- 1International Water Research Institute (IWRI), Mohammed VI Polytechnic University, Ben Guerir, Morocco
- 2HydroSciences Montpellier (University Montpellier, CNRS, IRD), Montpellier, France
- 3L3G Laboratory, Department of Earth Sciences, Faculty of Sciences and Techniques, Cadi Ayyad University, Marrakech, Morocco
- 4Center for Remote Sensing Applications (CRSA), Mohammed VI Polytechnic University, Ben Guerir, Morocco
In data-sparse regions and in developing countries such as Morocco, where flooding has serious socio-economic impacts, satellite-based precipitation products open new possibilities for monitoring and modelling water resources and floods. The objective of the study is to explore the possibility of using satellite precipitation products (SPPs) with hydrological models (CREST and MISDc) over 9 basins in Morocco. This work provides a hydrological assessment of three SPPs that have demonstrated good capabilities in reproducing precipitation over different basins in Morocco (GPM IMERG – PERSIANN CDR (PERCDR) and CHIRPS). The two hydrological models are coupled with a stochastic calibration method to provide the different ranges of uncertainties. In addition, we investigate the ability of SPPs on reproducing the November 2014 flood event that affected a large part of Morocco. The results indicated that, in calibration, both hydrological models provided similar performance to reproduce river discharge with observed precipitation or PERSIANN CDR. In validation, the combination of the MISDc model with PERSIANN CDR performed the best, notably allowing a good simulation of the flood hydrographs during the November 2014 event. Future analysis of relationships between SPPs, basin properties, and hydrological modelling technique will allow to find the appropriate combination for different application purposes.
1 Introduction
Ground-based precipitation measurements can be considered as a reference in different water resources studies. These measurements are based on rain gauges; however, due to the spatio-temporal variability of precipitation, it is hard to cover a large area with high precision and accuracy especially in the mountainous basins and developing countries that suffer from data scarcity (Kidd et al., 2017; El Khalki et al., 2018; Brocca et al., 2020). The accuracy in rainfall estimations is essential in many applications from climate change research, weather studies and hydrological modeling (Lu and Yong, 2018; Maggioni and Massari, 2018; Ricciardelli et al., 2018). Satellite observations are becoming a potential alternative to ground-based precipitation thanks to their ability to cover a large spatial scale with different temporal resolutions (Wagner et al., 2012; Camici et al., 2020; Massari et al., 2020).
The recent availability of Satellite Precipitation Products (SPPs) has stimulated the evaluation studies of different SPPs at different levels: precipitation level and hydrological assessment level. In Morocco, most SPPs evaluation studies are based on the comparison against observed precipitation in different regions (Seif-Ennasr et al., 2016; Ouatiki et al., 2017, 2023; El Alaoui El Fels et al., 2022; Rachdane et al., 2022; Salih et al., 2022). Most of these studies have used the Root Mean Square Error, Coefficient of Correlation, and the categorical scores. They concluded that TRMM (Huffman et al., 2010), CHIRPS (Funk et al., 2015), IMERG (Hou et al., 2014) and PERSIANN CDR (Ashouri et al., 2015) are the most accurate SPPs in the Moroccan context. However, only few studies have conducted hydrological assessments of SPPs using hydrological models using different time steps, from hourly data using GPM-IMERG Early Run for flood modeling (Saouabe et al., 2020) and daily for flood modeling (Ouaba et al., 2022) and Continuous modeling of a northern basin of Morocco and 12 basins in Morocco using different SPPs (Tramblay et al., 2016, 2023). Nonetheless, the hydrological evaluation is essential, especially in data scarce regions, to have general guidelines about which SPPs should be used for different hydrological applications.
The hydrological assessment of different SPPs at daily or sub daily time steps and at finer spatial resolution is challenging especially in semi-arid and Mediterranean basins which are characterized by highly variable hydrological response dynamics. Another limitation of SPPs use within hydrological models are the errors resulting from the measurement's nature and retrieval algorithms (Kummerow, 1998; Villarini et al., 2009). Indeed, recent studies have shown that there is an interest in evaluating SPPs and incorporate them in hydrological modeling approaches especially in operational applications (Casse et al., 2015; Tramblay et al., 2016; Beck et al., 2017; Elgamal et al., 2017; Camici et al., 2018, 2020; Massari et al., 2020; Saouabe et al., 2020; Ouaba et al., 2022). However, the selection of the best combination of hydrological model/SPPs may differ depending on the hydrological model formulation and complexity (Quintero et al., 2016; Tramblay et al., 2016), input data characteristics (Satgé et al., 2019), the basin's characteristics (i.e. soil moisture rate decay (El Khalki et al., 2020), topography complexity (Amjad et al., 2020) and quality of observed discharge data.
In order to limit these errors, several studies increased the hydrological performance by the application of bias correction methods (Thiemig et al., 2013; Camici et al., 2018). However, this would not be feasible for catchments with very sparse or even non-existent rain gauges (Tramblay et al., 2016). Using calibrated hydrological models is another way to compensate for the bias of different precipitation datasets, as shown in previous studies (Thiemig et al., 2013). The calibration of model's parameters can be driven by an ensemble of parameter sets instead of one or two for a given precipitation dataset (Heistermann and Kneis, 2011). Also, in case of a long time series of observed data, a differential split-sample test can be used (DSST, KlemeŠ, 1986). It consists in subdividing the whole period into sub-periods with the same length for calibration and validation steps. The limits of this method are the time coverage that is generally limited for the majority of SPPs.
The different hydrological applications using SPPs as input in Morocco are focused on water resources studies applied over one basin at daily time step (Tramblay et al., 2016; Camici et al., 2018; Saouabe et al., 2020; Karmouda et al., 2022; Ouaba et al., 2022) or on several basins using unevaluated satellite precipitation products against observed precipitation (Tramblay et al., 2023). This lack of hydrological applications studies using different hydrological models and SPPs, showed the need to validate hydrological simulations against observed discharge data to assess the capacity to build an operational system for water management and flood forecasting (Trigg et al., 2016).
In this context, three SPPs that have demonstrated good capabilities in reproducing precipitation over different basins in Morocco are considered in this study (Milewski et al., 2015; Tramblay et al., 2016; Ouatiki et al., 2017; Ahmed et al., 2021; El Alaoui El Fels et al., 2022; Karmouda et al., 2022; Salih et al., 2022). (1) IMERG (Integrated Multi-Satellite Retrievals, IMERG of the Global Precipitation Measurement Mission) (Hou et al., 2014), (2) PERSIANN CDR (Sorooshian et al., 2002) and (3) CHIRPS (Funk et al., 2015). These products were used to drive two hydrological models; MISDc (“ModelloIdrologico Semi-Distribuito in continuo”) (Brocca et al., 2011) and CREST (“Coupled Routing and Excess Storage”) (Wang et al., 2011) at daily time step among 9 different basins in terms of morphology and size. The objectives of this study are: (i) Investigating the usefulness of SPPs in simulating discharge to support water resources management activities in ground-data-poor regions, (ii) test the ability of the different SPPs and hydrological models on simulating a flood event in order to test the suitability of near real time hydrological application. The aim of this work is then to test over several basins if the discharge simulated from SPPs can outperform the simulated one from observed precipitation in ground-data-poor locations.
The paper is organized as follows; Section 2 describes the study area and dataset used for this study. Section 3 describes the methodology and the different hydrological models. The obtained results and conclusions are presented in Sections 4 and 5 respectively.
2 Study area and datasets
2.1 Study area
The study area is composed of 9 basins located in the central parts of Morocco (Central and High Atlas Mountains), with different areas ranging from 105 to 2500 km2. The basins' altitudes are ranging from 700 to 4167 m, with semi-arid conditions characterized by an average annual rainfall ranging from 280 to 410 mm/year. All the basins, except for Ait Ouchène and Tillouguite, are intermittent with a high number of days where flow is under 0.1 m3/s. The basins' selection is based on length and quality of discharge data and the absence of dams to study only the natural hydrological regime. The climate is semi-arid with rainfall occurring between November and April. Snowfall is noticed at altitudes above 2500 m (Marchane et al., 2021). To distinguish the snow cover ratio, we used the version 5 of MODIS/Terra Snow Cover Daily (MOD10A1) at 500 m resolution (Hall et al., 2002). The product was evaluated in different studies over the region (Marchane et al., 2017). The maximal average snow ratio showed that the snow-covered area is not very important for the majority of the basins, since the snow only appears from an altitude of 2500 m. The maximum snow ratio values are found for Ourika and Tillouguite with 23 and 24.4% respectively (Table 1). In terms of geology, the basins that are located in the southwest, in the Figure 1, are formed by impermeable formations in the form of plutonic and metamorphic rocks. However, the two northern basins are formed by carbonate formation (Bissour, 2018). The characteristics of these basins are mainly represented in Table 1.
