- Center for Ubiquitous Computing, University of Oulu, Oulu, Finland
According to previous research, humans are exceptionally skilled at distinguishing earth-congruent object trajectories from earth-discrepant ones. Inconsistent scale cues in Virtual Reality (VR), however, are shown to easily confuse VR users’ scale, size, and distance estimations. This paper describes the results of two studies, with 40 participants each, which tested the effects of self-scaling, and size cues from active virtual characters, on perceived physics coherence (i.e., subjective realism). In the first study (Study A), a humanoid robot the size of a doll manipulated household objects to demonstrate their rigid body dynamics. The demonstration sequence was performed twice, once using a correct approximation of physics, and once using an incorrect one in which gravity was simulated similarly to as if the doll-sized robot was human-sized and the surrounding room was enlarged. The participants observed both demonstrations, once while standing at the normal scale and once at a reduced scale, similar to that of the humanoid robot. The second study (Study B) was similar, except that the virtual character demonstrating the physics was a regular-sized cat. Our preregistered hypotheses predicted that participants would consider the correct approximation of physics as better matching their expectations at normal scale, and the incorrect one as better matching their expectations at the reduced scale. However, only the second of these hypotheses was supported. According to our exploratory analyses, the participant’s own scale was a poor predictor of physics preference, and instead there was a significant effect regarding the virtual character’s identity. Participants observing the virtual cat were about eight times more likely to select the realistic physics model compared to those observing the humanoid robot. The results indicate that familiar cues tied to virtual character identity overrode any potential effects related to changes in the participants’ own scale.
1 Introduction
Virtual Reality (VR) can provide experiences in which size and distance cues differ significantly from everyday experiences. Self-scaling is an example of this type of experience. For example, changing the virtual interpupillary distance (IPD) in VR alters the visual field so that the perception of sizes and distances scales as well, enabling the illusion of changing one’s own size relative to the world (Kim and Interrante, 2017; Pouke et al., 2020). In addition, body-ownership illusions can cause similar, albeit less intense, effects (Linkenauger et al., 2010; Van Der Hoort et al., 2011; Banakou et al., 2013; Weber et al., 2020). Understanding these effects is becoming more crucial as the body of applications that utilize forms of self-scaling continue to grow. Multiscale Collaborative Virtual Environments (mCVE) are a concept in which multiple VR users can collaborate while users coexist in different scales (Zhang and Furnas, 2005). mCVEs, and self-scaling in general, has been utilized in various applications, such as medical visualizations, collaborative mixed reality systems, and aiding design tasks (Billinghurst et al., 2001; Kopper et al., 2006; Piumsomboon et al., 2018a; Piumsomboon et al., 2018b; Zhang et al., 2020a; Zhang et al., 2020b). Self-scaling has also been utilized as a locomotion method in VR (Krekhov et al., 2018). In the field of robotics, VR has been suggested as a tool for teaching physics phenomena that become relevant (and difficult for users) in small-scale robotics operations (Sitti, 2007; Millet et al., 2008). The relationship between scale and physics is especially fascinating, since physics-related phenomena such as rigid body dynamics and friction at different scales greatly differ from our day-to-day experiences at human-scale (Zhou et al., 2000; Sitti, 2007; Millet et al., 2008; Pouke et al., 2021), and as applications that utilize self-scaling become more prominent, it will be crucial that we accurately characterize their associated perceptual effects so as to avoid unintended consequences. In our previous studies, we focused on how self-scaling using both IPD-based scaling and body-scaling affects our perception on rigid body dynamics (Pouke et al., 2020; Pouke et al., 2021; Pouke et al., 2022). In this paper, we present our results concerning the effects of self-scaling and scale cues on perceiving rigid body dynamics. In this study we specifically focus on how virtual characters can act as scale cues and distort our perceptions of rigid body dynamics of physically simulated objects.
1.1 Perceiving sizes and distances in VR
There has been much scientific interest concerning how humans perceive sizes and distances in VR. According to previous research, these perceptions are not entirely similar to judgments made in everyday life. For example, there is evidence that passability judgments in VR tend to be more conservative compared to real-world judgments (Bhargava et al., 2020). There also exists evidence that humans tend to underestimate egocentric distances in VR (Renner et al., 2013). It appears though that placing users in familiar environments, as well as providing them with familiar size cues in general, can alleviate distortions in the perception of sizes and distances (Renner et al., 2013; Deng and Interrante, 2019). Peillard et al. (2019) found a strong effect according to which objects placed to the sides are systematically perceived as farther away than objects placed directly in front when viewed in VR. Adding size cues in the form of textures and lighting did not change this effect significantly. Martin et al. found that distance perception in VR was significantly affected when earth-discrepant gravity was simulated using different body-orientations while using VR to keep visual stimuli in normal, upright orientation (Martin Calderon and Barnett-Cowan, 2022). In a similar vein, Morfoisse et al. (2024) found actual microgravity during a parabolic flight affecting visual and haptic perception of 3D shapes.
