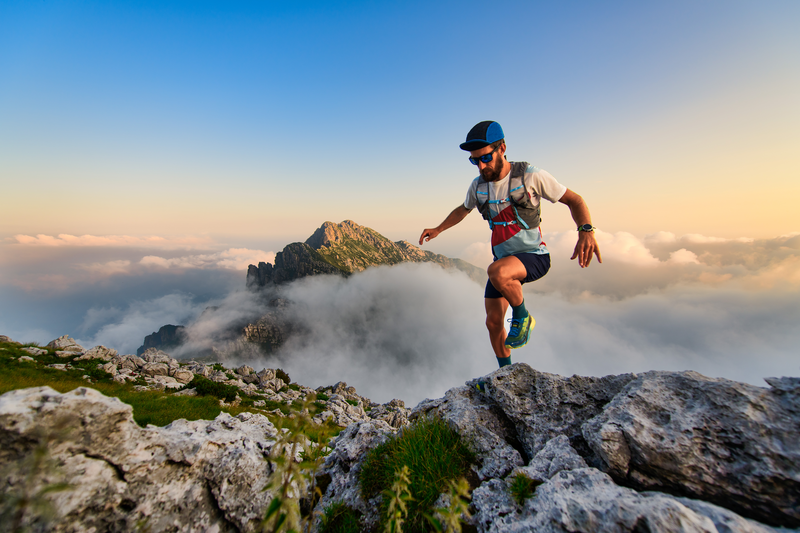
94% of researchers rate our articles as excellent or good
Learn more about the work of our research integrity team to safeguard the quality of each article we publish.
Find out more
ORIGINAL RESEARCH article
Front. Water , 11 April 2023
Sec. Water and Critical Zone
Volume 5 - 2023 | https://doi.org/10.3389/frwa.2023.1155623
This article is part of the Research Topic Investigating Connectivity to Advance the Predictive Understanding of Watershed Processes and the Earth’s Critical Zone View all 7 articles
Accumulations of wood in rivers can alter three-dimensional connectivity and facilitate channel bifurcations. Bifurcations divide the flow of water and sediment into secondary channels and are a key component of anastomosing rivers. While past studies illustrate the basic scenarios in which bifurcations can occur in anastomosing rivers, understanding of the mechanisms of bifurcations remains limited. We evaluate wood-induced bifurcations across thirteen anastomosing reaches in nine different streams and rivers in the U.S. Rocky Mountains to address conditions that favor different bifurcation types. We hypothesize that (1) wood-induced bifurcations exist as a continuum of different patterns in anastomosing rivers and (2) the position of a river segment along this continuum correlates with the ratio of erosive force to erosional resistance (F/R). We use field data to quantify F/R and compare varying F/R to bifurcation types across sites. Our results support these hypotheses and suggest that bifurcation types exist as a continuum based on F/R. At higher values of F/R, more channel avulsion is occurring and predominantly lateral bifurcations form. At lower values of F/R, banks are more resistant to erosive forces and wood-induced bifurcations are transitional or longitudinal with limited lateral extent. The relationship between F/R and bifurcation types is not linear, but it is progressive. Given the geomorphic and ecological functions associated with large wood and wood-induced channel bifurcations, it becomes important to understand the conditions under which wood accumulations can facilitate different types of bifurcations and the processes involved in these bifurcations. This understanding can inform river corridor restoration designed to enhance the formation of secondary channels, increase lateral and vertical connectivity, and promote an anastomosing planform.
River channels can split and merge at various spatiotemporal scales, creating and abandoning bifurcations as they migrate. Some of the earliest documentation of rivers depicts channels bifurcating (Carling et al., 2014, Figures 1, 2) and an extensive literature characterizes bifurcations (e.g., Bolla Pittaluga et al., 2003, 2015; Kleinhans et al., 2013). Bifurcations divide the flow of water and sediment into secondary channels and are a key component of delta distributary networks, braided planform, and anastomosing planform (Schumm, 1968; Knighton and Nanson, 1993; Burge, 2006). We consider anastomosing rivers to include at least two active parallel or sub parallel channels where flow rejoins downstream and vegetated islands or interfluves (≥ secondary channel width) are present between secondary channels. This description distinguishes anastomosing systems from distributary networks and braided planforms. Past work has described processes facilitating an anastomosing planform and planform distinctions (Schumm, 1968; Smith, 1973; Smith and Smith, 1980; Rust, 1981; Nanson et al., 1986; Schumann, 1989; Knighton and Nanson, 1993; Schumm et al., 1996; Makaske, 1998, 2001; Burge, 2006; Carling et al., 2014), as well as the characteristics of anastomosing rivers across diverse settings (e.g., Schumm, 1968; Baker, 1978; Smith and Smith, 1980; Nanson et al., 1986; Schumann, 1989; Harwood and Brown, 1993; Wohl et al., 2022). While these studies illustrate the basic scenarios in which bifurcation can occur, understanding of some of the mechanisms of bifurcations in anastomosing systems remains limited. We briefly review the conditions facilitating the formation of anastomosing channels and the role of logjams in creating channel bifurcations.
Figure 1. Conceptual illustration of jam-induced channel bifurcations. Lateral bifurcations (avulsive) form as a logjam forces overbank flow and bank erosion and the channel avulses laterally around the logjam. Longitudinal bifurcations (accretionary-bar splitting) form as a logjam creates lee deposition that is then stabilized by woody vegetation, forming a relatively short segment of split flow. Transitional bifurcations can have lateral and/or longitudinal bifurcations and depend on seasonal persistence of flow in secondary channels. An arrow showing increasing F/R with lateral bifurcation formation illustrates hypothesis 2.
Figure 2. A ternary diagram illustrating how we conceptualize the interactions among three primary controls influencing wood-induced channel bifurcations. The maximum value of each of these controls occurs at a triangle vertex and the minimum at the mid-point of the opposite side of the triangle. Letters indicate channel planforms (A anastomosing, B braided, M meandering, S straight) and the shading indicates the range of conditions in which anastomosing channel planform can occur. Multiple letters separated by commas indicate that more than one channel planform is possible in that portion of the diagram.
Anastomosing planforms have been attributed to diverse influences, including:
• Vegetation (e.g., Smith, 1976; Gradziński et al., 2003; Larsen, 2019)
• Sediment supply and bank cohesion (e.g., Rust, 1981; Smith, 1983; Gibling et al., 1998; Makaske, 1998)
• Tectonic uplift and basin subsidence (e.g., Rust et al., 1985; Smith, 1986)
• Increased flow magnitude (e.g., Smith and Smith, 1980; Knighton and Nanson, 1993)
• Channel obstructions such as large wood (e.g., Burge and Lapointe, 2005; Wohl, 2011; Collins et al., 2012), ice dams (e.g., King and Martini, 1984), and beaver dams (e.g., Woo and Waddington, 1990; Gurnell, 1998; Burchsted et al., 2010; Polvi and Wohl, 2012; Laurel and Wohl, 2019).
The combination underlying every scenario of anastomosing is (i) limited lateral migration due to either bank stability and/or limited stream power, which prevents the channel(s) from being sufficiently laterally mobile to create a braided planform and (ii) sufficient discharge and stream power to, at least episodically, overtop the banks and erode persistent secondary channels into the floodplain (Smith and Smith, 1980; Harwood and Brown, 1993; Makaske, 2001).
The distinction between anastomosing and other channel planforms is, to some extent, arbitrary because natural channel planforms occur along continua such as those between anastomosing and braided, anastomosing and meandering, or braided and meandering. Different positions along these channel-planform continua can represent differences in either the underlying processes driving bifurcation or the balance between hydraulic erosive force and erosional resistance. Carling et al. (2014), for example, described differences in bifurcations based on accretionary-bar flow splitting processes vs. avulsive processes. Accretionary alluvial islands can cause channel splitting as can avulsion across the floodplain (Carling et al., 2014).
