- 1Kenya Marine and Fisheries Research Institute, Kisumu, Kenya
- 2Kenya Marine and Fisheries Research Institute, Marigat, Kenya
- 3Kenya Marine and Fisheries Research Institute, Kisii, Kenya
- 4Kenya Marine and Fisheries Research Institute, Mombasa, Kenya
- 5Department of Fisheries and Aquatic Sciences, University of Eldoret, Eldoret, Kenya
The use of socioeconomic and cultural parameters in the assessment and biomonitoring of ecological health of aquatic ecosystems is still in its nascent stages. Yet, degradation of aquatic ecosystems has elicited concerns because of its bearing on social and economic development of communities consisting of marginalized and vulnerable groups, as well as the expenses and technical knowhow involved in biomonitoring approaches. In this study we developed a Citizen-based Index of Ecological Integrity (CIEI) for assessing and monitoring the ecological status of vulnerable African riverine ecosystems in Lake Victoria Basin, Kenya. The hypothesis is that the citizen-led socioeconomic and cultural metrics provides a more cost-effective broad view of ecosystems than other biomonitoring methods in the assessment of water resources in the developing countries. Selected rivers in the southern part of Lake Victoria (Rivers Kuja and Sondu-Mirui) recorded the highest CIEI than their northern counterparts (Rivers Yala and Nzoia) that had moderate to poor ecosystem integrities. The study demonstrates the usefulness of this approach to elucidate the source of impairment, the extent of impacts and provide a justifiable rationale to advice policy makers on developing guidelines for conservation and management of aquatic ecosystems. We recommend for adoption and promotion of the CIEI perspective in areas where such approaches appear defensible for the assessment of catchment-wide practices in areas with robust indigenous knowledge to provide a broad-view of the ecological health of the aquatic ecosystem.
Introduction
In working toward the protection of freshwater ecosystems, development of decision-support tools for monitoring changes in water quality and biological communities over time has been given a priority in many parts of the world (Statzner et al., 2001; Aura et al., 2020; Ko et al., 2020). Biological communities have especially become common indicators of change, based on the premise that the presence or absence of certain species or groups of species at a given site reflects its environmental quality (Barbour et al., 1999; Dallas et al., 2010). By monitoring how species respond to specific stressors in their environment and developing species-environmental relations along gradients of human influence, our understanding of how human disturbances can shape the structure and functioning of ecosystems has tremendously increased over the last 4 decades (Karr, 1981; Karr and Chu, 2000; Masese et al., 2009; Friberg, 2014). Continuous development and refinement of these approaches have yielded solid theoretical grounds upon which bioindication has flourished and operational biomonitoring programs have been developed (Dickens and Grahm, 2002; Kaaya et al., 2015).
Biomonitoring and bioassessment data and information is particularly important for aquatic systems management, because population growth, migration, and sociocultural activities are contributing to greater rates and extents of watershed development and impairment (Angel et al., 2011; Seto et al., 2011). These altered conditions negatively affect water quality, aquatic life, and functions of stream ecosystems (Smucker and Detenbeck, 2014) and have adverse socioeconomic consequences as well (Pickett et al., 2011). As a result, the proportion of impairment has become an important factor in urban planning and watershed management, because it is strongly associated with development intensity and stressors, and it can be readily quantified and regulated (Bellucci, 2007; Schueler et al., 2009).
Characterizing relationships between watershed conditions and water quality could help identify future priorities for monitoring and restoration (Faghihimani, 2012). However, the methods for data collection and study design could have consequences for interpreting results and for decision making (Carlsson and Berkes, 2005). Various methods of monitoring and assessment have been employed in characterization of aquatic relationships (Lozano et al., 2013). Monitoring actions traditionally focused on one aspect of ecological integrity that involved the determination of pollution from point sources which involves use of chemical and physical water quality, with regulatory efforts aimed at controlling individual parameters (Roux, 1997). With the failure of the chemical and physical water quality to provide information on the overall condition of the aquatic system, the use of biomonitoring approach emerged that was more integrated and holistic (Cairns, 2003). However, both physical-chemical and biological approaches require skills and knowledge as well as costs for their implementation (Masese et al., 2013), which is a major hindrance to continuous monitoring of vulnerable ecosystems in developing countries.
A number of factors determine the choice of a program for assessment and monitoring of ecosystems, such as research costs, human resources, and data needs (Wren et al., 2000). For African systems, biomonitoring of aquatic ecosystems lags behind other regions because of limited financial devotion, lack of technical capacity, and limited guides on biomonitoring. Despite these hindrances, a number of regional or country-specific indices and programs have been developed for biomonitoring (e.g., Dickens and Grahm, 2002; Aschalew and Moog, 2015; Kaaya et al., 2015). Most of these indices and programs, however, are based on biological communities and the physical and chemical parameters of the environment that can be measured using standard methods.
Despite their wide appeal and adoption, traditional ecological data collection methods, and biomonitoring programs are limited in the amount of data that can be gathered across large spatial and temporal scales (Pocock et al., 2017; Achieng' et al., 2020). As a result of these limitations, new approaches to environmental monitoring have been explored, and citizen-science (Conrad and Hilchey, 2011; Theobald et al., 2015; Chandler et al., 2017; Pocock et al., 2017) has emerged as one of the methods, which involve volunteer participation by community members in providing or collecting information following a protocol provided, designed and or validated by experts in the field. This is aligned to co-management approaches that give stakeholders a platform for sustainable management of aquatic resources (Obiero et al., 2015). The approach has among others factors such as cost led to the push toward the inclusion of citizens in stewardship and monitoring of the status of natural resources (Brooks et al., 2005; Mochizuki and Yarime, 2016). While African countries have made a lot progress in this regard, especially on the involvement of communities in top-down decision making, and monitoring and management of natural resources, such as fisheries (Imende et al., 2005; Etiegni et al., 2017), forestry (Crocker et al., 2020), water resources (Bannatyne et al., 2017) and effects of climate change (Tesfahunegn and Gebru, 2020), the development of citizen science as an environmental assessment and monitoring approach is quite limited, and in most cases, it is at its nascent state (Requier et al., 2020).
Managers and non-governmental organizations are increasing their use of citizen volunteers to enhance their ability to monitor and manage natural resources, through tracking of species at risk and conserving protected areas (Conrad and Hilchey, 2011; McKinley et al., 2017; Crocker et al., 2020). However, in many places a comprehensive approach has not been developed on the use of socio-cultural knowledge and experiences in the assessment of the status of aquatic ecosystems (Reid et al., 2010). Social value systems are transient and transitory, as is the environment in which they operate, and are by no means laws of nature (Stephenson et al., 2020). Several fundamental social and cultural values are associated with basic needs, determined by the biology of biota, thus being less subject to modification (Hjalte et al., 1977). Well-noted examples are human interactions with aquatic ecosystems that stimulate such physical surroundings (Tol, 1995). Thus, the indigenous knowledge of individuals who have long interaction with aquatic ecosystems can be utilized in understanding of ecosystem integrity.
In most African countries, biomonitoring, and bioassessment of aquatic ecosystem is often confounded by a lack of, or inadequate and incomplete, data and monitoring initiatives by professional scientists and government agencies (Masese et al., 2013; Mangadze et al., 2019). To fill the void, non-professionals and citizen organizations have emerged the world over to track trends and work toward effective and meaningful planning, management, and stewardship. This is because, data collection requires local inputs that are accompanied by the equitable participation of data users, including local communities which can lead to better monitored results and sustainability (Stephenson et al., 2020). One such approach is the application of sociocultural perspectives that uses indigenous knowledge and parameters in the creation of indices. More so, by integrating a large number of stakeholders, citizen science has the potential to directly connect scientists to the public and shares the importance of their work (Crocker et al., 2020). This could crack the challenge of communicating the value of scientific research to the public that is increasingly important yet in present world researchers typically have little support for outreach and education activities.
In this study we explored the use of sociocultural and economic perspectives, knowledge, and experiences to determine the pollution status of major rivers in the Lake Victoria Basin, Kenya. We used indigenous knowledge as one of the items in the toolbox of citizen science to develop a multimeric approach to bioassessment based on people's experiences and perspectives forged from living with and interacting with rivers in their localities. The approach has a potential for application in the assessment and monitoring the condition of vulnerable riverine ecosystems in Africa.
