- 1Ecothropic, Cimarron, CO, United States
- 2Ecothropic México A.C., San Cristóbal de las Casas, Mexico
- 3Water Resources Management Group, Department of Environmental Sciences, Wageningen University, Wageningen, Netherlands
Small-scale managed aquifer recharge (MAR) has significant potential as a bottom-up, community-based adaptation solution for increasing local groundwater availability and reducing the experience of drought for small-holder agriculturalists and rural populations. Using a suite of low-tech and low-cost techniques, small-scale MAR increases the infiltration of surface water runoff to replenish groundwater and deliver a suite of societal and ecosystem benefits. While the technique is hydrologically promising, populations may not act, implementation may not be permitted, interventions may not be effective for the population in question, or unexpected consequences (paradoxes) may result. For small-scale MAR to effectively reduce the experience of drought, it is imperative to unravel how such interventions play out within the complexity of the sociohydrological system in which they are implemented. Building on previous conceptualizations of the sociohydrological system, we apply the lens of political ecology to conceptualize the interplay between biophysical, climate, and social systems. Additionally, we explore considerations, feedbacks, and potential paradoxes in the uptake, implementation, and effectiveness of small-scale MAR interventions. We show that within the parameters of climate trends, small-scale MAR may serve to increase the functionality of ecosystems and reduce the impact of climate extremes, while protecting livelihoods and supporting society. In a positive feedback loop, small-scale MAR may both reduce the likelihood of experiencing drought while simultaneously increasing the ability and likelihood of the population to cope with or further avoid drought. Paradoxes and negative feedback processes, however, must be avoided. Specific factors, and how such factors interplay, will be different in each context where small-scale MAR is implemented. Conceptualizing the sociohydrological system in which small-scale MAR is implemented, including explicitly accounting for climate trends and using a power-sensitive approach, allows us to avoid overestimating or oversimplifying small-scale MAR as a solution, while supporting practical and effective implementation.
Introduction
Groundwater from replenishable unconfined shallow aquifers and non-replenishable confined aquifers is often a key resource for reliable and good quality water, especially for populations living in arid regions (Hoogesteger, 2022). Small-holder farmers often depend on water from shallow wells for livelihoods and household use. Water levels in shallow and unconfined aquifers is recharged by rainfall and surface water percolating through soil and substrates, streambeds, and terrestrial wetlands (Dillon et al., 2019). The unconfined aquifer can be considered a renewable resource when recharge rates are balanced with the rate of water withdrawals. Shallow groundwater is increasingly important for livelihoods as changing precipitation patterns challenge water availability in a context of increased competition for water and an increasing number of rural-urban water transfers (Molle and Berkoff, 2009; Molle et al., 2010; Hommes and Boelens, 2017; Hommes et al., 2019).
Human interventions in the landscape that increase the infiltration of surface water runoff have been shown to have significant impact on water tables, increasing groundwater availability (Patel and Prabhakar, 2012; Renganayaki and Elango, 2013; Malik et al., 2014; Rockström et al., 2014; Sadoff et al., 2015). With managed aquifer recharge (MAR) interventions, increasing the amount of water in the targeted aquifer is an explicit aim, while in other interventions (e.g., irrigation expansion, watershed restoration, soil conservation, and water harvesting) recharge may be an unintended effect and invisible benefit.
This paper explores MAR as a suite of socio-technical interventions that are intended to increase the infiltration of surface water runoff into groundwater systems through small (in infrastructural scale), low-tech, and low-cost techniques that can be easily implemented by local land managers. For example, small check dams built from stones, earth, cement or other materials may slow water flowing down small ravines, increasing rates of water infiltration into substrates. Contour bunds, linear mounds built horizontally along slopes (often in degraded or cultivated areas), use similar materials and function comparably, detaining water flowing down slopes. Rainwater runoff from roads can be channeled into porous roadside ditches or small-infiltration wells. Wetlands, which naturally mitigate flooding and maintain local water tables, can be protected. Such techniques have been used by human populations across diverse geographies for millennia for capturing seasonal rainfall and increasing long-term drought resilience (Kennedy, 1995; Pandey et al., 2003; Malik et al., 2014; Dillon et al., 2019; Ochoa-Tocachi et al., 2019).
The hydrological impact of MAR on increased groundwater levels has been widely discussed and documented (Patel and Prabhakar, 2012; Renganayaki and Elango, 2013; Malik et al., 2014; Rockström et al., 2014; Sadoff et al., 2015; Basel et al., 2020b). Interventions may also reduce soil erosion caused by runoff, and spread water availability more evenly throughout the year, reducing peak flows while increasing base flows (by slowing the movement of water through a watershed by passing it slowly through sediments instead of quickly overland (Dillon et al., 2019). Small-scale MAR techniques may reduce hydro-meteorological risks of drought and flooding, support soil humidity (mitigating the vulnerability of agriculture to climate trends), and regulate water tables that maintain vegetation and biodiversity (reducing local temperature extremes and regulating microclimates through evapotranspiration (Otieno and Anyah, 2012; Ribeiro, 2020; Seddon et al., 2020).
Small-scale MAR is increasingly relevant given current and projected climate trends and related hydro-meteorological extremes, and is recognized as a promising adaptation strategy for reducing vulnerability to climate impacts (Shah, 2009; Guyennon et al., 2017; International Groundwater Assessment Centre, 2020; IPCC, 2021). Rural and indigenous populations often have place-based and generational experience in managing local resources, and through daily interactions with resources are de facto resource managers (Berkes and Armitage, 2010; Boillat and Berkes, 2013; Reid, 2016; Basel et al., 2020a). Small-scale MAR interventions may then be particularly useful for people with land-based and groundwater-dependent livelihoods. As a small-scale, locally-driven, bottom-up measure, MAR is a promising strategy for community-based adaptation to climate change (Basel et al., 2020b).
As interventions can serve “...to protect, sustainably manage, and restore natural or modified ecosystems,” therefore “…providing human wellbeing and biodiversity benefits,” MAR can be also be considered a Nature-based Solution (Cohen-Shacham et al., 2016, p. 5). Given the potential benefits small-scale MAR offers for people and ecosystems, interventions may seem to be a nostrum for development interventionists working to address Sustainable Development Goal 6 which aims to “ensure availability and sustainable management of water and sanitation for all.” The potential hydrological impact of an intervention does not however ensure the intervention delivers increased water availability. Hydrological interventions are implemented within a complex socio-ecological system (SES) (Ostrom, 2009), and furthermore within a hydrological system in which:
“…humans influence water resources through behavior and infrastructural interventions. In turn, the availability of hydrological resources and hydro-climatic events influence human behavior and interventions, creating interplay and feedbacks between the two systems.” (Rusca and Di Baldassarre, 2019).
Furthermore, water management and water distribution are also political. The flow of water is not only guided by gravity but also the interplay of power relations. While small-scale MAR is hydrologically promising, populations may not act, implementation may not be permitted, interventions may not benefit the intended population, or unexpected consequences (paradoxes) may result.
Existing literature has largely focused on the hydrological and technical aspects of small-scale MAR; limited exceptions include Kennedy (1995), Pandey et al. (2003), Lasage and Verburg (2015), Lasage et al. (2015), and Ochoa-Tocachi et al. (2019). The complexity of the human-water interactions results in feedbacks and emergent dynamics that call for a more holistic approach (Blöschl et al., 2013). The implementation of small-scale MAR as a community-based adaptation strategy requires contextualizing these interventions within the complex sociohydrological system in which they are implemented. As a preliminary step to address this gap, this paper explores the following question: How can we conceptualize the sociohydrological system in which small-scale MAR interventions are implemented to increase groundwater availability in small or upper catchments?
To do this, we turn to and then further develop existing conceptualizations of the sociohydrological system. We propose climate trends as an additional and linked system that interacts with both human and hydrological systems. A critical lens is then applied to better understand the matrix of factors that may influence intervention outcomes, including possible paradoxes. Based on these understandings, the Methodology used is presented below. The Results section begins by applying a critical lens to solutions-oriented research and then presents the sociohydrological conceptualization of small-scale managed aquifer recharge from a critical sociohydrological perspective. The following three subsections on the biophysical, climate, and social systems are dedicated to exploring the interplay between these systems within the sociohydrological system in which small-scale MAR is implemented. The paper concludes with an overview of the conceptualization of the system and key findings.
Methodology
To situate small-scale MAR within existing sociohydrological understandings of linked human-water systems and explore system dynamics, we turned to and then further developed previous conceptualizations and models of the sociohydrological system. To identify relevant conceptualizations and models for analysis, we used a snowball approach. The search was started using Google Scholar, chosen for the diversity of resources represented in the platform. Appropriate literature to review was then selected using the following criterion: (1) relatively recent research (since the founding of the discipline in 2014);1 (2) applicable research topics; and (3) the presentation of a conceptualization/analysis of the sociohydrological system. Using this approach, nine papers, dating 2014–2021, were chosen for in-depth comparison and analysis2. The review was not intended to be exhaustive but rather representative, aimed at drawing on existing conceptualizations and understandings of the sociohydrological system to consider how small-scale MAR is situated within these understandings.
