- 1Freshwater Research Centre, Cape Town, South Africa
- 2Faculty of Science, Nelson Mandela University, Port Elizabeth, South Africa
Benthic macroinvertebrates are commonly used to assess water quality and ecological condition of aquatic ecosystems and they form the basis of several biotic indices. Many of these biotic indices are based on rapid bioassessment protocols (RBP). The first RBP based on macroinvertebrates, developed in Africa in the early 1990s, was the South Africa Scoring System (SASS). Since then SASS has been widely used in southern Africa and beyond, and has formed the basis of several other RBPs developed in Africa. This paper explores the RBPs and associated biotic indices currently used in Africa, primarily those that are rapid, field-based with low taxonomy (mostly family level) and which rely on sensitivity weightings of individual taxa to generate three metrics for interpreting water quality and ecological condition of aquatic ecosystems. Recommendations for future regional adaptation of RBPs, including calibration, validation, and modification of RBPs and biotic indices for new regions are provided. To date, five RBPs have been developed in Africa, while some existing biotic indices have been used outside their intended regional range. Key to the efficacy of any RBP and associated biotic index is the ability to detect a water quality impact, or change in river health. Important considerations when adapting an index for a new region or country include evaluating the suitability of the sampling protocol to local river conditions, evaluating the distribution of aquatic macroinvertebrate taxa in the region, assigning sensitivity weightings to new taxa in the region, evaluating the ability of the biotic index to detect impacts, evaluating within-country spatial and temporal variability in macroinvertebrate assemblages, and developing appropriate data interpretation guidelines based on metric scores and reference conditions. Often several iterations of a biotic index are needed, with improvement in efficacy with each version, following spatially and temporally comprehensive sampling. Future RBPs developed for bioassessment of rivers in Africa will promote the protection, conservation, and management of African riverine ecosystems.
Introduction
Benthic macroinvertebrates are commonly used to assess river water quality and form the basis of several biotic indices including those used in the United Kingdom (e.g., Armitage et al., 1983; Wright et al., 1998), Europe (e.g., Alba-Tercedor and Sánchez-Ortega, w1988; Camargo, 1993; Bonada et al., 2006), North America (e.g., Hilsenhoff, 1988; Rosenberg and Resh, 1993; Barbour et al., 1999), South America (e.g., Baptista et al., 2007; Buss and Vitorino, 2010), Asia (e.g., Morse et al., 2007; Hartmann et al., 2010; Blakely et al., 2014), Australia (e.g., Chessman, 1995, 2003; Smith et al., 1999), New Zealand (e.g., Stark, 1993, 1998; Stark and Maxted, 2007), and Africa (e.g., Chutter, 1972, 1998; Dickens and Graham, 2002; Palmer and Taylor, 2004; Ollis et al., 2006; Dallas, 2009; Kaaya et al., 2015; Dallas et al., 2018). Many of these biotic indices are based on rapid bioassessment protocols (RBPs), which provide a reliable, quick, and cost-effective method for evaluating water quality in perennial rivers. Macroinvertebrate-based biotic indices often form the primary tool for management of water quality and river health in riverine ecosystems (Ollis et al., 2006).
RBPs based on macroinvertebrates are typically qualitative, multihabitat (= multibiotope), rapid, field-based methods that derive metrics using sensitivity weightings of individual taxa, which reflect their water quality tolerances (Dallas, 1995, Dallas, 1997). For all RBPs, the associated biotic index generates three metrics, namely Total Score (sum of the sensitivity weightings of taxa recorded at a site: SASS Score, NASS Score, TARISS Score, ZISS Score, OKASS Score), Number of Taxa and Average Score Per Taxon (ASPT = Total Score divided by Number of Taxa). The sampling equipment, habitats or biotopes sampled, time or effort for sampling and processing of samples sometimes differ amongst RBPs (Bonada et al., 2006). In particular, choice of biotope sampled varies from multihabitat to single biotope, and combining samples from similar biotopes based on substrate similarities (e.g., all stone, all vegetation) vs. hydraulic similarities (e.g., all in-current samples). Processing of samples is commonly field-based to family-level, although processing varies amongst protocols, and the advantages of laboratory vs. field-based processing and taxonomic resolution have been argued (Carter and Resh, 2001; Bonada et al., 2006). Numerous biotic indices, especially those reliant on rapid, field-based protocols, use family-level taxonomic resolution because it is easier and less expensive (Bonada et al., 2006).
Although Chutter (1972) developed a biotic index for South African rivers in 1972, it was labor-intensive and thus never gained traction for biomonitoring (Chutter, 1998). Subsequently, a quicker and simpler index was developed, the South African Scoring System (SASS), which constituted the first RBP in Africa (Dallas, 1997; Chutter, 1998; Dickens and Graham, 2002). SASS was derived from the Biological Monitoring Working Party system (BMWP) (Hawkes, 1997) and modified for assessing water quality and condition of South African rivers (Dallas, 1997, Chutter, 1998). SASS5 (version 5) has been applied without modification in other regions of southern Africa, including Zimbabwe (Phiri, 2000; Ndebele-Murisa, 2012; Bere and Nyamupingidza, 2014; Mwedzi et al., 2016), Swaziland (Mthimkhulu et al., 2004), and Kenya (Oigara and Masese, 2017). Bere and Nyamupingidza (2014) confirmed the ability of SASS5 to reflect water quality and ecological health of lotic systems in Zimbabwe, which they attributed to the dominance of widely occurring macroinvertebrate taxa in their study (Bere and Nyamupingidza, 2014). In comparison, studies in more tropical regions such as Kenya, concluded that there is a need for testing and validation of the protocol before extending its use beyond South Africa (Oigara and Masese, 2017). Elias et al. (2014b) recommended that tropical African regions ideally need to develop their own RBP and biotic indices, rather than relying on indices developed from other geographical areas, especially non-tropical regions. SASS has also been used in other sub-Saharan regions of Africa including Ethiopia and West Africa (pers. Comm. R. Palmer).
SASS5 has been regionally modified for bioassessment of rivers in other African countries including Namibia: Namibian Scoring System (NASS; Palmer and Taylor, 2004), Tanzania: Tanzania River Scoring System (TARISS; Kaaya et al., 2015) and Zambia: Zambian Invertebrate Scoring System (ZISS; Dallas et al., 2018). It has also been adapted for non-wadeable, deltaic aquatic biotopes in the Okavango Delta in Botswana: Okavango Assessment System (OKASS; Dallas, 2009). TARISS has been used in Ugandan and Rwandan rivers to assess water quality (Dusabe et al., 2019; Tumusiime et al., 2019), although identifications of macroinvertebrates were done on preserved samples in the laboratory and not in the field, and in the case of Dusabe et al. (2019), the TARISS sampling protocol was not followed as biotopes were combined.
Several other studies have used other indices, including multi-metric indices such as B-IBI (Benthic Index of Biological Integrity, Barbour et al., 1999) for aquatic macroinvertebrates in rivers in Tanzania (Elias et al., 2014b) and Kenya (Masese et al., 2009). In these studies, the strength of the correlations between each metric and water quality allowed for the selection of a sub-set of metrics for inclusion in the final multi-metric index and subsequent calculation of integrity classes and B-IBI Scores. A laboratory-based macroinvertebrate biotic score system (ETHbios) has been developed in Ethiopia for assessing the ecological status of rivers (Aschalew and Moog, 2015). Other countries, including Nigeria, have used various biometric indices including Hilsenhoff's Biotic Index (Hilsenhoff, 1982) to evaluate water quality (Ogbeibu et al., 2013).
