- 1Department of Civil and Environmental Engineering, Colorado State University, Fort Collins, CO, United States
- 2Rocky Mountain Research Station, U.S. Forest Service, Fort Collins, CO, United States
While urban areas are being threatened by water shortage due to climate change and rapid population growth, effects of urban development patterns on future municipal water shortage are rarely investigated. We address this aspect of urbanization by assessing the impacts of sprawl vs. high-density patterns on future changes in the sub-annual water shortage intensity-duration-frequency (IDF) relationships. The City of Fort Collins, Colorado, water supply system is chosen as a representative region that is rapidly developing over the last decades. The future water supply is estimated using the Soil and Water Assessment Tool (SWAT) driven with a hot-dry climate model from the statistically downscaled Coupled Model Intercomparison Project, phase 5 (CMIP5) projections. Future water demand is projected using the Integrated Urban Water Model (IUWM) under both sprawl and high-density development patterns. The demonstration study reveals that urban areas under the sprawl development pattern are likely to experience water shortage events with higher intensity, duration, and frequency compared to the high-density pattern. Characterizing impacts of urban development patterns on future water shortage conditions is required for sustainable water management and smart urban growth and can help urban planners and water managers to develop an adaptive path to meet future water demand and decrease the vulnerability of municipal water supply systems to shortage.
Introduction
Municipal water shortage is a crucial problem around the world due to the integrated impacts of climate change and rapid urbanization (Mukherjee et al., 2018; Liang et al., 2020). Municipal water shortage can be defined as the lack of sufficient water supply to meet demand in urban areas (Foti et al., 2014; Zhao et al., 2019; Heidari et al., 2020a). Although water supply may be significantly affected in the future by climate change as a result of varying precipitation and increasing temperature (Heidari et al., 2020b, 2021b), water demand may increase over time due to rapid urbanization (Liu et al., 2020). Rapid urbanization can intensify imbalance between water supply and demand and lead to higher likelihood of water shortage conditions in developed areas in the future (Foti et al., 2012; Brown et al., 2019). The issues of increasing water shortage conditions and limited water resources may also restrict the farther urbanization (McDonald et al., 2011; Fan et al., 2017).
Rapid urbanization can be a consequence of either population growth or urban development (Bhatta et al., 2010; Leyk et al., 2020; Xu et al., 2020). Previous studies have mostly assessed the impacts of climate change or population growth on municipal water shortage (Foti et al., 2014; Rajsekhar et al., 2015; Hao et al., 2018; Warziniack and Brown, 2019; Sanchez et al., 2020), while the pattern of urban development itself can considerably affect the regional hydrological cycles through changing the geology of the river basins (e.g., slope, permeability, etc.) (McDonald et al., 2011; McGrane, 2016; Mukherjee et al., 2018; Hemmati et al., 2020; O'Donnell and Thorne, 2020). Well understanding of the role of urban development patterns as one of the driving forces of water shortage can provide a basis for decision-makers to alleviate water shortage conditions in the future (Li et al., 2020).
Urban development can be defined as the interaction of vertical growth (high-density) and horizontal growth (sprawl) patterns (Yan et al., 2018; Zhang et al., 2018; Zambon et al., 2019). Sprawl pattern refers to low-density urban areas with segregated land uses and homogenous populations, whereas a high-density pattern refers to urban areas with inhomogeneous populations accompanied by small areas of open or green space to accommodate large numbers of residential and commercial buildings (Western Resource Advocates, 2003). Urban development patterns are one of the main drivers of rising municipal water demand (House-Peters, 2010; Bouziotas et al., 2015). Sprawl development patterns can lead to increases in per capita rates of outdoor water use while the high-density development pattern can lead to lower outdoor water use (Western Resource Advocates, 2003; Sanchez et al., 2020).
Despite broad research on water shortage at different spatial and temporal scales, the importance of role of the urban development patterns remains less understood and discussed (Ioris, 2012; Chang et al., 2018; Li et al., 2020; Luo et al., 2020). Most previous national-scale studies have mainly investigated the distribution of water availability and water demand, and the mismatch between them (McDonald et al., 2011; Foti et al., 2012; Zhao et al., 2018; Brown et al., 2019; Warziniack and Brown, 2019; Heidari et al., 2020b), while previous regional-scale studies focused on urban development (Gober, 2010; Gober and Kirkwood, 2010; Gober et al., 2010; McDonald et al., 2011; Ren et al., 2018; Li et al., 2020) and highlighted the important role of land use change on the future regional water availability. McDonald et al. (2011) investigated how population growth and climate change will affect water availability for cities in developing countries and pointed out that effects of water shortage can be attenuated through efficient use of landscape resources (McDonald et al., 2011). Li et al. (2020) reported that both the urbanization levels and type had considerable impacts on water shortage. They conducted a research on the Beijing-Tianjin-Hebei megaregion in China and found the positive relation between human-induced water scarcity and landscape urbanization meaning that the increase in the built area would intensify water scarcity due to the increase in water consumed by the constructed land (Li et al., 2020).
Additionally, numerous studies reported that urban development can lead to urban heat island (UHI) effects in which urban regions have higher air temperature compared to the surrounding rural regions (Jenerette et al., 2007; Sodoudi et al., 2014; Kaloustian and Diab, 2015; Huang et al., 2019). Huang et al. (2019) reported that by 2050, urban land areas can be expanded by 78%-171% across the world leading to warming in air temperature of 0.5–0.7°C, up to~3°C in some regions (Huang et al., 2019). Previous studies reported that the physical form and land cover of cities can highly influence the intensity and pattern of the UHI (Gober et al., 2010). For example, people who living at lower density urbans use irrigated landscapes to lower daytime temperatures (Jenerette et al., 2007).
In the United States, the urban population has grown from 6 to 81% over the past 200 years (Leyk et al., 2020), and urban areas have increasingly expanded (Wheeler, 2008; Barrington-Leigh and Millard-Ball, 2015). The process of urban sprawl on the west and southwest of the United States has been exacerbated at tremendous rates (Hummel, 2020). While rapid urbanization seems inevitable in the United States, understanding a sustainable way to mitigate potential negative consequences on urban water resources in the future is an important challenge (Butler et al., 2017; Saraswat et al., 2017; Forrest et al., 2020; Heidari et al., 2021a).
Although aforementioned studies described the impacts of land use changes on water use (Gober, 2010; Gober et al., 2010; Santikayasa et al., 2014; Shrestha et al., 2018), enhanced characterizing the combined effects of population growth and climate change on future water shortage characteristics under different urban development pattern scenarios have been less investigated. Understanding connections between water supply and development patterns is vital to effectively manage and plan for future urban water resources. In other words, the importance of urban development patterns for future water shortage has received less attention compared to climate change and population growth as the main source of increasing the water shortage scarcity (Hemmati et al., 2020). Rapid urbanization compounded by climate change may pose significant pressures on municipal water supply systems in the future (McDonald et al., 2011; Brown et al., 2019; Heidari et al., 2020a). Understanding the important role of urban development patterns on municipal water resources in the future is required for smart urban development and sustainable water management (Saraswat et al., 2017; Bounoua et al., 2020; Forrest et al., 2020).
