- 1Department of Geography and Geospatial Sciences, Kansas State University, Manhattan, KS, United States
- 2Department of Sociology, Anthropology, and Social Work, Kansas State University, Manhattan, KS, United States
- 3Department of Biological and Agricultural Engineering, Kansas State University, Manhattan, KS, United States
- 4Department of Agricultural Economics, Kansas State University, Manhattan, KS, United States
Sociohydrology is a recent effort to integrate coupled human-water systems to understand the dynamics and co-evolution of the system in a holistic sense. However, due to the complexity and uncertainty involved in coupled human-water systems, the feedbacks and interactions are inherently difficult to model. Part of this complexity is due to the multi-scale nature across space and time at which different hydrologic and social processes occur and the varying scale at which data is available. This systematic review seeks to comprehensively collect those documents that conduct analysis within the sociohydrology framework to quantify the spatial-temporal scale(s) and the types of variables and datasets that were used. Overall, a majority of sociohydrology studies reviewed were primarily published in hydrological journals and contain more established hydrological, rather than social, models. The spatial extents varied by political and natural boundaries with the most common being cities and watersheds. Temporal extents also varied from event-based to millennial timescales where decadal and yearly were the most common. In addition to this, current limitations of sociohydrology research, notably the absence of an interdisciplinary unity, future directions, and implications for scholars doing sociohydrology are discussed.
Introduction
Earth is inherently dynamic (Stephens et al., 2021) with natural processes being impacted by societal and policy changes, exacerbating environmental change to unseen levels (Vogel et al., 2015; Blair and Buytaert, 2016; Di Baldassarre et al., 2019). There have been rapid global changes in land use and land management along with increasing water demands (Kumar et al., 2020). These changes have modified the hydrologic cycle at every scale resulting in the transformation of the landscape's hydrology around the world, significantly impacting societal development and ecosystem quality (Blair and Buytaert, 2015; Kumar et al., 2020; Li and Sivapalan, 2020). Sustainable water resource management is a critical component of food and energy production that is required to meet human demands (Roobavannan et al., 2018). Thus, the adequate availability of water is an important determinant for the socio-economic development of a country (Krahe et al., 2016). As water and society are both connected through mutually shaped relationships, any societal decision for water management, inevitably affects ecology, the organization of social groups, and water-society relationships (Riaux et al., 2020).
The need to better understand these feedbacks has brought on the creation of a new interdisciplinary field of sociohydrology (Sivapalan et al., 2011). Recent studies in the field of sociohydrology include a range of objectives and goals, but are united in a multidimensional, interdisciplinary effort with the aim of establishing generalizable models that hold across time at a global scale (Pande and Sivapalan, 2017; Sanderson, 2018; Di Baldassarre et al., 2019). Modeling sociohydrological systems is inherently difficult because these systems operate at multiple scales, both spatial and temporal, and because of the uncertainty associated with predicting and assessing human activities along with climate change (Li and Sivapalan, 2020; Stephens et al., 2021). Moreover, as the world becomes increasingly globalized, leading to increasingly interconnected sociohydrological systems, there is a need to extend sociohydrology to the space-time domain in order to continue to be able to model and understand real world sociohydrological issues, which implies significant challenges (Pande and Sivapalan, 2017). As Gober et al. (2014) noted, it is not possible to predict water cycle dynamics over decadal or longer periods without considering the interactions and feedbacks among natural and human components of the water system. However, the lack of appropriate data is central to many modeling challenges, including quantifying impacts of landscape change and human-water interactions at various scales (Krahe et al., 2016; Kumar et al., 2020; Gholizadeh Sarabi et al., 2021; Stephens et al., 2021).
As an interdisciplinary field, sociohydrology pulls from a variety of data sources. As Sivapalan et al. (2011) points out, the spatial-temporal scales must be carefully considered. The integration of different types of data from different fields is complex with quantitative and qualitative data (Blair and Buytaert, 2015). While methods for the collection of hydrological data are well-established, it is lacking in terms of having finite historical records for hydrological processes with a majority of the records collected in the past 100 years (Blair and Buytaert, 2015; Troy et al., 2015). The social data required is lacking due to valuable social variables being rarely monitored at the necessary spatial and temporal resolution to match the finer hydrologic data that is available to develop reliable inferences to the underlying dynamics (Blair and Buytaert, 2015; Troy et al., 2015; Krahe et al., 2016; Stephens et al., 2021). For example, U.S. Census data takes place every 10 years where streamflow data can be obtained daily from United States Geological Service (USGS). Also, data accessibility is a challenge where detailed information with social data is not available due to privacy reasons and the collection of new data can be costly in terms of time and money (Blair and Buytaert, 2015; Krahe et al., 2016; Srinivasan et al., 2018). However, historical data can be extended over longer timescales using a broad suite of proxy data such as paleo-climatological methods and hydrologic modeling (Troy et al., 2015). Data regarding social dynamics may need to be pieced together from multiple sources, such as narrative information, numerical records, pictorial information, or archaeological information.
A key challenge for hydrologists aiming to simulate environmental shifts is extrapolating physical relationships across different spatial and temporal scales to understand the feedbacks across those scales (Roobavannan et al., 2018; Stephens et al., 2021). In terms of space, the interactions that occur between natural (i.e., watersheds) and constructed scales (i.e., state boundaries) are superimposed with interactions occurring between local, regional, and global spatial scales (Kelly et al., 2013; Blair and Buytaert, 2015). For example, some sociohydrological issues occur at local scales but are experienced more widely (i.e., point-source pollution), where others are created globally but problems are experienced more locally (i.e., climate effects in the forms of floods and droughts). As far as temporal scales, there are interactions between slow and fast processes. For example, different policy options are appropriate on different timescales, with efforts such as rationing appropriate in the short-term, as opposed to infrastructure decisions and water rights changes being more appropriate in the long term. Thus, relevant spatial and temporal scales are needed for communicating, predicting, and understanding the dynamics of human-water related systems.
In addition to these challenges, there has been criticism over the novelty and necessity of sociohydrology. Blair and Buytaert (2015) claim that sociohydrology focuses on understanding the dynamics and co-evolution of coupled human-water systems in a holistic sense, which makes it different from other water management fields. Where Sivakumar (2012) and Madani and Shafiee-Jood (2020), find it important to recognize the decades of research that developed similar approaches and tools that are foundational to sociohydrology. Falkenmark (1977) was one of the first to introduce the need to include all interactions between man and water in the management planning process, later coining the phrase hydrosociology (Falkenmark, 1979). Since then, researchers introduced other interdisciplinary water resource fields like ecohydrology and integrated water resource management (IWRM), both with slightly different goals. Ecohydrology aimed to improve freshwater ecosystems' ability to adapt to human-induced stresses by integrating interactions between plant and animal life, climate, and hydrological processes into the decision-making process (Zalewski, 2000). Over 500 participants from 100 countries helped define the core principles of IWRM at the International Conference on Water and the Environment in 1992. Many countries and organizations define IWRM differently, but most aim to equitably maximize the economic and social welfare through coordinated use and development of water resource infrastructure (Xie, 2006). Additionally, Madani and Shafiee-Jood (2020) published an extensive review of research that employed similar, if not identical, methods to those described by the call to commentary on sociohydrology by Sivapalan et al. (2011). More recently, Ross and Chang (2020) reviewed sociohydrology and hydrosocial studies. They found that while both subfields focus on the coevolution of human-water systems, they show significant differences and, as response, they both offer new strengths to compensate for what the other may lack.
Each of these fields have received similar criticism for being divided in their approach, goals, and terminology (Kundzewicz, 2002; Hannah et al., 2004; Jeffrey and Gearey, 2006). Regardless of these criticisms, research published under each of these fields has provided valuable insights into the complex interactions between anthropogenic and natural systems before the introduction of sociohydrology. Regardless, this review is not intended to comment on the validity of sociohydrology as a new science, nor to provide an exhaustive history of spatial and temporal scale issues in water resource research, but to provide an overview of those spatial and temporal scales that are used under the umbrella of sociohydrology since its introduction in 2011. Therefore, this review has three main research questions, (1) what spatial and temporal scales do researchers use to study human-water systems in sociohydrology, (2) what physical and social components do researchers use to study human-water systems in sociohydrology, and (3) how have the spatial and temporal scales, as well as the components used changed since the introduction of sociohydrology in 2011?
Materials and Methods
The systematic review was designed to examine the spatial and temporal scales that researchers used to study human-water systems along with identifying gaps or challenges within sociohydrology. This was done by identifying the methodology and the data that was used for studies that were conducted within the sociohydrological framework. The Preferred Reporting Items for Systematic Reviews and Meta-Analysis (PRISMA) standards defined by Moher et al. (2009) was used to define the eligibility criteria, literature search, coding, and reporting conventions. The studies used in this systematic review are referred to as primary studies. The term coders used in the following sections refers to the first six authors. The coders share an interdisciplinary background with expertise in sociology, geography, economics and water resource engineering.
Eligibility Criteria
This review worked to include all searchable studies that have conducted research within the sociohydrological framework to provide a thorough and systematic examination of the types of data used and the methodology for overcoming the inherent spatial-temporal scale challenges within interdisciplinary research. Thus, the primary studies eligible for inclusion in this systematic review needed to meet the following criteria: (a) the study is conducted within the sociohydrological framework as defined by Sivapalan et al. (2011) in which the study considers both humans and water in a coupled system, (b) the study is performing analysis or implementing a model through a case study, (c) the study is from a peer-reviewed journal article.
Identification of Primary Studies
The primary studies were obtained from searching commonly used economic, social science, and science databases to include EBSCO, ERIC, Google Scholar, ProQuest, SCOPUS, Taylor and Francis, Web of Science, and Worldcat. A search string was determined through an iterative process to identify studies that used any of the search terms in the title, abstract, or keywords. The final search string was the following: (“socio-hydrology ORsociohydrology OR sociohydrological OR sociohydraulic OR human flood interactions OR coupled human-water systems OR human-water dynamics”). The search was further limited to the years 2011–2021 since it was assumed that the new study of sociohydrology was coined by Sivapalan et al. (2011). The search was not limited by geographic location but was limited to full-text studies available in English. The official search ended March 2021 and returned 4,606 studies (Table 1).
Due to the nature and limitations of the search, in particular, the need to have the studies in English, reduces and underrepresents the sample of collected studies. This is an inherent drawback in most systematic reviews, thus even though this search was systematic, the primary studies in the search may still be biased in terms of variations and methodology for dealing with differing spatial-temporal scales within the sociohydrological framework. While this does not necessarily detract from the importance and application of the findings, it is critical to note that this limits the scope of the work and the extent to which the results can be generalized.
Screening Studies Based Upon Eligibility Criteria
From the initial search of databases, a three-phase process was used to screen primary studies that met all eligibility criteria (Figure 1). In pairs, coders independently read the title and abstract for all the studies obtained in the original search using the search string and specified years. Each pair checked if the studies were conducting analysis within the sociohydrological frameworks by determining if both human and water components were included in the study to some degree. It was also important to distinguish between studies that were discussing sociohydrology and those that were proposing a framework or model compared to the studies that were actually implementing a framework or model. This distinction was made because the review only wanted to consider how differing spatial-temporal scales were used throughout the studies, thus a study had to actually be conducting sociohydrological analysis. For example, Di Baldassarre et al. (2015) proposed a novel framework for analyzing the dynamic interactions and feedbacks between flooding and societies but did not implement the framework through a case study. Additionally, Sivapalan (2015) discussed the benefits and challenges of endogenizing humans into hydrologic systems by broadening the hydrologic science field to include perspectives of both social and natural scientists but did not conduct data analysis. Thus, studies were only retained if they mentioned conducting analysis in sociohydrology or coupled human-water systems. Studies were also marked as “maybe” if they discussed sociohydrology or if it was unclear from the title or abstract if they met the criteria. Each pair checked if there were studies that were retained by one coder but not the other and discussed reasons for including or excluding studies. If there was still uncertainty, the group as a whole discussed the study and came to a consensus decision. A designated coder also reviewed the studies and removed any duplicates. The majority of the 4,606 studies examined did not discuss coupled human-water systems within the sociohydrology framework.