Each paper was reviewed to identify key themes, system variables, drivers, feedbacks, paradoxes, and possible research gaps. Resulting data were then compared across the papers to cluster similar and related concepts, and then identify agreements, patterns, and contradictions. Where the focus of the research was on agriculture, we extrapolated research findings as they would apply to water availability for combined agricultural and household use.
We then anchored these considerations in the framework presented in Di Baldassarre et al. (2018) and the conceptualization of the sociohydrological process of community-based adaptation presented in Basel et al. (2020b). These two frameworks were used as a “base map” for visualizing the relationships between the key biophysical, climate, and social considerations identified in the analysis of the selected papers, as related to small-scale MAR.
Results
Applying a critical lens to solutions oriented-research
Research on the interplay between human and water systems has largely been divided into two approaches. Hydrosocial literature applies a political ecology lens to examine social processes including power and scalar politics (for example Boelens, 2014; Linton and Budds, 2014; Boelens et al., 2016; Vos et al., 2020). Sociohydrology, rooted in the earth sciences, applies a (post) positivist approach to address specific issues (Wesselinck et al., 2017; Ross and Chang, 2020). According to Ross and Chang (2020, p. 1) “Without comprehending those interactive relationships, appropriate human responses to challenges and hazards involved in hydrologic systems become effectively impossible.” The sociohydrological literature therefore addresses the interactions between hydrology, hydro-climatic events, society, and interventions, seeking to understand how humans can alter or influence hydrological regimes and, circularly, how water availability and water related-hazards influence human behavior, which in turn, influences hydrological regimes (Pande and Sivapalan, 2016; Di Baldassarre et al., 2019; Rusca and Di Baldassarre, 2019; Basel et al., 2020b).
Applying the sociohydrological approach to small-scale MAR then supports “appropriate human responses” to the experience of drought. However, questions including who benefits, who loses, who has decision-making power, and the discourses that drive those decisions, also come into play. The answers to these questions influence how, when, by whom, and with what results small-scale MAR is implemented. These questions are inherently related to politics and power, across macro, micro, and basin scales. Therefore we assert that sociohydrological research has to increasingly account for social institutions, practices and norms by making research power-sensitive (see Melsen et al., 2018; Rusca and Di Baldassarre, 2019; Madani and Shafiee-Jood, 2020).
The following conceptualization draws on, consolidates, and further develops existing knowledge while recognizing that models simplify inherently complex systems, resulting in potential power issues and bias. Conceptualizing the system however allows us to recognize the complexity of the system, and identify important considerations, positive and negative feedback processes, and possible paradoxes. As we are reminded by Ostrom et al. (2007), there are no panaceas. Every context where small-scale MAR is implemented will represent a unique confluence of factors that must be considered accordingly. As political ecology further reminds us:
“Cross-regional and cross-cultural comparison may reveal important opportunities for mutual learning or even grassroots' cross-border engagement and solidarity, without falling in the trap of mainstream policies and neoliberal discourses promoting ‘good governance' and ‘best practices'…” (Vos et al., 2020, p. 9).
A sociohydrological conceptualization of small-scale managed aquifer recharge
A proposed conceptualization of the system in which small-scale MAR is implemented is depicted in Figure 1. The diagram shows a sociohydrological system made up of (i) biophysical, (ii) climate, and (iii) social subsystems. The structure and state of each subsystem respond to drivers within this sociohydrological system. Responses also act as drivers that circularly act on all three subsystems. The climate system drives local weather which drives the state, structure, and response of the social and biophysical subsystems. The state and structure of the biophysical subsystem respond with ecosystem/hydrological function and hazards which become drivers affecting local climate and the social subsystem, which may respond with socio-political and/or technological interventions.
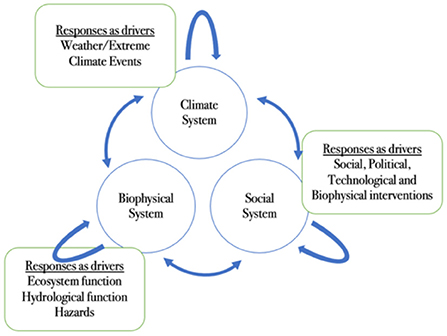
Figure 1. The proposed conceptualization of the sociohydrological system in which small-scale managed aquifer recharge interventions are implemented. Adapted from: Di Baldassarre et al. (2013), Di Baldassarre et al. (2018), Rusca and Di Baldassarre (2019), and Basel et al. (2020b).
Small-scale MAR is one response of the social system to climate, social, and biophysical drivers. Small-scale MAR interventions consequently drive the state, structure, and response of all subsystems; namely the biophysical, climate (particularly regarding microclimates), and social. The interaction between systems may result in feedbacks (between systems or a system acting upon itself) and/or paradoxes (unintended consequences). For example, an intervention could inadvertently increase the vulnerability of the population the intervention was intended to help. Paradoxes may result because human behavior is highly unpredictable (Wesselinck et al., 2017). Societies are vastly diverse with social dynamics, identities, and decision making-behavior developing across vastly divergent historical, cultural, and political pathways. Sociohydrology has identified multiple such phenomena that may apply to the sociohydrological system in which small-scale MAR is implemented (see Di Baldassarre et al., 2019). These include safe-development paradox (Kates et al., 2006); supply-demand cycle (Kallis, 2010); adaptation effect (Di Baldassarre et al., 2015); pendulum swing (see Gleick and Palaniappan, 2010); rebound effect (Kandasamy et al., 2014); and aggregation effect (see Olson, 1965; Ostrom, 1990; Zwarteveen et al., 2017).3 Recognizing and planning for the potential eventuality of phenomena and possible paradoxes is fundamental to designing effective solutions (i.e., small-scale MAR resulting in decreased experience of drought).
System dynamics (and the possible occurrence of paradoxes) are complicated by spatial and temporal scales. Small-scale MAR interventions are implemented at a basin or catchment level, but are influenced by, and connected to, both macro- and micro-spatial scales spanning hydrological, climatic, social, and political processes. Dynamic interactions between slow and fast variables across all elements of the system are also simultaneously at play (Elshafei et al., 2014). As Biggs et al. (2012; as quoted by Penny and Goddard, 2018, p. 40) explains, “Slow variables determine the underlying structure of the socio-ecological system (SES), whereas the dynamics of the system typically arise from interactions and feedback processes between fast variables that respond to conditions created by slow variables.” The structure (the condition of a system over an extended time frame—e.g., ~30 years) of each subsystem may be considered a slow changing variable, whereas the current state (rapidly changing conditions) of each subsystem might be considered a fast-changing variable, which overtime can affect the (slow-changing) structure of each subsystem. To exemplify the possible interactions (and paradoxes) between slow and fast variables, we consider the following: the construction of small-scale MAR infrastructure may be a relatively fast process, while watching wells fill may be a slow process, as infiltrated groundwater slowly moves through substrates. When people do not see results from their efforts, they may be less likely to continue to act. Alternatively, MAR interventions may be implemented after hydrological drought has increased the hydrophobia of soils and rainfall is inadequate to recharge water tables. As a result, intervention was too late to prevent the experience of impending drought, minimizing the impetus to create and maintain small-scale MAR infrastructure. Conversely, the experience of drought may be suddenly felt, while generating social will to build small-scale MAR infrastructure may take years.
In summary, the conceptualization of the sociohydrological system in which small-scale MAR is implemented is representative of the interplay between natural, social (defined by a diversity of local characteristics and unpredictability), and climatological processes (as further discussed below). The dynamic stage of small-scale MAR, including the complexities and range or potential drivers and responses, and value-driven decisions, suggests the system can be understood as a complex adaptive system (CAS), defined by “interconnectedness, innovation, [and] non-linear change”—in which uncertainly plays a central role (Bohensky et al., 2015, p. 142; Penny and Goddard, 2018; Clark and Harley, 2020).
The biophysical subsystem: Key variables and drivers
Water moves through the biophysical system guided by geomorphology, hydrological processes, ecological processes, and the climate regime. Slow-changing characteristics of the system including catchment size, type of aquifer, and a myriad of other factors explained in technical manuals, determine if, how, and where recharged water will naturally flow (Elshafei et al., 2014; Hale et al., 2015), including which human populations will benefit from increased water tables; how upstream recharge affects downstream populations, ecosystems, and flood/drought risk; or whether recharged water flows into inaccessible deep aquifers. The state of the biophysical system can be understood as the shorter-term responses of the biophysical system to interactions between social-climate-biophysical systems.4
The state of the system includes hydrologic function (including precipitation, evaporation, land use, basin modification, water balance, and water extraction rates), environmental degradation/alteration of landscape, and ecosystem health (including diversity, redundancy, and connectivity; Penny and Goddard, 2018). A sociohydrological approach suggests the structure, state, and functionality of the biophysical system, including environmental change factors, determine ecosystem services5,6 (Elshafei et al., 2014; Mao et al., 2017). The state of the system is a “…product of conflicting factors from both sides (social and hydrological), such as human demand and ecosystem supply, human disturbances and ecosystem regulation and regeneration, and human management and water resources” (Mao et al., 2017, p. 3,661). Increased hydrological extremes of flood and drought can result. As expressed by Anne von Loon,7 “drought and floods are caused by extremes of the same hydrological cycle and hence are correlated by dynamic feedback, strongly interlinked with human processes. Treating droughts and floods as independent phenomena, while ignoring their interaction with societal forces, leads to incomplete/distorted understanding of the processes that lead to the impacts experienced, and hence to possible underestimations of future risks.” The interplay between all of these factors is further responsive to changes in the climate system including extreme weather events, high temperatures, and precipitation trends.
