- 1Environmental Science, Radboud Institute for Biological and Environmental Science (RIBES), Radboud University, Nijmegen, Netherlands
- 2Animal Ecology and Physiology, Radboud Institute for Biological and Environmental Science (RIBES), Radboud University, Nijmegen, Netherlands
- 3Department River Dynamics and Inland Shipping, Deltares, Delft, Netherlands
Riverine systems are directly affected by plastic pollution and function as pathways for plastic transport to the sea. Plastic litter can enter the water by remobilization from the bed or from the riverbank, after which it can be transported with the flow. The process of remobilization is still poorly understood. To get a detailed understanding of the role rivers play in plastic transport and hence develop management measures to prevent or mitigate plastic transport it is vital to get a fundamental understanding of the factors contributing to remobilization of plastic on the riverbank. Therefore, we investigated how the wave action of inland navigation, one of the dominant actors in anthropogenic rivers, affects the remobilization of land-based plastics. Quantification of remobilization was performed along the riverbank of an intensely navigated river in the Netherlands (the river Waal) allowing for a real-world assessment of remobilization. Plastic pieces were placed at standardized distances from the average waterline to determine the plastic remobilization along the riverbank at different locations. Subsequently, wave actions of passing inland navigation was used to assess plastic remobilization. Inland navigation induced wave action was found to significantly cause remobilization of plastics present on the riverbank. The distance of the plastic object to the waterline combined with the wave height, riverbank slope and plastic size significantly affected remobilization probability. Overall, the remobilization effect was strongest on plastics present within ≤ 1.0 m from the waterline. With a wave height ≥ 0.5 m all plastics present up to 3.0 m from the waterline were remobilized and thereby potentially transported downstream through the river.
Introduction
Plastic pollution is an emerging anthropogenic threat to the environment and can potentially also be a hazard to human health (Mato et al., 2001; Teuten et al., 2007, 2009; Lang et al., 2008; Zalasiewicz et al., 2016). Predominantly entering the environment as waste, plastic is very persistent (Cole et al., 2011; Alimi et al., 2018). An estimated 76% of the total global plastic waste accumulated in natural environments or landfills up until 2017; this accounts for nearly 6,840 Mt (Geyer, 2020).
Even though much of the plastic pollution occurs on land, a large amount of plastic ends up in aquatic systems (Jambeck et al., 2015; OSPAR, 2017). The impact of plastics in aquatic systems is increasingly assessed, but the focus has largely been on marine systems (Blettler et al., 2018). However, research on plastic in riverine systems is also important because of direct effects as well as its contribution to the pollution of the oceans, functioning as pathways for land-based plastics (OSPAR, 2017; Schmidt et al., 2017). Yet, detailed knowledge of transport processes of plastics in rivers is limited but see Tramoy et al. (2020) and Newbould et al., 2021.
Plastic in rivers come in a wide variety of forms and can originate from multiple sources. Examples of sources are mismanaged waste, illegal dumping of waste often alongside rivers, generation of microplastic during mechanical recycling, littering, loss of fishing gear, wear and tear of tires, loss of nurdles, fiber release from the washing of synthetic clothing, and plastic from cosmetic products (Cole et al., 2011; GESAMP, 2016; Li et al., 2016; Zalasiewicz et al., 2016; OSPAR, 2017; Bernardini et al., 2020; The Pew Charitable Trusts SYSTEMIQ, 2020). Depending on the density (polymer), size and shape of a plastic item, it can be transported floating or submerged in the water column (GESAMP, 2016; OSPAR, 2017).
Plastic pollution can be harmful to riverine ecosystems and biota in multiple ways. Detrimental effects can occur through ingestion, animal entanglement, and accumulation and leakage of toxic compounds (van Emmerik and Schwarz, 2020). In freshwater systems, there is evidence that plastic is ingested by macroinvertebrates (Hurley et al., 2017; Windsor et al., 2019), fish (Horton et al., 2018; Urbanski et al., 2020), and birds (Holland et al., 2016). Furthermore, a recent study states that plastics are transferred throughout the food web (D'Souza et al., 2020), which could lead to bioaccumulation. Toxicity of plastic occurs through the leaching of additives into the environment or organisms (Oehlmann et al., 2009), or through accumulation and transfer of persistent organic pollutants (POPs) (Teuten et al., 2009).
A macroplastic item begins to be transported with the flow in the river after it is littered in an active river zone, or when stored macroplastics are remobilized (Liro et al., 2020). Remobilization can either occur from the surface of the riverbed or floodplain [e.g., at increasing flow velocities or during flooding of the floodplains Van Emmerik et al., 2022], or from the subsurface (e.g., by bank erosion). Waves generated by inland shipping can lead to remobilization of meso- and macroplastics stored both at the surface and in the subsurface. An item that is transported with the flow becomes stored, after it was deposited at the riverbed, entangled in vegetation, or stranded at the riverbank. The effect of remobilization and deposition of meso- and macroplastics determines the number of items in the water which are potentially transported with the river flow.
