- 1Department of Psychology, SUNY Old Westbury, Old Westbury, NY, United States
- 2SUNY Neuroscience Research Institute, SUNY Old Westbury, Old Westbury, NY, United States
- 3Department of Neurosurgery, CHRU Gui de Chauliac Hospital, Montpellier University Medical Center, Montpellier, France
- 4Institute of Functional Genomics, University of Montpellier, CNRS, INSERM, Montpellier, France
- 5Department of Neurosurgery, CHU Toulouse, Toulouse University Medical Center, Toulouse, France
- 6University of Montpellier / INSERM-MMDN, Montpellier, France
- 7Education Science School, LIRDEF, Montpellier University, Montpellier, France
The use of virtual reality (VR) training in medical school education has increased over the last decade partly due to coronavirus-19 (COVID-19) and due to technology advancements in this integrative area. As medical students are being trained in new pedagogical interventions, it is important to drill-down and focus on the areas of most translational concern for assessing their competency and proficiency. Thus, the present pilot study sought to evaluate a group of fourth-year medical students’ ability to learn a modified medical school curriculum on implementing a subdermal drain by first learning how to stitch on a silicone model (i.e., proper stitching only) and then being randomly assigned to either a third-person point-of-view (TP-POV) or first-person point-of-view (FP-POV) immersive VR group to learn how to conduct the surgical procedure (i.e., conceptually and procedurally). Then, the participants’ surgical procedural motor skills were assessed on a single attempt to demonstrate their competency and proficiency on a cadaver. The results showed that the pedagogical learning differences in FP-POV immersive VR improved the cleanliness and accuracy of the surgical dissection movement and drain placement with 100% proficiency in the first attempt at implementing this surgical technique on a cadaver. Additionally, there was statistically significant improvement in the dissection movement (p < 0.05*) and the tension test (p < 0.01**) in the FP-POV group compared to the TP-POV group, even with a small sample size in the present pilot study. These findings offer unique training opportunities to be considered when designing future VR curriculum with the suggested use of repeated-measures designs on the surgical procedural motor skills to assess learning curves and their potential translations in reducing operating room errors, costs, planning and scheduling times, and surgery overlaps, thereby positively contributing to a more efficient and safer OR surgical experience by staff and patients in the near future.
1 Introduction
The continued interest, advancement, and integration of virtual reality (VR) in the United States’ secondary and post-secondary educational training of both science, technology, engineering, and mathematics (STEM) and non-STEM majors have been increasing rapidly over the last decade (Qorbani et al., 2021; Christopoulos et al., 2020; Pellas et al., 2021; Pellas et al., 2020; Zhao et al., 2020a; Concannon et al., 2019; Nersesian et al., 2019), and this trend is also emerging world-wide as VR global healthcare education (Almousa et al., 2021; Barteit et al., 2021; Javaid and Haleem, 2020; Pottle, 2019). Some of these curricular changes directed toward more VR pedagogy may have been motivated by the shift in learning due to the coronavirus-19 (COVID-19) pandemic (Mehraeen et al., 2023; De Ponti et al., 2020; Neuwirth et al., 2021a). Moreover, the production of sophisticated, refined, and more sensitive visualization through VR has created technology that has arguably seen the fastest turnover in production (i.e., VR-e-waste) than smartphone technologies (Ashtari et al., 2020). Furthermore, this sequential educational interest coupled with the high demand of VR technology generally over the last decade has lent itself to swiftly evolve into a specialized high-demand field for students to anticipate the availability of and intentionally seek out VR educational learning applications and solutions when they enroll in the undergraduate- and graduate-level degree programs (Marks and Thomas, 2022; Botha et al., 2021; Reeves et al., 2021; Ismail and Hashim, 2020). This varied evolution of VR-educational demand situation presents with some interesting avenues for opportunities where more procedural knowledge can be offered within the curriculum at an accelerated rate (Rogers et al., 2021) that may further enhance undergraduate- and graduate-level educational courses (Jiang et al., 2022; Sultan et al., 2019) to upskill students specializing in the medical fields through their early career training, more so than the prior years. This need to upskill future generations of students is especially true for students that come from underrepresented and historically bypassed backgrounds in order to create a more inclusive and diverse global workforce (Neuwirth et al., 2021; Bayline et al., 2020; Neuwirth et al., 2018a; Neuwirth et al., 2018b; Mukherji et al., 2017).
Utilizing this premise, much VR work has been done on cadaver models for teaching both anatomy (Miltykh et al., 2023; Koucheki et al., 2023; Chen et al., 2020; Kolla et al., 2020) and a range of surgical procedures, such as mastoidectomy (Frendø et al., 2020), acetabulum reaming (Pelliccia et al., 2020), and cochlear implants (Frendø et al., 2020). The use of VR in medical education is becoming increasingly broad, yet only a few technologies have built a solid track-record for first-person point-of-view (FP-POV) VR educational experiences (for review, see Hatt et al., 2023; Tursø-Finnich et al., 2023) with validated learning gains within a range of educational contexts that permits the user to immerse themselves into the simulation that utilizes the mirror neuron system to reinforce self-perception of procedural motor skill learning (Fan and Luo, 2022; Choi et al., 2020). Moreover, there is yet another problem that arises when considering VR in training medical students beyond proficiency, which directly relates to the quality of the procedures they implement, the consistency of the procedure being conducted from one patient to another, and the reduced time and efficiency of the procedure being done accurately. All of which are characteristics that, when done improperly, will result in increased healthcare costs (i.e., both indirect and direct) that are related to the availability of proper surgical instruments, searching for procedure-matched instruments, errors using them, or improper functionality (Nichol and Saari, 2023; Lonner et al., 2021; Stockert and Langerman, 2014); surgical technique errors (Cima and Deschamps, 2013; Weller et al., 2015; Bharathan et al., 2013; Catchpole et al., 2008); efficiency, quality, and safety in the operating room (OR); and surgical planning, scheduling, and communication costs amongst the team (Dreyfus et al., 2020; Lee et al., 2019; Ugur et al., 2016; Wu and Aufses, 2012; Macario, 2006). What becomes evident is the need for not just medical school educational programs to increase their use of VR learning and pedagogical modalities in the modern day, but furthermore, for medical programs to become more intentional in evaluating whether distinct areas of the curriculum in which they teach such surgical techniques would be most likely to cause costly surgical errors by future surgeons. Thus, adding ways to the VR curriculum in which to target and assess the procedural quality and accuracy of the surgical techniques being trained may serve to best move the integrative medical and VR educational fields forward. Such a cogent educational model would potentially shift just learning procedures in VR to also trying to validate the areas of difficulty in which VR increases better learning outcomes and how well that learned VR experience might translate into the OR when implementing a learned surgical procedural skillset. In order to assess this theory, the present study evaluated fourth-year medical students’ surgical procedural motor skills using the Revinax Handbook® VR (i.e., hereon referred to as the VR software/application) method when compared to traditional video lecture learning during the medical school surgical curriculum and assessed how these VR vs. traditional pedagogical instructions on a surgical technique influenced the translation of their learned skills on implementing the surgical technique on a cadaver.
The VR software/application method when compared to traditional video lecture learning during the medical school surgical method is one such VR platform that has consistently showed and validated learning results using their FP-POV 180° stereoscopic recordings for VR experiences (Ros and Trives, 2023; Ros and Neuwirth, 2020; Ros et al., 2020a; Ros et al., 2020b; Ros et al., 2017), which have been applied broadly across a range of (post-)secondary training contexts for undergraduate neuroscience techniques in stereotaxic surgery (Neuwirth and Ros, 2021), dental education (Maret et al., 2022), medical education for lumbar punctures (Ros et al., 2021), external ventricular drainage (Ros et al., 2020a), and timely COVID-19 healthcare guidelines during the peak of the pandemic (Ros and Neuwirth, 2020). The literature is beginning to formulate several different frameworks by which VR can be used to further develop medical student procedural motor skill training, and the VR software/application method when compared to traditional video lecture learning during the medical school surgical method has demonstrated its versatility across several contexts with improved student learning outcomes. Building upon this pedagogical framework for utilizing VR in medical education, Revinax has put forth 11 principles aimed at helping educators and researchers adopt a method and medical devices, which are as follows: 1) enhancing the innovative dimension; 2) facilitating the discovery phase; 3) an alternative or supplement to in-person learning; 4) more easily showing all the benefits of the new device; 5) simulation of a real-life experience is one of the most efficient methods for learning and memorization; 6) VR technologies have a greater sensory and emotional impact than traditional teaching techniques; 7) subjective FP learning makes immersion and retention even better; 8) a faster learning curve and fewer errors; 9) the tutorials reduce the need for in-person training; 10) the tutorials can, by nature, be reused as many times as necessary; and 11) the tutorials are available at any time and under any circumstances (Revinax, 2021). In order to further build upon this emerging literature, the present pilot study sought to evaluate the use of the VR software/application FP-POV on its ability to influence the learning acquisition of medical students’ procedural motor skills conducting the surgical approaches to make incisions, dissect, and conduct an aponeurotomy (i.e., cutting the outermost skin layer to move the muscle back and inserting an outlet drainage tube through the skin from the surgical site) on a cadaver and a skin model. This surgical technique was chosen as it is frequently the most basic procedure that a student could do in the operating room to assist a surgeon.
