater arsenic remediation and wastewater management in West Bengal, India: a systematic review. Int. J. Adv. Technol. Eng. Explorat. 8, 797–823. doi: 10.19101/IJATEE.2021.874281
Kumar, S., Nair, R. R., Pillai, P. B., Gupta, S. N., Iyengar, M. A. R., and Sood, A. K. (2014). Graphene oxide–MnFe 2 O 4 magnetic nanohybrids for efficient removal of lead and arsenic from water. ACS Appl. Mater. Interfaces 6, 17426–17436. doi: 10.1021/am504826q
Lan, J., Wang, B., Bo, C., Gong, B., and Ou, J. (2023). Progress on fabrication and application of activated carbon sphere in recent decade. J. Indust. Eng. Chem. 120, 47–72. doi: 10.1016/j.jiec.2022.12.045
Lawson, M., Polya, D. A., Boyce, A. J., Bryant, C., and Ballentine, C. J. (2016). Tracing organic matter composition and distribution and its role on arsenic release in shallow Cambodian groundwaters. Geochim. Cosmochim. Acta 178, 160–177. doi: 10.1016/j.gca.2016.01.010
Lee, E., Han, Y., Park, J., Hong, J., Silva, R. A., Kim, S., et al. (2015). Bioleaching of arsenic from highly contaminated mine tailings using Acidithiobacillus thiooxidans. J. Environ. Manage. 147, 124–131. doi: 10.1016/j.jenvman.2014.08.019
Li, S., Wang, M., Yang, Q., Wang, H., Zhu, J., Zheng, B., et al. (2013). Enrichment of arsenic in surface water, stream sediments and soils in Tibet. J Geochem Explor 135, 104–116. doi: 10.1016/j.gexplo.2012.08.020
Li, Z., Jean, J.-S., Jiang, W.-T., Chang, P.-H., Chen, C.-J., and Liao, L. (2011). Removal of arsenic from water using Fe-exchanged natural zeolite. J. Hazard. Mater. 187, 318–323. doi: 10.1016/j.jhazmat.2011.01.030
Li, Z., Wang, L., Meng, J., Liu, X., Xu, J., Wang, F., et al. (2018). Zeolite-supported nanoscale zero-valent iron: new findings on simultaneous adsorption of Cd(II), Pb(II), and As(III) in aqueous solution and soil. J. Hazard. Mater. 344, 1–11. doi: 10.1016/j.jhazmat.2017.09.036
Lin, Y.-C., Chang-Chien, G.-P., Chiang, P.-C., Chen, W.-H., and Lin, Y.-C. (2013). Multivariate analysis of heavy metal contaminations in seawater and sediments from a heavily industrialized harbor in Southern Taiwan. Mar. Pollut. Bull. 76, 266–275. doi: 10.1016/j.marpolbul.2013.08.027
Liu, B., Jian, M., Liu, R., Yao, J., and Zhang, X. (2015). Highly efficient removal of arsenic(III) from aqueous solution by zeolitic imidazolate frameworks with different morphology. Colloids Surf. A Physicochem. Eng. Asp. 481, 358–366. doi: 10.1016/j.colsurfa.2015.06.009
Liu, C.-W., Liang, C.-P., Lin, K.-H., Jang, C.-S., Wang, S.-W., Huang, Y.-K., et al. (2007). Bioaccumulation of arsenic compounds in aquacultural clams (Meretrix lusoria) and assessment of potential carcinogenic risks to human health by ingestion. Chemosphere 69, 128–134. doi: 10.1016/j.chemosphere.2007.04.038
Liu, D.-G., Min, X.-B., Ke, Y., Chai, L.-Y., Liang, Y., Li, Y.-C., et al. (2018). Co-treatment of flotation waste, neutralization sludge, and arsenic-containing gypsum sludge from copper smelting: solidification/stabilization of arsenic and heavy metals with minimal cement clinker. Environ. Sci. Pollut. Res. 25, 7600–7607. doi: 10.1007/s11356-017-1084-x
Liu, Z., Zhang, F.-S., and Sasai, R. (2010). Arsenate removal from water using Fe3O4-loaded activated carbon prepared from waste biomass. Chemical Engineering Journal 160, 57–62. doi: 10.1016/j.cej.2010.03.003
Lodeiro, P., Kwan, S. M., Perez, J. T., González, L. F., Gérente, C., Andrès, Y., et al. (2013). Novel Fe loaded activated carbons with tailored properties for As(V) removal: Adsorption study correlated with carbon surface chemistry. Chem. Eng. J. 215–216, 105–112. doi: 10.1016/j.cej.2012.11.052
Luo, C., Routh, J., Luo, D., Wei, L., and Liu, Y. (2021). Arsenic in the Pearl River Delta and its related waterbody, South China: occurrence and sources, a review. Geosci. Lett. 8, 12. doi: 10.1186/s40562-021-00185-9
Macedo-Miranda, M. G., and Olguín, M. T. (2007). Arsenic sorption by modified clinoptilolite–heulandite rich tuffs. J. Incl. Phenom. Macrocycl. Chem. 59, 131–142. doi: 10.1007/s10847-007-9306-3