2.2 Datasets
In this section, we present the data sets used in this study including ground observations and satellite precipitation products (Table 2).
2.2.1 Ground observations
Observed rainfall, temperature and discharge data are composing the ground observations. We used the data networks of two Hydraulic Agencies in Morocco: Tensift and Oum Er Rbiaa, consisting of eleven rain gauges, nine discharge stations and two temperature stations with daily time steps, covering the period 2000–2018 (Figure 1). The two temperature stations are located in Tillouguite and Ghdat basins. For the evapotranspiration data, according to Er-Raki et al. (2010), we used the Hargreaves and Samani formula (Hargreaves and Samani, 1982) using minimum and maximum temperature data (Eq 1). This formula has been proved efficient in Morocco (Er-Raki et al., 2010) and is widely used in different studies over the study region (Marchane et al., 2017; El Khalki et al., 2021). Nine out of eleven rain gauges are situated at the basin outlets. Ait Ouchene and Tillouguite basins each have an extra rain gauge.
Where: PET is expressed in (mm/day), Ra is extraterrestrial radiation in (MJ/m2/day) as described by Allen et al. (1998), Ta is air temperature at 2 m height (°C), Tmin, and Tmax are the daily minimum and maximum air temperature respectively.
2.2.2 Satellite precipitation products
In this study, we used three satellite precipitation products over the study zone at daily time step covering the period 2000–2018:
IMERG is an advanced successor of the TRMM from NASA (National Aeronautics and Space Administration) and JAXA (Japan Aerospace Exploration Agency). It is equipped with a Dual-frequency Precipitation Radar (DPR) and a GPM Microwave Imager (GMI). The IMERG product has the ability to detect finer rain and snow by using two frequencies and multi-frequencies radars. IMERG has three different runs (products): IMERG Early: has 4 h latency and it is suitable for operational applications (Floods, landslide risk) (Hou et al., 2014). IMERG Late has 12 h latency and IMERG Final is the calibrated product using Global Precipitation Climatology Center (GPCC) and it has 3.5 months latency. In this study, we used the version 06 of IMERG Early that covers the period 2000-Present, with a temporal resolution of 30 min and a spatial resolution of ~10 km.
PERSIANN product is a precipitation satellite provided by the Center for Hydrometeorology and Remote Sensing with hourly time step and a spatial resolution of ~25 km. It is based on Infrared (IR) brightness temperature imagery generated from geostationary satellites (Hsu et al., 1997). The PERSIANN retrieval algorithm uses an Artificial Neural Network model to estimate precipitation from IR information. The network parameters are adapted using a calibration process based on satellite microwave data whenever rainfall estimates become available. The calibration process is done by integrating an adaptive training algorithm. PERSIANN-Climate Data Record (PERSIANN-CDR) was generated from the PERSIANN algorithm using Gridded Satellite (GridSat-BI), IR data and the National Centers for Environmental Prediction (NCEP) Stage IV radar data. The PERSIANN-CDR product is calibrated using Global Precipitation Climatology Project (GPCP) version 2.2 product at monthly time step (Ashouri et al., 2015). PERSIANN CDR is referred to here as “PERCDR.”
CHIRPS (Climate Hazards Group InfraRed Precipitation with Station data) combine three main data sources: The Climate Hazards group Precipitation climatology (CHPclim), global precipitation climatology based on observed data at 0.05° spatial resolution at monthly time step and TRMM 3B42 precipitation data. The CHPclim uses long-term average satellite rainfall that is used to drive climatological surfaces which has a direct impact on improving performance in mountainous regions. In this study, we used the version 2.2 of CHIRPS that is covering the period 1981 to near present with a spatial resolution of ~5.4 km and a temporal resolution of 1 day (Funk et al., 2015).
As the three satellite rainfall products possess diverse spatial resolutions ranging from 5.4 to 25 km, it is of utmost importance to display how the grids are distributed, represented as the pixel centroid, at the level of the 9 basins (Figure 2). The smallest basin (#7) is covered by 2 PERCDR pixels, 2 IMERG pixels, and 4 CHIRPS pixels.
3 Hydrological models
3.1 MISDc (continuous hydrological model)
The MISDc model (Brocca et al., 2011 - https://github.com/IRPIhydrology/MISDc) is a continuous and distributed two-layers hydrological model. It is predisposed to reproduce soil moisture and simulate the flow of a river over a long daily time series. As the model is lumped it requires as input data: precipitation and air temperature. The model simulates the different processes involved in the transformation of rain into flow (Infiltration, Evapotranspiration, excess soil saturation and percolation). Two components constitute the MISDc model; the first is the soil water balance model (Brocca et al., 2008) which simulates soil moisture for each time step and estimates the initial state of soil saturation. The second component continuously simulates the hydrograph of the observed flow. The two components group together gave 9 parameters to be calibrated (the flow diagram of the model can be found in the Supplementary material).
3.2 Coupled routing and excess storage
Coupled Routing and Excess Storage (CREST) is developed by Wang et al. (2011) and it is designed to simulate floods. CREST is a distributed model developed by the University of Oklahoma (www.hydro.ou.edu) and the NASA SERVIR project (www.servir.net). The version used in this work is the lumped version. The different components of the CREST model are: three layers which control the maximum storage, the routing from one cell to another using the kinematic wave function and a coupling between the flow generation and the routing function (For the case of the distributed version). This coupling allows real scaling of hydrological variables such as soil moisture. The model requires rain, evapotranspiration, basin area, and time step designation. There are 8 model parameters (Table 3).
4 Methods
4.1 Rainfall assessment
The assessment of SPPs was carried out by an intercomparison against observed precipitation at the scale of rain gauge and at daily time scale. Two metrics are calculated to measure the goodness of fit: Pearson correlation coefficient (CC) and the Root Mean Square Deviation (RMSD). The hydrological assessment is based on the basin wide average SPPs compared to the observed precipitation at gauge scale. We considered this approach for the hydrological assessment part because the satellite pixel, over the rain gauge, is covering a large portion of the basin especially for the seven High Atlas basins (< 500 km2).
4.2 Hydrological assessment
The evaluation metric for the hydrological assessment is based on the Kling-Gupta efficiency score (KGE, Gupta et al., 2009; Kling et al., 2012). The KGE values are ranging from -Inf to 1, if the KGE values are higher than −0.41 they indicate a better performance than the mean as a benchmark (Knoben et al., 2019). To investigate the uncertainties in discharge simulation induced by calibration, and to ensure that there are no subjective choices in the selection of calibration and validation samples, we applied a random bootstrap split sample test that combines discontinuous years (Coron, 2013; Arsenault et al., 2018). In this study, we randomly create 100 samples containing a sub-period of 10 years resampled from the whole period (2000–2018). Each sample with a length of 10 years is used in the calibration step. The calibrated parameters are then used in validation for each period when the years are not in common with calibration samples. We also tested the application of the 100 sets of parameters over the whole period in order to select the set of parameters that give the best KGE criteria. This step allows the selection of the appropriate set of parameters of each satellite product and catchment but also the uncertainty related to the choice of the parameter sets. For this step we added two different efficiency scores that are coefficient of correlation (Eq. 2) and Bias (Eq. 3) which are two out of three components of KGE equation.
Where: represents the mean observed discharge and represents the mean of simulate discharge from the different sources of precipitation products.
To assess the reliability of the satellite products on simulating extreme flood events, we selected a regional flood event that occurred in November 2014 covering the totality of the southwestern Mediterranean region (Faccini et al., 2015; Saidi et al., 2020). This event caused significant human and economic damage in Morocco, with a total of 47 individuals were either carried away by violent discharge or trapped under debris from collapsed homes The destructive event garnered widespread attention due to the extensive harm it caused (Saidi et al., 2020). The nine selected basins were also impacted by the event. We analyzed the simulated flood hydrograph using the best set of parameters that gave the best KGE in the calibration. This hydrograph is compared to the observed one as well as the various simulations to show how simulated discharge is affected by the precipitation input. Then we calculated the KGE criteria for the period 20–30 November 2014 only, to select the most appropriate satellite product and hydrological model for simulating an extreme flood event.