While, RBPs and associated biotic indices developed in one country or region may be applied in other countries or regions, it is recommended that they are calibrated, validated, and modified to ensure their effectiveness (Kaaya et al., 2015; Dallas et al., 2018). The aim of this paper is to examine and discuss RBPs and biotic indices currently used in rivers in Africa, in particular those RBPs that are rapid, field-based with low taxonomic resolution (mostly family level) and which rely on sensitivity weightings of individual taxa to generate three metrics for interpreting water quality and ecological condition of aquatic ecosystems. The paper further aims to provide recommendations for regional calibration, validation, and modification of these RBPs and associated biotic indices for application in new regions.
Methods
An extensive literature search was undertaken to source published studies on RBPs based on aquatic macroinvertebrates in African rivers (Figure 1). The literature search was conducted by means of Google Scholar, using a combination of the following search criteria: Africa, RBP, aquatic invertebrate, biomonitoring, ecological condition, macroinvertebrate, rapid bioassessment, and river health. Relevant articles were identified based on their titles, abstracts, methods, and results sections. Focus was on rapid, field-based protocols with low taxonomy (mostly family level) including SASS (Dickens and Graham, 2002), NASS (Palmer and Taylor, 2004), TARISS (Kaaya et al., 2015), and ZISS (Dallas et al., 2018), which are all RBPs based on aquatic macroinvertebrates developed for rivers. In addition, the Okavango Assessment System (OKASS; Dallas, 2009) was also included as this was adapted from SASS for use non-wadeable, deltaic aquatic biotopes in the Okavango Delta, Botswana. Each of these RBPs were evaluated to glean information on the sampling protocols used (biotopes sampled, duration/area sampled), sampling equipment used (kick sampling, equipment), aquatic macroinvertebrate taxonomy (taxonomic level, number of taxa, common and unique taxa), distribution and sensitivity weightings (range and actual value); methods for detecting and evaluating impacts (analyses used, human disturbance gradients, water quality correlations); spatial and temporal heterogeneity (spatial distribution of sites and seasonal sampling), and data interpretation guidelines (hierarchical spatial frameworks). In addition, the number of assessments used for the development of each RBP was ascertained. This provided an indication of the regional robustness of each biotic index. The results for each of these aspects are presented and discussed.
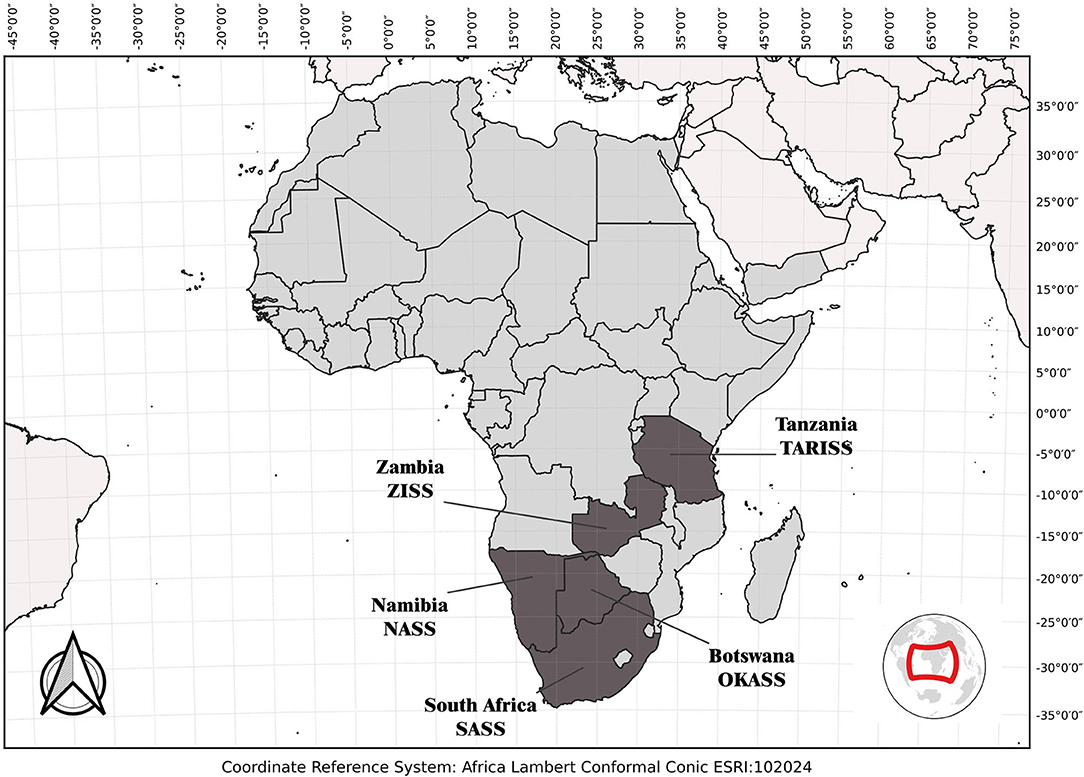
Figure 1. Rapid bioassessment protocols developed in Africa including the South African Scoring System (SASS; Dickens and Graham, 2002), the Namibian Scoring System (NASS; Palmer and Taylor, 2004), the Okavango Assessment System (OKASS; Dallas, 2009), the Tanzania River Scoring System (TARISS; Kaaya et al., 2015), and the Zambian Invertebrate Scoring System (ZISS; Dallas et al., 2018).
Results
Sampling Protocols
The SASS, NASS, TARISS, and ZISS sampling protocols are all applied in wadeable (<1 m depth, <20 m wide) rivers, while the ZISS protocol also includes a method for larger non-wadeable rivers (>20 m wide). These protocols are intended for use in low to moderate flows and are not to be used during high flow (flood) events, or in lentic systems such as wetlands and impoundments, or in estuaries or ephemeral rivers (Dickens and Graham, 2002). The sampling protocol of these four RBPS are similar since they were all derived from SASS. An overview of the sampling protocols is provided in Table 1. Importantly, sampling is undertaken per biotope, namely stones (stones in current and stone out of current), vegetation (marginal and aquatic), and gravel/sand/mud, with effort for each biotope standardized by time or area (Table 1). The OKASS sampling protocol was adapted from SASS and is applied in non-wadeable, deltaic aquatic biotopes, with sampling undertaken per biotope including marginal vegetation in current (channel), marginal vegetation out of current (lagoon), floating vegetation, submerged vegetation, and seasonally-inundated floodplain.
