- 1Institute for Sport and Movement Science, University of Stuttgart, Stuttgart, Germany
- 2Institute for Games, Stuttgart Media University, Stuttgart, Germany
Introduction: Motor-cognitive learning is crucial for achieving and maintaining wellbeing. Exergames can effectively facilitate this type of learning due to their inherent qualities of exertion and game-related disciplines. These qualities can create effectiveness, enjoyment, and meaning in the lives of individuals. To address these aspects equally, the design process for exergame interventions needs to be interdisciplinary from the beginning.
Objective: This paper aims to (1) enhance an exergame design process model for interdisciplinary co-creation (CIEM) by an Extended Reflection part (CIEMER). Furthermore, it aims to (2) show a formal process for making the abstract model applicable. In doing so, (3) this paper will also derive methods for conducting the process in an academic seminar.
Methods: The study employed the CIEMER to conduct a 2-month academic seminar with 20 students. The seminar consisted of a 3-day intensive workshop, a 6-week work phase, and a 1-week testing phase, creating four Extended Reality prototypes. We used a mixed methods approach to evaluate the model, including feedback interviews with external experts, internal surveys, and written reflections from student designers.
Results: Four motor-cognitive learning prototypes in Extended Reality were created using the CIEMER. External expert evaluations highlighted the prototypes’ alignment with effective, enjoyable, and meaningful objectives and potential efficacy while noting shortcomings in discipline-specific theoretical application. Internal feedback from students, collected via surveys and reflections, consistently showed positive outcomes in interdisciplinary collaboration and learning, underscoring the importance of an integrated approach in achieving project goals.
Conclusion: The formal process within CIEMER effectively yielded four promising prototypes, demonstrating its sufficiency. Students positively acknowledged the benefits of interdisciplinary collaboration, finding it supportive and competence-enhancing. Additionally, the Extended Reflections enabled rapid and targeted iterations, streamlining the reflection of the current state and Creation process.
1 Introduction
Age-associated declines in cognition, perception, and motor skills can lead to a loss of independence in older adults, making them reliant on external assistance (Seidler and Schott, 2015). Therefore, it is crucial to investigate and measure cognitive and motor changes throughout aging to develop effective training methods that promote successful aging. These methods should foster motor-cognitive learning to counteract these declines and motivate learners to actively engage in such activities. A novel approach in this domain using of exergames, which innovatively blend physical exertion with interactive video gaming (Oh and Yang, 2010). These exergames stand out due to their inherent interdisciplinary nature, integrating aspects of both exertion and game-related disciplines. Despite the multifaceted potential of exergames to be effective, enjoyable, and meaningful (EEM) (Retz et al., 2023a), their combined potential often remains untapped (Sinclair et al., 2009; Martin-Niedecken et al., 2019).
A common limitation developing of exergames is the tendency to design them from a narrow, single-domain scientific perspective, focusing primarily on specific objectives relevant to that domain. These perspectives, while grounded in valuable methods and theoretical frameworks, fail to fully leverage the extensive potential of exergames (Subramanian et al., 2020; Martin-Niedecken et al., 2020; Isbister, 2016; Florian‘Floyd’Mueller, 2017; Marshall et al., 2016; Kajastila and Hämäläinen, 2015). It is crucial to create interdisciplinary solutions that combine discipline-specific goals into a common approach to unlock the full potential of exergames. Effective ways must be found to teach people from different disciplines how to work together seamlessly in interdisciplinary exergame design to create EEM to foster motor and cognitive learning experiences. A new approach to foster this by addressing collaboration in exergame design is our Co-Creative Interdisciplinary Exergame Design Process Model (CIEM) (Retz et al., 2023a). Although the CIEM focuses on the Creation aspect of exergame design, a gap in the model regarding interdisciplinary reflection becomes apparent. This challenge arises because exertion and game-related disciplines not only have different focuses but also rely on distinct evaluation methods.
In this work, our objective is to enhance the CIEM by an Extended Reflection part (CIEMER) for applying feedback and adopting an iterative approach. In doing so, we show a formal process to make the theoretical CIEMER practically applicable. We introduce specific methods developed for implementing the full process of CIEMER in an academic seminar. Our work demonstrates the application of CIEMER by creating four motor-cognitive learning prototypes in Extended Realities (XR). A mixed-methods approach was adopted to evaluate the model, including obtaining feedback from external experts through interviews and conducting internal surveys and written reflections with the student designers.
2 Related work
This section presents a review related to exergames, XR, and design methods. Additionally, it provides a theoretical foundation on motor and cognitive learning to demonstrate the application of our model and process and articulate our contributions.
2.1 Exergames and Extended Realities
Exergames, combine physical and cognitive activities with game experiences. They allow players to engage physically through body movements or the use of specific body segments to interact with sensor-based systems. (Oh and Yang, 2010; Subramanian et al., 2020; Röglin et al., 2023). There is an inherent potential in the properties of exergames, primarily due to their interdisciplinary composition that fuses games with exertion (Sinclair et al., 2009; Kappen et al., 2019; Martin-Niedecken et al., 2019). This fusion results in interventions aimed at achieving the primary objectives of the involved disciplines, such as effectiveness, enjoyment, and meaningfulness (EEM) (Retz et al., 2023a). The effectiveness of exergames arises from their composition, which incorporates both motor and cognitive challenging dual tasks (Oh and Yang, 2010; Schott and Klotzbier, 2018; Martin-Niedecken et al., 2019; Wang et al., 2021). Enjoying and positive player experiences can be created through the application of game design methods (Belchior et al., 2012; Marston, 2013; Lyons, 2015; Isbister, 2016; Kappen et al., 2019; Martin-Niedecken et al., 2020). The player experiences are multi-dimensional, encompassing various aspects such as need fulfillment, psychological state attainment, and the consequences of player actions (Abeele et al., 2020). Meaningfulness can be created by addressing the psychological needs of the target audience (Gerling et al., 2012; Chao et al., 2015; Ge et al., 2022; Ringgenberg et al., 2022; Retz et al., 2023a). The resulting experiences can be divided into a lived experience (“Erlebnis”) that is created during the intervention and a reflected experience (“Erfahrung”) that includes lived experiences and extends beyond the intervention (Mueller et al., 2020). This distinction is important for creating EEM experiences with exergames.
Further studies have developed guidelines and frameworks that can be used to address various issues within the exergame context like immersive social interaction (Márquez Segura et al., 2021a), virtue-based lenses focused on sports activities (Mueller et al., 2018), guidelines for movement-based games (Mueller and Isbister, 2014), the perspective of the responding, moving, sensing, and relating body to augment exertion interactions (Mueller et al., 2011), goal-setting theory (Toscos et al., 2006), behavior change theory (Consolvo et al., 2014) and joy of movement using dance theory (Loke and Robertson, 2013). Approaches which define quality criteria (Caserman et al., 2020) and modules (Wiemeyer, 2020) exist for serious games that can be applied to specific application domains, such as exergames. They emphasize the necessity of interdisciplinary teams to work collaboratively with the target audience to establish a balance between disciplinary standards and interdisciplinary integration.
While these guidelines and approaches can address specific aspects of exergame design or indicate which criteria or domains should be integrated and considered, they do not offer a comprehensive and holistic design process and show how to collaborate across disciplines in exergame design. Hence, interdisciplinary approaches are crucial to bridge these gaps (Subramanian et al., 2020; Martin-Niedecken et al., 2019; Florian‘Floyd’Mueller, 2017; Isbister, 2016; Marshall et al., 2016; Kajastila and Hämäläinen, 2015) and strengthen all aspects of EEM beyond the boundaries of a single discipline (Retz et al., 2023a).
Innovative approaches to exergame design, as illustrated by the Co-creative Interdisciplinary Exergame Design Process Model (CIEM) (Retz et al., 2023a), could address these shortcomings in the field. The model emphasizes interdisciplinary collaboration in a co-creative process from the inception of the design process. This CIEM provides a formal approach for co-creative interdisciplinary work that divides the Creation Part into an Experts Relation and Expert Human Relations. The aim of the Expert Relation is to develop a common structure and overarching goals. In Expert Human Relations each discipline defines discipline-specific goals and uses its unique methods and processes to create discipline-specific artifacts. These artifacts are referred to as a discipline’s passive prototype. Construction kits are created by deconstructing and translating these passive prototypes into a shared thesaurus. These construction kits build up the foundation for Active Design. In the Active Design phase, elements from various construction kits are merged and transformed through the vast space of possibilities offered by technology, resulting in a novel construction kit 2. The resulting prototypes are evaluated from an expert and experiencer perspective and can potentially include the target group as experts for their own needs in the subsequent design process (Retz et al., 2023a; b).
XR’s broad potential paves the way for designing exergames tailored to health contexts. It facilitates the accurate depiction of physical activities in virtual or real-world environments while also integrating cognitive tasks, game-based approaches, and addressing emotional needs using digital elements. On a continuum, XR encompasses various technologies that differ in their potential degree of immersion, ranging from low immersion in Augmented Reality (AR) to increasing immersion in Mixed Reality (MR) to high immersion in Virtual Reality (VR) (Milgram and Kishino, 1994; Skarbez et al., 2021). Immersion represents the objective degree to which an XR system has the potential to involve users in the experience. The subjective perception of immersion can be described as presence (Slater and Wilbur, 1997; Slater, 2009).
2.2 Design methods
The Design Thinking process represents a human-centered, iterative methodology for problem-solving and innovation that, according to d. School (Stanford Design School), consists of five distinct phases: Empathize, Define, Ideate, Prototype, and Test. These phases do not follow a rigid linear sequence but can be performed concurrently and repeatedly to ascertain the best possible solutions (Brown et al., 2008). Co-creation is an integrative approach characterized by the active involvement of stakeholders and their respective goals and methods within the design process, thereby adding value (Galvagno and Dalli, 2014; Leask et al., 2019). In game design, curation of the player’s experience is paramount. This experience is not explicitly designed but originates from the player’s unique choices throughout gameplay. However, choosing design elements indirectly impacts the overall game experience (Tekinbas and Zimmerman, 2003; Hunicke et al., 2004; Schell, 2008).
However, since exergames involve body movements as input, movement-based design methods are fundamental. Movement-based design methods (Gerling et al., 2012; Loke and Robertson, 2013; Márquez Segura et al., 2016) focus primarily on physical movements as a key element in the interaction design process. These methods are typically applied in domains where human movement is integral to the interaction or experience. They often consider the body’s capabilities, spatial requirements, and the emotional, cognitive, and social implications of movement and interaction. In a design process, these methods can utilize various techniques that incorporate human movements by performing or observation, such as sensitizing, bodystorming, and embodied sketching (Márquez Segura et al., 2016). These methods aim to create more intuitive, engaging, and immersive experiences by centering the design process around human movement. Further work focuses here, for example, on greater naturalness of interaction (Wigdor and Wixon, 2011), innovative forms of interaction techniques (Buruk and Özcan, 2018), or experimental approaches (Kajastila et al., 2016).