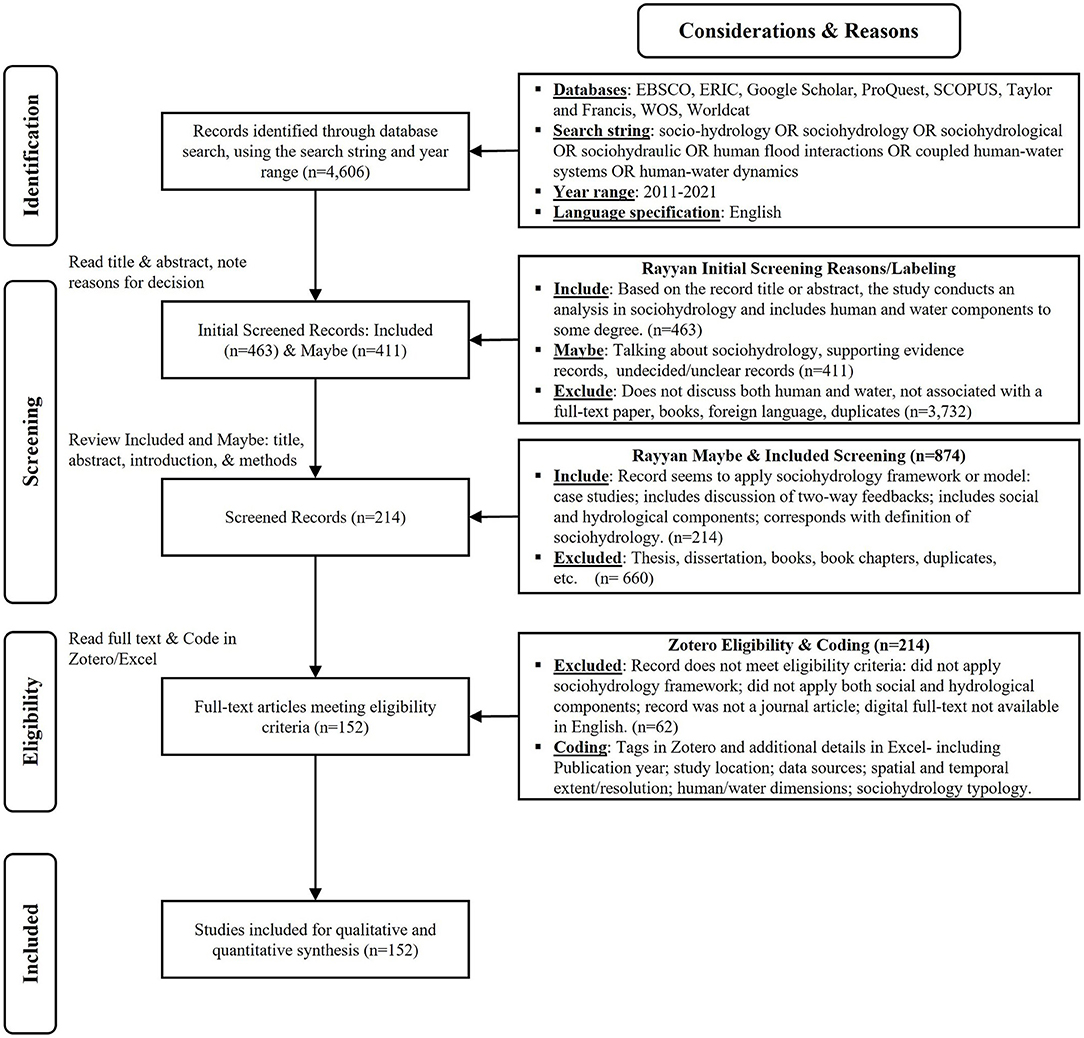
Figure 1. PRSMA flow diagram detailing the systematic review process with study counts and exclusion criteria.
In phase two, 463 studies were marked “included” and 411 studies were marked “maybe” totaling 874 studies left for additional screening. Using Rayann (Ouzzani et al., 2016), pairs of coders independently assessed whether each study fit the eligibility criteria outlined above by reading the title, abstracts, introduction, and methods. The coders discussed any discrepancies and made exclusion decisions together and if needed, the group as a whole resolved any disagreement between coders. In this phase, articles were excluded if two-way feedbacks with both social and hydrological components were not discussed. Studies were also excluded if they were published as a book, thesis, dissertation, or if there was not a digital full-text in English. After screening, there were 152 studies left for further analysis.
Included Studies for Synthesis
Coders were trained together on how and what to code using two studies as examples. Each coder then independently coded the 152 eligible studies using a common coding schema (Table 2). Some of the key information included publication year, spatial and temporal extent and resolution(s), geographic location(s) of the study, data source(s), human and water components, typology, and methodological approach. Disagreements or concerns were resolved through group discussions and a consensus was met. As an additional check two coders were designated to review the coding for consistency and accuracy across all studies. Throughout the screening and coding process, coders met regularly and had weekly check-ins with the group.
Results
While issues of scale are foremost of concern for this review, there are non-scale themes across studies that warrant comparison. These include the components of the water bodies and social variables studied, the data sources and their types, the methodological approach used, and finally the feedbacks and interactions. Examined in order are these non-scale themes followed by temporal scales, spatial scales, and spatial location of primary studies, where each is analyzed descriptively with relevant, non-exhaustive examples. Lastly, risk of bias is addressed. Some studies did not provide sufficient detail for the coder to confidently classify the category. In these cases, the code was not recorded. It should be noted that 2021 was not fully reported as the primary studies were defined between 2011 and March 2021. Additionally, there were no studies in 2011 that fit our criteria, instead 2012 was the first year of publication for the primary studies.
Water Components Used in Primary Studies
The non-scale themes were identified and analyzed to determine the commonalities of the types of data and variables used to understand the sociohydrological process. There was not a clear temporal trend for water components addressed in the primary studies, rather the trend was more related to an increase in articles published under the umbrella of sociohydroly since 2011. The first non-scaler theme is water body component that describes the hydrological process considered in the primary study. Eight water components were identified and coded: water resource management, flood management and stormwater structures, farming and irrigation management, groundwater, water quality, reservoir and lakes, drought, and coastal zones. The most common water component was water resource management (39%; Table 3). This was not surprising because water resource management was defined very broadly to include any study that considered an area's water system as a whole instead of focusing on a specific water or hydrologic component. In other words, if a primary study did not fit into the other seven components then water resource management was coded. It should be noted that the percentage of the primary studies does not add to 100% given several of the articles featured multiple components. For instance, Iwanaga et al. (2018) covered four components, reservoir, water resource management, groundwater, and irrigation, in their integrated models and scenarios based on stakeholders in the basin region. Water resource management, reservoir, and drought often overlapped, as did groundwater and irrigation. Additionally, water components imbue a social-use connotation, such as irrigation and water resource management, as well as an implied social response, such as drought, flooding, or tsunamis in coastal areas.
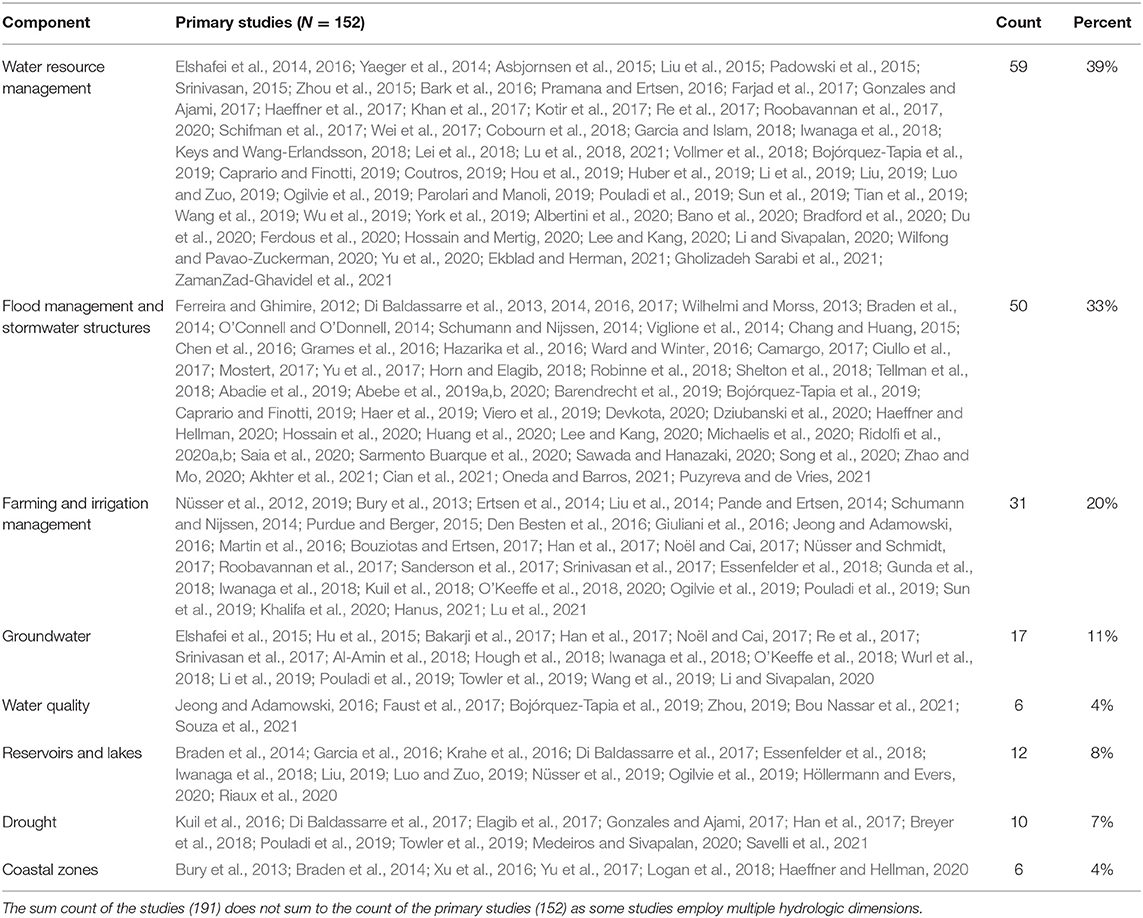
Table 3. The different water components considered in the primary studies along with the source of the study that used each.
Social Components Used in Primary Studies
In addition to water components, social components were also identified throughout the primary studies. This was important because the overreaching goal of sociohydrology is to no longer treat the human factor as a stationary external force, but to consider humans and their actions as a part of the water cycle dynamics (Sivapalan et al., 2011). As previously noted, modeling across and within global scales is challenging (Pande and Sivapalan, 2017). The same is true when scaling social science indicators. As hard as it is to capture social components spanning macro, meso, micro, and psycho-social levels, it is even harder to delineate social components due to their interrelation. In many cases authors use multiple of each, and/or indexes, scales, or theories which incorporate various, non-exclusive components. Due to this circumstance, social components were identified qualitatively. Nevertheless, five overarching social components were identified: socioeconomic, vulnerability/health, memory, demography, and behavior.
There exists a wide range of social in sociohydrology, the most common was socioeconomic indicator, included in 20% of the studies. Although, this social component featured a vast range of variables. For instance, Wang et al. (2019) approximated social by assessing population and Gross Domestic Product (GDP) yearly (basin scale) whereas Wilhelmi and Morss (2013) measured it using physical/language abilities and access to resources. Sun et al. (2019) measured socioeconomic with population, regional GDP, proportion of the secondary and tertiary industry, labor compensation per capita, and proportion of urban residents. Luo and Zuo's (2019) socioeconomic index included per capita GDP, per capita net income of rural residents, per capita disposable income of urban residents, tertiary industry share of GDP, population density, urbanization rate, Engel's coefficient, number of hospital beds per 10,000 people, per capita urban road area, and residents' satisfaction with the environment. Huber et al. (2019), in addition to households, economy, water supply, etc., included tourism. In addition, socioeconomic factors are sometimes explicitly used to approximate inequalities in water distributions or effects from conservation efforts. For instance, Breyer et al. (2018) found outdoor water conservation for reservoirs at the city scale produced, among other effects, downward redistribution of water along a socioeconomic gradient. In other words, as a result of the conservation, people with lower socioeconomic status had less water access than those with higher status.
Social vulnerability generally measures how individuals and communities are able to withstand shocks, including natural hazards. Cian et al. (2021) analyzed Northeast Italy's frequent flooding every 5 years using the flood vulnerability index, or FVI, which the authors claim to allow for a dynamic and adaptable assessment of vulnerability, necessary in preparation for the worst of climate change. Human development index (ZamanZad-Ghavidel et al., 2021). Hazarika et al. (2016) Dhemaji in the Upper Brahmaputra floodplain, indigenous knowledge and adaptations has allowed floodplain dwellers to be able to coexist with floodplain dwellers. Vulnerability can be tied to human resource depletion or ecosystem interference beyond the natural rate or environment (Liu et al., 2014). Related to vulnerability is human health. Keys and Wang-Erlandsson (2018) considered child malnutrition as an indicator of food security with moisture recycling. Baker et al. (2015) utilized social health risk, instead of the more widely-used contaminant concentration rate, as an optimization variable improves water management decisions aimed at maximizing social well-being.
Several authors assessed the social component via social memory, or the shared histories and experiences of groups of people in a place. In addition to the commonly-used variables of community awareness or sensitivity, Yu et al. (2017) add institutions for collective action and connections to an external economic system to their assessment of flood memory. Grames et al. (2016) include social memory and the sensitivity of social awareness which feedback on flood adaptation within the timespan of economic agents' decision-making.
Demography was frequently used as a control or endogenous variable (Braden et al., 2014; Roobavannan et al., 2017; Breyer et al., 2018; Ferdous et al., 2018; Garcia and Islam, 2018; Sarmento Buarque et al., 2020). A majority of sociohydrological research containing issues of scale and feedbacks do not utilize demographic variables to featurein analysis the latent structural sinequalities that operate at the center of the human-water nexus (Sanderson, 2018). Studies that did include these structural inequalities featured the typical demographic categories of race, age, sex, gender. Demographic factors and their influence on behavior were also examined. For example, Huang et al. (2020) find demographics and flood risk perception do not have direct impacts on protective coping behaviors with flooding, but are mediated by flood risk knowledge and flood risk attitude. If demographics are used, they are included in indexes with more traditional socioeconomic variables income, population, GDP, and so forth (e.g., Huber et al., 2019).