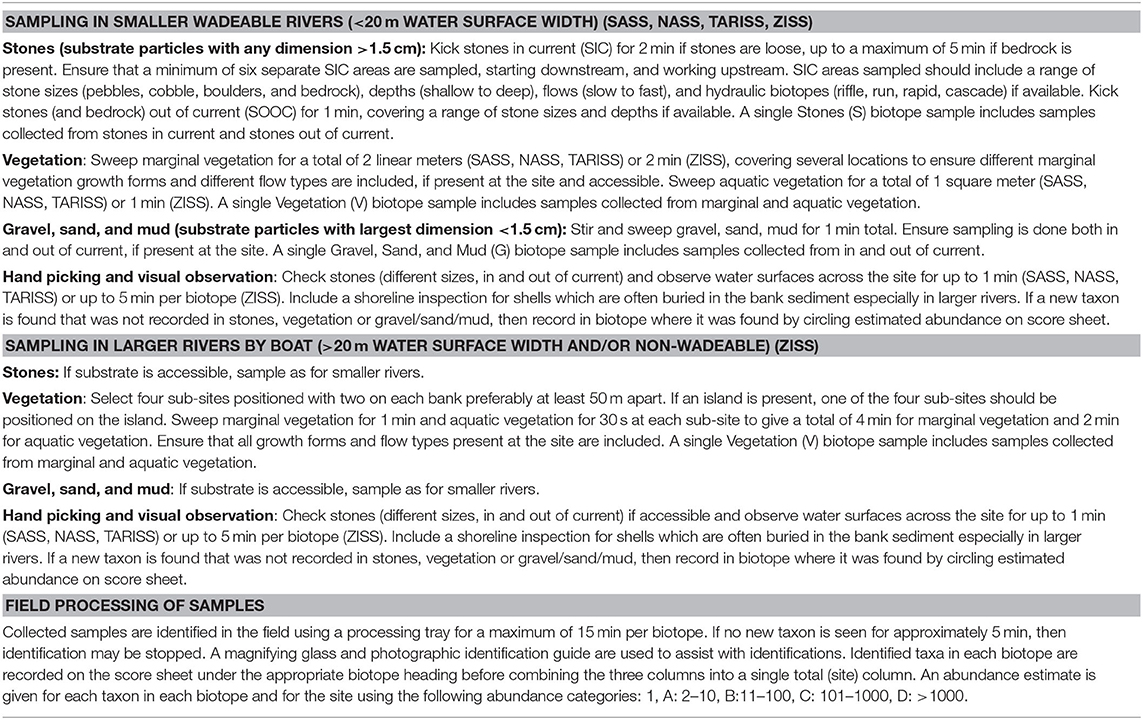
Table 1. Standardized rapid bioassessment protocol for rivers based on aquatic macroinvertebrates used for South African Scoring System (SASS), Namibian Scoring System (NASS), Tanzania River Scoring System (TARISS), and Zambian Invertebrate Scoring System (ZISS).
Aquatic Macroinvertebrate Taxonomy, Distribution, and Sensitivity Weightings
For existing RBPs, a taxon's tolerance to water quality impairment is reflected in its sensitivity weighting, with highly sensitive taxa assigned a weighting of 15, while highly tolerant taxa have a weighting of one. For all RBPs in Africa, sampled macroinvertebrates are identified to family level (or for a few taxa, e.g., Porifera, to a higher level; or a lower level, e.g., Baetidae and Hydropsychidae) on site using a magnifying glass. The total number of taxa in each RBP are 99 (SASS5), 93 (NASS2), 96 (TARISS1), 90 (ZISS1), and 58 (OKASS1). The number after the RBP name denotes the version, with SASS already in version 5, NASS in version 2, and the others in version 1. When the four RBPs developed for rivers are examined, 81 taxa are common to all (Table 2). Regional endemics such as those common in the south-western Cape, South Africa, and tropical taxa resulted in some taxa being absent from one or more RBPs.

Table 2. Taxa and sensitivity weightings for Rapid Bioassessment Protocols currently used in Africa, including the South African Scoring System (SASS, Dickens and Graham, 2002), Namibian Scoring System (NASS, Palmer and Taylor, 2004), Tanzania River Scoring System (TARISS, Kaaya et al., 2015) and Zambian Invertebrate Scoring System (ZISS, Dallas et al., 2018), and Okavango Assessment System (OKASS, Dallas, 2009).
SASS sensitivity weightings were initially derived using expert opinion and subsequently validated through field testing and correlation with impact. SASS underwent five revisions (SASS1 to SASS version 5) following extensive testing in several regions within South Africa by a number of river ecologists (Dallas, 1995, 1997; Chutter, 1998; Dickens and Graham, 2002) with more than 1,000 SASS assessments undertaken during this testing phase. This facilitated an iterative approach to the development of SASS, with new taxa added and sensitivity weightings adjusted based on observed data. Since then, more than 12,162 SASS assessments have been undertaken in South Africa (Freshwater Biodiversity Information System (FBIS), 2020) and it forms the backbone of river health assessment in South Africa. In comparison, NASS comprised approximately 50 assessments on the perennial rivers in Namibia (pers comm. R Palmer), TARISS comprised 101 assessments on 85 rivers in four freshwater ecoregions of Tanzania (Kaaya et al., 2015), ZISS comprised 151 assessments on 95 rivers across the country (Dallas et al., 2018), while OKASS comprised 103 assessments on 54 deltaic sites in three regions of the Okavango delta (Dallas, 2009). For all of these RBPs further use of the respective indices has taken place and will most likely be used to further improve the index. When an RBP is used in a new region, as part of the validation process, sensitivity weightings of existing taxa need to be checked and sensitivity weightings of new taxa assigned. Palmer and Taylor (2004) assigned sensitivity weightings to new taxa based on observed data and similarity with existing taxa. Canonical analysis of principal coordinates has been used to predict sensitivity weightings of new macroinvertebrate taxa along the disturbance gradient (Kaaya et al., 2015), as well as correlation of occurrence to impact ratings, evaluation of closely related SASS families, known life-history modes, and anatomical adaptations (Dallas et al., 2018).
Impact Detection and Evaluation
To evaluate the efficacy of the biotic index to demonstrate changes in river water quality, data on anthropogenic activities and ecosystem disturbance need to be collected at each site by assessing catchment, channel and habitat impacts. Both TARISS (Kaaya et al., 2015) and ZISS (Dallas et al., 2018) utilized versions of the site characterization protocol (Dallas, 2005) and Index of Habitat Integrity (Kleynhans, 1996), developed as part of the SASS testing phase in South Africa. This protocol evaluates the quantity and severity of anthropogenic impacts at a site and integrates potential impacts into an index of habitat integrity; in addition to assessing water quality impacts, modification to the channel, condition of the local catchment, and land-use (Dallas, 2005). For TARISS, levels of human disturbance across sites were derived by evaluating local catchment disturbance, instream and riparian habitat integrity (Kaaya et al., 2015). In Dallas et al. (2018) distinction between “impacted” and minimally impacted (or “unimpacted”) reference sites were used to generate thresholds of impact for each of the groups of impact. For OKASS, potential anthropogenic disturbances were used to calculate a Human Disturbance Score for each site (Dallas, 2009). To evaluate the ability of the biotic index to detect impacts and disturbance, metrics are correlated with the disturbance gradient to determine how each metric responds to the disturbance gradient, and to test differences among metrics from sites classified as impacted or reference.
