- Faculty of Information Technology and Communication Sciences, Tampere University, Tampere, Finland
Enhancing the experience of virtual reality (VR) through haptic feedback could benefit applications from leisure to rehabilitation and training. Devices which provide more realistic kinesthetic (force) feedback appear to hold more promise than their simpler vibrotactile counterparts. However, our understanding of kinesthetic feedback on virtual embodiment is still limited due to the novelty of appropriate kinesthetic devices. To contribute to the line of this research, we constructed a wearable system with state-of-the-art kinesthetic gloves for avatar full-body control, and conducted a between-subjects study involving an avatar self-touch task. We found that providing a kinesthetic sense of touch substantially strengthened the embodiment illusion in VR. We further explored the ability of these kinesthetic gloves to present virtual objects haptically. The gloves were found to provide useful haptic cues about the basic 3D structure and stiffness of objects for a discrimination task. This is one of the first studies to explore virtual embodiment by employing state-of-the-art kinesthetic gloves in full-body VR.
1 Introduction
Immersive virtual reality (VR) experience offers unparalleled visual immersion and natural-like interactions with unique affordances driving the pervasiveness of such applications in leisure, work, and educational contexts. Irrespective of the application domain, virtual embodiment, or the body ownership illusion (Maselli and Slater, 2013; Slater et al., 2010), remains one of the central phenomena in VR. Supported by movement tracking and visuomotor synchrony between the physical and the virtual body or avatar, it presents the core of our interactions within VR, whether in serious or gameful experiences (e.g., Freeman and Maloney, 2021; Fitton et al., 2023). However, multimodal VR peripherals are gaining traction and are promising to reshape our immersive interactions including virtual embodiment.
Not surprisingly, one such fast-developing area is in haptic devices which enable mimicking the sense and experience of touching virtual objects. Vibrotactile devices as a type of haptic devices can provide cutaneous tactile sensation (El Saddik et al., 2011), which have been used to enhance immersion and embodiment by mediating information through vibration signal (e.g., Cui and Mousas, 2021; Moon et al., 2023). However, vibrotactile feedback is relatively simple and limited, which cannot provide realistic touch sensation and thus has a limited application range in VR. Kinesthetic devices have become increasingly prominent with new cutting-edge gloves such as HaptX1 and Sense2. They enable natural bidirectional touch-based interactions with realistic movement-based kinesthetic (force) sensation from the muscles, tendons, and joints (El Saddik et al., 2011), enhancing the users’ perception and interaction with objects in VR and potentially our embodied experience.
Indeed, the potential of various kinesthetic devices has somewhat been explored in the contexts of embodiment, with encouraging results. For example, early studies have adopted grounded kinesthetic devices (e.g., Touch X3) to support presence in a collaborative environment (Sallnäs et al., 2000), improve virtual hand illusion (Choi et al., 2016; Fröhner et al., 2018), and enhance embodied tasks such as drawing (Richard et al., 2021).
However, these kinesthetic studies on virtual embodiment have their own limitations. Firstly, the grounded kinesthetic devices used in their studies (e.g., Sallnäs et al., 2000; Choi et al., 2016; Richard et al., 2021) could only enable single-point (pen-type) kinesthetic interaction and have a small and limited hand-movement workspace due to the fixed length of the mechanical arms of the devices. Therefore, this type of kinesthetic devices is not suited well for interactions in large VR environments, which limits their application and also potentially affects virtual embodiment. Current commercial wearable kinesthetic devices (e.g., HaptX and Sense gloves) adopt an exoskeleton structure to provide free and natural finger-based kinesthetic interaction. These devices are specifically designed for VR and could be used to design more appropriate experiment tasks (e.g., self-touch) for evaluating kinesthetic feedback on virtual embodiment. Furthermore, most of these studies still used a 2D screen-based display to present the experimental virtual environment and tasks (e.g., Sallnäs et al., 2000; Fröhner et al., 2018), instead of using a VR headset. In addition, because of the equipment used, most studies only focused on measuring embodiment based on a virtual hand instead of the whole body (Sallnäs et al., 2000; Choi et al., 2016; Fröhner et al., 2018). In sum, although previous studies were valid to examine the effects of kinesthetic feedback on virtual embodiment, their experimental setups and tasks were restricted by the equipment available at that time, which might have negatively affected virtual embodiment, and the practical effects of kinesthetic feedback on embodiment, including ownership, agency, and change (Roth and Latoschik, 2020), in full-body VR scenarios remain unclear.
Therefore, a complete evaluation of virtual embodiment based on full-body immersive VR avatars using cutting-edge VR equipment and wearable kinesthetic devices is necessary, which impacts individuals, performance, and social interaction (Steed et al., 2016; Pan and Steed, 2019; Bujić et al., 2021; Beyea et al., 2023). To contribute to the line of this research, we constructed a full-body motion tracking system using multiple motion trackers (HTC VIVE4) and a pair of current state-of-the-art wearable kinesthetic gloves (HaptX), which allowed users to control avatars in VR. The purpose was to examine the effects of kinesthetic feedback on virtual embodiment through an avatar self-touch task. This experiment task adopted a between-subjects design with two participant groups (haptic and non-haptic groups). The reason of adopting the between-subjects design, instead of the within-subjects design, was to avoid the context effect. The feelings of participants in the haptic condition would highly likely affect how they feel and respond in the non-haptic condition, and vice versa.
Furthermore, another object discrimination task was designed to ask users to subjectively assess the kinesthetic feedback from the gloves for presenting haptic properties of virtual objects as well as discriminate them through kinesthetic feedback solely. There were two motivations to conduct this additional task. First, this task could explore the ability of the gloves for haptically presenting objects and help understand their feedback characteristics which caused the change in virtual embodiment in the self-touch task. Second, grounded kinesthetic devices generate force feedback through actuators and use a mechanical arm to transfer the force to the user’s hand, providing pen-type kinesthetic interaction. This kind of kinesthetic devices has been explored well for virtual object recognition (e.g., Martínez et al., 2013). Kinesthetic gloves such as HaptX have an independent haptic-generated system and adopt the exoskeleton structure to apply the force on each fingertip, providing finger-based kinesthetic interaction. These gloves have been proposed for model recognition in professional fields [e.g., medicine (Pooryousef et al., 2019)], but its performance has not been explored in this field, and the task results could benefit future technical development for wearable kinesthetic devices. We adopted a within-subjects design for this task with data including self-reported subjective data, objective data of task accuracy and free comments.
Overall, this study uniquely contributes to the issues mentioned through an exploratory two-task mixed-method experiment approach using cutting-edge HaptX gloves in immersive virtual reality by focusing on the following research questions (RQs):
2 Embodiment in immersive VR
2.1 Virtual embodiment and avatars
Virtual embodiment, or body ownership illusion, in VR refers to the sensation that the player’s movements, actions, emotions, and other cognitive or physiological factors are connected to the virtual body (avatar), just as if it was the user’s own physical body (Costa et al., 2013; Beyea et al., 2023). Embodiment is often a crucial feature of VR, especially in terms of function and task performance. For example, in medicine, VR embodiment has been used for motor rehabilitation (Tokgöz et al., 2022), as enhancing virtual embodiment could assist the rehabilitation process (Dallaire-Côté et al., 2016). In entertainment, strong virtual embodiment illusion could largely improve the presence and engagement for VR gaming (Kalina and Johnson-Glenberg, 2020). Furthermore, VR has been used as an online platform for social activities (e.g., Meta Horizon5 and VRChat6), and enhancing virtual embodiment could promote communication and collaboration among people and contribute to social activities (Latoschik et al., 2017; Freeman and Maloney, 2021).