Ships navigating the river generate (primary and secondary) waves, return currents and propeller-induced currents. The primary wave can result in a drawdown (Goransson et al., 2014; Gabel et al., 2017; Mao and Chen, 2020). The wave action and ship-generated flow can, for example, lead to a decrease in potential aquatic macrophytes (Thunnissen et al., 2019), increased turbidity, and riverbank erosion (Goransson et al., 2014; Gabel et al., 2017; Mao and Chen, 2020). In groyne fields the effect of passing vessel is that the water level rises when the vessel approaches the groyne field, and a drop in water level when it leaves the groyne field. Moreover, the short waves generated by the vessel result in water level variation in the groyne field. These effects are largest for large ships with a relatively small drought (loaded vessels) that navigate close to the groyne field. Especially at the point where the groyne is connected to the riverbank the wave run-up can be large.
By generating waves and eroding the riverbank, land-based plastics can be mobilized toward the water (Newbould et al., 2021). This would be especially relevant for riverbanks near urbanized and densely populated areas where the amount of (recreational) littering is high (Battulga et al., 2019; Luo et al., 2019; Huang et al., 2020). However, the impact of inland navigation induced wave action on this remobilization, and thereby the potential riverine plastic transport, remains to be investigated. Therefore, this study is the first study that aims to quantitatively asses the contribution of inland navigation induced wave action on the remobilization of land-based plastics into the water, and thereby on plastic transport and fate in riverine systems.
The objective of the present study is to unravel the effect of inland navigation on the process of remobilization of meso- and macroplastics from the riverbank into the river. We hypothesize that inland navigation induced wave action significantly contributes to the remobilization of land-based plastics into the water and that the effect becomes stronger with increased wave intensity. The research was performed at the Waal near Nijmegen (the Netherlands), which is one of the most intensely navigated rivers in Europe (Pauli, 2010; Reeze et al., 2017).
The effect of navigation on plastic mobilization was measured in two distinctly different locations along the river. Since the river Waal has a traditional river training system (i.e., groynes), those two different locations are represented by two different groyne field classes (Sukhodolov et al., 2002). Additionally, we hypothesized that the effect of inland navigation would be different for different locations within the groyne fields. Therefore, all measurements were performed in three locations within the groyne fields (left, middle and right). Furthermore, we hypothesized that the slope of the riverbank, the distance of the plastics to the average waterline, and the plastic size would affect the impact of inland navigation induced wave action. Therefore, those parameters were included in our analysis.
Materials and Methods
Experimental Setup
Quantification of Plastic Remobilization From Riverbank to Water
The remobilization of plastics from the riverbank in a groyne field to the water was quantified by placing pieces of marked plastic in lines parallel to the waterline at 0.5, 1.0, and 3.0 meter from the waterline (Figure 1). The distance to the waterline was determined compared to the average water level when no inland navigation was passing. At each distance 20 pieces of flat standardized plastic items were placed. Two size classes were included; meso- (>5 mm ∧ <5 cm) and macroplastics (>5 cm) (van Emmerik and Schwarz, 2020), and all plastics used were polypropylene (PP), meaning it were hard, floating plastic pieces. Subsequently, the plastic remobilization from the riverbank into the water was quantified during a 15-min interval. Remobilization for each piece of plastic was categorized into two possible binary outcomes; (1) remobilization, or (2) no remobilization. Plastic that entered the water was immediately recaptured using a handheld net to minimize the introduction of plastic to the river (21 plastic pieces were lost, which accounts for 2.92% of total plastic pieces used). Experiments were conducted on days with little to no wind (bf ≤ 3) to exclude the effect of wind on the remobilization.
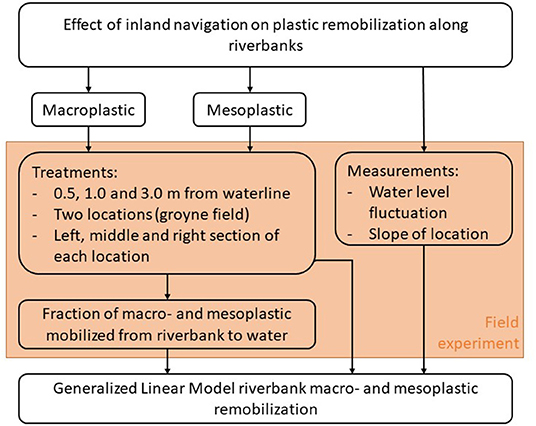
Figure 1. Flow chart of the used experimental set-up to assess macro- and mesoplastic remobilization under the influence of inland navigation induced waves and the resulting model on remobilization.