5 Results
5.1 Satellite precipitation products assessment
The performances of the three satellite precipitation products against observed precipitation are shown in Figure 3. The assessment was made over the whole period (2000–2018) at daily time scale. The results showed that PERCDR and CHIRPS give, practically, the same results in terms of correlation. We noticed a slight improvement in correlation values toward north, where we found that Ait Ouchene and Tillouguite (basins 8 and 9) gave slightly better results, especially for IMERG and CHIRPS, with a correlation equal to 0.43 and 0.4, respectively, whereas the correlation with PERCDR was equal to 0.37, same results were found in studies conducted by Ouatiki et al. (2023) in the Oum Er Rbiaa basin in Morocco and Salih et al. (2022) in the Tensift region. The RMSD obtained with CHIRPS is slightly greater, up to 9 mm/day, than the two other products, while the IMERG product provided the smallest values of RMSD (under 5 mm/day). Therefore, the limitation of the corrected (PERCDR and CHIRPS) and non-corrected (IMERG) precipitation product is the degradation of both metrics (CC and RMSD) with the slope's degree increases. These results are in parallel with the finds of Amjad et al. (2020) over Turkey where they investigated the impact of topography on the precipitation products accuracy. Moreover, it is worth noting that the inadequate effectiveness of SPPs can be attributed to the fact that the majority of rain gauge stations are located in mountainous areas, where the effectiveness of SSPs is generally limited (Rachdane et al., 2022; Ouatiki et al., 2023).
5.2 Hydrological assessment
The hydrological assessment is performed by using the MISDc and CREST hydrological models and using observed and satellite precipitation products as inputs. The simulated discharge from observed precipitation (QS-obs) is considered as a benchmark simulated river discharge data. Figure 4 shows the calibration and validation results of the two models using the DSST bootstrap method as explained in Sectiond 4–1. According to the calibration results, satellite precipitation products showed different hydrological performances depending on the hydrological model. Taking the number of basins where the median is above 0.5 in KGE, QPERCDR gave similar results (4 basins with KGE > 0.5) as QS-obs (4 basins with KGE > 0.5) using the two models. However, a slight improvement is obtained with the CREST model (5 basins with KGE > 0.5) with a small range of KGE values. QCHIRPS under CREST model showed good results in calibration (all the basins with KGE > 0) compared to MISDc model (4 basins with KGE > 0). The performance of satellite precipitation products and hydrological models deteriorated during the validation phase, with the majority of KGE values falling between 0.08 and 0.5. With comparable outcomes to QS-obs and QPERCDR, the CREST model performed better when employing the four precipitation data sources with a larger range of KGE values compared to MISDc. Whereas, using the measured precipitation and PERCDR, the MISDc model produced good results. Overall, the MISDc model provides best results in terms of uncertainties in validation phase using the different satellite products across the basins. The PERCDR remains the best in the 9 catchments during calibration and validation phases. The IMERG product showed a strong variability in its performances to simulate discharge over the basins. Its performance strongly depends on the hydrological model and the basin, especially high-altitude mountainous basins. This is also the case for CHIRPS that showed a strong variability in its performances depending on the basin and hydrological model, case of basins 2, 8 and 9, in the validation phase, for both models, where the range of KGE values is larger than the other basins, especially for CREST model. Furthermore, in the 100 validation simulations, both the CREST and MISDc models showed acceptable median correlation coefficient ranging between 0.1 and 0.6 for CREST and between 0 and 0.65 for MISDc with higher values using PERCDR. The smallest basin (#7) had the lowest values. CREST had larger uncertainties in bias criteria compared to MISDc for all the precipitation products. Nevertheless, the median values of bias ranged from 0.5 to 2.5 mm/day for CREST and 0.5 to 1.8 mm/day for MISDc (Additional details in the Supplementary material). The models' low performance has been observed in various studies conducted in semi-arid regions (Massari et al., 2014; Camici et al., 2018; Tramblay et al., 2023). This can be attributed to the high interannual variability of climate conditions (Bouizrou et al., 2023). After investigating the 100 set of parameters using the DSST bootstrap method, the best set of parameters from the 100 sets was selected for each satellite precipitation product and models across the basins. The results in calibration and validation showed the same conclusion as above, with best results provided by PERCDR (Figure 5, a detailed figure on coefficient of correlation and bias are presented in Supplementary material). The MISDc showed good results using observed precipitation (and to some extent using PERCDR). The basins #8 and #9 gave good results in term of KGE compared to the other basins when considering observed precipitation for both hydrological models, this is likely to be due to the strong interactions between the quality of the observed discharge data in the different catchments, which can influence model calibration and validation (Tramblay et al., 2023). The KGE of the best set of parameters from the 100 sets is compared to the area of the basins for each product. We found that the models' performance improves as the basin's size increases. The combination of observed precipitation/MISDc followed by PERCDR/MISDc combinations yields a higher correlation coefficient (0.8). On the contrary, the IMERG product shows a negative correlation (-0.7) with basin area, in disagreement with CHIRPS, PERCDR and observed precipitation (Supplementary Figures 1–3). This can be explained by the fact that the large basins represented in this study are mountainous, which results in the low performance of the IMERG product. Tramblay et al. (2023) tested IMERG on the same largest basins (#8 and #9) and obtained the lowest performance by this product.
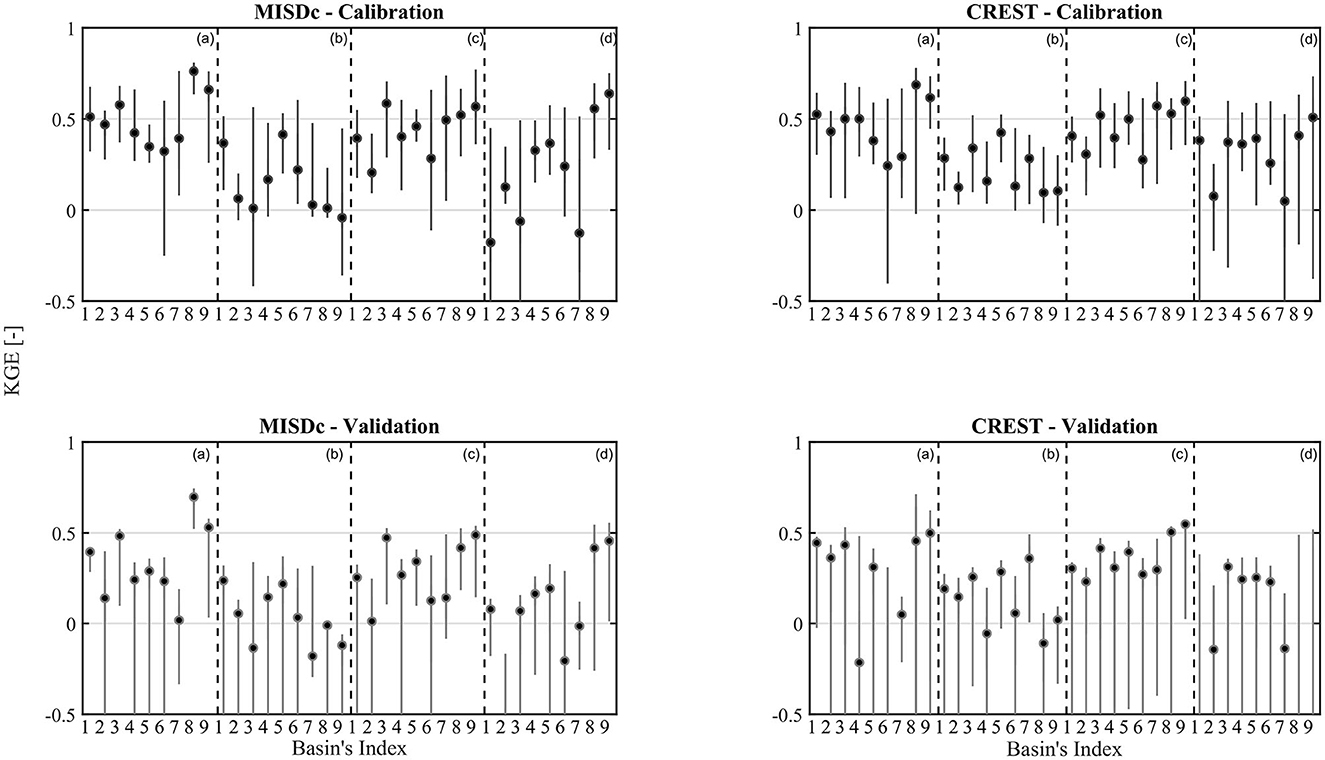
Figure 4. Boxplots of calibration and validation results of the 100 samples of each basin. The observed precipitation is the block (a), IMERG is (b), (c) is PERCDR and (d) represents CHIRPS. The black dot represents the median KGE of each basin represented as index number as referred in the Table 1.