The biophysical system is therefore not simply a clearly delineated product of the earth sciences, but plastic, being molded by the dynamic and constant interactions within and between systems. As a social system intervention in the biophysical system, small-scale MAR is simply one lever within this complex system. The illustrative examples of possible interactions are innumerous. Changes in vegetative landcover and soil permeability including deforestation, reforestation, afforestation, and urbanization may affect local microclimates (i.e., average local temperatures, high and low temperature extremes, ambient humidity, and local precipitation events), which in turn affect the water balance in a localized area. Changes in local climate regimes can further reduce precipitation, runoff, and soil permeability, shifting baseline hydrological conditions and trajectories. Land and agricultural management that results in decreased soil humidity can compound the impact of extended periods of high temperatures and correlating high evaporation rates. The result is hydrophobic soil conditions which further reduce soil humidity and recharge rates. Water resource use and transfer, including through virtual water exports8 can lower water tables, affecting vegetative cover, biodiversity, and local and regional climates (Otieno and Anyah, 2012; Keune et al., 2016). The functionality of the biophysical system then reflects not only the natural sciences but the normative goals and political choices of the people managing and interacting with the biophysical system (Mao et al., 2017), which could include reducing the risk of drought and increasing ecosystem function to facilitate positive system feedback processes (i.e., increasing local groundwater levels).
The social system is also molded by the biophysical environment through the relationship between people and functional ecosystems. This relationship provides for livelihoods, food, and water, while also supporting and influencing culture, knowledge, and social cohesion (see Hale et al., 2015; Basel et al., 2020a,b). The reciprocal relationship between people and the natural environmental is especially important for traditional and subsistence-based populations which may be the most likely to implement small-scale MAR interventions (Basel et al., 2020b). While decreased ecosystem functionality increases the biophysical possibility of drought, decreased ecosystem services may simultaneously increase community sensitivity and overall vulnerability to drought through inhibiting the ability for the population to adapt (cope, avoid, or reduce the impact of the hazard) (Elshafei et al., 2014; Kuil et al., 2016; Roobavannan et al., 2017; Basel et al., 2020b). For example, drought may reduce agricultural production and livelihoods, limiting food availability and/or financial resources populations have to acquire food from alternative sources. Decreased livelihoods may encourage migration, deteriorating local social coherence (reducing the likelihood of collective action to implement small-scale MAR) and the presence of physically capable individuals to build MAR infrastructure (further discussed below).
The climate subsystem: Key variables and drivers
“Although climate is inarguably changing society, social practices are also impacting on the climate. Nature and culture are deeply entangled, and researchers must examine how each is shaping the other. But they are largely failing to do so.” Hulme (2011, p. 177).
Climate drives the water cycle. Current climate trends, largely driven by human actions, are changing precipitation patterns, rates of evapotranspiration, intensity of rainfall, extreme weather events, and feedbacks within the climate system. As a result, climate change is increasing the frequency and intensity of drought (and floods), while driving reverberating consequences throughout the biophysical and social systems (Hulme, 2011; Jiménez Cisneros et al., 2014; IPCC, 2021). The intensity of climate change related impacts is then further exacerbated by social system drivers (e.g., changes in land use; water exports; over extraction of water resources).
While sociohydrological research is frequently framed within, and justified by, the context of changing climate trends and resulting hydro-meteorological extremes, existing literature largely fails to explicitly account for climate variables because of the complexity this would add to analysis [see Kumar et al. (2020)9 and Elshafei et al. (2014)].10 In the case of small-scale MAR, the complexity of accounting for the climate system is attenuated by small-scale MAR being a bottom-up intervention that can be considered a community-based adaptation strategy (see Blöschl et al., 2013; Basel et al., 2020b). Community-based adaptations are intended to increase the capacity of the system to resist a range of possible hazards, without the functionality of the system fundamentally changing. Endogenizing the climate system into the sociohydrological framework does not then require incorporating precise climate models. Climate trends (e.g., intensity of precipitation events, distribution of precipitation throughout the year, heat waves, and high temperature averages) are key limiting and determining factors in the quantity of water recharged and thresholds that will lead to flooding and drought. Regional long-term trends and extremes must be incorporated into the design of interventions, if interventions are to be effective within the extreme ranges of possible climate conditions (e.g., extreme rainfall events and how much water structures should be able to retain/resist; or evaporation trends and scarce rainfall that prevent structures from capturing sufficient water to raise water tables). Interventions may be ineffectual if they are designed for the current state and function of the biophysical and social system, without accounting for how both of these systems may be altered by changing climatic conditions, extreme events, and social interventions.
As a community-based adaptation strategy and Nature-based Solution, small-scale MAR interventions specifically increase the functionality of both the biophysical and social systems across potential climate extremes (i.e., reducing drought, flooding, soil erosion, and preserving ecosystem and hydrological function). For example, surface water runoff that has been redirected to recharge the aquifer may also reduce local evaporation rates, compounding the positive long-term effect on local water balances over time (Steenbergen et al., 2010). When small-scale MAR is implemented through collective action, the same collective action to implement small-scale MAR (discussed below) may also strengthen social systems, including identity, knowledge and social cohesion, which may further drive collective action and implementation of interventions (Basel et al., 2020a).
Climate trends and extremes within the sociohydrological system must further be contextualized across both temporal and spatial scales. At the temporal scale, the interplay between fast and slow variables may create both positive and negative system feedback processes. For example, industry, policy, and the cumulative effect of personal decisions determine macro-scale climate trends. There is a significant lag time, however, between emissions (or emissions reductions) and climate impacts. The effects of policy and individual behavior on emissions are therefore temporarily disconnected from the experience of subsequent climate-related impacts on both the biophysical (e.g., hydrological and ecosystem function) and social systems (e.g., increased risk due to increased probability of hazard occurrence). This disconnection may result in a lack of concentrated action to mitigate greenhouse gas emissions. Contrarily, a sudden climate-related disaster event may also spur a population to implement small-scale MAR interventions, directly impacting the biophysical subsystem.
The interaction between spatial scales also has implications. Higher groundwater levels may affect the local and regional climate, creating microclimates that alter ambient humidity, air temperatures, and local precipitation (Keune et al., 2016). Such shifts may make the local biophysical system more able to withstand macro-scale climatic extremes, and may even mitigate (to some extent) the local experience of drought. The implementation of small-scale MAR may therefore support positive feedback processes that moderate local climates. Scale is also important for the potential impact of social system variables on climate (and biophysical systems). The sum total of political and personal choices determines the total possible impact of the social system on the local climate system.
Drawing on these illustrative scenarios, climate trends and their relationship with economic, socio-political, and biophysical factors adds yet more complexity to an already dynamic sociohydrological system. However, assessing the potential effectiveness of small-scale MAR interventions and their implementation, depends on explicitly accounting for the climate subsystem. This requires not only using historical records for planning, but conducting climate vulnerability assessments, including how the climate regime is likely to shift, and incorporating temperature/precipitation trends, extremes, and seasonality into small-scale MAR designs.11
The social subsystem: Key variables and drivers
People and power work in tandem with hydrogeology to direct the flow of water. The social system causes cascading and dynamic effects throughout the biophysical and climate systems where small-scale MAR is implemented. Regardless, as the title of Savelli et al. (2021) “Don't Blame the Rain” eloquently illustrates, the experience of drought is often blamed on erratic weather and climate change. While climate trends are increasing the frequency and intensity of drought (IPCC, 2021), blaming the rain allows us to ignore that the modern experience of drought is often a result of inequitable and/or inefficient distribution of water (Savelli et al., 2021). Using this understanding as a point of departure, we conceptualize the social aspects of the sociohydrological system, including factors that may enable or inhibit self-organization and collective action for small-scale MAR. We aim to explore this issue by addressing the following interrelated questions: Do higher scales of governance enable or undermine local action? What variables may increase the likelihood that people will act?
Do higher scales of governance enable or undermine local action?
Do people have the power to act? As a bottom-up solution, implementing small-scale MAR suggests the need for self-organization and collective action. These literatures are highly developed and will be further explored below. Action does not happen in a vacuum, but within a setting of “contextual factors and referential environment” (Vos et al., 2020, p. 5). The flow of water and the legal right to implement small-scale MAR are both dependent on economics, politics, and resource competition: local actors are subject to an array of external factors that may support or impede small-scale MAR initiatives and success. Vos et al. (2020, p. 5) suggest that key components of this broader setting include strength and involvement of the state bureaucracy, academic and epistemological environment, strength of civil society and room to maneuver, techno-physical and agro-ecological environment, and the functioning of capitalist markets in the water sector.