Therefore, virtual embodiment plays a significant role in enhancing the overall experience and immersion for users. Currently, embodiment has been investigated on anthropomorphic and non-anthropomorphic avatars alike, including animals (Krekhov et al., 2019; Weidner et al., 2023). Anthropomorphic and non-anthropomorphic avatars both come in a variety of different styles and with a range of options (e.g., different costumes). Enabling the agency in embodied visual self-representation through these avatars not only potentially supports users’ experience and satisfaction with VR systems (e.g., Bujić et al., 2023), but also opens possibilities for manipulating one’s perceptions, affect, and cognition (e.g., Beyea et al., 2023). However, compared with non-anthropomorphic avatars, anthropomorphic avatars are preferred for the research on virtual embodiment (Roth and Latoschik, 2020).
2.2 Visual modality for virtual embodiment
To enhance virtual embodiment, most of the current VR interaction systems could already provide realistic visual feedback for interaction with virtual objects and avatars. For example, head-mounted displays, such as HTC VIVE and Meta Quest7, could provide high-resolution visual signals with a flexible viewing perspective following the movement and orientation of the user’s head. Furthermore, motion trackers allow the user’s natural body movement as the input for interacting with the virtual environment. Relying on these VR devices, many studies have studied the effects of visual modality and developed related techniques to further enhance virtual embodiment (Fribourg et al., 2020; Roth and Latoschik, 2020).
Visual modality was mainly studied to enhance virtual embodiment due to their unique affordance for full-body illusions which have far-reaching implications for our virtual interactions. Experiential aspects affecting embodiment are found in, for example, viewing a self-avatar (Mohler et al., 2010), first-person perspective and realistic humanoid textures (Maselli and Slater, 2013), and low-latency motor actions such as body and eye movements (Skarbez et al., 2017). In addition, the level of detail of the self-representation could also affect embodiment (Fribourg et al., 2020). These studies provide a crude overview of directions when investigating embodiment (Roth and Latoschik, 2020) but already clearly indicate an extensive focus on the relationship between the visual modality and embodiment. This is unsurprising considering that VR is primarily a visually immersive technology and that visuomotor synchrony appears to clearly elicit a certain level of embodiment. However, the illusion can be further strengthened and manipulated by employing other modalities.
2.3 Haptic modality for virtual embodiment and object recognition
Following the recent advances in haptic technologies and kinesthetic devices in particular, the development of commercial and research haptic devices provides an opportunity for more extensive exploration of the relationship between haptic feedback and embodiment as well as embodied interactions and haptic-based cognition.
Previous studies that used haptic modality to enhance virtual embodiment predominantly relied on vibrotactile interaction systems. In human-computer interaction (HCI), vibrotactile interaction, as a type of haptic interaction, provides relatively simple skin-based haptic feedback for interaction (i.e., vibration), and the related devices are simple, cheap and easy to use, such as linear actuators (El Saddik et al., 2011). These devices are commonly used in current game controllers, smartphones and data gloves (e.g., Almeida et al., 2019) for providing vibrotactile feedback. It has been widely acknowledged that adding such feedback in gaming could improve the sense of presence and enhance virtual embodiment (Orozco et al., 2012). Because of that, multiple vibrotactile interaction systems have been proposed and examined for different games. For example, we have seen the development of a surround haptic interaction system by attaching a tactile pad on a custom-made gaming chair to improve the immersion of driving games (Israr et al., 2012) or exploration of different tactile feedback patterns for combat games (Cui and Mousas, 2021). When it comes to embodiment, there have been efforts to examine its relationship to vibrotactile feedback through, for example, the effect of coordination of visual and vibrotactile feedback on virtual hand illusion (Padilla-Castañeda et al., 2014; Zoulias et al., 2016) or through a comparison of the effects of vibrotactile feedback from different devices including motion controllers and data gloves on users’ sense of presence and engagement (Moon et al., 2023). However, vibrotactile interaction is insufficient to provide users with realistic touch feelings, which thus affects virtual embodiment and also has a limited range of application.
On the other hand, kinesthetic interaction, as another type of haptic interaction, could provide realistic kinesthetic (force) feedback and is more promising for VR applications (El Saddik et al., 2011). Previous studies have explored to use force feedback from kinesthetic devices, such as grounded kinesthetic devices (e.g., Touch X and Force Dimension8), to enhance virtual embodiment. For example, a grounded kinesthetic device has been used in a collaborative computer task, and the results demonstrated that force feedback enhanced the participants’ perceived virtual presence in the collaborative virtual environment (Sallnäs et al., 2000). Furthermore, a grounded kinesthetic device (Choi et al., 2016) and a hand-based custom kinesthetic device (Fröhner et al., 2018) have been used to explore whether force feedback could foster the embodiment of a virtual hand in object manipulation tasks, and both studies demonstrated positive results. In addition, force feedback from a grounded kinesthetic device has been compared with vibrotactile feedback and no haptic feedback for enhancing virtual embodiment based on a drawing task, and the results showed the benefits of force feedback regarding embodiment and subjective performance (Richard et al., 2021).
However, the grounded devices require to be placed on a table and are not suitable for large VR environments due to their limited workspace. Wearable kinesthetic gloves are more suitable for interactions in VR. Compared with the grounded devices that use multiple actuators to generate force, kinesthetic gloves are relatively novel and adopt a different hardware (i.e., exoskeleton structure) with an independent hydraulic, pneumatic (e.g., HaptX), or electromechanical (e.g., Sense) haptic-generated system. Their strengths on enhancing virtual embodiment in VR, as well as limitations, still remain unclear. Furthermore, previous kinesthetic studies have demonstrated that kinesthetic feedback from the grounded kinesthetic devices could provide sufficient haptic cues for haptically presenting object structure (e.g., Martínez et al., 2013). However, some studies argued that kinesthetic feedback solely is difficult to provide the structure details for complex objects (Suga et al., 2023). Because of the novel and different mechanical system as well as the provided finger-based interaction, it is interesting to investigate the performance of wearable kinesthetic gloves for object recognition. More importantly, it would be beneficial for understanding how strong kinesthetic feedback was, which could cause significant embodiment enhancement. Overall, our study is one of the first to explore employing state-of-the-art kinesthetic gloves in full-body VR and investigate their kinesthetic feedback for object recognition.
3 Methods
To answer the research questions, we conducted a two-task study using an immersive virtual reality environment coupled with body tracking and a wearable kinesthetic device (HaptX gloves). We first constructed the prototype experiment system and then designed two experiment tasks: an avatar self-touch task and an object discrimination task.
3.1 Prototype experiment system
The development of the prototype experiment system involved both hardware and software systems (see Figure 1). The hardware system included a head-mounted display (VIVE headset), a pair of kinesthetic gloves (HaptX) and two additional motion trackers (VIVE trackers).