Materials and Methods
Study Area
This study was done in the lower reaches of major rivers draining the Kenyan part of the Lake Victoria Basin (LVB), specifically Rivers Nzoia, Yala, Sondu-Miriu, and Kuja (Figure 1). These rivers constitute over 45% of the total discharge to the lake (Twesigye et al., 2011). The rivers represent the major river catchments with a gradient of disturbance, and most notable biodiversity hotspots around Lake Victoria (Masese et al., 2020). These rivers support an artisanal fishery, particularly during the rainy seasons (Balirwa et al., 2003) and act as a source of water for livestock, irrigation, industries, and domestic uses. They are threatened by catchment activities such as conversion of wetlands into farms, urban developments, poor management of domestic, and industrial wastes and the leaching of agrochemical residues. These activities cause decrease in forest cover, increases in soil erosion and rivers pollution (Balirwa et al., 2003).
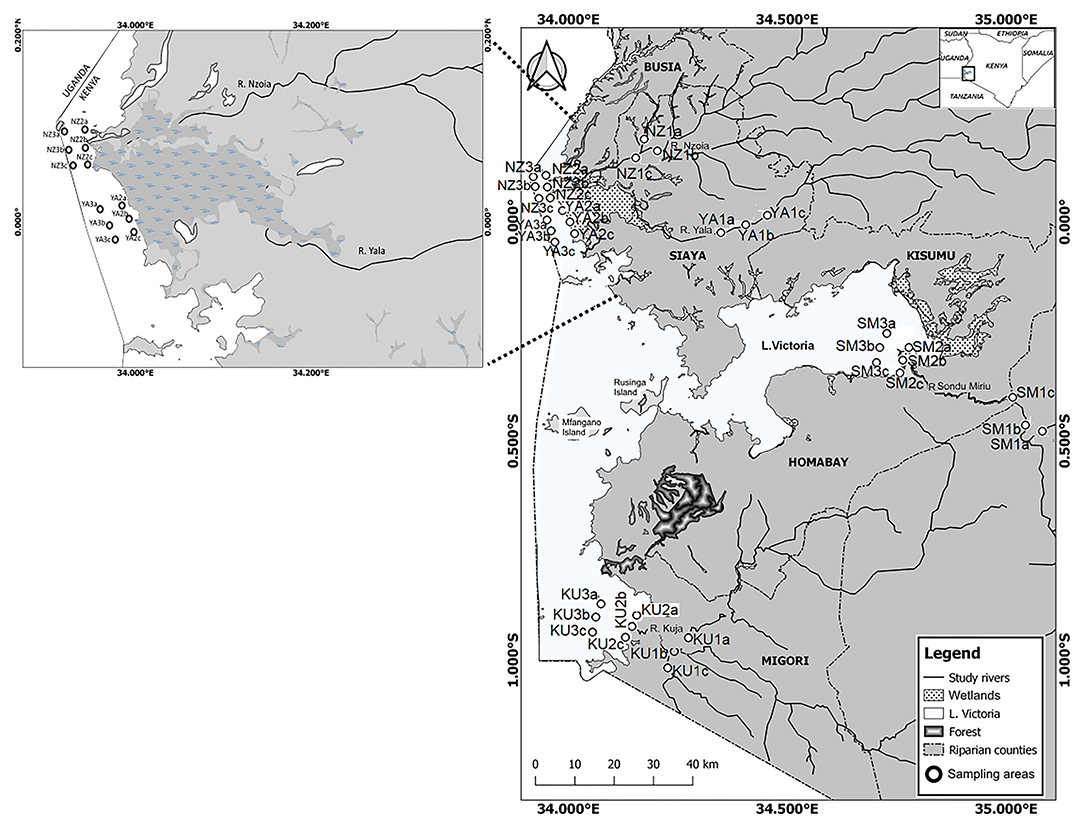
Figure 1. Location of the study sites within the stations of (a) River Kuja (KU), (b) River Sondu-Miriu (SM), (c) River Yala (YA), and (d) River Nzoia (NZ). Sampling sites with at least three replicates as representative of microhabitats included River Kuja: KU1a, b, c—River Kuja upstream channel; KU2a, b, c—Kuja river mouth before discharge; KU3a, b, c—Kuja river mouth after discharge. Sondu–Miriu: SM1a, b, c—River Sondu-Miriu upstream; SM2a, b, c—Sondu-Miriu river mouth before discharge; SM3a, b, c—Sondu-Miriu River mouth after discharge. River Yala: YA1a, b, c—River Yala upstream; YA2a, b, c—Yala river mouth before discharge, YA3a, b, c—Yala river mouth after discharge. River Nzoia: NZ1a, b, c—River Nzoia upstream, NZ2a, b, c—Nzoia river mouth before discharge, NZ3a, b, c—Nzoia river mouth after discharge. The labels (a–d) represents sampled replicates of each site.
Rivers Nzoia and Yala constitute the northern section of this study and Rivers Kuja and Sondu-Mirui are the southern representatives. The Lake Victoria Basin delivers important ecosystem services to more than 40 million people in the three riparian countries. These include fisheries, transport, and water for domestic, agricultural and industrial uses (Aura et al., 2013). The Kenyan part of the lake includes the Winam Gulf (Kavirondo Gulf or Nyanza Gulf), which is joined to the main lake by the Rusinga channel with major river discharges that have been believed to influence pollution status of the lake (Kundu et al., 2017; Aura et al., 2019). Nutrient enrichment in the Winam Gulf of Lake Victoria (Gikuma-Njuru and Hecky, 2005; Guya, 2013) has been attributed to increased extrinsic nutrient loadings associated with changes in land-use activities within its catchment (Verschuren et al., 2002). Due to the combined effects of human population growth, land use and land cover changes in the catchments of major rivers, elevated sedimentation have been reported to occur in the rivers over the years (Masese and McClain, 2012).
Study Design
The participation of riparian communities situated within 5 km of the river were selected on the basis of their involvement in the riverine changes and land use that foster the utilization and dependency on riverine products and their alternatives in this study. This underscores the application of citizen science into perspective. The riverine community benefits from a river site in their neighborhood in terms of anthropogenic activities such as fishing, water abstraction, waste disposal, and other socioeconomic and cultural activities that can be quantified into a measurement of a parameter or value, herein referred to as a metric (Aura et al., 2017; Masese et al., 2020). As a result of these practices and changes, this study aims to determine the role of the riparian communities in assessing the status of riverine systems.
Criteria for Sample Size Determination
The target population in the lower reaches of the selected major river catchments was identified from riparian communities. This consisted of local residents living within 5 km from the boundary of the river and the leaders of the community riverine associations. The northern riparian catchment had a total population of 102,321 people while the southern part had a total population of 110,321 people (National Population Census, 2019). The sample size was arrived at using the equation below by Cochran (1963):
Where:
n = the desired sample size (if the population is >10,000)
z = the standard normal deviate at the required confidence level
p = the proportion in the target population estimated to have had characteristics being measured (0.15)
q = 1-p
d = the level of statistical significance set at (0.05)
By using the above equation 400 people were sampled with each river having 100 participants from the entire population to be used for the study. In this case, the sample size method was chosen in order to ensure evenness in the distribution of the targeted sample population.
Criteria for Participants Selection in Interviews
The data were collected through interviews with community members, literature review, and field observations using transect walks to ensure evenness and appropriate representation of the target population. Qualitative data were collected using interviews that were administered to participants. Sampling (riparian community) was done within 5 km because it has been shown that natural systems' use reduces beyond this distance (Ewel, 1999). In the study, one among the elderly members in each household was randomly selected and interviewed for representation.
Data were collected during the wet season (March) and dry season (July) of 2018 and 2019. The participants were interviewed with the help of a local assistant who acted as an interpreter in cases where the participant could not respond in English. Before the interviewers and interpreter were trained on the contents of the questions, and this made it easier for him/her to understand the concept and the research. Closed and open-ended questions were used to extract relevant information from participants and also understand their views. The interview questions consisted of both one response and multi-response questions.
The questions were divided into four sections representing socioeconomic and cultural perspectives classified into structure, scale, pattern, and network (Zhou et al., 2019) in relation to the local situations. The participant's perspectives sought under aforesaid sections on structure and scale and pattern and network metrics are presented in Table 1 under the socioeconomic and cultural metrics.