Self-scaling adds a new dimension to the questions of perceiving sizes and distances in VR: how does the VR user perceive which parts of a scale-varying VR experience are “normal-sized” and which ones are not? When faced with conflicting size cues in VR or remote environments, it appears that body cues, both visual as well as proprioceptive, can act as the primary reference metric according to which we compare sizes and distances. As mentioned earlier, previous studies have shown that the illusory ownership of a virtual body of abnormal size can affect size and distance perceptions even when IPD has remained unaltered. This effect is often called the body-scaling effect in VR literature: when inhabiting a small body, sizes and distances are perceived as bigger, and vice versa (Linkenauger et al., 2010; Van Der Hoort et al., 2011; Banakou et al., 2013; Weber et al., 2020). It appears this effect can be achieved by using purely visual (e.g., Weber et al., 2020), as well as purely proprioceptive (e.g., Van Der Hoort and Ehrsson, 2016) cues. The existence of the body-scaling effect implies that when faced with multiple conflicting size cues, the size of our own body (or what we perceive to be the size of our own body) would be the “ground truth” of scale for humans. The results of our previous studies concerning self-scaling and the perception rigid-body dynamics support this claim (Pouke et al., 2020; Pouke et al., 2021). In these studies, we did not directly investigate the perception of sizes and distances but instead focused on the subjective perception of physics while participants were scaled in VR and interacting with physically simulated objects. When judging the realism of multiple physics models simulating object trajectories, participants most often picked the model in which objects behaved as if the participants’ scale was normal and the surrounding environment was scaled instead (Pouke et al., 2020; Pouke et al., 2021, for more details, see section 1.2).
It appears, however, that in some cases, size cues from external virtual characters can similarly act as the main perceptual ruler. Langbehn et al. (2016) investigated the perception of dominant scale in VR by having participants interact in a virtual environment depicting different combinations of size cues. According to their findings, groups of virtual characters could override environmental scale cues and even the scale of the participant’s own avatar; it appears the common scale of these virtual characters acted as the most important scale cue in this study (Langbehn et al., 2016). A somewhat similar, albeit more anecdotal, finding was made in one of our follow-up studies investigating the relationship between scale and rigid-body dynamics (Pouke et al., 2022). In this study, we compared the subjective realism of two physics models when demonstrated by a scaled-down virtual humanoid robot under two body-scaling conditions. Contrary to our hypotheses, the participants preferred a physics model that matched the virtual robot’s scale regardless of the body-scaling condition. Due to this outcome, we reasoned that the humanoid robot was, in fact, the most important scale cue according to which the participants matched their expectations of rigid body dynamics.
1.2 Perceiving rigid body dynamics
According to previous research, humans are typically accustomed to physical phenomena taking place at normal scale, under normal gravity. In their survey, Jörges and López-Moliner (2017) argue that gravity is a strong Bayesian prior for humans; it is rather difficult for humans to interact, or adapt in unstable gravity conditions. For example, McIntyre et al. (2001), as well as Senot et al. (2005) have found evidence according to which humans are more capable at intercepting objects when they accelerate according to normal gravity instead of non-earth-like trajectories. Using an apparatus that synthesized haptic cues simulating a rolling ball inside a tube, Yao and Hayward (2006) observed that their participants were capable of estimating simulated tube lengths in a way that suggests that they utilized an internal model of gravity in addition to direct perceptual cues. Pouke et al. (2024) found evidence that minor temporary adjustments to this internal model gravity could be achieved by using a repetitive throwing task under simulated hypergravity.
However, although humans are accustomed to observing normal gravity at regular scale, this capability is not perfect. Ullman et al. (2017) suggest that our inborn understanding of earth-congruent physics somewhat resembles physics models of contemporary game engines, utilizing reasonable “good-enough” assumptions instead of accurate, always-reliable computations. Mijatović et al. (2014) demonstrated this limitation in a study that investigated predictions of physics for rolling objects following either a linear ramp, or a tautochrone curve in normal and reversed gravity. A tautochrone is a geometric shape that is utilized in, for example, skate parks. The unique property of this curve is that time taken by an object to slide at the bottom of the curve is independent of its starting point. According to their findings, unfamiliarity with this geometric property hindered participants’ ability to make accurate physics predictions. La Scaleia et al. (2020) argue that visual cues, as well as affordances to motor interaction with a visual scene affect perceptual sensitivity to physics in VR.