To assess variability in riverbank—water remobilization between different groyne fields the experiment was performed in two groyne field classes present in the river Waal: namely groyne field class three and six. Groyne field class identification was based on morphological patterns according to Sukhodolov et al. (2002) and was determined from areal pictures. Groyne field class three and six correspond with upstream shaped triangular deposition and uniform partial deposition, respectively. Groyne field class six [51.8714975, 5.8190625] was chosen due to the prevalence in the river Waal, thereby being representative for the inland navigation induced effects on plastic remobilization. Groyne field class three [51.8692691, 5.8910346] was included due to a different deposition pattern compared to groyne field class six, potentially impacting plastic remobilization. Within each groyne field the experiment was performed in the right and left corner, and in the middle (Figure 1), resulting in a total of 36 different experimental conditions. At every experimental location the slope of transect was measured using high resolution RTK GPS (PolarisGNSS).
During the 15-min duration of the remobilization experiment continuous pressure measurements were collected (1 Hz: HOBO Onset Water Level Data Logger U20L-04). The logger was attached to a brick to ascertain that it stayed in place despite the wave action of inland navigation. The brick was placed at a water depth of ~0.70 m, directly in front of the plastics (Figure 1). An additional water level logger was used as a barometric control to calculate the water depth of the logger placed in the water.
Data Processing
The raw data from the water level loggers was retrieved after which the water level was determined using HOBOware. The Water depth range was calculated per remobilization experiment of 15 mins as the maximum minus the minimum water level.
Data analysis was performed in R Version 4.0.3 (R Core Team, 2020) and consisted of an analysis of the plastic remobilization in relation to ship passage and several other variables (Table 1). Data distribution was determined with the “fitdistrplus” package (Delignette-Muller and Dutang, 2015). Model performance was assessed using the “MuMIn” (Burnham and Anderson, 2002) and “performance” package (Gelman and Hill, 2007). Data visualization was performed with “ggplot2” (Wickham, 2016) and the “viridis” color scheme was used (Garnier, 2018).
Analysis of Plastic Remobilization
Prior to modelbuilding variables were tested for collinearity. The correlation of variables was assessed using the “corrplot” package (Taiyun and Simko, 2017). To be able to determine the correlation coefficient, categorical variables were first transformed to numerical values; either ordinal or nominal based on the logic of the variable. Variables with a high correlation coefficient (≥ 0.70) were never included in the model simultaneously (Tabachnick and Fidell, 1996). To determine which of the correlated parameters should be included separate models were built for the correlating variables, and the best performing model was selected based on the Akaike's Information Criterion with a correction for small sample size (AICc).
A binomial generalized linear model (GLM) was used to analyze the impact of the measured variables on the fraction of plastics mobilized to the water. The analysis was performed using the glm function in R (R Core Team, 2020). The GML was built stepwise, and interactions were only included if they significantly improved the model. A Chi-square test was used to analyze the difference in deviance between the models, and the selection for significant model improvement was based hereon. Final model selection was performed by assessing the models based on the AICc and by testing if models were significantly different. During the process of modelbuilding the data for 3.0 meter from the water were dropped as it skewed the model results and strongly lowered the performance of the models. Therefore, all data presented as model output are based upon a model with only 0.5 meter and 1.0 meter as levels for the variable Distance to the water. Data predicted by the GLM were visualized using lines, and the raw data the prediction was based upon were depicted as point data.
Results
Data Exploration
The local water level fluctuation was higher after inland navigation passes than without inland navigation (Figure 2). The maximum Water depth range after ship passage based on the visualized data is 0.6 meter (Figure 2). During the experiments nearly always all plastics at 0.5 and 1.0 meter from the waterline were mobilized toward the water. On the other hand, during most of the experiments the remobilization fraction for plastics at 3.0 meters from the riverbank was zero, indicating no remobilization (Figure 3).
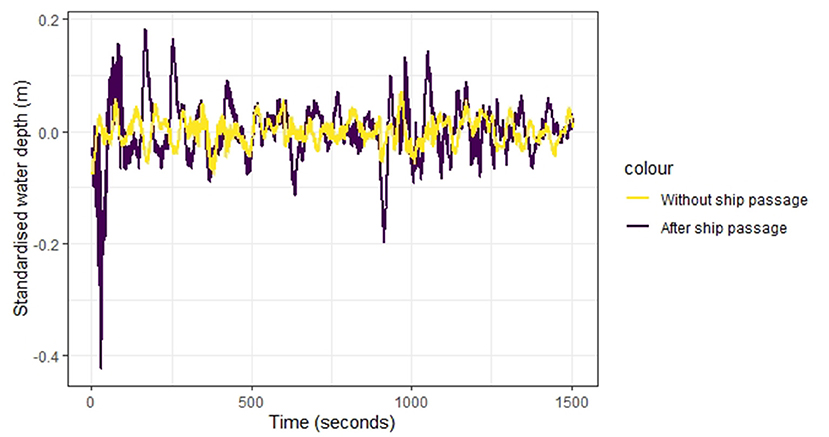
Figure 2. Water depth fluctuation with and without ship passage. These data are continuous measurements of the water depth and are presented as a standardized water depth (i.e., fluctuations around the mean water depth, which was set to zero). Two ship passages are shown (i.e., at ~t = 0 and at t = 900).