A single piece of large wood or a logjam can facilitate differences in bifurcations based on accretionary-bar flow splitting or avulsive processes. A logjam can force overbank flow, bank erosion, and lateral bifurcation via avulsion (Wohl, 2011; Wohl and Cadol, 2011; Collins et al., 2012). A logjam can also create lee deposition that is then stabilized by woody vegetation, forming a relatively short segment of split flow and a longitudinal bifurcation via accretionary-bar flow splitting processes (Gurnell and Bertoldi, 2020) (Figure 1). Here, we build on this process-based understanding by examining the conditions under which logjams obstructing a channel can facilitate avulsive lateral channel bifurcations vs. accretionary-bar flow splitting longitudinal bifurcations.
Wood accumulations can alter the three-dimensional connectivity of a river corridor. Here, connectivity refers to the degree to which matter (water, solutes, sediment, organic matter) and organisms can move among components of a landscape or ecosystem (Wohl, 2017). Accumulations of large wood can obstruct flow and facilitate channel bifurcations. We propose that the occurrence and characteristics of wood-induced bifurcations are influenced by (i) the hydrologically connected width, (ii) wood blockage, and (iii) the ratio of erosive force to erosional resistance (F/R) (Figure 2).
Hydrologically connected width is governed by valley-floor topography and the magnitude of overbank flow, which together limit the maximum possible lateral extent of channel bifurcations and whether there is sufficient space to form a multichannel (braided or anastomosing) planform. As suggested in Figure 2, a narrow valley floor will support only a single channel.
Wood blockage refers to the ratio of logjam frontal area to channel cross-sectional area. Even a logjam that does not span the entire channel can create sufficient blockage and enough hydraulic roughness to enhance overbank flow and initiate splays or avulsion channels (Brummer et al., 2006; Collins et al., 2012), although channel-spanning jams are more likely to deflect flow overbank and create backwater effects (Jeffries et al., 2003; Livers and Wohl, 2021). Even though multichannel planforms can occur in the absence of wood obstructions, they are more likely to occur where these obstructions are present (Collins et al., 2012).
F/R values can control whether overbank flow creates a new channel, as well as the spatial and longitudinal extent and cross-sectional area of secondary channels. Erosive forces exerted against the channel banks and floodplain surface, including shear stress, thermal erosion, and abrasion by ice, typically increase with discharge. Erosional resistance or bank erodibility results from the frictional properties of sediments, the effective normal stress of the bank, and effective cohesion (i.e., cohesion added from vegetation roots; Simon et al., 2000). Banks erode as individual grains detach from the bank or a mass failure occurs. Erosional resistance is commonly influenced by grain size distribution, stratigraphy, moisture level, vegetation (Järvelä, 2004; Pollen-Bankhead and Simon, 2010), large wood (Wohl, 2013), and topographic heterogeneity (Güneralp and Rhoads, 2011). The ratio of erosive force to erosional resistance, as originally conceptualized by Schumm (1985) based on gradient, sediment load, and bank composition, correlates with channel planform along a spectrum from a single, straight channel (low F/R) to a braided channel (high F/R).
Both dispersed pieces of large wood and logjams can have varying influences on erosive force and erosional resistance depending on the location, movement, and characteristics of the wood. Dispersed pieces of large wood within a channel can increase hydraulic roughness and thus reduce velocity and erosive force exerted against the banks (Manga and Kirchner, 2000; Brooks et al., 2003; Daniels and Rhoads, 2004). A jam can have the same effect and create a backwater that reduces bank erosion upstream (Triska, 1984; Le Lay et al., 2013). A jam or large wood piece can also deflect flow toward the bank, which promotes bar growth and lateral channel movement, and over the bank across the floodplain in a manner that promotes formation of secondary channels that branch from and then rejoin the main channel downstream (O'Connor et al., 2003; Collins et al., 2012; Martín-Vide et al., 2014). The movement of wood can increase erosional force as a jam moves in congested transport (Piegay, 1993) or the lack of movement can increase erosional resistance as jams remain stable for long periods of time, forming buried hard points (Collins et al., 2012).
Our primary objective is to compare types of wood-induced channel bifurcations on multiple rivers and streams of differing size to address the conditions that favor one scenario over the other. We focus on systems where valley confinement, and thus hydrologically connected width, is not limited and where wood obstructions are abundant. We hypothesize that (1) wood-induced bifurcations exist as a continuum of different patterns in anastomosing rivers, as illustrated in Figures 1, 2, and the position of a river segment along this continuum correlates with F/R.
We focus our work across nine rivers and streams of diverse size within the montane and mesic montane zone of the Rocky Mountains in Colorado and Montana, USA. We have thirteen study reaches across the nine study sites. Each river or stream reach has sufficient lateral space to create multiple channels (at least two) within the study areas.
Study sites in Montana include Nyack Creek, the Swan River and two tributaries, Lost Creek and Goat Creek (Figure 3). We distinguish between upper and lower sites along the Swan River, Lost Creek, and Goat Creek. The Swan River (1,676 km2 drainage area) runs north along a valley bounded by the Mission Range to the west and the Swan Range to the east before draining into the Flathead River. Both Goat Creek (~94 km2 drainage area) and Lost Creek (~85 km2 drainage area) are within the Swan River Basin. Nyack Creek (~200 km2 drainage area) flows west into the Middle Fork Flathead River before draining into the Flathead River.
Figure 3. Montana field sites (Goat Creek, Lost Creek, Nyack Creek, Swan River). Note the different scale bars for the Nyack Creek and the Swan River watershed maps. Photos depict representative reach characteristics. We include two sites at Lost Creek, Goat Creek, and the Swan River in our results, but only show a photo of the upper reach for each site here.
The Swan basin receives ~750 mm of mean annual precipitation and the Nyack receives ~1500 mm of mean annual precipitation. Precipitation varies significantly with topography and rain shadow effect from the Rocky Mountains. Rainfall, snowmelt, and rain-on-snow precipitation can all produce peak flows, but the largest annual peak flow is typically associated with spring snowmelt (MacDonald and Hoffman, 1995). The hydrology of the region is dominated by the accumulation and melting of seasonal snowpack, with high flow occurring during the spring and low flow occurring during the late summer, autumn, and winter months.
The region is underlain by the Proterozoic-age Belt Supergroup, which mostly consists of weakly metamorphosed, fine-grained sedimentary rocks. The area is within the Intermountain Seismic Belt, a seismically active zone characterized by range-bounding normal faults (Hofmann and Hendrix, 2010). In addition to continuing uplift, topography in the region was shaped by Pleistocene glaciations. Lacustrine glacial lake deposits along with volcanic ash layers overlie rocky glacial till (Locke, 1995; Hofmann and Hendrix, 2010). Soils are thin, have poorly developed profiles (Antos and James, 1981), and are mostly gravelly loamy sand (USDA, 2022). Channels primarily have cobble- to boulder-bed substrate with pool-riffle sequences. Valley width along the Swan is ~1–2 km with an average channel gradient of 0.5%. Valley width along Nyack Creek averages 400–500 m with an average channel gradient of 0.2%.
Valley floors in the region are primarily covered with mesic montane conifer forests and wetlands, with some areas of subalpine forest. Shade-intolerant species include western larch (Larix occidentalis), western white pine (Pinus monticola), and Douglas-fir (Pseudotsuga menziesii). Climax, shade-tolerant species include grand fir (Abies grandis) and western redcedar (Thuja plicata) (Antos, 1977). Despite a history of patch timber harvest and stand-replacing fires in the upland portions of the valley (Antos and James, 1981; Parks et al., 2015), substantial portions of old-growth forest remain (Lesica, 1996) and the floodplain has experienced little development. The Swan River and Nyack Creek corridors have a high volume of downed wood within the channel and floodplain (Wohl et al., 2018).