Since humans are very much accustomed to perceiving rigid body dynamics under normal gravity, physically simulated object behavior has often been used for various purposes in plausibility-related research in VR. The presence-related concept of plausibility has been associated with realism, credibility, and the extent to which the stimuli we perceive in VR match our expectations (Skarbez et al., 2017). More specifically, Slater (2009) and Slater et al. (2022) defined Plausibility Illusion (PSI) as specifically referring to the VR user’s sensation that the events inside the VR experience are actually occurring even though the user knows otherwise. Skarbez, on the other hand, suggested the concept of coherence that roughly corresponds to the content-related factors of the VR experience that either block or lead to PSI, similar to how immersion (hardware support for perceptual stimuli through natural sensorimotor actions) leads to Spatial Presence/Place Illusion (PI). Coherence is therefore described as the extent to which the VR experience’s content is reasonable and matches the user’s expectations. For example, studies by Hofer et al. (2020); Brübach et al. (2022); Skarbez et al. (2020); Pouke et al. (2020, Pouke et al., 2021, Pouke et al., 2022) have used various earth-discrepant physics models and object behavior to purposefully disrupt plausibility in research experiments.
1.3 The relationship between scale and the phenomenological perception of gravity
As mentioned earlier, besides directly changing the perception of sizes and distances, self-scaling affects how a VR user perceives the object trajectories of physically simulated objects Pouke et al. (2020, Pouke et al., 2021). In terms of simulating rigid body dynamics, virtual self-scaling has essentially the same effect as changing gravity; an object falling from a height of 1.5 m reaches the ground in approximately 0.55 s, whereas an object falling from a height of 15 cm reaches the ground in only 0.17 s. This means that simulating rigid body dynamics at 0.1x scale is essentially the same as simulating tenfold gravity, which often appears unnatural for anyone experiencing this type of simulation; accelerations and object velocities appear unnaturally fast, and it becomes difficult to throw objects since they appear to hit the ground as soon as they are released.
In our previous studies (Pouke et al., 2020; Pouke et al., 2021), we investigated participants’ perceptions of rigid body dynamics in VR when users were scaled at 0.1x as well as 10x scale by changing their viewpoint height, virtual IPD, and translational tracking. In both studies, the participant’s task was to drop as well as throw physically simulated objects under two gravity conditions followed by a subjective evaluation of the realism of both models. At 0.1x scale, the participants were interacting with soda can tabs, whereas at 10x scale, the physically simulated objects were logs of timberwork. Unsurprisingly, in both studies, a majority of participants considered a realistic approximation of physics (dubbed True Physics in the original studies) unrealistic and preferred a model in which rigid body dynamics followed the participants’ scale, similar to a situation in which the participant scale had remained unchanged, and the environment was scaled instead. We dubbed this incorrect model Movie Physics, referring to the common convention in Hollywood movies, such as Honey I shrunk the Kids, in which abnormal scales always seem to depict gravity that scales with the main characters (the names of the models were obviously hidden from the participants). These studies still left multiple questions unanswered, such as whether the phenomenon exists when the participants do not perform the interaction tasks themselves but instead observe tiny or large virtual characters interact with objects.
A follow-up study (Pouke et al., 2022) investigated whether the body-scaling effect can similarly bias physics perceptions when other properties, such as IPD and tracking properties, remain at human-scale. The body-scaling effect was elicited similarly as described in the study of Van Der Hoort and Ehrsson (2016), in which participants were leaning back on an exam table, and visuo-synchronous stimuli were used to elicit the illusion of possessing an invisible body. In our study, the participants then observed a scaled-down robot character to perform a similar interaction task that was performed by human participants themselves in the earlier studies Pouke et al. (2020, 2021). In this case, we expected the participants to prefer Movie Physics when body-scaling was taking place, and True Physics when not. Surprisingly however, Movie Physics was again preferred in both conditions, with and without body-scaling. We speculated whether this result was caused by the virtual character demonstrating the physics in that study: the humanoid virtual character at 0.2x scale could have caused a similar effect that was found by Langbehn et al. (2016) according to which virtual characters dictated the perception of dominant scale, and therefore the perception of physics models.