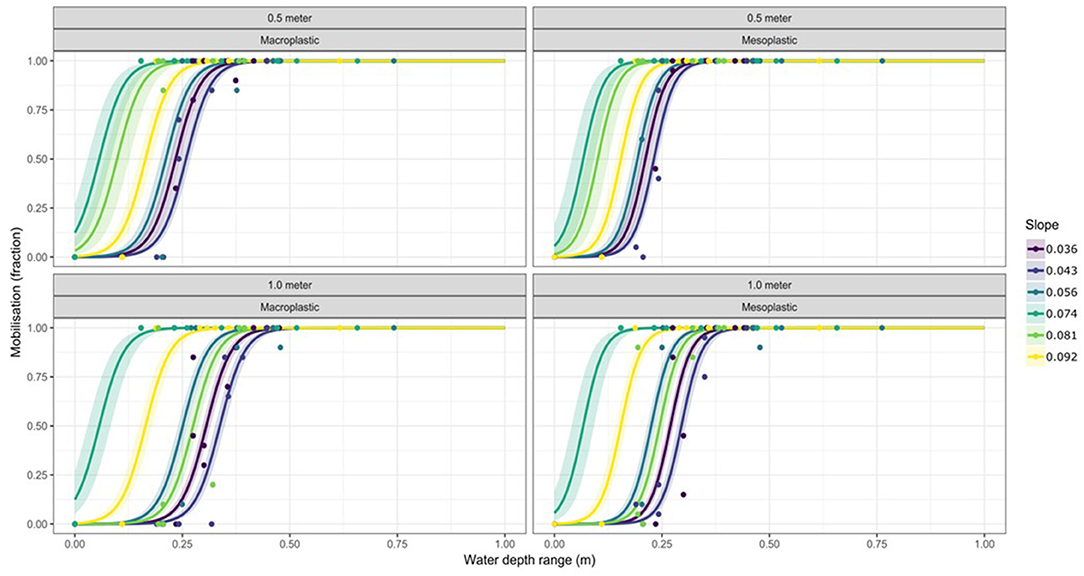
Figure 3. Generalized linear model output with the fraction of plastics mobilized from the shore to the water as a function of the water depth (0–1m), different plastic types (microplastic, macroplastic), distances to water (0.5 and 1 meter) and slopes (0.036–0.092). Contours depict the 95% CI.
Generalized Linear Model Results
The best GLM describes the fraction of plastic mobilized from the riverbank to the water with the Water depth range, the Slope, the Distance to the water, the Plastic type, the interaction between the Water depth range and the Plastic type, and the interaction between the Slope and the Distance to the water as explanatory variables. All explanatory variables significantly contributed to the model performance (Table 2). The AICc for this model was 1,024.5 and the Tjur's R2 was 0.872.
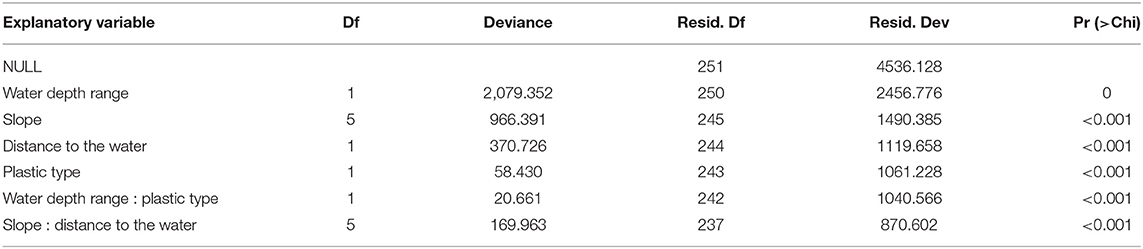
Table 2. Generalized linear model output with explanatory variables, degrees of freedom (Df), deviance, residual degrees of freedom (Resid. Df), residual deviance (Resid. Dev), and the p-values based on a Chi squared test[Pr(>Chi)].
With an increase in Water depth range the fraction of plastics mobilized increases. However, the steepness of the Slope impacts this relationship by reducing the effect of an increase in the Water depth range with an increase in Slope steepness. Additionally, there is a difference in the effect of the Water depth range for Plastic type, with a higher Water depth range required for the remobilization of macroplastics than for mesoplastics. Lastly, Distance to the water also impacts the effects of Water depth range on plastic remobilization. Plastics closer to the water (at 0.5 m) are mobilized at a lower Water depth range than plastics further from the water (1.0 m) (Figure 3).