2 Methods
2.1 Participants
The present study was funded by an educational grant intended to pilot improving curricular development of medical student course modules through the University of Montpellier (Montpellier, France). The pilot study was conducted on a subset of (N = 24) fourth-year medical students that were undergoing surgical training techniques in their curriculum modules. The study was approved by the Neurosurgical Society’s Institutional Review Board (IRB) consistent with other studies (Ros et al., 2021) and the University of Montpellier to conduct the pedagogical intervention on medical students. The participants consented to voluntarily take part in the pilot study to assess whether FP-POV VR provided any pedagogical and learning outcome advantages on increasing the proficiency of their procedural motor skills during their surgical training module during their medical school curriculum. The participants were randomly selected to participate in the pilot study from the fourth-year cohort that year and were, subsequently, again randomized to be assigned to a traditional video lecture condition or a FP-POV VR non-traditional learning condition using the Revinax Handbook®.
2.2 Design and procedures
The (N = 24) participants from both groups during their medical curriculum were instructed to learn several different types of suturing techniques (e.g., how to make a clean incision and dissect through the skin layers until performing an aponeurotomy, how to correctly place the needle through the skin, how to properly use a surgical needle holder and make the drainage outlet, how to make correct surgical stitching knots, and to practice stitching on a silicon model (i.e., superficial stitching on the skin, that were not deep nor subcutaneous knots)). In the medical school pedagogical curriculum, students first learn to conduct these surgical techniques on a silicon skin model (i.e., proper stitching only) and then, once that surgical procedural skill proficiency is achieved, they are then able to practice these techniques on a cadaver to exhibit proper translations of the conceptual and procedural knowledge, prior to being able to assist a surgeon in the operating room (OR) on live patients. Next, the (N = 24) participants were all exposed to an immersive tutorial in VR (i.e., which covered an entirely different medical procedure—lumbar puncture; Ros et al., 2021) to ensure everyone had at least tried and was familiar with engaging in a VR experience using the Revinax Handbook®. This allowed the researchers to control for a proper VR experience-dependent baseline amongst all participants and control for psychological-dependent and emotional-dependent anticipation and expectation factors as potential confounding variables if the participants were assigned to only the video or the VR group and lacked such an experience (i.e., potential disappointment that might negatively skew learning and performance outcomes when assessed, following the pedagogical intervention). During this immersive tutorial in VR, the participants were shown how to navigate through and use the Revinax Handbook® to proficiently understand the pedagogical alternative learning environment in VR.
The participants were then subsequently randomized into two groups: 1) third-person point-of-view (TP-POV; n = 12) using a video displayed on a screen and 2) first-person point-of-view (FP-POV; n = 12) using 180° stereoscopic VR through an Oculus Go Device—standalone VR headset with controller—64 GB (Meta Quest; Menlo Park, CA). Following the TP-POV (i.e., control condition) and the FP-POV (i.e., experimental condition) educational interventions, all participants then went to the surgical anatomy laboratory, where they were instructed to reproduce the procedural motor skills they had just learned on a cadaver to simulate assisting a surgeon in the OR. They were then evaluated for their proficiencies in completing the procedural surgical skills correctly and cleanly across four different measures: 1) the procedural motor skills, 2) the accuracy of the spatial repairing, 3) the duration of the procedure, and 4) the quality of their work. An illustration of the research design flow chart is provided in Figure 1. Unfortunately, in the middle of the procedural motor skill testing on the cadaver, a participant had a family emergency and had to leave. This resulted in the FP-POV group’s n-size being reduced to (n = 11), which was beyond our control.
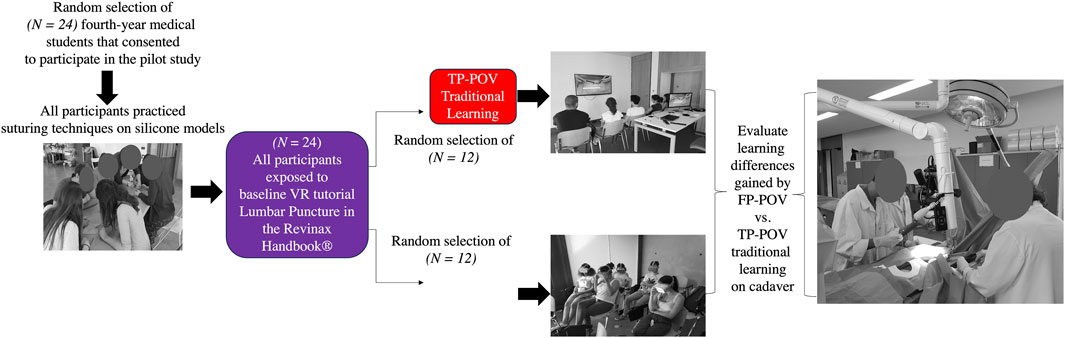
Figure 1. Research design as a flow chart. Twenty-four randomly selected fourth-year medical students consented to participate in the pilot study. All participants were first instructed how to perform several suturing techniques in their medical curriculum and then were trained and shown how to use the VR experience using the Revinax Handbook®. Then, participants were randomly assigned to the third-person point-of-view (TP-POV; n = 12) traditional learning from a video screen (control group) or the first-person point-of-view (FP-POV; n = 12) immersive VR (experimental group). Immediately following the pedagogical intervention of learning the surgical techniques, all participants were brought to the surgical anatomy laboratory to be evaluated on their procedural motor skill knowledge just learned, conducting the same procedure on a cadaver to simulate assisting a surgeon in the OR. The scoring for evaluating the participants’ surgical procedural skill proficiencies was across four areas, namely, 1) incision, 2) dissection, 3) drain, and 4) closing, which are outlined with their individualized components in the Appendix.
2.3 Materials
The participants were shown how to suture using standardized silicone skin models that are commercially available with consumable needles, needle holders, drainage and suction devices, and thread. The VR software/application experience and Oculus Go headsets were provided at no cost to the participants to learn with the tutorial and engage in the FP-POV experience for the randomly assigned participants. The participants in the TP-POV non-traditional learning condition were shown the video-captured surgical training procedure during a standard lecture, and then, the participants were assessed for their comprehension practicing the learned surgical motor skills on a cadaver. The TP-POV participants were not able to watch again or stop the video in their condition during the experimental run (i.e., to ensure methodologically that both groups were presented with the same duration of viewing the material), but could do so afterward. For the FP-POV VR non-traditional learning condition, the same surgical techniques that were shown in the video traditional lecture condition were newly recorded on a cadaver to create the VR software/application with an interactive experience which corresponded to the first steps that a medical student could actively participate in the OR to assist a skilled surgeon. The FP-POV and the TP-POV groups were afforded the same learning activities as the recordings covered the following surgical approaches: incision, dissection, installation of the Redon drainage outlet and its proper fixation, and closure of the skin.
The surgical technique was recorded from two different points-of-view (POVs) to ensure proper visualization of the behavioral procedural components for learning the surgical skill and completing the technique with proper mastery. The usual POV typically found when reviewing operative videos is conducted using a camera placed in the OR light above the head of the surgeon. To proactively prevent any unforeseen viewing obstacles from this OR light camera, an additional camera was positioned to record from the opposite POV angle of the OR light. Concurrent with these recordings, the surgeon was wearing a 180° stereoscopic camera rig to record their FP-POV experience (for review, see Ros and Trives, 2023) to be embedded in the VR software/application consistent with prior studies (Maret et al., 2022; Ros and Neuwirth, 2020; Ros et al., 2021; Neuwirth and Ros, 2021; Ros, 2021; Ros et al., 2020a; Ros et al., 2020b; Ros and Neuwirth, 2020; Ros et al., 2017). The FP-POV display and TP-POV were then synchronized and edited. The FP-POV had an extra content layer to create the specific VR software/experience for the medical students. Accordingly, FP-POV was subsequently broken down into short chapter learning modules covering specific procedural motor skill acquisition steps covering the surgical technique: incision, dissection, drain, and closure (Figure 2). The recordings were then used to create an immersive tutorial learning experience in FP-POV VR from the recorded procedures in the OR as a movie that could be walked through at the pace of the learner with the ability to go back to prior steps of the surgical technique. In contrast, the TP-POV participants following the experimental run had the opportunity to set their own pace with the ability to go back to prior steps of the surgical technique, but it was more difficult to experimentally control and was not done on an individual basis, given the cohort of medical students that participated in the pilot study. Moreover, the 180° stereoscopic FP-POV VR was displayed on a central screen, whereas the chapters could be selected by the learner at top of the VR environment (Figure 2).
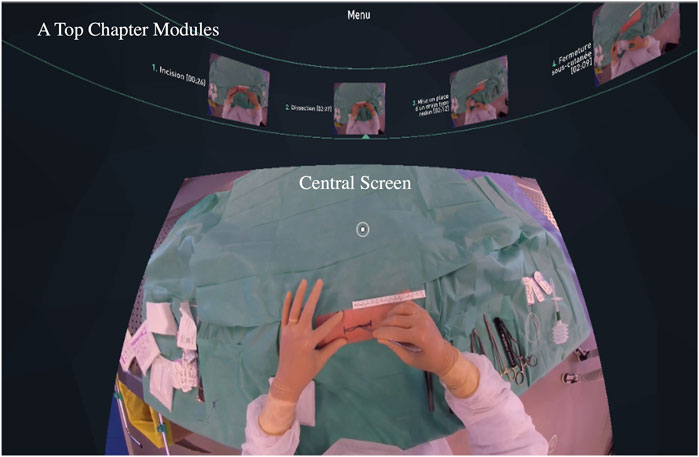
Figure 2. Screenshot of the first-person point-of-view (FP-POV) within the immersive virtual reality (VR) headset. The middle of the central screen, when looking down, permits the participant to review the recorded movie of the step-by-step surgical procedure (i.e., in this case an incision). At the top of the screen are the different chapter modules (i.e., shown in French) for the participant to move sequentially through the entire procedure or to go back to revisit an aspect of the procedure.