Central to understanding small-scale MAR within this context is the nature of water as a common-pool resource (CPR) “for which exclusion of users is difficult to achieve and for which joint use reduces the availability of benefits derived from the resource for others” (Steins and Edwards, 1999, p. 539). Water is vied for and contested. Water is also the lynchpin in supporting growing populations, urbanization, industrialization, and neoliberal development. The needs of numerous stakeholders, with various levels of influence, overlap creating complex interactions and competition (Hoogesteger and Wester, 2015). The interaction between stakeholders determines water governance, which simultaneously occurs at multiple levels through policies and measures, while water flows into and out of local systems. These policies and measures are a key driver affecting biophysical, climate, and social subsystems. While the role of governance and decision making is often highlighted within sociohydrological models (as an example see Penny and Goddard, 2018), the complexity of these interactions is arguably underestimated. Water governance and existing sociohydrological models/frameworks are largely based on neoliberal socio-economic productive models that may not be applicable to the cultural values of populations in question and may exacerbate injustice (Zwarteveen and Boelens, 2014; Vos and Boelens, 2018). Additionally, while tension between state and local governance may be circumstantial, Molle et al. (2009, p. 340) highlight that “Centralized water bureaucracies are also threatened by decentralization of power to the regional or local levels…” The bottom-up nature of small-scale MAR initiatives may make recharge undesirable by those in power, as it may be perceived as threatening their control over resource management. Local action to implement small-scale MAR (and benefit from increased water availability) may be prevented, limited, or regulated by state or federal legal frameworks (Cruz-Ayala and Megdal, 2020). Alternatively, downstream populations might believe that upstream small-scale MAR is stealing their water, further resulting in policies and regulations that prevent small-scale MAR; meanwhile, MAR infrastructure might actually be benefiting downstream populations with flood/drought mitigation and increased groundwater levels.
Conversely, the top-down involvement of government authorities in supporting local small-scale MAR initiatives may even undermine processes of self-organization and collective action. Top-down policy making tends to ignore the complexity and diversity of local water management systems (Boelens, 2009), potentially undermining the functionality of local systems. State recognition of local initiatives might paradoxically delegitimize local systems as effective square pegs of local management do not fit in the round whole of state discourse. Recognition and “equality” may translate into a process of “disciplining” of diverse systems of water management; through being recognized and accepted, diverse approaches to managing water must adapt to the system that has endogenized them, potentially undermining the prior effectiveness of the water management system (Boelens, 2009).
Government interventions in local initiatives can unknowingly contradict what the implementing population construes as equitable benefits (e.g., distributing water to people who did not do the work) potentially creating conflict and undermining the system of cooperation and collective action that made the intervention possible (Hoogesteger, 2015; Basel et al., 2020b; Dupuits et al., 2020).
“Well-intended” state and market interventions may push local production systems toward overexploitation of resources (i.e., water) that results in reduced resource access by underserved populations, resulting in further resource exploitation by these populations, increased environmental degradation, and reduced ecosystem services, impacting livelihoods and further exacerbating poverty (Robbins, 2012; Hoogesteger and Wester, 2017). Both the biophysical and social systems are impacted. This trend may be especially pronounced when state governments privatize previously collective land/resource rights or impose external governance systems on local actors (Trawick, 2003; Boelens et al., 2015; Hall, 2015). Among state and market interventions is a growing national and international focus on efficiency. State/non-profit initiatives to address water crises are often based on these efficiency discourses, which direct the flow of water toward the most efficient users and those that deliver the greatest economic benefit to the state (e.g., large-scale export producers or mining). The focus on using water “efficiently” effectively diverts water away from small-scale users that could be the most likely to implement small-scale MAR, while simultaneously disempowering local management, rights, and leaving local populations dispossessed of agricultural lands, livelihoods, and cultural identity (Mehta et al., 2012; Dell'Angelo et al., 2018; Vos and Boelens, 2018). Ironically, more “efficient” water users may effectively drain limited local water resources and drive local water crises with virtual water exports (Vos and Boelens, 2018).
These governance-related factors demonstrate paradoxes that may further drive community vulnerability to drought, while decreasing the likelihood of collective action and the implementation of small-scale MAR. Larger-scale governance can help avoid such paradoxes and enable local implementation of small-scale MAR (while increasing cooperation, collective action, and water justice) by (1) seeking to understand diversity, including recognizing and allowing for the different ways people understand and manage water; (2) taking a full inventory of the externalities of efficiency discourses; (3) external governance structures or policy makers taking the role of facilitator instead of expert; and (4) building on organizational structures that already exist (Hoogesteger and Verzijl, 2015).
What variables may increase the likelihood that people will act?
“…impacts and perceptions of natural hazards influence sociotechnical vulnerabilities, governance, and institutions, while at the same time social behavior, technical measures, and policy interventions alter the frequency, magnitude, and spatial distribution of natural hazards. Reciprocal effects at the local scale are also influenced by global drivers. Climate and environmental change can alter the frequency and severity of extreme weather events, while socioeconomic trends (including population growth, urbanization, and interdependent infrastructures) can increase exposure to natural hazards.” Di Baldassarre et al. (2018, p. 307).
Even if people have the power to act, will they? “Change (whether drastic or incremental) acts as a catalyst to response” (Elshafei et al., 2014, p. 2,143; Roobavannan et al., 2017). Populations act when conditions (e.g., drought) become sufficiently extreme, resulting in direct experience of the hazard, a shared memory of the experience, and/or perceived risk (Di Baldassarre et al., 2013; Viglione et al., 2014; Hale et al., 2015; Kuil et al., 2016; Penny and Goddard, 2018). While experiencing a hazard directly drives people to action to safeguard themselves, collective memory draws on local and social knowledge of previous experience to recognize biophysical thresholds and respond to emerging conditions (Kuil et al., 2016). Such collective memory is strongest immediately after experiencing the hazard and erodes over time, only to be renewed when the event is again experienced (Di Baldassarre et al., 2013; Viglione et al., 2014; Kuil et al., 2016). Collective memory increases social pressure to cooperate (Penny and Goddard, 2018), while eroded memory reduces the impetus for collective action. Social pressure may also be self-reinforcing: a behavior (e.g., action to address drought) is more likely to become widely adopted once 10% of the population has adopted the behavior (Elshafei et al., 2014). Perception of risk is rooted in the local context and individual/group understanding of the surrounding world (determined by cultural, agroecological, economic, political, and social factors) (Bebbington, 1996; Viglione et al., 2014; Hale et al., 2015; Penny and Goddard, 2018). Ostrom (2000, 2009) refer to this as Mental Models and Knowledge of the Social-ecological System, Haugaard (2012) as the sum of practical and discursive consciousness, Zwarteveen and Boelens (2014) as situated knowledge, and Feindt and Oels (2005) as discourse. Social connectivity and spaces for dialogue facilitate the development of shared understanding, including perception of risk, that can drive action (Benford and Snow, 2000; Ostrom, 2009; Penny and Goddard, 2018). Such understandings determine what is considered reasonable, possible, desirable, and important - and consequently influence how a population acts (and knows how to act) to address a problem (Kuil et al., 2016). Understandings may actually perpetuate inaction or result in actions that appear paradoxical (Bebbington, 1996; Cleaver, 2018). Factors that determine perception of risk are not static but constantly in flux as people adapt to changing conditions and contexts (Bebbington, 1996). When concerning small-scale MAR, factors of experience, memory, and perceived risk apply across governance scales, influencing policies and measures, individual behavior, and collective action (Elshafei et al., 2014; Hale et al., 2015; Di Baldassarre et al., 2019; Basel et al., 2020a; Kumar et al., 2020).
These factors interplay with social variables for enabling self-organization for small-scale MAR as a strategy for community-based adaptation highlighted by Basel et al. (2020b) and drawn from Ostrom (2009). Bottom-up and collective-action for small-scale MAR may be more likely among populations that have generational and situated knowledge with groundwater, established spaces or mechanisms for engaging in dialogue and debate, the opportunity for individuals to directly participate in building small-scale MAR infrastructure (allowing them to learn first-hand about the purpose and value of such activities), and access to technical and practical information about interventions (Basel et al., 2020a). Referred to by Ostrom (2009) as collective-choice rules, people are more likely to act if they are able to directly participate in resource governance, have political representation, and have access to information, tools, and funding for implementing small-scale MAR interventions (Penny and Goddard, 2018; Basel et al., 2020b). Self-organization for collective action is further enabled or inhibited by the number of water users. Users must be well-matched for the carrying capacity of the biophysical system (Kuil et al., 2016), having a large enough population both to contribute to organized/voluntary labor for building infrastructure, and to consolidate into a network that can access government support (Basel et al., 2020b). Simultaneously a population must be small enough to be able to engage directly in decision-making (see collective-choice rules) and feel that their participation makes a difference in the outcome (Basel et al., 2020b). As such, populations may also be unified by a shared challenge and believing that small-scale MAR will be effective (and is worth the effort invested) (Basel et al., 2020b). The lag time between intervention and benefit (resulting from the biophysical system described above) might reduce the will and social pressure to act. Belief in potential success, however, may be driven by implementing small-scale MAR in an iterative process that aims for short-term wins (Basel et al., 2020a).