Discussion
The results from this study clearly show that inland navigation contributes to the remobilization of plastics from riverbank to water in riverine systems, thereby illustrating that shipping affects plastic transport and fate. Specifically, water depth fluctuation caused by the inland navigation, the slope of the riverbank, the plastic size category, and the distance to the average waterline are relevant in explaining the remobilization of plastics. Five main conclusions can be deduced from the GLM predictions. First and foremost, Water depth range fluctuation induced by inland navigation shows a strong positive relation with the fraction of plastics mobilized. Secondly, the Water depth range needs to be larger to have the same amount of remobilization with a steeper slope. However, this pattern is not entirely consistent as the order of the predicted lines does not always correspond to the ordinal steepness of the slopes. Therefore, this pattern should be treated as a general pattern. Thirdly, macroplastics require a larger fluctuation in water depth range to have the same remobilization fraction as mesoplastics. Fourthly, plastics at 0.5 meter from the average water line are mobilized with less fluctuation in the water depth range than plastics at 1.0 meter from the water. Lastly, based on these predictions, independent of plastic type, slope, or distance to the water, all plastic pieces are mobilized to the water with a Water depth range of ≥0.5 meter at 0.5 and 1.0 meter from the waterline. Furthermore, the pattern in water depth fluctuation after ship passage is in accordance with that presented by De Roo et al. (2012) with regards to the primary and secondary wave systems.
Although the GLM model was simple and based on limited field data, the explained variability was high (R2 = 0.87), illustrating that the simplicity is not per se problematic. Even though the results of this study might not be comprehensive—there are undoubtedly more factors that are at play when considering the effect of inland navigation on plastic remobilization—we would like to stress that from the fieldwork we can visually confirm that the waves caused by inland navigation led to the remobilization of plastics. The bigger the waves, the more plastics were picked up. At the rare intervals at which no ships passed virtually no plastic was mobilized (an occasional one or two pieces were mobilized due to variation in wave action without ship passage). However, no studies were found that have quantitively investigated the effect of inland navigation on plastic transport and fate in riverine systems. The only reference to the potential effect of shipping is made by van Emmerik et al. (2020), stating that inland navigation might contribute to plastic transport and accumulation.
Having confirmed that inland navigation affects plastic transport and fate in riverine systems, we would like to mention that the results on plastic remobilization do not solely determine the number of plastics in the water, since some of the items may strand (again) at the riverbank or deposit to the bed. During the collection of field-data the plastics were immediately recaptured using a handheld net if they entered the water. Additionally, plastics that were picked up by waves, but deposited on the riverbank were counted as mobilized. The latter was decided upon with respect to the first point mentioned: in recapturing every piece of plastic that entered the water deposition on the riverbank was prevented, thereby leading to an unreliable amount of plastic deposition on the riverbank. This leads to an overestimation of plastic remobilization to the water, since not all plastics would per se end up in the water which were deposited on the riverbank and maybe some plastics that entered the water would be redeposited onto the riverbank. In future research it would be interesting to study the net effect of remobilization and stranding, by not immediately recapturing plastics that enter the water and by including more possible outcomes in the analysis.
Initially, we also attempted to perform the experiment with soft film plastics (biodegradable binbags). However, the effect of wind on this plastic category was very high, and therefore we excluded that category from the experiments. Based on this aspect we can hypothesize that for this specific plastic category the wind may be relevant in determining transport and fate.
One might argue that extreme events like floods are more influential in plastic remobilization between the riverbank and water than inland-navigation induced waves. However, floods occur a few times per year and even though the effect of a single ship-induced wave is limited, inland navigation induced waves are a near continuous stressor for intensely navigated waterways. To give an indication of the number of ships passing at the river Waal at Nijmegen: in 2015 ~105,000 ships passed (Reeze et al., 2017). Thereby, it is among the most intensely navigated rivers in Europe (Pauli, 2010; Reeze et al., 2017), resulting in virtually no moments without ship passage. Furthermore, extreme flood events predominantly occur in winter when the amount of recreational littering is limited. On the other hand, inland navigation continues all year round, and thereby exerts it pressure during summer also—the time of the year when recreational littering is more prevalent.
These data are relevant for both the scientific community and for policy makers. For the scientific community it is relevant because it aids in fundamentally understanding the drivers for plastic pathways in riverine systems. Thereby, the results might be useful in modeling attempts, which could possibly lead to better understanding the relative importance of the drivers of plastic transport and fate in riverine systems. For policy makers it is relevant because the results are meaningful in mitigating plastic transport and understanding plastic fate in riverine systems. For example, they can decide to implement measures that reduce the effect of the waves of inland navigation like longitudinal training dams (Collas et al., 2018). Furthermore, these results show that especially plastics close to the waterline are mobilized to the water which could help in efficiently focus cleaning efforts. Furthermore, the data are relevant since the intensity of inland navigation is only expected to increase over the coming years (European Commission, 2001; Pauli, 2010; Radmilovic and Vladislav, 2011).
This research contributes to a more holistic overview of plastic transport and fate in riverine systems and to fundamental understanding of the effect of inland navigation on those pathways. A better understanding of the drivers of riverbank to water remobilization is relevant in tackling the plastic pollution problem and implementing evidence-based techniques to reduce the plastic load in rivers.
Data Availability Statement
The datasets presented in this study were stored in the DANS EASY repository of the Radboud University, Nijmegen (https://doi.org/10.17026/dans-xjc-a2pp).