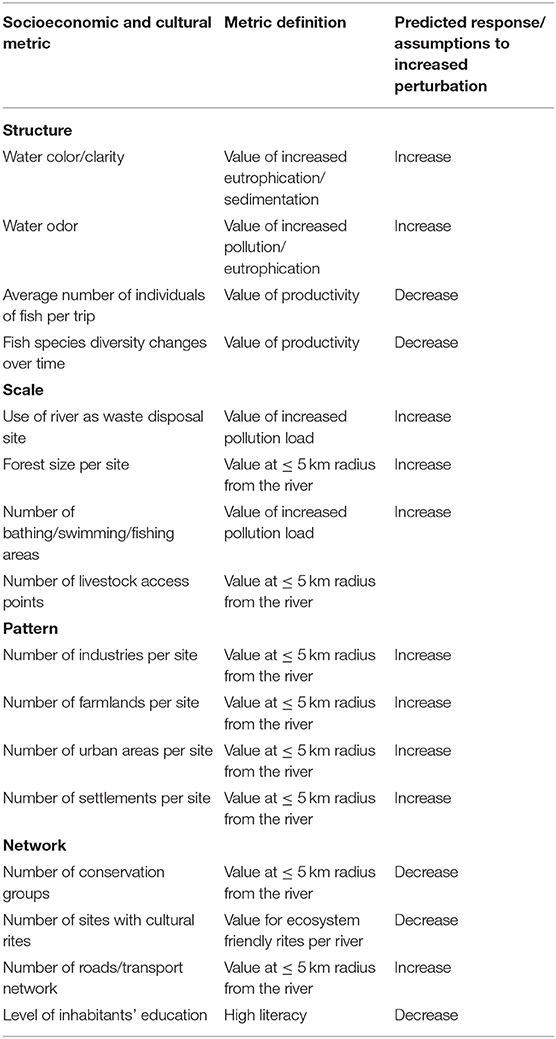
Table 1. Socioeconomic and cultural metrics and definitions that were evaluated for the development of the Citizen Index of Ecological Integrity (CIEI) for major rivers in the lower reaches of Lake Victoria Basin, Kenya and their predictive responses to increased levels of perturbation.
Before conducting the interviews, Key informant interviews were conducted among community leaders in the riverine regions. A total of 16 Key Informant Interviews (KIIs) were conducted, with each major riverine site having 4 KIIs who were mainly organizational heads and leaders. The responses of ≥ 50% choice of a reference site helped in the determination of a control site as well as the appropriate method of collecting community perceptions in each of the studied rivers during the scoring process. Furthermore, before interviews, consent of the participants was sought. If they chose not to participate, the next household member was picked. The interview questions was pre-tested on 40 participants (10 from each of the riverine systems) who were not part of the 400 interviewees. Following the pre-testing, a few changes were made to the structure and wording of the questions for clarity.
The participants were purposively chosen with special regard to proximity of residence from the river-line, involvement in socioeconomic and cultural activities related to the river-system and the duration of stay in proximity to the riverine system (Zhang et al., 2018). Those who stayed in close proximity to the river, depended on the river economically and socially, and had at least 20 years of continuous residence were chosen for interviews. This was based on the assumption that they could answer more accurately on the observed changes that the river systems have undergone over time. Snow-ball sampling technique was used when more information was required (Aura et al., 2018).
Field Observations
To assess and identify water quality status for supplementation and validation with the Citizen Index of Ecological Integrity (CIEI) developed, physico-chemical observations were conducted. In this case, the field parameter measurements would show the scientific and verifiable output of the ecosystem which would be compared with the CIEI in assessing the degree of appropriateness. Each sampling expedition took an average of 10 days in July and March for each year from 2016 to 2018 with specific experts in water quality. This was done to add to the information collected through questionnaires. Microhabitats including riffle, pool, run, and open water were sampled in triplicate (Aura et al., 2010). General environmental observations about each site such as the maximum depth, time of sampling, site characteristics, and Global Positioning System (GPS) location were noted before sampling for physico-chemical characteristics as they could have an influence on physico-chemical comprehensions.
The selected physico-chemical parameters that were measured using standard methods for in situ data collection and sampling included temperature (°C), dissolved oxygen (DO, mg L−1), pH, and conductivity (μS cm−1). These physico-chemical parameters were measured using portable electronic water quality meters. Water transparency was measured with a standard Secchi disk (APHA, 2005). The water samples were further collected directly from the sampling sites using pre-treated 1 L polyethylene sample bottles for nutrient analyses. The bottles were individually labeled, filled, preserved using sulphuric acid and stored in cool boxes, for laboratory analysis using photometric methods for total nitrogen (TN, μg L−1) and total Phosphorus (TP, μg L−1) (APHA, 2005).
Citizen Index of Ecological Integrity (CIEI)
Figure 2 shows the flow chart used in the attainment of the CIEI. The index is developed using socioeconomic and cultural attributes and validated using physic-chemical parameters and a previous index developed for the region. This is because hydromorphological changes, socioeconomic and cultural shifts within the major river catchments of Lake Victoria have modified the ecology of most of these rivers from a desirable to less desirable condition (Masese and McClain, 2012; Guya, 2019). Thus, the community perceptions on the changes in socioeconomic and cultural perspectives that were classified into structure, scale, pattern, and network were used to develop a citizen-based index for assessing the pollution status of major rivers draining into Lake Victoria, Kenya (Figure 3).
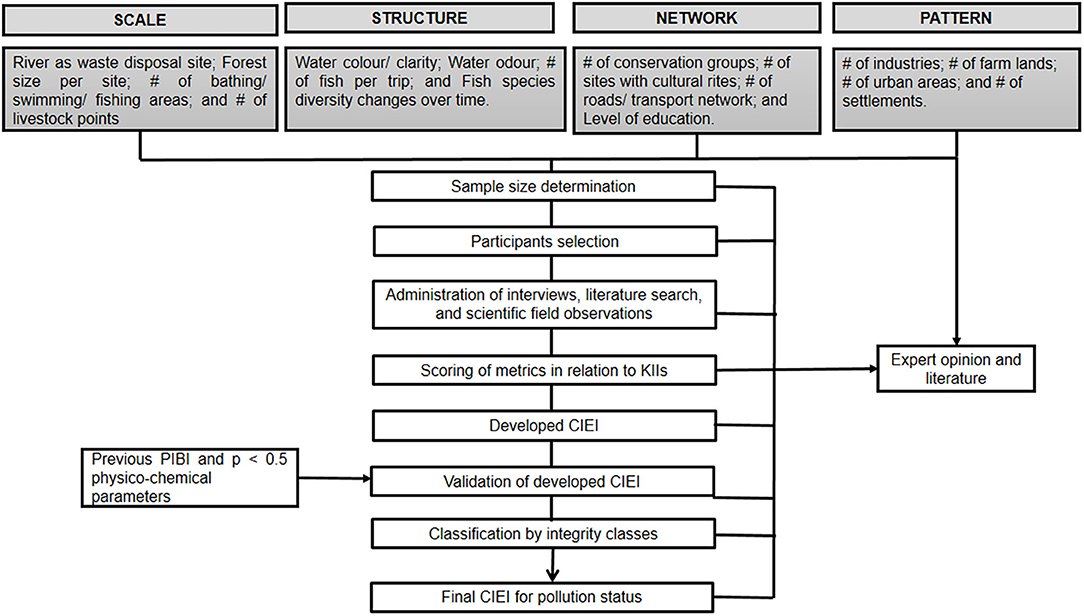
Figure 2. Flow chart showing the steps involved in the development of the Citizen Index of Ecological Integrity (CIEI) novelty for assessing the pollution status of major river catchments in Lake Victoria, Kenya.
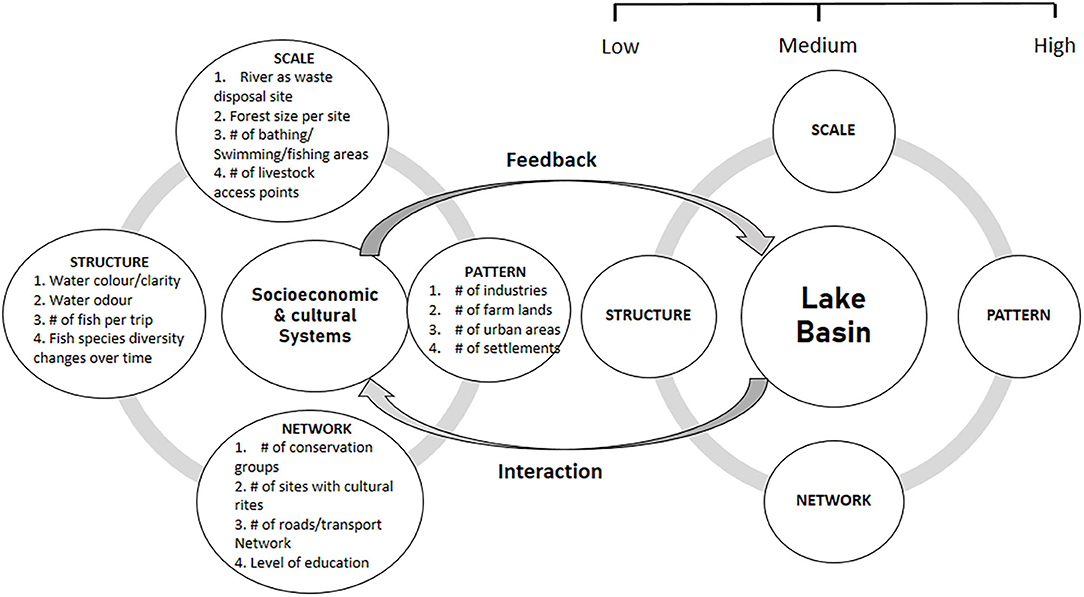
Figure 3. Schematic representation of the socioeconomic and cultural metrics for the development of the Citizen Index of Ecological Integrity (CIEI) novelty for assessing the pollution status of major river catchments in Lake Victoria, Kenya.