Improved understanding of the role of urban development patterns on municipal water shortage not only helps decision-makers to preserve municipal water supply resources by decreasing water demand, but also can mitigate the negative consequences of future water shortage events on water supply systems (Butler et al., 2017). The main contribution of this study is to focus on investigating the role of urban development patterns particularly in changes in intensity, duration, and frequency of future water shortage events by comparing sprawl vs. high-density development patterns. Increasing water use along with climate change may lead to more frequent imbalance between municipal water demand and supply. In this case, the impacts of urban development patterns on municipal water shortage can be represented by changes in properties of water shortage events, where a water shortage event is defined as the condition in which the quantities of water demand exceeds the quantities of water supply (Foti et al., 2014; Brown et al., 2019; Warziniack and Brown, 2019; Heidari et al., 2020a). This study highlights the importance of urban development patterns, i.e., sprawl vs. high-density, to future municipal water shortage for the city of Fort Collins as a required task for sustainable water management and smart urban development. The objectives are to: (1) assess how urban development patterns can affect municipal water demand; (2) evaluate the combined impacts of climate change, population growth and land use change on future water shortage; and (3) investigate the role of urban development patterns on future IDF properties of water shortage events.
The city of Fort Collins, Colorado was selected as a water shortage-prone area (Heidari et al., 2020a) to provide an insight into the importance of urban patterns on future water shortage. The city is experiencing substantial population growth and urban development in recent decades (Sharvelle et al., 2017; Forrest et al., 2020; Heidari et al., 2020a). A statistical approach proposed by Heidari et al. (2020a) was applied to assess the integrated effects of shifts in water supply and water demand on intensity-duration-frequency (IDF) relationships of water shortage events under climate change and population growth. The probabilistic approach uses a mixture Gamma-GPD distribution model at sub-annual scale that leads to improved assessment of water shortage properties under considerable changes in both water supply and water demand. In this study, the same probabilistic approach was applied to compare future IDF characteristics under sprawl vs. high-density development patterns.
Materials and Methods
Based on the definition of water shortage events, climate change, future water supply, and water demand projections are needed for the estimation of future water shortage properties. The Soil and Water Assessment Tool (SWAT) and the Integrated Urban Water Model (IUWM) were respectively used to estimate future water supply and water demand for the city of Fort Collins under a hot-dry climate model from the statistically downscaled Coupled Model Intercomparison Project, phase 5 (CMIP5) projections. Then, a probabilistic approach was used under sprawl and high-density patterns to assess the impacts of urban development patterns on intensity, duration, and frequency of water shortage events.
Study Region and Data
Fort Collins in northern Colorado is a growing small city characterized by mostly low to medium density development (AMEC Environment Infrastructure, 2014) was selected as an appropriate case study to characterize the role of future urban development patterns on municipal water shortage. The population of Fort Collins has increased by 14 percent from 2010 to 2017 (Bureau, 2011), forcing urban planners to develop a plan to support the growth.
The observed climate data were obtained from the Global Historical Climatology Network (GHCN), the Colorado Agricultural Meteorological Network (CoAgMet), and Northern Colorado Water Conservancy District (NCWCD). Future Climate changes were projected under the hot-dry climate model (ipsl-cm5a-mr) with the representative concentration pathway (RCP) 8.5 obtained from the CMIP5 dataset (U.S. Bureau of Reclamation, 2013) which represents the worst-case conditions in the future. The hot-dry climate model represents the CMIP5 model with the highest increase in average temperature and the highest decrease in average precipitation from current to future conditions. The future climate data including precipitation and temperature were statistically downscaled for meteorological stations in the region using the Quantile Mapping technique (Themeßl et al., 2012). Readers are also referred to Heidari et al. (2020a) for the detailed descriptions of future climate model selection. Supplementary Figures 1, 2 represent changes in the 30-year normal annual precipitation and temperature, respectively.
Future Water Supply
The selected hot-dry climate model was used to estimate water supply for the city of Fort Collins from 1986 to 2065. Horsetooth Reservoir and the Cache la Poudre (CLP) river are the two main water sources for the city of Fort Collins (AMEC Environment Infrastructure, 2014). Water deliveries to the city of Fort Collins are based on the annual quota set by Northern Water (AMEC Environment Infrastructure, 2014). Here, we assumed that the historical coefficients of water deliveries to the city of Fort Collins will be maintained as current conditions.
Future water yield in the CLP river basin at the Mouth of Canyon Station [National Water Information System (NWIS), 2019] was estimated using the Soil and Water Assessment Tool (SWAT) model (Arnold et al., 1998) driven with the hot-dry climate model. The SWAT model is a hydrological model that has been widely used to simulate streamflow in response to climate change (Chien et al., 2013; Ficklin et al., 2013; Gassman et al., 2014). Readers are referred to Havel et al. (2018) for the details about the SWAT model set up, calibration, and evaluation for the CLP river basin.
Future Water Demand
The Integrated Urban Water Model (IUWM)was applied to estimate future water demand for the city of Fort Collins. The IUWM is a process-based water balance model that has been used to project urban water demand (Sharvelle et al., 2017). The model was calibrated and evaluated for the city of Fort Collins by Sharvelle et al. (2017). The parameter estimates for the current condition were the same as used for this research the same as those determined in Sharvelle et al. (2017). The IUWM model was driven with the hot-dry climate model and the population growth scenario obtained from the U.S. Census Bureau with an estimated population of 165,000 for the middle of the century. Readers are referred to Sharvelle et al. (2017) for the details about the IUWM model set up, calibration, and evaluation for the city of Fort Collins. IUWM estimates indoor water use based on number of households and population and outdoor water use based on climate date, evapotranspiration, and several behavioral characteristics (e.g., landscape selection, percentage of evapotranspiration met, accounting for precipitation events, and selection of irrigation systems; Sharvelle et al., 2017). In the absence of adaptation actions, while the indoor water use was assumed to similarly increase to 54 gallons per capita per day (gpcd) under both sprawl and high-density development patterns, the outdoor water use was assumed to increase to about 80 gpcd under the high-density development pattern and 107 gpcd under the sprawl development pattern by the middle of the century. The calibrated value for plant factor applied in this study is 0.88 (Supplementary Table 1), consistent with Sharvelle et al. (2017) and Neale et al. (2020). Current condition landscape in Fort Collins, CO includes a mix of turf grass, drip irrigated landscape areas, and some xeriscape. Future scenarios developed for this study assumed behavior parameters (Supplementary Table 1) do not change, and account for changes in land use, climate, and population.
Housing density development has been identified as one of the factors that influences water use in urban areas (Western Resource Advocates, 2003). Thus, the future water demand for the city of Fort Collins was estimated under two high-density and sprawl development patterns using the IUWM model.
Table 1 provides changes in population and population density (people per sq. mile) for the city of Fort Collins from 2010 to 2050. Population was estimated to increase by 165,000 under both sprawl and high-density scenarios. This assumption helps to consider only the effects of different urban development patterns on IDF relationships of water shortage events in response to rapid urbanization. Population density was assumed to increase over time under high-density development by 4,263 (people per sq. mile) while it was assumed to decrease by 2,762 (people per sq. mile) under sprawl development.