Study sites in Colorado include a site along Little Beaver Creek (LBC), two sites along North Saint Vrain Creek (NSV), and one site along each of three NSV tributaries, Cony Creek, Ouzel Creek, and Hunters Creek (Figure 4). Cony Creek (~ 20 km2 drainage area), Ouzel Creek (~15 km2 drainage area), and Hunters Creek (~12 km2 drainage area) are all within the NSV drainage. NSV (drainage area 345 km2) and LBC (drainage area 40 km2) lie within the watershed of the South Platte River in the Colorado Front Range. The region receives ~550 mm of precipitation per year with variation based on elevation and has a mean annual temperature of 8.3°C (Barry, 1973). These sites are snowmelt dominated with a sustained seasonal peak, but summer convective storms can produce brief floods of higher magnitude (Jarrett, 1990).
Figure 4. Colorado field sites (Cony Creek, Hunters Creek, Ouzel Creek, North Saint Vrain Creek, and Little Beaver Creek). Note different scale bars for Little Beaver Creek and the sites within the North Saint Vrain Creek watershed. Photos depict representative reach characteristics.
Front Range catchments are underlain by Precambrian Silver Plume granite (Braddock and Cole, 1990; Cole et al., 2010). Valley geometry is highly variable longitudinally (Wohl et al., 2017), largely as a result of variations in bedrock joint density (Ehlen and Wohl, 2002). Fracture patterns in the granite create downstream alternations between relatively steep, narrowly confined valley segments and lower-gradient, less confined segments at lengths of 101-102 m. Channel planforms typically alternates between step-pool channels with boulder substrate in the most confined sections to anastomosing channels with pool-riffle bedforms or wood-forced steps and pools, and a cobble substrate, in the wider valley segments. Average channel gradient varies from 6 (upper portion NSV site) to 2% (Cony Creek) and channel substrate averages 45–60 mm diameter clasts, except in logjam backwaters where sand and fine gravel are present. Lower gradient and less confined reaches have anastomosing planforms with abundant channel-spanning logjams (Wohl, 2011) or beaver dams (John and Klein, 2004; Polvi and Wohl, 2012).
The region has old-growth montane forest. Dominant species include ponderosa pine (Pinus ponderosa), Engelmann spruce (Picea engelmannii), Douglas-fir (Pseudotsuga menziesii), aspen (Populus tremuloides), and willows (Salix spp.). Large wood is recruited to channels primarily from bank erosion and individual tree fall and channel-spanning logjams are abundant in the channels (Jackson and Wohl, 2015). Both watersheds have experienced recent disturbances by fire, flooding, and mass movements that significantly altered the watersheds and river corridors (Sibold et al., 2006; Rathburn et al., 2017; Sutfin and Wohl, 2019; Wohl et al., 2022). Most recently, the 2020 Cameron Peak fire burned substantial portions of the LBC watershed. New anastomosing reaches were created in both burned and unburned portions of the watershed during post-fire flash floods.
At each of our study sites, we characterized channel bifurcations and associated jams. We measured bank height, root depth/organic layer thickness, and coarse layer thickness, and collected one representative bank sample for organic layers and one for coarse layers to use in grain size analysis at locations where bifurcations occurred. Bank samples were sieved for grain size distribution. We measured the width of logjams relative to the width of the channel to calculate blockage ratios and used the most up-to-date Google Earth imagery at each site (spanning 2014–2022) to measure hydrologically connected width using the built-in measure tool (https://earth.google.com/web/).
We used our field data to quantify metrics of F and R. Our primary indicator of F is total stream power, which is the product of discharge, channel gradient, and the specific weight of water. Stream power is a useful predictor of channel form and dynamics because it quantifies the amount of geomorphic work that can be done by a stream, such as moving sediment on the bed or in the banks of the river (i.e., erosion or sediment transport). More specifically, stream power is commonly used as a tool to investigate the lateral stability of river channels (e.g., Chang, 1979; Nanson and Croke, 1992; Makaske, 1998). We used USGS StreamStats (https://streamstats.usgs.gov/ss/) to determine average snowmelt peak flow as a discharge value at which we expect wood can move. We used field measurements and LiDAR data to extract reach-scale channel gradient for each study site. We used the bank stability and toe erosion model (BSTEM; https://www.ars.usda.gov/southeast-area/oxford-ms/national-sedimentation-laboratory/watershed-physical-processes-research/research/bstem/) from the National Sedimentation Laboratory to provide comparative estimates of R (Simon et al., 2011). BSTEM integrates grain size, bank height and angle, sediment layer thicknesses, and vegetation data and is a commonly used tool for modeling streambank erosion and failure (Simon et al., 2000, 2011; Curran and Hession, 2013; Rinaldi and Nardi, 2013; Klavon et al., 2017). We use F/R to determine how much force relative to resistance is acting at each site and to compare the relative ratios across sites. We did not attempt to calculate a dimensionally correct ratio with respect to units. Rather, we use this ratio as an indicator of potential excess energy available for bank and floodplain erosion associated with channel bifurcations.
We manually measured total sinuosity and the ratio of channel migration width to average channel width as river corridor geometric variables using the built-in measure tool in Google Earth (https://earth.google.com/web/). Measurements were averaged across 500-m reaches at our study sites. Total sinuosity is the ratio of total channel length of all active channels/valley length (Hong and Davies, 1979; Egozi and Ashmore, 2008). Channel migration width is measured as the meander belt or total width between the outermost active channel edges in a multi-channel system. The ratio of this value to main channel bankfull width provides a dimensionless indicator of the lateral extent of active channel bifurcations. We used both total sinuosity and channel migration width/channel width as metrics for the degree to which a river has bifurcated. The larger the value of either metric, the more the river approaches the lateral bifurcation endmember in Figure 1; smaller values indicate the longitudinal bifurcation endmember.
Given our small dataset, we ran both parametric and non-parametric statistical analyses to assess the relationship between F/R and bifurcations. We used RStudio to perform the statistical analyses (R Core Team., 2022). We ran a simple linear regression and calculate the Pearson correlation coefficient (r) from the multiple R-squared. We calculated the Spearman rank correlation coefficient (ρ) as a non-parametric measure of the relationship between F/R and bifurcations. Given the repetition in values for some F/R ratios, we also calculated the Kendall rank correlation coefficient (τ) to ensure there is no impact from ties in our analyses (Hollander et al., 2014). All correlation coefficients were calculated using the cor.test() function in base R. We conducted the same analyses looking at the correlation between F/R and total sinuosity and F/R and channel migration width/channel width. The Pearson, Spearman, and Kendall correlation coefficients all measure the strength of the relationship between two variables (F/R and total sinuosity and F/R and channel migration width/channel width) for parametric (Pearson) or non-parametric (Spearman and Kendall) datasets. We used an alpha (probability of rejecting the null hypothesis when the null hypothesis is true) of 0.05 in all statistical analyses.
Results support both our hypotheses that (1) wood-induced bifurcations exist as a continuum of different patterns in anastomosing rivers and (2) the position of a river segment along this continuum correlates with F/R. Our full dataset is included as Supplementary Table 1. We plot F/R with both total sinuosity and channel migration width/channel width as proxies for the types of bifurcations. Our results for both suggest that bifurcation types exist as a continuum based on the ratio of F/R. We report only the Pearson Correlation Coefficients given the agreement between parametric and non-parametric correlation coefficients, but Spearman and Kendall correlation coefficients are included as Supplementary Table 2. Pearson correlation coefficients suggest a positive relationship between F/R and bifurcations (r = 0.837 for total sinuosity and r = 0.829 for channel migration width/channel width). At higher values of F/R, more channel avulsion is occurring and lateral bifurcations form (Figures 5, 6). At lower values of F/R, jam bifurcations are transitional or longitudinal but are not lateral (Figures 5, 6). The relationship between F/R and bifurcation type is not linear, but it is progressive.
