- 1Division of Cognitive Psychology, Perception and Research Methods, Institute of Psychology, University of Bern, Bern, Switzerland
- 2Education & Digital Technologies Lab, Institute of Research, Development and Evaluation, Bern University for Teacher Education, Bern, Switzerland
Introduction: Virtual reality (VR) offers new possibilities for learning in educational settings by navigating through large 3D virtual environments. When designing VR-based learning applications, developers have to decide between different locomotion techniques to navigate through VR. Since physical activity and walking have been shown to enhance learning, physical walking in VR should increase learning compared to locomotion techniques without physical activity.
Methods: In this study, we examined if learners who are able to walk freely in VR differ regarding their declarative knowledge acquisition from learners who are teleported in VR.
Results: Learning outcomes did not differ between these two conditions, neither immediately after learning in VR nor after a one-day delay. Also, participants’ sense of presence in the virtual environment did not differ between the two conditions.
Discussion: These findings suggest that both teleportation and walking are suitable for declarative knowledge acquisition in VR, and that teleportation may be sufficient enough.
1 Introduction
Virtual reality (VR) opens exciting new possibilities for immersive learning experiences by allowing users to navigate three-dimensional virtual worlds. These environments can range from emulating medieval cities and museums to chemical laboratories (Checa and Bustillo, 2020; Giangreco et al., 2019; Hu-Au and Okita, 2021). Moreover, these environments also allow to teach abstract content like climate change in an efficient way [e.g., Markowitz et al. (2018), Thoma et al. (2023)]. Presenting learning content in VR is a promising alternative to conventional learning materials. For instance, earlier research compared knowledge acquisition between a group of students who completed an architecture lesson using VR and a group who completed the lesson using presentation slides, videos, and pictures. The students who used VR for learning showed higher scores in a subsequent knowledge test (Wu et al., 2021). In a similar vein, Gloy et al. (2022) compared immersive VR anatomy atlases with anatomy textbooks. Results showed that students who learned in VR completed the test faster and achieved a higher proportion of correct answers. Indeed, the beneficial effects of VR on learning have been shown in multiple meta-analyses [e.g., Villena-Taranilla et al. (2022), Wu et al. (2020), Yu (2021)]. Learning often involves the acquisition of declarative knowledge which refers to an individual’s knowledge about facts or ideas (Anderson, 1976; Shavelson et al., 2005). Webster (2016) compared the acquisition of declarative knowledge between a VR setting and a lecture-based setting, showing that VR enhanced the acquisition of declarative knowledge. Thus, VR is also effective for learning new declarative knowledge.
In VR, locomotion is a key element to optimally exploit the benefits of VR, leading to better immersion and improved presence (e.g., Kim and Rhiu (2021), Langbehn et al. (2018)). The methods of navigating in VR vary, with navigation relying on joysticks, teleportation, or physical walking. Each method for navigation in VR has its benefits and disadvantages. The usage of a joystick for movement has been shown to result in more motion sickness than walking and teleportation (Buttussi and Chittaro, 2019; Caputo et al., 2023; Frommel et al., 2017; Langbehn et al., 2018). However, walking in VR requires more physical effort, time to traverse a VR environment, and a larger physical area compared to movement using a joystick or teleportation (Bozgeyikli et al., 2016; Buttussi and Chittaro, 2019; Kim and Rhiu, 2021; Shewaga et al., 2017). Additional hardware like omnidirectional treadmills could resolve the problem of available physical space but they are currently still expensive. Redirected walking solves the problem of available space by adjusting participants’ path as soon as they reach the end of their available physical space (e.g., Banakou and Slater (2023)). Based on users’ preferences, Langbehn et al. (2018) highlighted that both teleportation and redirected walking should be favored over movement by joystick in VR. The development of current VR headsets like the Meta Quest 3 seems to align with these suggestions by offering a dynamic shift between teleportation and walking–for instance in the hub environment. Despite this, Sayyad et al. (2020) observed a preference for walking over teleportation in their study, and multiple studies also showed that physically walking through VR increases the sense of presence and spatial orientation compared to teleportation (Kim and Rhiu, 2021; Langbehn et al., 2018; Shewaga et al., 2017; Slater et al., 1995; Usoh et al., 1999). It remains unclear how walking and teleportation affect the acquisition of declarative knowledge. More research is needed to investigate which type of locomotion is best suited for declarative knowledge acquisition in VR, and this has motivated us to conduct this study.
Embodied cognition theories suggest that the acquisition of knowledge is linked to the sensory experience of one’s body and its movements, highlighting the significance of physical engagement and real-world context in learning (Barsalou, 1999). Indeed, the body is involved in a wide array of cognitive functions, including language comprehension (Glenberg and Kaschak, 2002), numerical understanding (Link et al., 2013), or metaphorical understanding (Wilson and Gibbs, 2007). However, some educational contents merely rely on the acquisition of purely declarative knowledge with limited potential for embodied alignment. Nevertheless, embodiment also enables the potential of physical activation, which has been shown to enhance academic performance and achievements (Rasberry et al., 2011; Wretman, 2017; Zabriskie and Heath, 2019). Moreover, physical activation through movement breaks during classes has been shown to increase students’ attention (Daly-Smith et al., 2018; Lynch et al., 2022). These studies suggest that physical activities in the learning process may be crucial. Notably, the amount of physical activation is possibly more intense in these studies compared to walking. However, studies in the educational context also showed the positive effects of walking on learning (Biber and Heidorn, 2021; Weight et al., 2021). The walking classroom is a didactic approach in which students listen to education podcasts while walking. This approach has been shown to increase students’ long-term retention, as well as their self-perceived learning efficacy, happiness, and energy (Biber and Heidorn, 2021; Erwin et al., 2021; Weight et al., 2021). The walking classroom also enhances students’ alertness and information processing (Erwin et al., 2021; Weight et al., 2021) which might facilitate the acquisition of declarative knowledge. While findings from the walking classroom approach show the cognitive benefits of physical activity, it is an open question to what extent the same mechanisms also apply to walking in VR environments.
Previous research has shown that walking in VR has a positive effect – for example regarding presence and motion sickness (Ibánez et al., 2016; Saredakis et al., 2020). However, further research is needed to investigate the effect of physically walking in VR on learning – especially regarding the acquisition of declarative knowledge. This aspect is particularly important for designers of future educational applications in VR, as they are faced with the important decision of whether to include physical walking or teleportation in VR. The enhanced learning and cognitive functioning during the walking classroom suggest that walking in VR enhances declarative knowledge acquisition. However, the walking classroom shows that continuous walking during knowledge acquisition enhances learning. In VR applications, however, walking is rather used to move between points of interest at which declarative knowledge can be acquired. The short physical activity of walking between points of interest might also enhance cognitive functioning and thereby enhance learning. Riecke et al. (2010) have shown enhanced performance in a navigation task when participants navigated through VR by physical walking instead of using joysticks. Earlier studies could not corroborate these findings (Moreno and Mayer, 2002), but VR headsets have dramatically improved in usability and movement possibilities over the last decade. Recent research by Queiroz et al. (2023) found that movement in VR reduces the amount of learning compared to sitting in VR. Contrary, Johnson-Glenberg et al. (2021) showed enhanced learning due to movement, albeit their study varied movement only in regard of hand movements. Consequently, there has not been any conclusive evidence if walking and teleportation in VR might affect the outcome of declarative knowledge acquisition differently.