Figures 1A,B represent shifts in developed area including open, low, medium, and high intensities under the sprawl and high-density development patterns respectively. Under current conditions, it was assumed that there is 38.7 (sq. miles) developed area for the city of Fort Collins including open (9.2 sq. miles), low (18.3 sq. miles), medium (8.8 sq. miles), and high (2.4 sq. miles) intensities (Supplementary Tables 2, 3). Under sprawl scenario, total developed area will increase from 38.7 (sq. miles) to 59.7 (sq. miles) by the middle of century with the highest growth in low intensity area (14.3 sq. miles), and no change in high intensity area. Open and medium intensity area were assumed to increase by 4 (sq. miles), and 2.7 (sq. miles), respectively.
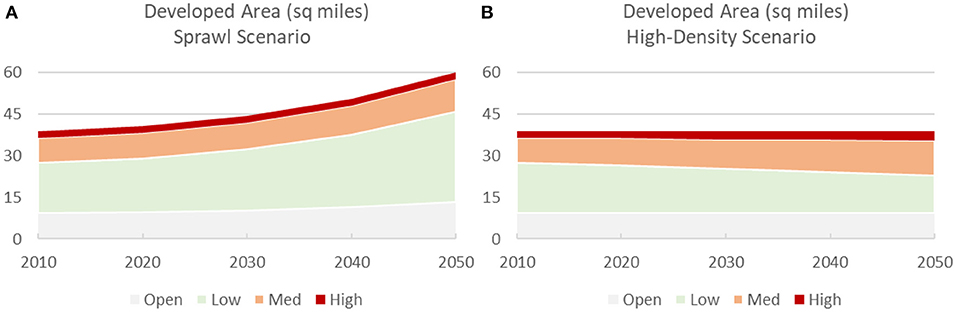
Figure 1. Estimation of population density (in sq. miles) for each developed area under high-density development. (A) Developed area (sq miles) sprawl scenario. (B) Developed area (sq miles) high-density scenario.
Under high-density development, total developed area was assumed to remain constant; however, the proportion of intensity will change with the highest decrease in low intensity area (−4.6 sq. miles), and no change in open area by the middle of century. Medium and high intensity areas were assumed to increase by 3.7 sq. miles, and 0.9 sq. miles, respectively.
Water Shortage IDF Projection
The probabilistic approach developed by Heidari et al. (2020a) was applied for the city of Fort Collins to evaluate the important role of urban development patterns on municipal water shortage induced by changes in future IDF characteristics of water shortage events. A sub-annual water shortage event can be defined as a succession of consecutive months with water demand greater than water supply (Yevjevich, 1967; Heidari et al., 2020a).
Water shortage events can be identified by duration, magnitude, intensity, and frequency (Supplementary Figure 3) (Yevjevich, 1967; Salas et al., 2005; Heidari et al., 2020a). Duration is the number of consecutive months in which water demand is greater than water supply. Magnitude is summation of monthly water deficit over the duration period. Intensity is the ratio of magnitude to duration and frequency is the number of times that an event occurs over a specific period. Intensity, duration and frequency (IDF) properties can be used for design of municipal water supply systems (Salas et al., 2005; Hallack-Alegria and Watkins, 2007; Ramazanipour et al., 2011; Wang et al., 2011; Mann and Gleick, 2015; Rajsekhar et al., 2015; Heidari et al., 2020a).
Heidari et al. (2020a) showed that characterization of water shortage IDF relationships can be improved by applying a mixture Gamma-GPD distribution model at a sub-annual scale (Supplementary Figure 4). The mixture probability models have been widely used to simultaneously capture bulk and tail of distribution (MacDonald et al., 2011; Stephens et al., 2018; Ghanbari et al., 2019, 2020). Heidari et al. (2020a) proposed the cumulative distribution function of mixture Gamma-GPD model as below:
where G(x|r, a) is the Gamma distribution function (Equation 2), g(x| ξ, β, u) is the unconditional GPD function (Equation 3), u is the threshold, and ϕu is the probability of x being above the threshold (Behrens et al., 2004; MacDonald et al., 2011).
Consequently, the joint probability distribution can be described as follows:
where D0 and I0 are respectively given values of duration and intensity. The term P (I > I0| D > D0) and P (D > D0) can be obtained from Equation 1 using F(I > I0| D > D0) and F (D > D0), respectively.
Moreover, return period and frequency amplification factor can be respectively defined as Equations (5, 6):
where E(D > D0) is the expected interarrival time for a water shortage event with D > D0, and PC(I > I0) and Pt(I > I0) are the exceedance events probability with I > I0 for current and future conditions, respectively. Amplification factor is applied in this study to quantify changes in frequencies of water shortage events from current to future periods under non-stationary conditions.
Future assessment of municipal water shortage using the proposed approach not only enhances understanding the effects of future urban patterns on urban water demand, but also provides an insight into how urban development patterns and to what extent leads to changes in future water shortage properties by considering sub-annual shifts in both water supply and water demand conditions (Heidari et al., 2020a). Note that in this study it was assumed the socioeconomic drivers of water demand will be unchanged over time. Readers are referred to Heidari et al. (2020a) for further explanation of water shortage IDF characteristics.
Results
While water supply decreases due to climate change, water demand increases in the future by the growing population and water use. Urban areas under sprawl patterns are likely to have water shortage events with higher intensity, duration, and frequency compared to the high-density pattern. The finding highlights that the sprawl development plays an important role in increasing vulnerability of cities to water shortage conditions. The 1986–2015 and 2035–2065 periods were used to respectively represent the current and mid-century conditions.
Future Water Supply and Demand Assessment
We characterized the potential amount of available water supply and water demand for the city of Fort Collins and did not account for problems such as water deliveries or water conservation and storage in the future. Figure 2A presents 12-month average of projected monthly water supply and water demand for the City of Fort Collins under both sprawl and high-density development and the hot-dry climate model by the middle of the century. Although water demand for the city of Fort Collins increases over time due to population growth, sprawl development results in higher total water demand compared to the high-density development pattern. Sprawl development pattern can lead to about 0.5 more million cubic meter in water demand by the middle of the century compared to the high-density development pattern. This would be due to rising in per capita water use for keeping landscapes green and irrigation purposes.
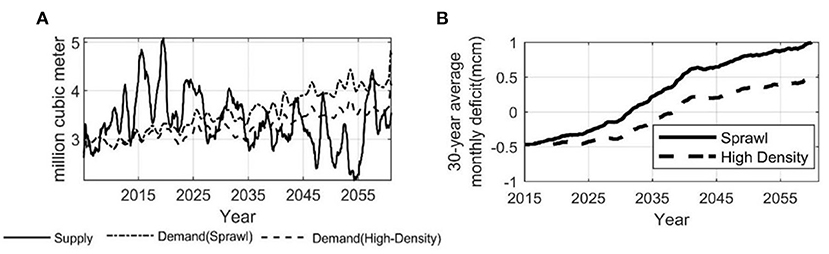
Figure 2. (A) 12-month average of estimated water supply and water demand; and (B) 30-year average of monthly water deficit in million cubic meters (mcm) under the hot-dry climate model.