2.3 Motor and cognitive learning
Motor-cognitive learning within XR applications can benefit from human movement science theories and methodologies to foster effectiveness. Therefore, understanding motor learning is fundamental to comprehending how individuals acquire, refine, and adapt movements to meet specific demands (Krakauer et al., 2019). This process encompasses developing and enhancing motor skills, including mastering new sports techniques and refining coordination. Integral to this understanding is the classification of motor skills, a vital aspect within the broader field of motor learning and movement sciences. A commonly used system for classifying motor skills is the one-dimensional classification system, which categorizes skills based on a single criterion. Differentiation based on the size of primary muscle involvement (gross vs fine motor skills), the beginning and end of movement (discrete vs continuous), and the dependency on environmental context (closed vs open) is conceivable. One-dimensional classification systems offer a simple and straightforward means of categorizing and understanding motor skills. However, they may also have limitations as they might not fully capture the entire spectrum of complexity and diversity inherent in motor skills (Magill and Anderson, 2010). A multidimensional classification scheme for movement tasks, as developed by Gentile (2000), provides a comprehensive framework for understanding task difficulty and optimizing motor learning. This classification scheme represents a differentiation and extension of the distinction between open and closed skills. On one dimension, the variability requirements for movement execution are mapped: (1) neither body (locomotion) nor object manipulation, (2) only object manipulation, (3) only body (locomotion), (4) both object manipulation and body (locomotion) are required. The second dimension maps the requirements concerning the variability of the environmental requirements. These include (1) neither a movement-related variability nor an environment-related variability, (2) only an environment-related variability, (3) only a movement-related variability, and (4) both an environment-related variability and a movement-related variability. This taxonomy helps to determine the degree of difficulty of a task and serves as a guideline for increasing the effectiveness of learning motor skills. By integrating learning phase models with Gentile’s classification system, trainers and professionals can optimize tasks’ difficulty for each phase of the learning process. In the initial stages of learning, where movement quality is relatively low and cognitive impact is high, tasks could be designed to require less variability and focus more on closed skills. As the learner progresses to more advanced learning phases and movement execution becomes more stable and effective, tasks can be designed with greater variability to promote skill adaptability and transferability.
Learning phase models are considered (“stages of skill acquisition”) to determine the learning phase in which a person is situated. Classical models of motor learning generally distinguish three phases: an early cognitive phase, a subsequent associative phase, and a final autonomous phase (Fitts and Posner, 1967). Other three-phase models assign a central function to movement coordination. In these models, the motor learning process is divided into three stages: the development of gross coordination, the development of fine coordination, and the stabilization of fine motor coordination. In his three-stage model, Bernsteĭn (1967) primarily describes changes in the control of degrees of freedom. These changes are as follows: 1. Freezing degrees of freedom; 2. Releasing degrees of freedom; 3. Incorporating and utilizing the mechanical inertia properties of the motor system in the movement. Despite different definitions of the individual learning phases, the learning models have in common that early learning phases are characterized by poor movement quality, high cognitive influence, and dependence on external feedback. Conversely, in the final stages of learning, movement execution becomes stable, effective, and variable. The learning phase a person is in is crucial for the design of the task, that is, for the cognitive requirements.
In addition, Schmidt’s schema theory (Schmidt, 2003) can also play an important role in adjusting the difficulty of tasks. Individuals could improve their adaptability by designing tasks that require different variations within a schema. In the early stages of learning, tasks can limit the variations within a particular schema to provide a solid foundation for movement execution. In more advanced stages, tasks could allow for greater variations within the schema, thus promoting flexibility and adaptability. Schema theory also posits that greater parameter variability (e.g., a variation in the use of force) during practice facilitates schema learning or the targeted use of movement parameters compared to monotonous practice. Two types of motor memory are distinguished: recall schema and recognition schema. The former contains open-loop components and rules for the relationship between initial conditions, parameters for the schema, and the result of the movement. The latter contains closed-loop components and rules for the relationship between initial conditions, sensory consequences, and the result of the movement. From the schema theory arises the importance of variable practice. By practicing different movement situations and contexts, learners strengthen their ability to adapt and execute basic schemata in different situations flexibly. However, the extent of variability must always be considered against the background of the learning phase in which the target group finds itself. Theoretically, it is initially advantageous for beginners to acquire a generalized motor program with low variability. However, increasing variability becomes crucial as the learning process progresses to refine the scheme accordingly (Magill and Anderson, 2010). In addition to program-theoretical approaches, the system dynamics perspective offers valuable insights into the modulation of task complexity. Schöllhorn’s application of system dynamics to differential learning emphasizes that introducing variability in training conditions can enhance motor learning (Schöllhorn et al., 2012; 2015). This is achieved by encouraging exploratory behavior and adaptation among learners. Initially, tasks might incorporate minimal variability to help establish fundamental movement patterns. As learners progress, increasing the variability in tasks promotes motor adaptability and robustness. This approach moves beyond conventional closed-loop models by highlighting the critical role of nonlinear dynamics in motor control. Differential learning prompts learners to explore a range of movement solutions instead of strictly adhering to predefined strategies. This exploration is vital given the complex nature of motor learning processes, where variability in training conditions aids in discovering more effective and adaptable motor strategies (Tassignon et al., 2021). However, it is noteworthy that a recent meta-analysis by Tassignon et al. (2021) on differential learning demonstrated high heterogeneity during the acquisition phase (I2 = 77%) and retention phase (I2 = 79%), along with a high risk of bias in many of the reviewed studies. Consequently, the effect sizes were small (0.26) in the acquisition and medium (0.61) in the retention phase. These findings indicate that differential learning might be more effective in the long term compared to other motor learning methods.
Another approach to effectively managing the cognitive demands in the training process and adapting to the respective learning phase is the inclusion of dual-task (DT) paradigms. The DT paradigm examines a person’s ability to manage several tasks simultaneously and effectively distribute attention resources (Raisbeck and Diekfuss, 2015). After years of training, the concrete planning and execution of complex movement sequences, such as dancing in a group or tying shoes, is mainly unconscious, appears almost effortless, and no longer requires special attention to detail. With a high rate of practice, the cognitive load on the working memory decreases overall, and the corresponding movement sequences become primarily automated. This results in a high resistance to interference, enabling routine movements with little use of cognitive control resources in different contexts (e.g., driving a car on a challenging route while conversing). Performance losses in dual tasks (dual-task costs) are therefore regarded as an indicator of the necessity for attentive control and their reduction as an indication of increasing automation (Schott, 2019). In the DT paradigm, the primary task is the task of interest in the test procedure. In the case of motor learning, this is, therefore, the movement task. A secondary task is employed for testing purposes. This secondary task is typically a cognitively demanding task that requires attention, resources, and working memory. Blischke et al. (2010) found that simultaneous motor and cognitive training improves DT integration but does not always automate movements. Initial DT performance losses disappeared with practice but reappeared with new tasks, challenging full automation. They conclude that DT practice mainly enhances cognitive strategies for simultaneous processing.
Exercise variability involves diverse practice approaches such as schema theory, context interference hypothesis, bilateral practice, and differential learning. Context interference theory suggests that frequent task alternations optimize learning. Serial or randomized task switching benefits both execution parameters and program variations. Varying practice conditions enhance skill transfer to real-life applications (Magill and Hall, 1990). Boutin and Blandin (2010) examined cognitive processes in contextual interference, finding that randomized schedules improve learning outcomes, especially for similar tasks. Task similarity influences cognitive processing and learning outcomes, with practice amount also impacting learning benefits. Task similarity and practice schedule modulate contextual interference’s effects, supporting its role in motor learning. These findings offer insights for designing effective training plans.
3 Materials and methods
This section presents the design of four different prototypes of exergame interventions for motor-cognitive learning in XR. To achieve this, an academic seminar was conducted for students of educational movement science and experience and game design. The co-creative exergame design process model CIEM was used, which we extended with a reflection part to apply CIEM. Furthermore, we transform this abstract model into a formal process by integrating concepts from design thinking. As part of the academic seminar, we assigned advanced methods to the process. We conducted a mixed-methods evaluation with internal and external perspectives to evaluate the formal process and the extended model.
3.1 Expanding the Co-creative Interdisciplinary Exergame Design Process Model
3.1.1 Extended Reflections as a Consumption Part
In order to exploit the EEM potential of exergames, iterative CIEM was used because it combines disciplines related to exertion, game and experience in an interdisciplinary design process. This model establishes an equal footing between the disciplines involved through a co-creation process. In addition to the Creation Part of CIEM, it was necessary to develop another part which improves the prototype design process through evaluation and feedback for further iterations. Therefore, the consumption perspective of the Mechanics Dynamic Aesthetics (MDA) Model (Hunicke et al., 2004) was integrated into the model resulting in two distinct parts: The Creation and the Consumption. The Creation Part consists of the CIEM (Retz et al., 2023a). The Consumption Part is based on the Retz et al. (2023b) perspective, categorizing consumers as experiencers and experts. The expert assessment aims to assess whether and how the objectives specific to each discipline and the overarching objectives have been achieved. The experiencers evaluate the extent to which their needs have been considered. Consequently, Consumption is divided into two categories: those related to XR-training and those focusing on human criteria (see Figure 1).
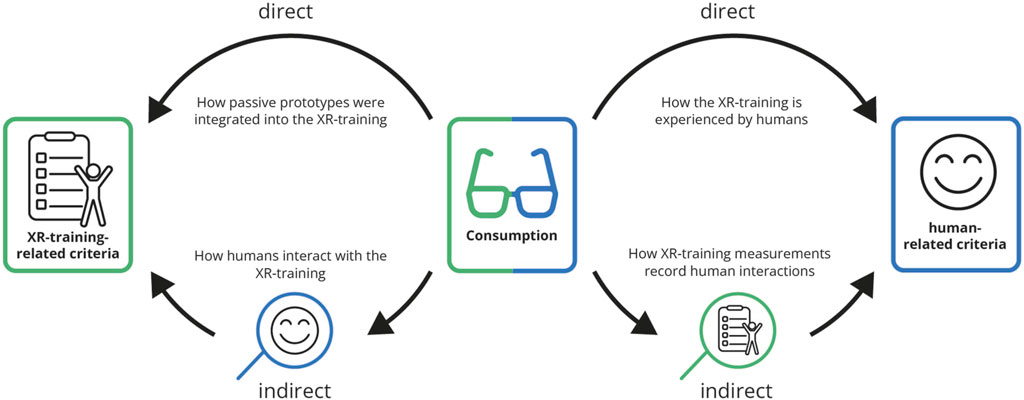
Figure 1. Consumption perspectives of exergame design: The dual pathways for evaluating exergame design. On the left, training-related criteria illustrate how goals are achieved through Consumption. The direct pathway evaluates the integration of passive prototypes, whereas the indirect pathway assesses how individuals interact with the XR-training, which is crucial for experts optimizing the XR-training process. On the right, human-related criteria are depicted, with the direct pathway evaluating individuals’ experiences of the XR-training and the indirect pathway examining the interaction between humans and the XR-training through various measures.