In this study, we examined if learners who are able to walk in VR differ regarding their declarative knowledge acquisition from learners who are teleported in VR. Recent meta-analyses have shown that VR enhances learning (Wu et al., 2020; Yu, 2021), and these effects were also observed for the acquisition of declarative knowledge (Webster, 2016). However, these studies did not compare whether the type of movement in VR affects knowledge acquisition. Previous research on embodied cognition suggests that physical activity is beneficial for learning [e.g., Rasberry et al. (2011), Weight et al. (2021), Wretman (2017), Zabriskie and Heath (2019)]. Therefore, we expected that in VR the outcomes of declarative knowledge acquisition of walking learners are better than those of teleported learners. To investigate whether the knowledge acquired in VR is still available on the following day, we compared immediate and delayed recall.
2 Methods
2.1 Participants
Seventy-five students participated in a laboratory experiment in exchange for course credit. A power analysis performed with G*Power (Faul et al., 2007) produced a minimum sample size of 60 participants (effect size of 0.2 for a between-within interaction with a power of .85, a p-value of .05, measurements and groups of 2, and correlations among the repeated measurements of .5). The exclusion criteria included wearing a pacemaker, hearing implants or hearing aids, susceptibility to migraine, epilepsy, as well as neurological or psychiatric disease. Eleven participants were excluded due to technical issues during the experiment. The final sample consisted of 64 participants. 44 participants were female (68.75%), and 20 participants were male (31.25%). Participants’ age ranged from 18‒29 years (M = 22.3 years, SD = 2.0 years). None of the participants were enrolled in a curriculum that relates to the learning content of the experiment (i.e., astronomy). All participants gave their written informed consent and could withdraw from the study at any time. The study protocol was reviewed and approved by the ethics committee of the faculty of human sciences of the authors’ institution.
2.2 Experimental design
A 2 × 2-mixed-factor design was conducted with locomotion type (walking vs. teleportation) in VR and measurement time (immediately after vs. 24 h after learning) as independent variables. Participants were randomly assigned to the walking or teleportation condition and explored a VR environment exhibiting the solar system. The main dependent variable was the acquired knowledge about the solar system measured with a quiz described below. Additionally, participants’ experience in the virtual environment (e.g., presence, motion sickness) was assessed by means of the questionnaires also described below.
2.3 Material
2.3.1 Virtual environment
A 3D virtual environment was developed using Unreal Engine, version 4.27 (Epic Games, 2019). This virtual environment was modelled as a museum. The museum consisted of six rooms which were connected by an elevator. Each room measured 4.94 × 3.87 m and the elevator 1.88 × 1.16 m. The museum showed an exhibition of the solar system. The first room showed a model of the solar system with all eight planets labeled with their respective names (see Figure 1). In the subsequent four rooms, each room presented two planets of the planetary solar system which were placed in opposite corners (see Figure 2). The distance between the points where the participants had to study the planet spanned 3 m. Overall, the expected pathway in each room consisted of approximately 9 m for each room. The presentation of these planets aligned with the order of the planets in the solar system. For each planet, four facts were presented alongside the miniature (e.g., “orbital period around the Sun: 84 years”, “ice giant”, “3rd largest planet”, or “named after Greek god” for Uranus; see Figure 3 for an example). The final room showed all planets in order of the solar system (see Figure 4). The virtual environment was displayed using a wireless, motion-tracked head-mounted display (HTC Vive Pro 2; 2,448 × 2,448 pixels per eye) and a desktop PC (using a NVIDIA GeForce GTX 1080 graphics card and an Intel Core i7 processor).
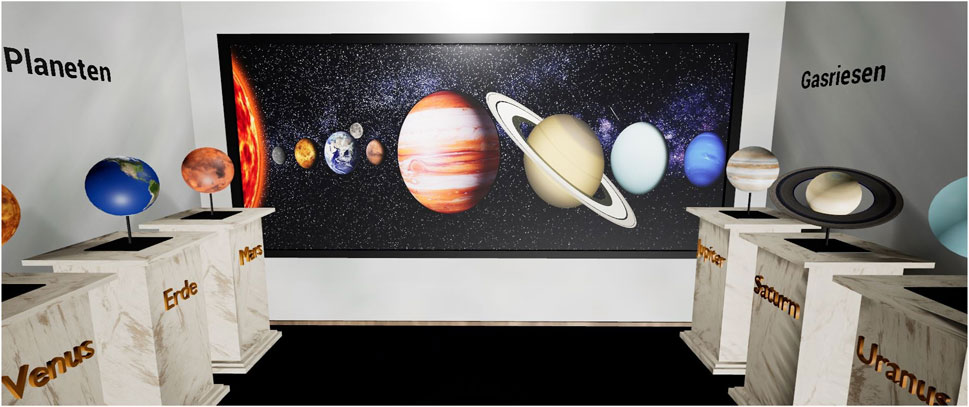
Figure 1. The first room of the VR museum which shows all eight planets labeled with their respective names.
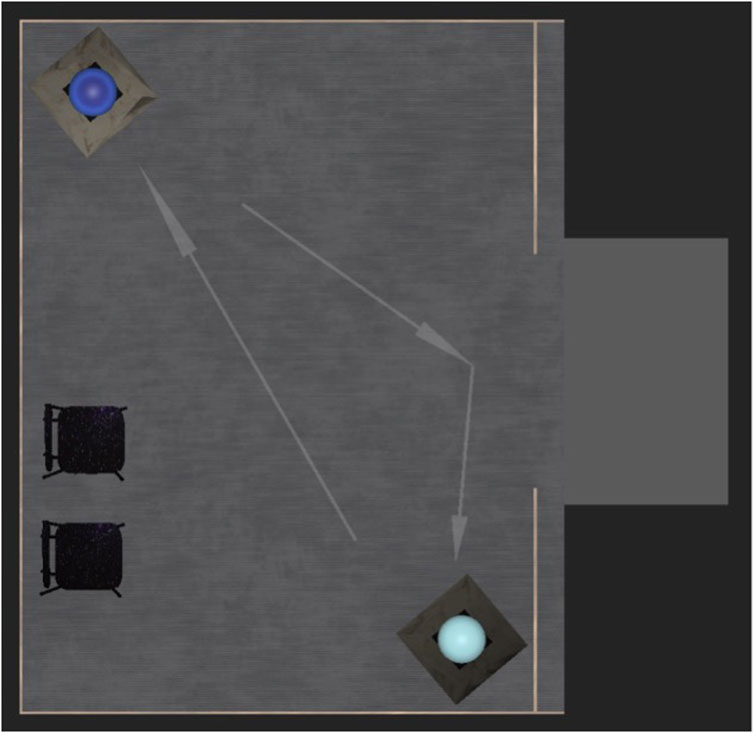
Figure 2. An example room from the bird’s perspective. Two planets are depicted in the top left and bottom right corner. In the whole-body movement condition, arrows sequentially emerge on the floor, guiding participants step by step to their destinations.