When small-scale MAR is not already part of existing mental models and discourse, strong leadership, paired with an openness to experimentation and learning, supports action (Penny and Goddard, 2018; Basel et al., 2020b). Norms and social capital translate into connective processes that allow for organizational structures, social interaction and cooperation, shared awareness, social/political cohesion, and community participation (Elshafei et al., 2014; Hale et al., 2015; Penny and Goddard, 2018; Basel et al., 2020b). Norms and social capital based on shared values, reciprocity between people and with nature, and shared work, may further support implementation of small-scale MAR (Basel et al., 2020b).
Financial drivers within this system may result in paradoxes. For example, if livelihoods depend on groundwater-irrigated agriculture and demand for agricultural production increases, the area of land developed for agriculture may expand (Roobavannan et al., 2017). As a result, water use may increase, reducing local groundwater levels and driving the implementation of MAR. In turn, MAR may then support further increased agricultural development and production, resulting in further increased groundwater use. When small-scale MAR is driven strictly by financial drivers without being tempered with other considerations, interventions could paradoxically increase local vulnerability to drought (Basel et al., 2020b). Furthermore, Elshafei et al. (2014) link low per person gross domestic product (GDP) to reduced resilience and increased sensitivity; the experience in Oaxaca Basel et al. (2020b) and the arguments above suggest this correlation may be biased to neoliberal economic models. While such models certainly apply in certain socio-economic and cultural contexts, the implementation of small-scale MAR and resulting social and ecological benefits may result from water being important for social/cultural reasons instead of purely economic ones (Basel et al., 2020b).
Discussion and conclusions
In this paper we have conceptualized the sociohydrological system in which small-scale MAR interventions are implemented to increase groundwater availability in small or upper catchments. To do so, we have drawn on, consolidated, and advanced existing conceptualizations of the sociohydrological system. Sociohydrology addresses how humans affect hydrological regimes and how, in turn, water resources and water-related hazards influence human behavior including water management. The approach is solutions-oriented, seeking to understand these human-water interactions to identify effective responses to water resource challenges and hazards. Responses to water related problems, such as small-scale MAR, are not neutral phenomenon but are implemented by people: factors including who has decision-making power, who benefits, who loses, and the discourses that drive these decisions, influence how, when, by whom, and with what results small-scale MAR is implemented. In the conceptualization of the sociohydrological system presented in here, we have brought to light complex interplay between biophysical, climate, and social systems, accounting for social institutions, practices, and norms to make this research power-sensitive.
In the interplay between biophysical, climate, and social systems, water moves through the biophysical system guided by geomorphology, ecological processes, and human interventions. The climate system cycles water into and out of local landscapes. People and power within the social system guide the flow of water and are changing the climate system. Within this dynamic context, fast and slow changing factors intersect with spatial and governance scales. The dynamics among and between these systems can result in positive or negative feedback processes or paradoxes (unintended consequences), decreasing or increasing the local experience of drought. By conceptualizing the system and exploring the interplay between these three systems, we can identify important considerations, opportunities for engagement and learning, and possible paradoxes.
The structure and state of the biophysical system determine ecosystem function, including hydrological flows. Ecosystem functions benefit human populations, including through the provision of water resources, food, livelihoods, and the regulation of floods and drought. The relationship between people and the surrounding landscape also supports and influences place-based knowledge, social dynamics, and identity that enable or inhibit the implementation of small-scale MAR. The biophysical system is not static or only subject to the laws of the natural sciences, but is plastic: both climatic processes and the normative goals and political choices of the people managing and interacting with the biophysical system help determine the state of the landscape and resulting ecosystem function.
Interplay between biophysical, climate, and social systems is unfolding within the context of increasing hydroclimatic extremes, including droughts and floods, related to climate change. Historical climate data are no longer representative for understanding system dynamics and planning small-scale MAR interventions. Climate molds the biophysical system, which in turn helps regulate both local climates and the global climate system. Climate also indisputably affects society, while people are driving changing climate trends across both global and microclimate scales. As a community-based adaptation strategy and Nature-based Solution, small-scale MAR interventions specifically increase the ability of both the biophysical and social systems to weather climate extremes (e.g., mitigating drought and flooding, preserving ecosystem/hydrological function, and reinforcing social factors that enable collective action).
The profound influence of climate on the interplay between biophysical, climate, and social systems indicates that assessing the potential effectiveness of small-scale MAR interventions and their implementation depends on explicitly accounting for the climate system. Designing small-scale MAR in the current context of climate change requires not only using historical records for planning, but conducting climate vulnerability assessments, including how the climate regime is likely to shift including incorporating temperature/precipitation trends, extremes, and shifting seasonality.
Meanwhile, society often blames drought on climate change and erratic weather. Climate trends are increasing and intensifying drought events. The social system also informs the state and functionality of both biophysical and climate systems in a myriad of ways. The social system moreover drives the experience of drought through inequitable and/or inefficient water management. The implementation of small-scale MAR, occurs within this broader socio-political context in which water is a contested resource. The complexity of governance and decision-making is routinely under recognized, while having ricocheting effects not only on the implementation of small-scale MAR interventions and water use, but throughout the entire sociohydrological system described in this paper. Higher-level governance may enable or inhibit action to address drought (e.g., small-scale MAR). Even when the state aims to support action, state involvement may inadvertently deteriorate or delegitimize the social capital that support the implementation of MAR, or push the sociohydrological system toward overexploitation of water resources, weakening the biophysical system, fueling climate change, and exacerbating poverty.
People will be more prone to implement small-scale MAR when biophysical, climatic conditions, and inequitable distribution of water become sufficiently extreme, resulting in the experience, recent and collective memory, or perceived risk of drought. These factors combine with social pressure to act; cultural, agroecological, socio-economic, and political factors; framing and discourse about the problem and perceived potential for action; and the principles for self-organization for small-scale MAR (see Basel et al., 2020b). While livelihoods and economic trends may drive implementation, implementation of small-scale MAR may actually be driven by water being important for social/cultural reasons linked to the landscape, instead of financial ones.
Despite the promise of small-scale MAR, applying a critical lens to understanding the interplay between biophysical, climate, and social systems and resulting feedback processes and paradoxes, allows us to not overestimate or oversimplify small-scale MAR as a bottom-up solution and community-based adaptation strategy. Within the parameters of climate trends, small-scale MAR may serve to increase the functionality of ecosystems and reduce the impact of climate extremes, while protecting livelihoods and supporting society. In an additional positive feedback loop, small-scale MAR may both reduce the biophysical likelihood of experiencing drought (and mitigate flooding) while simultaneously increasing the social ability to further cope with or avoid drought. Paradoxes and negative feedback processes, however, must be avoided.
Specific factors, and how such factors interplay, will be different in each context where small-scale MAR is implemented. Conceptualizing the sociohydrological system in which small-scale MAR is implemented, including explicitly accounting for climate trends and using a power-sensitive approach, allows us to recognize and account for the complexity of this system. Important considerations, positive and negative feedback processes, and possible paradoxes can be identified. Applying these understandings to case studies offers a rich opportunity for future research.
Data availability statement
The original contributions presented in the study are included in the article/supplementary material, further inquiries can be directed to the corresponding author/s.
Author contributions
BB and JH conceptualized the paper and the methodology. BB performed the literature review, curated the data, conducted the formal analysis, and wrote the first draft of the manuscript. BB, JH, and PH reviewed and edited the first draft before submission and throughout the peer-review process.
Acknowledgments
We would like to thank the Delft International Conference on Sociohydrology and Frontiers for the opportunity to present our preliminary research at the conference and for supporting the open-access publication of this manuscript. We would like to further thank our colleagues working with sociohydrology for their time and for many valuable discussions which contributed to the development and refinement of the conceptualization presented in this paper. Finally, we would like to thank the reviewers for their time and insightful comments that helped us to improve this manuscript.
Conflict of interest
Author BB is the founder of Ecothropic and co-founder of Ecothropic México A.C.
The remaining authors declare that the research was conducted in the absence of any commercial or financial relationships that could be construed as a potential conflict of interest.
Publisher's note
All claims expressed in this article are solely those of the authors and do not necessarily represent those of their affiliated organizations, or those of the publisher, the editors and the reviewers. Any product that may be evaluated in this article, or claim that may be made by its manufacturer, is not guaranteed or endorsed by the publisher.
Footnotes
1 While extensive previous research on human and water systems exists (see Results section for further detail), the sociohydrological discipline for exploring system interactions was proposed in 2014 by Sivapalan et al. (2014).