Therefore, sprawl development leads to a higher gap between water supply and water demand by the middle of the century. By the middle of the century, sprawl development is likely to approximately double monthly water deficits for the city of Fort Collins, water supply system compared to the high-density development pattern as it is shown in Figure 2B. Note that outdoor landscape, irrigation behavior, and indoor water use is assumed to stay constant in response to water shortage conditions in this study so that water deficits here represent those based on current behavior. Water deficits may be reduced via water conservation and reuse strategies due to large potential for these strategies to reduced demand for traditional supplies (Sharvelle et al., 2017; Neale et al., 2020).
Figure 2B indicates that developing cities under the high-density pattern can lead to saving a significant amount of water demanded and decreases pressure on municipal water supply systems in the future under climate changes and rapid population growth by assuming that other influencing factors remain constant.
Changes in Sub-annual Water Shortage IDF Properties
Sub-annual water shortage events can be characterized by the estimated monthly water deficit according to definitions and the applied probabilistic framework (Heidari et al., 2020a). While the expected interarrival time is forecasted to change significantly from the current conditions (1986–2015) to future conditions (2036–2035) under both sprawl and high-density patterns, sprawl development can lead to higher decreases in the expected interarrival than high-density development as it is shown in Figure 3A. Water shortage events with longer durations are more sensitive to urban development patterns than events with a duration of less than a year. For water shortage events with longer duration, urbanization under high-density pattern can approximately double the expected interarrival time between two water shortage events. However, events with short duration of less than a year (D <12) would be less influenced by changing urban development patterns. The results indicate that events with short duration of less than a year can be less affected by the choice of urban development patterns.
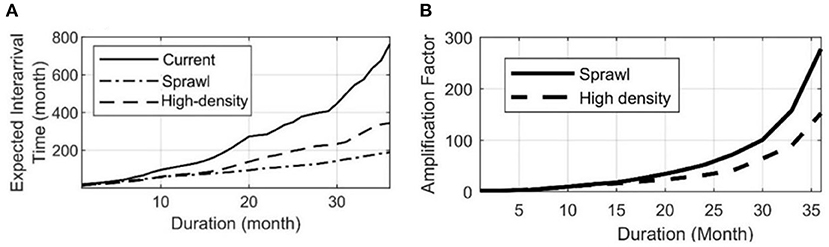
Figure 3. (A) Expected interarrival time; and (B) Frequency amplification factor curves for water shortage events.
Figure 3B shows changes in frequency amplification factors under sprawl and high-density development patterns with various durations. Although the frequencies of sub-annual water shortage events are estimated to increase as a result of climate change and population growth, changes in their frequencies are not the same under sprawl and high-density development patterns. The results indicate that the frequency for the study system was substantially higher for the sprawl development pattern compared to the high-density development pattern. Similar to results from the expected interarrival time, the amplification factor of events with short duration of less than a year is less affected by the urban development patterns while for water shortage events with longer durations, sprawl pattern can even double the frequency of water shortage events.
Changes in IDF curves of sub-annual water shortage events were obtained using the developed approach by Heidari et al. (2020a). Future IDF curves were generated with a duration greater than 1, 6, 12, 24, and 36 months for the sprawl and high-density development patterns respectively (Figures 4A,B). The comparison of the IDF curves for the sprawl and high-density development patterns highlights the importance of the pattern of urban growth on increasing sub-annual water shortage events.
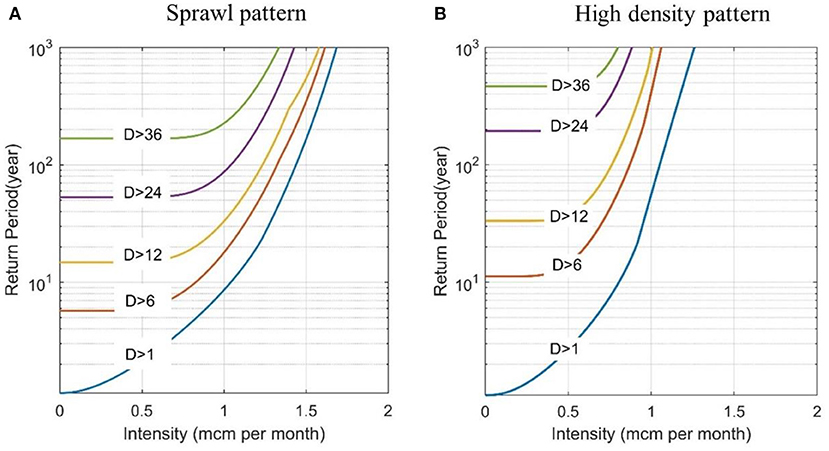
Figure 4. Intensity-duration-frequency (IDF) curves for future conditions under (A) Sprawl patterns and (B) High-density pattern for duration (D) greater than 1, 6, 12, 24, and 36 months.
The return period of events under sprawl development (Figure 4A) is smaller than high-density development (Figure 4B) consistently under all varying durations. For a given return period, events with higher intensity and longer duration were estimated under the sprawl pattern compared to the high-density pattern for the middle of the century. The results indicate that urban development patterns can substantially affect future IDF curves. IDF curves are widely applied for the design of municipal water supply systems. Thus, the urban development patterns can directly affect design of water supply systems in the future.
Quantitative parameters were estimated to demonstrate effects of urban development patterns on water shortage properties (Table 2). The average magnitude, duration, intensity, and maximum water deficit were calculated under both sprawl and high-density development patterns. The magnitude, duration, and intensity substantially increase under the sprawl pattern with a higher rate in duration and magnitude. These results highlight that the sprawl development pattern can substantially affect future municipal water shortage and play a key role in sustainable development and water use.
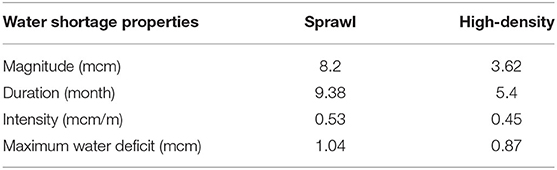
Table 2. Impacts of urban development patterns (sprawl vs. high-density) on water shortage properties.
Discussion
Rapid urbanization can change the behavior of river basins through changing their topology, geology, and hydrology and have some significant negative consequences on air, energy, land, and water noted in various studies (Wilson and Chakraborty, 2013). Urban development can alter the dynamic of water supply and demand as the combination of changes in land use and water consumption. The urban development pattern can directly impact the municipal water demand and cause a significant imbalance between water supply and water demand in developing areas. Although climate change and population growth are well-known as two major causes of water shortage in urban areas, the results of this study in overall identified that the urban sprawl development pattern, in particular, is as a fundamental factor contributing to raising intensity, duration, and frequency of water shortage events by growing future water consumption. It should be noted that sprawl development itself can also lead to increases in pollutant emissions such as carbon monoxide over the long-term (Stone et al., 2007; Zhang et al., 2020), and hence exacerbating climate change.