The feedback loop of the metrics (Table 1) in comparison with increased levels of perturbations was assigned community perception scores of either low (assigned a score of 1), medium (assigned a score of 3) or high (assigned a score of 5) as illustrated in Table 2. During the KIIs, 98% of them disagreed with the use of a continuous Likert-scale of 1–10 as it was deemed to be long and difficult to differentiate one score to another due to the closeness of the numbers (data not provided). Thus, although the criterion of 5, 3, and 1 has been heavily criticized by various authors such as Stoddard et al. (2008), it was deemed relevant for this study (Table 2). The 5, 3, and 1 system has also commonly been used for metrics biomonitoring and assessment (e.g., Aura et al., 2010, 2017), and its similar use could give better comparisons with other indices. For each socioeconomic and cultural metric that was expected to decrease with increased pollution levels in relation to the control site, the community awarded a score of 1, because the metrics showed the greatest deviation from the reference KIIsparticipants. Those sites were deemed to be close to the control site by the community were scored as 3, and values above the control site were scored as 5. The scoring by the community was reversed for socioeconomic and cultural metrics expected to increase with pollution levels. The scores for each metric were summed to arrive at the final CIEI value for each sampling site.
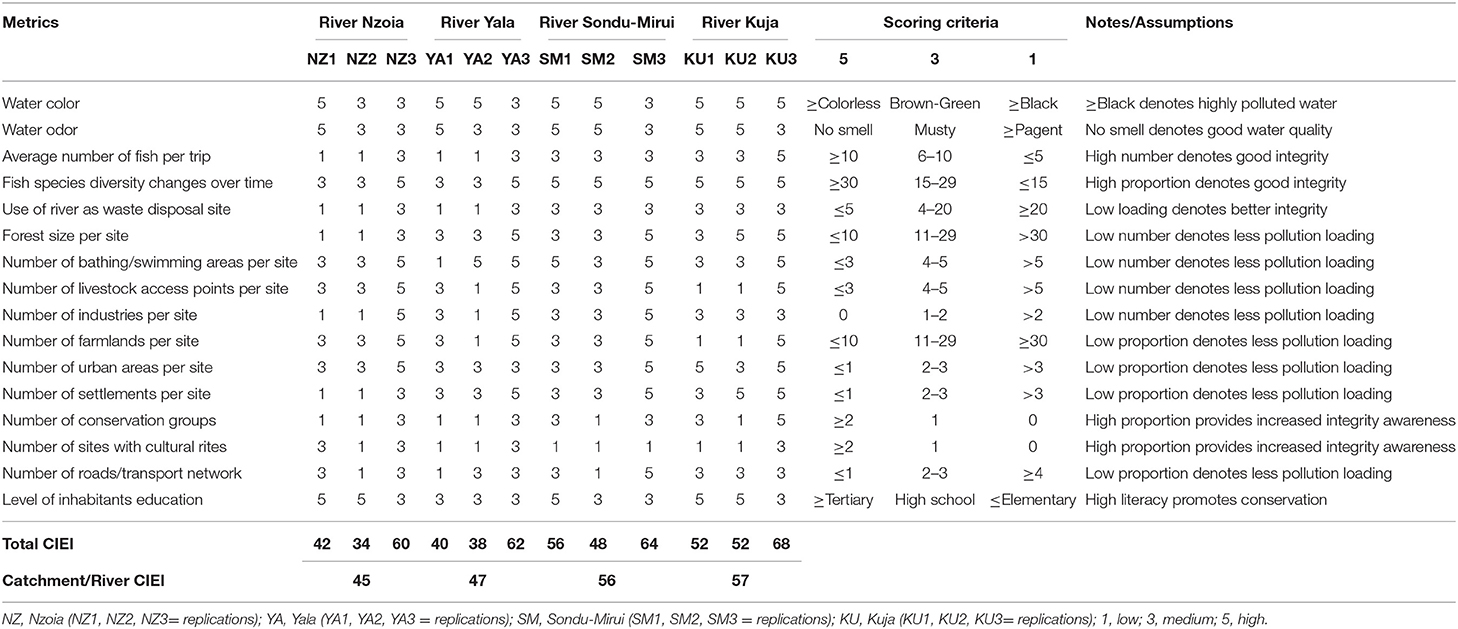
Table 2. Developed Citizen Index of Ecological Integrity (CIEI) for ranking of major river catchments in relation to community perceptions on the pollution status in the lower reaches of Lake Victoria, Kenya.
Based on the CIEI final scores, the study used integrity classes of good, fair, and poor as quantitative levels to come out with a scenario that could easily be interpreted by the policy makers (Aura et al., 2010) that were based on site visits and field records. The highest and lowest threshold ranges of > 45 and <57 points were used to avoid a large deviation from all the CIEI final values.
Data Analysis
The interviews were examined to ensure that they were completely and consistently filled. The interview questions were then coded and summary tables and figures produced for the various responses. In order to ascertain the degree of accuracy of the CIEI in determining the pollution status of the sampled ecosystem, previously developed Phytoplankton Index of Biotic Integrity (PIBI) values in similar study sites were used for the validation of the novelty approach (Aura et al., 2020). The similarity of the study sites would help establish the degree of performance and robustness of the CIEI as a citizen-led tool in relation to the previous PIBI developed as a scientific-led output. The physico-chemical data were not normally distributed and attempts to normalize the data by transformations were unsuccessful. The physico-chemical data was compared spatially and temporarily using the non-parametric Kruskal-Wallis one-way ANOVA to examine the uncertainty of values and variations through pair-wise comparisons. The CIEI was correlated with significant (p < 0.05) physico-chemical parameters to further validate and strengthen the results of the final index. The study employed the use of SPSS version 21 (SPSS Inc., Chicago, IL, USA) and R version 3.5.0 (R Core Team, 2014) for statistical analyses. Significant differences for all analyses were determined at p < 0.05.
Results
The participants in the study fell within the age group of between 55 and 70 years olds depicting participants' good experience. Though unquantified in the study, majority of the participants hesitated in answering questions on the scale metrics. The easiness to answer interview questions were in the order of structure, pattern, network, and scale from the least hesitant to the most hesitant metrics.
Table 2 shows the calculated final CIEI scores of the lower reaches of Lake Victoria, Kenya. In the final CIEI, River Kuja emerged with the highest average CIEI (57 points), while River Nzoia recorded the lowest CIEI (45 points) (Table 3) portraying good and poor ecosystem integrity accordingly. Thus, CIEI was in the order of Rivers Kuja, Sondu-Mirui, Yala, and Nzoia, from the highest to the lowest, respectively.
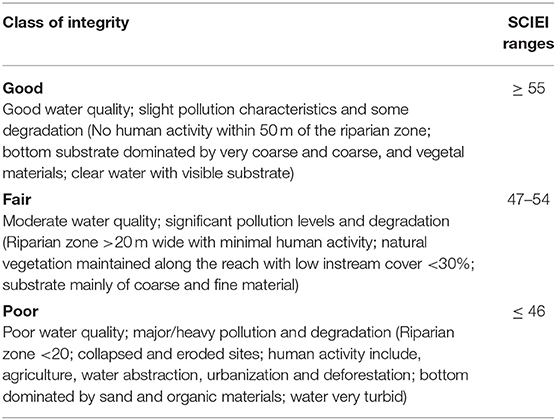
Table 3. Suggested threshold values of ecosystem integrity classes for final Citizen Index of Ecological Integrity (CIEI) development showing the classification level and ranges for ranking pollution levels in the lower reaches of Lake Victoria, Kenya during the study period (Adopted and modified from Aura et al., 2010).
The validation and strengthening of the final CIEI scores showed significant but weak relationship (R2 = 0.37; p = 0.02) with the PIBI scores (Figure 4). Notably, the CIEI depicted a similar trend with the previous index from North of Lake Victoria to the South. The only difference was the highest scores recorded in CIEI due to use of high number of metrics.