Spatial and Temporal Heterogeneity
Dallas (2004a), Kaaya (2015), and Dallas et al. (2018) demonstrated that macroinvertebrate assemblages differed among river types and recommended the inclusion of a hierarchical spatial framework within which bioassessment data is interpreted. SASS, TARISS, and ZISS, all use two-level hierarchical spatial frameworks to offer geographic partitions within which macroinvertebrate assemblages are expected to be similar, thereby assisting in interpretation of bioassessment data (Table 3). Level I relate to broad geographic regions, while level II relates to longitudinal zonation of river systems. In South Africa, for Level I, 31 ecoregions were derived from vegetation and terrain, with inclusion of geology, soil, altitude, rainfall, air temperature, and runoff variability (Kleynhans et al., 2005). For Level II, simplified geomorphological zonation was used to differentiate rivers into Upland or Lowland (Dallas, 2007a), based on research that demonstrated macroinvertebrate assemblages are typically divided into upland and lowland assemblages, with little differentiation at the finer level, e.g., Mountain Stream vs. Upper Foothill (Dallas, 2004a). In Tanzania, for Level I, Kaaya (2015) used freshwater ecoregions of the world (Abell et al., 2008) and five climatic zones (Indeje et al., 2000) to divide the country into 12 ecoregions based on hydrological (catchment) boundaries and climatic characteristics. For the Level II, 12 landform features and three slope classes were used to generate a geomorphologic classification (Kaaya, 2015). In Zambia, for Level I, freshwater ecoregions of the world (Abell et al., 2008) were used, and for Level II, stream order (“high” = stream orders 7–9 and “low” = stream order 3–6) was used (Dallas et al., 2018). Further partitioning of spatial variability is also included at biotope level, with all RBPs undertaking sampling for each biotope separately, and in some cases also interpreting metrics per biotope (e.g., ZISS). In comparison, examination of spatial variation of aquatic macroinvertebrate assemblages in the Okavango Delta (Dallas and Mosepele, 2007, 2020) showed that assemblages did not vary significantly amongst different region of the Delta, although macroinvertebrate assemblages differed amongst aquatic biotopes (Table 3). On this basis, Dallas (2009) proposed preliminary data interpretation guidelines based on two dominant deltaic habitats, namely marginal vegetation in current, and marginal vegetation out of current.
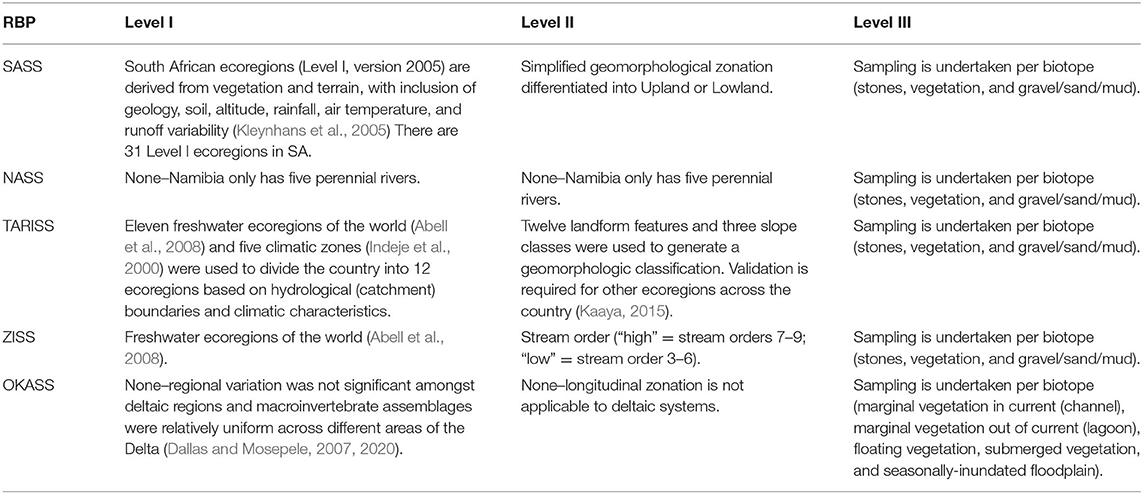
Table 3. Hierarchical spatial frameworks for Rapid Bioassessment Protocols (RBP) currently used in Africa, including the South African Scoring System (SASS, Dickens and Graham, 2002), Namibian Scoring System (NASS, Palmer and Taylor, 2004), Tanzania River Scoring System (TARISS, Kaaya et al., 2015) and Zambian Invertebrate Scoring System (ZISS, Dallas et al., 2018), and Okavango Assessment System (OKASS, Dallas, 2009).
Temporal variability was only examined for SASS (Dallas, 2004b) and OKASS (Dallas, 2009), with the latter focusing on high-water level period (July) and the low-water period (October), which is more appropriate for the deltaic biotopes. The influence of sampling season on macroinvertebrate assemblages, taxon occurrence, and SASS Scores was investigated and seasonal variability was shown to be more prevalent in some regions of South Africa and some biotopes (Dallas, 2004b), with SASS Scores in the stones in current biotope varying seasonally, inter-annually and in response to wet and dry cycles, as well as antecedent flow events, water temperature and abundance of benthic algae (Palmer, 1997). For OKASS, although more taxa were generally recorded during October, the low-water period, compared to July, the high-water period, this was not statistically significant (Dallas, 2009).
Data Interpretation Guidelines
SASS data interpretation guidelines are most advanced for South Africa, with guidelines provided for each “ecoregion-geomorphological zone” using the relationship between SASS Score and ASPT (Dallas, 2007a; Dallas and Day, 2007). Specifically, the number of biotopes sampled was positively correlated with SASS Score and number of taxa, while ASPT was negatively correlated with the number of biotopes (Dallas, 2007b). Data interpretation guidelines were not developed for NASS (Palmer and Taylor, 2004) as Namibia only has five perennial rivers, namely the Orange, Kunene, Okavango, Zambezi, and Chobe, while NASS has only been used in the north-eastern region. Data interpretation guidelines have not yet been developed TARISS (Kaaya et al., 2015). Provisional data interpretation guidelines have been produced for ZISS, although these require further testing and validation (Dallas et al., 2018). ZISS guidelines are based on metric scores (ZISS Score, Number of Taxa and ASPT) generated for reference sites within each “ecoregion-stream order-biotope” combination (e.g., Zambezian Headwaters-low order-stones). Dallas (2009) proposed preliminary data interpretation guidelines based OKASS Score for two deltaic biotopes, namely marginal vegetation in current, and marginal vegetation out of current.
Discussion
Sampling Protocols
The rationale for sampling biotopes separately is to ensure more accurate data interpretation since differences in availability of biotopes at a site may affect macroinvertebrate assemblages, given biotope preferences of some macroinvertebrate taxa (Dallas, 2007b). The availability of biotopes typically varies longitudinally down a river in response to broad geomorphological characteristics, with upper reaches dominated by stones, while lower reaches may only have vegetation and sand (Dallas, 2004a). When an RBP is being considered for use in a region outside the country where it was developed, it is important to consider the river systems prevalent in the region, including the variety of aquatic biotopes across rivers in the region. Biotopes sampled for all RBPs are dependent on which biotopes are present at the site. Where biotopes of the region resemble those of existing RBPs, then the sampling protocol can be adapted without much revision. For example, for ZISS, Dallas et al. (2018) concluded that stones and vegetation biotopes were more reliable than gravel/sand/mud in differentiating impacted from reference sites. Similarly, Dallas (2007b) noted that gravel/sand/mud biotope added very little to the SASS Scores or number of taxa in SASS. The earlier RBPs were limited to wadeable rivers, but since the development of OKASS and ZISS, non-wadeable, larger rivers, and deltaic biotopes may also be sampled. It should be emphasized that if the sampling protocol of an RBP is not followed (as summarized in Table 1), then it is not legitimate to assign sensitivity weightings to taxa recorded at a site to generate metrics for interpreting the impact of water quality impairment or deriving an ecological condition for the site. In particular, a result generated from a chemically preserved sample is not a legitimate SASS/NASS/TARISS/ZISS/OKASS result. Typical examples of where a protocol has not been adhered to include laboratory identification of preserved taxa instead of time controlled field-based identification, use of surber or box sampling instead of kick sampling, and only sampling one biotope at a site. The strict adherence to established sampling protocols is to ensure quality control and standardization so that results may be compared (Dickens and Graham, 2002).