Mahanta, N., and Chen, J. P. (2013). A novel route to the engineering of zirconium immobilized nano-scale carbon for arsenate removal from water. J. Mater. Chem. A Mater. 1, 8636. doi: 10.1039/c3ta10858a
Mahesh, N., Balakumar, S., Shyamalagowri, S., Manjunathan, J., Pavithra, M. K. S., Babu, P. S., et al. (2022). Carbon-based adsorbents as proficient tools for the removal of heavy metals from aqueous solution: a state of art-review emphasizing recent progress and prospects. Environ. Res. 213, 113723. doi: 10.1016/j.envres.2022.113723
Marcillo, C. E., García Prado, G., Copeland, N., and Krometis, L. H. (2020). Drinking water quality and consumer perceptions at the point-of-use in San Rafael Las Flores, Guatemala. Water Pract. Technol. 15, 374–385. doi: 10.2166/wpt.2020.025
Martinson, C. A., and Reddy, K. J. (2009). Adsorption of arsenic(III) and arsenic(V) by cupric oxide nanoparticles. J. Colloid Interface Sci. 336, 406–411. doi: 10.1016/j.jcis.2009.04.075
McCleskey, R. B., Nordstrom, D. K., Hurwitz, S., Colman, D. R., Roth, D. A., Johnson, M., et al. (2022). The source, fate, and transport of arsenic in the Yellowstone hydrothermal system - an overview. J. Volcanol. Geotherm. Res. 432, 107709. doi: 10.1016/j.jvolgeores.2022.107709
Medrano, M. J., Boix, R., Pastor-Barriuso, R., Palau, M., Damián, J., Ramis, R., et al. (2010). Arsenic in public water supplies and cardiovascular mortality in Spain. Environ. Res. 110, 448–454. doi: 10.1016/j.envres.2009.10.002
Mishra, A. K., and Ramaprabhu, S. (2011). Functionalized graphene sheets for arsenic removal and desalination of sea water. Desalination 282, 39–45. doi: 10.1016/j.desal.2011.01.038
Mishra, P. K., and Rai, P. K. (2019). Ultrafast removal of arsenic using solid solution of aero-gel based Ce1-XTixO2-Y oxide nanoparticles. Chemosphere 217, 483–495. doi: 10.1016/j.chemosphere.2018.11.003
Mladenov, N., Wolski, P., Hettiarachchi, G. M., Murray-Hudson, M., Enriquez, H., Damaraju, S., et al. (2014). Abiotic and biotic factors influencing the mobility of arsenic in groundwater of a through-flow island in the Okavango Delta, Botswana. J. Hydrol (Amst) 518, 326–341. doi: 10.1016/j.jhydrol.2013.09.026
Mohan, D., and Pittman, C. U. (2007). Arsenic removal from water/wastewater using adsorbents—A critical review. J. Hazard. Mater. 142, 1–53. doi: 10.1016/j.jhazmat.2007.01.006
Mohd-Salleh, S. N. A., Shaylinda, M. Z. N., Othman, N., Azizan, M. O., Yashni, G., and Afnizan, W. M. W. (2020). Sustainability analysis on landfilling and evaluation of characteristics in landfill leachate: a case study. IOP Conf. Ser. Mater. Sci. Eng. 736, 072002. doi: 10.1088/1757-899X/736/7/072002
Mojiri, A., and Zhou, J. (2023). Production of biochar from biowaste and its application in wastewater treatment. Curr. Dev. Biotechnol. Bioeng. 7, 149–193. doi: 10.1016/B978-0-323-91873-2.00001-7
Mojiri, A., Zhou, J. L., Robinson, B., Ohashi, A., Ozaki, N., Kindaichi, T., et al. (2020). Pesticides in aquatic environments and their removal by adsorption methods. Chemosphere 253, 126646. doi: 10.1016/j.chemosphere.2020.126646
Mondal, P., Balo Majumder, C., and Mohanty, B. (2007). Removal of Trivalent Arsenic (As(III)) from Contaminated Water by Calcium Chloride (CaCl 2)-Impregnated Rice Husk Carbon. Ind. Eng. Chem. Res. 46, 2550–2557. doi: 10.1021/ie060702i
Mondal, P., Majumder, C. B., and Mohanty, B. (2008). Effects of adsorbent dose, its particle size and initial arsenic concentration on the removal of arsenic, iron and manganese from simulated ground water by Fe3+ impregnated activated carbon. J. Hazard. Mater. 150, 695–702. doi: 10.1016/j.jhazmat.2007.05.040
Moradi, Z., Alihosseini, A., and Ghadami, A. (2023). Adsorption removal of arsenic from aqueous solution by carboxy methyl Cellulose(CMC) modified with montmorillonite. Results Mater. 17, 100378. doi: 10.1016/j.rinma.2023.100378
Mosai, A. K., Richards, H., and Tutu, H. (2022). “Modelling the transport of pollutants from mine dump and landfill sites to groundwater and surface water,” in IMWA 2022–Reconnect, eds. J. Pope, C. Wolkersdorfer, R. Rait, D. Trumm, H. Christenson, and K. Wolkersdorfer (Bella Vista, AR: IMWA), 273–279.