Author Contributions
JC, FB, and FC contributed to the conception and design of the study. JC, SO, and FC performed the fieldwork. JC organized the database, performed the statistical analysis, and wrote the first draft of the manuscript. JC, SO, FB, AH, and FC wrote sections of the manuscript. All authors contributed to manuscript revision, read, and approved the submitted version.
Conflict of Interest
The authors declare that the research was conducted in the absence of any commercial or financial relationships that could be construed as a potential conflict of interest.
Publisher's Note
All claims expressed in this article are solely those of the authors and do not necessarily represent those of their affiliated organizations, or those of the publisher, the editors and the reviewers. Any product that may be evaluated in this article, or claim that may be made by its manufacturer, is not guaranteed or endorsed by the publisher.
Acknowledgments
We would like to thank Mayra van der Vrande and Paul Arts for their help during the fieldwork. Moreover we would like to thank Margriet Schoor (Directorate-General for Public Works and Water Management) for organizing the exemption to release plastics into the water.
References
Alimi, O. S., Budarz, J. F., Hernandez, L. M., and Tufenkji, N. (2018). Microplastics and nanoplastics in aquatic environments: aggregation, deposition, and enhanced contaminant transport. Environ. Sci. Technol. 52, 1704–1724. doi: 10.1021/acs.est.7b05559
Battulga, B., Kawahigashi, M., and Oyuntsetseg, B. (2019). Distribution and composition of plastic debris along the river shore in the Selenga River basin in Mongolia. Environ. Sci. Pollut. Res. 26, 14059–14072. doi: 10.1007/s11356-019-04632-1
Bernardini, G. A. J., McConville, D., and Castillo, A. C. (2020). Macro-plastic pollution in the tidal Thames: an analysis of composite on and trends for the optimization of data collection. Marine Policy 119, 1064. doi: 10.1016/j.marpol.2020.104064
Blettler, M. C. M., Abrial, E., Khan, F. R., Sivri, N., and Espinola, L. A. (2018). Freshwater plastic pollution: Recognizing research biases and identifying knowledge gaps. Water Res. 143, 416–424. doi: 10.1016/j.watres.2018.06.015
Burnham, K. P., and Anderson, D. R. (2002). Model Selection and Multimodel Inference: A Practical Information-Theoretic Approach, 2nd Edn. New York, NY: Springer-Verlag.
Cole, M., Lindeque, P., Halsband, C., and Galloway, T. S. (2011). Microplastics as contaminants in the marine environment: a review. Marine Pollut. Bull. 62, 2588–2597. doi: 10.1016/j.marpolbul.2011.09.025
Collas, F. P. L., Buijse, A. D., van den Heuvel, L., van Kessel, N., Schoor, M. M., and Eerden, H. (2018). Longitudinal training dams mitigate effects of shipping on environmental conditions and fish density in the littoral zones of the river Rhine. Sci. Total Enviro. 619, 1183–1193. doi: 10.1016/j.scitotenv.2017.10.299
De Roo, S., Vanhaute, L., and Troch, P. (2012). “Impact of ship waves on the sediment transport in a nature friendly bank protection,” in River Flow, Vol. 1 and Vol. 2, ed R. M. Munoz.
Delignette-Muller, M. L., and Dutang, C. (2015). fitdistrplus: An R Package for fitting. Distributions 64, 4. doi: 10.18637/jss.v064.i04
D'Souza, J. M., Windsor, F. M., Santillo, D., and Ormerod, S. J. (2020). Food web transfer of plastics to an apex riverine predator. Global Change Biol. 26, 3846–3857. doi: 10.1111/gcb.15139
European Commission (2001). WHITE PAPER—European Transport Policy for 2010: Time to Decide. edited by Office for Official Publications of the European Communities. Luxembourg.
Gabel, F., Lorenz, S., and Stoll, S. (2017). Effects of ship-induced waves on aquatic ecosystems. Sci. Total Environ. 601, 926–939. doi: 10.1016/j.scitotenv.2017.05.206
Garnier, S.. (2018). “viridis: Default Color Maps from ‘matplotlib'.” R package version 0, 5.1. Available online at: https://CRAN.R-project.org/package=viridis (accessed March 1, 2022).
Gelman, A., and Hill, J. (2007). Data analysis using regression and multilevel/hierarchical models. Cambridge; New York, NY: Cambridge University Press. doi: 10.1017/CBO9780511790942
GESAMP (2016). “Sources, fate and effects of microplastics in the marine environment: part two of a global assessment,” in IMO/FAO/UNESCO-IOC/UNIDO/WMO/IAEA/UN/UNEP/UNDP Joint Group of Experts on the Scientific Aspects of Marine Environmental Protection.