2 The nine papers, dating 2014–2021, which were chosen for in-depth comparison and analysis, are as follows: (1) Elshafei et al. (2014); (2) Hale et al. (2015); (3) Kuil et al. (2016); (4) Kumar et al. (2020); (5) Penny and Goddard (2018); (6) Roobavannan et al. (2017); (7) Savelli et al. (2021); (8) Sivapalan et al. (2014); (9) Vanelli and Kobiyama (2021).
3 Please see Di Baldassarre et al. (2019, p.6331) for the characteristics and implications of these sociohydrological phenomena and related sub-phenomena.
4 Referred to as Environmental Change by Di Baldassarre et al. (2018).
5 i.e., the function of ecosystems in determining water availability and hydrologic extremes.
6 The term ecosystem services shows a linguistic bias toward being human-centric (humans benefited by ecosystems). While, Mao et al. (2017) present a critique of standard approaches to ecosystem services by aiming to address human-water coupled systems, the focus arguably maintains this bias without recognizing other worldviews which may be oriented toward a less extractivist and more reciprocity-based and integrated perspective of human/ecosystem relationships which may be at play when small-scale recharge is implemented (see Basel et al., 2020b).
7 From: https://vu.nl/en/about-vu/more-about/perfectstorm-storylines-of-future-extremes-ivm. Accessed July 9, 2022.
8 See Dell'Angelo et al. (2018) and Vos and Boelens (2018).
9 Kumar et al. (2020, p. 3) propose, “Researchers fail to attribute risk associated with climate stress and variability since the features of the climatic system are complex and interconnected with other economic and social phenomena.”
10 Elshafei et al. (2014, p. 2,142) write: “Finally, the specific vulnerability and responsiveness that the hydrological coupled system displays in regard to climate change (Ribeiro Neto et al., 2014) presents an additional challenge.”
11 Illustrating the importance of the temporal shift in precipitation: Even while the same amount of rain may fall throughout the year, it may increasingly fall in heavy rainfall events instead of being evening distributed (as per the historical record). As a result, rainfall concentrates as surface runoff, flood risk increases, and natural recharge is reduced, resulting in hydrological drought even at the same annual rate of precipitation.
References
Basel, B., Goby, G., and Johnson, J. (2020a). Community-based adaptation to climate change in villages of Western Province, Solomon Islands. Mar. Poll. Bulletin. 156, 111266. doi: 10.1016/j.marpolbul.2020.111266
Basel, B., Hernández Quiroz, N., Velasco Herrera, R., Santiago Alonso, C., and Hoogesteger, J. (2020b). Bee mietii rak rkabni nis (the people know how to seed water): a Zapotec experience in adapting to water scarcity and drought. Climate Dev. 13, 792–806. doi: 10.1080/17565529.2020.1855100
Bebbington, A. (1996). “Movements, modernizations, and markets: indigenous organizations and agrarian strategies in Ecuador,” in Liberation Ecologies. Environment, Development, Social Movements, eds Peet, R., and Watts, M., (London; New York, NY: Routledge), 86–109.
Benford, R. D., and Snow, D. A. (2000). Framing processes and social movements: an overview and assessment. Ann. Rev. Sociol. 26, 611–639. doi: 10.1146/annurev.soc.26.1.611
Berkes, F., and Armitage, D. (2010). Co-management institutions, knowledge, and learning: adapting to change in the Arctic. Études/Inuit/Studies 34, 109–131. doi: 10.7202/045407ar
Biggs, R., Schlüter, M., Biggs, D., Bohensky, E. L., BurnSilver, S., Cundill, G., et al. (2012). Toward principles for enhancing the resilience of Ecosystem Services. Ann. Rev. Environ. Resour. 37, 421–448. doi: 10.1146/annurev-environ-051211-123836
Blöschl, G., Viglione, A., and Montanari, A. (2013). Emerging Approaches to Hydrological Risk Management in a Changing World. Climate Vulnerability: Understanding and Addressing Threats to Essential Resources. (Elsevier Inc.; Academic Press), 3–10. ISBN: 9780123847034. doi: 10.1016/B978-0-12-384703-4.00505-0
Boelens, R. (2009). The politics of disciplining water rights'. Dev. Change 40, 307–331. doi: 10.1111/j.1467-7660.2009.01516.x
Boelens, R. (2014). Cultural politics and the hydrosocial cycle: water, power and identity in the Andean highlands. Geoforum 57, 234–247. doi: 10.1016/j.geoforum.2013.02.008
Boelens, R., Hoogesteger, J., and Baud, M. (2015). Water reform governmentality in Ecuador: neoliberalism, centralization, and the restraining of polycentric authority and community rule-making. Geoforum 64, 281–291. doi: 10.1016/j.geoforum.2013.07.005
Boelens, R., Hoogesteger, J., Swyngedouw, E., Vos, J., and Wester, P. (2016). Hydrosocial territories: a political ecology perspective. Water Int. 41, 1–14. doi: 10.1080/02508060.2016.1134898
Bohensky, E. L., Evans, L. S., Anderies, J. M., Biggs, D., and Fabricius, C. (2015). Principle 4—Foster Complex Adaptive Systems Thinking. Principles for Building Resilience: Sustaining Ecosystem Services in Social-Ecological Systems. Cambridge: Cambridge University Press, 142–173. doi: 10.1017/CBO9781316014240.007
Boillat, S., and Berkes, F. (2013). Perception and interpretation of climate change among Quechua farmers of Bolivia: indigenous knowledge as a resource for adaptive capacity. Ecol. Soc. 18, 421. doi: 10.5751/ES-05894-180421
Clark, W. C., and Harley, A. G. (2020). Sustainability science: toward a synthesis. Ann. Rev. Environ. Resour. 45, 331–386. doi: 10.1146/annurev-environ-012420-043621
Cleaver, F. (2018). “Everyday water injustice and the politics of accommodation,” in Water Justice, eds Boelens, R., Perreault, T., and Vos, J., (Cambridge: Cambridge University Press), 246–258. doi: 10.1017/9781316831847.016
Cohen-Shacham, E., Walters, G., Janzen, C., and Maginnis, S. (2016). Nature-Based Solutions to Address Global Societal Challenges. Gland: IUCN, xiii + 97. doi: 10.2305/IUCN.CH.2016.13.en
Cruz-Ayala, M. B., and Megdal, S. B. (2020). An overview of managed aquifer recharge in Mexico and its legal framework. Water 12, 474. doi: 10.3390/w12020474
Dell'Angelo, J., Rulli, M. C., and D'Odorico, P. (2018). The global water grabbing syndrome. Ecol. Econ. 143, 276–285. doi: 10.1016/j.ecolecon.2017.06.033
Di Baldassarre, G., Nohrstedt, D. J., Burchardt, S., Albin, C., and Bondesson, S. (2018). An integrative research framework to unravel the interplay of natural hazards and vulnerabilities. Earth's Fut. 6, 305–310. doi: 10.1002/2017EF000764
Di Baldassarre, G., Sivapalan, M., Rusca, M., Cudennec, C., Garcia, M., Kreibich, H., et al. (2019). Sociohydrology: scientific challenges in addressing the sustainable development goals. Water Resour. Res. 55, 6327–6355. doi: 10.1029/2018WR023901
Di Baldassarre, G., Viglione, A., Carr, G., Kuil, L., Salinas, J. L., and Blöschl, G. (2013). Socio-hydrology: conceptualising human-flood interactions. Hydrol. Earth Syst. Sci. 17, 3295–3303. doi: 10.5194/hess-17-3295-2013
Di Baldassarre, G., Viglione, A., Carr, G., Kuil, L., Yan, K., Brandimarte, L., et al. (2015). Debates—perspectives on socio-hydrology: capturing feedbacks between physical and social processes. Water Resour. Res. 51, 4770–4781. doi: 10.1002/2014WR016416
Dillon, P., Stuyfzand, P., Grischek, T., Lluria, M., Pyne, R. D. G., Jain, R. C., et al. (2019). Sixty years of global progress in managed aquifer recharge. Hydrogeol. J. 27, 1–30. doi: 10.1007/s10040-018-1841-z
Dupuits, E., Baud, M., Boelens, R., de Castro, F., and Hogenboom, B. (2020). Scaling up but losing out? Water commons' dilemmas between transnational movements and grassroots struggles in Latin America. Ecol. Econ. 172, 106625. doi: 10.1016/j.ecolecon.2020.106625
Elshafei, Y., Sivapalan, M., Tonts, M., and Hipsey, M. R. (2014). A prototype framework for models of socio-hydrology: identification of key feedback loops and parameterisation approach. Hydrol. Earth Syst. Sci. 18, 2141–2166. doi: 10.5194/hess-18-2141-2014
Feindt, P. H., and Oels, A. (2005). Does discourse matter? Discourse analysis in environmental policy making. J. Environ. Pol. Plan. 7, 161–173. doi: 10.1080/15239080500339638