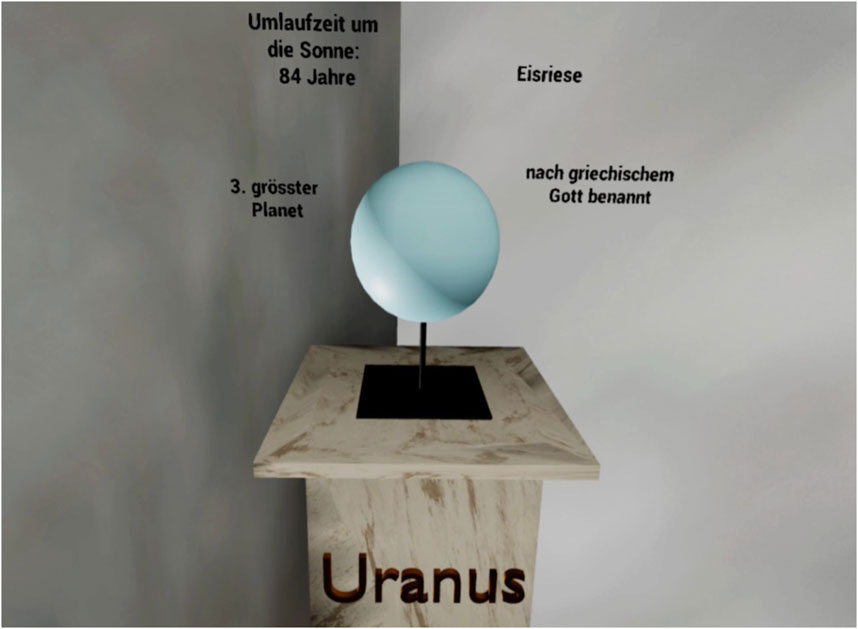
Figure 3. An example room of the virtual museum showing Uranus and the corresponding information about the planet.
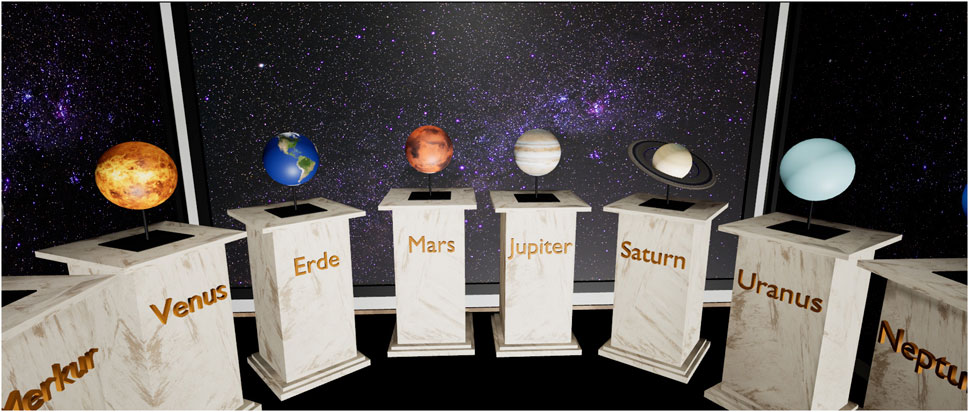
Figure 4. The last room of the VR museum which shows all eight planets in the order of the solar system.
2.3.2 Measurement of learning outcomes
A quiz consisting of 14 open-ended questions was created to evaluate participants’ declarative knowledge of the planetary solar system after exploration of the virtual environment. Table 1 shows each question and the percentage of correct answers given by participants across all conditions. The quiz was conducted using Qualtrics (https://www.qualtrics.com).
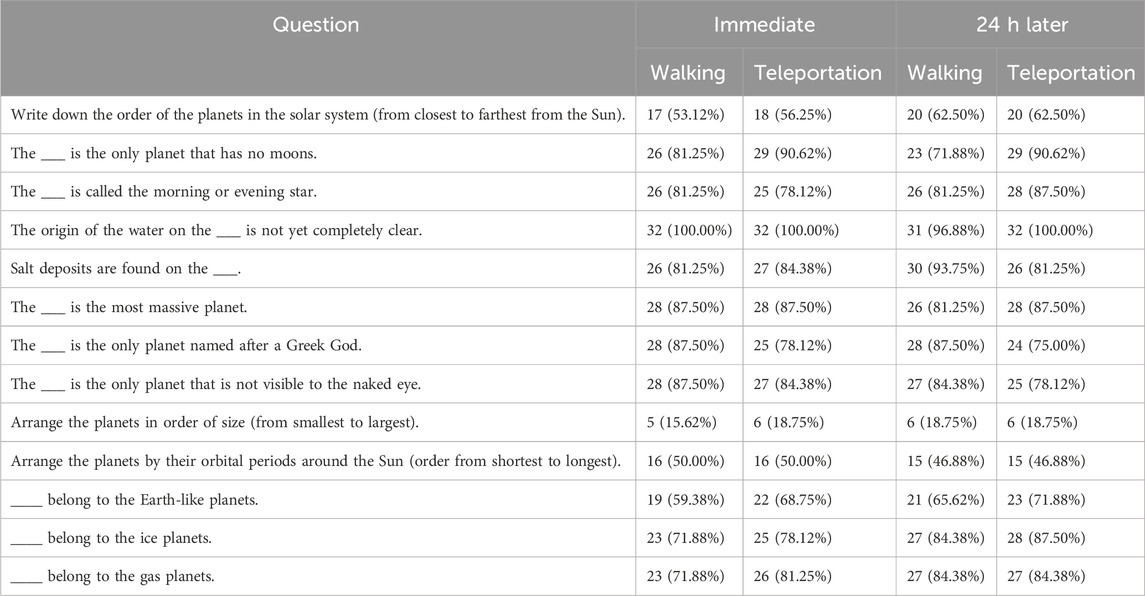
Table 1. Questions of the quiz for evaluating participants’ declarative knowledge immediately after and 24 h after learning in VR. For each condition, the frequency of correct answers is displayed with the percentage of correct answers for the respective condition in brackets.