Finally, human behavior was the last social component identified throughout the primary studies. There are no universally accepted laws of human behavior as there are for physical systems. Therefore, the variables and methods which measure behavior are wide-ranging. One aspect of behavior was public participation, such as capacity building, participatory activities, and workshop discussions (Kotir et al., 2017; Re et al., 2017; Bojórquez-Tapia et al., 2019; Bradford et al., 2020). These were conducted with surveys, interviews, workshops, or data from previous studies. Public participation is generally considered to signal bottom-up socio-behavioral change over time. A socio-behavioral measure considered to have greater top-down determination is policy preference, choice, action, or intention. In fact, 21% of the studies included variables representing a socio-behavioral dimension including policies or a policy (Liu et al., 2015; Srinivasan, 2015; Di Baldassarre et al., 2016; Garcia and You, 2016; Giuliani et al., 2016; Grames et al., 2016; Bakarji et al., 2017; Farjad et al., 2017; Gonzales and Ajami, 2017; Haeffner et al., 2017; Han et al., 2017; Khan et al., 2017; Kotir et al., 2017; Noël and Cai, 2017; Re et al., 2017; Sanderson et al., 2017; Essenfelder et al., 2018; Keys and Wang-Erlandsson, 2018; Robinne et al., 2018; Abebe et al., 2019a,b; York et al., 2019; Bradford et al., 2020; Du et al., 2020; Wilfong and Pavao-Zuckerman, 2020; Bou Nassar et al., 2021; Hanus, 2021; Oneda and Barros, 2021; Savelli et al., 2021; ZamanZad-Ghavidel et al., 2021).
Studies not only examine the outward-facing socio-behavioral measures, but those more inward, deeply-seated, and traditionally sociological. These include value systems, attitudes (Ciullo et al., 2017; Huang et al., 2020; Bou Nassar et al., 2021), beliefs (e.g., Souza et al., 2021) and norms (e.g., Braden et al., 2014). The authors consider perceptions (Elagib et al., 2017; Devkota, 2020) behavioral because they are often conceptualized and operationalized in ways that assume that by perceiving something in a particular light, the agent's behavior or action will follow accordingly.
Data Source Type
The data source types identified and coded for the primary studies were primary, secondary, simulated, and combinations thereof (Table 4). Primary data source is defined as data collected by the authors. Secondary data sources includes data that the authors retrieved from another source, such as local, regional, and state-based sources, however, most came from governmental surveys, such as the USDA (Dziubanski et al., 2020), Census Bureau (Sanderson et al., 2017), CIRD (Camargo, 2017), international organizations (ZamanZad-Ghavidel et al., 2021) which often provided economic and social variables. Simulated data was defined as data that the authors simulated for the study. The most common data source type throughout the primary studies was secondary data (47%) followed by primary and secondary (30%). There were 21 studies which included simulated data (e.g., Garcia and You, 2016; Gonzales and Ajami, 2017).
Data Types Throughout the Primary Studies
Following the identification of the data type source, eight unique data types emerged from the review: remote sensing, meteorological, survey (interview), survey (site investigation), survey (government), archaeological/historical, hydrologic, and maps. The results show that hydrologic data was included in 63 studies (41% of total), by far the most (Table 5). This is not surprising given 24% of the journals in this review were primarily hydrological (Table 6), with 20 alone coming from Hydrology and Earth System Sciences. Examples of hydrologic data included in the primary studies were stream gauge data (e.g., Garcia et al., 2016), soil moisture (e.g., Den Besten et al., 2016), groundwater and irrigation rates (e.g., Tellman et al., 2018; Gholizadeh Sarabi et al., 2021), and hydropower (e.g., Huber et al., 2019). Maps were defined as those not derived from remote sensing and were used the least (9%). In most cases, maps were used in addition historical or archeological studies (e.g., Nüsser et al., 2012; Baker et al., 2015; Chang and Huang, 2015; Caprario and Finotti, 2019; Cian et al., 2021; Gholizadeh Sarabi et al., 2021). Where historical and archaeological data was only used in 10% of the primary studies, by showing how human and water systems co-evolve over time, from ancient Rome (Di Baldassarre et al., 2017) to West Africa (Coutros, 2019) to the Tarim River basin in Western China and beyond (Liu et al., 2014).
Typology and Methodological Approach of Primary Studies
Typology and methodological approaches were coded in this review to understand what types of methods were used to combine social and hydrological variables within the sociohydrological framework. Three typologies were coded based upon the types of sociohydrology analysis identified in Pande and Sivapalan (2017). These include process, historical, and comparative. These typology codes were used to identify how the methods and tools are used, including what types of questions they help answer about a system. Additionally, the following methodological approaches were identified throughout the primary studies: established modeling, system dynamic models, agent-based models, and synthesis analysis. The methodological codes provide insight into the diversity of tools and methods being used in sociohydrological research, as well as drawing attention to approaches unique to the developing field. Typologies were coded based upon the types of sociohydrology analysis identified in Pande and Sivapalan (2017). These include historical, comparative, and process sociohydrology. Each of these approaches varies from traditional hydrologic studies or modeling because they endogenize social factors into their analysis. Sociohydrologic approaches focus less on calculating flow rates and finite numerical outcomes and focus more on understanding system the nature of system evolution. Specifically, historical sociohydrology was defined as having the aim to understand a coupled system from its immediate or distant past and often leverages historical accounts of specific events. A major emphasis in historical sociohydrology is understanding the major events that forced a system to evolve to its current state. Examples of these major events include, but are not limited to, wars, regime changes, major infrastructure projects, policy changes, droughts, and other severe weather events. Comparative sociohydrology was defined as a study that compares different systems across space or time, with the goal of understanding how different systems respond to similar events, or testing if certain generalizations can be made about interactions in larger human-water systems. Lastly, process sociohydrology was defined as trying to understand and hypothesize about the nature of observed processes. Process sociohydrology often asks “what if” questions to understand how a system would respond to certain changes. Such changes can come in the form of new policies, the addition of new stakeholders, reductions in resource availability, or technological improvements. While models are not specifically required for sociohydrology analysis, they greatly assist process sociohydrology. The most common typologies were process and historical, with process being the most common in the year 2020 (Figure 2). Comparative studies peeked in 2019 with 4 studies.
Next, methodological approaches was coded to identify how methods or tools are used. Firstly, established models are models which have been replicated, used, and modified across studies and over time. Established models were identified in 22% of the primary studies and examples include MIKE SHE/MIKE 11 (Farjad et al., 2017), SWAT (Baker et al., 2015; Martin et al., 2016; Khan et al., 2017; Essenfelder et al., 2018), and ISBA-MODCOU (Medeiros and Sivapalan, 2020). By nature, these models are most familiar to traditional hydrologists but are modified to incorporate additional social parameters to study system feedbacks.
In contrast, t system dynamic models represented frameworks developed specifically for sociohydrologic analysis (e.g., Den Besten et al., 2016; Sanderson et al., 2017; Gholizadeh Sarabi et al., 2021). System dynamic models represent top-down feedback approaches utilizing multi-loop, non-linear structures. They can be as simple as a causal loop, or as complex as a system of differential equations. While these models should mimic general interactions between stakeholders and resources, they generally do not require extensive calibration (Ding et al., 2018). System dynamic models were used in 42% of the primary studies and were useful to studies spanning the global scale (e.g., Elshafei et al., 2014; Hou et al., 2019; Liu et al., 2019; Hossain and Mertig, 2020). Hou et al. (2019) examined the social water cycle fluxes across 39 countries assessing total water use of countries and evolution mechanisms. Liu et al. (2019) looked at global virtual water use across 44 nations and regions. Hossain and Mertig (2020) considered water footprint or “virtual water consumption” by looking at energy, world position, beef consumption, and urbanization.
Agent-based modeling (ABM) simulates the interactions between water systems and one or multiple agents, which can be conceptualized as individuals or collective groups (Akhbari and Grigg, 2013). Rather than using causal loops or differential equations to describe interactions, ABMs rely on computational information streams and predetermined rules. The predetermined rules in ABMs govern how each agent will respond to model scenarios and interact with other agents. While ABM is similar to system dynamic models in that they model interactions, the authors established separate categories for these approaches because ABM is considered a bottom-up approach to modeling sociohydrological processes while system dynamic models are considered top-down models (Ding et al., 2018). ABM appeared in 13% of the primary studies and has been used to analyze farmer's agency shaping their financial situation and their water conservation (Pouladi et al., 2019) and can be used as a decision support tool for watershed management (Huber et al., 2019). ABM are flexible and dynamic and can incorporate established models. For instance, Khan et al. (2017) develop an ABM framework that includes a SWAT model to simulate the impacts of water resource management decisions that affect the food–water–energy–environment (FWEE) nexus at a watershed scale.
Finally, synthesis analyses integrate varying perspectives, data types, and approaches to provide a narrative of the system. Synthesis analyses generally attempt to describe the current state of a system by analyzing individual components and making inferences about how the states of those components are linked. While not always the case, this approach is often qualitative in nature, and as a minimum requirement, incorporates some form of qualitative data in the analysis. For instance, Haeffner et al. (2017) examined the historical, biophysical, economic, and cultural relations embedded in the development of urban water infrastructure in an urban Mexico watershed. Vollmer et al. (2018) created the Freshwater Health Index that integrates governance mechanisms, ecosystem dynamics, and stakeholder perceptions to better engage stakeholders in addressing multiple freshwater demands. In addition, Zhao and Mo (2020) provide a comprehensive analysis of the Holocene hydro-environmental evolution in Jianghan-Dongting Basin in China by studying sediment cores.
Spatial-Temporal Interactions and Feedbacks
Variables used for studying feedbacks were coded qualitatively. This was done because of the complexity and variety of variables that the primary studies used to quantitatively or qualifiedly describe the sociohydrology interactions. A few notable examples of feedbacks used are Bojórquez-Tapia et al.'s (2019) Analytic Network Process-situated feedback loop which involved synchronous and parallel updating of the geographic data river discharge time series (streamflow/inflow to reservoir). Roobavannan et al. (2017) mimicked and explained feedback between various subsystems of the human-water system, water availability, agriculture, environment health, manufacturing and services, technology, human population, and derived reservoir outflow from variation in water storage. Puzyreva and de Vries (2021) examined historical exposure to flooding, the community response and preparedness, evolution of risk acceptance, community engagement, perceptions of flooding causes, and how they feedback one another. Towler et al. (2019) drought feedbacks were influenced by science, technology, as well as historical lessons learned and management strategies. Robinne et al. (2018) included education capacity in their social variables. Baker et al. (2015) included media coverage and comments into their feedbacks. These are but a few of the feedback variables and mechanisms—creative, useful, and potentially transformative—found throughout the primary studies.
Temporal Scale
Temporal scale was investigated through both the temporal extent of a study and the temporal resolution at which the sociohydrological processes in the study was analyzed. Temporal extent was determined using the following categories: millennia, century, decadal, yearly, and event-based. A study was categorized as “millennia” if the temporal extent of the sociohydrological processes was over the course of one or multiple millennia. A study was categorized as “century” if the process was analyzed over the course of one or multiple centuries but less than a millennium. All other temporal extents followed this classification method with the exception of event-based studies. Event-based temporal extents referred to studies that did not specify a time frame within which analysis took place, but instead analyzed sociohydrological processes for the duration of a specific event or events. This was most common for studies that used simulated data to examine sociohydrological processes. For example, multiple studies simulated flood events to better understand pathways for city development (Viglione et al., 2014) or observe interactions between flood risk and behavior (Ridolfi et al., 2020a). Alternatively, some studies used models to simulate changes in a sociohydrology system over the course of the implementation of a specific policy (Du et al., 2020) or flood management strategy (Albertini et al., 2020). In these cases, temporal extent is not explicitly stated, but there is an implicit understanding that these events occur over a definite amount of time.
Results of coding showed primary studies analyzing sociohydrological processes over decadal (28%), yearly (30%), and event-based (18%) temporal extents to be the most common (Table 7). This trend could be indicative of the availability of necessary data to analyze human-water systems. For example, multiple studies reviewed relied on social data from either the Australian census (Elshafei et al., 2014, 2015; Roobavannan et al., 2017; Wei et al., 2017), which takes place every 5 years, or the United states census (Sanderson et al., 2017), which takes place on a decennial basis, providing reliable quantitative social data for studies investigating sociohydrologic processes over multiple decades. Moreover, data necessary to model hydrological processes is more abundant at these time scales as well, such as yearly water budgets (Keys and Wang-Erlandsson, 2018), water footprints (Souza et al., 2021), and data from Resource Bulletins that provide yearly data on water use and reservoir flows (Hou et al., 2019; Li et al., 2019; Luo and Zuo, 2019; Sun et al., 2019). Studies analyzing processes over centuries or millennia are more limited by data availability and often rely on archeological data (Pande and Ertsen, 2014; Wei et al., 2017; Lu et al., 2018; Zhao and Mo, 2020; Puzyreva and de Vries, 2021) or are limited to specific geographical areas that have well-documented hydrological and social data (Di Baldassarre et al., 2017). Examining the frequency of studies using these different temporal extents over time revealed that decadal and yearly temporal extents steadily increased as publications in sociohydrology increased (Figure 3). However, in 2020 studies employing decadal and yearly temporal extents decreased, and event-based studies increased.