Gleick, P., and Palaniappan, M. (2010). Peak water limits to freshwater withdrawal and use. Proc. Natl. Acad. Sci. U.S.A. 107, 11155–11162. doi: 10.1073/pnas.1004812107
Guyennon, N., Salerno, F., Portoghese, I., and Romano, E. (2017). Climate change adaptation in a Mediterranean semi-arid catchment: testing managed aquifer recharge and increased surface reservoir capacity. Water 9, 1–18. doi: 10.3390/w9090689
Hale, R. L., Armstrong, A., Baker, M. A., Bedingfield, S., Betts, D., Buahin, C., et al. (2015). iSAW: integrating structure, actors, and water to study socio-hydro-ecological systems. Earth's Fut. 3, 2014EF000295. doi: 10.1002/2014EF000295
Hall, R. J. (2015). Divide and conquer: privatizing indigenous land ownership as capital accumulation. Stud. Polit. Econ. 96, 23–46. doi: 10.1080/19187033.2015.11674936
Haugaard, M. (2012). Rethinking the four dimensions of power: domination and empowerment. J. Polit. Power 5, 33–54. doi: 10.1080/2158379X.2012.660810
Hommes, L., and Boelens, R. (2017). Urbanizing rural waters: rural-urban water transfers and the reconfiguration of hydrosocial territories in Lima. Polit. Geogr. 57, 71–80. doi: 10.1016/j.polgeo.2016.12.002
Hommes, L., Boelens, R., Harris, L. M., and Veldwisch, G. J. (2019). Rural–urban water struggles: urbanizing hydrosocial territories and evolving connections, discourses and identities. Water Int. 44, 81–94. doi: 10.1080/02508060.2019.1583311
Hoogesteger, J. (2015). Normative structures, collaboration and conflict in irrigation; a case study of the Pillaro North Canal Irrigation System, Ecuadorian Highlands. Int. J. Commons. 9, 398–415. doi: 10.18352/ijc.521
Hoogesteger, J. (2022). Regulating agricultural groundwater use in arid and semi-arid regions of the Global South: challenges and socio-environmental impacts. Curr. Opin. Environ. Sci. Health 2022, 100341. doi: 10.1016/j.coesh.2022.100341
Hoogesteger, J., and Verzijl, A. (2015). Grassroots scalar politics: insights from peasant water struggles in the Ecuadorian and Peruvian Andes. Geoforum 62, 13–23. doi: 10.1016/j.geoforum.2015.03.013
Hoogesteger, J., and Wester, P. (2015). Intensive groundwater use and (in) equity: Processes and governance challenges. Environ. Sci. Policy 51, 117–124. doi: 10.1016/j.envsci.2015.04.004
Hoogesteger, J., and Wester, P. (2017). Regulating groundwater use: The challenges of policy implementation in Guanajuato, Central Mexico. Environ. Sci. Policy 77, 107–113. doi: 10.1016/j.envsci.2017.08.002
International Groundwater Assessment Centre (2020). Managed Aquifer Recharge (MAR). Available online at: https://www.un-igrac.org/areas-expertise/managed-aquifer-recharge-mar (accessed June 9 2020).
IPCC. (2021). “Summary for policymakers,” in Climate Change 2021: The Physical Science Basis. Contribution of Working Group I to the Sixth Assessment Report of the Intergovernmental Panel on Climate Change, eds Masson-Delmotte, V., Zhai, P., Pirani, A., Connors, S. L., Péan, C., Berger, S., Caud, N., Chen, Y., Goldfarb, L., Gomis, M. I., Huang, M., Leitzell, K., Lonnoy, E., Matthews, J. B. R., Maycock, T. K., Waterfield, T., Yelekçi, O., Yu, R., and Zhou, B., (Cambridge University Press).
Jiménez Cisneros, B. E., Oki, T., Arnell, N. W., Benito, G., Cogley, J. G., Döll, P., et al. (2014). “Freshwater resources,” in Climate Change 2014: Impacts, Adaptation, and Vulnerability. Part A: Global and Sectoral Aspects. Contribution of Working Group II to the Fifth Assessment Report of the Intergovernmental Panel on Climate Change, eds Field, C. B., Barros, V. R., Dokken, D. J., Mach, K. J., Mastrandrea, M. D., Bilir, T. E., (Cambridge: Cambridge University Press), 229–269.
Kallis, G. (2010). Coevolution in water resource development: The vicious cycle of water supply and demand in Athens, Greece. Ecol. Econ. 69, 796–809. doi: 10.1016/j.ecolecon.2008.07.025
Kandasamy, J., Sounthararajah, D., Sivabalan, P., Chanan, A., Vigneswaran, S., and Sivapalan, M. (2014). Socio-hydrologic drivers of the pendulum swing between agriculture development and environmental health: A case study from Murrumbidgee river basin, Australia. Hydrol. Earth Syst. Sci. 18, 1027–1041. doi: 10.5194/hess-18-1027-2014
Kates, R. W., Colten, C. E., Laska, S., and Leatherman, S. P. (2006). Reconstruction of New Orleans after Hurricane Katrina: A research perspective. Proc. Natl. Acad. Sci. U.S.A. 103, 14653–14660. doi: 10.1073/pnas.0605726103
Kennedy, D. (1995). Water supply and use in the southern Hauran, Jordan. J. Field Archaeol. 22, 275–290. doi: 10.1179/009346995791974198
Keune, J., Gasper, F., Goergen, K., Hense, A., Shrestha, P., Sulis, M., et al. (2016). Studying the influence of groundwater representations on land surface-atmosphere feedbacks during the European heat wave in 2003. J. Geophys. Res. Atmos. 121, 2016JD025426. doi: 10.1002/2016JD025426
Kuil, L., Carr, G., Viglione, A., Prskawetz, A., and Blöschl, G. (2016). Conceptualizing socio-hydrological drought processes: the case of the Maya collapse. Water Resour. Res. 52, 6222–6242. doi: 10.1002/2015WR018298
Kumar, P., Avtar, R., Dasgupta, R., Johnson, B. A., Mukherjee, A., Ahsan, M. N., et al. (2020). Socio-hydrology: a key approach for adaptation to water scarcity and achieving human wellbeing in large riverine islands. Progr. Disast. Sci. 8, 100134. doi: 10.1016/j.pdisas.2020.100134
Lasage, R., Aerts, J. C. J. H., Verburg, P. H., and Sileshi, A. S. (2015). The role of small scale sand dams in securing water supply under climate change in Ethiopia. Mitig. Adapt. Strateg. Glob. Change. 20, 317–339. doi: 10.1007/s11027-013-9493-8
Lasage, R., and Verburg, P. H. (2015). Evaluation of small scale water harvesting techniques for semi-arid environments. J. Arid Environ. 118, 48–57. doi: 10.1016/j.jaridenv.2015.02.019
Linton, J., and Budds, J. (2014). The hydrosocial cycle: defining and mobilizing a relational-dialectical approach to water. Geoforum 57, 170–180. doi: 10.1016/j.geoforum.2013.10.008
Madani, K., and Shafiee-Jood, M. (2020). Socio-hydrology: a new understanding to unite or a new science to divide? Water 12, 1941. doi: 10.3390/w12071941
Malik, R., Giordano, M., and Sharma, V. (2014). Examining farm-level perceptions, costs, and benefits of small water harvesting structures in Dewas, Madhya Pradesh. Agri. Water Manag. 131, 204–211. doi: 10.1016/j.agwat.2013.07.002
Mao, F., Clark, J., Karpouzoglou, T., Dewulf, A., Buytaert, W., and Hannah, D. (2017). HESS Opinions: a conceptual framework for assessing socio-hydrological resilience under change. Hydrol. Earth Syst. Sci. 21, 3655–3670. doi: 10.5194/hess-21-3655-2017
Mehta, L., Veldwisch, G. J., and Franco, J. (2012). Introduction to the Special Issue: water grabbing? Focus on the (re)appropriation of finite water resources. Water Alternativ. 5, 193–207. Available online at: https://www.water-alternatives.org/index.php/tp1-2/1881-vol5/213-issue5-2
Melsen, L. A., Vos, J., and Boelens, R. (2018). What is the role of the model in socio-hydrology? Discussion of “Prediction in a socio-hydrological world”. Hydrolog. Sci. J. 63, 1435–1443. doi: 10.1080/02626667.2018.1499025
Molle, F., and Berkoff, J. (2009). Cities vs. agriculture: a review of intersectoral water re-allocation. Nat. Resour. For. 33, 6–18. doi: 10.1111/j.1477-8947.2009.01204.x
Molle, F., Mollinga, P. P., and Wester, P. (2009). Hydraulic bureaucracies and the hydraulic mission: flows of water, flows of power. Water Alternativ. 2, 328–349.
Molle, F., Wester, P., and Hirsch, P. (2010). River basin closure: processes, implications and responses. Agri. Water Manag. 97, 569–577. doi: 10.1016/j.agwat.2009.01.004
Ochoa-Tocachi, B. F., Bardales, J. D., Antiporta, J., Pérez, K., Acosta, L., Mao, F., et al. (2019). Potential contributions of pre-Inca infiltration infrastructure to Andean water security. Nat. Sustainabil. 2, 584–593. doi: 10.1038/s41893-019-0307-1
Olson, M. (1965). The Logic of Collective Action: Public Goods and the Theory of Groups. Cambridge, MA: Harvard University Press.