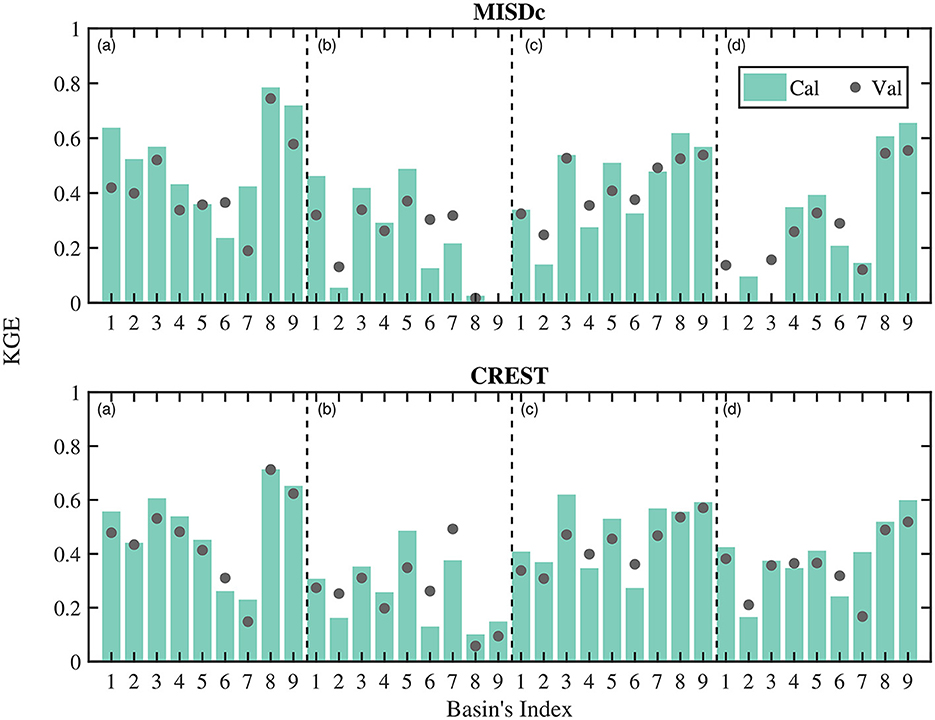
Figure 5. Calibration and validation results given by the best set of parameters of the two models for each basin using four different rainfall products. (a) Observed precipitation, (b) IMERG, (c) PERCDR and (d) CHIRPS.
Knowing that some basins have a limited percentage of snow cover of < 25%, it is essential to determine the influence of snow on the simulation of discharge by the two hydrological models. For this, we sought to know if the satellite products reproduced the observed hydrological cycle of the different basins. Figure 6 shows the average monthly discharge for the period 2000–2018. The MISDc model was forced by the three satellite and observed products to compare the simulations against the observed discharge. The presence of snow did not affect the simulation result since the two basins that receive < 25% of the snow ratio (3 and 9) are still well simulated especially during spring, this can be explained by the fact that the contribution of snow even if it exists, but it is not important. According to El Khalki et al. (2018) and Saidi et al. (2020) the hydrological response is controlled by rainfall.
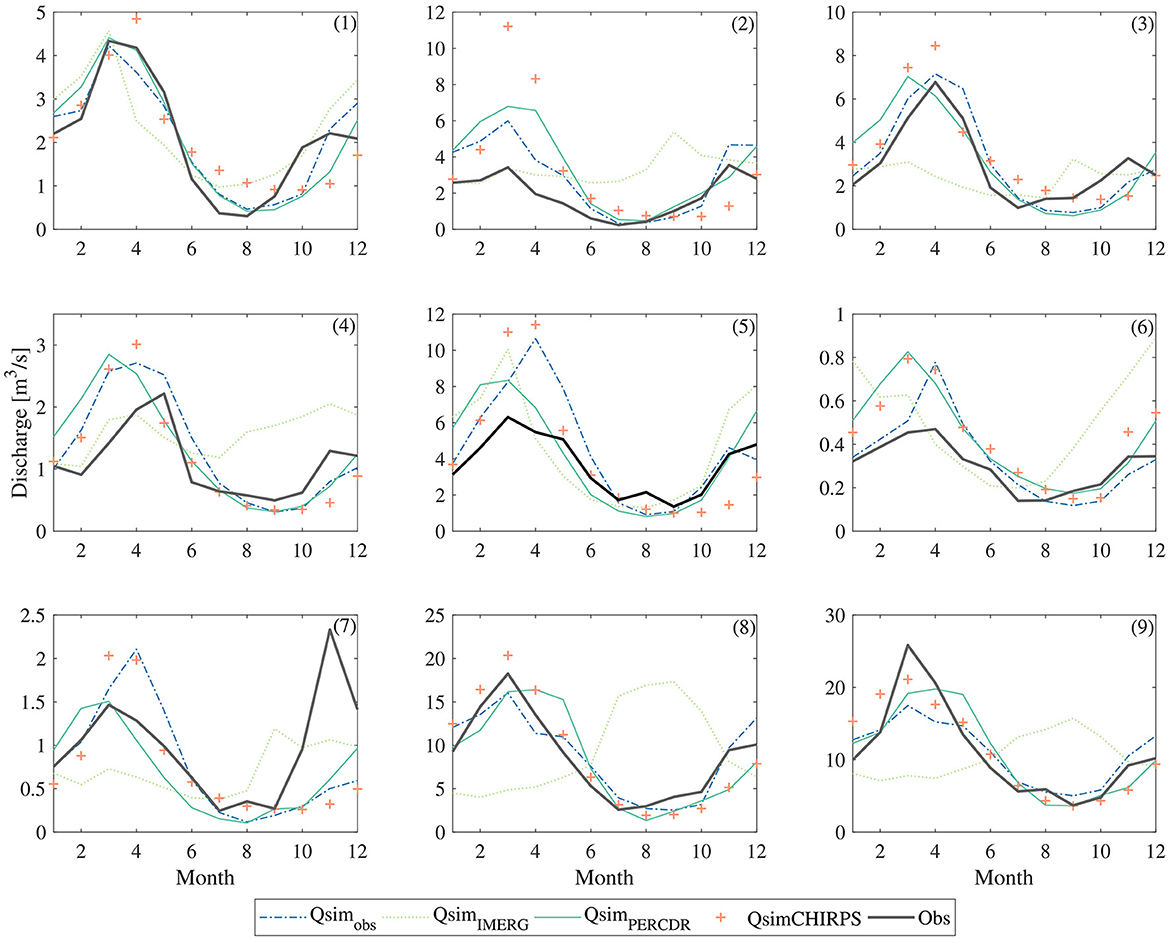
Figure 6. Average monthly discharge for the period 2000–2018 simulated by MISDc model using the satellite products and observed rain gauge to simulate the observed discharge. The numbers represent the basin's index.
The comparison between the precipitation products showed that the discharge simulated by PERCDR and the observed rainfall are almost the same for most of the basins with a mean KGE of 0.58 and 0.59 respectively. This shows that a single rain gauge is still sufficient to reproduce the continuous discharge at small basins and provide comparable results than with satellite rainfall. This result changes when we have a large basin, which is the case for basins 8 and 9 whose discharge simulated by PERCDR is better than that simulated by the observed precipitation. CHIRPS gave accepting results with an underestimation during summer and autumn. Concerning the IMERG product, the discharge is overestimated during the summer and autumn seasons with a peak during September for the small basins (from 1 to 6) and during the two seasons for the larger basins (8 and 9). According to Rachdane et al. (2022) and Salih et al. (2022), the IMERG product overestimates rainfall in high mountain areas during autumn.