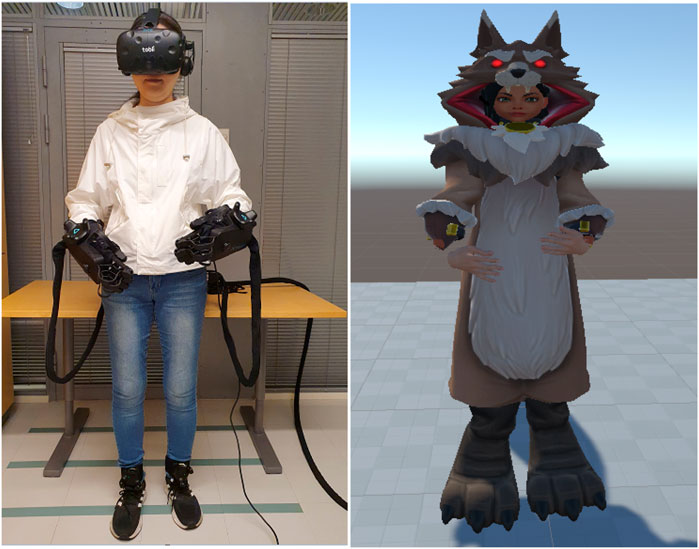
Figure 1. The prototype system including the wearable hardware system and the in-VR view of the controlled avatar: the user is touching the body of her avatar (the avatar self-touch task).
HaptX gloves were used aiming for high haptic fidelity (Muender et al., 2022). As one of the state-of-the-art kinesthetic gloves, HaptX gloves could provide kinesthetic feedback on each fingertip and also vibration feedback on the palm area. Because of the objective of this study, we disabled the vibration feedback on the palm to control the experimental variables. Each kinesthetic glove has a motion tracker which could detect hand motions, and their exoskeleton structure could track the positions of all fingers. The head-mounted display could track the position and orientation of the user’s head and thus could change the user’s view perspective in VR based on the user’s head movement. Two additional motion trackers were attached to the user’s ankles to map leg motions.
The software system was built using Unity development platform (version 2021.3.4f1)9. To control an avatar in the virtual environment, the position and orientation data from the five trackers were transferred to Unity to enable full-body tracking and control. In addition, multiple plugins have been used in the software system. Steam VR plugin10 was used to connect the VR headset, HapX SDK was used to connect the kinesthetic gloves and provide haptic rendering system and collision algorithm for kinesthetic feedback, and FinallK plugin11 was used to customise the avatars.
When the users wore the prototype system, they could touch their avatar using natural hand movements and experience the sensation of touch. Also, the users could perform free limb movement, such as waving, clapping, pointing, and walking, within a 1.5 m
3.2 Tasks
3.2.1 The avatar self-touch task
Task 1 (self-touch) employed a between-subjects design to avoid the context effect, with the goal of understanding the role of kinesthetic feedback on embodiment in VR. A within-subjects design with counterbalanced conditions was also considered. However, because of the increased experiment duration and workload for each participant and their potential significant influences on virtual embodiment when using the within-subjects design, the between-subjects design was adopted for this task.
Moreover, as the HaptX gloves could not provide vibrotactile feedback on fingertips when touching virtual object, this task only involved haptic and non-haptic conditions without vibrotactile condition. Changing or adding extra tactile devices would complicate the experimental variables and affect virtual embodiment (e.g., the changing device weight). Two conditions (haptic vs. non-haptic) would be sufficient to demonstrate the effects of kinesthetic feedback on embodiment.
In addition, avatars used in the task were created through ReadyPlayerMe12. They were used for multiple reasons relating to the current literature and usability. Primarily, such anthropomorphic avatars (see Figure 1) are most commonly used avatars for the research with virtual embodiment (Roth and Latoschik, 2020). Furthermore, on the usability, it was important to eliminate instances when the user’s hands would reach his/her physical body before the kinesthetic feedback is triggered by the boundaries of the avatar, which would negatively affect virtual embodiment. Therefore, the avatars with large costumes were selected, which provided enough distance between the boundaries of the avatar and the user’s physical body. Finally, to reduce incongruency and avoid confounding effects of self-representation (Schulze et al., 2019), two versions of the avatar with arguably masculine and feminine facial features were used in this task for the different genders of the participants (see Figure 2).
The process of this task was as follows. As synchronous motor action is a common method for inducing virtual embodiment (Maselli and Slater, 2013; Roth and Latoschik, 2020), participants were first instructed to perform a scripted series of movements in front of a mirror (see Figure 2) while embodying a gender-congruent avatar. This included head rotation, waving, finger gestures, and walking. Then, they were instructed to touch and explore their avatar using their virtual hands, and the virtual hands would stop when reaching the boundaries of the avatar, providing visual feedback (non-haptic group). Those in the haptic group had kinesthetic feedback coupled with the visual one. To control the effects of different viewing perspectives on virtual embodiment (Maselli and Slater, 2013), participants were only allowed to watch their movement and touch behaviors through the mirror.
3.2.2 The object discrimination task
Task 2 (object discrimination) employed a within-subjects design and focused on the assessment of the kinesthetic feedback from the gloves. The task included two groups of objects: simple objects and avatars. For each group, the task required participants to assess the kinesthetic feedback for presenting different haptic properties of objects (subjective data) and also select the correct object relying on kinesthetic feedback solely (objective data). Simple objects and avatars had clear differences in the complexity of 3D models, and the avatars had more complex structures than the simple objects. Comparing task results between these two groups could help understand the ability of the gloves for haptically presenting objects. To avoid making the task unnecessary difficult, the selected objects had clear visual differences in structure among them. For the simple objects, the selected objects included ball, cube, cylinder and pyramid. For the avatars, the required touch places were the costume heads, and their differences were mainly on the major characteristics (e.g., animal head with big ears vs. animal head with no ears vs. normal head), instead of small characteristics (see Figure 3). Generally, the objects used in the task would be easily discriminated if we could use real hands to directly touch.
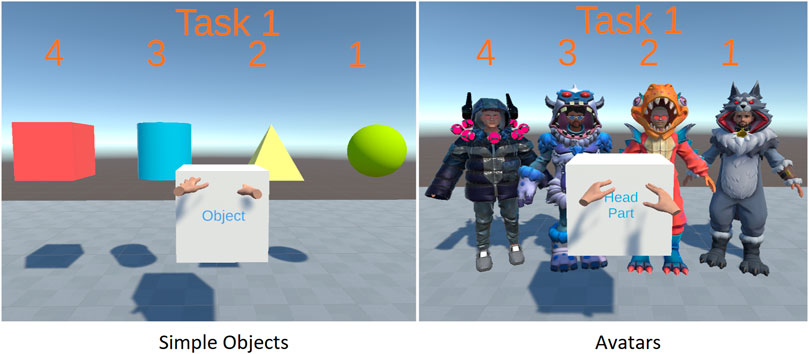
Figure 3. In-VR view of the two group objects in the object discrimination task - simple object and avatar head discrimination relying on kinesthetic feedback from the object hidden inside a visual box.
The process of this task was as follows. Participants performed two groups of the task: one in discriminating simple objects and the other in discriminating avatar heads. In both instances, they could see all four options, and an object or avatar was randomly selected and visually hidden inside an image of a white box. Each object or avatar could be only selected once from the four options by the system (this detail was not available to the participants). Participants performed four trials for each group of the task and then assess the feedback through a questionnaire.