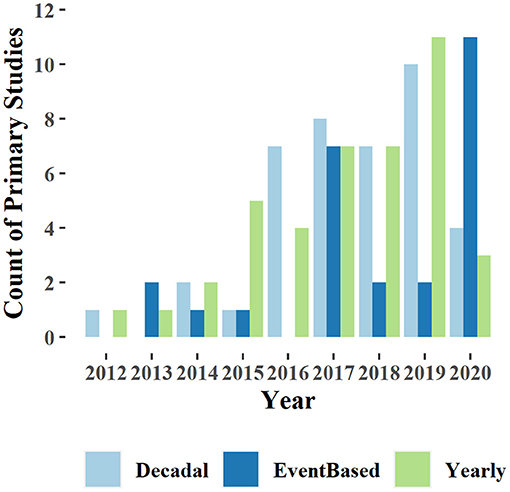
Figure 3. Count of the primary studies' three most common temporal extents by year of publication between 2012 and 2020.
Temporal resolution was also coded by recording the overall temporal resolution for a study or individually recording the temporal resolutions of the different data used in a study. Temporal resolution varied from groundwater level data collected every 15 min (O'Keeffe et al., 2020) to sociohydrological interactions over 6,000-year periods (early-Neolithic, middle-Neolithic, and late-Neolithic; Zhao and Mo, 2020). However, this variable is not reported quantitatively as the majority of studies reviewed did not provide sufficient information for coders to confidently identify temporal resolution. Lack of clear, explicitly stated temporal resolution emerged as a trend among the sociohydrology studies reviewed and was especially prominent among studies that used solely simulated data. Further, in the event that temporal resolution was reported for each data type used in a study, explanation of how these data types of differing temporal resolutions were integrated was often not present.
Spatial Scale
Similar to temporal scale, spatial scale for each primary study was examined through spatial extent and spatial resolution. Spatial extent was determined by identifying the spatial boundaries of a study as they fit into one of the following categories: global, international, national, regional, state, county, city, social network, watershed, or water body network. Primary studies categorized as having a “regional” spatial extent include studies that were analyzing sociohydrological processes at a spatial extent that did not conform to political boundaries (nation, state, county) but also did not belong to the water or network based categories (watershed, social network, water body network). For example, Sun et al. (2019) examined sociohydrological processes in a region covering 31 provincial-level administrative areas in China to better understand virtual water trade and water consumptions patterns. A study was categorized as having the spatial extent of a “water body network” when the study boundaries were determined by a specific geographical area surrounding a water body or system, such as an irrigation system (Khalifa et al., 2020), floodplain (Ferdous et al., 2018), or aquifer (Towler et al., 2019). A study was coded as having the spatial extent of a “social network” when the study area was defined by a social structure but did not conform to any of the more common social boundaries, such as county or state. For example, Bojórquez-Tapia et al. (2019) defined their study area by the sewage system in Mexico City. As a sewage system is socially constructed, this spatial extent qualified as a social network. Lastly, some primary studies were coded with more than one spatial extent as they analyzed areas of varying spatial extent for the purpose of comparison. For example, Akhter et al. (2021) compared sociohydrolocial processes at the national, state, and county level to gain a better understanding of flood risk management in floodplains across the United States.
The results of coding for spatial extent showed that the most common were watershed (37%), regional (24%), and city (23%; Table 8). Coders also observed that specific watersheds were of particular interest to researchers. For example, multiple primary studies focused on the Heihe River Basin in China (Lu et al., 2016; Wang et al., 2019; Du et al., 2020) and the Murrumbigee River Basin in Australia (Roobavannan et al., 2017, 2018, 2020). Further, studies analyzing sociohydrological processes within watersheds often prioritized water resource management, especially as it concerned the sociohydrological processes of irrigation (Roobavannan et al., 2017; Sanderson et al., 2017; Nüsser et al., 2019; Ogilvie et al., 2019; O'Keeffe et al., 2020) and drought (Di Baldassarre et al., 2017; Pouladi et al., 2019; Medeiros and Sivapalan, 2020). Studies analyzing sociohydrological processes at the city level were often focused on water resource management as it related to stormwater (Tellman et al., 2018; Oneda and Barros, 2021), wastewater infrastructure (Faust et al., 2017; Tellman et al., 2018; Souza et al., 2021), and human water consumption (Li et al., 2019; Savelli et al., 2021).
Watershed, regional, and city spatial extents being the most common among primary studies is also important for understanding the lens through which sociohydrology studies are framed. One of the key challenges of sociohydrology is appropriately integrating water systems that do not conform to political boundaries, and human systems that do not naturally align with hydrologic boundaries or networks. Reconciling these differences to model human-water systems or synthesize data to better understand sociohydrological processes requires careful consideration of the spatial extent of the study. Studies using watershed spatial extents inherently prioritize hydrological processes in the human-water system. Similarly, studies using city level spatial extents prioritize social processes in the human-water system. As regions can be defined both physically and socially, the popularity of this spatial extent potentially demonstrates the general flexibility of not using specific political or hydrological boundaries. Additionally, examining the use of these spatial extents over time, it can be observed that the use of regional spatial extents peaks in 2017, while the use of the watershed and city spatial extents steadily increases, peaking in 2020 (Figure 4).
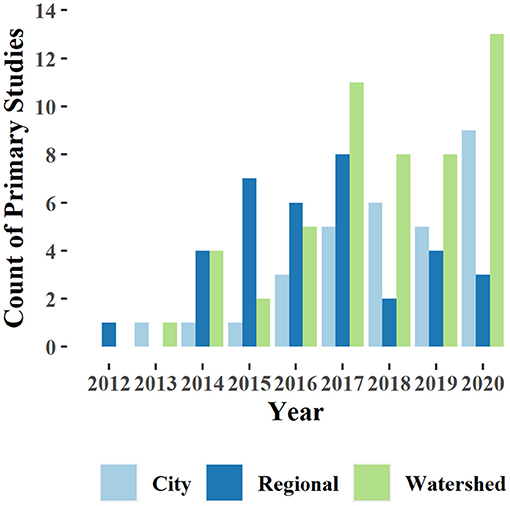
Figure 4. Count of the primary studies' three most common spatial extents by year of publication between 2012 and 2020.
Spatial resolution was also coded for by recording the overall spatial resolution for a study or individually recording the spatial resolutions of the different data used in a study. However, similar to the data gathered for temporal resolution, spatial resolution was not reported consistently or clearly enough in the primary studies reviewed for the coders to confidently identify the spatial resolution of each study and is therefore not reported quantitatively. Qualitatively, though, the coders observed that spatial resolution was reported more frequently for raster data than it was for vector data. In studies that used both vector and raster data for their modeling or analysis, this is especially notable given vector and raster data are inherently incompatible and must be manipulated to reach compatibility. Converting raster to vector or vector to raster to create compatible data layers comes with its own trade-offs (Congalton, 1997), such as loss of data quality due to aggregation or lessened reliability of data due to interpolation. However, transforming and manipulating data within the same data format to adjust for different scales still requires careful consideration of how the data and its subsequent interpretation and representation will be affected. Without explicit reporting of the spatial scales of these data sources or how these data sources are being manipulated within a model or series of analyses, it is difficult to critically reflect on scale issues in sociohydrology, as having access to this information is key to understanding the implications of scale issues on methodological approach and study results.
There were few studies that engaged in full transparency when reporting, though. For example, Wilhelmi and Morss (2013) conducted a case study of Fort Collins, Colorado, to gain a better understanding of interactions between social vulnerability and extreme precipitation events. The authors listed the data being used in the social vulnerability index in a table and included spatial and temporal scale information for each data source. Further, the authors explained how precipitation data was measured, manipulated, and ultimately integrated with the social vulnerability measures of sensitivity and coping capacity. In this study, a combination of tables, figures, and text explanation were used to explicitly communicate spatial and temporal scale, data manipulation as it related to scale, and data integration, allowing the reader to critically interrogate the results of the study.
Spatial Distribution of Research in Sociohydrology
The spatial distribution of the countries where the primary studies reviewed took place revealed high frequencies of studies in the United States, Australia, and China (Figure 5). This is undoubtedly partially due to the authors limiting their systematic literature search to English-only journal articles. However, this could also be indicative of a focus in sociohydrology literature on areas that are facing difficult social and water situations. For example, Australia has been experiencing extreme droughts and water shortages that are having severe effects on agriculture and farmers (Di Baldassarre et al., 2017; Roobavannan et al., 2017). A focus on sociohydrology issues in Australia is thus not surprising. Lastly, data availability plays an important role in the distribution of sociohydrology papers reviewed in this study.
Risk of Bias Across Primary Studies
There is a risk of coding error, as official intercoder reliability scores were not calculated. That said, the authors aimed to minimize error by randomly checking each other's work ex post facto for accuracy and detail. It is acknowledged that selective reporting of complete studies, such as publication bias or “outcome reporting bias” within individual studies, may occur in our methods and reporting results (Moher et al., 2009). The implications of these biases may be unclear, yet there is little dispute that selective outcome reporting typically occurs in the context of systematic reviews (Moja et al., 2005). To minimize biases, Moher et al.'s (2009) PRISMA checklist was used. Future systematic reviews could adapt this scale for those in the natural or social sciences, to more rigorously check for, and alleviate, these biases. However, such a re-tooling is outside the scope of this review.
Discussion
This review used categorization of the spatial-temporal scales and physical and social components used to study human-water systems to provide an overview of sociohydrology since 2011. This categorization and overview combined to form the first step in identifying challenges, specifically spatial-temporal scale challenges, in the nascent field of sociohydrology. It was found that the most common temporal extents were yearly and decadal with event-based studies becoming more popular in recent years. In addition, watersheds, cities, and regions were the most used spatial extents with regions becoming less used in recent years. China and the United States were the locations of the most studies where there is a larger hydrological focus demonstrated through a majority of publications in hydrological focused journals compared to sociology journals. The results also showed data coming largely from secondary sources relying primarily on hydrologic data and government surveys. It was also demonstrated that research within sociohydrology considers water from a variety of perspectives, such as water quantity, water use, and water management. However, with only 4% of studies focusing on water quality, agricultural studies generally focusing on irrigation management and water use, and no studies focusing on mining and industry. There is a clear lack of research in sociohydrology targeting issues of water quality. While definitions of sociohydrology do not explicitly state that the focus of this interdisciplinary field is not on water quality, the results indicate that the interactions and feedbacks between humans and water quality is not the focus of sociohydrological issues.
Sociohydrology by definition tries to be distinct from other coupled human-water fields of study as it considers humans to be endogenous to the system (Pande and Sivapalan, 2017). However, the authors observed a wide variety of social variables that represent different interpretations of what it means for humans to be “endogenous” to a system. Studies varied from accounting for social components of the human-water system exclusively through agent-based modeling or GDP (Krahe et al., 2016; Wang et al., 2019) to conducting surveys with stakeholders (Bakarji et al., 2017; Bano et al., 2020). Even among studies that used similar components to represent humans in sociohydrology, models varied in how those components were measured. For instance, Dodge et al. (2012) reviews and explains “well-being,” by identifying multiple definitions and indexes ranging in spatial (individual to social to global) and temporal (past, present, future, or generational well-being) scales. While the authors agree that treating humans as endogenous variables of human-water systems is important and a distinct characteristic of sociohydrology, differing interpretations of what it means for humans to be treated endogenously has led to a wide variety of research within sociohydrology that does not necessarily align with the original goals set out by Sivapalan et al. (2011) The absence of a common understanding of endogonization of humans in human-water systems leads to isolated knowledge that is not likely to accumulate.
However, the most apparent observation resulting from this systematic literature review of sociohydrology research is there are little to no norms for how to present methods, data, and results as there are in most disciplines. If sociohydrology's aim is to situate itself as its own field, then norms need to develop and be explicitly stated and followed. The authors of this review do not intend to restrict the ideas, scales, or variables used in sociohydrology. Rather, the authors intend to advocate for some level of uniformity in how sociohydrology research is communicated to improve understanding of differing methods across multiple disciplines. As it stands, sociohydrology reporting depends on the original discipline of the authors. There is risk of inconsistency in measuring and reporting scale, both spatially and temporally, and minimizing the ability to truly reach an interdisciplinary audience. Bridging the messiness that comes hand-in-hand with interdisciplinary research is not a new challenge; if scale issues are to be “resolved,” or at least mitigated as much as possible, then future studies wishing to resolve those issues may find the best approach to be starting from a common parameter of reporting and method-making. For example, the authors observed significant variety in the reporting of spatial and temporal resolution, with many primary studies not reporting these measures at all. Further, even among studies that reported both spatial and temporal scale (extent and resolution), reporting varied from general statements of study scale to detailed tables of data scale and text-based explanations of how data was integrated to achieve compatible scales for analysis (Wilhelmi and Morss, 2013). These inconsistencies point to an overarching issue—that is, there are large discrepancies between the spatial and temporal resolutions that social and hydrological factors are being monitored. Clearly, assumptions must be made to combine these datasets into some greater understanding, but the assumptions researchers made to integrate these datasets were not consistently well-documented. Establishing norms for reporting scale within the field of sociohydrology would allow for interdisciplinary audiences to better understand and critically engage in sociohydrology literature. Additionally, consistency in the reporting of spatial and temporal scale is essential addressing challenges and issues concerning scale in sociohydrology.