Additionally, to investigate the ability of satellite precipitation products to simulate extreme flooding, Figure 7 shows the ability of different satellite precipitation products and hydrological models to simulate the November 2014 event that occurred over all nine basins using the best set of parameters. The results showed that the PERCDR/MISDc combination gave the highest KGE values (> 0.4) compared to the satellite and observed products. In order to deepen this analysis, we compared for each basin, which satellite product gave the best simulation in validation over the whole period using only the MISDc simulations. This analysis showed that PERCDR product gave the highest KGE in 5 basins against 4 for the observed one (Figure 8). In order to analyse the shape of the simulated flood hydrograph to the observed one, we plotted the November 2014 flood simulated by PERCDR against the observed and all 100 simulations. The analysis was conducted between 20 and 30 November. However, to illustrate the before and after of the event, we have shown 20 days instead of 10 (Figure 8). This comparison showed that the 100 simulations reproduce well the shape of the flood hydrograph, with an underestimation of the first peak discharge when using observed precipitation which can be related to the poor coverage of precipitation over the basin which is not the case when using PERCDR. This result confirmed the capability of PERCDR in flood hydrograph simulation.
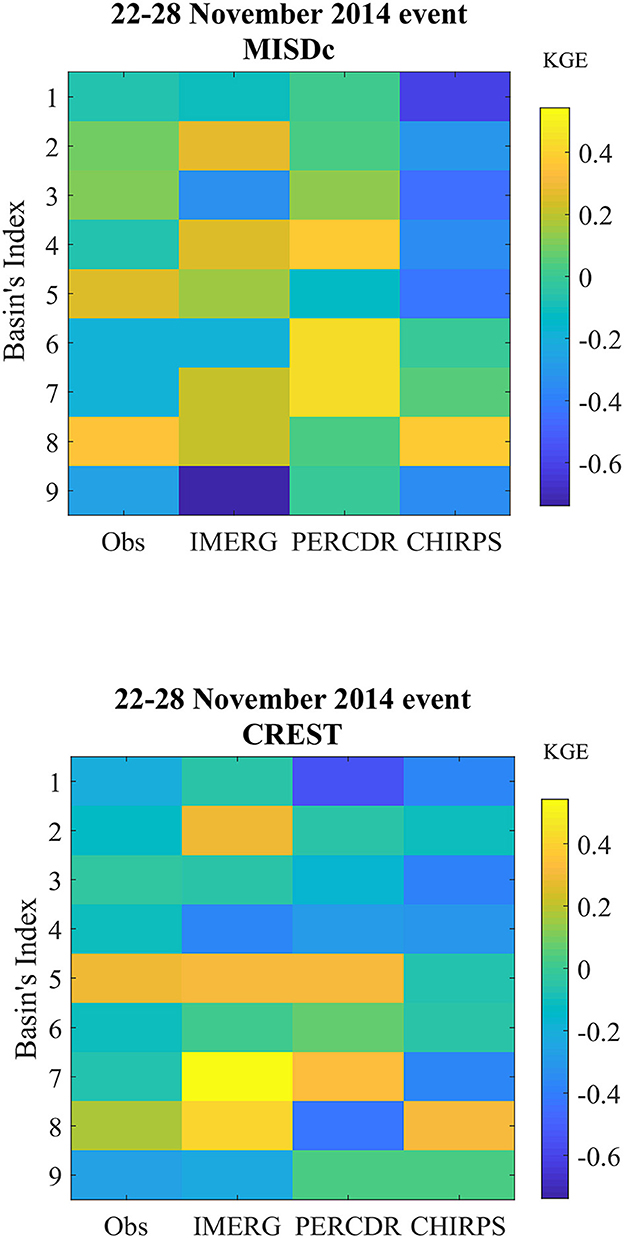
Figure 7. KGE plot of the flood event simulated in validation by MISDc driven by observed precipitation, IMERG, PERCDR and CHIRPS.
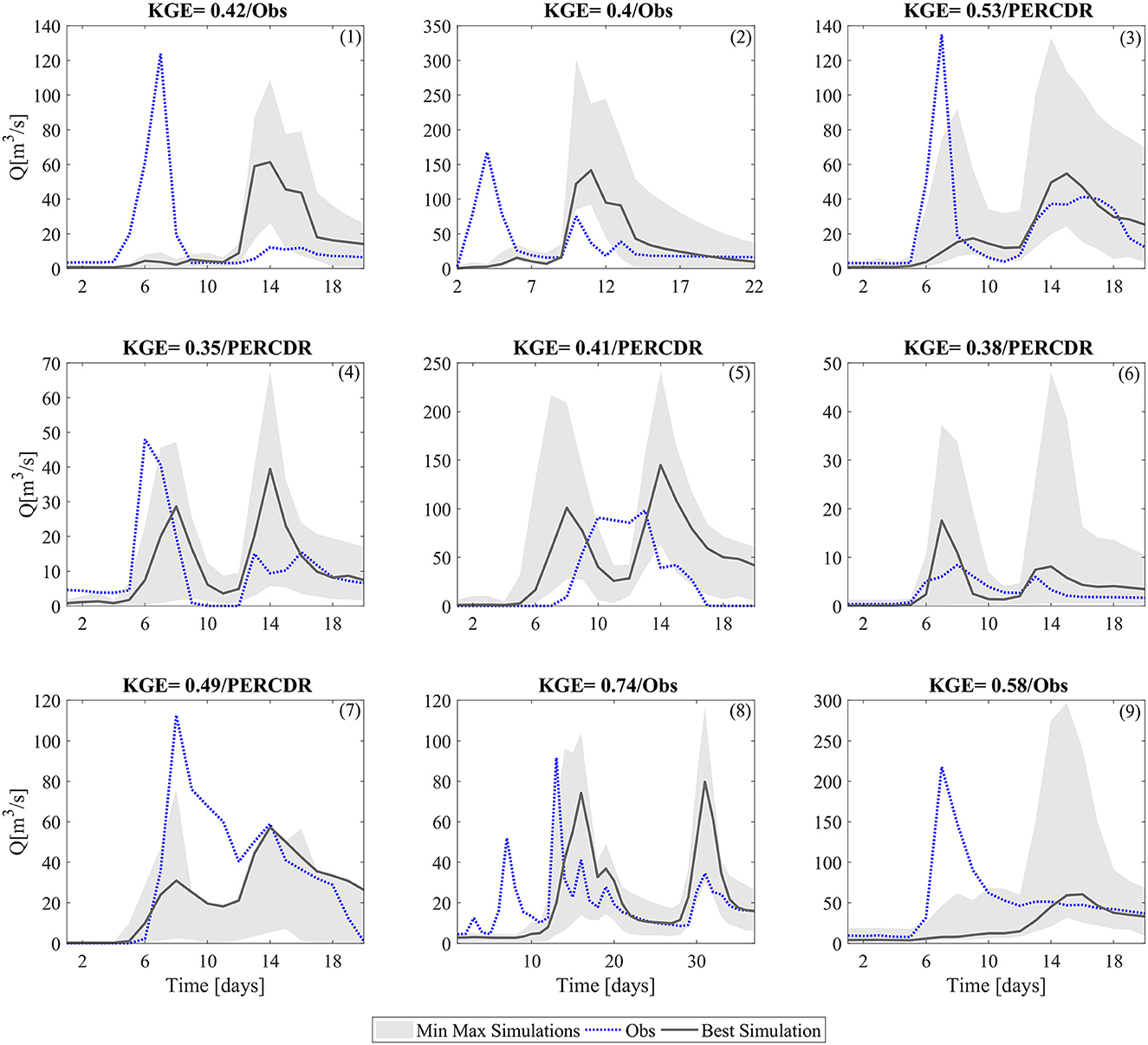
Figure 8. Best precipitation product of each basin for the validation results of November 2014 flood event simulated by MISDc model. The min max simulations represent the 100 set of parameters, the Obs: the observed discharge and the Best simulation: the best set of parameters. The KGE is calculated for the whole validation period. One to nine values are the basin's index.