2.3.3 Questionnaires
The sense of presence was measured using an adapted German version of the presence scale (Kim and Biocca, 1997). Participants rated the nine items using a 7-point Likert scale. The items were reformulated by changing “broadcast” and “television” to “experience in virtual reality”. Additionally, the pictorial presence self-assessment-manikins (PP-SAM; Weibel et al., 2015) was used to measure sub-dimensions of presence using pictorial manikins. This questionnaire consists of six items, each measuring a different aspect of presence (self-location, possible actions, attention allocation, spatial situation model, higher cognitive involvement, and suspension of disbelief). The virtual reality sickness questionnaire (VRSQ; Kim et al., 2018) was used to measure potential symptoms of motion sickness. This questionnaire contains 9 symptoms for which participants indicate the experienced intensity using a 4-point Likert scale (0 = not at all, 1 = slightly, 2 = moderately, and 3 = very). The VRSQ covers two subdomains of symptoms (oculomotor and disorientation), which can be summed to a total score of symptoms ranging from 0%‒100%. Additionally, participants were asked to indicate the amount of prior VR experience by choosing between “not at all”, “little” or “many”, as well as describing their prior knowledge about the presented topic using an open-ended question.
2.4 Procedure
Participants were randomly assigned to the walking or teleportation condition with each condition comprising 32 participants. Participants were informed that the study investigates the potential of VR for declarative learning and that they should remember the displayed information in VR. Participants explored the virtual environment either by physically walking or teleportation. In both conditions, the order of the rooms was kept the same. Participants started by standing in the elevator. When the elevator opened, participants could step into the first room. In the first room, they were instructed to study the model of the solar system and become acclimated to the virtual environment. Subsequently, they had to return into the elevator. After stepping back into the elevator, the doors closed before opening again on the next floor, where they began to explore the next room. This was the first room in which two planets were presented. Each participant followed the same path which was signaled by arrows on the floor. Whenever the participants would stand in front of a planet, the corresponding information would appear. After 30 s, the information disappeared, signaling to the participant to move on. This pattern was repeated across all rooms. In the walking condition, the participants could physically walk along the signaled path to the second planet in the room. In the teleportation condition, participants remained in a standing position and did not move in real life. Teleportation occurred automatically so that participants were not required to use a controller. This was done to reduce both motor activity and interference due to the use of the controllers. Furthermore, this allowed us to align the time needed for teleportation with the expected time for walking through the VR environment.
Right after the VR learning experience, participants were asked to complete the declarative knowledge quiz. Subsequently, presence, motion sickness, and the PP-SAM were assessed, and participants were asked about their prior knowledge and VR experience. On the next day, participants returned to the lab and answered the knowledge quiz again. Participants were not told about a second knowledge test in advance. Instead, they were told that they would visit a different virtual world on the second day. This was done to prevent participants from learning more about the presented topics between the two sessions.
2.5 Data analysis
Responses to the quiz questions were checked manually with one point per correct answer. Misspelled answers (e.g., “Markury” instead of “Mercury”) were also counted as correct. Data analysis was performed using R Studio (Posit Team, 2023) and R (R Core Team, 2023). To assess the acquisition of knowledge, a mixed ANOVA was conducted with the movement condition (walking vs. teleportation) as between-subject factor and the measurement time (immediately vs. 24 h after the VR experience) as within-subject factor. Independent sample t-tests were conducted to compare the effects of the movement condition on presence and motion sickness. If the assumptions for an independent sample t-test were violated, Kruskal–Wallis tests were used instead. Given the absence of significant differences between the movement conditions, additional equivalence tests were performed for participants’ knowledge, presence, and motion sickness using jamovi (The jamovi project, 2024).
3 Results
3.1 Knowledge
Participants could score up to 14 points in the knowledge quiz. Their scores in the quiz ranged from 1 to 13 (M = 9.54, SD = 2.54). Table 2 shows the mean values for each condition. Participants’ scores did not differ, F(1, 62) = 0.12, p = .734, ηp2 < .01, between the walking (M = 9.44, SD = 2.59) and the teleportation condition (M = 9.64, SD = 2.51). Moreover, participants’ scores immediately after learning in VR (M = 9.42, SD = 2.72) did not differ, F(1, 62) = 0.95, p = .333, ηp2 = .02, from their scores after 24 h (M = 9.66, SD = 2.37). There was no interaction between experimental condition and time, F(1, 62) = 0.11, p = .746, ηp2 < .01.
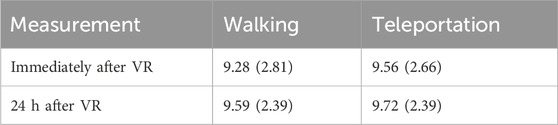
Table 2. Means and standard deviation (in brackets) of the scores in the quiz for each condition immediately and 24 h after learning in VR.
Most participants reported having no prior knowledge about the learning content presented in VR and no or only little prior experience using VR. The findings regarding the learning outcomes reported above remain the same when only looking at participants stating no prior knowledge. Similarly, the findings remain the same if the sample is split according to participants’ previous experience with VR. The sample characteristics for both groups are shown in Table 3.
3.2 Sense of presence
Scores in the presence questionnaire ranged from 2.5 to 6. Presence scores did not differ, t(61.83) = 0.49, p = .627, d = 0.12, between the walking (M = 4.42, SD = 0.72) and the teleportation condition (M = 4.34, SD = 0.68). Participants did also not differ in any of the dimensions measured by the PP-SAM (see Table 4).
3.3 Motion sickness
The motion sickness scores measured with the VRSQ ranged from 33.3 to 75.8. Shapiro-Wilk test (W = 0.87, p < .001) showed that the data were not normally distributed, and therefore, we used a Kruskal–Wallis test. There was no difference, χ2(1) = 1.7, p = .192, η2 = .01, in motion sickness between the walking condition (M = 42.71, SD = 8.82) and the teleportation condition (M = 46.20, SD = 10.17). The scores in the oculomotor subscale of the VRSQ ranged from 33.3 to 91.6, and the data did not follow a normal distribution (W = 0.87, p < .001). The walking condition (M = 44.79, SD = 11.74) did not differ regarding the scores in the oculomotor subscale from the teleportation condition (M = 49.48, SD = 13.71), χ2(1) = 2.22, p = .136, η2 = .02. The scores in the disorientation subscale of the VRSQ ranged from 33.33 to 60, and the data did not follow a normal distribution (W = 0.86, p < .001). Again, the walking condition (M = 40.62, SD = 7.45) did not differ, χ2(1) = 1.28, p = .258, η2 < .00, from the teleportation condition (M = 42.92, SD = 8.28). The distribution of the scores in the VRSQ is shown in Figure 5.