2.4 Statistical analyses
As this was a pilot study to evaluate the potential of using and incorporating more FP-POV immersive VR in the medical student training curriculum at the University of Montpellier, the statistical analyses solely focused on the educational outcome scores assessed on the surgical procedural motor skills that the participants exhibited on the cadavers post the educational intervention (i.e., TP-POV vs. FP-POV), absent of any other demographics. The dependent variables measured were the correct implementation of the procedure (i.e., % correct) for the overall four-chapter module areas in the curriculum, the within-chapter module areas, and the duration required to complete the procedural skills on the cadaver, following the educational intervention. The assessments were conducted by an independent rater blind to each of the educational intervention conditions to prevent any scoring biases. Each scored point of the assessment tool was converted into the % correct by dividing the points scored over the total maximal points and multiplying by 100 for both the overall curricular areas and the individual curricular components. The duration of the surgical technique was converted into seconds to draw more refined comparisons. The statistics were conducted using a traditional between-subjects design. To assess the normality of the participant sample distribution, a Levene’s test was used to assess the equality of variances. This was followed by an independent samples t-test and a Hedge’s G correction, given the smaller sample size (< n = 20) for determining the effect size when appropriate to evaluate the differences in learning the procedural motor skills between the two groups. All statistical analyses were conducted using SPSS version 24 (IBM®: Armonk, New York, United States). The criteria for statistical significance was set at α = ≤ 0.05 with a confidence interval of 95% (CI = 95%). Data are presented visually as bar and line graphs ± standard error of the means (SEM) and in table form for reporting full statistical analyses.
3 Results
In order to assess whether the FP-POV VR influenced the participants procedural motor skill performances when implementing the surgical technique on the cadaver over the traditional TP-POV video lecture, first, the overall four curricular areas in which the procedural motor skills were evaluated were compared (i.e., incision, dissection, drain, and closing). Figure 3 shows that across all four curricular areas, the FP-POV participants showed similar performance as the TP-POV participants on their demonstrated procedural motor skills, with a statistically emerging trend that the installation of the drain might have reached significance in the FP-POV group with a larger sample size. Otherwise, the participants performed similarly between these two educational interventions across each curricular chapter module area. Next, each of the four curricular areas were further evaluated for their respective individualized components of the learning chapter modules to see if the FP-POV experience influenced a specific procedural motor skillset when learning the surgical technique and the participants’ ability to exhibit better surgical motor skill acquisition.
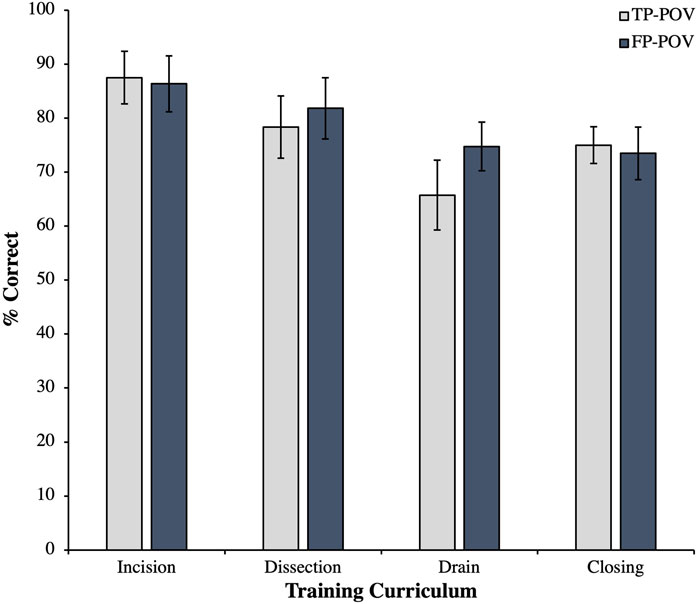
Figure 3. Participant’s percent correct surgical procedural skill proficiencies on the cadaver based upon the four evaluative areas in the curriculum: 1) incision, 2) dissection, 3) drain, and 4) closing between the third-person point-of-view (TP-POV) and the first-person point-of-view (FP-POV). The data show that FP-POV and the TP-POV produce similar procedural motor skill proficiencies as learning outcomes. However, despite the small sample size, there was an emerging trend observed approaching significance for the drain area of the curriculum, potentially favoring FP-POV over TP-POV if a larger sample size (n = 24 per group) was obtained. Data are presented as the mean ± SEM.
Table 1 shows the statistical findings from all the overall four surgical curricular areas broken down by their individual chapter module components to assess the procedural motor skills learned. Across all the areas, there were significant improvements in FP-POV over that of TP-POV only in dissection when assessing using dissection movements (Levene’s test F(21) = 30.130, p = 0.001***; t(11) = −1.915, p = 0.041*; Hedges G = −0.736) and in drain when assessing using the tension test (Levene’s test F(21) = 1.881, p = 0.185 n/s; t(21) = −2.295, p = 0.016**; Hedges G = −0.736) (Figure 4A). Remarkably, there was 100% correct responding with corroborating results in the incision area for the straight incision line and in the drain area for the number of stitches used between both groups (Figure 4A; denoted with [‡] as there was no standard deviation between either group to conduct a proper independent samples t-test as both groups performed identically).
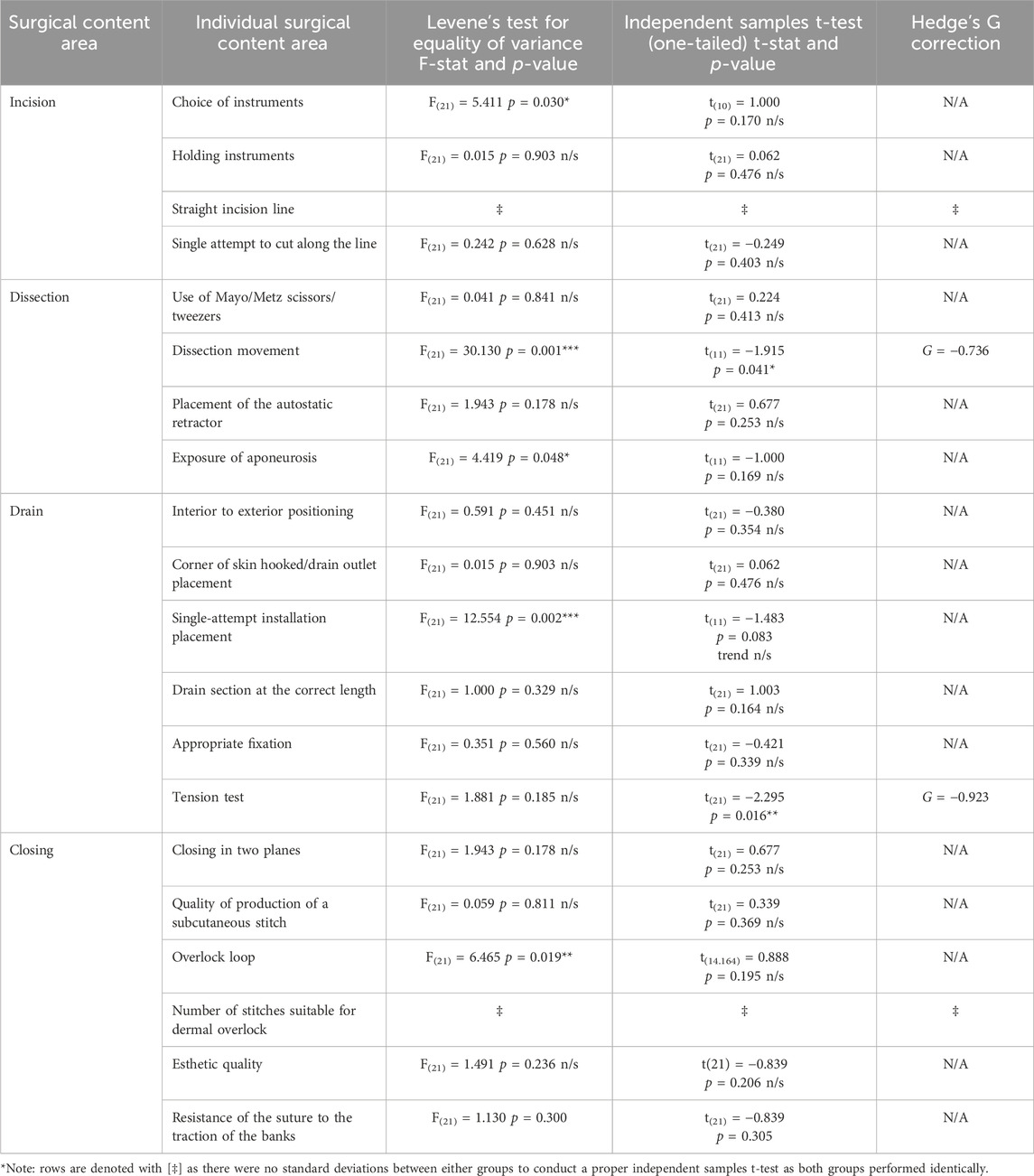
Table 1. Statistical analyses of the participant’s surgical procedural skill proficiencies on the cadaver.