However, since the follow-up study (Pouke et al., 2022) did not provide direct evidence of whether the virtual character was overriding body-size cues, we extended this work with new experiments. In this paper, we present the results of two studies in which participants observed virtual characters performing the physics demonstration task depicted originally in (Pouke et al., 2022). In the first study (Study A), a humanoid robot the size of a doll manipulated objects to demonstrate their rigid body dynamics using True Physics and Movie Physics in a counterbalanced order. The participants observed both demonstrations, once while standing at the normal scale and once at a reduced scale, similar to that of the humanoid robot. The second study (Study B) was similar, except that the virtual character demonstrating the physics was a regular-sized cat. Contrary to our preregistered hypotheses, self-scaling was a poor predictor for preferred physics. However, participants observing the virtual cat were eight times more likely to pick True Physics, suggesting that the identity of the virtual character acted as a stronger cue for perceiving rigid body dynamics. Comparing the results of these studies, we can acquire new knowledge on how environmental cues, such as virtual characters and their scale, can affect the perception of physics realism besides VR user’s own scale.
2 Materials and methods
As described earlier, we utilized two studies in which participants observed a virtual character performing a sequence of interactions demonstrating the rigid body dynamics of virtual soda can tabs. The dataset for Study A was originally collected in the conjunction of (Pouke et al., 2022, see associated preregistration), but was not described in the original paper. In this study, the virtual character was the doll-sized humanoid robot used also in Pouke et al. (2022) (0.2 to human scale, see Figure 1). In Study B, the virtual character performing the physics demonstrations was a regular-sized cat (see Figure 2). The choice for using virtual soda can tabs for the physics demonstration comes from our earlier studies (Pouke et al., 2020; Pouke et al., 2022); we reasoned the tabs would be easily recognizable as familiar, small lightweight objects that one could interact with in our scale of interest. Using the same objects consistently makes our new results more readily comparable to the earlier studies.
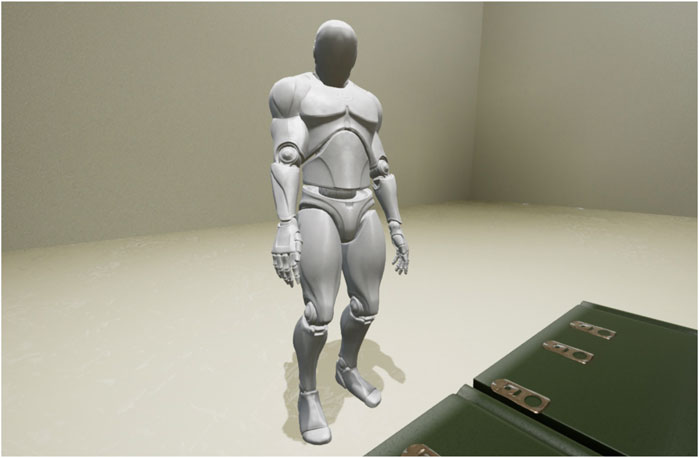
Figure 1. The humanoid robot used in Study A was the default Unreal Engine 4 mannequin performing motion captured animations.
The motivation for using a scaled-down robot and a regular-sized cat as the virtual characters is as follows. Firstly, the scaled-down humanoid allowed us to examine the relationship between the VR user’s own scale and that of a virtual character. Secondly, we chose the humanoid virtual character to resemble a robot since we reasoned it would be less confounding than using a scaled-down human character; a robot-like character could be perceived, for example, as a toy, whereas a scaled-down human would be a more conflicting size cue. For Study B, we chose a regular-sized cat so that this study would have a character that was ordinary-sized yet something that could plausibly interact with the same simulated objects at our scale of interest. In this case, because the cat’s size matched human priors for a cat’s size in this type of environment, there would be little impetus to assume that the character had been scaled in any way.
In each study, the participants repeated the observation task twice, both at normal scale (conditions A1 and B1) and at 0.2 scale (conditions A2 and B2). At each scale, the sequence of interactions was performed twice, demonstrating the two physics models: True Physics and Movie Physics. Similarly to our earlier studies (Pouke et al., 2020; Pouke et al., 2021; Pouke et al., 2022), True Physics refers to a correct approximation of physics whereas Movie Physics refers to a less accurate model in which rigid body dynamics behave as if our scale of interest (0.2) was the normal scale and the environment was five times larger instead. The names of the models were not disclosed to the participants. The participants’ task was to choose which one of the interaction sequences matched their expectations better in terms of object trajectories in each study at each scale. The order of scales as well as physics demonstrations were counterbalanced for participants in both studies (see counterbalancing sheet in Appendix 1 as well as preregistrations for reference). An overview of the Study protocol in each study is summarized in Figure 3.