Figure 5. Observed continuum of channel bifurcations based on total sinuosity and F/R with an exponential line of best fit.
Figure 6. Observed continuum of channel bifurcations based on channel migration width/channel width and F/R with an exponential line of best fit.
Our results suggest that bifurcation types exist as a continuum based on F/R. We observe a relationship between bifurcation type and F/R, where at higher values of F/R, more channel avulsion is occurring and lateral bifurcations with an increasing number of secondary channels and relative lateral extent form. We see the highest F/R and number of lateral bifurcations at the Swan River sites. Not surprisingly, this site has the highest stream power, and we assume highest transport capacity for wood. Visual observations in the field suggest that wood is regularly reworked within the Swan. We observed newly forming anastomosing reaches along the Swan where wood accumulations appear to have been transported and deposited during high flows, causing new bifurcations to form as flow avulsed laterally around logjams. The Swan also has a smaller root depth relative to the coarse layer of sediment, which contributes to more erodible banks relative to other sites. We see the lowest F/R and number of bifurcations along the upper portions of Goat Creek and Lost Creek as well as Hunters Creek. At each of these sites, conifers (>2 m in height) had established on the longitudinal bifurcations and buried pieces of wood were observed along the length of the bifurcation, suggesting the persistence and presence of older logjams. The transition from longitudinal to lateral bifurcations is progressive as F/R increases. This likely reflects a combination of increasing stream power and decreased root depth relative to the coarse layer in the banks, causing less erosional resistance. In other words, a combination of the flow having more erosive power and the banks being less stable as the root depth relative to the total bank height changes drives a transition from longitudinal to lateral bifurcations.
Understanding the conditions that facilitate different types of wood-induced bifurcations has important implications for the broader physical and biological processes in a river corridor. Wood-induced channel bifurcations support beneficial hydrologic, geomorphic, and ecologic function. In-channel logjams split flow and trap sediment, creating multiple channels and islands that dissipate energy during high flows (Brummer et al., 2006; Wohl, 2011; Collins et al., 2012). The ratio of wood blockage in a channel and F/R can influence the degree to which longitudinal and vertical connectivity is increased and lateral connectivity is decreased. Wood-induced bifurcations promote connectivity in the lateral (Baxter et al., 2005; Kondolf et al., 2006; Wohl, 2013) and vertical (Hester and Doyle, 2008; Sawyer et al., 2011; Marttila et al., 2018; Wilhelmsen et al., 2021) dimensions of a river corridor. Multiple, wood-rich channels that are laterally connected to the floodplain and vertically connected to the hyporheic zone support abundant and diverse habitat and species (Dolloff and Warren, 2003; Herdrich et al., 2018; Venarsky et al., 2018). The connectivity driven by wood-induced bifurcations provides refugia and resilience during disturbances such as flood, drought, and wildfire (Benda et al., 2004). For example, high water table, deep pools, and lateral connectivity provide drought refugia and more stable base flows (Boulton et al., 1998; Bêche et al., 2009; Dixon, 2016; Puttock et al., 2017). Increased lateral connectivity facilitated by logjams and secondary channels helps to attenuate flood peaks and diffuse flood flows across the floodplain (Junk et al., 1989; Poff et al., 1997), making habitats persistent and more resistant to natural and anthropogenic disturbances (Amoros and Bornette, 2002; Henning et al., 2006; Jeffres et al., 2008).
Hydrologic connectivity and interactions with wood are accompanied by hydraulic effects. Wood obstructions can divert and concentrate flow, creating local areas of high velocity and shear stress separated by wood-sheltered areas where velocities and shear stresses are drastically reduced (Gurnell, 2013; Matheson et al., 2017). Wood-induced bifurcations maximize hydraulic heterogeneity through partitioning of flow between branches that widen the range of in-channel depths and velocities (Gordon et al., 1992). Logjams reduce flow velocity and create pools (Beechie and Sibley, 1997; Montgomery and Buffington, 1997; Abbe and Montgomery, 2003) and bifurcations create multiple, marginal zones of slower flow. Both attributes promote bed heterogeneity and maximize morphological features in the channel and on the floodplain (Montgomery et al., 1996; Buffington and Montgomery, 1999), providing high capacity to store sediment and cycle nutrients (Parker et al., 2017). Frequent, small channel adjustments and a high, reliable water table also create optimal settings for germination and growth of aquatic and riparian vegetation (Nadler and Schumm, 1981; Tal and Paola, 2007; Braudrick et al., 2009). Wet woodlands on islands and floodplains supply and retain wood and widespread vegetation proximal to the channel (Fetherston et al., 1995; Gurnell et al., 2001; Montgomery and Abbe, 2006), supporting habitat and increased retention of organic matter and nutrients for other organisms (Bilby, 1981; Flores et al., 2011). Dense, diverse riparian vegetation provides abundant shade which, together with efficient hyporheic exchange, ameliorates water temperatures (Montgomery et al., 1999; Beechie et al., 2005).
The results summarized here suggest additional questions with respect to wood-induced channel bifurcations. These include potential thresholds of wood blockage, persistence of wood accumulations, and erosive mechanisms. First, is there a threshold size of wood accumulation or wood volume relative to channel dimensions that is necessary to drive bifurcations? Second, how long must wood accumulations persist relative to recurrence of peak flows to create persistent bifurcations? We were not able to directly observe interactions between potentially transient wood accumulations and channel bifurcations. We suspect, based on inference and multi-year field observations of some sites, that one large peak flow is sufficient to form a new bifurcation, even if the peak flow removes the wood accumulation that initiated the process of bifurcation. However, this inference has not been quantitatively tested. Third, what specific erosional mechanisms create channel bifurcations? Wood accumulations can cause preferential local bank erosion that could develop into a longitudinal bifurcation, for example. Our observations also indicate that diffuse overbank flow across the floodplain can concentrate into secondary channels over multiple seasons of peak flow. We have also observed overbank flow that returns to the channel downstream, creating a floodplain knickpoint at the point of re-entry to the channel during flows below bankfull stage. This knickpoint could presumably erode headward and help to stabilize a secondary channel.
Additional work is also needed across diverse settings to understand whether wood is a key driver of bifurcations in rivers with different hydrologic, wood, and sediment regimes. Our study focuses on snowmelt-dominated rivers with floodplains that are highly erodible (relatively uncohesive upper sediment layers and shallow-rooted conifers) except where willows grow in relatively dense stands. Additional work looking at the effects of flashier peak flow in rainfall-dominated rivers, highly cohesive silts and clays in floodplain alluvium, or different vegetation communities with greater hydraulic roughness and root resistance, would all provide valuable insight into understanding bifurcations across diverse settings.
Our results suggest that bifurcation types exist as a positive continuum based on the ratio of F/R. Longitudinal and lateral bifurcations occur at either end of the continuum, with transitional bifurcation types between. At higher values of F/R, more channel avulsion is occurring and lateral bifurcations form across a broader portion of the floodplain. At lower values of F/R, banks are more resistant to erosive forces and wood-induced bifurcations are transitional or longitudinal with a much lower lateral extent. More work considering thresholds of drivers forming new bifurcations across diverse settings will provide further insight into our understanding of the mechanisms behind bifurcations. As the need for resilient and more connected river systems increases in the face of accelerated environmental change, understanding the conditions under which wood accumulations can facilitate different types of bifurcations and the processes involved in these bifurcations is both significant and timely to river corridor science and management.