Aquatic Macroinvertebrate Taxonomy, Distribution, and Sensitivity Weightings
Variation in geology and climate may influence physico-chemistry of river water, which may affect the distribution and sensitivity of macroinvertebrate assemblages (Day and King, 1995). When calibrating and validating a biotic index for a new region, it is important to undertake extensive sampling of macroinvertebrate assemblages across a range of sites in the region so that the full variety of taxa present in the region may be established. This is often done iteratively as a community of biomonitoring practitioners is formed within a region. Normally, when developing a RBP for a new region, macroinvertebrate samples collected from each biotope using the RBP would be preserved, and the identification of each taxon confirmed in the laboratory. This also facilitates the creation of a reference collection of aquatic macroinvertebrates for the region, which is a particularly useful resource for new biomonitoring programmes. Extensive sampling and taxonomic confirmation enable the detection of new taxa, not previously included in existing RBPs, including regionally endemic taxa, and provides evidence of the absence of other taxa in a region. Unfortunately, the development and adaptation RBPs and biotic indices is often hindered by poorly known taxonomy (e.g., tropical East Africa, Elias et al., 2014a, Ochieng et al., 2019). Fortunately, the RBPs described in this paper are mostly family level, which has greater taxonomic confidence than genus or species. Indeed, the use of predominantly family-level taxonomy in RBPs is a prerequisite since they are field-based.
The full range of taxa present may differ within a region, depending on latitudinal differences and regional endemism. The majority of taxa in the four RBPs developed for rivers were common to all (81 taxa), with a few regional endemics and less common taxa comprising the balance. Some taxa (Dicercomyzidae, Ephemerythidae, Machadorythidae, Sisyridae, Curculionidae, Sciomyzidae, Stratiomyidae, Ampulariidae, Bithyniidae, and Mutelidae) included in NASS, TARISS, and ZISS should ideally be included in future versions of SASS as these taxa have been recorded in the South Africa, and a further revision of SASS is likely. In addition, a recent study in Uganda noted five new taxa to be included in a modified TARISS, namely Chordodidae, Ptilodactylidae, Aspidytidae, Leptopodidae, and Paraecnomidae, which were not included in the TARISS (Tumusiime et al., 2019), although the latter is not a recognized family and is included in Ecnomidae. Importantly, prior to any new taxon being included in a RBP developed for a new region, it is recommended that all identifications be confirmed by a recognized institute and their taxonomic classification be verified on the taxonomic backbone of the Global Biodiversity Information Facility. Taxa excluded from the list of taxa during the validation process for a new region, are normally because they were not sampled in the new region. In some instances, this may be due to limited sampling or because the taxon is rare, or in other cases it may be because the taxon does indeed not occur in the region, such as those taxa endemic to the south-western Cape, South Africa. This emphasizes the importance of undertaking extensive sampling across the region to ensure that all potential taxa are sampled and included in the updated list of taxa for a new region. It is also useful to calculate the frequency with which each taxon is recorded in a region to provide an indication of taxon rarity.
When a RBP and biotic index are developed for a new region, attention should be given to validating the sensitivity weightings assigned to each macroinvertebrate family in the region. Existing indices apply different weightings for two families (Baetidae and Hydropsychidae) based on the number of species within each family sampled and recorded at a site. Families that have many species may exhibit a wider range of within-family tolerance compared to families with few species (Bonada et al., 2006). As part of the validation process, sensitivity weightings of existing taxa need to be checked and sensitivity weightings of new taxa assigned based on correlation with disturbance gradients, sensitivity weightings of closely related families, known life-history modes, anatomical adaptations, and expert knowledge. It is recommended that the RBP be assigned a version to facilitate keeping track of revisions and that an iterative approach be adopted when developing a RBP for a new region.
Impact Detection and Evaluation
Biotic indices should be able to detect changes in water quality and ecological condition of the aquatic ecosystem they are designed to be used in. As part of the regional validation process it is necessary for the index to be applied across a range of sites exhibiting a gradient of impacts. During the testing of the index, and undertaken concurrently with sampling of macroinvertebrates, data on anthropogenic activities, and ecosystem disturbance need to be collected at each site by assessing catchment, channel, and habitat impacts. Strong correlations between the metrics and human disturbance provides confidence in the biotic indices to detect impacts and disturbance. The relationships between metrics and disturbance can be evaluated for each biotope (Dallas et al., 2018) and for the site as a whole (Kaaya et al., 2015). Recently, Tumusiime et al. (2019) showed that macroinvertebrate assemblages differed between test (= impacted) and reference sites, which provided confidence in the ability of the TARISS to distinguish impacted and reference sites, thus validating the efficacy of TARISS in Uganda.
Spatial and Temporal Heterogeneity
Lotic systems are intrinsically heterogeneous with spatial and temporal variability occurring at multiple scales. Spatial variability may result from catchment-scale factors such as altitude, geology, channel slope; site-scale factors such as canopy cover, stream width, stream depth; and habitat-scale factors such as substratum, biotope availability, hydraulics (Dallas, 2007c). These factors may effect macroinvertebrate assemblage structure and an understanding of spatial variation in macroinvertebrate assemblages and the use of biotic indices based on these assemblages, is thus needed. Spatial frameworks are often used to overcome this intrinsic spatial variability and improve the reliability of data interpretation. The hierarchical spatial frameworks developed for SASS, TARISS, and ZISS, facilitated partitioning of intrinsic spatial variability, although further testing has been recommended for TARISS (Kaaya, 2015). The inclusion of biotope level sampling ensures that substrate, which has been identified as an important predictor for classification of macroinvertebrates in rivers (Dallas, 2007c), is considered. During the initial validation of the SASS protocol, the issue arose as to whether to combine samples based on substrate similarities (e.g., all stone, all vegetation) or hydraulic similarities (e.g., all in-current samples), with the former selected for SASS. Subsequent comparison of two RBPs, SASS, and the Iberian Peninsula (IB-protocol), which combined hydraulic biotopes (Prat et al., 2000), confirmed the similarity of the bioassessment results and the ability of each RBP to detect water quality impacts even though the RBPs used different biotope combinations (substrate vs. hydraulic) and had different sampling equipment (mesh diameter), sampling and laboratory processing methods (Bonada et al., 2006).
Temporal variability in macroinvertebrate assemblages may occur in response to seasonal variability in factors such as water temperature (Dallas, 2008), biotope availability (Armitage et al., 1995), and stream flow (McElravy et al., 1989). Given that seasonal variability was prevalent in some regions of South Africa and some biotopes (Dallas, 2004b), Dickens and Graham (2002) recommended that season be factored into SASS data interpretation, as some natural intra- and inter-annual variation in macroinvertebrate assemblages is likely. Kaaya et al. (2015) recognized the potential influence of seasonal variability on TARISS and recommended that it be examined for each river type in Tanzania.
Data Interpretation Guidelines
Developing appropriate data interpretation guidelines is perhaps the most challenging aspect when developing RBPs and biotic indices for a new region. However, it is critical to ensure that impacts reflected in the metrics are real and not merely a consequence of intrinsic spatial and temporal variability of macroinvertebrate assemblages, as this may affect our ability to interpret bioassessment data. An understanding of variability is important to facilitate the establishment of reference conditions, including expected macroinvertebrate assemblages (Dallas, 2004a,b; Dallas and Day, 2007). Reference conditions represent the natural or least-impacted condition for a particular type of river, and are used as a measure with which impacted sites are compared. Highly variable systems may lead to patchiness of taxa, which need to be considered when developing the mechanism for interpreting bioassessment data (Dallas, 2004a,b; Dallas, 2007b; Dallas and Day, 2007). The basis for interpretation of metrics for a particular sample is understanding the “natural” variability of metrics from the site or similar sites (i.e., variability of reference sites) and whether the metric for a test site falls within (unimpacted = reference) or outside (impacted) that variation.