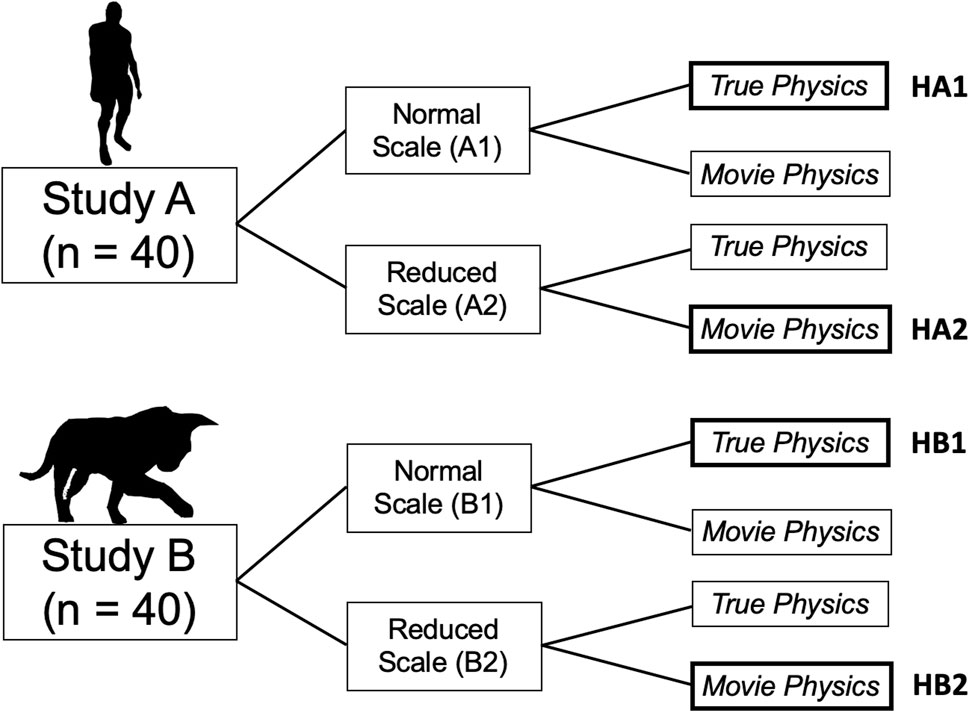
Figure 3. Studies A and B both utilized different sets of participants. Scale conditions and physics conditions were counterbalanced within-subjects. Physics conditions associated with a hypothesis are highlighted.
In total, 80 participants naive for the purposes of the experiments were recruited from the local campus community (Study A, 20 females and 20 males, Study B, 19 females, 21 males). The participants of Study B were pre-screened to prevent those of Study A from participating again. The participants gave written informed consent in accordance with the local Ethics Review Board (ERB). The sample sizes were based on the studies reported in (Pouke et al., 2020; Pouke et al., 2021, 44 and 40 participants, respectively). In Study A, the participants’ ages ranged from 18 to 46, the average age being 27.5 (SD 5.23). In Study B, the participants’ ages ranged from 21 to 40, the average age being 27 (SD 3.97). In both studies, participants self-reported their VR and video game experience using a scale from one (no experience) to seven (daily use); the number of participants giving each response is reported in Table 1.
2.1 Hypotheses
We hypothesized that participants’ own scales would act as the main metric and, therefore, the participants would prefer True Physics when they were themselves at normal scale regardless of the virtual character. Similarly, while scaled-down, the participants would always prefer Movie Physics consistent with our earlier findings. Our preregistered hypotheses are as follows.
The preregistration to hypotheses HA1 and HA2 can be viewed at https://osf.io/4gjv3 (please note that hypotheses HA1 and HA2 correspond to hypotheses H3 and H4 in the preregistration). The preregistration to hypotheses HB1 and HB2 can be viewed at https://osf.io/qnshf.
2.2 Virtual environment
In both studies, the task of the participants was to observe a specific virtual character perform two sequences of interactions with virtual soda can tabs. The sequences took place in the virtual room that was also used in the study reported in Pouke et al. (2022), containing the virtual character and its associated objects in one end, and a door next to two pieces of furniture in the other end (See Figure 4). The apparatus used in Study A was developed in Unreal Engine 4.26.2, whereas Study B used Unreal Engine 5.0.1 to utilize more advanced fur materials. In both studies, the animated sequences were initiated by the researcher after the participant had comfortably adjusted to wearing the headset and could observe the environment naturally.