Mueller, B., and Hug, S. J. (2018). Climatic variations and de-coupling between arsenic and iron in arsenic contaminated ground water in the lowlands of Nepal. Chemosphere 210, 347–358. doi: 10.1016/j.chemosphere.2018.07.024
Muensri, P., and Danwittayakul, S. (2017). Removal of arsenic from groundwater using nano-metal oxide adsorbents. Key Eng. Mater. 751, 766–772. doi: 10.4028/www.scientific.net/KEM.751.766
Mukherjee, A., Fryar, A. E., Eastridge, E. M., Nally, R. S., Chakraborty, M., and Scanlon, B. R. (2018). Controls on high and low groundwater arsenic on the opposite banks of the lower reaches of River Ganges, Bengal basin, India. Sci. Total Environm. 645, 1371–1387. doi: 10.1016/j.scitotenv.2018.06.376
Naseem, T., and Durrani, T. (2021). The role of some important metal oxide nanoparticles for wastewater and antibacterial applications: a review. Environm. Chem. Ecotoxicol. 3, 59–75. doi: 10.1016/j.enceco.2020.12.001
Navarathna, C. M., Karunanayake, A. G., Gunatilake, S. R., Pittman, C. U., Perez, F., Mohan, D., et al. (2019). Removal of Arsenic(III) from water using magnetite precipitated onto Douglas fir biochar. J. Environ. Manage. 250, 109429. doi: 10.1016/j.jenvman.2019.109429
Neamtiu, I., Bloom, M. S., Gati, G., Goessler, W., Surdu, S., Pop, C., et al. (2015). Pregnant women in Timis County, Romania are exposed primarily to low-level 10μg/l) arsenic through residential drinking water consumption. Int. J. Hyg. Environ. Health 218, 371–379. doi: 10.1016/j.ijheh.2015.01.004
Nguyen, P. M., and Mulligan, C. N. (2023). Study on the influence of water composition on iron nail corrosion and arsenic removal performance of the Kanchan arsenic filter (KAF). Journal of Hazard. Mater. Adv. 10, 100285. doi: 10.1016/j.hazadv.2023.100285
Niazi, N. K., Bibi, I., Shahid, M., Ok, Y. S., Shaheen, S. M., Rinklebe, J., et al. (2018). Arsenic removal by Japanese oak wood biochar in aqueous solutions and well water: Investigating arsenic fate using integrated spectroscopic and microscopic techniques. Sci. Total Environm 621, 1642–1651. doi: 10.1016/j.scitotenv.2017.10.063
Nikić, J., Watson, M., Tenodi, K. Z., Dalmacija, B., and Agbaba, J. (2023). Pilot study on arsenic removal from phosphate rich groundwater by in-line coagulation and adsorption. Journal of Hazardous Materials Advances 10, 100280. doi: 10.1016/j.hazadv.2023.100280
Nwankwo, C. B., Hoque, M. A., Islam, M. A., and Dewan, A. (2020). Groundwater constituents and trace elements in the basement aquifers of africa and sedimentary aquifers of Asia: medical hydrogeology of drinking water minerals and toxicants. Earth Syst. Environm. 4, 369–384. doi: 10.1007/s41748-020-00151-z
Nyanza, E. C., Dewey, D., Thomas, D. S. K., Davey, M., and Ngallaba, S. E. (2014). Spatial distribution of mercury and arsenic levels in water, soil and cassava plants in a community with long history of gold mining in Tanzania. Bull. Environ. Contam. Toxicol. 93, 716–721. doi: 10.1007/s00128-014-1315-5
Oladoye, P. O. (2022). Natural, low-cost adsorbents for toxic Pb(II) ion sequestration from (waste)water: a state-of-the-art review. Chemosphere 287, 132130. doi: 10.1016/j.chemosphere.2021.132130
Ortiz Letechipia, J., González-Trinidad, J., Júnez-Ferreira, H. E., Bautista-Capetillo, C., Robles-Rovelo, C. O., Contreras Rodríguez, A. R., et al. (2022). Aqueous arsenic speciation with hydrogeochemical modeling and correlation with fluorine in groundwater in a semiarid region of Mexico. Water (Basel) 14, 519. doi: 10.3390/w14040519
Otter, R. R., Bailey, F. C., Fortner, A. M., and Adams, S. M. (2012). Trophic status and metal bioaccumulation differences in multiple fish species exposed to coal ash-associated metals. Ecotoxicol. Environ. Saf. 85, 30–36. doi: 10.1016/j.ecoenv.2012.08.022
Ouattara, A. A., Yao, K. M., Soro, M. P., Diaco, T., and Trokourey, A. (2018). Arsenic and trace metals in three west african rivers: concentrations, partitioning, and distribution in particle-size fractions. Arch. Environ. Contam. Toxicol. 75, 449–463. doi: 10.1007/s00244-018-0543-9
Outa, J. O., Kowenje, C. O., Plessl, C., and Jirsa, F. (2020). Distribution of arsenic, silver, cadmium, lead and other trace elements in water, sediment and macrophytes in the Kenyan part of Lake Victoria: spatial, temporal and bioindicative aspects. Environ. Sci. Pollut. Res. 27, 1485–1498. doi: 10.1007/s11356-019-06525-9