XR-training-related Consumption aims to evaluate the exergame itself, which can be done directly via the training or indirectly via the experiencer. For example, direct XR-training-related Consumption can be assessed via the integration of the training plan or indirectly via the execution of the training by experiencers. Thus, human-related Consumption intends to capture statements about the perceptions of the experiencers, either directly via the experiencers or indirectly through their interaction with the XR-training. For example, direct statements from experiencers are their immediate feelings or reactions post-gameplay. The indirect human-related Consumption can be, for example, the monitoring of a user’s interaction data, such as the number of mistakes they made during the exergame. We call this extended model the Co-creative Interdisciplinary Exergame Design Process Model and Extended Reflection (CIEMER). We illustrate this model in Figure 2.
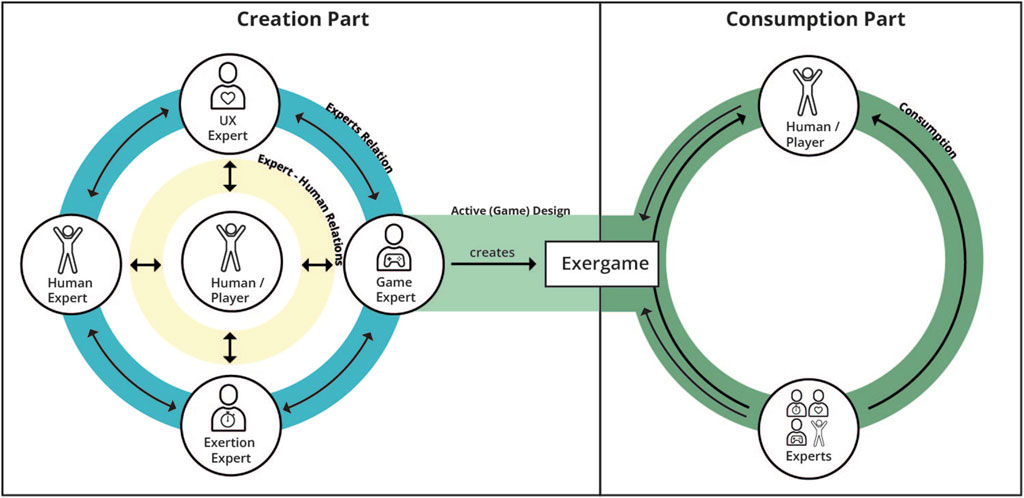
Figure 2. Resulting Co-creative Interdisciplinary Exergame Design Process Model and Extended Reflection (CIEMER) (Modified after Retz et al. (2023a)).
3.1.2 Developing a formal process
In order to render the abstract CIEMER practical, we developed a formal process inspired by the design thinking approach, which enables us to navigate through CIEMER effectively. Given the variability in exergame design following the framework, we assigned a sub-process to each of the three stages: Experts Relation, Expert-Human Relations, and Active Design. These involve one Experts Relation and multiple discipline-specific Expert-Human Relations. These processes can be executed sequentially or in parallel, as illustrated in Figure 3. The interaction between these concurrent process steps is designed to be seamless, enabling potential iterations of the corresponding processes.
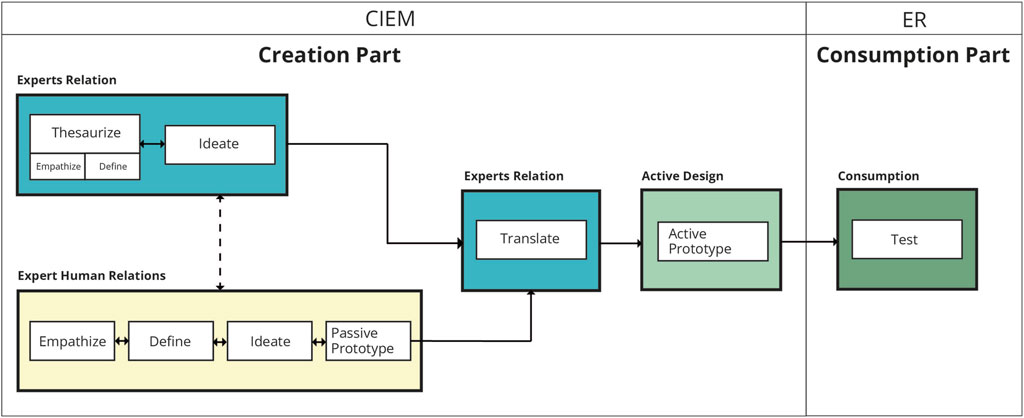
Figure 3. Formal process of the CIEMER: The two main parts are the Creation and Consumption. The Creation Part involves several subprocesses, including Experts Relation, multiple Expert-Human Relations, and Active Design. These are comprised of formal steps designed to create an Active Prototype. The Consumption Part focuses on testing the Active Prototype to gather insights about the XR-training and human experience.
The process begins with the Experts Relation within the Creation Part, which aims to combine the expertise of different disciplines into a common process. This sub-process starts with the phase Thesaurize to create a common thesaurus, establishing a shared language register essential for collaboration. This thesaurus aids in empathizing participants with the topic and understanding the goals and tasks within and between different disciplines. This phase is based on the steps Empathize and Define of the Design Thinking process. The established thesaurus then serves as a foundation for the Ideate phase, which focuses on generating as many shared solutions as possible for the defined goals and tasks. The most promising ideas emerging from this phase are further developed into design artifacts within the Expert Human Relations.
The primary goal of Expert Human Relations is to define and develop discipline-specific goals and artifacts. The sub-processes commence with the phases Empathize, Define, and Ideate, which involve discipline experts understanding their target humans, deriving individual goals, and elaborating ideas. The outcomes and ideas from other disciplines shared in the Experts Relation thesaurus are considered in these phases. Based on this, each discipline develops prototypes, the so-called Passive Prototypes, tailored to address the discipline-specific human problem with the expertise of that discipline. During Passive Prototyping, discipline-specific artifacts such as concepts, sketches, and prototypes are created. These artifacts are then translated into the shared language register in the Translate phase, serving as the Active Design platform.
This translation into construction kits catalyzes Active Prototyping, enabling active designers to distinguish between mandatory and variable artifacts. Through the holistic understanding thus acquired, the active designers perform this task in an iterative process, making Active Design decisions within the construction kits to create the prototype. After creating the exergame prototype, the Consumption Part follows, which consists of the phase test.
3.2 Methods and theories in the Creation Part
The Creation Part describes the process from empathizing to the development of the active prototype. This section outlines methods and theories for the phases of our formal process. These have been further developed to suit interdisciplinary co-creative efforts, with an emphasis on iterative progression through the phases.
3.2.1 Thesaurize: glasses
At the beginning of the Theaurize phase, an overarching concept is the adoption of diverse perspectives. Consequently, we have devised the method glasses, symbolized by using physical glasses. The color of the glasses represents the disciplines involved. The objective extends beyond mutual understanding and promotes self-awareness of personal goals and needs. The glasses act as an instrument that both initiated this self-awareness at an early stage and actively contributed to formulating a shared thesaurus.
3.2.2 Thesaurize: mood setters
The objective of mood setters, based on Márquez Segura et al. (2021b) and Elbæk et al. (2022), is to prepare students mentally, physically, and socially for movement-based design activities. In our adoption, we employed this method to reduce the barrier to social interaction and establish a starting point for a foundation of trust, which is essential for interdisciplinary collaboration and forming a shared thesaurus. The mood setters prioritized the design of exergames in XR and considered the expertise of other individuals as crucial for their realization.
3.2.3 Thesaurize: sensitizing
The method sensitizing (Bowen, 2006; Márquez Segura et al., 2016), aims to access and help designers understand users’ embodied experiences. In our adaptation, the shared thesaurus is refined by deepening the understanding of the user experience from the perspective of the other discipline. The sensitizing method entails adopting t the experience from the first-person view through testing. As a result, the objective is to embrace the perspective of the other discipline to gain insight into the user’s experience during gameplay or training.
3.2.4 Thesaurize: XR personas
The purpose of a persona is to create a fictional yet realistic representation of an individual from a specific target group to understand their needs, goals, behaviors, and preferences (Cooper, 1999). In our adaption, this method was applied in the context of motor-cognitive learning in XR. We developed XR personas to design interdisciplinary XR exergames in a motor-cognitive learning context, in which we included additional categories. These included their familiarity with technology, interests in sports, and motor and cognitive objectives. This was achieved by integrating discipline-specific perspectives.
3.2.5 Thesaurize: value proposition
The purpose of a value proposition is to present a product’s value and benefits to the consumer in a specific statement (Osterwalder et al., 2015). Our focus was on strengthening the Experts Relation by adopting the target group’s perspectives of the Expert Human Relations. By considering both perspectives, the value proposition summarized the persona’s problem statement and solution approach. It should also emphasize one’s role in interdisciplinary work by elaborating the values and benefits of both disciplines in a specific statement. To this end, the value proposition was expanded by an additional clause addressing a second customer pain point, one exertion-based and one experience-based.
3.2.6 Ideate: brainstorming
The Ideate phase started with group brainstorming (Al-Samarraie and Hurmuzan, 2018) sessions. During these sessions, ideas were cultivated from the shared thesaurus to design an exergame with the specific problem statement of the value proposition and objectives.
3.2.7 Ideate: embodied sketching
The objective of embodied sketching (Márquez Segura et al., 2016; Márquez Segura et al., 2021a) is to derive ideas and concepts during the Ideate phase. Embodied sketching emphasizes lived experiences and physical movement concepts by creating sketches with one’s body and using physical materials to visualize thoughts. The essence of this method lies in transforming the social and spatial surroundings into valuable design resources. We enhanced this visual framework, with familiar resources from all disciplines involved, to create a familiar learning atmosphere that illustrates different points of view and makes them understandable for all participants involved in the interdisciplinary project.