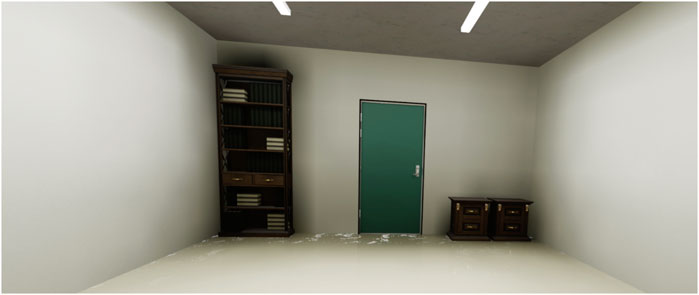
Figure 4. The virtual environment used in both studies was a plain room containing a small number of size cues.
2.3 Animated sequences
In Study A, the tabs were placed on a stack of books. In the animated sequence, a doll-sized humanoid robot picked up and dropped three tabs. Then, two tabs were thrown. The skeletal mesh model of the robot was the default Unreal Engine four mannequin. The animated sequence was the same as the one used in the previous study Pouke et al., 2022: the sequence was captured using the Vive MoCap plugin for Unreal Engine from a researcher physically demonstrating the sequence while wearing Valve Index HMD and controllers, as well as Vive 2.0 Trackers at ankles, hip, chest, and elbows. The picking up, dropping and throwing of the tabs were implemented using the Unreal Engine grab and drop events that are available in the Unreal Engine template VR motion controller implementation meant for interactive manipulation of virtual objects in VR. During grab events, the tabs were attached to a socket placed in the virtual character’s hands, whereas a drop event would release the tab while the tab’s velocity at time of release is carried over. Normally these events are triggered by a VR user using motion controllers. In this implementation, however, the events were programmatically triggered at corresponding frames within the captured animation sequence to mimic human grabbing, dropping and throwing. For the sake of consistency, we used the same assets for the pop tabs that were also used in our previous studies (Pouke et al., 2020; Pouke et al., 2021; Pouke et al., 2022). The rigid body dynamics of tabs after dropping and throwing took place were handled by the internal Unreal Engine physics engine, similarly as if human had performed these actions to physically simulated objects using motion controllers.
In Study B, the tabs were roughly at the same height as in Study A. Instead of stack of books, however, they were placed on a platform containing a cat carrier crate and the virtual cat within. In the animation sequences, the virtual cat emerged from the carrier and proceeded to knock the tabs off the platform. The cat pushed three tabs so that they were dropped next to the platform, after which she used greater strength to knock two tabs further away (to mimic the throwing performed by the humanoid robot). The cat was animated manually using Blender and the Unreal Engine five sequencer, using videos of cats as references. Unlike the humanoid robot, the cat did not utilize ‘grab’ and ‘throw’ events to move the tabs. Instead, the movement of the tabs was simply initiated through the collider in the cat’s animated paw interacting with those in tabs. A figure depicting the virtual characters in each condition can be seen Figure 5. A video demonstration can be viewed at https://youtu.be/01Sx4a2dQZg.
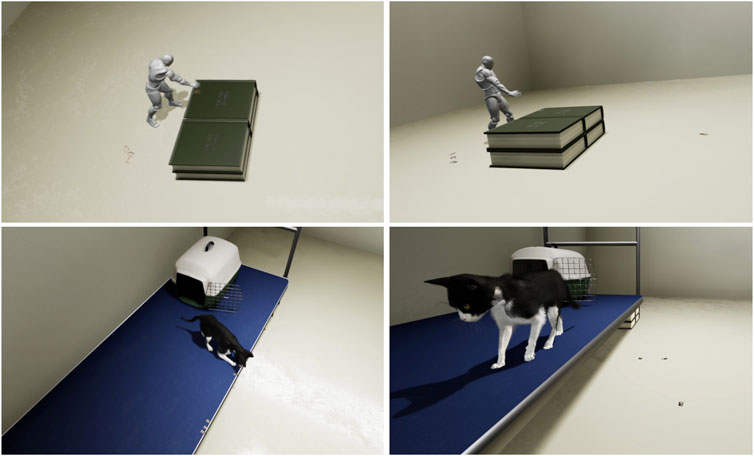
Figure 5. Virtual characters used in the studies at various scales. Upper images demonstrate conditions A1 and A2 whereas lower images demonstrate conditions B1 and B2.