3.3 Measurement
Task 1 - self-touch. For assessing virtual embodiment, Virtual Embodiment Questionnaire - VEQ (Roth and Latoschik, 2020) including the three-dimensional 7-point Likert validated scale was used for the measurement. The three factors includes Ownership (the feeling of that the user could possess the virtual body as own body), Agency (the feeling of that the user could control and perform actions with the virtual body) and Change (the feeling of that the user’s appearance and weight have been changed), and each of them has four questionnaire statements (all statements can be found in this study). They demonstrated good to excellent internal consistency when examined using the robust McDonald’s Omega (.751, .804, and .853, respectively) (McNeish, 2018).
Task 2 - object discrimination. Based on haptic material properties (Baumgartner et al., 2015), we constructed a 7-point Likert-type scale for the assessment of ease of perception of different properties of the objects. Each property was represented and assessed using a single item: “The object is perceived three-dimensional” (3D), “The object is perceived as having stiffness” (Stiffness), “The surface is perceived as having friction” (Friction), “The shape of the object is perceivable” (Shape), and “The size of the object is perceivable” (Size). This self-report subjective data was further complemented with the objective data on the correct number of performed trials by the participants (i.e., object discrimination accuracy). This objective data could overall reflect the quality of kinesthetic feedback from the gloves for haptically presenting object structure, as participants could rely on both the shapes and the sizes of the objects (or their components) to discriminate them. At last, participants could provide free-form comments for using the wearable system.
3.4 Pilot study
To ensure the validity and reliability of the experiment designs, we first conducted a pilot study (n = 6). The main points of improvement in the avatar self-touch task were the positioning of wearable trackers, joint parameters of avatars, and adjustment of avatar sizes to ensure that participants would not touch their physical bodies before reaching the boundary of the avatar. In the object discrimination task, this entailed the position and size of the target-object masking box, the object selection, and the number of trials based on the required time for completion. In addition, kinesthetic feedback from all objects and avatars was calibrated to match their visual models and perceptible to participants for object touch and discrimination.
4 Experiment
4.1 Participants
The sample size (N = 32) was determined primarily based on previously reported large effect sizes of kinesthetic feedback on embodiment. For example, Sallnäs et al. (2000) has conducted a between-subjects study and demonstrated a practical large effect size (d
All participants were recruited from the local university community (16 female and 16 male) with ages between 19 and 48 years (M = 27.7, SD = 7.0). Among them, 21 had tried an immersive VR headset before but with limited experience (one to four times). None of the participants had experience with HaptX gloves or similar kinesthetic devices. All participants self-reported normal touch sensitivity and participated in both tasks.
4.2 Apparatus
The host computer used in the experiment was an MSI GS63VR 7RF Stealth Pro laptop with an Intel i7-7700HQ processor and a GeForce GTX 1060 graphics card. A HTC VIVE VR headset (first generation), a pair of HaptX gloves (DK2 version) and two VIVE motion trackers constituted the hardware system of the wearable prototype system. The software system was developed using Unity (2021.3.4f1), along with three plugins, including SteamVR, HaptX SDK and FinallK, to support the connection of VR headset, the calibration of HaptX gloves and the avatar customization.
4.3 Procedure
Firstly, the participants were presented with the informed consent form. The researchers received the information from the local committee that, according to the national and university-specific guidelines on research ethics, this study did not require ethics board approval due to no significant risks involved as long as voluntary and informed participation practices were followed. After signing the informed consent form, the participants completed the background questionnaire.
All participants were involved in the self-touch task (through one experimental condition only: haptic or non-haptic) followed by the object discrimination task (through both experimental conditions: simple objects and avatars), with the entire session lasting approximately an hour. In the self-touch task, the participants were randomly assigned into the two groups (haptic and non-haptic) while minding the gender distribution (eight male and eight female vs. eight male and eight female). The wearable kinesthetic system was calibrated for each participant, including height and head, limb, and finger movements. Those in the haptic group were additionally informed about the function of the wearable system with the force feedback. The predefined motions in front of the mirror lasted approximately 3 min, after which they had the freedom to explore their avatar for five additional minutes. Following the self-touch task, they were given up to 10 min to complete the virtual embodiment questionnaire and take a break.
The object discrimination task was done with the participants sitting in a chair and the order of the two groups of the task (simple objects and avatars) was randomised for counterbalancing with 3 min of rest in-between tasks. For each discrimination trial, the participants were given up to 2 min to touch and examine the hidden object with both hands. They were informed that the touched object would be randomly selected by the system for each trial and the same object might be selected multiple times (to maintain the selection probability, that is, one out of four options). After making the selection and reporting the answer to the researcher, the participants were given 1 min to rest before moving to the next trial. After completing four trials, they answered the measures relating to the quality of the kinesthetic feedback through the questionnaire. After completing the task with both object groups, they could provide free comments for the whole experiment.
5 Results
5.1 Task 1 - Avatar self-touch task
The relationship between kinesthetic feedback and embodiment was assessed using the three dimensions of virtual embodiment questionnaire (VEQ), namely Ownership, Agency, and Change. Because of each dimension had four questionnaire statements, the mean score of each dimension was used in the statistical analyses [as mentioned by Roth and Latoschik (2020)]. The descriptive statistics of the results are presented in Table 1 and the boxplots are shown in Figure 4. The Shapiro-Wilk Normality test showed that the data were normally distributed (all p > .05). Therefore, the data satisfied the assumptions for the independent samples t-test, prompting the use of that parametric test for a comparison of outcomes between the two experimental conditions.
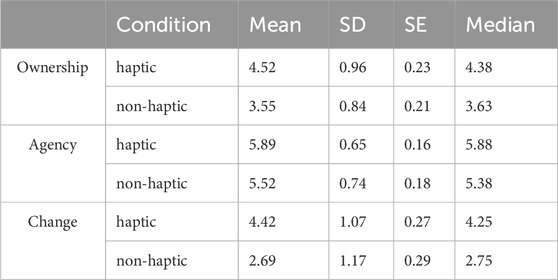
Table 1. Descriptive statistics of Ownership, Agency, and Change scores in haptic and non-haptic conditions, including mean, standard deviation (SD), standard error (SE) and median.
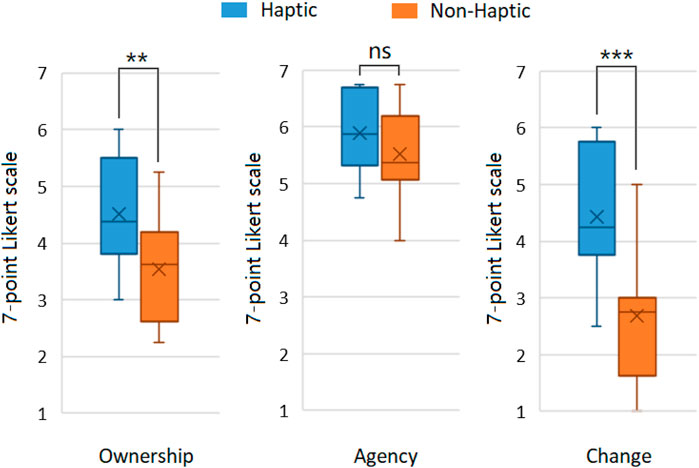
Figure 4. Boxplots for Ownership, Agency, and Change scores (the cross mark in the boxplot shows the mean value and the line shows the median value). Note: *p < .05, **p < .01, ***p < .001.