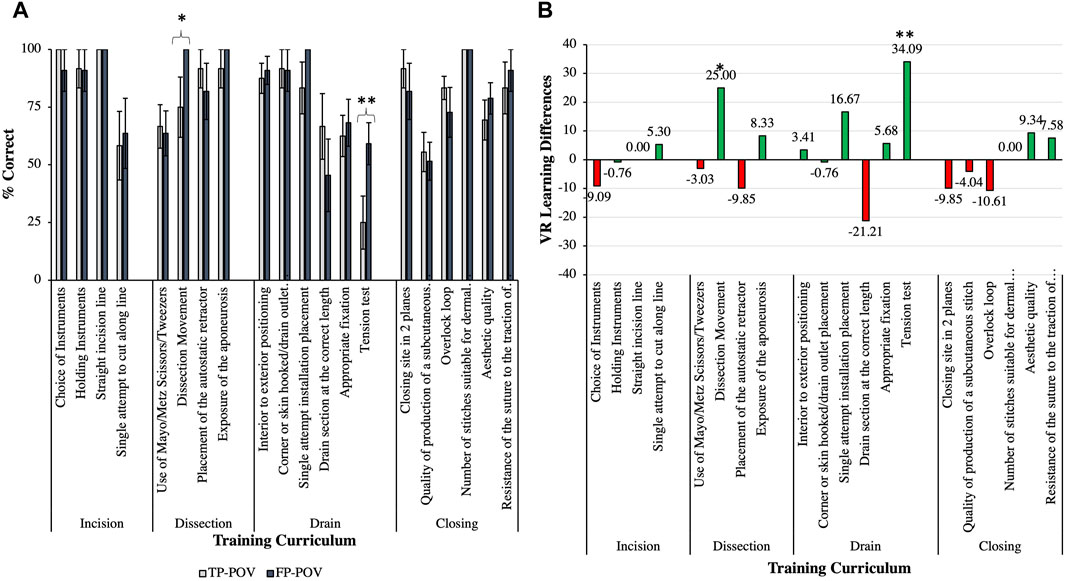
Figure 4. Individual curriculum components that were evaluated for assessing the proficiency of the surgical procedural motor skills that were demonstrated on the cadavers from the third-person point-of-view (TP-POV) and the first-person point-of-view (FP-POV) groups (A) and the virtual reality (VR) percent learning differences showing the influence of VR on the curriculum (B). The data show that in the dissection chapter module, the FP-POV group had a perfect dissection movement (p = 0.041*), (A, B) and that in the drain chapter module, a 34% greater proficiency (p = 0.016**) (A, B) was seen when compared to TP-POV on the tension test. The green bars indicate VR-induced learning enhancements, whereas the red bars indicate VR-induced learning deficits. Data are presented as the mean ± SEM with p < 0.05 * and p < 0.01** symbolic notations.
Figure 4B illustrates the % difference in VR learning when compared to TP-POV, whereby the green bars show the increased learning proficiency by way of VR and the red bars show the decreased learning proficiency when using FP-POV VR, with only the dissection movement (25% increase in learning in VR) and the tension test (i.e., 34.09% increase in learning in VR) reaching significance. Interestingly, there was no statistically significant difference in the duration to complete the surgical procedure on the cadaver accurately between the FP-POV and the TP-POV groups (i.e., ∼33 min), respectively. Finally, the efficiency by which the participants completed the surgical procedural motor skills on the cadavers was assessed between both groups. The data revealed that there was no significant difference in the duration (seconds) to complete the surgical procedure on the cadaver between the TP-POV and the FP-POV groups, respectively (Levene’s test F(21) = 0.122, p = 0.730 n/s; t(21) = −0.191, p = 0.425 n/s). Thus, each group was able to complete the surgical techniques in approximately 33 min with the same amount of accuracy, regardless of the intervention used.
4 Discussion
The pilot study sought to assess a very basic surgical procedure (i.e., an aponeurotomy by cutting the outermost skin layer to move the muscle back and inserting an outlet drainage tube through the skin from the surgical site) that medical students are required to learn during their surgical curriculum and whether learning through TP-POV (i.e., traditional video lecture) would produce different learning outcomes from learning through FP-POV (i.e., non-traditional immersive VR learning). The results showed that both TP-POV and FP-POV groups completed the surgical procedure motor skills on the cadaver within approximately ∼33 min duration, with no statistical differences observed between each group. Despite the groups being similar, the distribution for the FP-POV group duration to complete the surgical technique had more participants that completed the procedure 2 mins faster. Whether this finding would be sustained in a larger sample-size and whether it would be reproduced (i.e., as estimated with a sample size of n = 24 per each group using G*Power; Kang, 2021) remain to be elucidated. Previous studies indicated the benefits of a surgical efficiency savings duration, which may contribute to the surgical OR efficiency, planning, and scheduling concerns that remain in the field (Dreyfus et al., 2020; Lee et al., 2019; Ugur et al., 2016; Wu and Aufses, 2012; Macario, 2006) to move the surgeon on to other patients in need sooner. Whether the findings from the present pilot study corroborate with the surgical efficiency warrants further investigation. The present data show emerging trends of time savings in the FP-POV immersive VR group on the surgical procedure, which, even as a pilot study, appear to be consistent with the findings observed on learning acquisition of the lumbar puncture surgical procedural motor skills using the VR software/application (Ros et al., 2021), in which the FP-POV VR intervention resulted in a faster lumbar puncture. However, a larger sample size is required to confirm and validate these early trends and observations, along with a replicability study to be certain.
Furthermore, it is important to note that both the TP-POV and the FP-POV groups were able to complete the four surgical chapter modules (i.e., incision, dissection, drain, and closing) with nearly identical proficiency (Figure 3). The following proficiencies were demonstrated by the participants after completing the surgical procedure on the cadaver as a first attempt at practicing the required motor skills: incision (∼87% accuracy), dissection (∼79.5% accuracy), drain (69.5% accuracy), and closing (∼74% accuracy). Thus, despite the similar performances in proficiency, there was still room for motor skill improvement between both groups in the overall scoring of the surgical procedural techniques demonstrated by the participants. Thus, it is conceivable that the participants may have benefitted from a repeated-measures assessment/evaluation design in implementing the surgical procedural motor skills on the cadavers to fully assess a learning or proficiency curve to fully evaluate the degree by which FP-POV accelerates learning in this case, as shown and reported in the literature. However, the literature is scant and is lacking examples on repeated-measures design. Perhaps, this may pose a unique opportunity for the FP-POV VR literature to begin exploring ways to build more concrete educational and pedagogical assessment tools to truly capture these differences in learning performances with additional memory retention probes than the typical isolated single assessment of the contrived skill when performed.
In order to further investigate the emerging trend in the overall proficiency difference in the drain area of the curriculum, a more scrutinized analysis was conducted on the individual curricular components of the assessment scoring tool used to evaluate the participants' surgical procedural motor skills used on the cadaver. It was observed that there was increased performance in the single-attempt installation placement of the drain and the tension test, with the latter reaching significance (p < 0.01**; Figures 4A, B). This is an important finding as even though this was a very basic surgical procedure for medical students to learn, it is critical that when they implement the tension test that it is done so properly so that it holds its placement, does not get misaligned, or worse, the drain retracts back into the patient (i.e., which is rare). Notably, the FP-POV group also faced some challenges with ensuring that the drain section was at the proper length (i.e., -21.21% reduction, but it was not statistically significant). Whether this observation of the FP-POV group would reduce or enhance in error remains to be elucidated, but it is important to note that from a surgical perspective, the tension test for the drain is more critical than then length. The main concern with the drain tension test over the length is the possibility of it, post-operatively, being accidentally displaced or removed when a nurse is cleaning the area, renewing a cast or bandaging near it. However, in the case when a drain might be surgically placed for extra ventricular drainage (Ros et al., 2020a), this could be potentially life threatening if the tension was improper or compromised. Thus, depending on the placement of the drain, the risk can be quite different, and it is important that its tension and placement are correctly done for all other subsequent medical care that follows. If this tension test is done improperly, it can lead to a surgical error in the OR, prolong the surgery, reducing the ability for the surgeon to move on to the next patient, and perhaps have to re-operate on the patient to re-open the surgical site, further complicating the patients’ medical situation. Thus, the finding that the FP-POV superficially was able to increase the proficiency (i.e., measured by % correct surgical procedural skills observed on the cadaver) by nearly 34% is substantial for a first attempt at demonstrating the competency of the technique. These findings also support the idea that future studies may need to incorporate a repeated-measures design to ensure proficiencies are optimally achieved and sustained to reduce the surgical risks and errors in the OR, as outlined above.