Palmer, M. J., Chételat, J., Richardson, M., Jamieson, H. E., and Galloway, J. M. (2019). Seasonal variation of arsenic and antimony in surface waters of small subarctic lakes impacted by legacy mining pollution near Yellowknife, NT, Canada. Sci. Total Environm 684, 326–339. doi: 10.1016/j.scitotenv.2019.05.258
Pan, J., Pan, J.-F., and Wang, M. (2014). Trace elements distribution and ecological risk assessment of seawater and sediments from Dingzi Bay, Shandong Peninsula, North China. Mar. Pollut. Bull. 89, 427–434. doi: 10.1016/j.marpolbul.2014.10.022
Pedretti, D., Luoma, S., Ruskeeniemi, T., and Backman, B. (2019). A geologically-based approach to map arsenic risk in crystalline aquifers: analysis of the Tampere region, Finland. Geoscience Frontiers 10, 1731–1741. doi: 10.1016/j.gsf.2018.12.004
Pena, M., Meng, X., Korfiatis, G. P., and Jing, C. (2006). Adsorption Mechanism of Arsenic on Nanocrystalline Titanium Dioxide. Environ. Sci. Technol. 40, 1257–1262. doi: 10.1021/es052040e
Perera, P. C. T., Sundarabarathy, T. V., Sivananthawerl, T., Kodithuwakku, S. P., and Edirisinghe, U. (2016). Arsenic and cadmium contamination in water, sediments and fish is a consequence of paddy cultivation: evidence of river pollution in Sri Lanka. Achievements in the Life Sciences 10, 144–160. doi: 10.1016/j.als.2016.11.002
Peshut, P. J., Morrison, R. J., and Brooks, B. A. (2008). Arsenic speciation in marine fish and shellfish from American Samoa. Chemosphere 71, 484–492. doi: 10.1016/j.chemosphere.2007.10.014
Pham, T. T., Ngo, H. H., Tran, V. S., and Nguyen, M. K. (2020). Removal of As (V) from the aqueous solution by a modified granular ferric hydroxide adsorbent. Sci. Total Environm. 706, 135947. doi: 10.1016/j.scitotenv.2019.135947
Pillewan, P., Mukherjee, S., Kumar Meher, A., Rayalu, S., and Bansiwal, A. (2014). Removal of arsenic (III) and arsenic (V) using copper exchange zeolite-a. Environ. Prog. Sustain Energy 33, 1274–1282. doi: 10.1002/ep.11933
Powell, C. D., Guo, S., Godret-Miertschin, L. M., Ventura, K., Lounsbury, A. W., Clark, C. A., et al. (2020). Magnetically recoverable carbon-coated iron carbide with arsenic adsorptive removal properties. SN Appl. Sci. 2, 1690. doi: 10.1007/s42452-020-03491-7
Prasad Ahirvar, B., Das, P., Srivastava, V., and Kumar, M. (2023). Perspectives of heavy metal pollution indices for soil, sediment, and water pollution evaluation: an insight. Total Environm. Res. Themes. 6, 100039. doi: 10.1016/j.totert.2023.100039
Qiu, S., Yan, L., and Jing, C. (2019). Simultaneous removal of arsenic and antimony from mining wastewater using granular TiO2: Batch and field column studies. J. Environm. Sci. 75, 269–276. doi: 10.1016/j.jes.2018.04.001
Qu, G., Jia, P., Zhang, T., Li, Z., Chen, C., and Zhao, Y. (2022). UiO-66(Zr)-derived t-zirconia with abundant lattice defect for remarkably enhanced arsenic removal. Chemosphere 288, 132594. doi: 10.1016/j.chemosphere.2021.132594
Rahman, Z., and Singh, V. P. (2018). Assessment of heavy metal contamination and Hg-resistant bacteria in surface water from different regions of Delhi, India. Saudi J. Biol. Sci. 25, 1687–1695. doi: 10.1016/j.sjbs.2016.09.018
Raj, S. K., Sharma, V., Yadav, A., Indurkar, P. D., and Kulshrestha, V. (2023). Nano-alumina wrapped carbon microspheres for ultrahigh elimination of pentavalent arsenic and fluoride from potable water. J. Indust. Eng. Chem. 117, 402–413. doi: 10.1016/j.jiec.2022.10.028
Rajendran, S., Priya, A. K., Senthil Kumar, P., Hoang, T. K. A., Sekar, K., Chong, K. Y., et al. (2022). A critical and recent developments on adsorption technique for removal of heavy metals from wastewater-a review. Chemosphere 303, 135146. doi: 10.1016/j.chemosphere.2022.135146
Ramos Ramos, O. E., Cáceres, L. F., Ormachea Muñoz, M. R., Bhattacharya, P., Quino, I., Quintanilla, J., et al. (2012). Sources and behavior of arsenic and trace elements in groundwater and surface water in the Poopó Lake Basin, Bolivian Altiplano. Environ. Earth Sci. 66, 793–807. doi: 10.1007/s12665-011-1288-1
Rango, T., Bianchini, G., Beccaluva, L., and Tassinari, R. (2010). Geochemistry and water quality assessment of central Main Ethiopian Rift natural waters with emphasis on source and occurrence of fluoride and arsenic. J. Afric. Earth Sci. 57, 479–491. doi: 10.1016/j.jafrearsci.2009.12.005
Rowland, H. A. L., Omoregie, E. O., Millot, R., Jimenez, C., Mertens, J., Baciu, C., et al. (2011). Geochemistry and arsenic behaviour in groundwater resources of the Pannonian Basin (Hungary and Romania). Appl. Geochem. 26, 1–17. doi: 10.1016/j.apgeochem.2010.10.006