6 Conclusion
In this study, three satellite precipitation products for reproducing precipitation over Morocco are used in surface water simulation as well as extreme flood event reproduction over 9 basins with different physiographic conditions. The simulated discharge is obtained through MISDc and CREST hydrological models and compared with the one obtained with observed precipitation as input of the two models. An evaluation of the three satellite precipitation products was made, and we found that PERCDR and CHIRPS gave similar results in terms of correlation coefficients (median of 0.27) with a slight improvement toward the northern basins (0.4), these findings align with the studies of Rachdane et al. (2022) and Ouatiki et al. (2023), which indicate that the efficiency of SPPs improves with latitude, although efficiency may be lower in mountainous regions. In addition to the statistical evaluation, we introduced a random bootstrap method for cross-validation scheme to choose the best set of the model's parameters generated from the calibration process over 100 sub-period of 10 years to evaluate the uncertainties of the model parameters. This method allowed us to generate an ensemble of runoff simulations to extract the minimum and maximum bounds. The performance of satellite precipitation products in simulating daily discharge over the 9 basins was similar to that of observed precipitations, in terms of KGE, for the two models with less uncertainties found using MISDc model over the two phases: calibration and validation. However, the performance of PERCDR is quite identical to the performance of observed precipitations in water resource simulation, with an advantage to the latter over the small basin areas. For the larger basins (with two rain gauges) PERCDR is better in simulating discharge than observed precipitation. This showed that a single rain gauge is still sufficient to simulate discharge at small basins but not the opposite. Yet in most basins, a single rain gauge is not enough to have an adequate representation of rainfall spatial variability, even if the results here are satisfactory. Another analysis was performed on the reproduction of the complete flood hydrograph by comparing the observed hydrograph of the November 2014 event to the simulated one by the best SPPs and hydrological models. The highest KGE values (≥0.5) are obtained using PERCDR in combination with MISDc which is consistent with Camici et al. (2018), where the product was tested over a basin in northern Morocco using MISDc and the performance was satisfactory compared to the other basins tested in the same study.
PERCDR product can be a useful alternative to ground observations to simulate discharge for water resources management and hydrological applications at daily time step. Similar results are found in Tibetan plateau (Liu et al., 2017), Senegal (Bâ et al., 2018), Northeastern China (Zhang et al., 2020), Oklahoma (Ashouri et al., 2016). In addition, IMERG was not found reliable in hydrological applications which is in parallel with Tramblay et al. (2023) where IMERG product was not good in reproducing discharge. This highlights the necessity to test which SPP is more adapted to local climatic conditions.
Further work should aim in determining the relation between the SPPs, basin characteristics and the hydrological modeling approach to identify the best combination based on the application purpose for ungauged basins where only river discharge is available. Another aspect that we need to consider is integrating SPPs with other data (e.g., soil moisture, vegetation water content) to improve ground-level rainfall estimation especially in developing countries, where rain gauge data are sparse.
Data availability statement
The original contributions presented in the study are included in the article/Supplementary material, further inquiries can be directed to the corresponding author.
Author contributions
EMEK: Conceptualization, Data curation, Formal analysis, Methodology, Writing—original draft, Writing—review and editing. YT: Conceptualization, Methodology, Writing—review and editing. MES: Methodology, Writing—review and editing. AM: Methodology, Writing—review and editing. AC: Methodology, Writing—review and editing. All authors contributed to the article and approved the submitted version.
Acknowledgments
The authors would like to thank the Tensift and Oum Er Rbia basin agencies of Morocco for granting access to the observed discharge and rainfall data. The authors would like to thank Humberto Vergara-Arrieta for providing CREST model code and Luca Brocca for the MISDc model. The authors thank the African Geospatial Data Portal Frameworks for Science, Capacity-Building and Decision-Making Purposes project for its support within the Center for Remote Sensing Applications (CRSA-UM6P), Morocco (Accord spécifique n° 89 entre OCP S.A et UM6P).
Conflict of interest
The authors declare that the research was conducted in the absence of any commercial or financial relationships that could be construed as a potential conflict of interest.
Publisher's note
All claims expressed in this article are solely those of the authors and do not necessarily represent those of their affiliated organizations, or those of the publisher, the editors and the reviewers. Any product that may be evaluated in this article, or claim that may be made by its manufacturer, is not guaranteed or endorsed by the publisher.
Supplementary material
The Supplementary Material for this article can be found online at: https://www.frontiersin.org/articles/10.3389/frwa.2023.1243251/full#supplementary-material
References
Ahmed, M., Aqnouy, M., and Stitou El Messari, J. (2021). Sustainability of Morocco's groundwater resources in response to natural and anthropogenic forces. J. Hydrol. 603, 126866. doi: 10.1016/j.jhydrol.2021.126866
Allen, R., Pereira, G. L.S., Raes, D., and Smith, M. (1998). Crop evapotranspiration guide lines for computing crop water requirements. Irrigat. Drain 56, 300.
Amjad, M., Yilmaz, M. T., Yucel, I., and Yilmaz, K. K. (2020). Performance evaluation of satellite- and model-based precipitation products over varying climate and complex topography. J. Hydrol. 584, 124707. doi: 10.1016/j.jhydrol.2020.124707
Arsenault, R., Brissette, F., and Martel, J. L. (2018). The hazards of split-sample validation in hydrological model calibration. J. Hydrol. 566, 346–362. doi: 10.1016/j.jhydrol.2018.09.027
Ashouri, H., Hsu, K L., Sorooshian, S., Braithwaite, D. K., and Knapp, K. R., Cecil, L. D., et al. (2015). PERSIANN-CDR: daily precipitation climate data record from multisatellite observations for hydrological and climate studies. Bullet. Am. Meteorol. Soc. 96, 69–83. doi: 10.1175/BAMS-D-13-00068.1
Ashouri, H., Nguyen, P., Thorstensen, A., Hsu, K. L., Sorooshian, S., Braithwaite, D., et al. (2016). Assessing the efficacy of high-resolution satellite-based PERSIANN-CDR Precipitation product in simulating streamflow. J. Hydrometeorol. 17, 2061–2076. doi: 10.1175/JHM-D-15-0192.1
Bâ, K. M., Balcázar, L., Diaz, V., Ortiz, F., Gómez-Albores, M. A., and Díaz-Delgado, C. (2018). Hydrological evaluation of PERSIANN-CDR rainfall over upper senegal river and bani river basins. Remote Sensing 10, 1884. doi: 10.3390/rs10121884
Beck, H. E., Vergopolan, N., Pan, M., Levizzani, V., Dijk, A. I. J. M. V., Weedon, G. P., et al. (2017). Global-scale evaluation of 22 precipitation datasets using gauge observations and hydrological modeling. Hydrol. Earth Syst. Sci. 21, 6201–6217. doi: 10.5194/hess-21-6201-2017
Bissour, R. (2018). Ressources en eau de l'oued El Abid amont barrage Bin El Ouidane et agriculture irriguée : Caractérisation, Quantification et Valorisation. Cas du périmètre irrigué de Béni Moussa (Région Béni Mellal Khénifra). Beni-Mellal : Thèse de L'université Sultane Moulay Slimane, Maroc, 328.