Similarly, in the dissection area of the curriculum, the individual component of the dissection movement was shown to be significantly improved by FP-POV (p < 0.05*; Figures 4A, B) when compared to that with TP-POV, with nearly a 25% increase in performance that achieved 100% proficiency when evaluated on the cadaver. This is a noteworthy finding as it shows that the FP-POV pedagogical intervention produced an accelerated form of clean and accurate surgical incisions and opening in a single attempt. This finding corroborates with the FP-POV pedagogical intervention, also showing 100% proficiency on the single attempt to install the placement of the drain in the drain area of the curriculum. Thus, the FP-POV pedagogical intervention increased the maximal proficiency of 100% on the surgical dissection movements and the drain placement on the first attempt, which was not observed in the TP-POV group. The only other assessment measures that reached the maximal 100% proficiency was the use of a straight incision line to make the surgical site in the incision area of the curriculum and the number of stitches suitable for the dermal overlock in the closing area of the curriculum. It is important to note that having a straight incision line is a very important aspect of any surgery as it involves not just the cleanliness of the surgical entry point but also would be equally important to have a clean suture site. Interestingly, both the TP-POV and the FP-POV groups, given the importance of this step of the surgical procedure demanded the most of their attention. Alternatively, both the TP-POV and the FP-POV groups’ perfect use of the proper number of stitches could be attributed to the silicone skin model aspect of the curriculum in which the participants were trained prior to being randomly assigned to either the TP-POV or the FP-POV groups. Thus, what can be attributed clearly to the pedagogical learning differences in FP-POV immersive VR is the learning acquisition of the necessary motor skills required to properly conduct the surgical dissection movement and drain placement with 100% proficiency in the first attempt at implementing this surgical technique on a cadaver. This FP-POV enhanced learning acquisition of the motor skills required for the surgical procedure corroborates with prior FP-POV studies (Ros and Trives, 2023; Maret et al., 2022; Ros and Neuwirth, 2020; Neuwirth and Ros, 2021; Ros et al., 2020a; Ros et al., 2020b; Ros et al., 2017). In addition to the participants’ competency to learn in FP-POV, they were also able to evidence proficiency in the practice, efficiency, and cleanliness of the procedural motor skills consistent with the mirror neuron theory (Fan and Luo, 2022; Choi et al., 2020). Additionally, there was statistically significant improvement in the dissection movement and the tension test, even with a small sample size in the present pilot study.
5 Conclusion
The present pilot study sought to evaluate differences in fourth-year medical students’ procedural surgical skill learning outcomes between TP-POV and FP-POV on surgically implanting a subdermal drain in a cadaver. The findings from the pilot study showed that the FP-POV and the TP-POV produced similar procedural motor skill proficiencies as learning outcomes, with an emerging trend approaching significance for the drain area of the curriculum potentially favoring the FP-POV group (Figure 3). From the surgical curriculum on the incision, dissection, drain, and closing techniques, differences between the two groups were observed in the dissection and the drain techniques. In particular, in the dissection techniques, the FP-POV group had a perfect dissection movement (p = 0.041*; Figure 4A), and in the drain techniques, the FP-POV group had a greater proficiency on the tension test (p = 0.016**; Figure 4A). What also became apparent from the present pilot study’s findings were the VR learning differences by way of VR-induced learning enhancements and VR-induced learning deficits. The FP-POV showed significant learning enhancements in drain curriculum for the dissection movement (+25%) and the tension test (+34.09%; Figure 4B). There were also trends of VR-induced learning enhancements for single-attempt installation placement for the drain curriculum. Conversely, although there were no statistically significant VR learning deficits, data were trending downward for the drain curriculum for the drain section length (−21.21%) and for the closing curriculum for the overlock loop (−10.61%; Figure 4B). All other data below 10% were negligible for interpreting whether the VR-induced enhancement or deficit was remarkable from the data obtained in the present pilot study.
The implications from the present pilot study’s findings suggest that 4-year medical students can benefit from some critical procedural learning skills through FP-POV. Additionally, the increased precision in the dissection movement can serve to reduce scaring, offer patients a cleaner surgical incision with less complications and post-surgical intervention, and can offer a better area to work with in the rare event that another surgical incision may be needed to re-open or re-address the same or additional surgical interventions to the same area. The other benefit of the FP-POV was a more accurate tension test for the surgical drain, which, in terms of patient follow-up care, can be the most likely to have complications. These two core findings from this pilot study offer valuable insights for medical programs to pay more careful attention to these two areas of their practicum trainings, in which they assess these procedural skills in medical students. If FP-POV can be added to the curriculum, it may help compliment what medical programs are already doing in the curriculum and further serve to reduce surgical procedural complications that might otherwise have higher rates of error from medical students. Additionally, this concern may be circumvented if medical programs offer the FP-POV options earlier in the curriculum (i.e., in the first- or second-year medical student cohorts), as opposed to the fourth-year medical student cohorts, in which the present pilot study assessed. It is conceivable that if these FP-POV educational interventions were deployed earlier in the curriculum, the medical students could have more procedural knowledge and feedback through which they can further master these surgical skills through increased proficiency and reduced errors. These findings should be considered in the context for medical educators and researchers to consider for both modifying the future curriculum and further exploration in research that replicates and validates the findings found herein with larger sample sizes.
The present pilot study is not without limitations. First, the sample size was based upon the size of the fourth-year medical student cohort and the individuals that decided to volunteer for this extracurricular experience. After gaining new knowledge that there are benefits from the FP-POV experience when learning this procedure, it will increase the recruitment for a follow-up study with more medical students. The voluntary nature of the fourth-year medical students may have a prior learning history well-acclimated to video lecture-based learning than VR, which might explain why there were areas with reduced proficiency (Figure 4B red bars), but it is important to note that they were not statistically significant. Perhaps, future studies introducing these FP-POV surgical training techniques earlier in the first-year medical student curriculum may prove useful in accelerating more mastery of the surgical techniques. Conceivably, if there are hypothetically eight surgical learning modules in a traditional surgical training curriculum and FP-POV immersive VR can accelerate learning and proficiency in these techniques, then starting earlier with FP-POV immersive VR may permit more surgical techniques to be added to the conventional surgical training curriculum. This would have the advantage of medical schools having more graduates upskilled with more technical experiences and ability to take on additional OR surgical tasks, challenges, or medical complications when they move on to residency and/or employment in the medical and allied healthcare sectors. This type of training would also be predictive of increasing both the proficiency and efficiency in the OR, serving to reduce indirect and direct costs, reduce overlapping surgeries, and create more ease in scheduling flow through while also improving patients’ surgical and post-surgical outcomes. This could also serve to reduce the inherent surgical fatigue when the surgeon themselves has to move from patient-to-patient.
However, the latter remains to be tested, but it is within reason to speculate, given the abundance of work on VR in the medical field on increasing such efficiency. Whether an efficient surgeon that saves time between surgeries and reduces their own fatigue versus a surgeon that saves time between surgeries and the OR schedules more surgeries for them to conduct in a given day is a real possibility that may make an assessment of this factor less practical; in particular, as the surgeries might vary when considering medical emergencies and the surgeon’s area(s) of specialty. Therefore, the use of FP-POV may prove quite beneficial for medical students’ training in any stage of their medical school year in their program. Notably, earlier pedagogical intervention would be better. Additionally, the present study’s design evaluated the differences in surgical curriculum learning between the FP-POV and TP-POV groups. However, considering having the choice between these two groups for the medical students to elect which option they would prefer to choose was not explored in the present pilot study. Future work could investigate whether such a comparison matters or what additional effects it might cause on the medical student’s learning outcomes as different VR displays may cause such learning differences. Moreover, future comprehensive comparisons conducted on a larger-scale study could also consider differences in medical student learning immersion vs. shifting between a TP-POV and a FP-POV. However, these critical points were beyond the scope of the present pilot study, but they are not without merit to note as limitations that will guide the commencement of future explorations in these areas. More work will be required in these areas to further advance knowledge in the field to best guide educators and researchers seeking to use VR in pedagogical and meaningful ways to support the next generation of medical doctors and surgeons on cadaver-based curricular procedural skill training.
Regarding the latter point, VR has been used over the last 20 years in a variety of cadaver-based surgical trainings regarding dissecting cavernous sinus (Qian et al., 2018), reverse total shoulder arthroplasty (Crockatt et al., 2023), temporal bone dissection (Zhao et al., 2011), laparoscopic surgical training (Draper and Constantin, 2022; Van Bruwaene et al., 2015; Sharma and Horgan, 2012), comparisons between digital and virtual dissections of cadavers (Van Dijck, 2008), skeletal anatomy (Koucheki et al., 2023; Chen et al., 2020; González Izard et al., 2017), musculoskeletal anatomy (González Izard et al., 2017; Codd and Choudhury, 2021), and acetabulum reaming (Pelliccia et al., 2020), to name a few. These VR training modules have even begun to compare stereoscopic value (Brewer-Deluce et al., 2021), immersive VR (Kurul et al., 2020; Stepan et al., 2017), augmented VR (Uruthiralingam and Rea, 2020), and augmented VR and HoloLens-based augmented VR (Vergel et al., 2020), but the verdict remains unclear as each VR application offers different solutions for different educational and research needs (Zhao et al., 2020b). Notably, VR-based teaching has begun to live up to its prediction to be a pedagogically significant tool for the twenty-first century (Hoffman and Vu, 1997). However, the unique ability to blend VR to teach cadaver dissections during the COVID-19 pandemic (Miltykh et al., 2023; Nakai et al., 2021; Choi et al., 2020) offered the field invaluable insight with byproducts that have currently pressured universities to consider more timely technology-based cost-effective VR solutions to overcoming the more cost prohibitive maintenance and storage of cadavers (McMenamin et al., 2018; Neuwirth et al., 2018a), which places other universities at pedagogical and other resource (dis)advantages to teach their students. Since VR anatomy has been globally identified to be comparable with traditional teaching methods (Raheem et al., 2023; Kolla et al., 2020; Erolin et al., 2019; Yiasemidou et al., 2018; Fairén González et al., 2017; Codd and Choudhury, 2021) but provides arguably greater procedural knowledge over conceptual knowledge gains (Ros et al., 2020b; Neuwirth and Ros, 2021; Ros et al., 2021), this outcome serves to create more advantages and possibilities to close educational learning gaps for students, even at the surgical training levels in medical schools. Taken together, the growing body of literature in medical student training of traditional cadaver dissections is swiftly expanding in broad ways that utilize VR-based pedagogy in several dissection training modules to support curricular learning while causing a modern paradigm shift in the ways medical and surgical students are to be trained. Previous studies that piloted the use of VR on cadaveric dissections showed enhanced procedural learning in first-year medical students’ understanding of anatomy (Darras et al., 2019). The present pilot study added to the literature on the advantages of immersive FP-POV VR procedural learning training in conducting more accurate dissection incisions and improved performance in conducting the tension test for installing a subdermal drain on cadavers, all of which collectively seek to enhance the quality of the medical student’s procedural skillsets of conducting surgical techniques prior to being able to work on a living patient through VR-based pedagogical interventions.