Sabzehmeidani, M. M., Mahnaee, S., Ghaedi, M., Heidari, H., and Roy, V. A. L. (2021). Carbon based materials: a review of adsorbents for inorganic and organic compounds. Mater. Adv. 2, 598–627. doi: 10.1039/D0MA00087F
Sahu, N., Singh, J., and Koduru, J. R. (2021). Removal of arsenic from aqueous solution by novel iron and iron–zirconium modified activated carbon derived from chemical carbonization of Tectona grandis sawdust: Isotherm, kinetic, thermodynamic and breakthrough curve modelling. Environ. Res. 200, 111431. doi: 10.1016/j.envres.2021.111431
Saikia, R., Goswami, R., Bordoloi, N., Senapati, K. K., Pant, K. K., Kumar, M., et al. (2017). Removal of arsenic and fluoride from aqueous solution by biomass based activated biochar: Optimization through response surface methodology. J. Environ. Chem. Eng. 5, 5528–5539. doi: 10.1016/j.jece.2017.10.027
Salem Attia, T. M., Hu, X. L., and Yin, D. Q. (2014). Synthesised magnetic nanoparticles coated zeolite (MNCZ) for the removal of arsenic (As) from aqueous solution. J. Ex.p Nanosci. 9, 551–560. doi: 10.1080/17458080.2012.677549
Sanjrani, M. A., Zhou, B., Zhao, H., Bhutto, S. A., Muneer, A. S., and Xia, S. B. (2019). Arsenic contaminated groundwater in china and its treatment options, a review. Appl. Ecol. Environ. Res. 17, 1655–1683. doi: 10.15666/aeer/1702_16551683
Sarkar, A., and Paul, B. (2016). The global menace of arsenic and its conventional remediation - a critical review. Chemosphere 158, 37–49. doi: 10.1016/j.chemosphere.2016.05.043
Sattar, M. S., Shakoor, M. B., Ali, S., Rizwan, M., Niazi, N. K., and Jilani, A. (2019). Comparative efficiency of peanut shell and peanut shell biochar for removal of arsenic from water. Environ. Sci. Pollut. Res. 26, 18624–18635. doi: 10.1007/s11356-019-05185-z
Scandelai, A. P. J., Zotesso, J. P., Vicentini, J. C. M., Cardozo Filho, L., and Tavares, C. R. G. (2021). Intensification of supercritical water oxidation (ScWO) by ion exchange with zeolite for the reuse of landfill leachates. Sci. Total Environm. 794, 148584. doi: 10.1016/j.scitotenv.2021.148584
Schiopu, A.-M., and Gavrilescu, M. (2010). Municipal solid waste landfilling and treatment of resulting liquid effluents. Environ. Eng. Manag. J 9, 993–1019. doi: 10.30638/eemj.2010.133
Sener, E., Sener, S., and Bulut, C. (2023). Assessment of heavy metal pollution and quality in lake water and sediment by various index methods and GIS: a case study in Beyşehir Lake, Turkey. Mar. Pollut. Bull. 192, 115101. doi: 10.1016/j.marpolbul.2023.115101
Sener, S., and Karakuş, M. (2017). Investigating water quality and arsenic contamination in drinking water resources in the Tavşanli District (Kütahya, Western Turkey). Environ. Earth Sci. 76, 750. doi: 10.1007/s12665-017-7101-z
Senila, M., Levei, E., Cadar, O., Senila, L. R., Roman, M., Puskas, F., et al. (2017). Assessment of availability and human health risk posed by arsenic contaminated well waters from timis-bega area, Romania. J. Anal. Methods Chem. 2017, 1–7. doi: 10.1155/2017/3037651
Shah, A. Q., Kazi, T. G., Arain, M. B., Jamali, M. K., Afridi, H. I., Jalbani, N., et al. (2009). Accumulation of arsenic in different fresh water fish species – potential contribution to high arsenic intakes. Food Chem. 112, 520–524. doi: 10.1016/j.foodchem.2008.05.095
Shao, P., Ding, L., Luo, J., Luo, Y., You, D., Zhang, Q., et al. (2019). Lattice-defect-enhanced adsorption of arsenic on zirconia nanospheres: a combined experimental and theoretical study. ACS Appl. Mater. Interfaces 11, 29736–29745. doi: 10.1021/acsami.9b06041
Sharifi, R., Moore, F., and Keshavarzi, B. (2016). Mobility and chemical fate of arsenic and antimony in water and sediments of Sarouq River catchment, Takab geothermal field, northwest Iran. J. Environ. Manage. 170, 136–144. doi: 10.1016/j.jenvman.2016.01.018
Sharifi, Z., and Sinegani, A. (2012). Assessment of arsenic, nitrate and phosphorus pollutions in shallow groundwater of the rural area in Kurdistan province (Iran). Current World Environment 7, 233–241. doi: 10.12944/CWE.7.2.07
Sheikhmohammadi, A., Dahaghin, Z., Mohseni, S. M., Sarkhosh, M., Azarpira, H., Atafar, Z., et al. (2018). The synthesis and application of the SiO 2 @Fe 3 O 4 @MBT nanocomposite as a new magnetic sorbent for the adsorption of arsenate from aqueous solutions: modeling, optimization, and adsorption studies. J. Mol. Liq. 255, 313–323. doi: 10.1016/j.molliq.2018.01.164