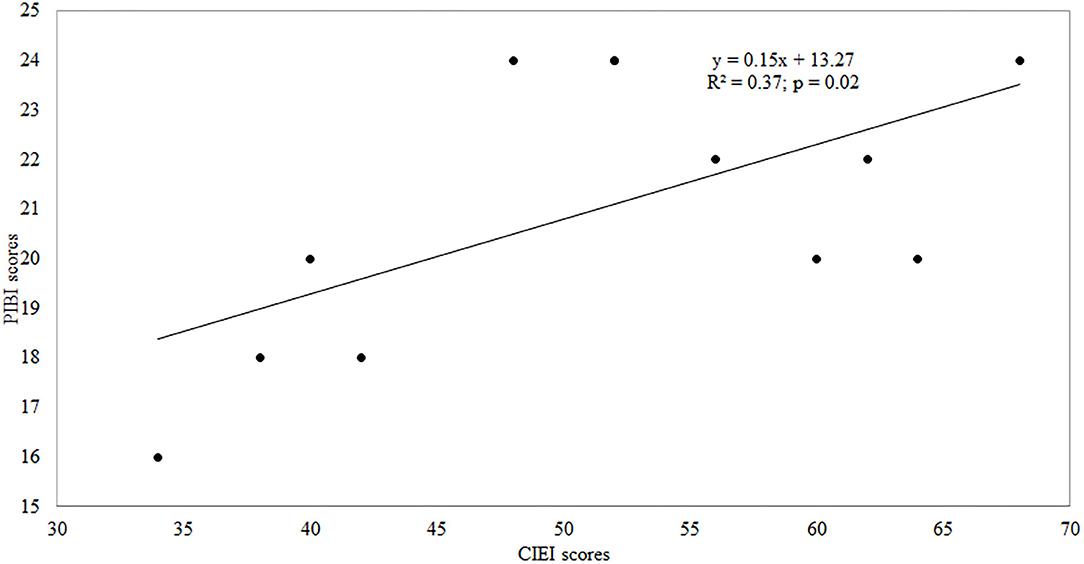
Figure 4. A correlation plot showing the validation of the Citizen Index of Ecological Integrity (CIEI) novelty performance using previously developed Phytoplankton Index of Biotic Integrity (PIBI).
In the field observations, only conductivity, TP and TN levels varied across the sites (Kruskal-Wallis ANOVA; p < 0.05) with no temporal variations (Table 4). Further validation of the CIEI in relation to physico-chemical parameters with spatial significant variations showed a negative relationship (p > 0.05; R < 0.5) between the CIEI scores and the physic-chemical parameters.
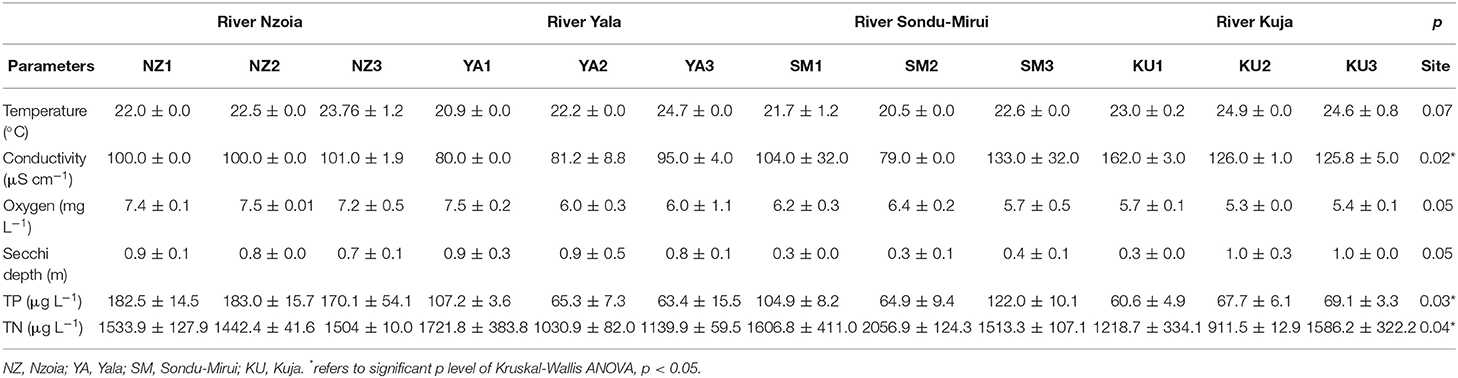
Table 4. Spatio-temporal mean (± SD) values of selected physico-chemical variables in Rivers Nzoia, Yala, Sondu-Mirui, and Kuja, Kenya.
Discussion
Just like other scientific indices, the evaluation in the use of citizen-led tool for bioindication has been found to have several assumptions and drawbacks. Citizens or communities who have lived side by side with the natural environment for millennia are often believed to have a comprehensive understanding of the ecosystem integrity, including function and structure (Zhang et al., 2018). However, this is not always the case. This is because different inhabitants could have different interests and socioeconomic valuations. Furthermore, knowledge on the integrity, structure and functioning of natural ecosystems such as rivers that is passed down over generations can be harvested in a distorted manner and end up to have no benefit on management and conservation. Notably, the classification of merits and attributes for citizen-based indices are expert-dependent (Masese et al., 2013). The expatriate point of view could therefore hinder objectivity and uniformity of the index output and thereby misinterpret conservation priority areas. This is especially the case in Africa where standard methods and tools for bioindication have not been fully developed for use by resource managers and conservationists. Additionally, other assumptions and premises are study dependent due to variability in geographical characteristics (Mangadze et al., 2019).
For example, the current study was based on the premise that pollution of major riverine catchments due to anthropogenic activities can cause longitudinal changes in water quality and habitat conditions that influence a lake basin. Community perceptions were noted to have indiscriminately determined the studied riverine catchments integrity variations as reflected in the spatial variability of metrics used as indicators of degradation. Metric variability and response of metrics to impaired sites indicated CIEI to have corresponded to the changes in the riverine ecosystem based on the physicochemical parameters reported in the study. However, the metrics used were not indiscriminately measured for redundancy and for elimination since each parameter was given a chance to contribute to the final index to ascertain the value and relevance of every participant (Zhou et al., 2017). This meant that each participant input was given a chance to contribute to the final CIEI to ensure full representation and to minimize bias. However, hesitation to answer interview questions on the scale metrics and as per the order of hesitation could be attributed to the riparian communities' activities that were directly linked to their relationships with contamination. Such behavioral responses from participants could be examined and quantified further in future citizen science studies that are related to pollution and environmental degradation.
However, understanding how socioeconomic and cultural perceptions of aquatic systems vary spatially has important implications for researchers, policy makers, environmental organizations aiming to reduce, or minimize pollution of such systems (Zhou et al., 2019). The findings from this study highlight the need for not only future socioeconomic and cultural studies on aquatic systems indices and models, but for outreach and educational efforts to occur in marginalized neighborhoods to increase the trust in the use of such systems and their safety. The improved trust will reduce the economic burden of conserving them and will also address the environmental sustainability issues surrounding pollution in aquatic systems.
The reported participants' age class of 55–70 years signified their longer residence time at the study areas, indicative of significant and valuable responses (Bachetti et al., 2008). This is because the CIEI was purely based on responses participantobtained during the interviews using semi-structured questionnaires and participant observation. This makes CIEI a major bottom-up tool that can be extensively employed to represent and explain ecosystem priority issues of the riparian community such as land use systems, pollution and water resource management.
Along the major river catchments studied herein, over-use of the riparian areas, sewage discharges and agriculture affected the quality of the riparian zones, banks and substrate quality; these were reflected in the developed CIEI (Tables 2, 3) and water quality at the sampling stations (Table 4). The order of index scoring of rivers from Kuja, Sondu-Mirui, Yala, and Nzoia was from the highest to the lowest, respectively. Observed inclination illustrated the gradient of influence on the pollution status of the lake basin from north to south. Thus, selected northern rivers are highly threatened by catchment activities. The main ones being conversion of wetlands into farms such as the Dominion Farm around River Yala, urban developments, poor management of domestic, and industrial wastes from industries such as Pan Paper Industry along River Nzoia, and leaching of agrochemical residues causing river pollution, decreased forest cover, and increased soil erosion. Conversely, selected southern rivers Sondu-Mirui and Kuja are influenced mainly by agricultural farms, domestic wastes, and urbanization (Balirwa et al., 2003). The variation in the ecosystem integrity could therefore be due to the varying degree of multiple sources of pollutants from agricultural fields, industries, and domestic sources in studied ecosystems (Kundu et al., 2017). Similarly, several authors have recorded differences in space for biota, nutrient variations, pollution levels, water quality, and indices (Lung'ayia et al., 2001; Gikuma-Njuru and Hecky, 2005; Aura et al., 2010; Haande et al., 2011; Masese et al., 2013).