Normally metrics are used for assessing ecological condition, either as tables or graphs showing different ecological categories. Embedding data interpretation within a spatial framework, as for SASS, provides a robust, easy to use system for evaluating change in water quality and ecological condition. Spatial and temporal variability may be accounted for by defining the reference condition as a band (Dallas and Day, 2007). Further, by utilizing the relationship between SASS Score and ASPT, between-site variation in the availability of biotopes is taken into account (Dallas and Day, 2007). The validity of this relationship for metrics derived from other RBPs and biotic indices in other regions, however, would need to be tested. Kaaya et al. (2015), while demonstrating that the validated TARISS technique is a dependable method for rapid bioassessment of rivers in Tanzania, advised that interpretation guidelines for each river type still need to be developed, using the “river type specific reference condition” approach. Since TARISS was only validated in two Tanzanian ecoregions, further validation in the other ecoregions is needed before it qualifies as a national biotic index.
Conclusion
This paper outlines important aspects that need to be considered when assessing water quality and river condition using RBPs and associated biotic indices. RBPs developed for new regions, and which are based on existing RBPs and indices, need to be comprehensively tested, calibrated, validated, and modified before they can be used with confidence in a new region. Of the five RBPs currently used in Africa, SASS has been the most widely tested across a range of river types, providing insight into spatial, and temporal variability of the biotic index. It has proven its value as a rapid, cost-effective and reliable tool for assessing river water quality and health. Other RBPs such as TARISS, ZISS, and OKASS require further within-region testing, especially in relation to spatial and temporal variability and interpretation of data. While TARISS1 has been developed within a spatial framework and validated for two regions in Tanzania, Kaaya et al. (2015) recommended further regional expansion and testing. Dallas et al. (2018) demonstrated that ZISS1 could detect moderate to high anthropogenic impacts on water quality and river condition. Dallas et al. (2018) recommended that ZISS only be done at sites with stones and/or vegetation biotope(s) and not at sites with only gravel/sand/mud, as these sites were not suitable for ZISS. Further sampling however is suggested to test this observation as well as the sensitivity of ZISS to a range of pollution types and intensities. Dallas (2009) recommended that further sampling of selected sites be undertaken to allow for the generation of additional data at both reference and monitoring sites, to help test and refine OKASS.
Confidence in the efficacy of an RBP and associated biotic index to assess river water quality and condition, is dependent on adequate calibration and validation of the index, including the development of appropriate data interpretation guidelines. The development of RBPs from other countries have shown that, whilst many RBPs are cost-effective, with less training and equipment requirements compared to more intensive monitoring protocols; the development of RBPs requires large amounts of data, collected iteratively together with testing and adaptation (Wright et al., 1998; Smith et al., 1999). Reliability of data is key. This is achieved by standardizing the RBP and including quality control measures such as practitioner accreditation (Dickens and Graham, 2002). Whilst RBPs currently used in Africa do not incorporate predictive models such as in RIVPACS (Wright et al., 1998) and AusRivAS (Smith et al., 1999), it is not beyond the scope of a calibrated and validation RBP and biotic index, and holistically implemented biomonitoring system, to facilitate the development of predictive models in the future. RBPs developed for bioassessment of rivers in Africa will promote the protection, conservation and management of African riverine ecosystems.
Author Contributions
The author confirms being the sole contributor of this work and has approved it for publication.
Conflict of Interest
The author declares that the research was conducted in the absence of any commercial or financial relationships that could be constructed as a potential conflict of interest.
References
Abell, R., Thieme, M., Revenga, C., Bryer, M., Kottelat, M., Bogustskaya, N., et al. (2008). Freshwater ecoregions of the world: a new map of biogeographic units for freshwater biodiversity conservation. BioScience 58, 403–414. doi: 10.1641/B580507
Alba-Tercedor, J., and Sánchez-Ortega, A. (1988). Un método rápido y simple para evaluar la calidad biológica de las aguas corrientes basado en el de Hellawell (1978). Limnetica 4, 51–56.
Armitage, P. D., Moss, D., Wright, J. F., and Furse, M. T. (1983). The performance of a new biological water quality score system based on macroinvertebrates over a wide range of unpolluted running-water sites. Water Res. 17, 333–347. doi: 10.1016/0043-1354(83)90188-4
Armitage, P. D., Pardo, I., and Brown, A. (1995). Temporal constancy of faunal assemblages in “mesohabitats”–application to management? Arch. Hydrobiol. 133, 367–387.
Aschalew, L., and Moog, O. (2015). Benthic macroinvertebrates based new biotic score “ETHbios” for assessing ecological conditions of highland streams and rivers in Ethiopia. Limnologica 52:11–19. doi: 10.1016/j.limno.2015.02.002
Baptista, D. F., Buss, D. F., Egler, M., Giovanelli, A., Silveira, M. P., and Nessimian, L. (2007). A multimetric index based on benthic macroinvertebrates for evaluation of Atlantic Forest streams at Rio de Janeiro State, Brazil. Hydrobiologia 575, 83–94. doi: 10.1007/s10750-006-0286-x
Barbour, M. T., Gerritsen, J., Snyder, B. D., and Stribling, J. B. (1999). Rapid Bioassessment Protocols for Use in Streams and Wadeable Rivers: Periphyton, Benthic Macroinvertebrates, and Fish, 2nd Edn. Report EPA 841-B-99-002. US Environmental Protection Agency, Office of Water, Washington, DC.
Bere, T., and Nyamupingidza, B. B. (2014). Use of biological monitoring tools beyond their country of origin: a case study of the South African Scoring System Version 5 (SASS5). Hydrobiologia 722, 223–232. doi: 10.1007/s10750-013-1702-7
Blakely, T. J., Eikaas, H. S., and Harding, J. S. (2014). The Singscore: a macroinvertebrate biotic index for assessing the health of Singapore's streams and canals. Raffles Bull. Zool. 62, 540–548.
Bonada, N., Dallas, H. F., Rieradevall, M., Prat, N., and Day, J.A. (2006). A comparison of rapid bioassessment protocols used in two regions with Mediterranean climates, the Iberian Peninsula and South Africa. J. North Am. Benthol. Soc. 25, 487–500. doi: 10.1899/0887-3593(2006)25[487:ACORBP]2.0.CO;2
Buss, D. F., and Vitorino, A. S. (2010). Rapid bioassessment protocols using benthic macroinvertebrates in Brazil: evaluation of taxonomic sufficiency. J. North Am. Benthol. Soc. 29, 562–571. doi: 10.1899/09-095.1
Camargo, J. A. (1993). Macrobenthic surveys as a valuable tool for assessing freshwater quality in the Iberian Peninsula. Environ. Monit. Assess. 24, 71–90. doi: 10.1007/BF00568800
Carter, J. L., and Resh, V. H. (2001). After site selection and before data analysis: sampling, sorting, and laboratory procedures used in stream benthic macroinvertebrate monitoring programs by USA state agencies. J. North Am. Benthol. Soc. 20, 658–682. doi: 10.2307/1468095
Chessman, B. C. (1995). Rapid assessment of rivers using macroinvertebrates: a procedure based on habitat-specific sampling, family level identification, and a biotic index. Aust. J. Ecol. 20, 122–129. doi: 10.1111/j.1442-9993.1995.tb00526.x
Chessman, B. C. (2003). New sensitivity grades for Australian river macroinvertebrates. Mar. Freshwater Res. 54, 95–103. doi: 10.1071/MF02114
Chutter, F. M. (1972). An empirical biotic index of the quality of water in South African streams and rivers. Water Res. 6, 19–30. doi: 10.1016/0043-1354(72)90170-4
Chutter, F. M. (1998). Research on the Rapid Biological Assessment of Water Quality Impacts in Streams and Rivers. WRC Report No. 422/1/98. Water Research Commission, Pretoria, South Africa.