Sheng, G., Li, Y., Yang, X., Ren, X., Yang, S., Hu, J., et al. (2012). Efficient removal of arsenate by versatile magnetic graphene oxide composites. RSC Adv. 2, 12400. doi: 10.1039/c2ra21623j
Shevade, S., and Ford, R. G. (2004). Use of synthetic zeolites for arsenate removal from pollutant water. Water Res. 38, 3197–3204. doi: 10.1016/j.watres.2004.04.026
Siddiqui, S. I., and Chaudhry, S. A. (2017). Iron oxide and its modified forms as an adsorbent for arsenic removal: A comprehensive recent advancement. Proc. Safety Environm. Prot. 111, 592–626. doi: 10.1016/j.psep.2017.08.009
Siddiqui, S. I., Singh, P. N., Tara, N., Pal, S., Chaudhry, S. A., and Sinha, I. (2020). Arsenic removal from water by starch functionalized maghemite nano-adsorbents: thermodynamics and kinetics investigations. Colloid Interface Sci. Commun. 36, 100263. doi: 10.1016/j.colcom.2020.100263
Singh, P., Sarswat, A., Pittman, C. U., Mlsna, T., and Mohan, D. (2020). Sustainable low-concentration arsenite [As(III)] removal in single and multicomponent systems using hybrid iron oxide–biochar nanocomposite adsorbents—a mechanistic study. ACS Omega 5, 2575–2593. doi: 10.1021/acsomega.9b02842
Šlejkovec, Z., Stajnko, A., Falnoga, I., Lipej, L., Mazej, D., Horvat, M., et al. (2014). Bioaccumulation of arsenic species in rays from the Northern Adriatic Sea. Int. J. Mol. Sci. 15, 22073–22091. doi: 10.3390/ijms151222073
Smedley, P. L., and Kinniburgh, D. G. (2002). A review of the source, behaviour and distribution of arsenic in natural waters. Appl. Geochem. 17, 517–568. doi: 10.1016/S.0883-2927(02)00018-5
Sø, H. U., Postma, D., Vi, M. L., Pham, T. K. T., Kazmierczak, J., Dao, V. N., et al. (2018). Arsenic in Holocene aquifers of the Red River floodplain, Vietnam: effects of sediment-water interactions, sediment burial age and groundwater residence time. Geochim. Cosmochim. Acta 225, 192–209. doi: 10.1016/j.gca.2018.01.010
Son, H. X., Hao, P., Van, V. H., Van Hai, N. T., Ngan, N. T. K., Minh, D. N., et al. (2018). Removal of arsenic from water using crumpled graphite oxide. Green Proc. Synth. 7, 404–408. doi: 10.1515/gps-2018-0018
Sprague, D. D., and Vermaire, J. C. (2018). Legacy arsenic pollution of lakes near cobalt, ontario, Canada: arsenic in lake water and sediment remains elevated nearly a century after mining activity has ceased. Water Air Soil Pollut. 229, 87. doi: 10.1007/s11270-018-3741-1
Su, H., Ye, Z., and Hmidi, N. (2017). High-performance iron oxide–graphene oxide nanocomposite adsorbents for arsenic removal. Colloids Surf. A Physicochem. Eng. Asp. 522, 161–172. doi: 10.1016/j.colsurfa.2017.02.065
Sultana, M., Rownok, M. H., Sabrin, M., Rahaman, M. H., and Alam, S. M. N. (2022). A review on experimental chemically modified activated carbon to enhance dye and heavy metals adsorption. Clean Eng. Technol. 6, 100382. doi: 10.1016/j.clet.2021.100382
Sunil, K., Karunakaran, G., Yadav, S., Padaki, M., Zadorozhnyy, V., and Pai, R. K. (2018). Al-Ti2O6 a mixed metal oxide based composite membrane: a unique membrane for removal of heavy metals. Chem. Eng. J. 348, 678–684. doi: 10.1016/j.cej.2018.05.017
Tolkou, A. K., Rada, E. C., Torretta, V., Xanthopoulou, M., Kyzas, G. Z., and Katsoyiannis, I. A. (2023). Removal of Arsenic(III) from water with a combination of graphene oxide (GO) and granular ferric hydroxide (GFH) at the optimum molecular ratio. Carbon (Basel) 9, 10. doi: 10.3390/c9010010
Tomiyasu, T., Nakagawa, M., Kodamatani, H., and Kanzaki, R. (2021). The influence of submarine volcano on seasonal changes in arsenic in the waters of Kagoshima Bay, southwestern Japan. Environ. Earth Sci. 80, 331. doi: 10.1007/s12665-021-09619-1
Torasso, N., Vergara-Rubio, A., Pereira, R., Martinez-Sabando, J., Baudrit, J. R. V., Cerveny, S., et al. (2023). An in situ approach to entrap ultra-small iron oxide nanoparticles inside hydrophilic electrospun nanofibers with high arsenic adsorption. Chem. Eng. J. 454, 140168. doi: 10.1016/j.cej.2022.140168
Uppal, H., Chawla, S., Joshi, A. G., Haranath, D., Vijayan, N., and Singh, N. (2019). Facile chemical synthesis and novel application of zinc oxysulfide nanomaterial for instant and superior adsorption of arsenic from water. J. Clean. Prod. 208, 458–469. doi: 10.1016/j.jclepro.2018.10.023