However, it should be paid attention that decisions on future land management is more complicated and should seek to balance impacts of water use and environmental impacts of urbanization. While urbanization under the high-density development pattern can lead to decreasing water shortage conditions in the future, it may have negative consequences on other aspects of urban water cycle such as an increase in stormwater due to more impervious areas. Thus, an integrated water resources and land management strategy is required to mitigate negative consequences on society, economy, and environment. Besides, the importance of water shortage between sprawl and high-density development patterns may be different so that shortage for the sprawl pattern may mean not irrigating lawns while for the high-density pattern may mean no showers.
The assumption for projection of water shortages for this research was that behavior for indoor and outdoor water use stay constant in future scenarios. It is important to note that increased efficiency in water use has the potential to decrease future water shortages. Sharvelle et al. (2017) demonstrated demand reduction for traditional water sources in the study city could be reduced by 7–45% through adoption of water conservation and reuse strategies at a pragmatic level (harvesting roof runoff : 7%; indoor conservation: 14%; aggressive irrigation conservation: 45%). Notable demand reduction could be achieved by selecting climate appropriate landscape and use of efficient irrigation systems (Sharvelle et al., 2017). The current landscape includes a large amount of turf grass (plant factor = 0.88; Supplementary Table 1) and notable decreases in projected outdoor water demand could be achieved by selection of climate appropriate landscape. The efficiency of irrigation systems assumed for this study was 71% (Supplementary Table 1), which also could be substantially improved. It was not within the scope of this research to investigate the role of water demand reduction strategies on water shortages in future conditions, however future research should address this. Water sensitive urban design can incorporate climate appropriate landscape selection to reduce water demand (Permana and Petchsasithon, 2020; Williams, 2020).
Furthermore, high density development typically results in high impervious area, and increased stormwater runoff with detrimental impacts for receiving water bodies and combined sewer overflow events (Bouziotas et al., 2015). Innovative stormwater management techniques such as low impact development (LID), and green infrastructure (GI) can reduce these negative impacts in high density development areas (Seo et al., 2017b; Zhang et al., 2017). However, it should be highlighted that LID/GI practices may not be of much assistance in reducing peak runoff for extreme precipitation events (Ahiablame et al., 2013; Seo et al., 2017a; Zhu et al., 2019) that are likely to increase in the future (Ghanbari et al., 2021).
The results of this study are also subject to several sources of uncertainty, including the choice and assumptions of the climate model, SWAT hydrological model, IUWM model, and probabilistic approach. Despite these uncertainties, comparisons of changes in water shortage characteristics under sprawl and high-density development patterns from the current to the future conditions are more likely to be accurately estimated than are the absolute amounts from which the differences are calculated.
This study aims to highlight the importance of urban development patterns on future water shortage conditions rather than policy implementation or planning strategies. Note that we investigated only the role of urban development patterns on municipal water shortage features and do not account for problems with adequate water conservation, stormwater management, water delivery, and water quality. The main purpose of this study was to identify the role of urban development patterns on municipal water shortage through two urban development scenarios as a comparative study. A comprehensive assessment of future water supply and demand conditions in response to climate change is beyond the scope of this study. Comprehensive planning regulation and strategies for sustainable urban development in the future needs an interdisciplinary collaborative work between hydrologist, geologist, policymakers, economist, and social scientist.
Conclusions
As population and urban areas continue to grow, enhanced urban planning and sustainable water resource management become more crucial. This study aimed to draw attention to the role of urban development patterns in future water shortage conditions to provide insights for decision makers, water, and land managers. Improved understanding of factors that reduce future water shortage conditions can help local and regional planners to identify policy solutions that result in more efficiency of future water use. Overall, four important conclusions can be made here:
1) The sprawl development pattern can result in more water consumption due to higher quantities of water used for outdoor activities such as landscape irrigation.
2) The sprawl development pattern can increase magnitude, intensity, duration, and frequency of water shortage events with higher impacts on magnitude and duration.
3) The high-density development pattern not only saves municipal water supply by decreasing future water use but also leads to decreasing impacts of water shortage events on municipal water supply systems by decreasing intensity, duration, and frequency compared to the sprawl development pattern during the long duration of water shortage events.
4) Water shortage events with short duration of less than a year are less sensitive to urban development patterns compared to events with long duration of more than a year.
The decision on the future urban development pattern can considerably affect the design and vulnerability of municipal water supply systems due to the significant change in the intensity, duration, and frequency of future water shortage events. The findings of this study recommended that modification to development densities can reduce vulnerability to water shortage. However, decisions on future urban development patterns must balance considerations of water shortage events with possible negative impacts of urbanization and include innovative strategies for stormwater management to mitigate those impacts. Note that water conservation practices can reduce water consumption and subsequently water shortage conditions. Therefore, future policies should be developed particularly for cities with the high-density development pattern to ensure sustainable and sufficient water use (Kaloustian and Diab, 2015). Future research should explore the potential for water conservation and reuse to further reduce water shortage events under both sprawl and high-density development patterns.
Data Availability Statement
The original contributions presented in the study are included in the article/Supplementary Material, further inquiries can be directed to the corresponding author.
Author Contributions
HH and MA: conceptualization. HH, TW, MA, and SS: methodology, validation, formal analysis, investigation, resources, and writing—review and editing. HH, MA, and SS: software. HH: writing—original draft preparation. All authors contributed to the article and approved the submitted version.
Funding
This work was funded by NSF Sustainability Research Network (SRN) Cooperative Agreement 1444758 and a cooperative agreement US Forest Service Research and Development, Rocky Mountain Research Station.
Conflict of Interest
The authors declare that the research was conducted in the absence of any commercial or financial relationships that could be construed as a potential conflict of interest.
Acknowledgments
The observed daily temperature and precipitation data were available from the Global Historical Climatology Network (GHCN), as provided by the National Oceanic and Atmospheric Administration, National Climatic Data Center (https://www.ncdc.noaa.gov/ghcn-monthly), the Colorado Agricultural Meteorological Network (CoAgMet, https://coagmet.colostate.edu/), and Northern Colorado Water Conservancy District (NCWCD, http://www.ncwcd.org). Future climate was obtained from the CMIP5 projections (Reclamation, 2013).
Supplementary Material
The Supplementary Material for this article can be found online at: https://www.frontiersin.org/articles/10.3389/frwa.2021.694817/full#supplementary-material
References
Ahiablame, L. M., Engel, B. A., and Chaubey, I. (2013). Effectiveness of low impact development practices in two urbanized watersheds: retrofitting with rain barrel/cistern and porous pavement. J. Environ. Manage. 119, 151–161. doi: 10.1016/j.jenvman.2013.01.019
AMEC Environment and Infrastructure (2014). Fort Collins Water Supply and Demand Management Policy Revision Report. AMEC Environment and Infrastructure.