Dallas, H. F. (1995). An evaluation of SASS (South African scoring system) as a tool for the rapid bioassessment of water quality (MSc thesis). University of Cape Town, Cape Town, South Africa.
Dallas, H. F. (1997). A preliminary evaluation of aspects of SASS (South African Scoring System) for the rapid bioassessment of water quality in rivers, with particular reference to the incorporation of SASS in a national biomonitoring programme. South Afr. J. Aquat. Sci. 23, 79–94. doi: 10.1080/10183469.1997.9631389
Dallas, H. F. (2004a). Spatial variability in macroinvertebrate assemblages: comparing regional and multivariate approaches for classifying reference sites in South Africa. Afr. J. Aquat. Sci. 29, 161–171. doi: 10.2989/16085910409503807
Dallas, H. F. (2004b). Seasonal variability of macroinvertebrate assemblages in two regions of South Africa: implications for aquatic bioassessment. Afr. J. Aquat. Sci. 29, 173–184. doi: 10.2989/16085910409503808
Dallas, H. F. (2005). River Health Programme: Site Characterisation Field-Manual and Field-Data Sheets. National Biomonitoring Programme Report Series No 18. Pretoria: Institute for Water Quality Studies, Department of Water Affairs and Forestry.
Dallas, H. F. (2007a). River Health Programme: South African Scoring System (SASS) Data Interpretation Guidelines. Prepared for the Institute of Natural Resources and the Resource Quality Services River Health. Department of Water Affairs and Forestry. The Freshwater Consulting Group, Cape Town, South Africa.
Dallas, H. F. (2007b). The influence of biotope availability on macroinvertebrate assemblages in South African rivers: implications for aquatic bioassessment. Freshwater Biology 52, 370–380. doi: 10.1111/j.1365-2427.2006.01684.x
Dallas, H. F. (2007c). The effect of biotope-specific sampling for aquatic macroinvertebrates on reference site classification and the identification of environmental predictors in Mpumalanga, South Africa. Afr. J. Aquat. Sci. 32, 165–173. doi: 10.2989/AJAS.2007.32.2.8.205
Dallas, H. F. (2008). Water temperature and riverine ecosystems: an overview of knowledge and approaches for assessing biotic responses, with special reference to South Africa. Water SA 34, 393–404. doi: 10.4314/wsa.v34i3.180634
Dallas, H. F. (2009). Wetland Monitoring Using Aquatic Macroinvertebrates. Technical Report. Report 5/2009 Prepared for the Biokavango Project, Harry Oppenheimer Okavango Research Centre, University of Botswana. The Freshwater Consulting Group, University of Cape Town, Cape Town, South Africa.
Dallas, H. F., and Day, J. A. (2007). Natural variation in macroinvertebrate assemblages and the development of a biological banding system for interpreting bioassessment data—a preliminary evaluation using data from upland sites in the south-western Cape, South Africa. Hydrobiologia 575, 231–244. doi: 10.1007/s10750-006-0374-y
Dallas, H. F., Lowe, S., Kennedy, M. P., Saili, K., and Murphy, K. J. (2018). Zambian Invertebrate Scoring System (ZISS): a macroinvertebrate-based biotic index for rapid bioassessment of southern tropical African river systems. Afr. J. Aquat. Sci. 43, 325–344. doi: 10.2989/16085914.2018.1517081
Dallas, H. F., and Mosepele, B. (2007). A survey of the aquatic invertebrates of the Okavango Delta, Botswana. Afr. J. Aquat. Sci. 32, 1–11. doi: 10.2989/AJAS.2007.32.1.1.138
Dallas, H. F., and Mosepele, B. (2020). Spatial variability of aquatic macroinvertebrate assemblages in the Okavango Delta, Botswana: considerations for developing a rapid bioassessment tool. Afr. J. Aquat. Sci. 45, 350–363. doi: 10.2989/16085914.2019.1704215
Day, J. A., and King, J. M. (1995). Geographical patterns and their origins, in the dominance of major ions in South African rivers. South Afr. J. Sci. 91, 299–306.
Dickens, C. W. S., and Graham, P. M. (2002). The South African Scoring System (SASS) Version 5: rapid bioassessment method for rivers. Afr. J. Aquat. Sci. 27, 1–10. doi: 10.2989/16085914.2002.9626569
Dusabe, M., Wronski, T., Gomes-Silva, G., Plath, M., Albrecht, C., and Apio, A. (2019). Biological water quality assessment in the degraded Mutara rangelands, northeastern Rwanda. Environ. Monit. Assess. 191:139. doi: 10.1007/s10661-019-7226-5
Elias, J. D., Ijumba, J. N., and Mamboya, F. A. (2014a). Effectiveness and compatibility of non-tropical bio-monitoring indices for assessing pollution in tropical rivers–a review. Int. J. Ecosyst. 4, 128–134. doi: 10.5923/j.ije.20140403.05
Elias, J. D., Ijumba, J. N., Mgaya, Y. D., and Mamboya, F. A. (2014b). Study on freshwater macroinvertebrates of some Tanzanian rivers as a basis for developing biomonitoring index for assessing pollution in tropical African regions. J. Ecosyst. 2014, 1–8. doi: 10.1155/2014/985389
Freshwater Biodiversity Information System (FBIS) (2020). FBIS Version 3. Available online at: https://www.freshwaterbiodiversity.orgon (accessed January 27, 2021).
Hartmann, A., Moog, O., and Stubauer, I. (2010). “HKH screening”: a field bio-assessment to evaluate the ecological status of streams in the Hindu Kush-Himalayan region. Hydrobiologia 651, 25–37. doi: 10.1007/s10750-010-0288-6
Hawkes, H. A. (1997). Origin and development of the biological monitoring working Party system. Water Res. 32, 964–968. doi: 10.1016/S0043-1354(97)00275-3
Hilsenhoff, W. L. (1982). Using a Biotic Index to Evaluate Water Quality in Streams. Technical Bulletin no.132. Madison, WI: Department of Natural Resources.