2.3.1 Physics conditions
In both studies, the interaction sequence was repeated with two physics conditions simulated by the Unreal Engine internal physics engine. Using the terminology introduced in (Pouke et al., 2020), these models are called True Physics: a correct approximation of rigid-body dynamics, and Movie Physics in which gravity was scaled at 0.2g, resulting in rigid body dynamics that were similar to if the doll-sized humanoid robot was the size of a human, and the room five times larger. In Study A, the rigid body dynamics were simulated real-time. In Study B, however, initially simulated trajectories were recorded and played as animations for the participants. This was to prevent the excessive randomness in tab trajectories stemming from Unreal Engine physics simulation when developing the apparatus used in Study B1. Both studies A and B used a single animation that was repeated across all physics demonstrations at both scales.
2.3.2 Scale conditions
In both studies, the observation of the animated sequence was carried out both at a normal scale (conditions A1 and B1), as well as at 0.2x scale (conditions A2 and B2). The scaling down of participants was executed by manipulating the Unreal Engine World to Meters parameter, which scales the player character’s viewpoint height, virtual IPD, and translational movement speed.
2.4 Data
The main dependent variable responding to all hypotheses HA1 - HB2 was the response to the forced-choice question “Thinking back how the pull tabs were behaving, which matched your expectations, the first or the second time?” The question was asked verbally after presenting both physics conditions at each scale, and a researcher filled in the participant’s responses to a data sheet.
In both studies, after finishing both scale conditions, the participants removed the VR hardware and filled in a post-experiment questionnaire consisting of the extended Slater-Usoh-Steed presence questionnaire (SUS) (Slater et al., 1994; Usoh et al., 2000) as well as a background questionnaire concerning age, sex, as well as video game and VR experience. The participants were then debriefed and compensated with a gift card worth 20€. For COVID-19 related precautions, all equipment and surfaces were disinfected using alcohol wipes. The Cleanbox device was used to disinfect the HMD between participants2. Researchers were wearing masks throughout both experiments. Masks and hand sanitizer were also available for participants in both studies.
3 Results
3.1 Confirmatory results
In both studies, we predicted that participants would prefer True Physics when they were observing physics demonstrations themselves being at normal scale (hypotheses HA1 and HB1) and Movie Physics when they were at 0.2x scale (hypotheses HA2 and HB2). For the most part, our results did not support these predictions. In Study A, 33 out of 40 participants chose Movie Physics at normal scale (condition A1) whereas 37 out of 40 participants chose Movie Physics while at 0.2x scale (condition A2). In Study B, however, neither model came out significantly more popular than the other. At normal scale, 17 out of 40 participants chose Movie Physics (condition B1) whereas 20 out of 40 chose Movie Physics at 0.2x scale (condition B2). Following the preregistered procedure, we tested each hypothesis HA1, HA2, HB1, and HB2 using an exact binomial test (one-tailed) against the null proportion (20/40). According the results of the tests, hypothesis HA1 was unsupported (condition 1A,
3.2 Exploratory results
Given the strong preference for movie physics in both conditions involving a humanoid (Study A), we tested whether movie physics was also significantly preferred in condition 1A. Contrary to hypothesis HA1, a two-tailed exact binomial test found that this preference was significant
While the within-subjects manipulation of scale had little effect, we were interested in whether the type of virtual character present in the scene produced significantly different patterns of responses between the A and B study participants. Pearson chi-squared tests (with Yates’ continuity correction) revealed that participants were more likely to choose true physics as realistic when physics were demonstrated by the cat virtual character as opposed to the humanoid, at both large (
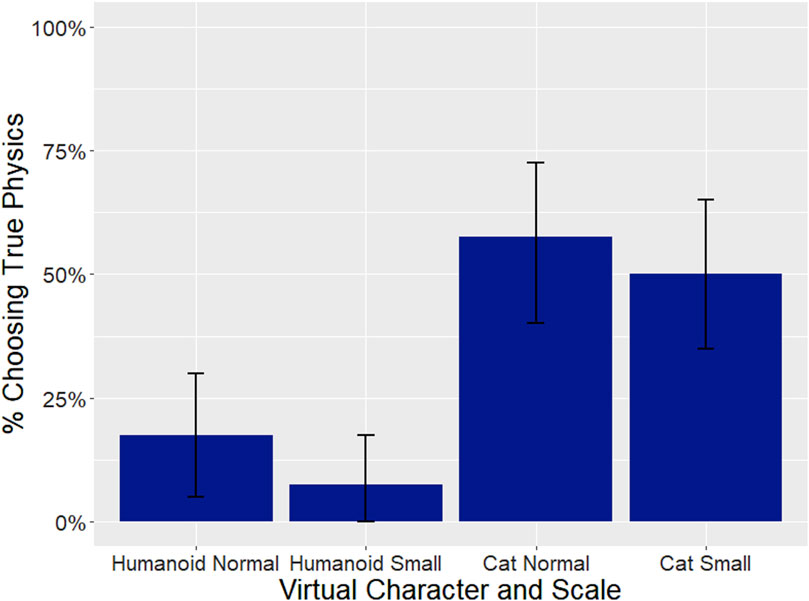
Figure 6. Percentage of “true physics” choices for all conditions. Error bars represent 95% bootstrapped confidence intervals.