Arnold, J. G., Srinivasan, R., Muttiah, R. S., and Williams, J. R. (1998). Large area hydrologic modeling and assessment part I: model development. J. Am. Assoc. Am. Water Resour. Assoc. Febr. 34, 73–89. doi: 10.1111/j.1752-1688.1998.tb05961.x
Barrington-Leigh, C., and Millard-Ball, A. (2015). A century of sprawl in the United States. Proc. Natl. Acad. Sci. U.S.A. 112, 8244–8249. doi: 10.1073/pnas.1504033112
Behrens, C. N., Lopes, H. F., and Gamerman, D. (2004). Bayesian analysis of extreme events with threshold estimation. Stat. Model. 4, 227–244. doi: 10.1191/1471082X04st075oa
Bhatta, B., Saraswati, S., and Bandyopadhyay, D. (2010). Quantifying the degree-of-freedom, degree-of-sprawl, and degree-of-goodness of urban growth from remote sensing data. Appl. Geogr. 30, 96–111. doi: 10.1016/j.apgeog.2009.08.001
Bounoua, L., Fathi, N., El Berkaoui, M., El Ghazouani, L., and Messouli, M. (2020). Assessment of sustainability development in urban areas of Morocco. Urban Sci. 4:18. doi: 10.3390/urbansci4020018
Bouziotas, D., Rozos, E., and Makropoulos, C. (2015). Water and the city: exploring links between urban growth and water demand management. J. Hydroinformatics 17, 176–192. doi: 10.2166/hydro.2014.053
Brown, T. C., Mahat, V., and Ramirez, J. A. (2019). Adaptation to future water shortages in the United States caused by population growth and climate change. Earths Future 7, 219–234. doi: 10.1029/2018EF001091
Bureau, U. S. C. (2011). 2010 Census of Population and Housing (Demographic Profile Summary File: Technical Documentation). Suitland-Silver Hill, MD: U.S. Census Bureau.
Butler, D., Ward, S., Sweetapple, C., Astaraie-Imani, M., Diao, K., Farmani, R., et al. (2017). Reliable, resilient, and sustainable water management: the Safe and SuRe approach. Glob. Challenges 1, 63–77. doi: 10.1002/gch2.1010
Chang, S., Graham, W., Geurink, J., Wanakule, N., and Asefa, T. (2018). Evaluation of impacts of future climate change and water use scenarios on regional hydrology. Hydrol. Earth Syst. Sci. 22, 4793–4813. doi: 10.5194/hess-22-4793-2018
Chien, H., Yeh, P. J. F., and Knouft, J. H. (2013). Modeling the potential impacts of climate change on streamflow in agricultural watersheds of the Midwestern United States. J. Hydrol. 491, 73–88. doi: 10.1016/j.jhydrol.2013.03.026
Fan, L., Gai, L., Tong, Y., and Li, R. (2017). Urban water consumption and its influencing factors in China: evidence from 286 cities. J. Clean. Prod. 166, 124–133. doi: 10.1016/j.jclepro.2017.08.044
Ficklin, D. L., Luo, Y., and Zhang, M. (2013). Climate change sensitivity assessment of streamflow and agricultural pollutant transport in California's Central Valley using Latin hypercube sampling. Hydrol. Process. 27, 2666–2675. doi: 10.1002/hyp.9386
Forrest, N., Stein, Z., and Wiek, A. (2020). Transferability and scalability of sustainable urban water solutions–a case study from the Colorado River Basin. Resour. Conserv. Recycl. 157:104790. doi: 10.1016/j.resconrec.2020.104790
Foti, R., Ramirez, J. A., and Brown, T. C. (2012). Vulnerability of U.S. Water Supply to Shortage: A Technical Document Supporting the Forest Service 2010 RPA Assessment. 295. Available online at: http://www.treesearch.fs.fed.us/pubs/42363 (accessed April 12, 2021).
Foti, R., Ramirez, J. A., and Brown, T. C. (2014). A probabilistic framework for assessing vulnerability to climate variability and change: the case of the US water supply system. Clim. Change 125, 413–427. doi: 10.1007/s10584-014-1111-6
Gassman, P. W., Sadeghi, A. M., and Srinivasan, R. (2014). Applications of the SWAT model special section: overview and insights. J. Environ. Qual. 43, 1–8. doi: 10.2134/jeq2013.11.0466
Ghanbari, M., Arabi, M., Kao, S., Obeysekera, J., and Sweet, W. (2021). Climate change and changes in compound coastal-riverine flooding hazard along the U.S. Coasts. Earth's Future 9:e2021EF002055. doi: 10.1029/2021EF002055
Ghanbari, M., Arabi, M., and Obeysekera, J. (2020). Chronic and acute coastal flood risks to assets and communities in Southeast Florida. https://www.researchgate.net/journal/Journal-of-Water-Resources-Planning-and-Management-0733-9496 J. Water Resour. Plan. Manag. 146, 1–10. doi: 10.1061/(ASCE)WR.1943-5452.0001245
Ghanbari, M., Arabi, M., Obeysekera, J., and Sweet, W. (2019). A Coherent statistical model for coastal flood frequency analysis under nonstationary sea level conditions. Earth's Future 7, 162–177. doi: 10.1029/2018EF001089
Gober, P. (2010). Desert urbanization and the challenges of water sustainability. Curr. Opin. Environ. Sustain. 2, 144–150. doi: 10.1016/j.cosust.2010.06.006
Gober, P., Brazel, A., Quay, R., Myint, S., Grossman-Clarke, S., Miller, A., et al. (2010). Using watered landscapes to manipulate urban heat island effects: how much water will it take to cool phoenix? J. Am. Plan. Assoc. 76, 109–121. doi: 10.1080/01944360903433113
Gober, P., and Kirkwood, C. W. (2010). Vulnerability assessment of climate-induced water shortage in Phoenix. Proc. Natl. Acad. Sci. U.S.A. 107, 21295–21299. doi: 10.1073/pnas.0911113107
Hallack-Alegria, M., and Watkins, D. W. (2007). Annual and warm season drought intensity-duration-frequency analysis for Sonora, Mexico. J. Clim. 20, 1897–1909. doi: 10.1175/JCLI4101.1
Hao, Z., Singh, V. P., and Xia, Y. (2018). Seasonal drought prediction: advances, challenges, and future prospects. Rev. Geophys. 56, 108–141. doi: 10.1002/2016RG000549
Havel, A., Tasdighi, A., and Arabi, M. (2018). Assessing the hydrologic response to wildfires in mountainous regions. Hydrol. Earth Syst. Sci. 22, 2527–2550. doi: 10.5194/hess-22-2527-2018
Heidari, H., Arabi, M., Ghanbari, M., and Warziniack, T. (2020a). A Probabilistic approach for characterization of sub-annual socioeconomic drought intensity- duration-frequency (IDF) relationships in a changing environment. Water 12:1522. doi: 10.3390/w12061522
Heidari, H., Arabi, M., Warziniack, T., and Kao, S.-C. (2021a). Shifts in hydroclimatology of U.S. megaregions in response to climate change. Environ. Res. Commun. 3:065002. doi: 10.1088/2515-7620/ac0617
Heidari, H., Arabi, M., Warziniack, T., and Kao, S. C. (2020b). Assessing shifts in regional hydroclimatic conditions of U.S. River basins in response to climate change over the 21st century. Earth's Future 8, 1–14. doi: 10.1029/2020EF001657
Heidari, H., Warziniack, T., Brown, T. C., and Arabi, M. (2021b). Impacts of Climate change on hydroclimatic conditions of U.S. National Forests and Grasslands. Forests 12:139. doi: 10.3390/f12020139
Hemmati, M., Ellingwood, B. R., and Mahmoud, H. N. (2020). The role of urban growth in resilience of communities under flood risk. Earths Future 8, 1–14. doi: 10.1029/2019EF001382
House-Peters, L. (2010). Examining the Effects of Climate Change and Urban Development on Water Demand: A Multi-Scale Analysis of Future Water Demand in Hillsboro, Oregon. Portland, OR: Portland State University.