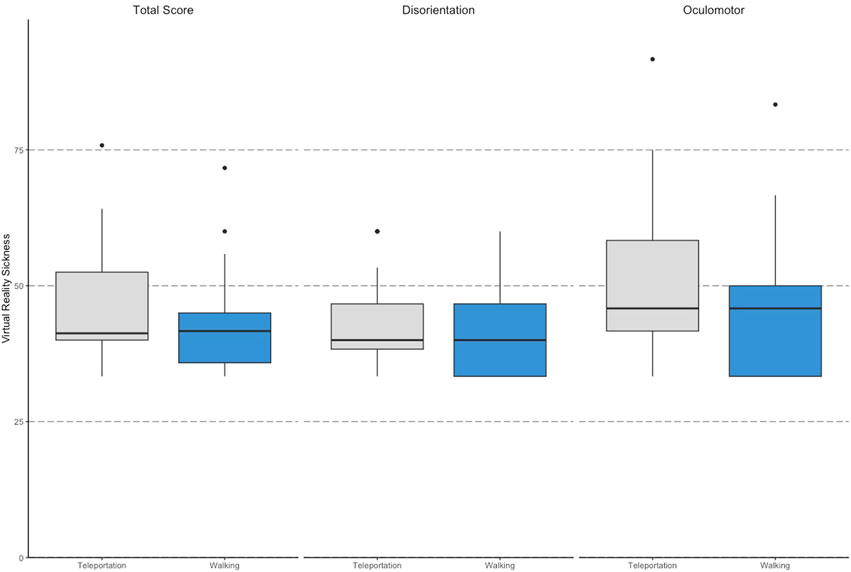
Figure 5. Scores in the virtual reality sickness questionnaire, both for the overall scores and the two subdimensions (disorientation and oculomotor).
3.4 Equivalence testing
Neither the equivalence test on participants’ declarative knowledge outcomes (t(61.83) = −1.00, p = .162, equivalence bounds ±0.4) nor the equivalence test on the VRSQ overall score (t(60.78) = −1.634, p = .054, equivalence bounds ±0.4) reached significance. For presence, the equivalence test reached significance (t(61.83) = −1.79, p = .040, equivalence bounds ±0.4). This suggests that walking results in the same amount of presence as teleportation.
4 Discussion
We examined if learners enabled to walk in VR differed regarding their declarative knowledge acquisition from learners that were teleported in VR. We found no evidence for an advantage of walking when compared to teleportation. However, a post hoc power analysis revealed that the power for the comparisons of the between-subjects factor was low. Thus, further studies will be needed to replicate the absence of differences in declarative knowledge acquisition between walking and teleportation. Moreover, we did not find any differences in motion sickness or presence between the two experimental conditions. Hence, our findings do not confirm previous findings showing enhanced learning due to physical walking or an increased sense of presence when walking in VR (Slater et al., 1995; Weight et al., 2021; Zabriskie and Heath, 2019).
Our study focused on the planetary solar system, and we assessed different types of declarative knowledge such as lexical knowledge (e.g., planet names), relational knowledge (e.g., the order of planets in relation to each other), and conceptual knowledge (e.g., identifying all gas planets). Hence, it is possible that walking in VR can affect the learning of other types of knowledge, especially that of procedural knowledge. This may be hypothesized to occur when walking movements in a learning task are meaningfully related to the process of knowledge acquisition (Skulmowski and Rey, 2018), for instance by being aligned with each other. VR allows for the creation of educational settings that align with body movements, and developers of VR applications should consider the advantages of VR during development (Bailenson, 2018).
Previous studies found evidence for the beneficial effect of walking classrooms on learning [e.g., Weight et al. (2021)]. Our study did not detect any noticeable improvement in learning attributed to walking in VR. This contradicts studies observing cognitive benefits after walking or physical activity (Erwin et al., 2021; Johnson-Glenberg et al., 2021; Oppezzo and Schwartz, 2014; Rasberry et al., 2011; Wretman, 2017; Zabriskie and Heath, 2019), but aligns with earlier studies finding no enhanced learning due to body movement in VR (Moreno and Mayer, 2002; Queiroz et al., 2023). One explanation might be that participants in the teleportation condition – despite not moving – remained in a standing position during the VR experience. Previous studies have shown that a standing position enhances both executive functions and working memory when compared to sitting (Mehta et al., 2016). These beneficial effects of standing could also improve the acquisition of declarative knowledge. However, there is no evidence for standing in a classroom setting having an advantage regarding learning outcomes (Chim et al., 2021). Therefore, it is unlikely that the standing position is responsible for the absence of differences in our experiment.
Teleportation has been shown to result in spatial disorientation compared to walking in VR (Cherep et al., 2020; Cherep et al., 2023). Thus, teleportation in VR could result in cognitive costs, whereby these costs can be reduced by the usage of rotational self-motion cues guiding marks, mini-maps, trails, or heatmaps (Kelly et al., 2020; Kraus et al., 2020; Lim et al., 2020). In our study, we used automatic teleportation to avoid inferences due to the usability and unfamiliarity of the teleportation system or hand movements. This could have affected the spatial orientation of our sample in the teleportation condition. However, spatial disorientation in the teleportation condition would probably have resulted in differences between the two conditions regarding the scores in the knowledge quiz, motion sickness, or presence. Instead, the usage of rather small museum rooms might have helped participants in the teleportation condition to keep spatial orientation despite being teleported automatically.
Our results remain unaffected by the hardware utilized for teleportation, as participants were teleported automatically without the use of controllers. Different controllers are used in VR, ranging from those featuring joysticks to others incorporating touchpads (Novacek and Jirina, 2020). This stands in contrast to the evolution of controller prototypes seen in console or PC gaming over decades, where standardized designs have emerged (Maggiorini et al., 2019). For VR, however, controllers show more variation in design, thus resulting in larger differences in usability between different hardware models. Consequently, the method of teleportation in VR using controllers may differ from automatic teleportation and thereby influence learning outcomes (Maraj et al., 2019). Thus, forthcoming studies comparing walking to teleportation should consider the use of controllers for teleportation.
No beneficial effects of walking in VR have been demonstrated in this study. It is by all means possible that there are positive effects of walking in VR which were not revealed in this study. For instance, walking has been shown to have a positive impact on health, including reduced risk for development of chronic diseases, reduced depressive symptoms, and better quality of life (Hanson and Jones, 2015; Lee and Buchner, 2008; Varma et al., 2014). Additionally, previous studies also highlight the beneficial effects of walking in other cognitive areas beyond mere acquisition of knowledge. Oppezzo and Schwartz (2014) observed enhanced creativity both during and after walking which also enhanced the formation of new and more qualitative analogies. As such, even if walking in VR does not enhance the acquisition of the displayed content, it can potentially increase the quality of the respective classroom lesson. Furthermore, the current study exclusively compared the effect of physical walking for knowledge acquisition in VR with teleportation in VR. Other types of physical activity like cycling or running involve a higher level of physical activity than walking (Zabriskie and Heath, 2019). The current findings are limited to walking and further research is needed to investigate how different types of physical activity in VR could affect the acquisition of declarative knowledge.
Finally, it is important to note that it was not the goal of our study to investigate if VR can enhance learning in general. Instead, it aimed at comparing specifically the effects of walking and teleportation in VR on declarative learning outcomes. Since this comparison represented the focus of the current study, we refrained from a pre-test to assess participants’ prior knowledge, which represents a limitation of the present study. A considerable body of research has shown that VR has a medium-large effect on learning outcomes (Villena-Taranilla et al., 2022; Wu et al., 2020; Yu, 2021), for instance for learning anatomy or landscape architecture (Gloy et al., 2022; Wu et al., 2021). Furthermore, immersive VR applications result in more pronounced learning benefits (Villena-Taranilla et al., 2022). Given the growing significance of VR, which is driven by more affordable headsets and wider usage scenarios, the number of educational applications for VR is likely to increase. Research needs to align with this trend, exploring how these applications should be designed to optimize learning in a VR environment.