The results suggested that Ownership (t (30) = 3.074, p = .004) and Change (t (30) = 4.379, p < .001) in particular significantly benefited from the added kinesthetic feedback, resulting in stronger illusions than when there was visual feedback only. On the other hand, there was no apparent difference when it comes to the perceived Agency over the virtual avatar (t (30) = 1.523, p = .138).
5.2 Task 2 - Object discrimination task
In the second task, the participants subjectively assessed the kinesthetic feedback from the gloves. Additionally, they were tasked with identifying the correct object or avatar out of four options (objective data) based on kinesthetic feedback solely.
The result boxplots are shown in Figure 5. Generally, the participants provided positive responses for perceiving simple objects, in terms of whether the objects were three-dimensional and had stiffness as well as whether their shapes and sizes were perceivable (all above the neutral level 4 in the 7-point Likert scale), except for whether the objects had friction. The participants provided positive responses for perceiving avatars, in terms of whether they were three dimensional and had stiffness, however, they provided negative responses for other three measurements (all below 4).
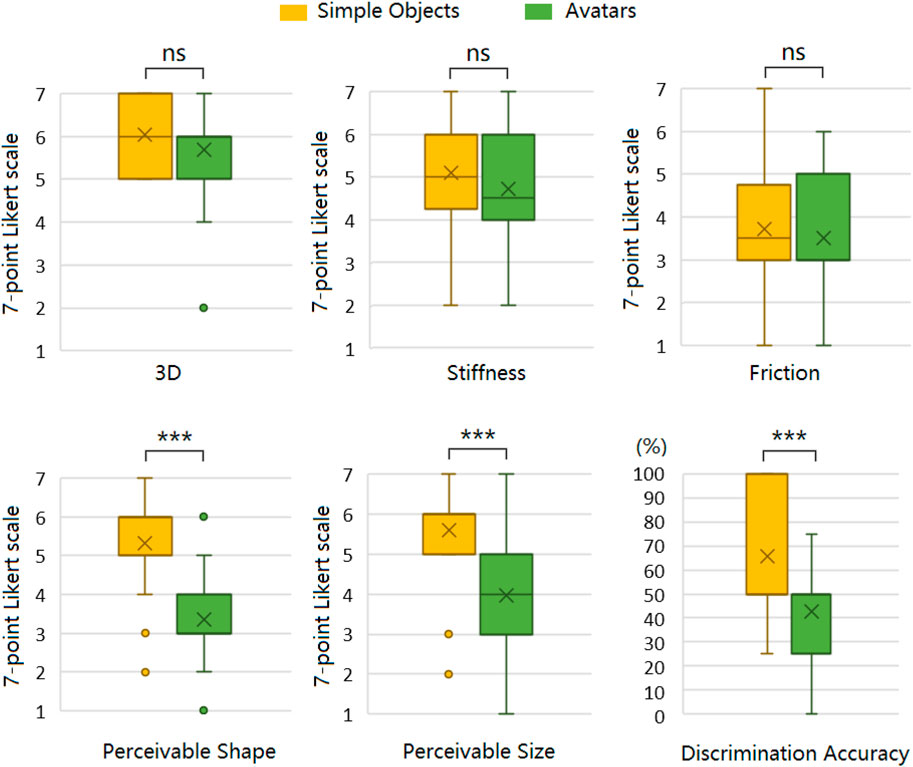
Figure 5. Boxplots for the feedback quality and object discrimination accuracy for simple objects and avatars (higher values mean better performance). Note: *p < .05, **p < .01, ***p < .001.
Because the data were not normally distributed (all p < .05 shown in the Shapiro-Wilk Normality test), Wilcoxon Signed-Rank non-parametric test was adopted. It showed that there were no statistically significant differences between the simple objects and avatars, in terms of whether they were three dimensional (Z = −1.432, p = .152) and had stiffness (Z = −1.846, p = .065) and friction (Z = −1.261, p = .207). However, the participants felt that the shapes (Z = −4.359, p < .001) and sizes (Z = −3.641, p < .001) of the simple objects were more perceivable than the ones of the avatars. In the objective data, the participants have a higher accuracy rate (percentage) for the object discrimination task with the simple objects (M = 0.66, SD = 0.25) than the avatars (M = 0.43, SD = 0.21; Z = −3.336, p < .001).
5.3 Free comments
Finally, free-form optional comments from the participants for the experiment were collected but scarce. However, some reported being intrigued by the possibility of touch in the virtual environment, while others emphasised the weight and overall lack of comfort when using the elaborate kinesthetic system. Worth noting are also a few comments for the task 2 reflecting on the usability of the system and the lack of congruence with the physical environment. Without visual feedback, they could easily move their hands beyond the edge of the hidden objects and into them, making it difficult and attention-heavy to consistently explore boundaries of the objects for the discrimination task.
6 Discussion
6.1 Virtual embodiment
To examine virtual embodiment including ownership, agency, and change, we divided the participants into two groups and both groups were asked to wear the full-body tracking system with the kinesthetic gloves. One group would embody the VR avatar through mirrored visuomotor synchrony known as a simple mechanism for inducing embodiment (Maselli and Slater, 2013; Slater et al., 2010; Skarbez et al., 2017) and another group would additionally explore their avatars via touch sensation.
In our study, the users’ sense of body ownership and perceptual change in their bodies were greatly positively affected by the added haptic stimulus, which is congruent with previous findings on the relationship between kinesthetic feedback and body ownership (Longo et al., 2008) but extended their results in physical environments to VR environments and embodied avatars. Moreover, previous research in the VR domain focused on stimuli outside of the visual self-representation and especially in interactions with other objects (Fröhner et al., 2018; Richard et al., 2021). We extended this vein of literature by focusing on the user and their experience of embodiment in VR, which is a prolific and highly impactful field (e.g., Bujić et al., 2021; Thibault and Bujić, 2020; Freeman and Maloney, 2021). Overall, our study demonstrated that, when users stepped into a VR environment and controlled a full-body avatar, allowing them to touch and feel their virtual body through kinesthetic feedback could greatly enhance their senses of body ownership and change.
On the other hand, the participants’ self-reported level or extent of agency over the virtual embodiment does not seem to significantly differ. Based on the test statistics in combination with the visual representation through boxplots (Figure 4), the effect on agency was notably smaller than for the other two dimensions. However, agency appeared to have been rated relatively high regardless of the conditions, which might hamper the benefits of the kinesthetic feedback. If our results did not present a false negative, the different results on agency between our study and previous studies might be because of the different experiment tasks. Our task was the avatar self-touch task which mainly used body movement to control the avatar, and kinesthetic feedback likely had less impact on this control experience. However, the previous studies showing benefits of haptic feedback on agency were based on object manipulation tasks (e.g., Padilla-Castañeda et al., 2014), and providing the multisensory feedback during such tasks could enhance the experience of object control, consequently bleeding into their experience of control over their virtual bodies.
Regardless of the nuances, adding kinesthetic feedback could significantly benefit the illusion of embodiment and could play a compelling role in our immersive VR interactions when the hardware becomes more affordable, reliable, and easy to use. Moreover, previous studies have additionally compared different haptic feedback (e.g., vibrotactile, kinesthetic, and no feedback) in this research field (Fröhner et al., 2018; Richard et al., 2021), to which we contribute by additionally examining the quality of kinesthetic feedback from the gloves for haptically presenting objects and exploring the direction of future technology improvement for wearable kinesthetic devices (discussed in the following section).