Ostrom, E. (1990). Governing the Commons: The Evolution of Institutions for Collective Action (Cambridge, MA: Cambridge University Press), 271. doi: 10.1017/CBO9780511807763
Ostrom, E. (2000). Reformulating the commons. Swiss Polit. Sci. Rev. 6, 29–52. doi: 10.1002/j.1662-6370.2000.tb00285.x
Ostrom, E. (2009). A general framework for analyzing sustainability ofsocial-ecological systems. Science 325, 419–422. doi: 10.1126/science.1172133
Ostrom, E., Janssen, M. A., and Anderies, J. M. (2007). Going beyond panaceas. Proc. Natl. Acad. Sci. U. S. A. 104, 15176–15178. doi: 10.1073/pnas.0701886104
Otieno, V. O., and Anyah, R. O. (2012). Effects of land use changes on climate in the Greater Horn of Africa. Climate Res. 52, 77–95. doi: 10.3354/cr01050
Pande, S., and Sivapalan, M. (2016). Progress in socio-hydrology: a meta-analysis of challenges and opportunities. WIREs Water 4, 1193. doi: 10.1002/wat2.1193
Pandey, D. N., Gupta, A. K., and Anderson, D. M. (2003). Rainwater harvesting as an adaptation to climate change. Curr. Sci. 85, 46–59. Available online at: http://www.jstor.org/stable/24107712
Patel, N. G., and Prabhakar, A. M. (2012). Analysis of rain water harvesting system for artificial recharge of groundwater for Gandhinagar District, Gujarat. Paripex Ind. J. Res. 3, 215–216. doi: 10.15373/22501991/July2014/83
Penny, G., and Goddard, J. J. (2018). Resilience principles in socio-hydrology: a case-study review. Water Secur. 4, 37–43. doi: 10.1016/j.wasec.2018.11.003
Reid, H. (2016). Ecosystem- and community-based adaptation: learning from community-based natural resource management. Climate Dev. 8, 4–9. doi: 10.1080/17565529.2015.1034233
Renganayaki, S. P., and Elango, L. (2013). A review on managed aquifer recharge by check dams: a case study Near Chennai, India. Int. J. Res. Eng. Technol. 2, 416–423. doi: 10.15623/ijret.2013.0204002
Ribeiro Neto, A., Scott, C. A., Lima, E. A., Montenegro, S. M. G. L., and Cirilo, J. A. (2014). Infrastructure sufficiency in meeting water demand under climate-induced socio-hydrological transition in the urbanizing Capibaribe River basin–Brazil. Hydrol. Earth Syst. Sci. 18, 3449–3459. doi: 10.5194/hess-18-3449-2014
Ribeiro, L. (2020). The Role of Ancestral Groundwater Techniques as Nature Based Solutions for Managing Water. EGU General Assembly 2020. Online, 4–8 May 2020, EGU2020-5959, 2020. doi: 10.5194/egusphere-egu2020-5959
Robbins, P. (2012). “Political vs. apolitical ecologies,” in Political Ecology: A Critical Introduction, 2nd Edn, Chapter 1. (Oxford: Wiley-Blackwell), 11–23.
Rockström, J., Falkenmark, M., Allan, T., Folke, C., Gordon, L., Jägerskog, A., et al. (2014). The unfolding water drama in the Anthropocene: towards a resilience-based perspective on water for global sustainability. Ecohydrology 7, 1249–1261. doi: 10.1002/eco.1562
Roobavannan, M., Kandasamy, J., Pande, S., Vigneswaran, S., and Sivapalan, M. (2017). Role of sectoraltransformation in the evolution ofwater management norms inagricultural catchments: asociohydrologic modeling analysis. Water Resour. Res. 53, 8344–8365. doi: 10.1002/2017WR020671
Ross, A., and Chang, H. (2020). Socio-hydrology with Hydrosocial Theory: two sides of the same coin? Hydrolog. Sci. J. 65, 1443–1457. doi: 10.1080/02626667.2020.1761023
Rusca, M., and Di Baldassarre, G. (2019). Interdisciplinary critical geographies of water: capturing the mutual shaping of society and hydrological flows. Water 11, 1973. doi: 10.3390/w11101973
Sadoff, C. W., Hall, J. W., Grey, D., Aerts, J. C. J. H., Ait-Kadi, M., Brown, C., et al. (2015). Securing Water, Sustaining Growth: Report of the GWP/OECD Task Force on Water Security and Sustainable Growth. (Oxford: University of Oxford), 180.
Savelli, E., Rusca, M., Cloke, H., and Di Baldassarre, G. (2021). Don't blame the rain: social power and the 2015–2017 drought in Cape Town. J. Hydrol. 594, 125953. doi: 10.1016/j.jhydrol.2020.125953
Seddon, N., Chausson, A., Berry, P., Girardin, C. A., Smith, A., and Turner, B. (2020). Understanding the value and limits of nature-based solutions to climate change and other global challenges. Philos. Trans. Royal Soc. B 375, 20190120. doi: 10.1098/rstb.2019.0120
Shah, T. (2009). Climate change and groundwater: India's opportunities for mitigation and adaptation. Environ. Res. Lett. 4, 035005. doi: 10.1088/1748-9326/4/3/035005
Sivapalan, M. M., Konar, V., Srinivasan, A, Chhatre, A., Wutich, C. A., Scott, J. L., et al. (2014). Socio-hydrology: use-inspired water sustainability science for the Anthropocene. Earth's Fut. 2, 225–230. doi: 10.1002/2013EF000164
Steenbergen, F., van Tuinhof, A., and van Beusekom, M. (2010). Managing the Water Buffer for Development and Climate Change Adaptation: Groundwater Recharge, Retention, Reuse and Rainwater Storage. Available online at: https://www.bebuffered.com/downloads/3r_managing_the_water_buffer_2009.pdf
Steins, N. A., and Edwards, V. M. (1999). Collective action in common-pool resource management: the contribution of a social constructivist perspective to existing theory. Soc. Nat. Resour. 12, 539–557. doi: 10.1080/089419299279434
Trawick, P. (2003). Against the privatization of water: an indigenous model for improving existing laws and successfully Governing the commons. World Dev. 31, 977–996. doi: 10.1016/S0305-750X(03)00049-4
Vanelli, F. M., and Kobiyama, M. (2021). How can socio-hydrology contribute to natural disaster risk reduction? Hydrolog. Sci. J. 66, 1758–1766. doi: 10.1080/02626667.2021.1967356
Viglione, A., Di Baldassarre, G., Brandimarte, L., Kuil, L., Carr, G., Salinas, J. L., et al. (2014). Insights from socio-hydrology modelling on dealing with flood risk–roles of collective memory, risk-taking attitude and trust. J. Hydrol. 518, 71–82. doi: 10.1016/j.jhydrol.2014.01.018
Vos, J., and Boelens, R. (2018). “Neoliberal water governmentalities, virtual water trade, and contestations,” in Water Justice, eds Boelens, R., Perreault, T., and Vos, J., (Cambridge: Cambridge University Press), 283–301. doi: 10.1017/9781316831847.019
Vos, J., Boelens, R., Venot, J.-P., and Kuper, M. (2020). Rooted water collectives: towards an analytical framework. Ecolog. Econ. 173, 106651. doi: 10.1016/j.ecolecon.2020.106651
Wesselinck, A., Kooy, M., and Warner, J. (2017). Socio-hydrology and hydrosocial analysis: toward dialogues across disciplines. WIREs Water 4, e1196. doi: 10.1002/wat2.1196
Zwarteveen, M., Kemerink-Seyoum, J. S., Kooy, M., Evers, J., Guerrero, T. A., Batubara, B., et al. (2017). Engaging with the politics of water governance. Wiley Interdiscip. Rev. Water 4:e1245. doi: 10.1002/wat2.1245
Keywords: managed aquifer recharge (MAR), water sowing and harvesting, groundwater recharge, sociohydrology, community-based adaptation, Nature-based Solution, climate adaptation, drought risk reduction
Citation: Basel B, Hoogesteger J and Hellegers P (2022) Promise and paradox: A critical sociohydrological perspective on small-scale managed aquifer recharge. Front. Water 4:1002721. doi: 10.3389/frwa.2022.1002721
Received: 25 July 2022; Accepted: 27 September 2022;
Published: 25 November 2022.
Edited by:
Jenia Mukherjee, Indian Institute of Technology Kharagpur, IndiaReviewed by:
Sara Nowreen, Bangladesh University of Engineering and Technology, BangladeshAugustin F. C. Holl, Xiamen University, China
Mayra Gutiérrez-González, Universidad Autónoma de Baja California Sur, Mexico
Copyright © 2022 Basel, Hoogesteger and Hellegers. This is an open-access article distributed under the terms of the Creative Commons Attribution License (CC BY). The use, distribution or reproduction in other forums is permitted, provided the original author(s) and the copyright owner(s) are credited and that the original publication in this journal is cited, in accordance with accepted academic practice. No use, distribution or reproduction is permitted which does not comply with these terms.
*Correspondence: Britt Basel, brittbasel@ecothropic.com