Huang, K., Li, X., Liu, X., and Seto, K. C. (2019). Projecting global urban land expansion and heat island intensification through 2050. Environ. Res. Lett. 14:114037. doi: 10.1088/1748-9326/ab4b71
Hummel, D. (2020). The effects of population and housing density in urban areas on income in the United States. Local Econ. 35, 27–47. doi: 10.1177/0269094220903265
Ioris, A. A. R. (2012). The geography of multiple scarcities: urban development and water problems in Lima, Peru. Geoforum 43, 612–622. doi: 10.1016/j.geoforum.2011.12.005
Jenerette, G. D., Harlan, S. L., Brazel, A., Jones, N., Larsen, L., and Stefanov, W. L. (2007). Regional relationships between surface temperature, vegetation, and human settlement in a rapidly urbanizing ecosystem. Landsc. Ecol. 22, 353–365. doi: 10.1007/s10980-006-9032-z
Kaloustian, N., and Diab, Y. (2015). Effects of urbanization on the urban heat island in Beirut. Urban Clim. 14, 154–165. doi: 10.1016/j.uclim.2015.06.004
Leyk, S., Uhl, J. H., Connor, D. S., Braswell, A. E., Mietkiewicz, N., Balch, J. K., et al. (2020). Two centuries of settlement and urban development in the United States. Sci. Adv. 6:eaba2937. doi: 10.1126/sciadv.aba2937
Li, W., Hai, X., Han, L., Mao, J., and Tian, M. (2020). Does urbanization intensify regional water scarcity? evidence and implications from a megaregion of China. J. Clean. Prod. 244:118592. doi: 10.1016/j.jclepro.2019.118592
Liang, Z., Wu, S., Wang, Y., Wei, F., Huang, J., Shen, J., et al. (2020). The relationship between urban form and heat island intensity along the urban development gradients. Sci. Total Environ. 708:135011. doi: 10.1016/j.scitotenv.2019.135011
Liu, X., Huang, Y., Xu, X., Li, X., Li, X., Ciais, P., et al. (2020). High-spatiotemporal-resolution mapping of global urban change from 1985 to 2015. Nat. Sustain. 3, 564–570. doi: 10.1038/s41893-020-0521-x
Luo, P., Sun, Y., Wang, S., Wang, S., Lyu, J., Zhou, M., et al. (2020). Historical assessment and future sustainability challenges of Egyptian water resources management. J. Clean. Prod. 263:121154. doi: 10.1016/j.jclepro.2020.121154
MacDonald, A., Scarrott, C. J., Lee, D., Darlow, B., Reale, M., and Russell, G. (2011). A flexible extreme value mixture model. Comput. Stat. Data Anal. 55, 2137–2157. doi: 10.1016/j.csda.2011.01.005
Mann, M. E., and Gleick, P. H. (2015). Climate change and California drought in the 21st century. Proc. Natl. Acad. Sci. U.S.A. 112, 3858–3859. doi: 10.1073/pnas.1503667112
McDonald, R. I., Green, P., Balk, D., Fekete, B. M., Revenga, C., Todd, M., et al. (2011). Urban growth, climate change, and freshwater availability. Proc. Natl. Acad. Sci. U.S.A. 108, 6312–6317. doi: 10.1073/pnas.1011615108
McGrane, S. J. (2016). Impacts of urbanisation on hydrological and water quality dynamics, and urban water management: a review. Hydrol. Sci. J. 61, 2295–2311. doi: 10.1080/02626667.2015.1128084
Mukherjee, S., Bebermeier, W., and Schütt, B. (2018). An overview of the impacts of land use land cover changes (1980-2014) on urban water security of Kolkata. Land 7:91. doi: 10.3390/land7030091
Neale, M. R., Sharvelle, S., Arabi, M., Dozier, A., and Goemans, C. (2020). Assessing tradeoffs of strategies for urban water conservation and fit for purpose water. J. Hydrol. X 8:100059. doi: 10.1016/j.hydroa.2020.100059
O'Donnell, E. C., and Thorne, C. R. (2020). Drivers of future urban flood risk. Philos. Trans. R. Soc. A Math. Phys. Eng. Sci. 378:20190216. doi: 10.1098/rsta.2019.0216
Permana, A. S., and Petchsasithon, A. (2020). Linking engineering approach and local wisdom in water sensitive urban design as an adaptation strategy to climate change. IOP Conf. Ser. Earth Environ. Sci. 447:012004. doi: 10.1088/1755-1315/447/1/012004
Rajsekhar, D., Singh, V. P., and Mishra, A. K. (2015). Integrated drought causality, hazard, and vulnerability assessment for future socioeconomic scenarios: an information theory perspective. J. Geophys. Res. 120, 6346–6378. doi: 10.1002/2014JD022670
Ramazanipour, M., Roshani, M., and Aveily, J. G. (2011). The relationship of drought intensity, duration and frequency in the Southern Coast of Caspian Sea. World Appl. Sci. J. 15, 1176–1180. Available online at: http://www.idosi.org/wasj/wasj15(8)11/19.pdf
Ren, D., Yang, Y., Yang, Y., Richards, K., and Zhou, X. (2018). Land-Water-Food Nexus and indications of crop adjustment for water shortage solution. Sci. Total Environ. 626, 11–21. doi: 10.1016/j.scitotenv.2018.01.071
Salas, J. D., Fu, C., Cancelliere, A., Dustin, D., Bode, D., Pineda, A., et al. (2005). Characterizing the severity and risk of drought in the Poudre River, Colorado. J. Water Resour. Plan. Manag. 131, 383–393. doi: 10.1061/(ASCE)0733-9496(2005)131:5(383)
Sanchez, G. M., Terando, A., Smith, J. W., García, A. M., Wagner, C. R., and Meentemeyer, R. K. (2020). Forecasting water demand across a rapidly urbanizing region. Sci. Total Environ. 730:139050. doi: 10.1016/j.scitotenv.2020.139050
Santikayasa, I. P., Babel, M. S., Shrestha, S., Jourdain, D., and Clemente, R. S. (2014). Evaluation of water use sustainability under future climate and irrigation management scenarios in Citarum River Basin, Indonesia. Int. J. Sustain. Dev. World Ecol. 21, 181–194. doi: 10.1080/13504509.2014.884023
Saraswat, C., Mishra, B. K., and Kumar, P. (2017). Integrated urban water management scenario modeling for sustainable water governance in Kathmandu Valley, Nepal. Sustain. Sci. 12, 1037–1053. doi: 10.1007/s11625-017-0471-z
Seo, M., Jaber, F., and Srinivasan, R. (2017a). Evaluating various low-impact development scenarios for optimal design criteria development. Water (Switzerland) 9:270. doi: 10.3390/w9040270
Seo, M., Jaber, F., Srinivasan, R., and Jeong, J. (2017b). Evaluating the impact of Low Impact Development (LID) practices on water quantity and quality under different development designs using SWAT. Water (Switzerland) 9:193. doi: 10.3390/w9030193