Bouizrou, I., Bouadila, A., Aqnouy, M., and Gourfi, A. (2023). Assessment of remotely sensed precipitation products for climatic and hydrological studies in arid to semi-arid data-scarce region, central-western Morocco. Remote Sens. Appl. Soc. Environ. 30, 100976. doi: 10.1016/j.rsase.2023.100976
Brocca, L., Camici, S., Massari, C., Ciabatta, L., Filippucci, P., Villarini, G., et al. (2020). Satellite Soil Moisture Improves Rainfall Just Where Needed. EGU General Assembly 2020, EGU2020-5303. doi: 10.5194/egusphere-egu2020-5303
Brocca, L., Melone, F., and Moramarco, T. (2008). On the estimation of antecedent wetness conditions in rainfall-runoff modelling. Hydrol. Proc. 12, 6629 doi: 10.1002/hyp.6629
Brocca, L., Melone, F., and Moramarco, T. (2011). Distributed rainfall-runoff modelling for flood frequency estimation and flood forecasting. Hydrol. Proc. 18, 8042. doi: 10.1002/hyp.8042
Camici, S., Ciabatta, L., Massari, C., and Brocca, L. (2018). How reliable are satellite precipitation estimates for driving hydrological models: A verification study over the Mediterranean area. J. Hydrol. 563, 950–961. doi: 10.1016/j.jhydrol.2018.06.067
Camici, S., Massari, C., Ciabatta, L., Marchesini, I., and Brocca, L. (2020). Which rainfall score is more informative about the performance in river discharge simulation? A comprehensive assessment on 1318 basins over Europe. Hydrol. Earth Syst. Sci 24, 4869–4885. doi: 10.5194/hess-24-4869-2020
Casse, C., Gosset, M., Peugeot, C., Pedinotti, V., Boone, A., Tanimoun, B. A., et al. (2015). Potential of satellite rainfall products to predict Niger River flood events in Niamey. Atmosph. Res. Int. Precip. Working Group 163, 162–176. doi: 10.1016/j.atmosres.2015.01.010
Coron, L. (2013). Les Modèles Hydrologiques Conceptuels, sont-ils Robustes Face à un Climat en Évolution? Diagnostic sur un Échantillon de Bassins Versants Français Et Australiens. Thèse de doctorat. Paris: AgroParisTech, 346.
El Alaoui El Fels, A., Saidi, M. E., and Alam, M. J. B. (2022). Rainfall frequency analysis using assessed and corrected satellite precipitation products in moroccan arid areas. the case of tensift watershed. Earth Syst. Environ. 6, 391–404. doi: 10.1007/s41748-021-00290-x
El Khalki, E. M., Tramblay, Y., Hanich, L., Marchane, A., Boudhar, A., Hakkani, B., et al. (2021). Climate change impacts on surface water resources in the Oued El Abid basin, Morocco. Hydrol. Sci. J. 66, 2132–2145. doi: 10.1080/02626667.2021.1982137
El Khalki, E. M., Tramblay, Y., Massari, C., Brocca, L., Simonneaux, V., Gascoin, S., et al. (2020). Challenges in flood modeling over data-scarce regions: how to exploit globally available soil moisture products to estimate antecedent soil wetness conditions in Morocco. Nat. Hazards Earth Syst. Sci. 20, 2591–2607. doi: 10.5194/nhess-20-2591-2020
El Khalki, E. M., Tramblay, Y., Saidi, M. E. M., Bouvier, C., Hanich, L., Benrhanem, M., et al. (2018). Comparison of modeling approaches for flood forecasting in the High Atlas Mountains of Morocco. Arab. J. Geosci. 11, 410. doi: 10.1007/s12517-018-3752-7
Elgamal, A., Reggiani, P., and Jonoski, A. (2017). Impact analysis of satellite rainfall products on flow simulations in the Magdalena River Basin, Colombia. J. Hydrol. Reg. Stu. 9, 85–103. doi: 10.1016/j.ejrh.2016.09.001
Er-Raki, S., Chehbouni, A., Khabba, S., Simonneaux, V., Jarlan, L., and Ouldbba, A., et al. (2010). Assessment of reference evapotranspiration methods in semi-arid regions: Can weather forecast data be used as alternate of ground meteorological parameters? J. Arid Environ. 74, 1587–1596. doi: 10.1016/J.JARIDENV.2010.07.002
Faccini, F., Luino, F., Sacchini, A., Turconi, L., and Graff, D. J.V. (2015). Geohydrological hazards and urban development in the Mediterranean area: an example from Genoa (Liguria, Italy). Nat. Hazards Earth Syst. Sci. 15, 2631–2652. doi: 10.5194/nhess-15-2631-2015
Funk, C., Peterson, P., Landsfeld, M., Pedreros, D., Verdin, J., Shukla, S., et al. (2015). The climate hazards infrared precipitation with stations—a new environmental record for monitoring extremes. Sci. Data 2, 150066. doi: 10.1038/sdata.2015.66
Gupta, H. V., Wagener, T., and Liu, Y. (2009). Reconciling theory with observations: elements of a diagnostic approach to model evaluation. Hydrol. Proc. 22, 3802–3813. doi: 10.1002/hyp.6989
Hall, D. K., Riggs, G. A., Salomonson, V. V., DiGirolamo, N. E., and Bayr, K. J. (2002). MODIS snow-cover products. Remote Sens. Environ. 83, 181–194. doi: 10.1016/S0034-4257(02)00095-0
Hargreaves, G. H., and Samani, Z. A. (1982). Estimating potential evapotranspiration. J. Irrig. Drainage Div. 108, 225–230. doi: 10.1061/JRCEA4.0001390
Heistermann, M., and Kneis, D. (2011). Benchmarking quantitative precipitation estimation by conceptual rainfall-runoff modeling. Water Res. Res. 47, 9153. doi: 10.1029/2010WR009153
Hou, A. Y., Kakar, R. K., Neeck, S., Azarbarzin, A. A., Kummerow, C. D., Kojima, M., et al. (2014). The global precipitation measurement mission. Bullet. Am. Meteorol. Soc. 95, 701–722. doi: 10.1175/BAMS-D-13-00164.1
Hsu, K., Gao, X., Sorooshian, S., and Gupta, H. V. (1997). Precipitation estimation from remotely sensed information using artificial neural networks. J. Appl. Meteor. 36, 1176–1190. doi: 10.1175/1520-0450(1997)036<1176:PEFRSI>2.0.CO;2
Huffman, G. J., Adler, R. F., Bolvin, D. T., and Nelkin, E. J. (2010). “The TRMM multi-satellite precipitation analysis (TMPA),” in Satellite Rainfall Applications for Surface Hydrology, eds M. Gebremichael and F. Hossain (Dordrecht: Springer). doi: 10.1007/978-90-481-2915-7_1
Karmouda, N., Kacimi, I., ElKharrim, M., Brirhet, H., and Hamidi, M. (2022). Geo-statistical and hydrological assessment of three satellite precipitation products over Ouergha basin (Northern Morocco). Arab J. Geosci. 15, 235. doi: 10.1007/s12517-021-09124-6
Kidd, C., Becker, A., Huffman, G. J., Muller, C. L., Joe, P., Skofronick-Jackson, G., et al. (2017). So, How much of the earth's surface is covered by rain gauges? Bullet. Am. Meteorol. Soc. 98, 69–78. doi: 10.1175/BAMS-D-14-00283.1
KlemeŠ, V. (1986). Operational testing of hydrological simulation models. Hydrol. Sci. J. 31, 13–24. doi: 10.1080/02626668609491024
Kling, H., Fuchs, M., and Paulin, M. (2012). Runoff conditions in the upper Danube basin under an ensemble of climate change scenarios. J. Hydrol. 424–425, 264–277. doi: 10.1016/j.jhydrol.2012.01.011
Knoben, W. J. M., Freer, J. E., and Woods, R. A. (2019). Technical note: inherent benchmark or not? Comparing Nash-Sutcliffe and Kling-Gupta efficiency scores. Hydrol. Earth Syst. Sci. 23, 4323–4331. doi: 10.5194/hess-23-4323-2019
Kummerow, C. (1998). Beamfilling errors in passive microwave rainfall retrievals. J. Appl. Meteorol. Climatol. 37, 356–370. doi: 10.1175/1520-0450(1998)037<0356:BEIPMR>2.0.CO;2
Liu, X., Yang, T., Hsu, K., Liu, C., and Sorooshian, S. (2017). Evaluating the streamflow simulation capability of PERSIANN-CDR daily rainfall products in two river basins on the Tibetan Plateau. Hydrol. Earth Syst. Sci. 21, 169–181. doi: 10.5194/hess-21-169-2017
Lu, D., and Yong, B. (2018). Evaluation and hydrological utility of the latest GPM IMERG V5 and GSMaP V7 precipitation products over the Tibetan Plateau. Remote Sens. 10, 2022. doi: 10.3390/rs10122022