The validity of the CIEI developed was also reinforced by the insignificant weak relationship with conductivity and nutrient concentrations (Figure 5), suggesting that the variation exhibited some level of congruent with habitat and water quality of the studied rivers. In this regard, the CIEI was to some extent, able to identify sources of impairment and to assess the level of degradation arising from human activities. This could be because no site in the studied rivers scored the maximum values for all metrics, it can be adduced that even areas considered to be relatively unimpaired were already experiencing the effects of degradation (Aura et al., 2010).
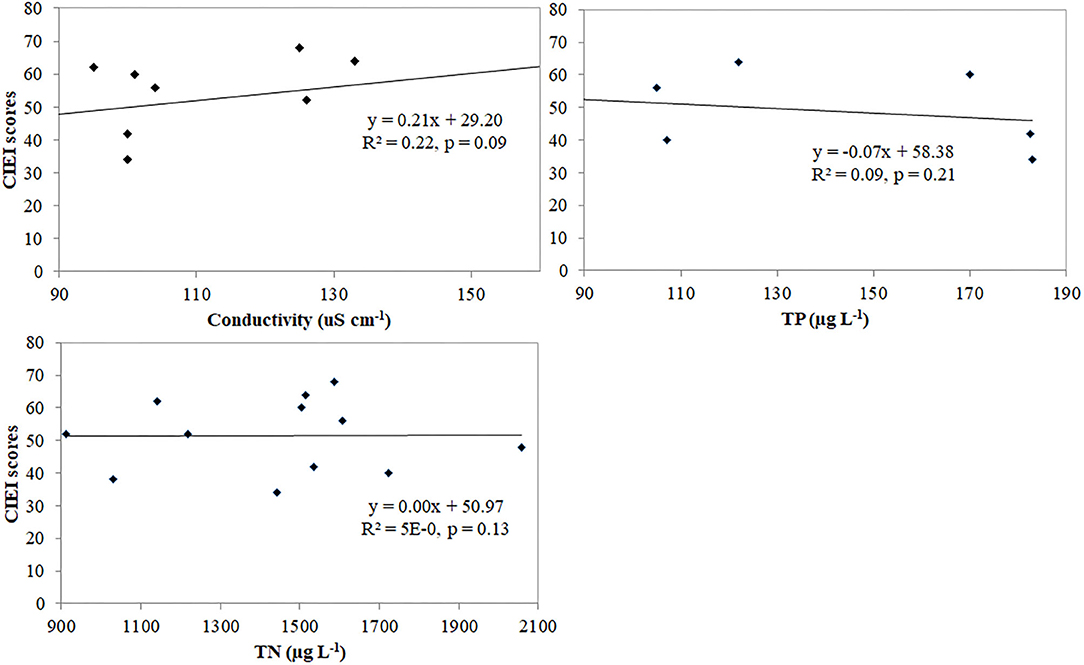
Figure 5. Correlation plots showing further validation and strengthening of the Citizen Index of Ecological Integrity (CIEI) novelty performance for assessing the pollution status of major river catchments in Lake Victoria, Kenya.
For the management of the Lake Victoria Basin, the metric scores and integrity classes are indicative of a changing environment under the influence of human activities. With increasing human population in the major riverine catchments, the situation is likely to be exacerbated. The challenge is to mitigate the current trend and to improve the CIEI scores at the sites and stations by improving both water and habitat quality. This can be achieved by riparian zone restorations, which have been found to be useful in improving riverine integrity (Kasangaki et al., 2008). Therefore, there is need to adopt this index for monitoring the Afrotropical riverine systems, because it is reliable not only for identifying sources of impairment, but also for assessing restoration successes. The CIEI developed herein in the current form or if further strengthened and reviewed, could offer a potential candidate decision support tool for the management of the lake basin at spatial and temporal level.
Conclusions
This study explores the preliminary use of socioeconomic and cultural parameters or index in the assessment of natural aquatic ecosystem integrity. The CIEI developed showed a robust approach to ascertain the various levels of ecosystem integrity using community metrics. In this case, southern ecosystem of Lake Victoria was ranked well than the northern counterpart in terms of pollution status of the lake basin. Thus, CIEI novelty herein could be used to show areas where conservation may be prioritized, with a broader applicability for snapshot studies in marginalized areas and with minimal expenses. There are some deficiencies in the current study that need to be improved in future research. Because the research area is a quite complex system, data with high spatio-temporal accuracy is required for better simulation. More adjustments for the CIEI may be required in the future to compare the upper sections of the catchments with the lower reaches as well as revision and up-scaling of socioeconomic and cultural metrics used.
Data Availability Statement
The raw data supporting the conclusions of this article will be made available by the authors, without undue reservation.
Ethics Statement
Ethical review and approval was not required for the study on human participants in accordance with the local legislation and institutional requirements. Written informed consent for participation was not required for this study in accordance with the national legislation and the institutional requirements.
Author Contributions
CA drafted the manuscript with support from CN, HO, CO, SM, JN, KN, and FM. All authors contributed to the final version of the manuscript.
Funding
This project was funded under National Research Fund (NRF), Kenya.
Conflict of Interest
The authors declare that the research was conducted in the absence of any commercial or financial relationships that could be construed as a potential conflict of interest.
Acknowledgments
Kenya Marine and Fisheries Research Institute (KMFRI) provided logistics and facilitated the research activities. Sincere thanks to all who gave their time to participate in the study, to our KMFRI technicians and interns.
References
Achieng', A. O., Masese, F. O., and Kaunda-Arara, B. (2020). Fish assemblages and size-spectra variation among rivers of Lake Victoria Basin, Kenya. in revision. Ecol. Indicators. 118:106745. doi: 10.1016/j.ecolind.2020.106745
Angel, S., Parent, J., Civco, D. L., Blei, A., and Potere, D. (2011). The dimensions of global urban expansion: estimates and projections for all countries, 2000-2050. Prog. Plan. 75, 53–107. doi: 10.1016/j.progress.2011.04.001
APHA (2005). American Public Health Association Standard Methods for the Examination of Water and Wastewater, 21st Edn. Washington, DC: APHA-AWWA-WEF.
Aschalew, L., and Moog, O. (2015). Benthic macroinvertebrates based new biotic score “ETHbios” for assessing ecological conditions of highland streams and rivers in Ethiopia. Limnologica 52, 11–19. doi: 10.1016/j.limno.2015.02.002
Aura, M. C., Kimani, E. N., Musa, S., Kundu, R., and Njiru, J. M. (2017). Spatio-temporal macroinvertebrate multi-index of biotic integrity (MMiBI) for a coastal river basin: a case study of River Tana, Kenya. Ecohydrol. Hydrobiol. 17, 113–124. doi: 10.1016/j.ecohyd.2016.10.001
Aura, M. C., Musa, S., Njiru, J., Ogello, E. O., and Kundu, R. (2013). “Fish-restocking of lakes in Kenya: should solemnly be an environmental issue,” in African Political, Social and Economic Issues: Kenya Political, Social and Environmental Issues, eds. W. A. Adoyo, and C. I. Wangai (NewYork, NY: NOVA Science Publishers, Inc), 39–60.