The authors recognize limitations in this systematic review based on several key decisions. First, the authors chose to narrow the review to only include peer-reviewed articles written in English with full texts available online. Therefore, this review neglects any sociohydrologic research presented in conference proceedings or textbooks. Only including research published in English biases the findings of the study toward Anglophone geographies and settings while underrepresenting others. Additionally, only research published after the introduction of sociohydrology by Sivapalan et al. (2011) was considered in this review. The authors recognize that other fields have studied coupled human-water systems at different scales decades before 2011, but the focus for this review limited the search timeframe to understand how coupled human-water systems are being studied since the introduction of sociohydrology. Lastly, the authors faced many challenges in identifying an appropriate classification system to summarize the main findings in the primary studies. The classification system was revised multiple times throughout the process to be as precise and inclusive as possible. However, the wide scope of data types, data sources, and methodologies found in the primary studies are likely not fully represented.
Despite these limitations, this review summarized key challenges in terms of interactions within coupled human-water systems and the varying spatial-temporal scales at which the interactions are occurring and the scale at which they are being studied. As Levy et al. (2016) titled their article “Wicked but worth it,” found that sociohydrology was “wicked” because of its unwieldy complexity and the challenges of scaling spatial-temporal boundaries and systems. Nevertheless, sociohydrology is “worth it,” the authors contended, because of sociohydrology's tremendous potential and benefit. The lead authors of this present review came to a similar conclusion, sociohydrology is multifaceted, multi-dimensional, and complex, which is needed to continue the good interdisciplinary work aiming to solve problems at the human-water nexus and incorporate feedbacks. However, if the aim of sociohydrology is unification around a more precise ontology, epistemology, and methodology of human-hydrology interactions, then sociohydrology has a way to go. This conclusion should not imply disciplinary ossification, but rather, a unified plurality aimed at solving a—nay, the—common crisis of our time.
Data Availability Statement
The raw data supporting the conclusions of this article will be made available by the authors, without undue reservation.
Author Contributions
MS conceived the presented theme. AF, JM, EN, TW, LK, and GP-Q carried out the review analysis and wrote the manuscript. SH and MS helped supervise the project. All authors contributed to the article and approved the submitted version.
Funding
This study was supported by NSF # 1828571 NRT R3. This study was supported in part by Kansas State University.
Conflict of Interest
The authors declare that the research was conducted in the absence of any commercial or financial relationships that could be construed as a potential conflict of interest.
Publisher's Note
All claims expressed in this article are solely those of the authors and do not necessarily represent those of their affiliated organizations, or those of the publisher, the editors and the reviewers. Any product that may be evaluated in this article, or claim that may be made by its manufacturer, is not guaranteed or endorsed by the publisher.
Acknowledgments
Thank you to the professors and colleagues in the NRT R3 cohort.
References
Abadie, L. M., Markandya, A., and Neumann, M. B. (2019). Accounting for economic factors in socio-hydrology: optimization under uncertainty and climate change. Water 11:2073. doi: 10.3390/w11102073
Abebe, Y. A., Ghorbani, A., Nikolic, I., Manojlovic, N., Gruhn, A., and Vojinovic, Z. (2020). The role of household adaptation measures in reducing vulnerability to flooding: a coupled agent-based and flood modelling approach. Hydrol. Earth Syst. Sci. 24, 5329–5354. doi: 10.5194/hess-24-5329-2020
Abebe, Y. A., Ghorbani, A., Nikolic, I., Vojinovic, Z., and Sanchez, A. (2019a). A coupled flood-agent-institution modelling (CLAIM) framework for urban flood risk management. Environ. Model. Software 111, 483–492. doi: 10.1016/j.envsoft.2018.10.015
Abebe, Y. A., Ghorbani, A., Nikolic, I., Vojinovic, Z., and Sanchez, A. (2019b). Flood risk management in Sint Maarten – A coupled agent-based and flood modelling method. J. Environ. Manage. 248:109317. doi: 10.1016/j.jenvman.2019.109317
Akhbari, M., and Grigg, N. S. (2013). A framework for an agent-based model to manage water resources conflicts. Water Resource Manage. 27, 4039–4052 doi: 10.1007/s11269-013-0394-0
Akhter, F., Mazzoleni, M., and Brandimarte, L. (2021). Analysis of 220 years of floodplain population dynamics in the US at different spatial scales. Water 13:141. doi: 10.3390/w13020141
Al-Amin, S., Berglund, E. Z., Mahinthakumar, G., and Larson, K. L. (2018). Assessing the effects of water restrictions on socio-hydrologic resilience for shared groundwater systems. J. Hydrol. 566, 872–885. doi: 10.1016/j.jhydrol.2018.08.045
Albertini, C., Mazzoleni, M., Totaro, V., Iacobellis, V., and Di Baldassarre, G. (2020). Socio-hydrological modelling: the influence of reservoir management and societal responses on flood impacts. Water 12:1384. doi: 10.5194/egusphere-egu2020-673
Asbjornsen, H., Mayer, A. S., Jones, K. W., Selfa, T., Saenz, L., Kolka, R. K., et al. (2015). Assessing impacts of payments for watershed services on sustainability in coupled human and natural systems. Bioscience 65, 579–591. doi: 10.1093/biosci/biv051
Bakarji, J., O'Malley, D., and Vesselinov, V. V. (2017). Agent-based socio-hydrological hybrid modeling for water resource management. Water Resources Manage. 31, 3881–3898. doi: 10.1007/s11269-017-1713-7
Baker, T. J., Cullen, B., Debevec, L., and Abebe, Y. (2015). A socio-hydrological approach for incorporating gender into biophysical models and implications for water resources research. Appl. Geogr. 62, 325–338. doi: 10.1016/j.apgeog.2015.05.008
Bano, R., Khiadani, M., and Burian, S. (2020). Socio-hydrological modelling to assess reliability of an urban water system under formal-informal supply dynamics. Water 12:2795. doi: 10.3390/w12102795
Barendrecht, M. H., Viglione, A., Kreibich, H., Merz, B., Vorogushyn, S., and Blöschl, G. (2019). The value of empirical data for estimating the parameters of a sociohydrological flood risk model. Water Resour. Res. 55, 1312–1336. doi: 10.1029/2018WR024128
Bark, R. H., Robinson, C. J., and Flessa, K. W. (2016). Tracking cultural ecosystem services: water chasing the Colorado River restoration pulse flow. Ecol. Econ. 127, 165–172. doi: 10.1016/j.ecolecon.2016.03.009
Blair and Buytaert (2016). Socio-hydrological modelling: a review asking “why, what and how?” Hydrol. Earth Syst. Sci. 20, 443–478. doi: 10.5194/hess-20-443-2016
Blair, P., and Buytaert, W. (2015). Modeling socio-hydrological systems: a review of concepts, approaches, and applications. Hydrol. Earth Syst. Sci. Discus. 12, 18761–18851. doi: 10.5194/hessd-12-8761-2015
Bojórquez-Tapia, L. A., Janssen, M., Eakin, H., Baeza, A., Serrano-Candela, F., Gómez-Priego, P., et al. (2019). Spatially-explicit simulation of two-way coupling of complex socio-environmental systems: Socio-hydrological risk and decision making in Mexico City. Socio Environ. Syst. Model. 1, 1–15. doi: 10.18174/sesmo.2019a16129
Bou Nassar, J. A., Malard, J. J., Adamowski, J. F., Ramírez Ramírez, M., Medema, W., and Tuy, H. (2021). Multi-level storylines for participatory modeling – involving marginalized communities in Tz'olöj Ya', Mayan Guatemala. Hydrol. Earth Syst. Sci. 25, 1283–1306. doi: 10.5194/hess-25-1283-2021
Bouziotas, D., and Ertsen, M. (2017). Socio-hydrology from the bottom up: a template for agent-based modeling in irrigation systems. Hydrol. Earth Syst. Sci. Discus. 1–27. doi: 10.5194/hess-2017-107
Braden, J., Jolejole-Foreman, M., and Schneider, D. (2014). Humans and the water environment: the need for coordinated data collection. Water 6, 1–16. doi: 10.3390/w6010001
Bradford, L., Thapa, A., Duffy, A., Hassanzadeh, E., Strickert, G., Noble, B., et al. (2020). Incorporating social dimensions in hydrological and water quality modeling to evaluate the effectiveness of agricultural beneficial management practices in a Prairie River Basin. Environ. Sci. Pollut. Res. 27, 14271–14287. doi: 10.1007/s11356-019-06325-1
Breyer, B., Zipper, S. C., and Qiu, J. (2018). Sociohydrological impacts of water conservation under anthropogenic drought in Austin, TX (USA). Water Resour. Res. 54, 3062–3080. doi: 10.1002/2017WR021155
Bury, J., Mark, B. G., Carey, M., Young, K. R., McKenzie, J. M., Baraer, M., et al. (2013). New geographies of water and climate change in peru: coupled natural and social transformations in the santa river watershed. Ann. Assoc. Am. Geogr. 103, 363–374. doi: 10.1080/00045608.2013.754665
Camargo, A. (2017). Land born of water: property, stasis, and motion in the floodplains of northern Colombia. Geoforum. 1–9. doi: 10.1016/j.geoforum.2017.11.006
Caprario, J., and Finotti, A. R. (2019). Socio-technological tool for mapping susceptibility to urban flooding. J. Hydrol. 574, 1152–1163. doi: 10.1016/j.jhydrol.2019.05.005
Chang, L.-F., and Huang, S.-L. (2015). Assessing urban flooding vulnerability with an emergy approach | Elsevier Enhanced Reader. Landsc. Urban Plan. 143, 11–24. doi: 10.1016/j.landurbplan.2015.06.004
Chen, X., Wang, D., Tian, F., and Sivapalan, M. (2016). From channelization to restoration: sociohydrologic modeling with changing community preferences in the Kissimmee River Basin, Florida. Water Resour. Res. 52, 1227–1244. doi: 10.1002/2015WR018194
Cian, F., Giupponi, C., and Marconcini, M. (2021). Integration of earth observation and census data for mapping a multi-temporal flood vulnerability index: a case study on Northeast Italy. Nat. Hazards 106, 2163–2184. doi: 10.1007/s11069-021-04535-w
Ciullo, A., Viglione, A., Castellarin, A., Crisci, M., and Di Baldassarre, G. (2017). Socio-hydrological modelling of flood-risk dynamics: comparing the resilience of green and technological systems (rayyan-142092706). Hydrol. Sci. J. 62, 880–891. doi: 10.1080/02626667.2016.1273527
Cobourn, K. M., Carey, C. C., Boyle, K. J., Duffy, C., Dugan, H. A., Farrell, K. J., et al. (2018). From concept to practice to policy: modeling coupled natural and human systems in lake catchments. Ecosphere 9, 1–15. doi: 10.1002/ecs2.2209
Congalton, R. G. (1997). Exploring and evaluating the consequences of vector-to-raster and raster-to-vector conversion. Photogramm. Eng. Remote Sensing 63, 425–434.
Coutros, P. R. (2019). A fluid past: socio-hydrological systems of the West African Sahel across the long durée. WIREs Water 6, 1–22. doi: 10.1002/wat2.1365
Den Besten, N. I., Pande, S., and Savenije, H. H. G. (2016). A socio-hydrological comparative assessment explaining regional variances in suicide rate amongst farmers in Maharashtra, India. Proc. Int. Assoc. Hydrol. Sci. 373, 115–118. doi: 10.5194/piahs-373-115-2016
Devkota, R. (2020). Assessing the past and adapting to future floods: a hydro-social analysis. Clim. Change. 163:18. doi: 10.1007/s10584-020-02909-w
Di Baldassarre, G., Kooy, M., Kemerink, J. S., and Brandimarte, L. (2013). Towards understanding the dynamic behaviour of floodplains as human-water systems. Hydrol. Earth Syst. Sci. 17, 3235–3244. doi: 10.5194/hess-17-3235-2013
Di Baldassarre, G., Martinez, F., Kalantari, Z., and Viglione, A. (2016). Drought and flood in the anthropocene: modelling feedback mechanisms. Earth Syst. Dynam. Discus. 1–24. doi: 10.5194/esd-2016-65
Di Baldassarre, G., Sacc,à, S., Aronica, G. T., Grimaldi, S., Ciullo, A., and Crisci, M. (2017). Human-flood interactions in Rome over the past 150 years. Adv. Geosci. 44, 9–13. doi: 10.5194/adgeo-44-9-2017
Di Baldassarre, G., Sivapalan, M., Rusca, M., Cudennec, C., Garcia, M., Kreibich, H., et al. (2019). Sociohydrology: scientific challenges in addressing the sustainable development goals. Water Resour. Res. 55, 6327–6355. doi: 10.1029/2018WR023901
Di Baldassarre, G., Viglione, A., Carr, G., Kuil, L., Yan, K., Brandimarte, L., et al. (2015). Debates-Perspectives on sociohydrology: capturing feedbacks between physical and social processes: a socio-hydrological approach to explore flood risk changes. Water Resour. Res. 51, 4770–4781. doi: 10.1002/2014WR016416
Di Baldassarre, G., Yan, K., Ferdous, Md. R., and Brandimarte, L. (2014). The interplay between human population dynamics and flooding in Bangladesh: a spatial analysis. Proc. Int. Assoc. Hydrol. Sci. 364, 188–191. doi: 10.5194/piahs-364-188-2014
Ding, Z., Gong, W., Li, S., and Wu, Z. (2018). System dynamics versus agent-based modeling: a review of complexity simulation in construction waste management. Sustainability 10:2484. doi: 10.3390/su10072484
Dodge, R., Daly, A., Huyton, J., and Sanders, L. (2012). The challenge of defining wellbeing. Int. J. Wellbeing 2, 222–235. doi: 10.5502/ijw.v2i3.4
Du, E., Tian, Y., Cai, X., Zheng, Y., Li, X., and Zheng, C. (2020). Exploring spatial heterogeneity and temporal dynamics of human-hydrological interactions in large river basins with intensive agriculture: a tightly coupled, fully integrated modeling approach. J. Hydrol. 591:125313. doi: 10.1016/j.jhydrol.2020.125313
Dziubanski, D., Franz, K. J., and Gutowski, W. (2020). Linking economic and social factors to peak flows in an agricultural watershed using socio-hydrologic modeling. Hydrol. Earth Syst. Sci. 24, 2873–2894. doi: 10.5194/hess-24-2873-2020
Ekblad, L., and Herman, J. D. (2021). Toward data-driven generation and evaluation of model structure for integrated representations of human behavior in water resources systems. Water Resources Res. 57:e2020WR028148. doi: 10.1029/2020WR028148
Elagib, N. A., Musa, A. A., and Sulieman H. M. (2017). “Socio-hydrological framework of farmer-drought feedback: Darfur as a case study,” in Water Resources in Arid Areas: The Way Forward, eds O. Abdalla, A. Kacimov, M. Chen, A. Al-Maktoumi, T. Al-Hosni, and I. Clark (Cham: Springer Water Springer).