Geyer, R.. (2020). Production, use, and fate of synthetic polymers. Plastic Waste Recycl. 20, 5. doi: 10.1016/B978-0-12-817880-5.00002-5
Goransson, G., Larson, M., and Althage, J. (2014). Ship-generated waves and induced turbidity in the gota alv river in Sweden. J. Waterway Port Coast. Ocean Eng. 140, 3. doi: 10.1061/(ASCE)WW.1943-5460.0000224
Holland, E. R., Mallory, M. L., and Shutler, D. (2016). Plastics and other anthropogenic debris in freshwater birds from Canada. Sci. Total Environ. 571, 251–258. doi: 10.1016/j.scitotenv.2016.07.158
Horton, A. A., Jurgens, M. D., Lahive, E., van Bodegom, P. M., and Vijver, M. G. (2018). The influence of exposure and physiology on microplastic ingestion by the freshwater fish andITRutilus rutilusandIT (roach) in the River Thames, UK. Environ. Pollut. 236, 188–194. doi: 10.1016/j.envpol.2018.01.044
Huang, Y. L., Tian, M., Jin, F., Chen, M. Y., Liu, Z. G., He, S. Q., et al. (2020). Coupled effects of urbanization level and dam on microplastics in surface waters in a coastal watershed of Southeast China. Marine Pollut. Bull. 20, 154. doi: 10.1016/j.marpolbul.2020.111089
Hurley, R. R., Woodward, J. C., and Rothwell, J. J. (2017). Ingestion of microplastics by freshwater tubifex worms. Environ. Sci. Technol. 51, 12844–12851. doi: 10.1021/acs.est.7b03567
Jambeck, J. R., Geyer, R., Wilcox, C., Siegler, T. R., Perryman, M., Andrady, A., et al. (2015). Plastic waste inputs from land into the ocean. Science 347, 768–771. doi: 10.1126/science.1260352
Lang, I. A., Galloway, T. S., Scarlett, A., Henley, W. E., Depledge, M., Wallace, R. B., et al. (2008). Association of urinary bisphenol A concentration with medical disorders and laboratory abnormalities in adults. Jama-J. Am. Med. Assoc. 300, 1303–1310. doi: 10.1001/jama.300.11.1303
Li, W. C., Tse, H. F., and Fok, L. (2016). Plastic waste in the marine environment: a review of sources, occurrence and effects. Sci. Total Environ. 566, 333–349. doi: 10.1016/j.scitotenv.2016.05.084
Liro, M., van Emmerik, T., Wyzga, B., Liro, J., and Mikus, P. (2020). Macroplastic storage and remobilization in rivers. Water 12, 7. doi: 10.3390/w12072055
Luo, W. Y., Su, L., Craig, N. J., Du, F. N., Wu, C. X., and Shi, H. H. (2019). Comparison of microplastic pollution in different water bodies from urban creeks to coastal waters. Environ. Pollut. 246, 174–182. doi: 10.1016/j.envpol.2018.11.081
Mao, L. L., and Chen, Y. M. (2020). Investigation of ship-induced hydrodynamics and sediment suspension in a heavy shipping traffic waterway. J. Marine Sci. Eng. 8, 424. doi: 10.3390/jmse8060424
Mato, Y., Isobe, T., Takada, H., Kanehiro, H., Ohtake, C., and Kaminuma, T. (2001). Plastic resin pellets as a transport medium for toxic chemicals in the marine environment. Environ. Sci. Technol. 35, 318–324. doi: 10.1021/es0010498
Newbould, R. A., Powell, D. M., and Whelan, M. J. (2021). Macroplastic debris transfer in rivers: a travel distance approach. Front. Water 3, 724596. doi: 10.3389/frwa.2021.724596
Oehlmann, J., Schulte-Oehlmann, U., Kloas, W., Jagnytsch, O., Lutz, I., Kusk, K. O., et al. (2009). A critical analysis of the biological impacts of plasticizers on wildlife. Philosophic. Transact. Royal Soc. B-Biologic. Sci. 364, 2047–2062. doi: 10.1098/rstb.2008.0242
OSPAR (2017). Environmental Impact of Human Activities Series Assessment document of land-based inputs of microplastics in the marine environment. London: OSPAR Commision.
Pauli, G.. (2010). Sustainable transport: a case study of Rhine navigation. Nat. Resour. Forum 34, 236–254. doi: 10.1111/j.1477-8947.2010.01309.x
PolarisGNSS. (2021). “RTK receiver [Apparatus and Software]”. Available online at: https://www.polaris-gnss.com/home (accessed April 1, 2021).
R Core Team (2020). “R: A Language and Environment for Statistical Computing.” Available online at: https://www.R-project.org/.
Radmilovic, Z., and Vladislav, M. (2011). “Role of danube Inland navigation in Europe.” Int. J. Traffic Transp. Eng. 1, 12.
Reeze, B., van Winden, A., Postma, J., Pot, R., Hop, J., and Liefveld, W. (2017). Watersysteemrapportage Rijntakken 1990-2015 : Ontwikkelingen waterkwaliteit en ecologie. edited by Bart Reeze Water and Ecologie. Harderwijk.