Varol, M., and Sen, B. (2012). Assessment of nutrient and heavy metal contamination in surface water and sediments of the upper Tigris River, Turkey. Catena (Amst) 92, 1–10. doi: 10.1016/j.catena.2011.11.011
Velarde, L., Nabavi, M. S., Escalera, E., Antti, M.-L., and Akhtar, F. (2023). Adsorption of heavy metals on natural zeolites: a review. Chemosphere 328, 138508. doi: 10.1016/j.chemosphere.2023.138508
Vitela-Rodriguez, A. V., and Rangel-Mendez, J. R. (2013). Arsenic removal by modified activated carbons with iron hydro(oxide) nanoparticles. J. Environ. Manage. 114, 225–231. doi: 10.1016/j.jenvman.2012.10.004
Wang, H., Qi, X., Yan, G., and Shi, J. (2023). Copper-doped ZIF-8 nanomaterials as an adsorbent for the efficient removal of As(V) from wastewater. J. Phys. Chem. Solids 179, 111408. doi: 10.1016/j.jpcs.2023.111408
Wang, J., Liu, R. H., Yu, P., Tang, A. K., Xu, L. Q., and Wang, J. Y. (2012). Study on the pollution characteristics of heavy metals in seawater of Jinzhou Bay. Procedia Environ. Sci. 13, 1507–1516. doi: 10.1016/j.proenv.2012.01.143
Wang, L., Wang, Y., Xu, C., An, Z., and Wang, S. (2011). Analysis and evaluation of the source of heavy metals in water of the River Changjiang. Environ. Monit. Assess. 173, 301–313. doi: 10.1007/s10661-010-1388-5
Wang, N., Ye, Z., Huang, L., Zhang, C., Guo, Y., and Zhang, W. (2022). Arsenic occurrence and cycling in the aquatic environment: a comparison between freshwater and seawater. Water (Basel) 15, 147. doi: 10.3390/w15010147
Wang, S., Gao, B., Li, Y., Mosa, A., Zimmerman, A. R., Ma, L. Q., et al. (2015). Manganese oxide-modified biochars: preparation, characterization, and sorption of arsenate and lead. Bioresour. Technol. 181, 13–17. doi: 10.1016/j.biortech.2015.01.044
Wang, Z., He, B., Pan, X., Zhang, K., Wang, C., Sun, J., et al. (2010). Levels, trends and risk assessment of arsenic pollution in Yangzonghai Lake, Yunnan Province, China. Sci. China Chem. 53, 1809–1817. doi: 10.1007/s11426-010-4039-3
Weber, P., Behr, E. R., Knorr, C. D. L., Vendruscolo, D. S., Flores, E. M. M., Dressler, V. L., et al. (2013). Metals in the water, sediment, and tissues of two fish species from different trophic levels in a subtropical Brazilian river. Microchem. J. 106, 61–66. doi: 10.1016/j.microc.2012.05.004
Wijesekara, S. S., Mayakaduwa, S. S., Siriwardana, A. R., de Silva, N., Basnayake, B. F. A., Kawamoto, K., et al. (2014). Fate and transport of pollutants through a municipal solid waste landfill leachate in Sri Lanka. Environ. Earth Sci. doi: 10.1007/s12665-014-3075-2
Wu, C., Chen, Y., Qian, Z., Chen, H., Li, W., Li, Q., et al. (2023). The effect of extracellular polymeric substances (EPS) of iron-oxidizing bacteria (Ochrobactrum EEELCW01) on mineral transformation and arsenic (As) fate. J. Environm. Sci. 130, 187–196. doi: 10.1016/j.jes.2022.10.004
Wu, L.-K., Wu, H., Liu, Z.-Z., Cao, H.-Z., Hou, G.-Y., Tang, Y.-P., et al. (2018a). Highly porous copper ferrite foam: a promising adsorbent for efficient removal of As(III) and As(V) from water. J. Hazard. Mater. 347, 15–24. doi: 10.1016/j.jhazmat.2017.12.048
Wu, L.-K., Wu, H., Zhang, H.-B., Cao, H.-Z., Hou, G.-Y., Tang, Y.-P., et al. (2018b). Graphene oxide/CuFe2O4 foam as an efficient absorbent for arsenic removal from water. Chem. Eng. J. 334, 1808–1819. doi: 10.1016/j.cej.2017.11.096
Wurl, J., Mendez-Rodriguez, L., and Acosta-Vargas, B. (2014). Arsenic content in groundwater from the southern part of the San Antonio-El Triunfo mining district, Baja California Sur, Mexico. J. Hydrol. (Amst) 518, 447–459. doi: 10.1016/j.jhydrol.2014.05.009
Yan, C., Che, F., Zeng, L., Wang, Z., Du, M., Wei, Q., et al. (2016). Spatial and seasonal changes of arsenic species in Lake Taihu in relation to eutrophication. Sci. Total Environm. 563–564, 496–505. doi: 10.1016/j.scitotenv.2016.04.132
Yang, F., Geng, D., Wei, C., Ji, H., and Xu, H. (2016). Distribution of arsenic between the particulate and aqueous phases in surface water from three freshwater lakes in China. Environ. Sci. Pollut. Res. 23, 7452–7461. doi: 10.1007/s11356-015-5998-x
Yang, X., Wan, Y., Zheng, Y., He, F., Yu, Z., Huang, J., et al. (2019). Surface functional groups of carbon-based adsorbents and their roles in the removal of heavy metals from aqueous solutions: a critical review. Chem. Eng. J. 366, 608–621. doi: 10.1016/j.cej.2019.02.119
Yang, Y., Wei, L., Cui, L., Zhang, M., and Wang, J. (2017). Profiles and risk assessment of heavy metals in great rift lakes, Kenya. Clean (Weinh) 45, 1600825. doi: 10.1002/clen.201600825