Elshafei, Y., Coletti, J. Z., Sivapalan, M., and Hipsey, M. R. (2015). A model of the socio-hydrologic dynamics in a semiarid catchment: isolating feedbacks in the coupled human-hydrology system. Water Resour. Res. 51, 6442–6471. doi: 10.1002/2015WR017048
Elshafei, Y., Sivapalan, M., Tonts, M., and Hipsey, M. R. (2014). A prototype framework for models of socio-hydrology: identification of key feedback loops and parameterisation approach. Hydrol. Earth Syst. Sci. 18, 2141–2166. doi: 10.5194/hess-18-2141-2014
Elshafei, Y., Tonts, M., Sivapalan, M., and Hipsey, M. R. (2016). Sensitivity of emergent sociohydrologic dynamics to internal system properties and external sociopolitical factors: Implications for water management. Water Resour. Res. 52, 4944–4966. doi: 10.1002/2015WR017944
Ertsen, M. W., Murphy, J. T., Purdue, L. E., and Zhu, T. (2014). A journey of a thousand miles begins with one small step – human agency, hydrological processes and time in socio-hydrology. Hydrol. Earth Syst. Sci. 18, 1369–1382. doi: 10.5194/hess-18-1369-2014
Essenfelder, A. H., Pérez-Blanco, C. D., and Mayer, A. S. (2018). Rationalizing systems analysis for the evaluation of adaptation strategies in complex human-water systems. Earths Future 6, 1181–1206. doi: 10.1029/2018EF000826
Falkenmark, M. (1979). Main problems of water use and transfer of technology. GeoJournal 3, 435–443. doi: 10.1007/BF00455982
Farjad, B., Pooyandeh, M., Gupta, A., Motamedi, M., and Marceau, D. (2017). Modelling interactions between land use, climate, and hydrology along with stakeholders' negotiation for water resources management. Sustainability 9:2022. doi: 10.3390/su9112022
Faust, K. M., Abraham, D. M., and DeLaurentis, D. (2017). Coupled human and water infrastructure systems sector interdependencies: framework evaluating the impact of cities experiencing urban decline. J. Water Resources Plan. Manage. 143:04017043. doi: 10.1061/(ASCE)WR.1943-5452.0000794
Ferdous, M. R., Di Baldassarre, G., Brandimarte, L., and Wesselink, A. (2020). The interplay between structural flood protection, population density, and flood mortality along the Jamuna River, Bangladesh. Region. Environ. Change 20:1–9. doi: 10.1007/s10113-020-01600-1
Ferdous, M. R., Wesselink, A., Brandimarte, L., Slager, K., Zwarteveen, M., and Di Baldassarre, G. (2018). Socio-hydrological spaces in the Jamuna River floodplain in Bangladesh. Hydrol. Earth Syst. Sci. 22, 5159–5173. doi: 10.5194/hess-22-5159-2018
Ferreira, S., and Ghimire, R. (2012). Forest cover, socioeconomics, and reported flood frequency in developing countries. Water Resour. Res. 48:1–13. doi: 10.1029/2011WR011701
Garcia, D. J., and You, F. (2016). The water-energy-food nexus and process systems engineering: a new focus. Comput. Chem. Eng. 91, 49–67. doi: 10.1016/j.compchemeng.2016.03.003
Garcia, M., and Islam, S. (2018). The role of external and emergent drivers of water use change in Las Vegas. Urban Water J. 15, 888–898. doi: 10.1080/1573062X.2019.1581232
Garcia, M., Portney, K., and Islam, S. (2016). A question driven socio-hydrological modeling process. Hydrol. Earth Syst. Sci. 20, 73–92. doi: 10.5194/hess-20-73-2016
Gholizadeh Sarabi, S., Davary, K., Ghahraman, B., and Shafiei, M. (2021). A perceptual socio-hydrological model of co-evolutionary coupled human–water system based on historical analysis, Mashhad basin, Iran. Hydrol. Sci. J. 66, 355–372. doi: 10.1080/02626667.2021.1873345
Giuliani, M., Li, Y., Castelletti, A., and Gandolfi, C. (2016). A coupled human-natural systems analysis of irrigated agriculture under changing climate. Water Resour. Res. 52, 6928–6947. doi: 10.1002/2016WR019363
Gober, P., White, D. D., Quay, R., Sampson, D. A., and Kirkwood, C. W. (2014). Socio-hydrology modeling for an uncertain future, with examples from the USA and Canada. Geol. Soc. 408, 183–199. doi: 10.1144/SP408.2
Gonzales, P., and Ajami, N. (2017). Social and structural patterns of drought-related water conservation and rebound. Water Resour. Res. 53, 10619–10634. doi: 10.1002/2017WR021852
Grames, J., Prskawetz, A., Grass, D., Viglione, A., and Blöschl, G. (2016). Modeling the interaction between flooding events and economic growth. Ecol. Econ. 129, 193–209. doi: 10.1016/j.ecolecon.2016.06.014
Gunda, T., Turner, B. L., and Tidwell, V. C. (2018). The influential role of sociocultural feedbacks on community-managed irrigation system behaviors during times of water stress. Water Resour. Res. 54, 2697–2714. doi: 10.1002/2017WR021223
Haeffner, M., Galvin, K., and Vázquez, A. E. G. (2017). Urban water development in La Paz, Mexico 1960-present: a hydrosocial perspective. Water Hist. 9, 169–187. doi: 10.1007/s12685-016-0180-z
Haeffner, M., and Hellman, D. (2020). The social geometry of collaborative flood risk management: a hydrosocial case study of Tillamook County, Oregon. Nat. Hazards 103, 3303–3325. doi: 10.1007/s11069-020-04131-4
Haer, T., Botzen, W. J. W., and Aerts, J. C. J. H. (2019). Advancing disaster policies by integrating dynamic adaptive behaviour in risk assessments using an agent-based modelling approach. Environ. Res. Lett. 14:044022. doi: 10.1088/1748-9326/ab0770
Han, S., Tian, F., Liu, Y., and Duan, X. (2017). Socio-hydrological perspectives of the co-evolution of humans and groundwater in Cangzhou, North China Plain. Hydrol. Earth Syst. Sci. 21, 3619–3633. doi: 10.5194/hess-21-3619-2017
Hannah, D. M., Wood, P. J., and Sadler, J. P. (2004). Ecohydrology and hydroecology: a ‘new paradigm’? Hydrol. Process. 18, 3439–3445. doi: 10.1002/hyp.5761
Hanus, K. (2021). The social logic of irrigation. Accessibility based approach towards evaluating the water management infrastructure of the archaeological site of Miran. Archaeol. Prospect. 28, 63–72. doi: 10.1002/arp.1797
Hazarika, N., Tayeng, T., and Das, A. K. (2016). Living in troubled waters: Stakeholders' perception, susceptibility and adaptations to flooding in the Upper Brahmaputra plain. Nat. Hazards 83, 1157–1176. doi: 10.1007/s11069-016-2366-1
Höllermann, B., and Evers, M. (2020). Identifying the sensitivity of complex human-water systems using a qualitative systems approach. Front. Water 2:25. doi: 10.3389/frwa.2020.00025
Horn, F., and Elagib, N. A. (2018). Building socio-hydrological resilient cities against flash floods: key challenges and a practical plan for arid regions. J. Hydrol. 564, 125–132. doi: 10.1016/j.jhydrol.2018.07.001
Hossain, M. B., and Mertig, A. G. (2020). Socio-structural forces predicting global water footprint: socio-hydrology and ecologically unequal exchange. Hydrol. Sci. J. 65, 495–506. doi: 10.1080/02626667.2020.1714052
Hossain, M. S., Ramirez, J. A., Haisch, T., Speranza, C. I., Martius, O., Mayer, H., et al. (2020). A coupled human and landscape conceptual model of risk and resilience in Swiss Alpine communities. Sci. Total Environ. 730:138322. doi: 10.1016/j.scitotenv.2020.138322
Hou, B., Yang, R., Zhou, Y., Xiao, W., Wang, J., Zhao, Y., et al. (2019). Evolution mechanisms and fundamental equations of social water cycle fluxes. Hydrol. Res. 50, 1344–1358. doi: 10.2166/nh.2019.115
Hough, M., Pavao-Zuckerman, M. A., and Scott, C. A. (2018). Connecting plant traits and social perceptions in riparian systems: ecosystem services as indicators of thresholds in social-ecohydrological systems. J. Hydrol. 566, 860–871. doi: 10.1016/j.jhydrol.2018.08.005
Hu, Y., Garcia-Cabrejo, O., Cai, X., Valocchi, A. J., and DuPont, B. (2015). Global sensitivity analysis for large-scale socio-hydrological models using Hadoop. Environ. Model. Software 73, 231–243. doi: 10.1016/j.envsoft.2015.08.015
Huang, D., Li, G., Liu, Q., and Sun, C. (2020). Exploring interactions in the local water-energy-food nexus (WEF-Nexus) using a simultaneous equations model. Sci. Total Environ. 703:135034. doi: 10.1016/j.scitotenv.2019.135034
Huber, L., Bahro, N., Leitinger, G., Tappeiner, U., and Strasser, U. (2019). Agent-based modelling of a coupled water demand and supply system at the catchment scale. Sustainability 11:6178. doi: 10.3390/su11216178
Iwanaga, T., Zare, F., Croke, B., Fu, B., Merritt, W., Partington, D., et al. (2018). Development of an integrated model for the Campaspe catchment: a tool to help improve understanding of the interaction between society, policy, farming decision, ecology, hydrology and climate. Proc. Int. Assoc. Hydrol. Sci. 379, 1–12. doi: 10.5194/piahs-379-1-2018
Jeffrey, P., and Gearey, M. (2006). Integrated water resources management: lost on the road from ambition to realisation? Water Sci. Technol. 53, 1–8. doi: 10.2166/wst.2006.001
Jeong, H., and Adamowski, J. (2016). A system dynamics based socio-hydrological model for agricultural wastewater reuse at the watershed scale. Agric. Water Manage. 171, 89–107. doi: 10.1016/j.agwat.2016.03.019
Kelly, R. A., Jakeman, A. J., Barreteau, O., Borusk, M. E., ElSawah, S., Hamilton, S. H., et al. (2013). Selecting among five common modelling approaches for integrated environmental assessment and management. Environ. Model. Softw. 47, 159–181. doi: 10.1016/j.envsoft.2013.05.005
Keys, P. W., and Wang-Erlandsson, L. (2018). On the social dynamics of moisture recycling. Earth Syst. Dynam. 9, 829–847. doi: 10.5194/esd-9-829-2018
Khalifa, M., Elagib, N. A., Ahmed, B. M., Ribbe, L., and Schneider, K. (2020). Exploring socio-hydrological determinants of crop yield in under-performing irrigation schemes: pathways for sustainable intensification. Hydrol. Sci. J. 65, 153–168. doi: 10.1080/02626667.2019.1688333
Khan, F., Pilz, J., and Ali, S. (2017). Improved hydrological projections and reservoir management in the Upper Indus Basin under the changing climate. Water Environ. J. 31, 235–244. doi: 10.1111/wej.12237
Kotir, J. H., Brown, G., Marshall, N., and Johnstone, R. (2017). Systemic feedback modelling for sustainable water resources management and agricultural development: an application of participatory modelling approach in the Volta River Basin. Environ. Model. Software 88, 106–118. doi: 10.1016/j.envsoft.2016.11.015
Krahe, P., Nilson, E., Knoche, M., and Eschenback, A. E. (2016). Modeling human-water-systems: towards a comprehensive and spatially distributed assessment of co-evolutions for river basins in Central Europe. Procc. IAHS 373, 119–123. doi: 10.5194/piahs-373-119-2016
Kuil, L., Carr, G., Viglione, A., Prskawetz, A., and Blöschl, G. (2016). Conceptualizing socio-hydrological drought processes: the case of the Maya collapse. Water Resour. Res. 52, 6222–6242. doi: 10.1002/2015WR018298
Kuil, L., Evans, T., McCord, P. F., Salinas, J. L., and Blöschl, G. (2018). Exploring the influence of smallholders' perceptions regarding water availability on crop choice and water allocation through socio-hydrological modeling. Water Resour. Res. 54, 2580–2604. doi: 10.1002/2017WR021420
Kumar, P., Avtar, R., Dasgupta, R., Johnson, B. A., Mukherjee, A., Ahsan, M. N., et al. (2020). Socio-hydrology: a key approach for adaptation to water scarcity and achieving human well-being in large riverine islands. Progress Disaster Sci. 8:100134. doi: 10.1016/j.pdisas.2020.100134
Kundzewicz, Z. W. (2002). Ecohydrology—Seeking consensus on interpretation of the notion/Ecohydrologie—À la recherche d'un consensus sur l'interprétation de la notion. Hydrol. Sci. J. 47, 799–804. doi: 10.1080/02626660209492982
Lee, S., and Kang, D. (2020). Analyzing the effectiveness of a multi-purpose dam using a system dynamics model. Water 12:1062. doi: 10.3390/w12041062
Lei, X., Zhao, J., Wang, D., and Sivapalan, M. (2018). A Budyko-type model for human water consumption. J. Hydrol. 567, 212–226. doi: 10.1016/j.jhydrol.2018.10.021
Levy, M. C., Garcia, M., Blair, P., Chen, X., Gomes, S. L., Gower, D. B., et al. (2016). Wicked but worth it: student perspectives on socio-hydrology. Hydrol. Process. 30, 1467–1472. doi: 10.1002/hyp.10791
Li, B., and Sivapalan, M. (2020). Long-term coevolution of an urban human-water system under climate change: Critical role of human adaptive actions. Water Resource Res. 55:e2020WR027931. doi: 10.1029/2020WR027931
Li, B., Sivapalan, M., and Xu, X. (2019). An urban sociohydrologic model for exploration of Beijing's water sustainability challenges and solution spaces. Water Resour. Res. 55, 5918–5940. doi: 10.1029/2018WR023816
Liu, D. (2019). Evaluating the dynamic resilience process of a regional water resource system through the nexus approach and resilience routing analysis. J. Hydrol. 578:124028. doi: 10.1016/j.jhydrol.2019.124028
Liu, D., Tian, F., Lin, M., and Sivapalan, M. (2015). A conceptual socio-hydrological model of the co-evolution of humans and water: case study of the Tarim River basin, western China. Hydrol. Earth Syst. Sci. 19, 1035–1054. doi: 10.5194/hess-19-1035-2015
Liu, Y., Tian, F., Hu, H., and Sivapalan, M. (2014). Socio-hydrologic perspectives of the co-evolution of humans and water in the Tarim River basin, Western China: the Taiji–Tire model. Hydrol. Earth Syst. Sci. 18, 1289–1303. doi: 10.5194/hess-18-1289-2014
Liu, Y., Wang, S., and Chen, B. (2019). Water–land nexus in food trade based on ecological network analysis. Ecol. Indicat. 97, 466–475. doi: 10.1016/j.ecolind.2018.10.042
Logan, T. M., Guikema, S. D., and Bricker, J. D. (2018). Hard-adaptive measures can increase vulnerability to storm surge and tsunami hazards over time. Nat. Sustain. 1, 526–530. doi: 10.1038/s41893-018-0137-6
Lu, Y., Tian, F., Guo, L., Borz,ì, I., Patil, R., Wei, J., et al. (2021). Socio-hydrologic modeling of the dynamics of cooperation in the transboundary Lancang–Mekong River. Hydrol. Earth Syst. Sci. 25, 1883–1903. doi: 10.5194/hess-25-1883-2021
Lu, Z., Wei, Y., Feng, Q., Xiao, H., and Cheng, G. (2016). Progress on socio-hydrology. Shuikexue Jinzhan Adv. Water Sci. 27, 772–783.