Sharvelle, S., Dozier, A., Arabi, M., and Reichel, B. (2017). A geospatially-enabled web tool for urban water demand forecasting and assessment of alternative urban water management strategies. Environ. Model. Softw. 97, 213–228. doi: 10.1016/j.envsoft.2017.08.009
Shrestha, S., Bhatta, B., Shrestha, M., and Shrestha, P. K. (2018). Integrated assessment of the climate and landuse change impact on hydrology and water quality in the Songkhram River Basin, Thailand. Sci. Total Environ. 643, 1610–1622. doi: 10.1016/j.scitotenv.2018.06.306
Sodoudi, S., Shahmohamadi, P., Vollack, K., Cubasch, U., and Che-Ani, A. I. (2014). Mitigating the urban heat island effect in megacity Tehran. Adv. Meteorol. 2014:547974. doi: 10.1155/2014/547974
Stephens, S. A., Bell, R. G., and Lawrence, J. (2018). Environmental research letters developing signals to trigger adaptation to sea-level rise developing signals to trigger adaptation to sea-level rise. Environ. Res. Lett 13:104004. doi: 10.1088/1748-9326/aadf96
Stone, B., Mednick, A. C., Holloway, T., and Spak, S. N. (2007). Is compact growth good for air quality? J. Am. Plan. Assoc. 73, 404–418. doi: 10.1080/01944360708978521
Themeßl, M. J., Gobiet, A., and Heinrich, G. (2012). Empirical-statistical downscaling and error correction of regional climate models and its impact on the climate change signal. Clim. Change 112, 449–468. doi: 10.1007/s10584-011-0224-4
U.S. Bureau of Reclamation (2013). Downscaled CMIP3 and CMIP5 Climate and Hydrology 795 Projections: Release of Downscaled CMIP5 Climate Projections, Comparison With Preceding 796 Information, and Summary of User Needs. Washington, DC: U.S. Bureau of Reclamation.
Wang, D., Hejazi, M., Cai, X., and Valocchi, A. J. (2011). Climate change impact on meteorological, agricultural, and hydrological drought in central Illinois. Water Resour. Res. 47, 1–13. doi: 10.1029/2010WR009845
Warziniack, T., and Brown, T. C. (2019). The importance of municipal and agricultural demands in future water shortages in the United States. Environ. Res. Lett. 14:084036. doi: 10.1088/1748-9326/ab2b76
Western Resource Advocates (2003). Smart Water: A Comparative Study of Urban Water Use Efficiency Across the Southwest. 178. Available online at: http://www.westernresourceadvocates.org/water/smartwater.php (accessed April 12, 2021).
Wheeler, S. M. (2008). The evolution of built landscapes in Metropolitan Regions. J. Plan. Educ. Res. 27, 400–416. doi: 10.1177/0739456X08315889
Williams, D. (2020). The influence of statutory land use planning on water sensitive urban design practices. Australas. J. Water Resour. 24, 60–72. doi: 10.1080/13241583.2020.1746173
Wilson, B., and Chakraborty, A. (2013). The environmental impacts of sprawl: emergent themes from the past decade of planning research. Sustainability 5, 3302–3327. doi: 10.3390/su5083302
Xu, F., Wang, Z., Chi, G., and Zhang, Z. (2020). The impacts of population and agglomeration development on land use intensity: new evidence behind urbanization in China. Land Use Policy 95:104639. doi: 10.1016/j.landusepol.2020.104639
Yan, Y., Zhou, R., Ye, X., Zhang, H., and Wang, X. (2018). Suitability evaluation of urban construction land based on an approach of vertical-horizontal processes. ISPRS Int. J. Geo-Information 7:198. doi: 10.3390/ijgi7050198
Yevjevich, V. (1967) An Objective Approach to Definitions and Investigations of Continental Hydrologic Droughts, Hydrology Paper no. 23, Colorado State University, Fort Collins, CO.
Zambon, I., Colantoni, A., and Salvati, L. (2019). Horizontal vs vertical growth: understanding latent patterns of urban expansion in large metropolitan regions. Sci. Total Environ. 654, 778–785. doi: 10.1016/j.scitotenv.2018.11.182
Zhang, D., Gersberg, R. M., Ng, W. J., and Tan, S. K. (2017). Conventional and decentralized urban stormwater management: a comparison through case studies of Singapore and Berlin, Germany. Urban Water J. 14, 113–124. doi: 10.1080/1573062X.2015.1076488
Zhang, W., Li, W., Zhang, C., Hanink, D. M., Liu, Y., and Zhai, R. (2018). Analyzing horizontal and vertical urban expansions in three East Asian megacities with the SS-coMCRF model. Landsc. Urban Plan. 177, 114–127. doi: 10.1016/j.landurbplan.2018.04.010
Zhang, W., Li, Y., Li, Z., Wei, X., Ren, T., Liu, J., et al. (2020). Impacts of climate change, population growth, and urbanization on future population exposure to long-term temperature change during the warm season in China. Environ. Sci. Pollut. Res. 27, 8481–8491. doi: 10.1007/s11356-019-07238-9
Zhao, G., Gao, H., Kao, S. C., Voisin, N., and Naz, B. S. (2018). A modeling framework for evaluating the drought resilience of a surface water supply system under non-stationarity. J. Hydrol. 563, 22–32. doi: 10.1016/j.jhydrol.2018.05.037
Zhao, M., Huang, S., Huang, Q., Wang, H., Leng, G., and Xie, Y. (2019). Assessing socio-economic drought evolution characteristics and their possible meteorological driving force. Geomatics Nat. Hazards Risk 10, 1084–1101. doi: 10.1080/19475705.2018.1564706
Keywords: water shortage, climate change, urban development, drought, sprawl, high density, water demand
Citation: Heidari H, Arabi M, Warziniack T and Sharvelle S (2021) Effects of Urban Development Patterns on Municipal Water Shortage. Front. Water 3:694817. doi: 10.3389/frwa.2021.694817
Received: 13 April 2021; Accepted: 21 June 2021;
Published: 09 July 2021.
Edited by:
Chingwen Cheng, Arizona State University, United StatesReviewed by:
Katarzyna Pietrucha-Urbanik, Rzeszów University of Technology, PolandErik Porse, California State University, Sacramento, United States
Copyright © 2021 Heidari, Arabi, Warziniack and Sharvelle. This is an open-access article distributed under the terms of the Creative Commons Attribution License (CC BY). The use, distribution or reproduction in other forums is permitted, provided the original author(s) and the copyright owner(s) are credited and that the original publication in this journal is cited, in accordance with accepted academic practice. No use, distribution or reproduction is permitted which does not comply with these terms.
*Correspondence: Hadi Heidari, hadi.heidari@colostate.edu