Yoon, K., Cho, D.-W., Tsang, D. C. W., Bolan, N., Rinklebe, J., and Song, H. (2017). Fabrication of engineered biochar from paper mill sludge and its application into removal of arsenic and cadmium in acidic water. Bioresour. Technol. 246, 69–75. doi: 10.1016/j.biortech.2017.07.020
Yu, X., Tong, S., Ge, M., Zuo, J., Cao, C., and Song, W. (2013). One-step synthesis of magnetic composites of cellulose@iron oxide nanoparticles for arsenic removal. J. Mater. Chem. A 1, 959–965. doi: 10.1039/C2TA00315E
Yürüm, A., Kocabaş-Atakli, Z. Ö., Sezen, M., Semiat, R., and Yürüm, Y. (2014). Fast deposition of porous iron oxide on activated carbon by microwave heating and arsenic (V) removal from water. Chemical Engineering Journal 242, 321–332. doi: 10.1016/j.cej.2014.01.005
Zaimee, M. Z. A., Sarjadi, M. S., and Rahman, M. L. (2021). Heavy metals removal from water by efficient adsorbents. Water (Basel) 13, 2659. doi: 10.3390/w13192659
Zhang, G., Ren, Z., Zhang, X., and Chen, J. (2013). Nanostructured iron(III)-copper(II) binary oxide: a novel adsorbent for enhanced arsenic removal from aqueous solutions. Water Res. 47, 4022–4031. doi: 10.1016/j.watres.2012.11.059
Zhang, N., Wei, C., and Yang, L. (2013). Occurrence of arsenic in two large shallow freshwater lakes in China and a comparison to other lakes around the world. Microchem. J. 110, 169–177. doi: 10.1016/j.microc.2013.03.014
Zhang, W., Guo, Z., Song, D., Du, S., and Zhang, L. (2018). Arsenic speciation in wild marine organisms and a health risk assessment in a subtropical bay of China. Sci. Total Environm. 626, 621–629. doi: 10.1016/j.scitotenv.2018.01.108
Zhang, W., Miao, A.-J., Wang, N.-X., Li, C., Sha, J., Jia, J., et al. (2022). Arsenic bioaccumulation and biotransformation in aquatic organisms. Environ. Int. 163, 107221. doi: 10.1016/j.envint.2022.107221
Zhang, W., Wang, W.-X., and Zhang, L. (2016). Comparison of bioavailability and biotransformation of inorganic and organic arsenic to two marine fish. Environ. Sci. Technol. 50, 2413–2423. doi: 10.1021/acs.est.5b06307
Zhao, R., Novak, J. T., and Douglas Goldsmith, C. (2013). Treatment of organic matter and methylated arsenic in landfill biogas condensate. Waste Manage. 33, 1207–1214. doi: 10.1016/j.wasman.2013.01.013
Zhu, H., Jia, Y., Wu, X., and Wang, H. (2009). Removal of arsenic from water by supported nano zero-valent iron on activated carbon. J. Hazard. Mater. 172, 1591–1596. doi: 10.1016/j.jhazmat.2009.08.031
Zhu, R., Chen, Q., Zhou, Q., Xi, Y., Zhu, J., and He, H. (2016). Adsorbents based on montmorillonite for contaminant removal from water: a review. Appl. Clay Sci. 123, 239–258. doi: 10.1016/j.clay.2015.12.024
Keywords: adsorption, arsenic removal, bentonite, graphene, nanoparticles, zeolite
Citation: Mojiri A, Razmi E, KarimiDermani B, Rezania S, Kasmuri N, Vakili M and Farraji H (2024) Adsorption methods for arsenic removal in water bodies: a critical evaluation of effectiveness and limitations. Front. Water 6:1301648. doi: 10.3389/frwa.2024.1301648
Received: 25 September 2023; Accepted: 22 January 2024;
Published: 07 February 2024.
Edited by:
Ioannis D. Manariotis, University of Patras, GreeceReviewed by:
Amita Nakarmi, University of Arkansas at Little Rock, United StatesCopyright © 2024 Mojiri, Razmi, KarimiDermani, Rezania, Kasmuri, Vakili and Farraji. This is an open-access article distributed under the terms of the Creative Commons Attribution License (CC BY). The use, distribution or reproduction in other forums is permitted, provided the original author(s) and the copyright owner(s) are credited and that the original publication in this journal is cited, in accordance with accepted academic practice. No use, distribution or reproduction is permitted which does not comply with these terms.
*Correspondence: Amin Mojiri, YW1pbi5tb2ppcmlAZ21haWwuY29t
Disclaimer: All claims expressed in this article are solely those of the authors and do not necessarily represent those of their affiliated organizations, or those of the publisher, the editors and the reviewers. Any product that may be evaluated in this article or claim that may be made by its manufacturer is not guaranteed or endorsed by the publisher.
Research integrity at Frontiers
Learn more about the work of our research integrity team to safeguard the quality of each article we publish.