3.2.8 Define: modular design brief
The purpose of a design brief is to provide a foundation for a design project (Roto et al., 2021). It is a document containing crucial information and requirements, serving as a communication tool between stakeholders. It establishes parameters such as background, objectives, target audience, and specific design requirements to establish a shared understanding and a clear foundation for the project. In our formal process, we employed a modular design brief. The modular structure of the brief served to provide students with a common foundation that encompasses the fundamental aspects of the disciplines involved. This foundation serves as a starting point for application and offers flexible opportunities for development.
3.2.9 Translate: the shared language register
The aim of the translation process (Retz et al., 2023a) is to translate discipline-specific passive prototype artifacts into a shared language register. This facilitates understanding for active designers. These artifacts, originating from Expert Human Relations, are developed based on the objectives, serving as a construction kit for the exergame design. Here, the construction kits can be divided into mandatory and variable aspects.
3.3 Study design and execution
This section illustrates our approach in applying CIEMER and its formal process of designing four exergame prototypes to facilitate meaningful motor-cognitive learning in XR for different personas. We focused on shaping the Experts Relation to foster interdisciplinary collaboration by implementing the methods described and integrating the Extended Reflection as a component of this iterative process.
3.3.1 Participants and structure
This seminar, a collaborative project between two universities, was aimed at students majoring in user experience and game design, and educational human movement science. The participants consisted of 20 students and graduates from these disciplines, with 10 students from each discipline. They were divided into four different teams.
The academic seminar was hosted at both universities, utilizing facilities such as a seminar room, a games lab, and a sports facility. The seminar structure included a 3-day intensive workshop, a 6-week period of independent work with scheduled feedback sessions, and a 1-week testing phase that concluded with a comprehensive presentation of the results. The seminar was led by the first two authors of this paper.
3.3.2 Course execution
The course started with an intensive 3-day workshop. We introduced the Thesaurize phase of the Experts Relation and the overarching glasses method at the outset. Each student was given a pair of glasses, the color of which represented their area of expertise (Figure 4). This visual cue was designed to heighten awareness of the differing perspectives. At the commencement of each subsequent exercise, students were instructed to don their glasses, thereby becoming aware of their discipline-specific goals and needs. In the following step, students were required to exchange glasses with the other discipline involved. The objective of this procedure was to promote openness and enable students to evaluate their perspectives from alternative viewpoints during the transfer. Furthermore, it was intended to enhance the understanding and appreciation of the expertise of the other discipline.
As a mood setter, we developed an embodied imagination exercise to encourage social interaction and introduce students to XR. In this exercise, students were tasked to imagine an object, express it through body movements, and then “throw” this imaginary object to another student, who then had to identify it. In subsequent exercises and phases, cognitive tasks were added to this activity, such as memorizing or incorporating real objects in various shapes.
We introduced the modular design brief as the next step to create a cohesive foundation for all participants. This brief served as a versatile yet unified structure, accommodating the execution of different projects under a shared conceptual umbrella. It was structured into overarching and discipline-specific categories, representing essential aspects of the two disciplines. These categories included potential options, theories, and approaches that could be combined, developed, and detailed, as shown in Table 1. Our modular design brief adheres to the Effective, Enjoyable, and Meaningful (EEM) approach, aiming to foster an experience that embodies these qualities. Categories under the overarching themes are “Target Group” and “Technology.” For effectiveness, the brief includes “Motor Learning” and “Cognitive Learning” categories. In contrast, “Experience” is the designated category for addressing the elements of enjoyment and meaning.
Two sessions were conducted to encourage sensitizing, one in a games lab and the other in a sports facility (see Figure 5). In the games lab, students could experiment with different exergames and XR applications. Furthermore, the students had to analyze the exergames based on various criteria to understand the components and their translation into the game and technology, as illustrated in Table 2. The aim was to sensitize the students to the translation of elements from the passive prototype into the exergame.
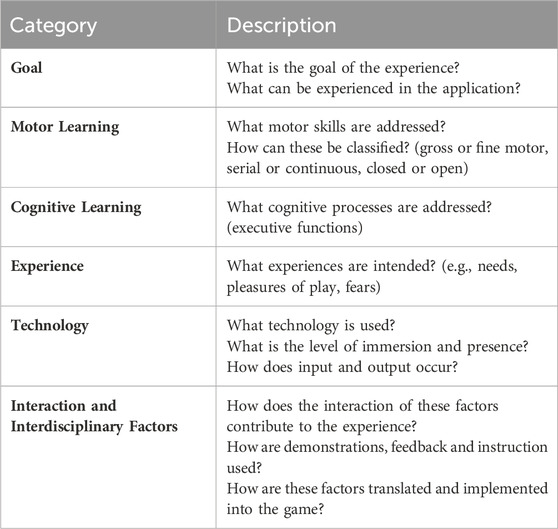
Table 2. Analysis of exergames: Goal, motor learning, cognitive learning, experience, technology, interaction and interdisciplinary factors.
The sensitizing was conducted per the tenets of movement science within the confines of a sports facility. Here, students had the opportunity to explore fundamental motor control and learning concepts of movements corresponding to the three options–throwing and catching, balance and mobility, and dancing—from the modular design brief. Students were furnished with the resources to utilize a plethora of sports equipment for experimentation. Subsequently, each group sensitized themselves to three fundamental exercises for a specific movement concept.
The modular design brief was provided to the participants as a basis for brainstorming. This brief contained five different categories. One element from each category was to be selected and used as the basis for ideation to generate as many ideas as possible. In particular, one group used aspects from the categories target group: older adults, motor learning: balance and mobility, cognitive learning: cognitive flexibility, emotions: family bonding, and technology: MR to derive the idea of an MR—Skiing-Experience. The brainstorming process concluded with selecting one of these ideas as a starting point for subsequent phases.
Following the brainstorming, we proceeded to the next method within the Thesaurize phase, namely, creating personas. In our specific context, we focused on a role-based persona tailored to XR training in sports and health. Each team created this persona collaboratively, considering the perspectives unique to each discipline involved. This development was based on demographic data, familiarity with XR, athletic interests, personality, usage context and everyday life, motivation, and frustration. Individual procedures and goals were derived from these factors. These goals were based on the EEM approach, which addresses motor skills, cognition, and emotion. In this context, it was crucial to differentiate between the lived and the reflected experience of the persona.
Afterwards, each group formulated a value proposition based on the previously created XR persona. This proposition addressed two main “pain points”: the first related to the persona’s motor and cognitive challenges, such as cognitive inflexibility and physical dissatisfaction; the second focused on experience-related issues, such as loneliness. For both types of pain, a corresponding gain for the XR persona was identified and articulated in a collective gain statement.
Progressing to the next stage, we delved deeper into the Ideation phase. To this end, we conducted an embodied sketching session in a sports facility (see Figure 6). The process of developing sketches was divided into three cycles, each comprising a 20-min Creation and a subsequent 10-min Consumption segment. For this purpose, students were given various tools from the human movement science discipline (e.g., sports equipment) and design disciplines (e.g., paper, cardboard, pens). The final ideas from the initial brainstorming and the selected basic exercises from sensitizing served as the starting point for the session. In the initial round, the students constructed an affordance canvas for the selected basic exercises using the material and resources available at the sports facility (Norman, 2013). Team members could be incorporated into the canvas as moving or operational parts. Once the initial Creation segment was completed, one team member from each group was selected to test the sketch and the anticipated experience on another team. The creators of the sketch prioritized the comprehensibility of affordances and the accurate execution of movements. Based on the findings from the Consumption, the sketch was refined and developed further in the second round. The subsequent Consumption assessed whether the integrated improvements accurately represented the intended experiences. It is worth noting that the test subject was not the same as in the initial round. The third round was designed to integrate the targeted technology and the persona’s experiences, such as the limited field of view induced by immersive VR technology. During subsequent Consumption, the tester attempted to emulate the target audience. For illustration, the tester could use a pair of glasses with paper strips on the sides to limit the field of view. Images, videos, and voice notes were recorded to document the results of the sketches, culminating in a compilation of a complete run-through of the sketch.
The students performed their Expert Human Relations using the ideas from the embodied sketching session. The human movement science students considered their group persona from their disciplinary perspective and defined them based on theoretical foundations to develop a training plan. As a result the ideation is focused on the content and timing of tasks.
Using Gentile’s taxonomy, the training plan was adjusted to increase the demands gradually. Taking dance and the persona’s goals of improving bodily awareness and reducing anxiety in social interactions, the students observed the dancer in motion (player in motion) and determined low intertrial variability (unpredictable situations). In order to increase the difficulty level in learning to dance, the students in this group considered the dimensions of this taxonomy by using various techniques and exercises. For example, partner exercises and varying virtual environments were incorporated to increase intertrial variability. In this way, a gradual progression was intended to help the learner master the skill under different conditions.
The DT paradigm explored the ability to manage multiple tasks simultaneously and allocate attentional resources effectively. It was used by a group that wanted to support Parkinson’s patients with daily living activities. The primary task was a motor challenge in which the participants had to walk a path in a park. A cognitive component was introduced as a secondary task. While following the path, players had to memorize the symbols they encountered; these were later quizzed. By applying the DT paradigm, the artifact aimed to minimize the interruption of everyday activities. The secondary task was intended to influence the primary task by shifting attention from maintaining balance (the primary task) to remembering objects. This should facilitate the automation of the primary task. Frequent practice and repetition should reduce motor-cognitive interference and interruptions in DT activities.
The amount of context interference was adjusted to the expertise to avoid over- or under-challenge. For example, the virtual environment and sequence of dance moves changed in the dance context, resulting in different transitions.
After creating the Passive Prototype artifacts, the next task was translating them into a shared language register. For example, the translation of training plans, was achieved by deconstructing the plan into its design components and dynamics using 3D storyboards to create the construction kit. This process involved translating the exercises into a sequence plan and visualizing and describing the execution of movements in the context of essential elements in the gaming environment (see Figure 71). Some groups opted for a detailed textual description of the concepts for their translation. These were divided into objectives, intended effects, and descriptions.