The original contributions presented in the study are included in the article/Supplementary material, further inquiries can be directed to the corresponding author.
AM and EW contributed to the conception and design of this study. AM collected field data in MT. EW collected field data in CO. Both authors contributed to the article and approved the submitted version.
Funding from the Tobacco Root Geological Society and an AGU Horton Research Grant, both awarded to AM, supported field data collection.
We thank Mckenna Pressley, Nathan Terault, and Hiromi Uno for assistance in the field, Bob Hall, Diane Whited, and the staff at Flathead Lake Biological Station for support at Montana field sites, and Juli Scamardo for feedback on conceptual figures. This manuscript benefited from comments from MK and DR as reviewers and MS as editor. We acknowledge that that the land this work was conducted on is the traditional and ancestral homelands of the Arapaho, Cheyenne, Ute, and Flathead Nations and recognize, with respect, the Indigenous peoples as original stewards of this land.
The authors declare that the research was conducted in the absence of any commercial or financial relationships that could be construed as a potential conflict of interest.
All claims expressed in this article are solely those of the authors and do not necessarily represent those of their affiliated organizations, or those of the publisher, the editors and the reviewers. Any product that may be evaluated in this article, or claim that may be made by its manufacturer, is not guaranteed or endorsed by the publisher.
The Supplementary Material for this article can be found online at: https://www.frontiersin.org/articles/10.3389/frwa.2023.1155623/full#supplementary-material
Abbe, T. B., and Montgomery, D. R. (2003). Patterns and processes of wood debris accumulation in the queets river basin, Washington. Geomorphology 51, 81–107. doi: 10.1016/S0169-555X(02)00326-4
Amoros, C., and Bornette, G. (2002). Connectivity and biocomplexity in waterbodies of riverine floodplains. Freshwater Biol. 47, 761–776. doi: 10.1046/j.1365-2427.2002.00905.x
Antos, J., and James, H. (1981). Successional Development in Abies Grandis (Dougl.) Forbes Forests in the Swan Valley, Western Montana. Northwest Science Missoula: University of Montana.
Antos, J. A. (1977). Grand Fir (Abies Grandis (Dougl.) Forbes) Forests of the Swan Valley, Montana. Missoula: University of Montana.
Baker, V. R. (1978). Large-Scale Erosional and Depositional Features of the Channeled Scabland. NASA Technical Reports. Washington, DC: The Channeled Scabland.
Barry, R. G. (1973). A climatological transect on the east slope of the front range, Colorado. Arctic Alpine Res. 5, 89–110. doi: 10.2307/1550251
Baxter, C. V., Fausch, K. D., and Saunders, W. C. (2005). Tangled webs: Reciprocal flows of invertebrate prey link streams and riparian zones. Freshwater Biol. 50, 201–220. doi: 10.1111/j.1365-2427.2004.01328.x
Bêche, L. A., Connors, P. G., Resh, V., and Merenlender, A. (2009). Resilience of fishes and invertebrates to prolonged drought in two california streams. Ecography 32, 778–788. doi: 10.1111/j.1600-0587.2009.05612.x
Beechie, T. J., Liermann, M., Beamer, E. M., and Henderson, R. (2005). A classification of habitat types in a large river and their use by juvenile salmonids. Trans. Am. Fisheries Soc. 134, 717–729. doi: 10.1577/T04-062.1
Beechie, T. J., and Sibley, T. H. (1997). Relationships between channel characteristics, woody debris, and fish habitat in northwestern washington streams. Trans. Am. Fisheries Soc. 126, 217–29. doi: 10.1577/1548-8659(1997)126<0217:RBCCWD>2.3.CO;2
Benda, L., Poff, L., Miller, D., Dunne, T., Reeves, G., Pess, G., et al. (2004). The network dynamics hypothesis: how channel networks structure riverine habitats. BioScience 54, 413–427. doi: 10.1641/0006-3568(2004)0540413:TNDHHC2.0.CO;2
Bilby, R. (1981). Role of organic debris dams in regulating the export of dissolved and particulate matter from a forested watershed. Ecology 62, 1234–1243. doi: 10.2307/1937288
Bolla Pittaluga, M., Coco, G., and Kleinhans, M. G. (2015). A unified framework for stability of channel bifurcations in gravel and sand fluvial systems. Geophys. Res. Lett. 42, 7521–7536. doi: 10.1002/2015GL065175
Bolla Pittaluga, M., Repetto, R., and Tubino, M. (2003). Channel bifurcation in braided rivers: equilibrium configurations and stability. Water Res. Res. 39:112. doi: 10.1029/2001WR001112
Boulton, A. J., Findlay, S., Marmonier, P., Stanley, E. H., and Valett, H. M. (1998). The functional significance of the hyporheic zone in streams and rivers. Ann. Rev. Ecol. Syst. 29, 59–81. doi: 10.1146/annurev.ecolsys.29.1.59
Braddock, W. A., and Cole, J. C. (1990). Geologic Map of Rocky Mountain National Park and Vicinity. Colorado. IMAP.
Braudrick, C. A., Dietrich, W. E., Leverich, G. T., and Sklar, L. S. (2009). Experimental evidence for the conditions necessary to sustain meandering in coarse-bedded rivers. Proc. Nat. Acad. Sci. 106, 16936–16941. doi: 10.1073/pnas.0909417106
Brooks, A. P., Brierley, G. J., and Millar, R. G. (2003). the long-term control of vegetation and woody debris on channel and flood-plain evolution: insights from a paired catchment study in southeastern Australia. Geomorphology 51, 7–29. doi: 10.1016/S0169-555X(02)00323-9
Brummer, C. J., Abbe, T. B., Sampson, J. R., and Montgomery, D. R. (2006). Influence of vertical channel change associated with wood accumulations on delineating channel migration zones, washington, USA. Geomorphology 80, 295–309. doi: 10.1016/j.geomorph.2006.03.002
Buffington, J. M., and Montgomery, D. R. (1999). Effects of hydraulic roughness on surface textures of gravel-bed rivers. Water Res. Res. 35, 3507–3521. doi: 10.1029/1999WR900138
Burchsted, D., Daniels, M., Thorson, R., and Vokoun, J. (2010). The river discontinuum: applying beaver modifications to baseline conditions for restoration of forested headwaters. BioScience 60, 908–922. doi: 10.1525/bio.2010.60.11.7
Burge, L. M. (2006). Stability, morphology and surface grain size patterns of channel bifurcation in gravel–cobble bedded anabranching rivers. Earth Surface Processes Landforms 31, 1211–1226. doi: 10.1002/esp.1325
Burge, L. M., and Lapointe, M. F. (2005). Understanding the temporal dynamics of the wandering renous river, new brunswick, Canada. Earth Surface Processes Landforms 30, 1227–1250. doi: 10.1002/esp.1196
Carling, P., Jansen, J., and Meshkova, L. (2014). Multichannel rivers: their definition and classification. Earth Surf. Processes Landforms 39, 26–37. doi: 10.1002/esp.3419
Chang, H. H. (1979). Minimum stream power and river channel patterns. J. Hydrol. 41, 303–327. doi: 10.1016/0022-1694(79)90068-4
Cole, J. C., Trexler, J. H., Cashman, P. H., Miller, I. M., Shroba, R. R., Cosca, M. A., et al. (2010). Beyond colorado's front range—a new look at laramide basin subsidence, sedimentation, and deformation in north-central Colorado. Field Guides 18, 55–76. doi: 10.1130/2010.0018(03)
Collins, B. D., Montgomery, D. R., Fetherston, K. L., and Abbe, T. B. (2012). The floodplain large-wood cycle hypothesis: a mechanism for the physical and biotic structuring of temperate forested alluvial valleys in the north pacific coastal ecoregion. Geomorphology. 139–140, 460–70. doi: 10.1016/j.geomorph.2011.11.011
Curran, J. C., and Hession, W. C. (2013). Vegetative impacts on hydraulics and sediment processes across the fluvial system. J. Hydrol. 505, 364–376. doi: 10.1016/j.jhydrol.2013.10.013
Daniels, M. D., and Rhoads, B. L. (2004). Effect of large woody debris configuration on three-dimensional flow structure in two low-energy meander bends at varying stages. Water Res. Res. 40:181. doi: 10.1029/2004WR003181
Dixon, S. J. (2016). A dimensionless statistical analysis of logjam form and process. Ecohydrology 9, 1117–1129. doi: 10.1002/eco.1710
Dolloff, C. A., and Warren, M. L. (2003). “Fish relationships with large wood in small streams,” in American Fisheries Society Symposium, Vol. 37 (Bethesda, MD), 179–193.