Hilsenhoff, W. L. (1988). Rapid field assessment of organic pollution with a family-level biotic index. J. North Am. Benthol. Soc. 7, 65–68. doi: 10.2307/1467832
Indeje, M., Semazzi, F., and Ogallo, L. (2000). ENSO signals in East African rainfall seasons. Int. J. Climatol. 20, 19–46. doi: 10.1002/(SICI)1097-0088(200001)20:1 <19::AID-JOC449>3.0.CO;2-0
Kaaya, L. T. (2015). Towards a classification of Tanzanian rivers: a bioassessment and ecological management tool. A case study of the Pangani, Rufiji, and Wami–Ruvu river basins. Afr. J. Aquat. Sci. 40, 37–45. doi: 10.2989/16085914.2015.1008970
Kaaya, L. T., Day, J. A., and Dallas, H. F. (2015). Tanzania River Scoring System (TARISS): a macroinvertebrate based biotic index for rapid bioassessment of rivers. Afr. J. Aquat. Sci. 40, 109–117. doi: 10.2989/16085914.2015.1051941
Kleynhans, C. J. (1996). A qualitative procedure for the assessment of the habitat integrity status of the Luvuvhu River (Limpopo system, South Africa). J. Aquat. Ecosyst. Health 5, 41–54. doi: 10.1007/BF00691728
Kleynhans, C. J., Thirion, C., and Moolman, J. (2005). A Level I Ecoregion Classification System for South Africa, Lesotho, and Swaziland. Pretoria: Department of Water Affairs and Forestry–Resource Quality Services.
Masese, F. O., Raburu, P. O., and Muchiri, M. (2009). A preliminary benthic macroinvertebrate index of biotic integrity (B-IBI) for monitoring the Moiben River, Lake Victoria Basin, Kenya. Afr. J. Aquat. Sci. 34, 1–14. doi: 10.2989/AJAS.2009.34.1.1.726
McElravy, E. P., Lamberti, G. A., and Resh, V. H. (1989). Year-to-year variation in the aquatic macroinvertebrate fauna of a northern California stream. J. North Am. Benthol. Soc. 8, 51–63. doi: 10.2307/1467401
Morse, J. C., Bae, Y. J., Munkhjargal, G., Sangpradub, N., Tanida, K., Vshivkova, T. S., et al. (2007). Freshwater biomonitoring with macroinvertebrates in East Asia. Front. Ecol. Environ. 5, 33–42. doi: 10.1890/1540-9295(2007)5[33:FBWMIE]2.0.CO;2
Mthimkhulu, S., Dallas, H., Day, J., and Hoko, Z. (2004). “Biological assessment of the state of the water quality in the Mbuluzi River, Swaziland,” in Proceedings of the IWA Specialist Group Conference on Water and Wastewater Management for Developing Countries (Livingstone).
Mwedzi, T., Bere, T., and Mangadze, T. (2016). Macroinvertebrate assemblages in agricultural, mining, and urban tropical streams, implications for conservation, and management. Environ. Sci. Pollut. Res. 23, 11181–11192. doi: 10.1007/s11356-016-6340-y
Ndebele-Murisa, M. R. (2012). Biological monitoring and pollution assessment of the Mukuvisi River, Harare, Zimbabwe. Lakes Reservoirs Res. Manag. 17, 73–80. doi: 10.1111/j.1440-1770.2012.00497.x
Ochieng, H., Okot-Okumu, J., and Odong, R. (2019). Taxonomic challenges associated with identification guides of benthic macroinvertebrates for biomonitoring freshwater bodies in East Africa: a review. Afr. J. Aquat. Sci. 44, 113–126. doi: 10.2989/16085914.2019.1612319
Ogbeibu, A. E., Omoigberale, M. O., Ezenwa, I. M., and Oboh, I. P. (2013). Application of some biometric indices in the assessment of the water quality of the Benin River, Niger Delta, Nigeria. Trop. Freshwater Biol. 22, 49–64. doi: 10.4314/tfb.v22i1.5
Oigara, D. K., and Masese, F. O. (2017). Evaluation of the South African Scoring System (SASS 5) biotic index for assessing the ecological condition of the Mara River, Kenya. Afr. J. Educ. Sci. Technol. 4, 41–51.
Ollis, D. J., Dallas, H. F., Esler, K. J., and Boucher, C. (2006). Rapid bioassessment of the ecological integrity of river ecosystems using aquatic macroinvertebrates: review with a focus on South Africa. Afr. J. Aquat. Sci. 31, 205–227. doi: 10.2989/16085910609503892
Palmer, R. W. (1997). Change in the Abundance of Invertebrates in the Stones-in-Current Biotope in the Middle Orange River Over Five Years. WRC Report No. KV130/00. Water Research Commission, sPretoria, South Africa.
Palmer, R. W., and Taylor, E. D. (2004). The Namibian Scoring System (NASS) version 2 rapid bio-assessment method for rivers. Afr. J. Aquat. Sci. 29, 229–234. doi: 10.2989/16085910409503814
Phiri, C. (2000). An assessment of the health of two rivers within Harare, Zimbabwe, on the basis of macroinvertebrate community structure and selected physico-chemical variables. Afr. J. Aquat. Sci. 25, 134–145. doi: 10.2989/160859100780177677
Prat, N., Munné, A., Rieradevall, M., Solà, C., and Bonada, N. (2000). ECOSTRIMED: protocol to establish the ecological status of Mediterranean rivers and streams. Barcelona: Diputació de Barcelona, À rea de Medi Ambient. Available online at: http://www.diba.es/mediambient/ecostrimed.asp
Rosenberg, D. M., and Resh, V. H. (1993). Freshwater Biomonitoring and Benthic Macroinvertebrates. London: Chapman and Hall.
Smith, M. J., Kay, W. R., Edward, D. H. D., Papas, P. J., Richardson, K. S. J., Simpson, J. C., et al. (1999). AUSRIVAS: using macroinvertebrates to assess ecological condition of rivers in Western Australia. Freshwater Biol. 41, 269–282. doi: 10.1046/j.1365-2427.1999.00430.x
Stark, J. D. (1993). Performance of the macroinvertebrate community index: effects of sampling method, sample replication, water depth, current velocity, and substratum on index values. N. Z. J. Mar. Freshwater Res. 27, 463–478. doi: 10.1080/00288330.1993.9516588
Stark, J. D. (1998). SQMCI: a biotic index for freshwater macroinvertebrate coded-abundance data. N. Z. J. Mar. Freshwater Res. 32, 55–66. doi: 10.1080/00288330.1998.9516805
Stark, J. D., and Maxted, J. R. (2007). A biotic index for New Zealand's soft-bottomed streams. N. Z. J. Mar. Freshw. Res. 41, 43–61. doi: 10.1080/00288330709509895
Tumusiime, J., Tolo, C. U., Dusabe, M., and Albrecht, C. (2019). Reliability of the Tanzania river scoring system (TARISS) macroinvertebrate index of water quality: a case study of the river Mpanga system, Uganda. J. Freshwater Ecol. 34, 541–557. doi: 10.1080/02705060.2019.1631895
Keywords: biomonitoring, ecological condition, spatial variability, river health, temporal variability, water quality
Citation: Dallas HF (2021) Rapid Bioassessment Protocols Using Aquatic Macroinvertebrates in Africa–Considerations for Regional Adaptation of Existing Biotic Indices. Front. Water 3:628227. doi: 10.3389/frwa.2021.628227
Received: 11 November 2020; Accepted: 04 February 2021;
Published: 25 February 2021.
Edited by:
Gordon O'Brien, University of Mpumalanga, South AfricaReviewed by:
Pankaj K. Gupta, University of Waterloo, CanadaTarik Bahaj, Mohammed V University, Morocco
Copyright © 2021 Dallas. This is an open-access article distributed under the terms of the Creative Commons Attribution License (CC BY). The use, distribution or reproduction in other forums is permitted, provided the original author(s) and the copyright owner(s) are credited and that the original publication in this journal is cited, in accordance with accepted academic practice. No use, distribution or reproduction is permitted which does not comply with these terms.
*Correspondence: Helen Dallas, aGVsZW5AZnJjc2Eub3JnLnph