Aura, M. C., Musa, S., Yongo, E., Okechi, J., Njiru, J. M., Ogari, Z., et al. (2018). Integration of mapping and socio-economic status of cage culture: towards balancing lake-use and culture fisheries in Lake Victoria, Kenya. Aquac. Res. 49, 532–545. doi: 10.1111/are.13484
Aura, M. C., Nyamweya, C., Njiru, J. M., Musa, S., Ogari, Z., and Wakwabi, E. (2019). Exploring the demarcation requirements of fish breeding sites to balance the management and conservation needs of the lake ecosystem. Fish. Manag. Ecol. 26, 451–459. doi: 10.1111/fme.12311
Aura, M. C., Odoli, C., Nyamweya, C., Njiru, J. M., Musa, S., Miruka, J. B., et al. (2020). Application of phytoplankton community structure in the ranking of major riverine catchments that influence pollution status of a lake basin. Lakes Reserv. Sci. Policy Manag. Sustain. Use 25, 3–17. doi: 10.1111/lre.12307
Aura, M. C., Raburu, P. O., and Herrmann, J. (2010). A Preliminary macroinvertebrate index of biotic integrity for bioassessment of the Kipkaren and Sosiani Rivers, Nzoia River Basin, Kenya. Lakes Reserv. Res. Manag. 15, 119–128. doi: 10.1111/j.1440-1770.2010.00432.x
Bachetti, P., MuCulloch, C. E., and Segal, M. R. (2008). Simple, defensible sample sizes based on cost efficiency. Biometrics 64, 577–585. doi: 10.1111/j.1541-0420.2008.01004_1.x
Balirwa, J. S., Chapman, C. A., Chapman, C. L., Cowx, I. A., Geheb, K., Kaufman, L., et al. (2003). Biodiversity and fishery sustainability in the Lake Victoria basin: an unexpected marriage? Bioscience 53, 703–716. doi: 10.1641/0006-3568(2003)053[0703:BAFSIT]2.0.CO;2
Bannatyne, L. J., Rowntree, K. M., van der Waal, B. W., and Nyamela, N. (2017). Design and implementation of a citizen technician–based suspended sediment monitoring network: lessons from the Tsitsa River catchment, South Africa. Water 43, 365–377. doi: 10.4314/wsa.v43i3.01
Barbour, C. D. M. T., Gerritsen, J., Snyder, B. D., and Stribling, J. B. (1999). Rapid Bioassessment Protocols for Use in Streams and Wadeable Rivers: Periphyton, Benthic Macroinvertebrates and Fish, 2nd Edn. Washington, DC: U.S. Environmental Protection Agency; Office of Water.
Bellucci, C. J. (2007). Stormwater and aquatic life: making the connection between impervious cover and aquatic life impairments for TMDL development in Connecticut streams. Proc. TMDL 5, 1003–1018. doi: 10.2175/193864707786619819
Brooks, N., Adger, W. N., and Kelly, P. M. (2005). The determinants of vulnerability and adaptive capacity at the national level and the implications for adaptation. Glob. Environ. Change 15, 151–163. doi: 10.1016/j.gloenvcha.2004.12.006
Cairns, J., Jr. (2003). “Biotic community response to stress,” in Biological Response Signatures: Indicator Patterns Using Aquatic Communities, ed T. P. Simon (Boca Raton, FL: CRC Press), 13–20.
Carlsson, L., and Berkes, F. (2005). Co-management: concepts and methodological implications. J. Environ. Manage 75, 65–76. doi: 10.1016/j.jenvman.2004.11.008
Chandler, M., See, L., Copas, K., Bonde, A. M., López, B. C., Danielsen, F., et al. (2017). Contribution of citizen science towards international biodiversity monitoring. Biol. Conserv. 213, 280–294. doi: 10.1016/j.biocon.2016.09.004
Conrad, C. C., and Hilchey, K. G. (2011). A review of citizen science and community-based environmental monitoring: issues and opportunities. Environ. Monit. Assess. 176, 273–291. doi: 10.1007/s10661-010-1582-5
Crocker, E., Condon, B., and Almsaeed, A., et al. (2020). TreeSnap: a citizen science app connecting tree enthusiasts and forest scientists. Plants People Planet 2, 47–52. doi: 10.1002/ppp3.41
Dallas, H. F., Kennedy, M., Taylar, J., Lowe, S., and Murphy, S. (2010). SAFRASS. South African Rivers Assessment Scheme, WP4. Review Paper.
Dickens, C. W. S., and Grahm, P. M. (2002). The South African Scoring System (SASS) version 5 rapid bioassessment method for rivers. Afr. J. Aqu. Sci. 27, 1–10. doi: 10.2989/16085914.2002.9626569
Etiegni, C. A., Irvine, K., and Kooy, M. (2017). Playing by whose rules? community norms and fisheries rules in selected beaches within Lake Victoria (Kenya) co-management. Environ. Dev. Sustain. 19, 1557–1575. doi: 10.1007/s10668-016-9799-2
Ewel, J. J. (1999). Natural systems as models for the design of sustainable systems of land use. Agrofor. Syst. 45, 1–21.
Faghihimani, M. (2012). Systemic approach for measuring environmental sustainability at higher education institutions: A case study of the University of Oslo. (Ph.D. thesis). Institute of Educational Research, Faculty of Education, University of Oslo, Oslo, Norway.
Friberg, N. (2014). Impacts and indicators of change in lotic ecosystems. Wiley Interdiscipl. Rev. Water 1, 513–531. doi: 10.1002/wat2.1040
Gikuma-Njuru, P., and Hecky, R. E. (2005). Nutrient concentrations in Nyanza Gulf, Lake Victoria, Kenya: light limits algal demand and abundance. Hydrobiologia 534, 131–140. doi: 10.1007/s10750-004-1418-9
Guya, F. J. (2013). Bioavailability of particle-associated nutrients as affected by internal regeneration processes in the Nyanza Gulf region of Lake Victoria. Lakes Reserv. Res. Manag. 18, 129–143. doi: 10.1111/lre.12031
Guya, F. J. (2019). Intrinsic and extrinsic sources of phosphorus loading into the Nyando River, Kenya. J. Lakes Reserv. Sci. Policy Manag. Sustain. Use 24, 362–371. doi: 10.1111/lre.12289
Haande, S., Rohrlack, T., Semyalo, R. P., Brettum, P., Edvardsen, B., Lyche-Solheim, A., et al. (2011). Phytoplankton dynamics and cyanobacterial dominance in Murchison Bay of Lake Victoria (Uganda) in relation to environmental conditions. Limnologica 41, 20–29. doi: 10.1016/j.limno.2010.04.001
Hjalte, K., Lidgren, K., and Stahl, I. (1977). Environmental Policy and Welfare Economics. Cambridge: Cambridge University Press.
Imende, S., Hoza, R., and Bakunda, A. (2005). “The status, development and role of Beach Managemnet Units (BMU's) in the management of the fishery resource,” in LVFO 2005: The State of The Fisheries Resources of Lake Victoria and Their Management Proceedings of the Regional Stakeholders Conferences, 24-25 February 2005 (Entebbe), 21–30.
Kaaya, L. T., Day, J. A., and Dallas, H. F. (2015). Tanzania River Scoring System (TARISS): a macroinvertebrate based biotic index for rapid bioassessment of rivers. Afr. J. Aquat. Sci. 40, 109–117. doi: 10.2989/16085914.2015.1051941
Karr, J. R. (1981). Assessment of biotic integrity using fish communities. Fisheries 6, 21–27. doi: 10.1577/1548-8446(1981)006<0021:AOBIUF>2.0.CO;2
Karr, J. R., and Chu, E. W. (2000). Sustaining living rivers. Hydrobiologia 422/423, 114. doi: 10.1023/A:1017097611303
Kasangaki, A., Chapman, L. J., and Balirwa, J. (2008). Land use and the ecology of benthic macroinvertebrate assemblages of high-altitude rainforest streams in Uganda. Freshwater Biol. 53, 681–697. doi: 10.1111/j.1365-2427.2007.01925.x
Ko, N. T., Suter, P., Conallin, J., Rutten, M., and Bogaard, T. (2020). The urgent need for river health biomonitoring tools for large tropical rivers in developing countries: preliminary development of a river health monitoring tool for Myanmar Rivers. Water 12:1408. doi: 10.3390/w12051408
Kundu, R., Aura, C. M., Nyamweya, C., Agembe, S., Sitoki, L., Lung'ayia, H. B. O., et al. (2017). Changes in pollution indicators in Lake Victoria, Kenya and their implications for lake and catchment management. Lakes Reserv. Res. Manage. 22, 199–214. doi: 10.1111/lre.12187
Lozano, R., Lukman, R., Lozano, F. J., Huisingh, D., and Lambrechts, W. (2013). Declarations for sustainability in higher education: becoming better leaders, through addressing the university system. J. Clean. Prod. 48, 10–19. doi: 10.1016/j.jclepro.2011.10.006
Lung'ayia, H., Sitoki, L., and Kenyanya, M. (2001). The nutrient enrichment of Lake Victoria (Kenyan waters). Hydrobiologia 458, 75–82. doi: 10.1023/A:1013128027773
Mangadze, T., Dalu, T., and Froneman, P. W. (2019). Biological monitoring in southern Africa: a review of the current status, challenges and future prospects. Sci. Environ. 648, 1492–1499. doi: 10.1016/j.scitotenv.2018.08.252
Masese, F. O., Achieng,', A. O., Raburu, P. O., Lawrence, T., Ives, J. T., Nyamweya, C., et al. (2020). Distribution patterns and diversity of riverine fishes of the Lake Victoria Basin, Kenya. Int. Rev. Hydrobiol. 105, 171–184. doi: 10.1002/iroh.202002039
Masese, F. O., and McClain, M. E. (2012). Trophic resources and emergent food-web attributes in rivers of the Lake Victoria Basin: a review with reference to anthropogenic influences. Ecohydrology 5, 685–707. doi: 10.1002/eco.1285
Masese, F. O., Muchiri, M., and Raburu, P. O. (2009). Macroinvertebrate assemblages as biological indicators of water quality in the Moiben River, Kenya. Afr. J. Aqu. Sci. 34, 15–26. doi: 10.2989/AJAS.2009.34.1.2.727
Masese, F. O., Omukoto, J. O., and Nyakeya, K. (2013). Biomonitoring as a prerequisite for sustainable water resources: a review of current status, opportunities and challenges to scaling up in East Africa. Ecohydrol. Hydrobiol. 13, 173–191. doi: 10.1016/j.ecohyd.2013.06.004
McKinley, D. C., Miller-Rushing, A. J., Ballard, H. L., Bonney, R., Brown, H., Cook-Patton, S. C., et al. (2017). Citizen science can improve conservation science, natural resource management, and environmental protection. Biol. Conserv. 208, 15–28. doi: 10.1016/j.biocon.2016.05.015
Mochizuki, Y., and Yarime, M. (2016). “Education for sustainabile development and sustainability science,” in Re-Purposing Higher Education and Research, eds M. Barth, G. Michelsen, I. Thomas, and M. Rieckmann (London: Routledge Handbook of Higher Education for Sustainable Development), 11–24.