Egozi, R., and Ashmore, P. (2008). Defining and measuring braiding intensity. Earth Surface Processes Landforms 33, 2121–2138. doi: 10.1002/esp.1658
Ehlen, J., and Wohl, E. (2002). Joints and landform evolution in bedrock canyons. Trans. Jap. Geomorphological Union 23, 237–255.
Fetherston, K. L., Naiman, R. J., and Bilby, R. E. (1995). Large woody debris, physical process, and riparian forest development in montane river networks of the pacific northwest. Geomorphology 13, 133–144. doi: 10.1016/0169-555X(95)00033-2
Flores, L., Larrañaga, A., Díez, J., and Elosegi, A. (2011). Experimental wood addition in streams: effects on organic matter storage and breakdown. Freshwater Biol. 56, 2156–2167. doi: 10.1111/j.1365-2427.2011.02643.x
Gibling, M. R., Nanson, G. C., and Maroulis, J.C. (1998). Anastomosing river sedimentation in the channel country of central Australia. Sedimentology 45, 595–619. doi: 10.1046/j.1365-3091.1998.00163.x
Gordon, N. D., McMahon, T. A., and Finlayson, B. L. (1992). Stream Hydrology: An Introduction for Ecologists. Toronto: Wiley.
Gradziński, R., Baryła, J., Doktor, M., Gmur, D., Gradziński, M., Kedzior, A., et al. (2003). Vegetation-controlled modern anastomosing system of the upper narew river (NE Poland) and its sediments. Sedimentary Geol. 157, 253–276. doi: 10.1016/S0037-0738(02)00236-1
Güneralp, Í., and Rhoads, B. L. (2011). Influence of floodplain erosional heterogeneity on planform complexity of meandering rivers. Geophys. Res. Letters 38, 2–7. doi: 10.1029/2011GL048134
Gurnell, A. M. (1998). The hydrogeomorphological effects of beaver dam-building activity. Progress in physical geography. Earth Environ. 22, 167–189. doi: 10.1177/030913339802200202
Gurnell, A. M. (2013). Wood in fluvial systems. Treatise Geomorphol. 9, 163–188. doi: 10.1016/B978-0-12-374739-6.00236-0
Gurnell, A. M., and Bertoldi, W. (2020). Extending the conceptual model of river island development to incorporate different tree species and environmental conditions. River Res. Appl. 36, 1730–1747. doi: 10.1002/rra.3691
Gurnell, A. M., Petts, G. E., Hannah, D. M., Smith, B. P. G., Edwards, P. J., Kollmann, J., et al. (2001). Riparian vegetation and island formation along the gravel-bed fiume tagliamento, Italy. Earth Surf. Processes Landforms 26, 31–62. doi: 10.1002/1096-9837(200101)26:1<31::AID-ESP155>3.0.CO;2-Y
Harwood, K., and Brown, A. G. (1993). Fluvial processes in a forested anastomosing river: flood partitioning and changing flow patterns. Earth Surface Processes Landforms 18, 741–748. doi: 10.1002/esp.3290180808
Henning, J. A., and Gresswell, R. A., and Fleming, I. A. (2006). Juvenile salmonid use of freshwater emergent wetlands in the floodplain and its implications for conservation management. J. Fisheries Manage. 26, 367–376. doi: 10.1577/M05-057.1
Herdrich, A. T., Winkelman, D. L., Venarsky, M. P., Walters, D. M., and Wohl, E. (2018). The loss of large wood affects Rocky Mountain trout populations. Ecol. Freshw. Fish 27, 1023–1036. doi: 10.1111/eff.12412
Hester, E. T., and Doyle, M. W. (2008). In-stream geomorphic structures as drivers of hyporheic exchange. Water Res. Res. 44, 5810. doi: 10.1029/2006WR005810
Hofmann, M. H., and Hendrix, M. S. (2010). Depositional processes and the inferred history of ice-margin retreat associated with the deglaciation of the cordilleran ice sheet: the sedimentary record from Flathead Lake, Northwest Montana, USA. Sedimentary Geol. 223, 61–74. doi: 10.1016/j.sedgeo.2009.10.004
Hollander, M., Wolfe, D. A., and Chicken, E. (2014). Nonparametric Statistical Methods. New York, NY: Wiley.
Hong, L. B., and Davies, T. R. H. (1979). A study of stream braiding. GSA Bulletin 90, 1839–1859. doi: 10.1130/GSAB-P2-90-1839
Jackson, K. J., and Wohl, E. (2015). Instream wood loads in montane forest streams of the colorado front range, USA. Geomorphology 234, 161–170. doi: 10.1016/j.geomorph.2015.01.022
Jarrett, R. D. (1990). Paleohydrologic techniques used to define the spatial occurrence of floods. Geomorphology 3, 181–195. doi: 10.1016/0169-555X(90)90044-Q
Järvelä, J. (2004). Determination of flow resistance caused by non-submerged woody vegetation. Int. J. River Basin Manage. 2, 61–70. doi: 10.1080/15715124.2004.9635222
Jeffres, C. A., Opperman, J. J., and Moyle, P. B. (2008). Ephemeral floodplain habitats provide best growth conditions for juvenile chinook salmon in a california river. Environ. Biol. Fishes 83, 449–458. doi: 10.1007/s10641-008-9367-1
Jeffries, R., Darby, S. E., and Sear, D. A. (2003). The influence of vegetation and organic debris on flood-plain sediment dynamics: case study of a low-order stream in the new forest, England. Geomorphology 51, 61–80. doi: 10.1016/S0169-555X(02)00325-2
John, S., and Klein, A. (2004). Hydrogeomorphic effects of beaver dams on floodplain morphology: avulsion processes and sediment fluxes in upland valley floors. Quaternaire 15219–231. doi: 10.3406/quate.2004.1769
Junk, W., Bayley, P., and Sparks, R. (1989). The flood pulse concept in river-floodplain systems. Can. Pub. Fish. Aquatic Sci. 106, 110–127.