6.2 Object discrimination
When it comes to object discrimination, the participants perceived that kinesthetic feedback from the gloves could provide haptic cues for basic 3D structure and stiffness for both simple objects and avatars, except friction. Furthermore, the participants felt easier in perceiving the shapes and sizes of simple objects than in perceiving the avatars. Our objective data showed similar results that the accuracy rate of the object discrimination task with simple objects was 66%, and the accuracy rate was decreased to 43% for the avatar discrimination. In fact, both accuracy rates were low especially considering the task accuracy rate through guess only could achieve between 17.5% and 32.5% approximately (32 participants completed 4 trials with 25% guess correct chance for each trial and 95% confidence intervals).
Firstly, it is no surprise that the participants provided positive responses for 3D and stiffness but negative responses for friction, regardless of simple objects and avatars. Because of the mechanical structure of the gloves, the force feedback was applied to the participants’ fingertips perpendicular to the finger pad surfaces, which made them have touch sensation for the stiffness and the basic 3D structure of the objects, but this could be also the main reason for the difficulty to perceive the friction.
However, based on both the subjective and objective data, perceiving the object shape and size was overall difficult. Our different results compared with the previous research could be because of the different kinesthetic devices used. Previous research has demonstrated that the kinesthetic feedback from the grounded devices could provide accurate virtual object recognition, which could even lead to a competitive task performance compared with using real fingers to directly touch physical objects (accurate rate: 94.2% vs. 96.9%) (Martínez et al., 2013). Grounded kinesthetic devices are point-based devices which generate force feedback based on the touch point (without size) on the object. The gloves we used, as well as other kinesthetic gloves, provide force feedback based on the contact areas between the fingertips and the object. The sizes of the contact areas are large (i.e., normally equal to the sizes of fingertip pads) which could cause the difficulty to provide accurate haptic cues for the surface parts smaller than the pad sizes. Therefore, the force form the gloves has a lower resolution compared with the one from the grounded devices. Furthermore, for perceiving 3D virtual objects with intricate shapes, force feedback solely has been argued insufficient and additional cutaneous sensory inputs are needed for perceiving structure details such as the edges of complex 3D objects (Suga et al., 2023).
In addition to the reasons above, as the participants mentioned in the comments, their hands could feel touch sensation while touching but also could move through the objects, which confused them when performing the discrimination task. This phenomenon was not an issue in the self-touch task, as the participants normally stopped moving their hands when they saw their virtual hands contacting on the surface of the avatar. However, in the object discrimination task, the participants might still move their hand because of the lack of the visual feedback (the object was hidden in the white box), which caused the difficulty to explore the boundary of the objects. Moreover, the gloves felt bulky and heavy which caused fatigue, especially after a prolonged period of interaction. Heightened fatigue could disturb our sense of position and affect estimating spatial positions (Allen and Proske, 2006), which might have contributed further to the difficulty in the object discrimination. All of these could contribute to understanding the difficulty in identifying the object shapes and sizes and the low task accuracy, especially for the avatars.
Connecting to the self-touch task, our results of the discrimination task implied that kinesthetic feedback that could provide haptic cues for the basic 3D structure and the stiffness of objects could already significantly enhance virtual embodiment. The HaptX gloves, as well as other kinesthetic gloves such as Sense gloves, commonly adopt the similar mechanical exoskeleton structure to provide finger-based kinesthetic interaction. Future development for these wearable kinesthetic gloves could focus on improving the resolution of the kinesthetic feedback, adding additional cutaneous sensory inputs, providing force feedback to the user’s whole hand in addition to their fingers to avoid the issue of moving through the objects (e.g., by adding an extra exoskeleton arm) and simultaneously reducing the size and weight of hardware. Addressing these issues could be challenging but beneficial for improving their usability in VR and enhancing virtual embodiment when using them to control avatars. Overall, technical advance in wearable kinesthetic devices could greatly expand their VR applications and benefit more professional works and social activities (e.g. Kim et al., 2023).
6.3 Limitations and future studies
Although the expected and obtained effect sizes are predominantly of notable strength (Sallnäs et al., 2000), generalizability of the results is still limited by the sample (e.g., different age groups). Our results can be expanded on by testing different samples, and also in conjunction with some of the suggestions below.
The first task to examine virtual embodiment entailed self-touch for a limited duration and with an unfamiliar system to the participants. It is unclear how experienced users would respond to the stimulus and whether there is a component of the novelty effect in our results. Moreover, it was a static and focused experience, while for example adding haptic feedback for object manipulation tasks could enhance the agency of the virtual hand and potentially improve performance (Padilla-Castañeda et al., 2014). Future studies could employ more complex interaction tasks in VR to examine the robustness of the embodiment enhancement. Additionally, these systems and their preliminary implications for the illusion of embodiment open new avenues for exploring social touch (Gallace and Girondini, 2022) and phantom touch (Alexdottir and Yang, 2022) in immersive virtual reality.
Furthermore, although previous studies have compared kinesthetic feedback with vibrotactile feedback regarding virtual embodiment and demonstrated similar performances (Fröhner et al., 2018; Richard et al., 2021), it is still interesting to explore their differences in VR scenarios with full-body avatars. Because of the technical limitation of the gloves used (i.e., the absence of vibrotactile feedback on fingertips), it was not involved in this experiment. Future studies could explore this area when appropriate kinesthetic devices are available.
In addition, this study adopted a questionnaire to measure virtual embodiment. This is currently the commonly used method for measuring virtual embodiment in VR (Roth and Latoschik, 2020). However, there are also objective validated methods for assessing the strength of aspects of the illusion, such as using neurophysiological markers through electroencephalography (EEG) (Jeunet et al., 2018), which could be tested and used in the future studies.
Lastly, kinesthetic gloves become increasingly popular and have been proposed for virtual object recognition tasks in professional fields, such as using kinesthetic gloves for model recognition of anatomical models in medicine (Pooryousef et al., 2019). Understanding the factors that influence object recognition for kinesthetic feedback could largely contributes to the development of current kinesthetic gloves and their application. This study investigated object recognition using kinesthetic gloves, which is the first but preliminary research in this field. The potential issues found included the low resolution force, the lack of cutaneous sensory inputs and the force for the whole hand. Future studies could continue to explore this area.
7 Conclusion
We presented a two-task study on haptics-mediated virtual embodiment and exploration of the used wearable kinesthetic devices for haptically presenting objects. In the first task, we used a between-subjects design to examine the effect of kinesthetic feedback from embodied boundaries coupled with visuomotoric synchrony on the strength of the embodiment illusion. In the second task, we used a within-subjects design and explored how users perceived the experience of kinesthetic feedback when examining hidden objects as well as their consequent accuracy when discriminating objects. Overall, our results suggested that embodiment is greatly enhanced through the addition of kinesthetic exploration of virtual selves in immersive environments but there are also several limitations in the current capabilities of the system when it comes to correctly perceiving complex objects. These system developments and their evaluations are not only relevant for user virtual experience but also for developing VR as versatile and highly accessible tools and media.
Data availability statement
The raw data supporting the conclusions of this article will be made available by the authors, without undue reservation.
Ethics statement
The studies involving humans were approved by Ethics Committee of the Tampere Region. The studies were conducted in accordance with the local legislation and institutional requirements. The participants provided their written informed consent to participate in this study.