In summary, our study could not support that walking in VR is beneficial for the acquisition of declarative knowledge compared to teleportation in VR. This does not question the benefits of VR for learning in general. Instead, our findings provide important information for the development of VR-based learning apps. Developers of such learning apps will need to consider whether their app should enable users to walk. In educational contexts, our findings suggest that teleportation can keep up with walking when declarative knowledge has been acquired. In these cases, teleportation in VR represents a promising avenue within future, immersive VR learning environments.
Data availability statement
The datasets for this study is publicly accessible in the open science framework (OSF): doi.org/10.17605/OSF.IO/EC32M.
Ethics statement
The studies involving humans were approved by Ethics committee of the faculty of human sciences of University of Bern. The studies were conducted in accordance with the local legislation and institutional requirements. The participants provided their written informed consent to participate in this study.
Author contributions
MR: Conceptualization, Formal Analysis, Funding acquisition, Project administration, Visualization, Writing–original draft. RS: Methodology, Software, Writing–review and editing. SA: Investigation, Methodology, Software, Writing–review and editing. FM: Conceptualization, Funding acquisition, Supervision, Writing–review and editing. MD: Conceptualization, Funding acquisition, Writing–review and editing.
Funding
The author(s) declare that financial support was received for the research, authorship, and/or publication of this article. This manuscript represents independent research funded by BeLEARN–a competence center for digitalization in education. Open access funding by the University of Bern.
Acknowledgments
A special thanks goes to Pavlos Konstantinidis from the Technology Platform of the Human Sciences Faculty of the University of Bern for developing the experiment in Unreal Engine. Moreover, we also thank Anteo Vicini and Danijela Radovic for their work during data collection.
Conflict of interest
The authors declare that the research was conducted in the absence of any commercial or financial relationships that could be construed as a potential conflict of interest.
Publisher’s note
All claims expressed in this article are solely those of the authors and do not necessarily represent those of their affiliated organizations, or those of the publisher, the editors and the reviewers. Any product that may be evaluated in this article, or claim that may be made by its manufacturer, is not guaranteed or endorsed by the publisher.
References
Bailenson, J. (2018). Experience on demand: what virtual reality is, how it works, and what it can do. W. W. Norton and Company.
Banakou, D., and Slater, M. (2023). A comparison of two methods for moving through a virtual environment: walking in place and interactive redirected walking. Front. Virtual Real. 4, 1294539. doi:10.3389/frvir.2023.1294539
Barsalou, L. W. (1999). Perceptual symbol systems. Behav. Brain Sci. 22, 577–660. doi:10.1017/S0140525X99002149
Biber, D. D., and Heidorn, J. (2021). Tailoring the walking classroom to promote college student engagement. Coll. Teach. 69 (3), 169–172. doi:10.1080/87567555.2020.1833177
Bozgeyikli, E., Raij, A., Katkoori, S., and Dubey, R. (2016). “Point and teleport locomotion technique for virtual reality,” in Proceedings of the 2016 annual symposium on computer-human interaction in play. Editor A. Cox (New York, NY: Association for Computing Machinery), 205–216. doi:10.1145/2967934.2968105
Buttussi, F., and Chittaro, L. (2019). Locomotion in place in virtual reality: a comparative evaluation of joystick, teleport, and leaning. IEEE Trans. Vis. Comput. Graph. 27 (1), 125–136. doi:10.1109/TVCG.2019.2928304
Caputo, A., Zancanaro, M., and Giachetti, A. (2023). “Eyes on teleporting: comparing locomotion techniques in Virtual Reality with respect to presence, sickness and spatial orientation,” in IFIP conference on human-computer interaction (Cham: Springer Nature Switzerland), 547–566. doi:10.1007/978-3-031-42286-7_31
Checa, D., and Bustillo, A. (2020). Advantages and limits of virtual reality in learning processes: briviesca in the fifteenth century. Virtual Real. 24 (1), 151–161. doi:10.1007/s10055-019-00389-7
Cherep, L. A., Kelly, J. W., Miller, A., Lim, A. F., and Gilbert, S. B. (2023). Individual differences in teleporting through virtual environments. J. Exp. Psychol. Appl. 29 (1), 111–123. doi:10.1037/xap0000396
Cherep, L. A., Lim, A. F., Kelly, J. W., Acharya, D., Velasco, A., Bustamante, E., et al. (2020). Spatial cognitive implications of teleporting through virtual environments. J. Exp. Psychol. Appl. 26 (3), 480–492. doi:10.1037/xap0000263
Chim, H. Q., de Groot, R. H. M., Gerven, P. W. M. V., oude Egbrink, M. G. A., Erkens, R. H. J., von Rango, U., et al. (2021). The effects of standing in tutorial group meetings on learning: a randomized controlled trial. Trends Neurosci. Educ. 24, 100156. doi:10.1016/j.tine.2021.100156
Daly-Smith, A. J., Zwolinsky, S., McKenna, J., Tomporowski, P. D., Defeyter, M. A., and Manley, A. (2018). Systematic review of acute physically active learning and classroom movement breaks on children’s physical activity, cognition, academic performance and classroom behaviour: understanding critical design features. BMJ Open Sport and Exerc. Med. 4 (1), e000341. doi:10.1136/bmjsem-2018-000341
Epic Games (2019). Unreal engine. Available at: https://www.unrealengine.com.
Erwin, H., Weight, E., and Harry, M. (2021). “Happy, healthy, and smart”: student responses to the walking classroom education program aimed to enhance physical activity. J. Sch. Health 91 (3), 195–203. doi:10.1111/josh.12990
Faul, F., Erdfelder, E., Lang, A.-G., and Buchner, A. (2007). G*Power 3: a flexible statistical power analysis program for the social, behavioral, and biomedical sciences. Behav. Res. Methods 39, 175–191. doi:10.3758/bf03193146
Frommel, J., Sonntag, S., and Weber, M. (2017). “Effects of controller-based locomotion on player experience in a virtual reality exploration game,” in Proceedings of the 12th international conference on the foundations of digital games, Hyannis Massachusetts, August 14 - 17, 2017, 1–6. doi:10.1145/3102071.3102082
Giangreco, I., Sauter, L., Parian, M. A., Gasser, R., Heller, S., Rossetto, L., et al. (2019). “Virtue: a virtual reality museum experience,” in Proceedings of the 24th International Conference on Intelligent User Interfaces, Marina del Ray California, March 17 - 20, 2019, 119–120. doi:10.1145/3308557.3308706
Glenberg, A. M., and Kaschak, M. P. (2002). Grounding language in action. Psychonomic Bull. and Rev. 9 (3), 558–565. doi:10.3758/BF03196313
Gloy, K., Weyhe, P., Nerenz, E., Kaluschke, M., Uslar, V., Zachmann, G., et al. (2022). Immersive anatomy atlas: learning factual medical knowledge in a virtual reality environment. Anat. Sci. Educ. 15 (2), 360–368. doi:10.1002/ase.2095
Hanson, S., and Jones, A. (2015). Is there evidence that walking groups have health benefits? A systematic review and meta-analysis. Br. J. Sports Med. 49 (11), 710–715. doi:10.1136/bjsports-2014-094157
Hu-Au, E., and Okita, S. (2021). Exploring differences in student learning and behavior between real-life and virtual reality chemistry laboratories. J. Sci. Educ. Technol. 30, 862–876. doi:10.1007/s10956-021-09925-0
Ibánez, M. L., Peinado, F., and Palmieri, O. (2016). “Walking in VR: measuring presence and simulator sickness in first-person virtual reality games,” in Proceedings of the third congress of the Spanish society for video games sciences.