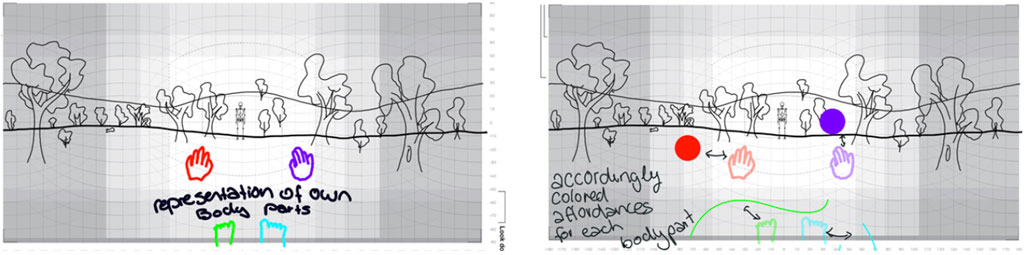
Figure 7. Example of translated training plan artifacts in a 3D storyboard: It highlights the viewer’s visual field, distinguishing between focused and peripheral areas. The background provides the contextual setting, while the foreground features representations of hands and feet, each uniquely colored to represent the viewer’s personal body perspective in this scene. Colored points depicted throughout the scene represent affordances that guide the viewer in learning movement sequences.
In the Active Design phase, each group created two prototypes representing the design status in different fidelity levels, including a conceptual prototype and, in two iterations, an XR prototype. The aim of the conceptual prototype was to visualize ideas simply and efficiently, facilitating rapid iterations and feedback loops. In this context, active designers illustrated how they intend to merge the mandatory artifacts into a unified prototype, and the variable artifacts settled on for this purpose, creating a construction kit 2. Students used simple sketches, storyboards, and 3D templates in our application. One group, aiming to assist adults at risk of falls in recreational activities, created structured sketches and descriptions following the navigation design. This entailed providing design concepts for levels, the difficulty rating of levels, the digital trainer, and introductions to activities, as well as describing and justifying the translation steps from training and effect to affordances and design decisions as displayed in Figure 8.
XR prototyping captured the functionality and basic interactivity of the application. In this stage, artifacts were simulated and integrated into the application using construction kit 2. In our hands-on application, students had the opportunity to implement the XR prototyping (see Figure 9) using various tools. The goal of the second iteration was to present the final concepts holistically. To this end, the XR prototypes from the first iteration were further developed and enhanced in terms of their level of detail. The prototypes were subsequently presented through a self-shot and edited video, demonstrating both the in-game and experiencer perspectives, with their movements and emotions during execution. One team, aiming to promote self-confidence through dancing, demonstrated various 3D worlds using the Unreal Engine to test the emotional experience and the feeling of presence in different environments. Another team, aiming to assist Parkinson’s patients with activities of daily living, designed six playable mini-exergames using DraftXR, all connected through a common hub. The focus here was on interactions, affordances, and navigation in the application.Once the Creation Part was complete, we proceeded to the Consumption Part. This part involved reflection on the Creation process and testing of the prototypes. The objective of our course was to evaluate the integration of the artifacts from construction kit 2 into the prototypes and determine if the exergame prototypes could potentially meet the intended EEM goals. This involved conducting an early user study and the assessment of the prototypes by experts within the discipline. Each group conducted an early player study with ten participants to evaluate human-related criteria. The aim was to assess the usability and comprehensibility of affordances, user flow, and appeal to intended emotions. The NeuroPark group, for example, used the User Experience Questionnaire (UEQ) (Laugwitz et al., 2008) in conjunction with the think-aloud method. This mixed-method approach was employed to test pragmatic qualities such as usability and comprehensibility of affordances while deriving insights into hedonic qualities such as stimulation and originality. The ConfiDance group emphasized the experience of different virtual environments. They employed a mixed-methods approach with a questionnaire based on Carbonell-Carrera et al. (2021), to capture aspects such as presence, motivation, flow, intrinsic motivation inventory, and the different environments.
3.4 Methods for evaluating CIEMER
To evaluate CIEMER, we adopted a mixed-method approach, assessing both the formal process and the extended model. This involved an analysis from two distinct perspectives, integrating external reflections from discipline-specific experts and internal feedback from participating students.
3.4.1 External reflection
The external reflection is conducted to evaluate the CIEMER based on the outcomes of the design process. Discipline-specific experts in exergame design are consulted for this purpose. The experts were one from the field of experience and game design and one from the field of human movement science. Neither of them participated in the Creation Part of the project. These experts review the phases carried out and evaluate the final XR prototypes, which are presented to them through videos and detailed presentations. The focus of the external reflection is on the XR prototypes, with an emphasis on their potential effectiveness and the degree of successful integration of theoretical concepts. This is done through feedback, which is provided verbally. The main points of this feedback are then paraphrased, translated from German into English, and analyzed using qualitative content analysis. For further details, please see Mayring (1994).
3.4.2 Internal reflection
The second part of the evaluation of CIEMER is the internal reflection. This is carried out by the students who created the prototypes using mixed-methods. The primary objective of this evaluation is to assess the applicability of our CIEMER, with a particular focus on interdisciplinary exergame design in XR, from the perspective of the students who participated in its practical application. Following the completion of the practical application, an anonymous survey was conducted. The survey consisted of a questionnaire divided into two sections: first, four questions were asked about interdisciplinary collaboration, and second, three questions related to personal learning outcomes and interest development. The questionnaire comprises five-point Likert scales, with ratings ranging from −2 to +2. The rating options were “strongly agree/very high,” “agree/high,” “neutral,” “disagree/low,” and “strongly disagree/very low.” Descriptive statistics were generated for all variables. A t-test was employed to assess the differences in students’ interest in participating in an interdisciplinary project before and after the academic seminar. This was based on a significance level of α = 0.05. Additionally, students provided written reflections on their experiences during the design process. Quotes from these reflections were translated from German into English.
4 Results
This section describes the XR prototypes developed during the academic seminar. As a contribution to the evaluation of CIEMER, we also describe an external reflection on these prototypes and an internal student reflection on the process using mixed-methods.
4.1 Introducing the final prototypes
ConfiDance is a first-person VR dance exergame designed to assist shy young adults in gaining more confidence in public situations. The goal is to enhance body awareness and diminish apprehension regarding social interactions by dancing in various virtual environments with increasing social components. The final prototype was developed using the Unreal Engine.
NeuroPark is a first-person VR exergame designed to assist older adults with Parkinson’s disease maintain their independence in motor and cognitive activities for as long as possible. It aims to train everyday motor and cognitive tasks (e.g., shopping) and improve activities. This is intended to reduce the loss of autonomy by providing assessment training for activities adapted to the person’s state of health. The final prototype was implemented with Draft XR.
DiscUp is an AR exergame intended to help performance-oriented decathletes optimize their discus-specific skills and abilities. It aims to reduce motor-cognitive interference and stimulate performance motivation by providing AR training focused on improving discus throwing performance through gamified exercises and statistical evaluation of throws. The final prototype was implemented using Figma in conjunction with video simulation.
The Golden Age Games Skiing Experience is an MR exergame designed to help older adults at risk of falling maintain daily mobility and actively participate in life. The exergame aims to counteract cognitive inflexibility and physical dissatisfaction and thereby reduce feelings of loneliness by providing a mobilizing and stimulating skiing experience in a safe MR environment. The final prototype was implemented using Game Studio for the Nintendo Switch.
4.2 External reflection on CIEMER
In the external reflection, the experts evaluated the design process results from their perspective and expertise (summarized in Table 3). For all prototypes, it was found that the implementation of the translated concepts was clearly visible and could be evaluated both individually and across the board. In addition, it was easy to understand why certain concepts were used and why design decisions were made. For example, the expert view clearly showed that the theoretical background chosen for the training plans was not always optimal for the persona. In addition, the extent to which the goals for the exergames were achieved was assessed. For example, from the perspective of human movement science, it was proposed that the designed tasks of the Parkinson exergame, which reference daily life, could assist with activities of daily living. Furthermore, the Active Design of the prototypes was evaluated in terms of design decisions. The application of suitable guidelines and heuristics, as well as the comprehensibility of affordances, were evaluated. It was also assessed whether the selected technological enabler successfully represents the concepts. For example, particular attention was paid to the respective persona and value proposition. Overall, the experts rated the prototypes positively in terms of collaboration and Active Design, such as the main concepts and the personas created. The main criticism related to the Passive Prototypes, such as the transfer of theory into the training plans, concerned the needs of the persona.
4.3 Internal reflection on CIEMER
The data from the students’ internal reflection suggested that there was only moderate interest in participating in an interdisciplinary project before the practical application, represented by a value of 0.41 ± 0.94. One student succinctly articulated their initial reservations: “After being assigned to the course […] I initially approached it with skepticism and had my doubts.” However, after practical application, interest significantly increased (p = 0.00003, t = 5.9) to 1.35 ± 0.79. As depicted in Figure 10 and Figure 11, both “very high” and “high” interest levels rose from 17.7% to 47.1% post-event. The same student commented: “My initial concern that I could not visualize the project was quickly alleviated by new insights. This apprehension transformed into enthusiasm as I delved into a new realm.” Another participant reflected on their experience, stating: “In summary, I can say that I thoroughly enjoyed the course […]. It was more than just an educational event for me.”
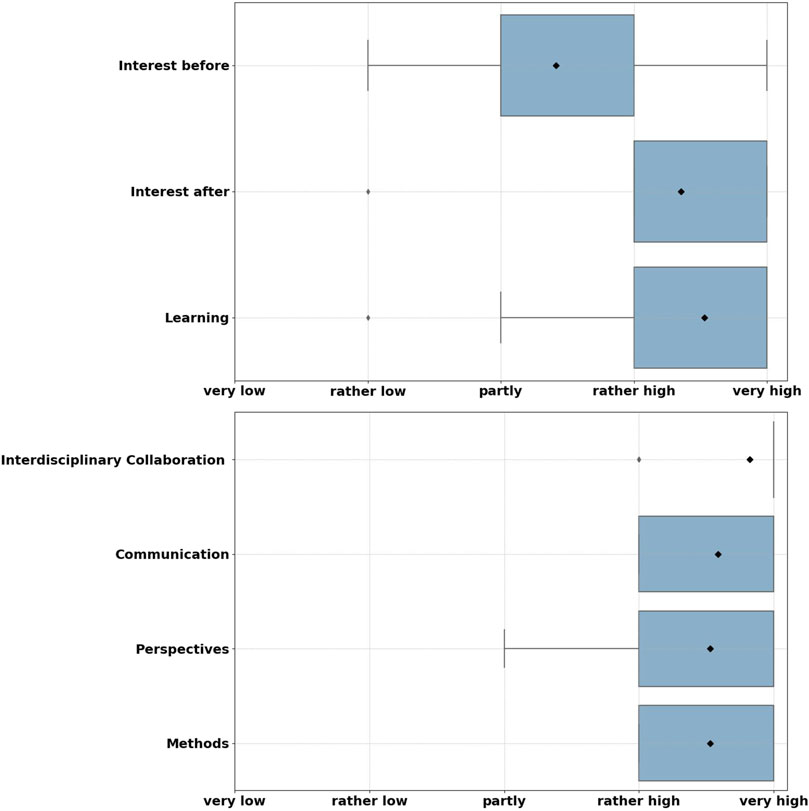
Figure 10. Box plot of responses regarding interdisciplinary collaboration, personal learning outcomes, and interest development from the internal reflection survey conducted among 20 students. The sample includes 10 students specialized in user experience and game design and 10 in educational human movement science. The central box represents the lower and upper quartiles of the distribution, while the diamante within the box indicates the mean value. The whiskers extend to 1.5 times the interquartile range above and below the box, and points outside this range are marked as outliers. The data indicates a significant increase in interest in interdisciplinary projects (p =0.00003).