However, as educators, physicians, surgeons, and researchers alike embark on this continued journey in integrating several VR applications into the medical school curriculum, there appears to be a gap in the field directed towards clear educational learning outcomes and an assessment from VR interventions. Therefore, it is the collective authors’ opinions that immersive FP-POV VR should be used with a repeated-measures design with a validated assessment tool to help refine the several different approaches that are being developed in VR for medical school student learning outcomes (i.e., especially, procedural motor skills). This is important as medical students will have the critical responsibility for caring for patients in the future. Currently, the board examinations, completion of residency, or multiple residencies are used to validate well-trained physicians and surgeons, but perhaps, there might also be a place for immersive FP-POV VR along this learning competency and proficiency continuum and ongoing continuing educational credits/units across different medical areas of specialty. These findings offer unique training opportunities to be considered when designing future VR curriculum with the suggested use of repeated-measures designs on the procedural surgical skills to assess learning curves and their potential translations in reducing operating room errors, costs, planning and scheduling times, and overlapping surgeries, thereby positively contributing to a more efficient and safer OR surgical experience for the staff and patients in the near future.
Data availability statement
The original contributions presented in the study are included in the article/supplementary material; further inquiries can be directed to the corresponding author.
Ethics statement
The studies involving humans were approved by The University of Montpellier’s Institutional Review Board (IRB). The studies were conducted in accordance with the local legislation and institutional requirements. The participants provided their written informed consent to participate in this study. Written informed consent was obtained from the individual(s) for the publication of any potentially identifiable images or data included in this article.
Author contributions
LN: conceptualization, data curation, formal analysis, investigation, software, visualization, writing–original draft, and writing–review and editing. SN: conceptualization, data curation, funding acquisition, investigation, methodology, project administration, resources, software, supervision, visualization, and writing–review and editing. SD: conceptualization, data curation, funding acquisition, investigation, methodology, project administration, resources, software, supervision, visualization, and writing–original draft. NL: conceptualization, data curation, funding acquisition, investigation, methodology, project administration, resources, software, supervision, visualization, and writing–review and editing. MR: conceptualization, data curation, formal analysis, funding acquisition, investigation, methodology, project administration, resources, software, supervision, validation, visualization, writing–original draft, and writing–review and editing.
Funding
The author(s) declare that financial support was received for the research, authorship, and/or publication of this article. The authors would like to thank the University of Montpellier’s Education Science School for funding the present study through the “Take Off Project” grants under the Center of Support for Pedagogical Innovation affiliated to a Platform Services called the Montpellier University of Excellence (MUSE). The grant, in part, was used to purchase the VR-headsets for this study.
Acknowledgments
The authors would like to thank the IRB at the College of Neurosurgery and the Administration in the Education Science School and Medical School at the University of Montpellier for permitting them the opportunity to explore the possibilities of this pilot study and future studies for implementing and assessing the value of FP-POV in the medical school curriculum to enhance students’ procedural skill acquisition during their early career training in hopes that it will make them better skilled in their future careers through such VR educational training. The authors would also like to thank S.D. for her life’s long work of dedication, care, and desire to help her patients through the OR. Unfortunately, S.D. passed away prior to the completion of this manuscript, and this study could not have been possible without her. Therefore, this study is dedicated to S.D. as her insights, tireless commitment to the efficiency in the OR, medical knowledge, and genuine care for patients and the teaching of medical students traditionally and through FP-POV have shaped a new generation of modern medical healthcare workers in the OR. For her unwavering dedication and service to the education of the profession and the profession itself, we are all forever grateful, and S.D. will not be forgotten.
Conflict of interest
MR declares being CEO of Revinax and that he completed his doctorate at the University of Montpellier, which might be perceived as a conflict of interest. All other authors declare that the research was conducted in the absence of any commercial or financial relationships that could be construed as a potential conflict of interest.
The author(s) declared that they were an editorial board member of Frontiers, at the time of submission. This had no impact on the peer review process and the final decision.
Publisher’s note
All claims expressed in this article are solely those of the authors and do not necessarily represent those of their affiliated organizations, or those of the publisher, the editors, and the reviewers. Any product that may be evaluated in this article, or claim that may be made by its manufacturer, is not guaranteed or endorsed by the publisher.
References
Almousa, O., Zhang, R., Dimma, M., Yao, J., Allen, A., Chen, L., et al. (2021). Virtual reality technology and remote digital application for tele-simulation and global medical education: an innovative hybrid system for clinical training. As 52 (5), 614–634. doi:10.1177/10468781211008258
Ashtari, N., Bunt, A., McGrenere, J., Nebeling, M., and Chilana, P. K. (2020). “Creating augmented and virtual reality application: current practices, challenges, and opportunities,” in Proceedings of the 2020 CHI conference on human factors in computing systems, 1–13. doi:10.1145/3313831.3376722
Barteit, S., Lanfermann, L., Bärnighausen, T., Neuhann, F., and Beiersmann, C. (2021). Augmented, mixed, and virtual reality-based head-mounted devices for medical education: systematic Review. JMIR Serious Games 9 (3), 329080. doi:10.2196/29080
Bayline, R. J., Morrison, M. E., Illig, K. R., Martinez-Acosta, V. G., Becker, L. A., Gavero, C. B., et al. (2020). Faculty for undergraduate neuroscience (FUN) statement on diversity, equity, and inclusion. J. Undergrad. Neurosci. Educ. 18 (2), E4–E5.
Bharathan, R., Aggarwal, R., and Darzi, A. (2013). Operating room of the future. Best Pract. and Res. Clin. Obstetrics and Gynaecol. 27 (3), 311–322. doi:10.1016/j.bpobgyn.2012.11.003
Botha, B. S., de Wet, L., and Botma, Y. (2021). Undergraduate nursing student experiences in using immersive virtual reality to manage a patient with a foreign object in the right lung. Clin. Simul. Nurs. 56, 76–83. doi:10.1016/j.ecns.2020.10.008
Brewer-Deluce, D., Bak, A. B., Simms, A. J., Sinha, S., Mitchell, J. P., Shin, D., et al. (2021). Virtual reality bell-ringer: the development and testing of a stereoscopic application for human gross anatomy. Anat Sci Educ. 14 (3), 330–341. doi:10.1002/ase.2074
Catchpole, K., Mishra, A., Handa, A., and McCulloch, P. (2008). Teamwork and error in the operating room: analysis of skills and roles. Ann. Surg. 247 (4), 699–706. doi:10.1097/SLA.0b013e3181642ec8
Chen, S., Zhu, J., Cheng, C., Pan, Z., Liu, L., Du, J., et al. (2020). Can virtual reality improve traditional anatomy education programs? A mixed-methods study on the use of a 3D skull model. BMC Med. Educ. 20 (1), 1–10. doi:10.1186/s12909-020-02255-6
Choi, J. W., Kim, B. H., Huh, S., and Jo, S. (2020). Observing actions through immersive virtual reality enhances motor imagery training. IEEE Trans. Neural Syst. Rehabilitation Eng. 28 (7), 1614–1622. doi:10.1109/tnsre.2020.2998123
Choi, N., Kim, E., Ahn, J. H., Ahn, J. H., Park, J. Y., Kim, S. H., et al. (2022). Blending virtual reality laboratories with cadaver dissection during COVID-19 pandemic. Anatomia 1 (2), 134–147. doi:10.3390/anatomia1020014
Christopoulos, A., Pellas, N., and Laakso, M. J. (2020). A learning analytics theoretical framework for STEM education virtual reality applications. Educ. Sci. 10 (11), 317. doi:10.3390/educsci10110317
Cima, R. R., and Deschamps, C. (2013). Role of the surgeon in quality and safety in the operating room environment. General Thorac. Cardiovasc. Surg. 61, 1–8. doi:10.1007/s11748-012-0111-6
Codd, A. M., and Choudhury, B. (2021). Virtual reality anatomy: is it comparable with traditional methods in the teaching of human forearm musculoskeletal anatomy? Anat. Sci. Educ. 4 (3), 119–125. doi:10.1002/ase.214
Concannon, B. J., Esmail, S., and Roduta, R. M. (2019). Head-mounted display virtual reality in post-secondary education and skill training. Front. Educ. 4, 80. doi:10.3389/feduc.2019.00080
Crockatt, W. K., Confino, J. E., Kopydlowski, N. J., Jobin, C. M., and Levine, W. N. (2023). Comparing skill acquisition and validity of immersive virtual reality with cadaver laboratory sessions in training for reverse total shoulder arthroplasty. JBJS Open Access 8 (3). doi:10.2106/jbjs.oa.22.00141
Darras, K. E., Spouge, R., Hatala, R., Nicolaou, S., Hu, J., Worthington, A., et al. (2019). Integrated virtual and cadaveric dissection laboratories enhance first year medical students’ anatomy experience: a pilot study. BMC Med. Educ. 19, 366–6. doi:10.1186/s12909-019-1806-5
De Ponti, R., Marazzato, J., Maresca, A. M., Rovera, F., Carcano, G., and Ferrario, M. M. (2020). Pre-graduation medical training including virtual reality during COVID-19 pandemic: a report on students’ perception. BMC Med. Educ. 20, 332. doi:10.1186/s12909-020-02245-8
Draper, K. M., and Constantin, A. G. (2022). The role of cadavers and virtual reality in laparoscopic training. UBC Med. J. 14 (1).