Author contributions
ZL: Conceptualization, Data curation, Formal Analysis, Investigation, Methodology, Software, Validation, Visualization, Writing–original draft, Writing–review and editing. MB;: Conceptualization, Formal Analysis, Methodology, Validation, Writing–original draft, Writing–review and editing. OB: Conceptualization, Methodology, Validation, Writing–review and editing. EB: Methodology, Validation, Writing–review and editing. SJ: Methodology, Validation, Writing–review and editing. JH: Funding acquisition, Methodology, Project administration, Resources, Supervision, Validation, Writing–review and editing.
Funding
The author(s) declare that financial support was received for the research, authorship, and/or publication of this article. The study was supported by POSTEMOTION project (Research Council of Finland, number: 342144).
Conflict of interest
The authors declare that the research was conducted in the absence of any commercial or financial relationships that could be construed as a potential conflict of interest.
Publisher’s note
All claims expressed in this article are solely those of the authors and do not necessarily represent those of their affiliated organizations, or those of the publisher, the editors and the reviewers. Any product that may be evaluated in this article, or claim that may be made by its manufacturer, is not guaranteed or endorsed by the publisher.
Footnotes
3https://www.3dsystems.com/haptics-devices/touch-x
8https://www.forcedimension.com/products
10https://store.steampowered.com/app/250820/SteamVR/
11https://assetstore.unity.com/packages/tools/animation/final-ik-14290
References
Alexdottir, S., and Yang, X. (2022). “Phantom touch phenomenon as a manifestation of the visual-auditory-tactile synaesthesia and its impact on the users in virtual reality,” in 2022 IEEE International Symposium on Mixed and Augmented Reality Adjunct (ISMAR-Adjunct), Singapore, 17–21 October 2022 (IEEE), 727–732.
Allen, T. J., and Proske, U. (2006). Effect of muscle fatigue on the sense of limb position and movement. Exp. Brain Res. 170, 30–38. doi:10.1007/s00221-005-0174-z
Almeida, L., Lopes, E., Yalçinkaya, B., Martins, R., Lopes, A., Menezes, P., et al. (2019). “Towards natural interaction in immersive reality with a cyber-glove,” in 2019 IEEE International Conference on Systems, Man and Cybernetics (SMC), Bari, Italy, 06-09 October 2019 (IEEE), 2653–2658.
Baumgartner, E., Wiebel, C. B., and Gegenfurtner, K. R. (2015). A comparison of haptic material perception in blind and sighted individuals. Vis. Res. 115, 238–245. doi:10.1016/j.visres.2015.02.006
Beyea, D., Ratan, R. R., Lei, Y. S., Liu, H., Hales, G. E., and Lim, C. (2023). “A new meta-analysis of the proteus effect: studies in vr find stronger effect sizes,” in PRESENCE: virtual and augmented reality, 1–30.
Bujić, M., Macey, A.-L., Järvelä, S., and Hamari, J. (2021). Playing with embodied social interaction: a thematic review of experiments on social aspects in gameful virtual reality. Interact. Comput. 33, 583–595. doi:10.1093/iwc/iwac012
Bujić, M., Macey, A.-L., Kerous, B., Belousov, A., Buruk, O., and Hamari, J. (2023). “Self-representation does (not) spark joy: experiment on effects of avatar customisation and personality on emotions in vr,” in 7th international GamiFIN conference (CEUR-WS), 96–105.
Choi, W., Li, L., Satoh, S., and Hachimura, K. (2016). Multisensory integration in the virtual hand illusion with active movement. BioMed Res. Int. 2016, 1–9. doi:10.1155/2016/8163098
Costa, M. R., Kim, S. Y., and Biocca, F. (2013). “Embodiment and embodied cognition,” in Virtual Augmented and Mixed Reality. Designing and Developing Augmented and Virtual Environments: 5th International Conference, VAMR 2013, Held as Part of HCI International 2013 (Las Vegas, NV: Springer), 333–342.
Cui, D., and Mousas, C. (2021). “Evaluating wearable tactile feedback patterns during a virtual reality fighting game,” in 2021 IEEE International Symposium on Mixed and Augmented Reality Adjunct (ISMAR-Adjunct), Bari, Italy, 04-08 October 2021 (IEEE), 328–333.
Dallaire-Côté, M., Charbonneau, P., Côté, S. S.-P., Aissaoui, R., and Labbe, D. R. (2016). “Animated self-avatars for motor rehabilitation applications that are biomechanically accurate, low-latency and easy to use,” in 2016 IEEE Virtual Reality (VR), Greenville, SC, 19-23 March 2016 (IEEE), 167–168.
El Saddik, A., Orozco, M., Eid, M., and Cha, J. (2011). Haptics technologies: bringing touch to multimedia. Springer Science and Business Media.
Fitton, I., Clarke, C., Dalton, J., Proulx, M. J., and Lutteroth, C. (2023). “Dancing with the avatars: minimal avatar customisation enhances learning in a psychomotor task,” in Proceedings of the 2023 CHI conference on human factors in computing systems, 1–16.
Freeman, G., and Maloney, D. (2021). Body, avatar, and me: the presentation and perception of self in social virtual reality. Proc. ACM human-computer Interact. 4, 1–27. doi:10.1145/3432938
Fribourg, R., Argelaguet, F., Lécuyer, A., and Hoyet, L. (2020). Avatar and sense of embodiment: studying the relative preference between appearance, control and point of view. IEEE Trans. Vis. Comput. Graph. 26, 2062–2072. doi:10.1109/tvcg.2020.2973077
Fröhner, J., Salvietti, G., Beckerle, P., and Prattichizzo, D. (2018). Can wearable haptic devices foster the embodiment of virtual limbs? IEEE Trans. haptics 12, 339–349. doi:10.1109/toh.2018.2889497
Gallace, A., and Girondini, M. (2022). Social touch in virtual reality. Curr. Opin. Behav. Sci. 43, 249–254. doi:10.1016/j.cobeha.2021.11.006
Israr, A., Kim, S.-C., Stec, J., and Poupyrev, I. (2012). Surround haptics: tactile feedback for immersive gaming experiences. CHI’12 Ext. Abstr. Hum. Factors Comput. Syst. (ACM), 1087–1090. doi:10.1145/2212776.2212392
Jeunet, C., Albert, L., Argelaguet, F., and Lécuyer, A. (2018). “do you feel in control?”: towards novel approaches to characterise, manipulate and measure the sense of agency in virtual environments. IEEE Trans. Vis. Comput. Graph. 24, 1486–1495. doi:10.1109/tvcg.2018.2794598
Kalina, E., and Johnson-Glenberg, M. C. (2020). “Presence and platform: effects of embodiment comparing a 2d computer and 3d vr game,” in 2020 6th International Conference of the Immersive Learning Research Network (iLRN), San Luis Obispo, CA, 21-25 June 2020 (IEEE), 31–37.
Kang, H. (2021). Sample size determination and power analysis using the g* power software. J. Educ. Eval. health Prof. 18, 17. doi:10.3352/jeehp.2021.18.17
Kim, S., Cherian, J., Ray, S., Lacy, A., Taele, P., Koh, J. I., et al. (2023). A wearable haptic interface for assisting blind and visually impaired students in learning algebraic equations. Ext. Abstr. 2023 CHI Conf. Hum. Factors Comput. Syst. 1–7. doi:10.1145/3544549.3585815
Krekhov, A., Cmentowski, S., and Krüger, J. (2019). “The illusion of animal body ownership and its potential for virtual reality games,” in 2019 IEEE Conference on Games (CoG), London, UK, 20-23 August 2019 (IEEE), 1–8.