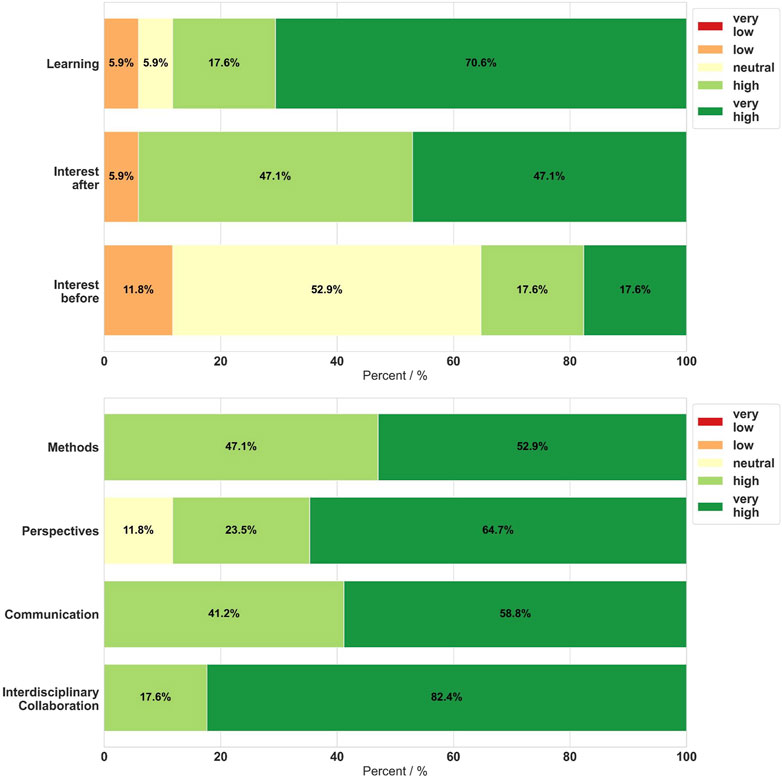
Figure 11. Frequency of a chosen answer of the questions on interdisciplinary collaboration, personal learning outcomes, and interest development from the internal reflection survey conducted among 20 students. The sample includes 10 students specialized in user experience and game design and 10 in educational human movement science.
The learning outcome was rated as very high, with a value of 1.53 ± 0.87. A total of 70.6% and 17.7% of participants rated their learning gain as “very high” and “high,” respectively. One student articulated: “Overall, I learned immensely and am grateful for this experience.” Another expressed: “I gleaned a lot for my future solely from the course’s design.”
Interdisciplinary collaboration was divided into the categories: collaboration, communication, perspectives, and methods. Collaboration was rated at a very high level of 1.87 ± 0.39.82.4% of students strongly agreed with the statement, “The interdisciplinary collaboration between the involved disciplines was very cooperative.” One student stated: “Collaborating within an interdisciplinary team was an enlightening overall experience. While challenges were present, they paved the way to integrate diverse perspectives and skills, leading to innovative solutions that I could not have achieved individually.”
Communication received a very high score of 1.59 ± 0.51.58.8% strongly agreed, and 41.2% agreed that communication between the disciplines worked well. One student elaborated: “Team communication and collaboration functioned effectively […]. Witnessing how team members collaborate efficiently with their varied backgrounds and skills was inspiring.” Another participant added: “I found the interdisciplinary exchange very successful as it broadened my horizons.”
The importance of including different perspectives and expertise received a high rating of 1.53 ± 0.72, with 64.7% of students strongly agreeing. This was supported by the statement: “Incorporating the viewpoints of designers and human movement science experts was pivotal for our project’s success.”
Methods employed during the process were also rated positively, with a score of 1.53 ± 0.51. The ratings exclusively ranged from “strongly agree” (52.9%) to “agree” (47.1%) to the claim that the methods fit into the design process. One student commented: “Despite my initial skepticism about this step of the design process, I believe that without the sensitizing in the sports facility initially, we would not have progressed as far with our prototype.”
5 Discussion
This section discusses CIEMER, examining its practical application and evaluating the resulting exergame prototypes from both external and internal perspectives. It is important to note that the evaluation does not focus on the direct results of the prototypes but rather on the design process model.
5.1 Reflection on CIEMER
The evaluation of our project included both internal and external perspectives on CIEMER. Our approach centered on creating prototypes for motor-cognitive exergame learning, focusing on achieving EEM outcomes.
This necessitated a strong interdisciplinary collaboration, integrating different theories and concepts from multiple disciplines, as highlighted in Retz et al. (2023a). A pivotal component of our model was the creation of a comprehensive thesaurus. This proved instrumental in fostering effective group communication and an open working culture, which was reflected in highly positive evaluations of interdisciplinary collaboration. This collaboration was not perceived as a burden but as an enrichment and fundamental element of the project. Effective communication is key to interdisciplinary work (Nancarrow et al., 2013), and our methods promoted this, particularly by emphasizing the importance of clearly explaining one’s expertise. The Extended Reflection Part aimed to promote interdisciplinary efforts within CIEMER. This approach was well-received by students; who found it compelling. One student suggested it could be a model for future interdisciplinary projects, while another commended its efficiency in managing large-scale interdisciplinary projects. This indicates that students perceive the model as easy to apply and competence-enhancing.
In the external perspective, the focus was on the exergames themselves, providing a lens on the quality of the design process. Experts effectively identified and assessed discipline-specific passive prototype artifacts, indicating the model’s success in addressing the EEM objectives. Furthermore, the process allows to focus on overarching and discipline-specific goals and provides various opportunities to evaluate and iterate their implementation.
In light of these factors and the creation of four potentially effective prototypes, we conclude that CIEMER and its associated processes are potentially sufficient for the design of motor-cognitive exergames.
5.2 Application of CIEMER
In this part, we discuss whether and how the employed methods and the formal process align with the overarching CIEMER.
5.2.1 Use of formal process and methods
The methods employed to build the thesaurus aimed to share expertise and combine it into a common language register. An overarching understanding of the project was derived from the common language register (Retz et al., 2023a). Furthermore, conclusions were drawn regarding the procedure and responsibilities. To this end, the subject-specific mental models were transferred to the physical object of the colored glasses. This facilitated empathizing with the other perspectives, which resulted in the concept of the thesaurus being easily applied. This was demonstrated by the fact that the glasses were repeatedly exchanged independently when using individual methods, such as embodied sketching.
In order to promote sensitizing of interdisciplinary collaboration during the thesaurus phase, targeted sessions were held in the games lab and at the sports facility. The positive experiences of these sensitizing sessions to promote mutual appreciation among discipline experts was apparent in the students’ reflections. They expressed that achieving their goals would not have been possible without the insights and perspectives of the co-designing disciplines. This was further evidenced by the post-sensitizing session activities, where tasks were not only shared but also independently allocated to the expert groups. This demonstrated that the method was effective in promoting sensitization. It is noteworthy that in traditional sensitizing sessions, as discussed in (Márquez Segura et al., 2016), the focus is often on the understanding of the target group’s lived experiences. However, our interdisciplinary sessions broadened the focus to include the needs and experiences of co-designers from different disciplines.
In the Ideate phase, many ideas were generated to identify potential solutions to the identified goals. In this phase, embodied sketching combined familiar tools from the disciplines involved. Familiarity with the sports equipment or the design materials was used to facilitate easy interactions with the tools. This intended effect was observed during the sessions at the sports facility.
The embodied sketching method was implemented to create sketches as efficiently and expediently as possible. To this end, time pressure was used as a motivator, and a constant alternation between Creation and Consumption was used to generate as much input as possible. This approach proved to be an effective and efficient means of generating iterations. One participant stated: “I do not think I have ever developed a concept with a team in such a short amount of time as in this course.” This method was described in all groups as highly productive and essential for interdisciplinary collaboration. It allowed for the practical meeting of perspectives and sketching with familiar, easy-to-use tools, as well as one’s own body.
In the Translate phase, construction kits were build from the passive prototypes. These differentiate between mandatory and variable artifacts from the Expert Human Relations. This ensured that the focus and the intended effects were integrated into the Active Design. The advantage of translating into the shared language register was that potential limitations, such as technological feasibility or conflicting expert passive prototype artifacts, can be identified early. This allows for the goals and artifacts to be iterated before Active Prototyping, as described by the external experts with respect to the training plan.
5.2.2 Overall model and Extended Reflection
The Creation Part of CIEMER, a component derived from CIEM, was conducted four times in our academic seminar, resulting in the creation of four exergame prototypes. The successful completion of these design processes demonstrated the suitability of both the Creation Part and the formal process in producing viable exergame prototypes.
Furthermore, the Extended Reflection allowed the designers to evaluate the potential effectiveness of their prototypes. This part revealed certain deficiencies in the passive prototypes, such as issues with the training plans, as highlighted by the experts’ feedback. Concurrently, user feedback was sought to enhance the prototypes, particularly regarding human-related parameters such as usability.
The CIEMER aims to balance the perspectives of experience and game design with those of human movement science to create EEM in exergames. This approach divers from view-specific approaches like (Toscos et al., 2006; Mueller et al., 2011; Loke and Robertson, 2013; Consolvo et al., 2014; Mueller and Isbister, 2014; Mueller et al., 2018; Márquez Segura et al., 2021b) as it focuses the process of interdisciplinary co-creation. The application of CIEMER and the formal process facilitated the transfer of knowledge and the transition into other relations, while also providing early feedback from diverse perspectives. The resulting analysis of the current state led to numerous quick and specific improvements and iterations, following a fail-fast approach.
It can be posited that other teams may benefit from CIEMER due to its preliminary stages, as it allows interdisciplinary teams to better understand and empathize with each other, which could improve overall results. Furthermore, this model can assist teams in focusing on their specific goals and expertise and structuring their project effectively. A shared thesaurus can enhance interdisciplinary cooperation and create a deeper understanding for project partners. Through Extended Reflection, other teams can utilize CIEMER to optimize and iterate their passive prototypes, thereby identifying gaps and suggest improvements for the Active Design phase.