Dreyfus, D., Nair, A., and Rosales, C. (2020). The impact of planning and communication on unplanned costs in surgical episodes of care: implications for reducing waste in hospital operating rooms. J. Operations Manag. 66 (1-2), 91–111. doi:10.1002/joom.1070
Erolin, C., Reid, L., and McDougall, S. (2019). Using virtual reality to complement and enhance anatomy education. J. Vis. Commun. Med. 42 (3), 93–101. doi:10.1080/17453054.2019.1597626
Fairén González, M., Farrés, M. M., Moyes Ardiaca, J., and Insa, E. (2017). “Virtual reality to teach anatomy,” in Eurographics 2017: education papers (European Association for Computer Graphics Eurographics), 51–58. doi:10.2312/eged.20171026
Fan, H., and Luo, Z. (2022). Functional integration of mirror neuron system and sensorimotor cortex under self-actions visual perception. Behav. Brain Res. 432, 113784.
Frendø, M., Konge, L., Cayé-Thomasen, P., Sørensen, M. S., and Andersen, S. A. W. (2020). Decentralized virtual reality training of mastoidectomy improves cadaver dissection performance: a prospective, controlled cohort study. Otology and Neurotol. 41 (4), 476–481. doi:10.1097/mao.0000000000002541
González Izard, S., Juanes Méndez, J. A., and Ruisoto Palomera, P. (2017). Virtual reality educational tool for human anatomy. J. Med. Syst. 41, 76. doi:10.1007/s10916-017-0723-6
Hatt, D., Zimmerman, E., Chang, E., Vane, J., Hollenback, K. A., and Shah, A. (2023). First-person point-of-view instructional video on lumbar puncture procedure. Pediatr. Emerg. Care 39 (12), 953–956. doi:10.1097/PEC.0000000000003084
Hoffman, H., and Vu, D. (1997). Virtual reality teaching tool of the twenty-first century? i(12), 1076–1081.
Ismail, S. M., and Hashim, H. (2020). Virtual reality-based education (VRBE): understanding students’ readiness and expectancies. Int. J. Innov. Technol. Explor. Eng. 9 (3), 172–176.
Javaid, M., and Haleem, A. (2020). Virtual reality applications toward medical field. Clin. Epidemiol. Glob. Health 8 (2), 600–605. doi:10.1016/j.cegh.2019.12.010
Jiang, H., Vimalesvaran, S., Wang, J. K., Lim, K. B., Mogali, S. R., and Car, L. T. (2022). Virtual reality in medical students’ education: scoping Review. JMIR Med. Educ. 8 (1), e34860. doi:10.2196/34860
Kang, H. (2021). Sample size determination and power analysis using the G*Power software. J. Educ. Eval. Health Prof. 18, 17. doi:10.3352/jeehp.2021.18.17
Kolla, S., Elgawly, M., Gaughan, J. P., and Goldman, E. (2020). Medical student perception of a virtual reality training module for anatomy education. Med. Sci. Educ. 30 (3), 1201–1210. doi:10.1007/s40670-020-00993-2
Koucheki, R., Lex, J. R., Morozova, A., Ferri, D., Hauer, T. M., Mirzaie, S., et al. (2023). Immersive virtual reality and cadaveric bone are equally effective in skeletal anatomy education: a randomized crossover noninferiority trial. J. Surg. Educ. 80 (7), 1028–1038. doi:10.1016/j.jsurg.2023.04.005
Kurul, R., Ögün, M. N., Neriman Narin, A., Avci, S., and Yazgan, B. (2020). An alternative method for anatomy training: immersive virtual reality. Anat. Sci. Educ. 13 (5), 648–656. doi:10.1002/ase.1959
Lee, D. J., Ding, J., and Guzzo, T. J. (2019). Improving operating room efficiency. Curr. Urol. Rep. 20 (28), 28–8. doi:10.1007/s11934-019-0895-3
Lonner, J. G., Goh, G. S., Sommer, K., Niggeman, G., Levicoff, E. A., Vernace, J. V., et al. (2021). Minimizing surgical instrument burden increases operating room efficiency and reduces perioperative costs in total joint arthroplasty. J. Arthroplasty 36 (6), 1857–1863. doi:10.1016/j.arth.2021.01.041
Macario, A. (2006). Are your hospital operating rooms “efficient”? A scoring system with eight performance indicators. J. Am. Soc. Anesthesiol. 105 (2), 237–240. doi:10.1097/00000542-200608000-00004
Maret, D., Barrere, S., Ros, M., and Geeraerts, T. (2022). Clinical gesture in dental education: presentation of a new immersive virtual reality application in first person point-of-view. J. Dent. Educ. 87 (S1), 920–922. doi:10.1002/jdd.13084
Marks, B., and Thomas, J. (2022). Adoption of virtual reality technology in higher education: an evaluation of five teaching semesters in a purpose-designed laboratory. Educ. Inf. Technol. 27 (1), 1287–1305. doi:10.1007/s10639-021-10653-6
McMenamin, P. G., McLachlan, J., Wilson, A., McBride, J. M., Pickering, J., Evans, D. J., et al. (2018). Do we really need cadavers anymore to learn anatomy in undergraduate medicine? Med. Teach. 40 (10), 1020–1029. doi:10.1080/0142159x.2018.1485884
Mehraeen, E., Dashti, M., Ghasemzadeh, A., Afsahi, A. M., Shahidi, R., Mirzapour, P., et al. (2023). Virtual reality in medical education during the COVID-19 pandemic. A Syst. Rev. doi:10.21203/rs.3.rs-2551708/v1
Miltykh, I., Kafarov, E. S., Covantsev, S., Dadashev, A. S., Skarlis, A. A., and Zenin, O. K. (2023). A new dimension in medical education: virtual reality in anatomy during COVID-19 pandemic. Clin. Anat. 36 (7), 1007–1015. doi:10.1002/ca.24098
Mukherji, B. R., Neuwirth, L. S., and Limonic, L. (2017). Making the case for real diversity: redefining underrepresented minority students in public universities. Sage Open 7 (2), 215824401770779. doi:10.1177/2158244017707796
Nakai, K., Terada, S., Takahara, A., Hage, D., Tubbs, R. S., and Iwanaga, J. (2021). Anatomy education for medical students in a virtual reality workspace: a pilot study. Clin. Anat. 35 (1), 40–44. doi:10.1002/ca.23783
Nersesian, E., Spryszynski, A., and Lee, M. J. (2019). “Integration of virtual reality in secondary STEM education,” in 2019 IEEE integrated STEM education conference (ISEC), 83–90.
Neuwirth, L. S., Dacius, T. F., and Mukherji, B. R. (2018a). Teaching neuroanatomy through a historical context. J. Undergrad. Neurosci. Educ. 16 (2), E26–E31.
Neuwirth, L. S., Ebrahimi, A., Mukherji, B. R., and Park, L. (2018b). “Addressing diverse college students and interdisciplinary learning experiences through online virtual laboratory instruction: a theoretical approach to error-based learning in biopsychology,” in Visual approaches to cognitive education with technology integration (IGI Global), 283–303.
Neuwirth, L. S., Jovic, S., and Mukherji, B. R. (2021). Reimagining higher education during and post-COVID-19: challenges and opportunities. J. Adult Continuing Educ. 27 (2), 141–156. doi:10.1177/1477971420947738
Neuwirth, L. S., Quadros-Menella, P. S., Kang, Y. Y., Linden, M. L., Nahmani, M., Abrams, M., et al. (2021a). Revisiting diversity, equity, and inclusion commitments and instituting lasting actionable changes in the faculty for undergraduate neuroscience. J. Undergrad. Neurosci. Educ. 20 (1), E1–E3.
Neuwirth, L. S., and Ros, M. (2021). Comparisons between first person point-of-view 180° video virtual reality head-mounted display and 3D video computer display in teaching undergraduate neuroscience students stereotaxic surgeries. Front. Virtual Real. 2, 706653. doi:10.3389/frvir.2021.706653
Nichol, P. F., and Saari, M. J. (2023). Risk modeling of errors in the surgical instrument cycle, insights into solutions for an expensive and persistent problem. Perioper. Care Operating Room Manag. 32, 100333. doi:10.1016/j.pcorm.2023.100333
Pellas, N., Dengel, A., and Christopolous, A. (2020). A-scoping review of immersive virtual reality in STEM education. IEEE Trans. Learn. Technol. 13 (4), 748–761. doi:10.1109/tlt.2020.3019405
Pellas, N., Mystakidis, S., and Kazanidis, I. (2021). Immersive virtual reality in K-12 and higher education: a systematic review of the last decade scientific literature. Virtual Real. 25 (3), 835–861. doi:10.1007/s10055-020-00489-9
Pelliccia, L., Lorenz, M., Heyde, C. E., Kaluschke, M., Klimant, P., Knopp, S., et al. (2020). A cadaver-based biomechanical model of acetabulum reaming for surgical virtual reality training simulators. Sci. Rep. 10 (1), 14545. doi:10.1038/s41598-020-71499-5
Pottle, J. (2019). Virtual reality and the transformation of medical education. Future Healthc. J. 6 (3), 181–185. doi:10.7861/fhj.2019-0036
Qian, Z. H., Feng, X., Li, Y., and Tang, K. (2018). Virtual reality model of the three-dimensional anatomy of the cavernous sinus based on a cadaveric image and dissection. J. Craniofacial Surg. 29 (1), 163–166. doi:10.1097/SCS.0000000000004046
Qorbani, H. S., Arya, A., Nowlan, N., and Abdinejad, M. (2021). “ScienceVR: a virtual reality framework for STEM education, simulation and assessment,” in 2021 IEEE international conference on artificial intelligence and virtual reality (AIVR), 267–275.