King, W. A., and Martini, I. P. (1984). Morphology and recent sediments of the lower anastomosing reaches of the attawapiskat River. Sedimentary Geology 37, 295–320. doi: 10.1016/0037-0738(84)90019-8
Klavon, K., Fox, G., Guertault, L., Langendoen, E., Enlow, H., Miller, R., et al. (2017). Evaluating a process-based model for use in streambank stabilization: insights on the bank stability and toe erosion model (BSTEM). Earth Surface Processes Landforms 42, 191–213. doi: 10.1002/esp.4073
Kleinhans, M. G., Ferguson, R. I., Lane, S. N., and Hardy, R. J. (2013). Splitting rivers at their seams: bifurcations and avulsion. Earth Surf. Processes Landforms 38, 47–61. doi: 10.1002/esp.3268
Knighton, D. A., and Nanson, G. C. (1993). Anastomosis and the continuum of channel pattern. Earth Surf. Processes Landforms 18, 613–625. doi: 10.1002/esp.3290180705
Kondolf, G. M., Boulton, A. J., O'Daniel, S., et al. (2006). Process-based ecological river restoration: visualizing three-dimensional connectivity and dynamic vectors to recover lost linkages. Ecol. Soc. 11, 5. doi: 10.5751/ES-01747-110205
Larsen, L. G. (2019). Multiscale flow-vegetation-sediment feedbacks in low-gradient landscapes. Geomorphology 334, 165–193. doi: 10.1016/j.geomorph.2019.03.009
Laurel, D., and Wohl, E. (2019). The persistence of beaver-induced geomorphic heterogeneity and organic carbon stock in river corridors. Earth Surf. Processes Landforms 44, 342–353. doi: 10.1002/esp.4486
Le Lay, Y., Piégay, H., and Rivière-Honegger, A. (2013). Perception of braided river landscapes: implications for public participation and sustainable management. J. Environ. Management 119, 1–12. doi: 10.1016/j.jenvman.2013.01.006
Lesica, P. (1996). Using fire history models to estimate proportions of old growth forest in northwest Montana, USA. Biol. Conserv. 77, 33–39. doi: 10.1016/0006-3207(95)00130-1
Livers, B., and Wohl, E. (2021). All logjams are not created equal. J. Geophys. Res. 126, e2021JF.006076. doi: 10.1029/2021JF006076
Locke, W. W. (1995). Modelling of icecap glaciation of the northern rocky mountains of Montana. Geomorphology 14, 123–130. doi: 10.1016/0169-555X(95)00053-5
MacDonald, L. H., and Hoffman, J. A. (1995). Causes of peak flows in northwestern Montana and northeastern Idaho. Water Resources Bulletin, 31, 79–95. doi: 10.1111/j.1752-1688.1995.tb03366.x
Makaske, B. (1998). Anastomosing Rivers: Forms, Processes and Sediments. Nederlandse Geografische Studies (Netherlands).
Makaske, B. (2001). Anastomosing rivers: a review of their classification, origin and sedimentary products. Earth Sci. Rev. 53, 149–196. doi: 10.1016/S0012-8252(00)00038-6
Manga, M., and Kirchner, J. W. (2000). Stress partitioning in streams by large woody debris. Water Res. Res. 36, 2373–2379. doi: 10.1029/2000WR900153
Martín-Vide, J. P., Amarilla, M., and Zárate, F. J. (2014). Collapse of the pilcomayo river. Geomorphology 205, 155–163. doi: 10.1016/j.geomorph.2012.12.007
Marttila, H., Turunen, J., Aroviita, J., Tammela, S., Luhta, P. L., Muotka, T., et al. (2018). Restoration increases transient storages in boreal headwater streams. River Res. Appl. 34, 1278–1285. doi: 10.1002/rra.3364
Matheson, A., Thoms, M., and Reid, M. (2017). Does reintroducing large wood influence the hydraulic landscape of a lowland river system? Geomorphology 292, 128–141. doi: 10.1016/j.geomorph.2017.03.035
Montgomery, D. R., and Abbe, T. B. (2006). Influence of logjam-formed hard points on the formation of valley-bottom landforms in an old-growth forest valley, queets River, Washington, USA. Quaternary Res. 65, 147–155. doi: 10.1016/j.yqres.2005.10.003
Montgomery, D. R., Abbe, T. B., Buffington, J. M., Peterson, N. P., Schmidt, K. M., Stock, J. D., et al. (1996). Distribution of bedrock and alluvial channels in forested mountain drainage basins. Nature 381, 587–589. doi: 10.1038/381587a0
Montgomery, D. R., Beamer, E. M., Pess, G. R., and Quinn, T. P. (1999). Channel type and salmonid spawning distribution and abundance. Can. J. Fish. Aquatic Sci. 56, 377–387. doi: 10.1139/f98-181
Montgomery, D. R., and Buffington, J. M. (1997). Channel-reach morphology in mountain drainage basins. GSA Bulletin 109, 596–611 doi: 10.1130/0016-7606(1997)109<0596:CRMIMD>2.3.CO;2
Nadler, C. T., and Schumm, S. A. (1981). Metamorphosis of south platte and arkansas rivers, eastern Colorado. Phys. Geography 2, 95–115. doi: 10.1080/02723646.1981.10642207
Nanson, G. C., and Croke, J. C. (1992). A genetic classification of floodplains. Geomorphology 4, 459–486. doi: 10.1016/0169-555X(92)90039-Q
Nanson, G. C., Rust, B. R., and Taylor, G. (1986). Coexistent Mud braids and anastomosing channels in an arid-zone river: cooper creek, central australia. Geology 14, 175–178. doi: 10.1130/0091-7613(1986)14<175:CMBAAC>2.0.CO;2
O'Connor, J. E., Jones, M. A., and Haluska, T. L. (2003). Flood plain and channel dynamics of the quinault and queets rivers, Washington, USA. Geomorphology 51, 31–59. doi: 10.1016/S0169-555X(02)00324-0
Parker, C., Henshaw, A. J., Harvey, G. L., and Sayer, C. D. (2017). Reintroduced large wood modifies fine sediment transport and storage in a lowland river channel. Earth Surf. Processes Landforms 42, 1693–1703. doi: 10.1002/esp.4123
Parks, S. A., Miller, C., Parisien, M., Holsinger, L. M., Dobrowski, S. Z., Abatzoglou, J., et al. (2015). Wildland fire deficit and surplus in the western United States, 1984–2012. Ecosphere 6, 1–13. doi: 10.1890/ES15-00294.1
Piegay, H. (1993). Nature, mass and preferential sites of coarse woody debris deposits in the lower ain valley (Mollon Reach), France. Regulated Rivers Res. Manage. 8, 359–372. doi: 10.1002/rrr.3450080406
Poff, L., Allan, J. D., Bain, M. B., Karr, J. R., Prestegaard, K. L., Richter, B. D., et al. (1997). The natural flow regime. BioScience 47, 769–784. doi: 10.2307/1313099
Pollen-Bankhead, N., and Simon, A. (2010). Hydrologic and hydraulic effects of riparian root networks on streambank stability: is mechanical root-reinforcement the whole story? Geomorphology 116, 353–362. doi: 10.1016/j.geomorph.2009.11.013
Polvi, L. E., and Wohl, E. (2012). The beaver meadow complex revisited – the role of beavers in post-glacial floodplain development. Earth Surf. Processes Landforms 37, 332–346. doi: 10.1002/esp.2261
Puttock, A., Graham, H. A., Cunliffe, A. M., Elliott, M., and Brazier, R. E. (2017). Eurasian beaver activity increases water storage, attenuates flow and mitigates diffuse pollution from intensively-managed grasslands. Sci. Total Environ. 576, 430–443. doi: 10.1016/j.scitotenv.2016.10.122
R Core Team. (2022). R: A Language and Environment for Statistical Computing. Vienna: R Foundation for Statistical Computing.
Rathburn, S. L., Bennett, G. L., Wohl, E. E., Briles, C., McElroy, B., Sutfin, N., et al. (2017). The fate of sediment, wood, and organic carbon eroded during an extreme flood, Colorado Front Range, USA. Geology 45, 499–502. doi: 10.1130/G38935.1
Rinaldi, M., and Nardi, L. (2013). Modeling interactions between riverbank hydrology and mass failures. J. Hydrol. Eng. 18, 1231–1240. doi: 10.1061/(ASCE)HE.1943-5584.0000716
Rust, B. R. (1981). Sedimentation in an arid-zone anastomosing fluvial system; cooper's creek, central Australia. J. Sedimentary Res. 51, 745–755. doi: 10.1306/212F7D97-2B24-11D7-8648000102C1865D
Rust, B. R., Gibling, M. R., and Legun, A. S. (1985). Coal Deposition in an Anastomosing-Fluvial System: The Pennsylvanian Cumberland Group South of Joggins, Nova Scotia, Canada. London: John Wiley and Sons, Ltd.