6 Limitations
As highlighted in the analysis, one significant drawback of the model is its preemptive nature. The model heavily emphasizes the preliminary stages and the creation of Passive Prototype artifacts, consuming substantial resources before initiating Active Design and developing a cohesive exergame prototype. Another limitation concerns the potential evaluator bias. Since the practical application was conducted within an academic seminar, the possibility of bias in survey responses and reflections exists. The student’s performance was evaluated exclusively by assessing the evaluation of the developed exergames, rather than through knowledge or performance tests. Moreover, the influence of instructors on the seminar cannot be evaluated. The focus of the application and evaluation was primarily on the interaction within the Experts Relation. This work demonstrates that the model can be applied to various types of exergames for different target groups. However, whether the model offers advantages in a controlled setting has not been tested. A notable limitation of this study is that the effectiveness of the early-stage prototypes was evaluated only by experts. Consequently, this work does not provide a direct assessment of their effectiveness from a broader perspective, as it was not tested with a sufficiently large sample of actual target group users. As this paper deals with the model’s applicability, it only provides potential, not absolute, statements regarding the fulfilment of EEM objectives.
7 Conclusion
This paper presents an enhanced Co-Creative Interdisciplinary Exergame Design Process Model by incorporating an Extended Reflection Part. This addition improved interdisciplinary collaboration and facilitated rapid iteration of the current state, following a fail-fast approach due to the constant feedback of diverse expressions in a common language. Furthermore, a formal process has been developed to operationalize this theoretical model, making it practically applicable. The process yielded four motor-cognitive learning exergame prototypes in extended realities, which have the potential to be effective for various target groups. Methods specific to this interdisciplinary co-creative process were derived from movement-based, experience-based, and game design. Those methods combined with theories from the disciplines human movement science as well as experience and game design helped to focus the aims of effectiveness, enjoyment and meaning. This was evident by a mixed-methods approach involving external experts and internal student designers. Future work could include employing the model with experienced experts, different team sizes, related disciplines, different disciplines for Active Design, and users as experts of their own needs. Our work focuses primarily on the Experts Relation. Further work should take a detailed look at the Expert Human Relations, e.g., using additional lenses and detailed theoretical sessions.
Data availability statement
The original contributions presented in the study are included in the article/supplementary material, further inquiries can be directed to the corresponding author.
Ethics statement
Ethical approval was not required for the studies involving humans because the results were general insights into teaching methods and content, not involving sensitive personal data, as confirmed by an internal institutional review. The studies were conducted in accordance with the local legislation and institutional requirements. The participants provided their written informed consent to participate in this study.
Author contributions
CR: Conceptualization, Data curation, Investigation, Methodology, Project administration, Writing–original draft, Writing–review and editing, Visualization. TK: Project administration, Writing–review and editing. SG: Supervision, Writing–review and editing. NS: Supervision, Writing–review and editing.
Funding
The author(s) declare that financial support was received for the research, authorship, and/or publication of this article. This research received no external funding. The University of Stuttgart funded the APC through the Institutional Open Access Program (IOAP).
Conflict of interest
The authors declare that the research was conducted in the absence of any commercial or financial relationships that could be construed as a potential conflict of interest.
Publisher’s note
All claims expressed in this article are solely those of the authors and do not necessarily represent those of their affiliated organizations, or those of the publisher, the editors and the reviewers. Any product that may be evaluated in this article, or claim that may be made by its manufacturer, is not guaranteed or endorsed by the publisher.
Footnotes
1https://blog.prototypr.io/vr-paper-prototyping-9e1cab6a75f3
References
Abeele, V. V., Spiel, K., Nacke, L., Johnson, D., and Gerling, K. (2020). Development and validation of the player experience inventory: a scale to measure player experiences at the level of functional and psychosocial consequences. Int. J. Human-Computer Stud. 135, 102370. doi:10.1016/j.ijhcs.2019.102370
Al-Samarraie, H., and Hurmuzan, S. (2018). A review of brainstorming techniques in higher education. Think. Ski. creativity 27, 78–91. doi:10.1016/j.tsc.2017.12.002
Belchior, P., Marsiske, M., Sisco, S., Yam, A., and Mann, W. (2012). Older adults’ engagement with a video game training program. Activities, Adapt. aging 36, 269–279. doi:10.1080/01924788.2012.702307
Blischke, K., Wagner, F., Zehren, B., and Brueckner, S. (2010). Dual-task practice of temporally structured movement sequences augments integrated task processing, but not automatization. J. Hum. Kinet. 25, 5–15. doi:10.2478/v10078-010-0026-1
Boutin, A., and Blandin, Y. (2010). On the cognitive processes underlying contextual interference: contributions of practice schedule, task similarity and amount of practice. Hum. Mov. Sci. 29, 910–920. doi:10.1016/j.humov.2010.07.011
Bowen, G. A. (2006). Grounded theory and sensitizing concepts. Int. J. Qual. methods 5, 12–23. doi:10.1177/160940690600500304
Buruk, O. T., and Özcan, O. (2018). “Extracting design guidelines for wearables and movement in tabletop role-playing games via a research through design process,” in Proceedings of the 2018 CHI Conference on Human Factors in Computing Systems, 1–13.
Carbonell-Carrera, C., Saorin, J. L., and Melián Díaz, D. (2021). User vr experience and motivation study in an immersive 3d geovisualization environment using a game engine for landscape design teaching. Land 10, 492. doi:10.3390/land10050492
Caserman, P., Hoffmann, K., Müller, P., Schaub, M., Straßburg, K., Wiemeyer, J., et al. (2020). Quality criteria for serious games: serious part, game part, and balance. JMIR serious games 8, e19037. doi:10.2196/19037
Chao, Y.-Y., Scherer, Y. K., and Montgomery, C. A. (2015). Effects of using nintendo wiiTM exergames in older adults: a review of the literature. J. aging health 27, 379–402. doi:10.1177/0898264314551171
Chou, Y. (2016) Actionable gamification: beyond points, badges, and leaderboards. California, United States: CreateSpace Independent Publishing Platform, 1–151.
Consolvo, S., Klasnja, P., McDonald, D. W., and Landay, J. A. (2014). Designing for healthy lifestyles: design considerations for mobile technologies to encourage consumer health and wellness. Found. Trends® Human–Computer Interact. 6, 167–315. doi:10.1561/1100000040
Elbæk, L., Lekbo, S., Hansen, R. E., Kaos, M., and Vestergaard, R. (2022). “Mind the gap: the 4m bridge between 4e-cognition and movement-based design,” in ECGBL 2022 16th European Conference on Game-Based Learning (Academic Conferences and publishing limited), 208–215.
Fitts, P. M., and Posner, M. I. (1967) Human performance. Belmont, CA: Brooks/Cole Publishing Company.
Florian‘Floyd’Mueller, D. Y., and Young, D. (2017). “Five lenses for designing exertion experiences,” in Proceedings of the 2017 CHI Conference on Human Factors in Computing Systems.
Galvagno, M., and Dalli, D. (2014). Theory of value co-creation: a systematic literature review. Manag. Serv. Qual. 24, 643–683. doi:10.1108/msq-09-2013-0187
Ge, L., Su, T.-T., An, Y., and Mejía, S. T. (2022). The effectiveness of exergames on fear of falling in community-dwelling older adults: a systematic review. Aging and Ment. Health 26, 1306–1317. doi:10.1080/13607863.2021.1950615
Gentile, A. M. (2000). Skill acquisition: action, movement, and neuromotor processes. Mov. Sci., 111–187.
Gerling, K., Livingston, I., Nacke, L., and Mandryk, R. (2012). “Full-body motion-based game interaction for older adults,” in Proceedings of the SIGCHI conference on human factors in computing systems, 1873–1882.
Hassenzahl, M., Diefenbach, S., and Göritz, A. (2010). Needs, affect, and interactive products–facets of user experience. Interact. Comput. 22, 353–362. doi:10.1016/j.intcom.2010.04.002
Hunicke, R., LeBlanc, M., and Zubek, R. (2004). “Mda: a formal approach to game design and game research,” in Proceedings of the AAAI Workshop on Challenges in Game AI, San Jose, CA, 1722.
Kajastila, R., and Hämäläinen, P. (2015). Motion games in real sports environments. Interactions 22, 44–47. doi:10.1145/2731182
Kajastila, R., Holsti, L., and Hämäläinen, P. (2016). “The augmented climbing wall: high-exertion proximity interaction on a wall-sized interactive surface,” in Proceedings of the 2016 CHI conference on human factors in computing systems (New York, NY: Association for Computing Machinery), 758–769. doi:10.1145/2858036.2858450
Kappen, D. L., Mirza-Babaei, P., and Nacke, L. E. (2019). Older adults’ physical activity and exergames: a systematic review. Int. J. Human–Computer Interact. 35, 140–167. doi:10.1080/10447318.2018.1441253
Krakauer, J. W., Hadjiosif, A. M., Xu, J., Wong, A. L., and Haith, A. M. (2019). Motor learning. Compr. Physiol. 9, 613–663. doi:10.1002/cphy.c170043
Laugwitz, B., Held, T., and Schrepp, M. (2008). “Construction and evaluation of a user experience questionnaire,” in HCI and Usability for Education and Work: 4th Symposium of the Workgroup Human-Computer Interaction and Usability Engineering of the Austrian Computer Society, USAB 2008, Graz, Austria, November 20-21, 2008 (Springer), 63–76.
Leask, C. F., Sandlund, M., Skelton, D. A., Altenburg, T. M., Cardon, G., Chinapaw, M. J., et al. (2019). Framework, principles and recommendations for utilising participatory methodologies in the co-creation and evaluation of public health interventions. Res. Involv. Engagem. 5, 2–16. doi:10.1186/s40900-018-0136-9
Loke, L., and Robertson, T. (2013). Moving and making strange: an embodied approach to movement-based interaction design. ACM Trans. Computer-Human Interact. (TOCHI) 20, 1–25. doi:10.1145/2442106.2442113
Lyons, E. J. (2015). Cultivating engagement and enjoyment in exergames using feedback, challenge, and rewards. Games health J. 4, 12–18. doi:10.1089/g4h.2014.0072
Magill, R., and Anderson, D. I. (2010) Motor learning and control. New York: McGraw-Hill Publishing.