Raheem, O. B., Omeijeh, B. O., and Ibeachu, C. (2023). Virtual reality: a new approach for learning anatomy. Afr. J. Humanit. Contemp. Educ. Res. 10 (1), 186–193.
Reeves, S. M., Crippen, K. J., and McCray, E. D. (2021). The varied experience of undergraduate students learning chemistry in virtual reality laboratories. Comput. and Educ. 175, 104320. doi:10.1016/j.compedu.2021.104320
Revinax (2021). 11 advantages of immersive virtual reality for optimizing the launch of new medical devices. Available at: https://www.revinax.net/en/blog/virtual-reality/11-advantages-of-immersive-virtual-reality-for-optimizing-the-launch-of-new-medical-devices/.
Rogers, M. P., DeSantis, A. J., Janjua, H., Barry, T. M., and Kuo, P. C. (2021). The future surgical training paradigm: virtual reality and machine learning in surgical education. Surgery 169 (5), 1250–1252. doi:10.1016/j.surg.2020.09.040
Ros, M. (2021). Les intérêts pédagogiques du tutoriel immersif sur l'apprentissage technique appliqué au domaine médico-chirurgical: études sur la rétention, la restitution de connaissances théoriques, l'apprentissage des techniques et sur la généralisation au monde réel d'une procédure technique apprise. Educ. Univ. Paul. Valéry - Montpellier. III, 2021. Français. ⟨NNT.
Ros, M., Debien, B., Cyteval, C., Molinari, N., Gatto, F., and Lonjon, N. (2020a). Applying an immersive tutorial in virtual reality to learning a new technique. Neurochirurgie 66 (4), 212–218. doi:10.1016/j.neuchi.2020.05.006
Ros, M., and Neuwirth, L. S. (2020). Increasing global awareness of timely COVID-19 healthcare guidelines through FPV training tutorials: portable public health crises teaching method. Nurse Educ. Today 91, 104479. doi:10.1016/j.nedt.2020.104479
Ros, M., Neuwirth, L. S., Ng, S., Debien, B., Molinari, N., Gatto, F., et al. (2021). The effects of an immersive virtual reality application in first person point-of-view (IVRA-FPV) on the learning and generalized performance of a lumbar puncture medical procedure. Educ. Technol. Res. Dev. 69, 1529–1556. doi:10.1007/s11423-021-10003-w
Ros, M., and Trives, J. V. (2023). Point-of-view recording device. U. S. Pat. Appl. Publ. pub. no. U. S. 2020/0257184 A1.
Ros, M., Trives, J. V., and Lonjon, N. (2017). From stereoscopic recording to virtual reality headsets: designing a new way to learn surgery. Neurochirurgie 63 (1), 1–5. doi:10.1016/j.neuchi.2016.08.004
Ros, M., Weaver, L., and Neuwirth, L. S. (2020b). “Virtual reality stereoscopic 180-degree video-based immersive environments: applications for training surgeons and other medical professionals,” in Cases on instructional design and performance outcomes in medical education (IGI Global), 92–119.
Sharma, M., and Horgan, A. (2012). Comparison of fresh-frozen cadaver and high-fidelity virtual reality simulator as methods of laparoscopic training. World J. Surg. 36 (8), 1732–1737. doi:10.1007/s00268-012-1564-6
Stepan, K., Zeiger, J., Hanchuk, S., Del Signore, A., Shrivastava, R., Govindaraj, S., et al. (2017). Immersive virtual reality as a teaching tool for neuroanatomy. Int. Forum Allergy and Rhinology 7 (10), 1006–1013. doi:10.1002/alr.21986
Stockert, E. W., and Langerman, A. (2014). Assessing the magnitude and costs of intraoperative inefficiencies attributable to surgical instrument trays. J. Am. Coll. Surg. 219 (4), 646–655. doi:10.1016/j.jamcollsurg.2014.06.019
Sultan, L., Abuznadah, W., Al-Jifree, H., Khan, M. A., Alsaywid, B., and Ashour, F. (2019). An experimental study on usefulness of virtual reality 360° in undergraduate medical education. Adv. Med. Educ. Pract. 10, 907–916. doi:10.2147/AMEP.S219344
Tursø-Finnich, T., Jensen, R. O., Jensen, L. X., Konge, L., and Thinggarrd, E. (2023). Virtual reality head-mounted displays in medical education: a systematic review. Simul. Healthc. 18 (1), 42–50. doi:10.1097/SIH.0000000000000636
Ugur, E., Kara, S., Yildirim, S., and Akbal, E. (2016). Medical errors and patient safety in the operating room. Age 66 (5), 593–597.
Uruthiralingam, U., and Rea, P. M. (2020). Augmented and virtual reality in anatomical education – a systematic review. In: Adv. Exp. Med. Biol., P. Rea (Eds). 1235. 89, 101. doi:10.1007/978-3-030-37639-0_5
Van Bruwaene, S., Schijven, M. P., Napolitano, D., De Win, G., and Miserez, M. (2015). Porcine cadaver organ or virtual-reality simulation training for laparoscopic cholecystectomy: a randomized, controlled trial. J. Surg. Educ. 72 (3), 483–490. doi:10.1016/j.jsurg.2014.11.015
Van Dijck, J. (2008). Digital cadavers and virtual dissection. Anat. Live Perform. Operating Theatre 1, 29–48. doi:10.1515/9789048501229-005
Vergel, R. S., Tena, P. M., Yrurzum, S. C., and Cruz-Neira, C. (2020). A comparative evaluation of a virtual reality table and a HoloLens-based augmented reality system for anatomy training. IEEE Trans. Human-Machine Syst. 50 (4), 337–348. doi:10.1109/THMS.2020.2984746
Weller, J., Cumin, D., Torrie, J., Boyd, M., Civil, I., Madell, D., et al. (2015). Multidisciplinary operating room simulation-based team training to reduce treatment errors: a feasibility study in New Zealand hospitals. N. Z. Med. J. 128 (1418), 40–51. Available at: http://hdl.handle.net/2292/30103.
Wu, R. L., and Aufses, A. H. (2012). Characteristics and costs of surgical scheduling errors. Am. J. Surg. 204 (4), 468–473. doi:10.1016/j.amjsurg.2012.01.011
Yiasemidou, M., Gkaragkani, E., Glassman, D., and Biyani, C. S. (2018). Cadaveric simulation: a review of reviews. Ir. J. Med. Sci. 187, 827–833. doi:10.1007/s11845-017-1704-y
Zhao, J., LaFemina, P., Carr, J., Sajjadi, P., Wallgrün, J. O., and Klippel, A. (2020a). “Learning in the field: comparison of desktop, immersive virtual reality, and actual field trips for place-based STEM education,” in 2020 IEEE conference on virtual reality and 3D user interfaces (VR), 893–902.
Zhao, J., Xu, X., Jiang, H., and Ding, Y. (2020b). The effectiveness of virtual reality-based technology on anatomical teaching: a meta-analysis of randomized controlled studies. BMC Med. Educ. 20 (127), 1–10. doi:10.1186/s12909-020-1994-z
Zhao, Y. C., Kennedy, G., Yukawa, K., Pyman, B., and O’Leary, S. (2011). Can virtual reality simulator be used as a training aid to improve cadaver temporal bone dissection? Results of a randomized blinded control trial. Laryngoscope 121 (4), 831–837. doi:10.1002/lary.21287
Appendix
Procedural surgical skill assessment evaluation tool for implementing the skillset on a cadaver. Each section is listed below with their total number of points that can be earned to demonstrate the competency and proficiency of the surgical skill.
Keywords: first-person point-of-view, third-person point-of-view, immersive virtual reality, surgical procedural motor skills, medical students, cadavers, subdermal drain, Revinax Handbook®
Citation: Neuwirth LS, Ng S, Devors S, Lonjon N and Ros M (2024) Comparing fourth-year medical students’ procedural surgical skill learning outcomes between third-person point-of-view video lectures and first-person point-of-view immersive virtual reality: a pilot study of surgically implanting a subdermal drain on cadavers. Front. Virtual Real. 5:1408092. doi: 10.3389/frvir.2024.1408092
Received: 27 March 2024; Accepted: 29 July 2024;
Published: 26 September 2024.
Edited by:
Panagiotis Kourtesis, American College of Greece, GreeceReviewed by:
Vangelis Lympouridis, University of Southern California, United StatesMauro Mocerino, Curtin University, Australia
Copyright © 2024 Neuwirth, Ng, Devors, Lonjon and Ros. This is an open-access article distributed under the terms of the Creative Commons Attribution License (CC BY). The use, distribution or reproduction in other forums is permitted, provided the original author(s) and the copyright owner(s) are credited and that the original publication in this journal is cited, in accordance with accepted academic practice. No use, distribution or reproduction is permitted which does not comply with these terms.
*Correspondence: Maxime Ros, bWF4aW1lcm9zQGdtYWlsLmNvbQ==