Johnson-Glenberg, M. C., Bartolomea, H., and Kalina, E. (2021). Platform is not destiny: embodied learning effects comparing 2D desktop to 3D virtual reality STEM experiences. J. Comput. Assisted Learn. 37 (5), 1263–1284. doi:10.1111/jcal.12567
Kelly, J. W., Ostrander, A. G., Lim, A. F., Cherep, L. A., and Gilbert, S. B. (2020). Teleporting through virtual environments: effects of path scale and environment scale on spatial updating. IEEE Trans. Vis. Comput. Graph. 26 (5), 1841–1850. doi:10.1109/TVCG.2020.2973051
Kim, H. K., Park, J., Choi, Y., and Choe, M. (2018). Virtual reality sickness questionnaire (VRSQ): motion sickness measurement index in a virtual reality environment. Appl. Ergon. 69, 66–73. doi:10.1016/j.apergo.2017.12.016
Kim, T., and Biocca, F. (1997). Telepresence via television: two dimensions of telepresence may have different connections to memory and persuasion. J. Computer-Mediated Commun. 3 (2), 0. doi:10.1111/j.1083-6101.1997.tb00073.x
Kim, Y. M., and Rhiu, I. (2021). A comparative study of navigation interfaces in virtual reality environments: a mixed-method approach. Appl. Ergon. 96, 103482. doi:10.1016/j.apergo.2021.103482
Kraus, M., Schäfer, H., Meschenmoser, P., Schweitzer, D., Keim, D. A., Sedlmair, M., et al. (2020). “A comparative study of orientation support tools in virtual reality environments with virtual teleportation,” in 2020 IEEE International Symposium on Mixed and Augmented Reality (ISMAR), Porto de Galinhas, Brazil, 09-13 November 2020 (IEEE), 227–238. doi:10.1109/ISMAR50242.2020.00046
Langbehn, E., Lubos, P., and Steinicke, F. (2018). “Evaluation of locomotion techniques for room-scale vr: joystick, teleportation, and redirected walking,” in Proceedings of the Virtual Reality International Conference-Laval Virtual, Laval France, April 4 - 6, 2018, 1–9. doi:10.1145/3234253.3234291
Lee, I. M., and Buchner, D. M. (2008). The importance of walking to public health. Med. and Sci. Sports and Exerc. 40 (7), 512–518. doi:10.1249/mss.0b013e31817c65d0
Lim, A. F., Kelly, J. W., Sepich, N. C., Cherep, L. A., Freed, G. C., and Gilbert, S. B. (2020). “Rotational self-motion cues improve spatial learning when teleporting in virtual environments,” in Proceedings of the 2020 ACM Symposium on Spatial User Interaction, Canada, 30 October 2020- 1 November 2020, 1–7. doi:10.1145/3385959.3418443
Link, T., Moeller, K., Huber, S., Fischer, U., and Nuerk, H. C. (2013). Walk the number line–An embodied training of numerical concepts. Trends Neurosci. Educ. 2 (2), 74–84. doi:10.1016/j.tine.2013.06.005
Lynch, J., O’Donoghue, G., and Peiris, C. L. (2022). Classroom Movement breaks and physically active learning are feasible, reduce sedentary behaviour and fatigue, and May increase focus in University students: a systematic review and Meta-analysis. Int. J. Environ. Res. Public Health 19 (13), 7775. doi:10.3390/ijerph19137775
Maggiorini, D., Granato, M., Ripamonti, L. A., Marras, M., and Gadia, D. (2019). “Evolution of game controllers: toward the support of gamers with physical disabilities,” in Computer-human interaction research and applications: first international conference, CHIRA 2017, funchal, madeira, Portugal, october 31–november 2, 2017, revised selected papers. Editors A. Holzinger, H. P. Silva, and M. Helfert (Springer International Publishing), 66–89. doi:10.1007/978-3-030-32965-5_4
Maraj, C., Hurter, J., Ferrante, S., Horde, L., Carter, J., and Murphy, S. (2019). “Oculus rift versus HTC vive: usability assessment from a teleportation task,” in Virtual, augmented and mixed reality. Multimodal interaction: 11th international conference, VAMR 2019, held as part of the 21st HCI international conference, HCII 2019, orlando, FL, USA, july 26–31, 2019, proceedings, Part I. Editors J. Y. C. Chen, and G. Fragomeni (Springer International Publishing), 247–257. doi:10.1007/978-3-030-21607-8_19
Markowitz, D. M., Laha, R., Perone, B. P., Pea, R. D., and Bailenson, J. N. (2018). Immersive virtual reality field trips facilitate learning about climate change. Front. Psychol. 9, 2364. doi:10.3389/fpsyg.2018.02364
Mehta, R. K., Shortz, A. E., and Benden, M. E. (2016). Standing up for learning: a pilot investigation on the neurocognitive benefits of stand-biased school desks. Int. J. Environ. Res. Public Health 13 (1), 59. doi:10.3390/ijerph13010059
Moreno, R., and Mayer, R. E. (2002). Learning science in virtual reality multimedia environments: role of methods and media. J. Educ. Psychol. 94 (3), 598–610. doi:10.1037/0022-0663.94.3.598
Novacek, T., and Jirina, M. (2020). Overview of controllers of user interface for virtual reality. PRESENCE Virtual Augmented Real. 29, 37–90. doi:10.1162/pres_a_00356
Oppezzo, M., and Schwartz, D. L. (2014). Give your ideas some legs: the positive effect of walking on creative thinking. J. Exp. Psychol. Learn. Mem. Cognition 40 (4), 1142–1152. doi:10.1037/a0036577
Posit Team (2023). RStudio: integrated development environment for R. Posit software. Boston, MA: PBC. Available at: http://www.posit.co.