Lu, Z., Wei, Y., Feng, Q., Xie, J., Xiao, H., and Cheng, G. (2018). Co-evolutionary dynamics of the human-environment system in the Heihe River basin in the past 2000years. Sci. Total Environ. 635, 412–422. doi: 10.1016/j.scitotenv.2018.03.231
Luo, Z., and Zuo, Q. (2019). Evaluating the coordinated development of social economy, water, and ecology in a heavily disturbed basin based on the distributed hydrology model and the harmony theory. J. Hydrol. 574, 226–241. doi: 10.1016/j.jhydrol.2019.04.042
Madani, K., and Shafiee-Jood, M. (2020). Socio-hydrology: a new understanding to unite or a new science to divide? Water Water 12:1941. doi: 10.3390/w12071941
Martin, E., Gascoin, S., Grusson, Y., Murgue, C., Bardeau, M., Anctil, F., et al. (2016). On the use of hydrological models and satellite data to study the water budget of river basins affected by human activities: examples from the Garonne Basin of France. Surveys Geophys. 37, 223–247. doi: 10.1007/s10712-016-9366-2
Medeiros, P., and Sivapalan, M. (2020). From hard-path to soft-path solutions: slow–fast dynamics of human adaptation to droughts in a water scarce environment. Hydrol. Sci. J. 65, 1803–1814. doi: 10.1080/02626667.2020.1770258
Michaelis, T., Brandimarte, L., and Mazzoleni, M. (2020). Capturing flood-risk dynamics with a coupled agent-based and hydraulic modelling framework. Hydrol. Sci. J. 65, 1458–1473. doi: 10.1080/02626667.2020.1750617
Moher, D., Liberati, A., Tetzlaff, J., Altman, D. G., and The PRISMA Group. (2009). Preferred reporting items for systematic reviews and meta-analyses: the PRISMA statement. PLoS Med. 6:e1000097. doi: 10.1371/journal.pmed.1000097
Moja, L. P., Telaro, E., D'Amico, R., Moschetti, I., Coe, L., and Liberati, A. (2005). Assessment of methodological quality of primary studies by systematic reviews: results of the metaquality cross sectional study. BMJ 330:1053. doi: 10.1136/bmj.38414.515938.8F
Mostert, E. (2017). An alternative approach for socio-hydrology: case study research. Hydrol. Earth Syst. Sci. 22, 317–329. doi: 10.5194/hess-2017-299
Noël, P. H., and Cai, X. (2017). On the role of individuals in models of coupled human and natural systems: lessons from a case study in the Republican River Basin. Environ. Model. Software 92, 1–16. doi: 10.1016/j.envsoft.2017.02.010
Nüsser, M., Dame, J., Kraus, B., Baghel, R., and Schmidt, S. (2019). Socio-hydrology of “artificial glaciers” in Ladakh, India: assessing adaptive strategies in a changing cryosphere. Region. Environ. Change 19, 1327–1337. doi: 10.1007/s10113-018-1372-0
Nüsser, M., and Schmidt, S. (2017). Nanga parbat revisited: evolution and dynamics of sociohydrological interactions in the Northwestern Himalaya. Ann. Am. Assoc. Geogr. 107, 403–415. doi: 10.1080/24694452.2016.1235495
Nüsser, M., Schmidt, S., and Dame, J. (2012). Irrigation and development in the upper Indus basin: characteristics and recent changes of a socio-hydrological system in central Ladakh, India. Mountain Res. Dev. 32, 51–62. doi: 10.1659/MRD-JOURNAL-D-11-00091.1
O'Connell, P. E., and O'Donnell, G. (2014). Towards modelling flood protection investment as a coupled human and natural system. Hydrol. Earth Syst. Sci. 18, 155–171. doi: 10.5194/hess-18-155-2014
Ogilvie, A., Riaux, J., Massuel, S., Mulligan, M., Belaud, G., Le Goulven, P., et al. (2019). Socio-hydrological drivers of agricultural water use in small reservoirs. Agric. Water Manage. 218, 17–29. doi: 10.1016/j.agwat.2019.03.001
O'Keeffe, J., Moulds, S., Bergin, E., Brozovi,ć, N., Mijic, A., and Buytaert, W. (2018). Including farmer irrigation behavior in a sociohydrological modeling framework with application in North India. Water Resources Res. 54, 4849–4866. doi: 10.1029/2018WR023038
O'Keeffe, J., Moulds, S., Scheidegger, J. M., Jackson, C. R., Nair, T., and Mijic, A. (2020). Isolating the impacts of anthropogenic water use within the hydrological regime of north India. Earth Surface Processes Landforms 45, 1217–1228. doi: 10.1002/esp.4799
Oneda, T. M. S., and Barros, V. G. (2021). On stormwater management master plans: comparing developed and developing cities. Hydrol. Sci. J. 66, 1–11. doi: 10.1080/02626667.2020.1853131
Ouzzani, M., Hammady, H., Fedorowicz, Z., and Elmagarmid, A. (2016). Rayyan — a web and mobile app for systematic reviews. Syst. Rev. 5:210. doi: 10.1186/s13643-016-0384-4
Padowski, J. C., Gorelick, S. M., Thompson, B. H., Rozelle, S., and Fendorf, S. (2015). Assessment of human–natural system characteristics influencing global freshwater supply vulnerability. Environ. Res. Lett. 10:104014. doi: 10.1088/1748-9326/10/10/104014
Pande, S., and Ertsen, M. (2014). Endogenous change: on cooperation and water availability in two ancient societies. Hydrol. Earth Syst. Sci. 18, 1745–1760. doi: 10.5194/hess-18-1745-2014
Pande, S., and Sivapalan, M. (2017). Progress in socio-hydrology: a meta-analysis of challenges and opportunities: progress in socio-hydrology. Wiley Interdiscip. Rev. Water 4:e1193. doi: 10.1002/wat2.1193
Parolari, A. J., and Manoli, G. (2019). Power law growth and delayed feedbacks in socio-hydrological systems. Earths Future 7, 1220–1231. doi: 10.1029/2019EF001185
Pouladi, P., Afshar, A., Afshar, M. H., Molajou, A., and Farahmand, H. (2019). Agent-based socio-hydrological modeling for restoration of Urmia Lake: application of theory of planned behavior. J. Hydrol. 576, 736–748. doi: 10.1016/j.jhydrol.2019.06.080
Pramana, K. E. R., and Ertsen, M. W. (2016). Towards systematic planning of small-scale hydrological intervention-basedresearch. Hydrol. Earth Syst. Sci. 20, 4093–4115. doi: 10.5194/hess-20-4093-2016
Purdue, L. E., and Berger, J.-F. (2015). An integrated socio-environmental approach to the study of ancient water systems: the case of prehistoric Hohokam irrigation systems in semi-arid central Arizona, USA. J. Archaeol. Sci. 53, 586–603. doi: 10.1016/j.jas.2014.11.008
Puzyreva, K., and de Vries, D. H. (2021). ‘A low and watery place’: a case study of flood history and sustainable community engagement in flood risk management in the County of Berkshire, England. Int. J. Disaster Risk Reduct. 52:101980. doi: 10.1016/j.ijdrr.2020.101980
Re, V., Sacchi, E., Kammoun, S., Tringali, C., Trabelsi, R., Zouari, K., et al. (2017). Integrated socio-hydrogeological approach to tackle nitrate contamination in groundwater resources. The case of Grombalia Basin (Tunisia). Sci. Total Environ. 593–594, 664–676. doi: 10.1016/j.scitotenv.2017.03.151
Riaux, J., Ogilvie, A., and Jenhaoui, Z. (2020). More than just water! Hydraulic materiality and the process of resource making: a sociohydroligcal reading of Tunisian hillside reservoirs. J. Rural Stud. 79, 125–135. doi: 10.1016/j.jrurstud.2020.08.041
Ridolfi, E., Albrecht, F., and Di Baldassarre, G. (2020a). Exploring the role of risk perception in influencing flood losses over time. Hydrol. Sci. J. 65, 12–20. doi: 10.1080/02626667.2019.1677907
Ridolfi, E., Mondino, E., and Di Baldassarre, G. (2020b). Hydrological risk: modeling flood memory and human proximity to rivers. Hydrol. Res. 52, 241–252. doi: 10.2166/nh.2020.195
Robinne, F.-N., Bladon, K. D., Miller, C., Parisien, M.-A., Mathieu, J., and Flannigan, M. D. (2018). A spatial evaluation of global wildfire-water risks to human and natural systems. Sci. Total Environ. 610–611, 1193–1206. doi: 10.1016/j.scitotenv.2017.08.112
Roobavannan, M., Emmerik, T. H. M., Elshafei, Y., Kandasamy, J., Sanderson, M. R., Vigneswaran, S., et al. (2018). Norms and values in sociohydrological models. Hydrol. Earth Syst. Sci. 22, 1337–1349. doi: 10.5194/hess-22-1337-2018
Roobavannan, M., Kandasamy, J., Pande, S., Vigneswaran, S., and Sivapalan, M. (2017). Allocating environmental water and impact on basin unemployment: role of a diversified economy. Ecol. Econ. 136, 178–188. doi: 10.1016/j.ecolecon.2017.02.006
Roobavannan, M., Kandasamy, J., Pande, S., Vigneswaran, S., and Sivapalan, M. (2020). Sustainability of agricultural basin development under uncertain future climate and economic conditions: a socio-hydrological analysis. Ecol. Econ. 174:106665. doi: 10.1016/j.ecolecon.2020.106665
Ross, A., and Chang, H. (2020). Socio-hydrology with hydrosocial theory: two sides of the same coin? Hydrol. Sci. J. 65, 1443–1457. doi: 10.1080/02626667.2020.1761023
Saia, S. M., Suttles, K. M., Cutts, B. B., Emanuel, R. E., Martin, K. L., Wear, D. N., et al. (2020). Applying climate change risk management tools to integrate streamflow projections and social vulnerability. Ecosystems 23, 67–83. doi: 10.1007/s10021-019-00387-5
Sanderson, M. R. (2018). Everything flows…unevenly: Social stratification in coupled socio-ecological systems. Curr. Opin. Environ. Sustain. 33, 51–57. doi: 10.1016/j.cosust.2018.04.012
Sanderson, M. R., Bergtold, J. S., Heier Stamm, J. L., Caldas, M. M., and Ramsey, S. M. (2017). Bringing the “social” into sociohydrology: conservation policy support in the Central Great Plains of Kansas, USA: bringing the social into sociohydrology. Water Resources Res. 53, 6725–6743. doi: 10.1002/2017WR020659
Sarmento Buarque, A. C., Bhattacharya-Mis, N., Fava, M. C., Souza, F. A. A. D., and Mendiondo, E. M. (2020). Using historical source data to understand urban flood risk: a socio-hydrological modelling application at Gregório Creek, Brazil. Hydrol. Sci. J. 65, 1075–1083. doi: 10.1080/02626667.2020.1740705
Savelli, E., Rusca, M., Cloke, H., and Di Baldassarre, G. (2021). Don't blame the rain: social power and the 2015–2017 drought in Cape Town. J. Hydrol. 594:125953. doi: 10.1016/j.jhydrol.2020.125953
Sawada, Y., and Hanazaki, R. (2020). Socio-hydrological data assimilation: analyzing human-flood interactions by model-data integration. Hydrol. Earth Syst. Sci. 24, 4777–4777. doi: 10.5194/hess-24-4777-2020
Schifman, L. A., Ossola, A., Herrmann, D. L., Shuster, W. D., Garmestani, A., and Hopton, M. E. (2017). Situating green infrastructure in context: a framework for adaptive socio-hydrology in cities. Water Resources Res. 53, 10139–10154. doi: 10.1002/2017WR020926