Maggioni, V., and Massari, C. (2018). On the performance of satellite precipitation products in riverine flood modeling: a review. J. Hydrol. 558, 214–224. doi: 10.1016/j.jhydrol.2018.01.039
Marchane, A., Boudhar, A., Baba, M. W., Hanich, L., and Chehbouni, A. (2021). Snow lapse rate changes in the atlas mountain in Morocco based on MODIS time series during the period 2000–2016. Remote Sens. 13, 3370. doi: 10.3390/rs13173370
Marchane, A., Tramblay, Y., Hanich, L., Ruelland, D., and Jarlan, L. (2017). Climate change impacts on surface water resources in the Rheraya catchment (High Atlas, Morocco). Hydrol. Sci. J. 62, 979–995. doi: 10.1080/02626667.2017.1283042
Massari, C., Brocca, L., Barbetta, S., Papathanasiou, C., Mimikou, M., Moramarco, T., et al. (2014). Using globally available soil moisture indicators for flood modelling in Mediterranean catchments. Hydrol. Earth Syst. Sci. 18, 839–853. doi: 10.5194/hess-18-839-2014
Massari, C., Brocca, L., Pellarin, T., Abramowitz, G., Filippucci, P., Ciabatta, L., et al. (2020). A daily 25 km short-latency rainfall product for data-scarce regions based on the integration of the Global Precipitation Measurement mission rainfall and multiple-satellite soil moisture products. Hydrol. Earth Syst. Sci. 24, 2687–2710. doi: 10.5194/hess-24-2687-2020
Milewski, A., Elkadiri, R., and Durham, M. (2015). Assessment and comparison of TMPA satellite precipitation products in varying climatic and topographic regimes in Morocco. Remote Sens. 7, 5697–5717. doi: 10.3390/rs70505697
Ouaba, M., El Khalki, E. M., Saidi, M. E., and Alam, M. J. B. (2022). Estimation of flood discharge in ungauged basin using GPM-IMERG satellite-based precipitation dataset in a Moroccan arid zone. Earth Syst. Environ. 6, 541–556. doi: 10.1007/s41748-022-00296-z
Ouatiki, H., Boudhar, A., and Chehbouni, A. (2023). Accuracy assessment and bias correction of remote sensing–based rainfall products over semiarid watersheds. Theor. Appl. Climatol. 9, 1–8. doi: 10.1007/s00704-023-04586-y
Ouatiki, H., Boudhar, A., Tramblay, Y., Jarlan, L., Benabdelouhab, T., Hanich, L., et al. (2017). Evaluation of TRMM 3B42 V7 rainfall product over the oum er rbia watershed in Morocco. Climate 5, 1. doi: 10.3390/cli5010001
Quintero, F., Krajewski, W. F., Mantilla, R., Small, S., and Seo, B. C. (2016). A spatial–dynamical framework for evaluation of satellite rainfall products for flood prediction. J. Hydrometeorol. 17, 2137–2154. doi: 10.1175/JHM-D-15-0195.1
Rachdane, M., El Khalki, E. M., Saidi, M. E., Nehmadou, M., Ahbari, A., Tramblay, Y., et al. (2022). Comparison of high-resolution satellite precipitation products in sub-saharan Morocco. Water 14, 3336. doi: 10.3390/w14203336
Ricciardelli, E., Di Paola, D., Gentile, F., Cersosimo, S., Cimini, A., Gallucci, D., et al. (2018). Analysis of livorno heavy rainfall event: examples of satellite-based observation techniques in support of numerical weather prediction. Remote Sens. 10, 1549. doi: 10.3390/rs10101549
Saidi, M. E. M., Saouabe, T., Fels, A. E. A. E., Khalki, E. M. E., and Hadri, A. (2020). Hydro-meteorological characteristics and occurrence probability of extreme flood events in Moroccan High Atlas. J. Water Clim. Change 11, 310–321. doi: 10.2166/wcc.2020.069
Salih, W., Chehbouni, A., and Epule, T. E. (2022). Evaluation of the performance of multi-source satellite products in simulating observed precipitation over the tensift basin in Morocco. Remote Sens. 14, 1171. doi: 10.3390/rs14051171
Saouabe, T., Khalki, E. M. E., Saidi, M. E. M., Najmi, A., Hadri, A., Rachidi, S., et al. (2020). Evaluation of the GPM-IMERG precipitation product for flood modeling in a semi-arid mountainous basin in Morocco. Water 12, 2516. doi: 10.3390/w12092516
Satgé, F., Ruelland, D., Bonnet, M. P., Molina, J., and Pillco, R. (2019). Consistency of satellite-based precipitation products in space and over time compared with gauge observations and snow- hydrological modelling in the Lake Titicaca region. Hydrol. Earth Syst. Sci. 23, 595–619. doi: 10.5194/hess-23-595-2019
Seif-Ennasr, M., Zaaboul, R., Hirich, A., Caroletti, G. N., Bouchaou, L., Morjani, Z. E. A. E., et al. (2016). Climate change and adaptive water management measures in Chtouka Aït Baha region (Morocco). Sci. Total Environ. 573, 862–875. doi: 10.1016/j.scitotenv.2016.08.170
Sorooshian, S., Gao, X., Hsu, K., Maddox, R. A., Hong, Y., Gupta, H. V., et al. (2002). Diurnal variability of tropical rainfall retrieved from combined GOES and TRMM satellite information. J. Climate 15, 983–1001. doi: 10.1175/1520-0442(2002)015<0983:DVOTRR>2.0.CO;2
Thiemig, V., Rojas, R., Zambrano-Bigiarini, M., and De Roo, A. (2013). Hydrological evaluation of satellite-based rainfall estimates over the Volta and Baro-Akobo Basin. J. Hydrol. 499, 324–338. doi: 10.1016/j.jhydrol.2013.07.012
Tramblay, Y., El Khalki, E. M., Ciabatta, L., Camici, S., Hanich, L., Saidi, M. E., et al. (2023). River runoff estimation with satellite rainfall in Morocco. Hydrol. Sci. J. 68, 474–487. doi: 10.1080/02626667.2023.2171295
Tramblay, Y., Thiemig, V., Dezetter, A., and Hanich, L. (2016). Evaluation of satellite-based rainfall products for hydrological modelling in Morocco. Hydrol. Sci. J. 61, 2509–2519. doi: 10.1080/02626667.2016.1154149
Trigg, M. A., Birch, C. E., Neal, J. C., Bates, P. D., Smith, A., Sampson, C. C., et al. (2016). The credibility challenge for global fluvial flood risk analysis. Environ. Res. Lett. 11, 094014. doi: 10.1088/1748-9326/11/9/094014
Villarini, G., Krajewski, W. F., and Smith, J. A. (2009). New paradigm for statistical validation of satellite precipitation estimates: application to a large sample of the TMPA 0.25° 3-hourly estimates over Oklahoma. J. Geophys. Res. Atmosphere. 114, 1475. doi: 10.1029/2008JD011475
Wagner, P. D., Fiener, P., Wilken, F., Kumar, S., and Schneider, K. (2012). Comparison and evaluation of spatial interpolation schemes for daily rainfall in data scarce regions. J. Hydrol. 465, 388–400. doi: 10.1016/j.jhydrol.2012.07.026
Wang, J., Hong, Y., Li, L., Gourley, J. J., Khan, S. I., Yilmaz, K. K., et al. (2011). The coupled routing and excess storage (CREST) distributed hydrological model. Hydrol. Sci. J. 56, 84–98. doi: 10.1080/02626667.2010.543087
Keywords: floods, Morocco, MISDc, PERSIANN CDR, discharge
Citation: El Khalki EM, Tramblay Y, Saidi ME, Marchane A and Chehbouni A (2023) Hydrological assessment of different satellite precipitation products in semi-arid basins in Morocco. Front. Water 5:1243251. doi: 10.3389/frwa.2023.1243251
Received: 20 June 2023; Accepted: 29 November 2023;
Published: 20 December 2023.
Edited by:
Reza Kerachian, University of Tehran, IranReviewed by:
Luca Ciabatta, National Research Council (CNR), ItalyAnsoumana Bodian, Gaston Berger University, Senegal
Copyright © 2023 El Khalki, Tramblay, Saidi, Marchane and Chehbouni. This is an open-access article distributed under the terms of the Creative Commons Attribution License (CC BY). The use, distribution or reproduction in other forums is permitted, provided the original author(s) and the copyright owner(s) are credited and that the original publication in this journal is cited, in accordance with accepted academic practice. No use, distribution or reproduction is permitted which does not comply with these terms.
*Correspondence: El Mahdi El Khalki, ZWxtYWhkaS5lbGtoYWxraUB1bTZwLm1h