Sawyer, A. H., Bayani Cardenas, M., and Buttles, J. (2011). Hyporheic exchange due to channel-spanning logs. Water Res. Res. 47:484. doi: 10.1029/2011WR010484
Schumann, R. R. (1989). Morphology of red creek, wyoming, an arid-region anastomosing channel system. Earth Surf. Processes Landforms 14, 277–288. doi: 10.1002/esp.3290140404
Schumm, S. A. (1968). River Adjustment to Altered Hydrologic Regimen, Murrumbidgee River and Paleochannels. Australia. U.S. Government Printing Office.
Schumm, S. A. (1985). Patterns of alluvial rivers. Ann. Rev. Earth Planet. Sci. 13, 5–27. doi: 10.1146/annurev.ea.13.050185.000253
Schumm, S. A., Erskine, W. D., and Tilleard, J. W. (1996). Morphology, hydrology, and evolution of the anastomosing ovens and king rivers, Victoria, Australia. GSA Bulletin 108, 1212–1224. doi: 10.1130/0016-7606(1996)108<1212:MHAEOT>2.3.CO;2
Sibold, J. S., Veblen, T. T., and Gonzalez, M. E. (2006). Spatial and temporal variation in historic fire regimes in subalpine forests across the Colorado Front Range in Rocky Mountain National Park, Colorado, USA. J. Biogeography 32, 631–647. doi: 10.1111/j.1365-2699.2005.01404.x
Simon, A., Curini, A., Darby, S. E., and Langendoen, E. J. (2000). Bank and near-bank processes in an incised channel. Geomorphology 35, 193–217. doi: 10.1016/S0169-555X(00)00036-2
Simon, A., Pollen-Bankhead, N., and Thomas, R. E. (2011). Development and application of a deterministic bank stability and toe erosion model for stream restoration. Sci. Approach. Anal. Tools 194, 453–474. doi: 10.1029/2010GM001006
Smith, D. G. (1973). Aggradation of the alexandra-north saskatchewan river, banff park, Alberta. Fluvial Geomorphology. 47, 201–19.
Smith, D. G. (1976). Effect of vegetation on lateral migration of anastomosed channels of a glacier meltwater river. GSA Bulletin 87, 857–860. doi: 10.1130/0016-7606(1976)87<857:EOVOLM>2.0.CO;2
Smith, D. G. (1983). Anastomosed Fluvial Deposits: Modern Examples from Western Canada. Modern and Ancient Fluvial Systems. London: John Wiley and Sons, Ltd.
Smith, D. G. (1986). Anastomosing river deposits, sedimentation rates and basin subsidence, magdalena river, northwestern Colombia, South America. Sedimentary Geol. 46, 177–196. doi: 10.1016/0037-0738(86)90058-8
Smith, D. G., and Smith, N. D. (1980). Sedimentation in anastomosed river systems; examples from alluvial valleys near banff, alberta. J. Sedimentary Res. 50, 157–164. doi: 10.1306/212F7991-2B24-11D7-8648000102C1865D
Sutfin, N. A., and Wohl, E. (2019). Elevational differences in hydrogeomorphic disturbance regime influence sediment residence times within mountain river corridors. Nat. Commun. 10, 2221. doi: 10.1038/s41467-019-09864-w
Tal, M., and Paola, C. (2007). Dynamic single-thread channels maintained by the interaction of flow and vegetation. Geology 35, 347–350. doi: 10.1130/G23260A.1
Triska, F. J. (1984). Role of wood debris in modifying channel geomorphology and riparian areas of a large lowland river under pristine conditions: a historical case study. SIL Proc. 22, 1876–1892. doi: 10.1080/03680770.1983.11897589
USDA. (2022). Natural Resource Conservation Service Web Soil Survey. Available online at: https://www.nrcs.usda.gov/wps/portal/nrcs/main/soils/survey/ (accessed January, 2023).
Venarsky, M. P., Walters, D. M., Hall, R. O., Livers, B., and Wohl, E. (2018). Shifting stream planform state decreases stream productivity yet increases riparian animal production. Oecologia 187, 167–180. doi: 10.1007/s00442-018-4106-6
Wilhelmsen, K., Sawyer, A. H., Marshall, A., McFadden, S., Singha, K., Wohl, E., et al. (2021). Laboratory flume and numerical modeling experiments show log jams and branching channels increase hyporheic exchange. Water Res. Res. 57, 030299. doi: 10.1029/2021WR030299
Wohl, E. (2011). Threshold-induced complex behavior of wood in mountain streams. Geology 39, 587–590. doi: 10.1130/G32105.1
Wohl, E. (2013). Floodplains and wood. Earth Sci. Reviews 123, 194–212. doi: 10.1016/j.earscirev.2013.04.009
Wohl, E. (2017). Connectivity in rivers. Prog. Phys. Geography 41, 345–362. doi: 10.1177/0309133317714972
Wohl, E., and Cadol, D. (2011). Neighborhood matters: patterns and controls on wood distribution in old-growth forest streams of the colorado front range, USA. Geomorphology 125, 132–146. doi: 10.1016/j.geomorph.2010.09.008
Wohl, E., Lininger, K. B., and Scott, D. N. (2018). River beads as a conceptual framework for building carbon storage and resilience to extreme climate events into river management. Biogeochemistry 141, 365–383. doi: 10.1007/s10533-017-0397-7
Wohl, E., Marshall, A. E., Scamardo, J., White, D., and Morrison, R. R. (2022). Biogeomorphic influences on river corridor resilience to wildfire disturbances in a mountain stream of the southern rockies, USA. Sci. Environ. 820, 153321. doi: 10.1016/j.scitotenv.2022.153321
Wohl, E., Rathburn, S., Chignell, S., Garrett, K., Laurel, D., Livers, B., et al. (2017). Mapping longitudinal stream connectivity in the north vrain Creek watershed of Colorado. Geomorphology 277, 171–181. doi: 10.1016/j.geomorph.2016.05.004
Keywords: large wood, channel planform, bifurcations, anastomosing, river restoration, resilience, connectivity
Citation: Marshall A and Wohl E (2023) The continuum of wood-induced channel bifurcations. Front. Water 5:1155623. doi: 10.3389/frwa.2023.1155623
Received: 31 January 2023; Accepted: 20 March 2023;
Published: 11 April 2023.
Edited by:
Mohammad Safeeq, University of California, Merced, United StatesReviewed by:
Masato Kobiyama, Federal University of Rio Grande do Sul, BrazilCopyright © 2023 Marshall and Wohl. This is an open-access article distributed under the terms of the Creative Commons Attribution License (CC BY). The use, distribution or reproduction in other forums is permitted, provided the original author(s) and the copyright owner(s) are credited and that the original publication in this journal is cited, in accordance with accepted academic practice. No use, distribution or reproduction is permitted which does not comply with these terms.
*Correspondence: Anna Marshall, YW1hcnNoMDFAY29sb3N0YXRlLmVkdQ==
Disclaimer: All claims expressed in this article are solely those of the authors and do not necessarily represent those of their affiliated organizations, or those of the publisher, the editors and the reviewers. Any product that may be evaluated in this article or claim that may be made by its manufacturer is not guaranteed or endorsed by the publisher.
Research integrity at Frontiers
Learn more about the work of our research integrity team to safeguard the quality of each article we publish.