Queiroz, A. C., Fauville, G., Abeles, A. T., Levett, A., and Bailenson, J. N. (2023). The efficacy of virtual reality in climate change education increases with amount of body movement and message specificity. Sustainability 15 (7), 5814. doi:10.3390/su15075814
Rasberry, C. N., Lee, S. M., Robin, L., Laris, B. A., Russell, L. A., Coyle, K. K., et al. (2011). The association between school-based physical activity, including physical education, and academic performance: a systematic review of the literature. Prev. Med. 52, S10–S20. doi:10.1016/j.ypmed.2011.01.027
R Core Team (2023). R: a language and environment for statistical computing. Vienna, Austria: R Foundation for Statistical Computing. Available at: https://www.R-project.org/.
Riecke, B. E., Bodenheimer, B., McNamara, T. P., Williams, B., Peng, P., and Feuereissen, D. (2010). “Do we need to walk for effective virtual reality navigation? physical rotations alone may suffice,” in International conference on spatial cognition (Berlin, Heidelberg: Springer Berlin Heidelberg), 234–247.
Saredakis, D., Szpak, A., Birckhead, B., Keage, H. A., Rizzo, A., and Loetscher, T. (2020). Factors associated with virtual reality sickness in head-mounted displays: a systematic review and meta-analysis. Front. Hum. Neurosci. 14, 96. doi:10.3389/fnhum.2020.00096
Sayyad, E., Sra, M., and Höllerer, T. (2020). “Walking and teleportation in wide-area virtual reality experiences,” in 2020 IEEE international symposium on mixed and augmented reality (ISMAR), Porto de Galinhas, Brazil, 9-13 November 2020 (IEEE), 608–617. doi:10.1109/ISMAR50242.2020.00088
Shavelson, R. J., Ruiz-Primo, M. A., and Wiley, E. W. (2005). Windows into the mind. High. Educ. 49 (4), 413–430. doi:10.1007/s10734-004-9448-9
Shewaga, R., Uribe-Quevedo, A., Kapralos, B., and Alam, F. (2017). A comparison of seated and room-scale virtual reality in a serious game for epidural preparation. IEEE Trans. Emerg. Top. Comput. 8 (1), 218–232. doi:10.1109/tetc.2017.2746085
Skulmowski, A., and Rey, G. D. (2018). Embodied learning: introducing a taxonomy based on bodily engagement and task integration. Cognitive Res. Princ. Implic. 3, 6–10. doi:10.1186/s41235-018-0092-9
Slater, M., Usoh, M., and Steed, A. (1995). Taking steps: the influence of a walking technique on presence in virtual reality. ACM Trans. Computer-Human Interact. (TOCHI) 2 (3), 201–219. doi:10.1145/210079.210084
The jamovi project (2024). Jamovi (Version 2.5). Available at: https://www.jamovi.org.
Thoma, S. P., Hartmann, M., Christen, J., Mayer, B., Mast, F. W., and Weibel, D. (2023). Increasing awareness of climate change with immersive virtual reality. Front. Virtual Real. 4, 897034. doi:10.3389/frvir.2023.897034
Usoh, M., Arthur, K., Whitton, M. C., Bastos, R., Steed, A., Slater, M., et al. (1999). “Walking> walking-in-place> flying, in virtual environments,” in Proceedings of the 26th annual conference on Computer graphics and interactive techniques (New York: ACM Press), 359–364. doi:10.1145/311535.311589
Varma, V. R., Tan, E. J., Wang, T., Xue, Q. L., Fried, L. P., Seplaki, C. L., et al. (2014). Low-intensity walking activity is associated with better health. J. Appl. Gerontology 33 (7), 870–887. doi:10.1177/0733464813512896
Villena-Taranilla, R., Tirado-Olivares, S., Cozar-Gutierrez, R., and González-Calero, J. A. (2022). Effects of virtual reality on learning outcomes in K-6 education: a meta-analysis. Educ. Res. Rev. 35, 100434. doi:10.1016/j.edurev.2022.100434
Webster, R. (2016). Declarative knowledge acquisition in immersive virtual learning environments. Interact. Learn. Environ. 24 (6), 1319–1333. doi:10.1080/10494820.2014.994533
Weibel, D., Schmutz, J., Pahud, O., and Wissmath, B. (2015). Measuring spatial presence: introducing and validating the pictorial presence SAM. Presence Teleoperators Virtual Environ. 24 (1), 44–61. doi:10.1162/PRES_a_00214
Weight, E. A., Harry, M., and Erwin, H. (2021). The walking classroom: measuring the impact of physical activity on student cognitive performance and mood. J. Phys. Activity Health 18 (7), 818–825. doi:10.1123/jpah.2020-0263
Wilson, N. L., and Gibbs, R. W. (2007). Real and imagined body movement primes metaphor comprehension. Cognitive Sci. 31 (4), 721–731. doi:10.1080/15326900701399962
Wretman, C. J. (2017). School sports participation and academic achievement in middle and high school. J. Soc. Soc. Work Res. 8 (3), 399–420. doi:10.1086/693117
Wu, B., Yu, X., and Gu, X. (2020). Effectiveness of immersive virtual reality using head-mounted displays on learning performance: a meta-analysis. Br. J. Educ. Technol. 51 (6), 1991–2005. doi:10.1111/bjet.13023
Wu, W. L., Hsu, Y., Yang, Q. F., and Chen, J. J. (2021). A spherical video-based immersive virtual reality learning system to support landscape architecture students’ learning performance during the COVID-19 era. Land 10 (6), 561. doi:10.3390/land10060561
Yu, Z. (2021). A meta-analysis of the effect of virtual reality technology use in education. Interact. Learn. Environ. 31 (8), 4956–4976. doi:10.1080/10494820.2021.1989466
Keywords: walking, teleportation, learning, education, physical activity, knowledge acquisition, immersion
Citation: Rihs M, Steuri RA, Aeschlimann SA, Mast FW and Dobricki M (2024) Comparison of teleportation and walking in virtual reality in a declarative learning task. Front. Virtual Real. 5:1423911. doi: 10.3389/frvir.2024.1423911
Received: 26 April 2024; Accepted: 29 August 2024;
Published: 08 November 2024.
Edited by:
Erwan David, Le Mans Université, FranceReviewed by:
Julian Keil, Ruhr University Bochum, GermanyMina C. Johnson-Glenberg, Arizona State University, United States
Estelle Michinov, University of Rennes 2 – Upper Brittany, France
Inas Redjem, EA1285 Laboratoire de Psychologie, Cognition, Comportement, Communication (LP3C), France, in collaboration with reviewer EM
Copyright © 2024 Rihs, Steuri, Aeschlimann, Mast and Dobricki. This is an open-access article distributed under the terms of the Creative Commons Attribution License (CC BY). The use, distribution or reproduction in other forums is permitted, provided the original author(s) and the copyright owner(s) are credited and that the original publication in this journal is cited, in accordance with accepted academic practice. No use, distribution or reproduction is permitted which does not comply with these terms.
*Correspondence: Michael Rihs, bWljaGFlbC5yaWhzQHVuaWJlLmNo