Schmidt, C., Krauth, T., and Wagner, S. (2017). Export of plastic debris by rivers into the sea. Environ. Sci. Technol. 51, 12246–12253. doi: 10.1021/acs.est.7b02368
Sukhodolov, A., Uijttewaal, W. S. J., and Engelhardt, C. (2002). On the correspondence between morphological and hydrodynamical patterns of groyne fields. Earth Surface Process. Landforms. 27, 289–305. doi: 10.1002/esp.319
Tabachnick, B. G., and Fidell, L. S. (1996). Using Multivariate Statistics. California State University, Northridge: Harper Collins.
Taiyun, W., and Simko, V. (2017). “R package “corrplot”: Visualization of a Correlation Matrix. Available online at: https://github.com/taiyun/corrplot.
Teuten, E. L., Rowland, S. J., Galloway, T. S., and Thompson, R. C. (2007). Potential for plastics to transport hydrophobic contaminants. Environ. Sci. Technol. 41, 7759–7764. doi: 10.1021/es071737s
Teuten, E. L., Saquing, D. R. U., Knappe, M. A., Barlaz, S., Jonsson, A., Bjorn, S. J., et al. (2009). Transport and release of chemicals from plastics to the environment and to wildlife. Philosophic. Transact. Royal Soc. B-Biologic. Sci. 364, 2027–2045. doi: 10.1098/rstb.2008.0284
The Pew Charitable Trusts and SYSTEMIQ (2020). “Breaking the plastic wave—a comprehensive assessment of pathways towards stopping ocean plastic pollution,” in The Pew Charitable Trusts. Available online at: https://www.pewtrusts.org/-/media/assets/2020/07/breakingtheplasticwave_report.pdf (accessed 16-06-2022).
Thunnissen, N. W., Collas, F. P. L., Hendriks, A. J., and Leuven, R. S. E. W. (2019). Effect of shipping induced changes in flow velocity on aquatic macrophytes in intensively navigated rivers. Aquatic Bot. 159, 103145. doi: 10.1016/j.aquabot.2019.103145
Tramoy, R., Gasperi, J., Colasse, L., Silvestre, M., Dubois, P., Noûs, C., et al. (2020). Transfer dynamics of macroplastics in estuaries—New insights from the Seine estuary: Part 2. Short-term dynamics based on GPS-trackers. Marine Pollut. Bull. 160, 111566. doi: 10.1016/j.marpolbul.2020.111566
Urbanski, B. Q., Denadai, A. C., Azevedo-Santos, V. M., and Nogueira, M. G. (2020). First record of plastic ingestion by an important commercial native fish (Prochilodus lineatus) in the middle Tiete River basin, Southeast Brazil. Biota Neotropica 20, 3. doi: 10.1590/1676-0611-bn-2020-1005
Van Emmerik, T., Mellink, Y., Hauk, R., Waldschläger, K., and Schreyers, L. (2022). Rivers as plastic reservoirs. Front. Water 3, 786936. doi: 10.3389/frwa.2021.786936
van Emmerik, T., Roebroek, C., Winter, W., Vriend, P., Boonstra, M., et al. (2020). Riverbank macrolitter in the Dutch Rhine-Meuse delta. Environ. Res. Lett. 15, 10. doi: 10.1088/1748-9326/abb2c6
van Emmerik, T., and Schwarz, A. (2020). Plastic debris in rivers. Wires Water. 7, e1398. doi: 10.1002/wat2.1398
Wickham, H.. (2016). ggplot2: Elegant Graphics for Data Analysis. Available online at: https://ggplot2.tidyverse.org. doi: 10.1007/978-3-319-24277-4
Windsor, F. M., Tilley, R. M., Tyler, C. R., and Ormerod, S. J. (2019). Microplastic ingestion by riverine macroinvertebrates. Sci. Total Environ. 646, 68–74. doi: 10.1016/j.scitotenv.2018.07.271
Keywords: groyne field, shipping, pollution, wave action, meso- and macroplastic
Citation: Climo JD, Oswald SB, Buschman FA, Hendriks AJ and Collas FPL (2022) Inland Navigation Contributes to the Remobilization of Land-Based Plastics Into Riverine Systems. Front. Water 4:888091. doi: 10.3389/frwa.2022.888091
Received: 02 March 2022; Accepted: 21 June 2022;
Published: 04 August 2022.
Edited by:
Constantinos V. Chrysikopoulos, Technical University of Crete, GreeceReviewed by:
Sedat Gundogdu, Çukurova University, TurkeyAgnieszka Deja, Maritime University of Szczecin, Poland
Copyright © 2022 Climo, Oswald, Buschman, Hendriks and Collas. This is an open-access article distributed under the terms of the Creative Commons Attribution License (CC BY). The use, distribution or reproduction in other forums is permitted, provided the original author(s) and the copyright owner(s) are credited and that the original publication in this journal is cited, in accordance with accepted academic practice. No use, distribution or reproduction is permitted which does not comply with these terms.
*Correspondence: Frank P. L. Collas, f.collas@science.ru.nl