Magill, R. A., and Hall, K. G. (1990). A review of the contextual interference effect in motor skill acquisition. Hum. Mov. Sci. 9, 241–289. doi:10.1016/0167-9457(90)90005-x
Márquez Segura, E., Rogers, K., Martin-Niedecken, A. L., Niedecken, S., and Vidal, L. T. (2021a). “Exploring the design space of immersive social fitness games: the imsofit games model,” in Proceedings of the 2021 CHI Conference on Human Factors in Computing Systems, 1–14.
Márquez Segura, E., Turmo Vidal, L., Rostami, A., and Waern, A. (2016). “Embodied sketching,” in Proceedings of the 2016 CHI Conference on Human Factors in Computing Systems, 6014–6027.
Márquez Segura, E., Turmo Vidal, L., Waern, A., Duval, J., Parrilla Bel, L., and Altarriba Bertran, F. (2021b). “Physical warm-up games: exploring the potential of play and technology design,” in Proceedings of the 2021 CHI Conference on Human Factors in Computing Systems (New York, NY, USA: Association for Computing Machinery). CHI ’21.
Marshall, J., Mueller, F. F., Benford, S., and Pijnappel, S. (2016). Expanding exertion gaming. Int. J. Human-Computer Stud. 90, 1–13. doi:10.1016/j.ijhcs.2016.02.003
Marston, H. R. (2013). Digital gaming perspectives of older adults: content vs. interaction. Educ. Gerontol. 39, 194–208. doi:10.1080/03601277.2012.700817
Martin-Niedecken, A. L., Mahrer, A., Rogers, K., de Bruin, E. D., and Schättin, A. (2020). “hiit” the exercube: comparing the effectiveness of functional high-intensity interval training in conventional vs. exergame-based training. Front. Comput. Sci. 2, 33. doi:10.3389/fcomp.2020.00033
Martin-Niedecken, A. L., Rogers, K., Turmo Vidal, L., Mekler, E. D., and Márquez Segura, E. (2019). “Exercube vs. personal trainer: evaluating a holistic, immersive, and adaptive fitness game setup,” in Proceedings of the 2019 CHI conference on human factors in computing systems, 1–15.
Mayring, P. (1994). “Qualitative inhaltsanalyse,” in Texte verstehen: konzepte, methoden, werkzeuge. Editors A. Boehm, A. Mengel, and T. Muhr (Konstanz: UVK Univ.-Verl. Konstanz), 159–175. vol. 14 of Schriften zur Informationswissenschaft.
Milgram, P., and Kishino, F. (1994). A taxonomy of mixed reality visual displays. IEICE Trans. Inf. Syst. 77, 1321–1329.
Mueller, F., Edge, D., Vetere, F., Gibbs, M. R., Agamanolis, S., Bongers, B., et al. (2011). Designing sports: a framework for exertion games. Proc. sigchi Conf. Hum. factors Comput. Syst., 2651–2660. doi:10.1145/1978942.1979330
Mueller, F., and Isbister, K. (2014). “Movement-based game guidelines,” in Proceedings of the sigchi conference on human factors in computing systems, 2191–2200.
Mueller, F., Matjeka, L., Wang, Y., Andres, J., Li, Z., Marquez, J., et al. (2020). ““ erfahrung and erlebnis” understanding the bodily play experience through German lexicon,” in Proceedings of the Fourteenth International Conference on Tangible, Embedded, and Embodied Interaction, 337–347.
Mueller, F., and Young, D. (2018). 10 lenses to design sports-hci. Found. Trends® Human–Computer Interact. 12, 172–237. doi:10.1561/1100000076
Nancarrow, S. A., Booth, A., Ariss, S., Smith, T., Enderby, P., and Roots, A. (2013). Ten principles of good interdisciplinary team work. Hum. Resour. Health 11, 19–11. doi:10.1186/1478-4491-11-19
Oh, Y., and Yang, S. (2010). “Defining exergames and exergaming,” in Proceedings of meaningful play 2010, 21–23.
Osterwalder, A., Pigneur, Y., Bernarda, G., and Smith, A. (2015) Value proposition design: how to create products and services customers want, Vol. 2. John Wiley and Sons.
Raisbeck, L. D., and Diekfuss, J. A. (2015). Fine and gross motor skills: the effects on skill-focused dual-tasks. Hum. Mov. Sci. 43, 146–154. doi:10.1016/j.humov.2015.08.007
Retz, C., Braun, A., Klotzbier, T. J., Ghellal, S., and Schott, N. (2023a). “Towards co-creative interdisciplinary exergame design processes: a theory-based approach of a vr-exergame fall prevention training,” in Companion Proceedings of the Annual Symposium on Computer- Human Interaction in Play (CHI PLAY ’23 Companion).
Retz, C., Klotzbier, T. J., Ghellal, S., and Schott, N. (2023b). “Interdisciplinary design process of a mixed reality exergame for motor-cognitive health training based on the trail-walking-test,” in 2023 IEEE 11th International Conference on Serious Games and Applications for Health(SeGAH), Athens, Greece, 28-30 August 2023.
Ringgenberg, N., Mildner, S., Hapig, M., Hermann, S., Kruszewski, K., Martin-Niedecken, A. L., et al. (2022). Exerg: adapting an exergame training solution to the needs of older adults using focus group and expert interviews. J. NeuroEngineering Rehabilitation 19, 89–17. doi:10.1186/s12984-022-01063-x
Röglin, L., Martin-Niedecken, A. L., and Ketelhut, S. (2023). “Exercising digitally: a multi-perspective analysis of exergames for physical activity and health promotion,” in Creating digitally: shifting boundaries: arts and technologies—contemporary applications and concepts (Springer), 79–116.
Roto, V., Lee, J.-J., Lai-Chong Law, E., and Zimmerman, J. (2021). “The overlaps and boundaries between service design and user experience design,” in Designing Interactive Systems Conference 2021, 1915–1926.
Ryan, R. M., and Deci, E. L. (2000). Self-determination theory and the facilitation of intrinsic motivation, social development, and well-being. Am. Psychol. 55, 68–78. doi:10.1037/0003-066x.55.1.68
Schmidt, R. A. (2003). Motor schema theory after 27 years: reflections and implications for a new theory. Res. Q. Exerc. sport 74, 366–375. doi:10.1080/02701367.2003.10609106
Schöllhorn, W. I., Eekhoff, A., and Hegen, P. (2015). System dynamics and differential learning. Sportwissenschaft 45, 127–137. doi:10.1007/s12662-015-0366-z
Schöllhorn, W. I., Hegen, P., and Davids, K. (2012). The nonlinear nature of learning-a differential learning approach. Open Sports Sci. J. 5, 100–112. doi:10.2174/1875399x01205010100
Schott, N. (2019). Dual-task performance in developmental coordination disorder (dcd): understanding trade-offs and their implications for training. Curr. Dev. Disord. Rep. 6, 87–101. doi:10.1007/s40474-019-00163-z
Schott, N., and Klotzbier, T. J. (2018). Profiles of cognitive-motor interference during walking in children: does the motor or the cognitive task matter? Front. Psychol. 9, 947. doi:10.3389/fpsyg.2018.00947
Seidler, R. D., and Schott, N. (2015). Mechanismen altersassoziierter abnahmen im motorischen lernprozess. Z. für Sportpsychol. 20, 18–24. doi:10.1026/1612-5010/a000089
Sinclair, J., Hingston, P., and Masek, M. (2009). “Exergame development using the dual flow model,” in Proceedings of the Sixth Australasian Conference on Interactive Entertainment, 1–7.
Skarbez, R., Smith, M., and Whitton, M. C. (2021). Revisiting milgram and kishino’s reality-virtuality continuum. Front. Virtual Real. 2, 647997. doi:10.3389/frvir.2021.647997
Slater, M. (2009). Place illusion and plausibility can lead to realistic behaviour in immersive virtual environments. Philosophical Trans. R. Soc. B Biol. Sci. 364, 3549–3557. doi:10.1098/rstb.2009.0138
Slater, M., and Wilbur, S. (1997). A framework for immersive virtual environments (five): speculations on the role of presence in virtual environments. Presence Teleoperators Virtual Environ. 6, 603–616. doi:10.1162/pres.1997.6.6.603
Subramanian, S., Skjæret-Maroni, N., and Dahl, Y. (2020). Systematic review of design guidelines for full-body interactive games. Interact. Comput. 32, 367–406. doi:10.1093/iwc/iwaa026
Tassignon, B., Verschueren, J., Baeyens, J.-P., Benjaminse, A., Gokeler, A., Serrien, B., et al. (2021). An exploratory meta-analytic review on the empirical evidence of differential learning as an enhanced motor learning method. Front. Psychol. 12, 533033. doi:10.3389/fpsyg.2021.533033
Toscos, T., Faber, A., An, S., and Gandhi, M. P. (2006). Chick clique: persuasive technology to motivate teenage girls to exercise. CHI’06 Ext. Abstr. Hum. factors Comput. Syst., 1873–1878. doi:10.1145/1125451.1125805
Wang, R.-Y., Huang, Y.-C., Zhou, J.-H., Cheng, S.-J., and Yang, Y.-R. (2021). Effects of exergame-based dual-task training on executive function and dual-task performance in community-dwelling older people: a randomized-controlled trial. Games health J. 10, 347–354. doi:10.1089/g4h.2021.0057
Wiemeyer, J. (2020). “Towards a generic framework for serious games,” in Proceedings of the 12th International Symposium on Computer Science in Sport (IACSS 2019) (Springer), 193–200.
Keywords: learning, design process, exergame, interdisciplinary, co-creation, extended reality, motor, cognitive
Citation: Retz C, Klotzbier TJ, Ghellal S and Schott N (2024) CIEMER in action: from development to application of a co-creative, interdisciplinary exergame design process in XR. Front. Virtual Real. 5:1376572. doi: 10.3389/frvir.2024.1376572
Received: 25 January 2024; Accepted: 13 May 2024;
Published: 30 May 2024.
Edited by:
Bastian Kordyaka, University of Bremen, GermanyReviewed by:
Josef Wiemeyer, Darmstadt University of Technology, GermanyMartina Seemann, Chemnitz University of Technology, Germany
Copyright © 2024 Retz, Klotzbier, Ghellal and Schott. This is an open-access article distributed under the terms of the Creative Commons Attribution License (CC BY). The use, distribution or reproduction in other forums is permitted, provided the original author(s) and the copyright owner(s) are credited and that the original publication in this journal is cited, in accordance with accepted academic practice. No use, distribution or reproduction is permitted which does not comply with these terms.
*Correspondence: Celina Retz, c3QxODE2NTNAc3R1ZC51bmktc3R1dHRnYXJ0LmRl