Schumann, A., and Nijssen, d. (2014). Shortage and surplus of water in the socio-hydrological context. Proc. Int. Assoc. Hydrol. Sci. 364, 292–298. doi: 10.5194/piahs-364-292-2014
Shelton, R. E., Baeza, A., Janssen, M. A., and Eakin, H. (2018). Managing household socio-hydrological risk in Mexico city: a game to communicate and validate computational modeling with stakeholders. J. Environ. Manage. 227, 200–208. doi: 10.1016/j.jenvman.2018.08.094
Sivakumar, B. (2012). Socio-hydrology: not a new science, but a recycled and re-worded hydrosociology. Hydrol. Proces. 26, 3788–3790. doi: 10.1002/hyp.9511
Sivapalan, M. (2015). Debates-perspectives on socio-hydrology: changing water systems and the “tyranny of small problems”-socio-hydrology: changing water systems and the “tyranny of small problems.” Water Resources Res. 51, 4795–4805. doi: 10.1002/2015WR017080
Sivapalan, M., Savenije, H. H. G., and Blöschl, G. (2011). Socio-hydrology: a new science of people and water: invited commentary. Hydrol. Proces. 26, 1270–1276. doi: 10.1002/hyp.8426
Song, J., Yin, Y., Xu, H., Wang, Y., Wu, P., and Sun, S. (2020). Drivers of domestic grain virtual water flow: a study for China. Agric. Water Manage. 239:106175. doi: 10.1016/j.agwat.2020.106175
Souza, F. A. A., Bhattacharya-Mis, N., Restrepo-Estrada, C., Gober, P., Taffarello, D., Tundisi, J. G., et al. (2021). Blue and grey urban water footprints through citizens' perception and time series analysis of Brazilian dynamics. Hydrol. Sci. J. 66, 408–421. doi: 10.1080/02626667.2021.1879388
Srinivasan, V. (2015). Reimagining the past – use of counterfactual trajectories in socio-hydrological modelling: The case of Chennai, India. Hydrol. Earth Syst. Sci. 19, 785–801. doi: 10.5194/hess-19-785-2015
Srinivasan, V., Penny, G., Lele, S., Thomas, B. K., and Thompson, S. (2017). Proximate and underlying drivers of socio-hydrologic change in the upper Arkavathy watershed, India. Hydrol. Earth Syst. Sci. Discus. 1–28. doi: 10.5194/hess-2017-543
Srinivasan, V., Sanderson, M., Garcia, M., Konar, m., Bloschl, G., and Sivapalan, M. (2018). Moving socio-hydrology modelling forward: unpacking hidden assumptions, values and model structure by engaging with stakeholders: reply to “What is the role of the model in socio-hydrology?” Hydrol. Sci. J. 63, 1444–1446. doi: 10.1080/02626667.2018.1499026
Stephens, C. M., Lall, U., Johnson, F. M., and Marshall, L. A. (2021). Landscape changes and their hydrologic effects: interactions and feedbacks across scales. Earth Sci. Rev. 212:103466. doi: 10.1016/j.earscirev.2020.103466
Sun, S. K., Song, J. F., Wang, F. F., Wu, P. T., and Wang, Y. B. (2019). Evaluating the impacts of socio-economic factors on regional grain virtual water flows in China using a structural equation modeling approach. J. Hydrol. 571, 132–141. doi: 10.1016/j.jhydrol.2019.01.042
Tellman, B., Bausch, J., Eakin, H., Anderies, J., Mazari-Hiriart, M., Manuel-Navarrete, D., et al. (2018). Adaptive pathways and coupled infrastructure: seven centuries of adaptation to water risk and the production of vulnerability in Mexico City. Ecol. Soc. 23:1–19. doi: 10.5751/ES-09712-230101
Tian, F., Lu, Y., Hu, H., Kinzelbach, W., and Sivapalan, M. (2019). Dynamics and driving mechanisms of asymmetric human water consumption during alternating wet and dry periods. Hydrol. Sci. J. 64, 507–524. doi: 10.1080/02626667.2019.1588972
Towler, E., Lazrus, H., and PaiMazumder, D. (2019). Characterizing the potential for drought action from combined hydrological and societal perspectives. Hydrol. Earth Syst. Sci. 23, 1469–1482. doi: 10.5194/hess-23-1469-2019
Troy, T. J., Konar, M., Srinivassan, V., and Thompson, S. (2015). Moving sociohydrology forward: a synthesis across studies. Hydrol. Earth Syst. Sci. 19, 3667–3679. doi: 10.5194/hess-19-3667-2015
Viero, D. P., Roder, G., Matticchio, B., Defina, A., and Tarolli, P. (2019). Floods, landscape modifications and population dynamics in anthropogenic coastal lowlands: the Polesine (northern Italy) case study. Sci. Total Environ. 651, 1435–1450. doi: 10.1016/j.scitotenv.2018.09.121
Viglione, A., Di Baldassarre, G., Brandimarte, L., Kuil, L., Carr, G., Salinas, J. L., et al. (2014). Insights from socio-hydrology modelling on dealing with flood risk – Roles of collective memory, risk-taking attitude and trust. J. Hydrol. 518, 71–82. doi: 10.1016/j.jhydrol.2014.01.018
Vogel, R. M., Lall, U., Cai, X., Rajagopalan, B., Weiskel, P. K., Hooper, R. P., et al. (2015). Hydrology: the interdisciplinary science of water. Water Resources Res 51, 4409–4430. doi: 10.1002/2015WR017049
Vollmer, D., Shaad, K., Souter, N. J., Farrell, T., Dudgeon, D., Sullivan, C. A., et al. (2018). Integrating the social, hydrological and ecological dimensions of freshwater health: the freshwater health index. Sci. Total Environ. 627, 304–313. doi: 10.1016/j.scitotenv.2018.01.040
Wang, Z., Nguyen, T., and Westerhoff, P. (2019). Food–Energy–water analysis at spatial scales for districts in the Yangtze River Basin (China). Environ. Eng. Sci. 36, 789–797. doi: 10.1089/ees.2018.0456
Ward, E. W., and Winter, K. (2016). Missing the link: urban stormwater quality and resident behaviour. Water SA 42:571. doi: 10.4314/wsa.v42i4.07
Wei, J., Wei, Y., and Western, A. (2017). Evolution of the societal value of water resources for economic development versus environmental sustainability in Australia from 1843 to 2011. Global Environ. Change 42, 82–92. doi: 10.1016/j.gloenvcha.2016.12.005
Wilfong, M., and Pavao-Zuckerman, M. (2020). Rethinking stormwater: analysis using the hydrosocial cycle. Water 12:1273. doi: 10.3390/w12051273
Wilhelmi, O. V., and Morss, R. E. (2013). Integrated analysis of societal vulnerability in an extreme precipitation event: a Fort Collins case study. Environ. Sci. Policy 26, 49–62. doi: 10.1016/j.envsci.2012.07.005
Wu, X. D., Guo, J. L., Li, C. H., Shao, L., Han, M. Y., and Chen, G. Q. (2019). Global socio-hydrology: an overview of virtual water use by the world economy from source of exploitation to sink of final consumption. J. Hydrol. 573, 794–810. doi: 10.1016/j.jhydrol.2019.03.080
Wurl, J., Gámez, A. E., Ivanova, A., Imaz Lamadrid, M. A., and Hernández-Morales, P. (2018). Socio-hydrological resilience of an arid aquifer system, subject to changing climate and inadequate agricultural management: a case study from the Valley of Santo Domingo, Mexico. J. Hydrol. 559, 486–498. doi: 10.1016/j.jhydrol.2018.02.050
Xie, M. (2006). Integrated water resources management (IWRM)–introduction to principles and practices. In: Africa Regional Workshop on IWRM, Nairobi, Oct.
Xu, X., Li, X., Chen, M., Li, X., Duan, X., Zhu, G., et al. (2016). Land-ocean-human interactions in intensively developing coastal zone: demonstration of case studies. Ocean Coastal Manage. 133, 28–36. doi: 10.1016/j.ocecoaman.2016.09.006
Yaeger, M. A., Housh, M., Cai, X., and Sivapalan, M. (2014). An integrated modeling framework for exploring flow regime and water quality changes with increasing biofuel crop production in the U.S. Corn Belt. Water Resources Res. 50, 9385–9404. doi: 10.1002/2014WR015700
York, A. M., Sullivan, A., and Bausch, J. C. (2019). Cross-scale interactions of socio-hydrological subsystems: examining the frontier of common pool resource governance in Arizona. Environ. Res. Lett. 14:125019. doi: 10.1088/1748-9326/ab51be
Yu, D., Chang, H., Davis, T., Hillis, V., Marston, L., Oh, W. S., et al. (2020). Socio-hydrology: an interplay of design and self-organization in a multilevel world. Ecol. Soc. 25:22. doi: 10.5751/ES-11887-250422
Yu, D. J., Sangwan, N., Sung, K., Chen, X., and Merwade, V. (2017). Incorporating institutions and collective action into a sociohydrological model of flood resilience. Water Resources Res. 53, 1336–1353. doi: 10.1002/2016WR019746
Zalewski, M. (2000). Ecohydrology—The scientific background to use ecosystem properties as management tools toward sustainability of water resources. Ecol. Eng. 16, 1–8. doi: 10.1016/S0925-8574(00)00071-9
ZamanZad-Ghavidel, S., Bozorg-Haddad, O., and Goharian, E. (2021). Sustainability assessment of water resource systems using a novel hydro-socio-economic index (HSEI). Environ. Dev. Sustain. 23, 1869–1916. doi: 10.1007/s10668-020-00655-8
Zhao, C., and Mo, D. (2020). Holocene hydro-environmental evolution and its impacts on human activities in Jianghan-Dongting Basin, Middle Reaches of the Yangtze River, China. Dili Xuebao Acta Geographica Sinica 75, 529–543. doi: 10.1007/s11442-020-1735-6
Zhou, S., Huang, Y., Wei, Y., and Wang, G. (2015). Socio-hydrological water balance for water allocation between human and environmental purposes in catchments. Hydrol Earth Syst. Sci. 19, 3715–3726. doi: 10.5194/hess-19-3715-2015
Keywords: scale issues, sociohydrology, spatial-temporal data, coupled human-water systems, systematic review
Citation: Fischer A, Miller JA, Nottingham E, Wiederstein T, Krueger LJ, Perez-Quesada G, Hutchinson SL and Sanderson MR (2021) A Systematic Review of Spatial-Temporal Scale Issues in Sociohydrology. Front. Water 3:730169. doi: 10.3389/frwa.2021.730169
Received: 24 June 2021; Accepted: 27 August 2021;
Published: 30 September 2021.
Edited by:
Pieter van Oel, Wageningen University and Research, NetherlandsReviewed by:
Maurizio Mazzoleni, Uppsala University, SwedenJeroen Vos, Wageningen University and Research, Netherlands
Copyright © 2021 Fischer, Miller, Nottingham, Wiederstein, Krueger, Perez-Quesada, Hutchinson and Sanderson. This is an open-access article distributed under the terms of the Creative Commons Attribution License (CC BY). The use, distribution or reproduction in other forums is permitted, provided the original author(s) and the copyright owner(s) are credited and that the original publication in this journal is cited, in accordance with accepted academic practice. No use, distribution or reproduction is permitted which does not comply with these terms.
*Correspondence: Matthew R. Sanderson, bWF0dHJzJiN4MDAwNDA7a3N1LmVkdQ==
†These authors have contributed equally to this work and share first authorship