National Population Census (2019). 2019 Kenya Population and Housing Census, Volume I: Population by County and Sub-county. Nairobi: Kenya National Bureau of Statistics, 49.
Obiero, K. O., Abila, R. O., Njiru, M. J., Raburu, P. O., Achieng, A. O., Kundu, R., et al. (2015). The challenges of management: recent experiences in implementing fisheries co-management in Lake Victoria, Kenya. Lakes Reserv. Res. Manag. 20, 139–154. doi: 10.1111/lre.12095
Pickett, S. T. A., Cadenasso, M., Grive, M., and Boone, C. G. (2011). Urban ecological systems: scientific foundations and a decade of progress. J. Environ. Manag. 92, 331–362. doi: 10.1016/j.jenvman.2010.08.022
Pocock, M. J., Tweddle, J. C., Savage, J., Robinson, L. D., and Roy, H. E. (2017). The diversity and evolution of ecological and environmental citizen science. PLoS ONE 12:e0172579. doi: 10.1371/journal.pone.0172579
R Core Team (2014). R: A Language and Environment for Statistical Computing. Vienna: R Foundation for Statistical Computing.
Reid, W. V., Chen, D., Goldfarb, L., Hackmann, H., Lee, Y. T., Mokhele, K., et al. (2010). Earth system science for global sustainability: grand challenges. Science 330, 916–917. doi: 10.1126/science.1196263
Requier, F., Andersson, G. K., Oddi, F. J., and Garibaldi, L. A. (2020). Citizen science in developing countries: how to improve volunteer participation. Front. Ecol. Environ. 18, 101–108. doi: 10.1002/fee.2150
Roux, D. J. (1997). National Aquatic Ecosystem Biomonitoring Programme: Overview of the Design Process and Guidelines for Implementation. NAEBP Report Series No. 6. Pretoria: Institute for Water Quality Studies, Department of Water Affairs and Forestry, South Africa.
Schueler, T. R., Fraley-McNeal, L., and Cappiella, K. (2009). Is impervious cover still important? review of recent research. J. Hydrol. Eng. 14, 309–315. doi: 10.1061/(ASCE)1084-0699(2009)14:4(309)
Seto, K. C., Fragkias, M., Guneralp, B., and Reilly, M. K. (2011). A meta-analysis of global urban land expansion. PLoS ONE 6:e23777. doi: 10.1371/journal.pone.0023777
Smucker, N. J., and Detenbeck, N. E. (2014). Meta-analysis of lost ecosystem attributes in urban streams and the effectiveness of out-ofchannel management practices. Restor. Ecol. 22, 741–748. doi: 10.1111/rec.12134
Statzner, B., Bis, B., Dolédec, S., and Usseglio-Polatera, P. (2001). Perspectives for biomonitoring at large scales: a unified measure for the functional composition of invertebrate communities in European running waters. Basic Appl. Ecol. 2, 73–85. doi: 10.1078/1439-1791-00039
Stephenson, P. J., Ntiamoa-Baidu, Y., and Simaika, J. P. (2020). The use of traditional and modern tools for monitoring wetlands biodiversity in africa: challenges and opportunities. Front. Environ. Sci. 8:61. doi: 10.3389/fenvs.2020.00061
Stoddard, J. L., Herlihy, A. T., Peck, D. V., Hughes, R. M., Whittier, T. R., and Tarquinio, E. (2008). A process for creating multimetric indices for large-scale aquatic surveys. J. North Am. Benthol. Soc. 27, 878–891. doi: 10.1899/08-053.1
Tesfahunegn, G. B., and Gebru, T. A. (2020). Smallholder farmers' level of understanding on the impacts of climate change on water resources in northern Ethiopia catchment. GeoJournal. doi: 10.1007/s10708-020-10265-6
Theobald, E. J., Ettinger, A. K., Burgess, H. K., DeBey, L. B., Schmidt, N. R., Froehlich, H. E., et al. (2015). Global change and local solutions: tapping the unrealized potential of citizen science for biodiversity research. Biol. Conserv. 181, 236–244. doi: 10.1016/j.biocon.2014.10.021
Tol, R. (1995). The damage costs of climate change: towards more comprehensive calculations. Environ. Res. Econ. 5, 353–374. doi: 10.1007/BF00691574
Twesigye, C. K., Onywere, S. M., Getenga, Z. M., Mwakalila, S. S., and Nakiranda, J. K. (2011). The impact of land use activities on vegetation cover and water quality in the Lake Victoria watershed. Open Environ. J. 4, 66–77. doi: 10.2174/1874829501104010066
Verschuren, D., Johnson, T. C., Kling, H. J., Edgington, D. N., Leavitt, P. R., Brown, E. T., et al. (2002). History and timing of human impact on Lake Victoria, East Africa. Proc. R. Soc. Lond. B 269, 289–294. doi: 10.1098/rspb.2001.1850
Wren, D. G., Barkdoll, B. D., Kuhnle, R. A., and Derrow, R. W. (2000). Field techniques for suspended-sediment measurement. J. Hydraul. Eng. 126, 97–104.
Zhang, F., Zhou, B., Liu, L., Liu, Y., Fung, H. H., Lin, H., et al. (2018). Measuring human perceptions of a large-scale urban region using machine learning. Landsc. Urban Plan. 180, 148–160. doi: 10.1016/j.landurbplan.2018.08.020
Zhou, X. Y., Lei, K., Meng, W., and Khu, S. T. (2017). Industrial structural upgrading and spatial optimization based on water environment carrying capacity. J. Clean. Prod. 165, 1462–1472. doi: 10.1016/j.jclepro.2017.07.246
Keywords: citizen science, community-based monitoring, ecological integrity, water pollution, catchment management
Citation: Aura CM, Nyamweya CS, Owiti H, Odoli C, Musa S, Njiru JM, Nyakeya K and Masese FO (2021) Citizen Science for Bio-indication: Development of a Community-Based Index of Ecosystem Integrity for Assessing the Status of Afrotropical Riverine Ecosystems. Front. Water 2:609215. doi: 10.3389/frwa.2020.609215
Received: 22 September 2020; Accepted: 02 December 2020;
Published: 07 January 2021.
Edited by:
Marcus Nüsser, Heidelberg University, GermanyReviewed by:
Barbara Anna Willaarts, International Institute for Applied Systems Analysis (IIASA), AustriaCarly Maynard, Scotland's Rural College, United Kingdom
Copyright © 2021 Aura, Nyamweya, Owiti, Odoli, Musa, Njiru, Nyakeya and Masese. This is an open-access article distributed under the terms of the Creative Commons Attribution License (CC BY). The use, distribution or reproduction in other forums is permitted, provided the original author(s) and the copyright owner(s) are credited and that the original publication in this journal is cited, in accordance with accepted academic practice. No use, distribution or reproduction is permitted which does not comply with these terms.
*Correspondence: Christopher Mulanda Aura, YXVyYW11bGFuZGEmI3gwMDA0MDt5YWhvby5jb20=; YXVyYS5tdWxhbmRhJiN4MDAwNDA7Z21haWwuY29t