Latoschik, M. E., Roth, D., Gall, D., Achenbach, J., Waltemate, T., and Botsch, M. (2017). “The effect of avatar realism in immersive social virtual realities,” in Proceedings of the 23rd ACM symposium on virtual reality software and technology, 1–10.
Longo, M. R., Schüür, F., Kammers, M. P., Tsakiris, M., and Haggard, P. (2008). What is embodiment? a psychometric approach. Cognition 107, 978–998. doi:10.1016/j.cognition.2007.12.004
Martínez, J., García, A. S., Molina, J. P., Martínez, D., and González, P. (2013). An empirical evaluation of different haptic feedback for shape and texture recognition. Vis. Comput. 29, 111–121. doi:10.1007/s00371-012-0716-x
Maselli, A., and Slater, M. (2013). The building blocks of the full body ownership illusion. Front. Hum. Neurosci. 7, 83. doi:10.3389/fnhum.2013.00083
McNeish, D. (2018). Thanks coefficient alpha, we’ll take it from here. Psychol. methods 23, 412–433. doi:10.1037/met0000144
Mohler, B. J., Creem-Regehr, S. H., Thompson, W. B., and Bülthoff, H. H. (2010). The effect of viewing a self-avatar on distance judgments in an hmd-based virtual environment. Presence 19, 230–242. doi:10.1162/pres.19.3.230
Moon, H. S., Orr, G., and Jeon, M. (2023). Hand tracking with vibrotactile feedback enhanced presence, engagement, usability, and performance in a virtual reality rhythm game. Int. J. Human–Computer Interact. 39, 2840–2851. doi:10.1080/10447318.2022.2087000
Muender, T., Bonfert, M., Reinschluessel, A. V., Malaka, R., and Döring, T. (2022). “Haptic fidelity framework: defining the factors of realistic haptic feedback for virtual reality,” in Proceedings of the 2022 CHI conference on human factors in computing systems, 1–17.
Orozco, M., Silva, J., El Saddik, A., and Petriu, E. (2012). The role of haptics in games. Haptics Render. Appl. 217–234. doi:10.5772/32809
Padilla-Castañeda, M. A., Frisoli, A., Pabon, S., and Bergamasco, M. (2014). The modulation of ownership and agency in the virtual hand illusion under visuotactile and visuomotor sensory feedback. Presence 23, 209–225. doi:10.1162/pres_a_00181
Pan, Y., and Steed, A. (2019). “Avatar type affects performance of cognitive tasks in virtual reality,” in Proceedings of the 25th ACM symposium on virtual reality software and technology, 1–4.
Pooryousef, V., Brown, R., and Turkay, S. (2019). “Shape recognition and selection in medical volume visualisation with haptic gloves,” in OzCHI ’19: proceedings of the 31st Australian conference on human-computer-interaction, 433–436.
Richard, G., Pietrzak, T., Argelaguet, F., Lécuyer, A., and Casiez, G. (2021). Studying the role of haptic feedback on virtual embodiment in a drawing task. Front. Virtual Real. 1, 573167. doi:10.3389/frvir.2020.573167
Roth, D., and Latoschik, M. E. (2020). Construction of the virtual embodiment questionnaire (veq). IEEE Trans. Vis. Comput. Graph. 26, 3546–3556. doi:10.1109/tvcg.2020.3023603
Sallnäs, E.-L., Rassmus-Gröhn, K., and Sjöström, C. (2000). Supporting presence in collaborative environments by haptic force feedback. ACM Trans. Computer-Human Interact. (TOCHI) 7, 461–476. doi:10.1145/365058.365086
Schulze, S., Pence, T., Irvine, N., and Guinn, C. (2019). “The effects of embodiment in virtual reality on implicit gender bias,” in Virtual, Augmented and Mixed Reality. Multimodal Interaction: 11th International Conference, VAMR 2019, Held as Part of the 21st HCI International Conference (Orlando, FL: Springer), 361–374.
Skarbez, R., Brooks, F. P., and Whitton, M. C. (2017). A survey of presence and related concepts. ACM Comput. Surv. (CSUR) 50, 1–39. doi:10.1145/3134301
Slater, M., Spanlang, B., Sanchez-Vives, M. V., and Blanke, O. (2010). First person experience of body transfer in virtual reality. PloS one 5, e10564. doi:10.1371/journal.pone.0010564
Steed, A., Pan, Y., Zisch, F., and Steptoe, W. (2016). “The impact of a self-avatar on cognitive load in immersive virtual reality,” in 2016 IEEE virtual reality (VR) (IEEE), 67–76.
Suga, Y., Miyakami, M., Mizoguchi, I., and Kajimoto, H. (2023). “3d shape presentation by combination of force feedback and electro-tactile stimulation,” in 2023 IEEE World Haptics Conference (WHC), Delft, Netherlands, 10-13 July 2023, 361–367.
Thibault, M., and Bujić, M. (2020). Vrban-strategies of representation and degrees of freedom in virtual cities. Meaning-Making Ext. Real. doi:10.4399/978882553432010
Tokgöz, P., Stampa, S., Wähnert, D., Vordemvenne, T., and Dockweiler, C. (2022). Virtual reality in the rehabilitation of patients with injuries and diseases of upper extremities. Healthc. (MDPI) 10, 1124. doi:10.3390/healthcare10061124
Weidner, F., Boettcher, G., Arboleda, S. A., Diao, C., Sinani, L., Kunert, C., et al. (2023). A systematic review on the visualization of avatars and agents in ar and vr displayed using head-mounted displays. IEEE Trans. Vis. Comput. Graph. 29, 2596–2606. doi:10.1109/tvcg.2023.3247072
Zoulias, I. D., Harwin, W. S., Hayashi, Y., and Nasuto, S. J. (2016). “Milliseconds matter: temporal order of visuo-tactile stimulation affects the ownership of a virtual hand,” in Haptics: Perception, Devices, Control, and Applications: 10th International Conference (London, UK: Springer), 479–489.
Keywords: virtual embodiment, kinesthetic feedback, wearable, virtual reality, body ownership illusion, agency
Citation: Li Z, Bujić M, Buruk O‘, Bampouni E, Järvelä S and Hamari J (2024) Haptics-mediated virtual embodiment: Impact of a wearable avatar-controlling system with kinesthetic gloves on embodiment in VR. Front. Virtual Real. 5:1439724. doi: 10.3389/frvir.2024.1439724
Received: 28 May 2024; Accepted: 23 August 2024;
Published: 04 September 2024.
Edited by:
Stephen Palmisano, University of Wollongong, AustraliaReviewed by:
Richard Skarbez, La Trobe University, AustraliaJoel Teixeira, University of Wollongong, Australia
Copyright © 2024 Li, Bujić, Buruk, Bampouni, Järvelä and Hamari. This is an open-access article distributed under the terms of the Creative Commons Attribution License (CC BY). The use, distribution or reproduction in other forums is permitted, provided the original author(s) and the copyright owner(s) are credited and that the original publication in this journal is cited, in accordance with accepted academic practice. No use, distribution or reproduction is permitted which does not comply with these terms.
*Correspondence: Zhenxing Li, WmhlbnhpbmcuTGlAdHVuaS5maQ==