Responses choosing True Physics as more realistic were coded as “1” and those choosing Movie Physics were coded as “0,” serving as a response variable for all models, and a random effect was fit to capture variance related to multiple responses deriving from the same participants. Nested models were compared using likelihood-ratio tests and the strength of predictors are reported as slope estimates with 95% confidence intervals. The fully saturated model containing predictors for scale, avatar type, and their interaction, significantly outperformed the null model (
3.2.1 Confound analysis
An effect of familiar size cues of virtual agents on physics realism would be an interesting result, and we thus wanted to check whether there were any other differences that could explain differences in responses, given that different groups of participants experienced the humanoid or the cat demonstrations. Both groups were divided roughly equally in terms of sex (20 women and 20 men in Study A; 19 women and 21 men in Study B). Wilcox ranked-sum tests showed no difference in age (
Using the modeling approach described previously, we tested whether experience using VR could predict physics coherence choices. A model using only VR experience significantly outperformed the null model (
Finally, we investigated demographic information and condition order effects. VR headsets tend to fit men better than women, sometimes leading to worse experiences for women (Stanney et al., 2020), and viewing from a small or large initial scale, or seeing True or Movie physics demonstrations first, could provide an anchoring effect that could bias future responses. None of knowing a participant’s sex, history of videogame experience, presence in the VE as indicated by SUS score, nor knowing which condition dimensions were experienced first could reliably predict physics coherence choices in any model (all
4 Discussion
Although only one out of four hypotheses was supported (HA2), the studies presented here bring new insights regarding physics coherence in virtual environments. Looking at previous studies concerning the coherence of rigid body dynamics at different scales, Movie Physics has been an overwhelmingly popular choice among participants. In a study concerning interactive manipulation of objects at 0.1x scale, Movie Physics was considered the realistic one by roughly 3/4 of participants and as better matching their expectations by 9/10 of participants (Pouke et al., 2020). In a study concerning 10x scale, Movie Physics was similarly considered as the realistic model, although participants were split more evenly concerning which of the models matched their expectations better (Pouke et al., 2021). In a follow-up study, no evidence was found to support the notion that the body scaling effect can affect physics coherence similarly to the IPD and viewpoint height based scaling in the previous studies; surprisingly though, Movie Physics was found to match participants’ expectations better in both conditions this study investigated, perhaps due to the humanoid virtual character used to demonstrate the physics conditions (Pouke et al., 2022). In the studies presented in this paper, the results of Study A were in line with previous ones, especially those presented in Pouke et al. (2022). This is not true, however, for the results of Study B, which suggests that the virtual character demonstrating the rigid body dynamics of objects might indeed act as a cue according to which participants could base their physics judgments.
It appears the virtual cat character could not make True Physics become more popular than Movie Physics, however. Instead, neither physics model was significantly preferred in participant responses. In our exploratory analysis, we also compared participant responses across both studies. According to these results, the participants of Study A were significantly more likely to pick Movie Physics both when the participants were themselves at normal scale or when they were scaled down. Although these results are not conclusive, they lend greater support to the anecdotal finding made in Pouke et al. (2022), according to which a virtual character can act as a size cue when making physics judgments. This is also in line with the finding reported by Langbehn et al. (2016), according to which virtual characters could act as dominant cues when participants were asked to make judgments across conflicting scale cues.
4.1 Implications for coherence in VR
In their article, Skarbez et al. (2020) discussed many open presence-related question, among which was the question regarding manipulation of coherence: “How and to what extent can we manipulate users’ expectations, for example, by “priming” the user with stimuli that are related to those that will appear in the virtual environment?” This question refers to the important question of preventing breaks in PSI by ensuring that the VR content is in line with users’ expectations (Slater et al., 2022). In previous plausibility literature, the effect of context on coherence is usually discussed as the overall setting of the VR experience. This can mean, for example, a sci-fi or fantasy setting in which objectively unnatural behavior, such as dragons or earth-discrepant gravity, would be acceptable by users (Hofer et al., 2020; Skarbez et al., 2020; Slater et al., 2022). In this work, however, we utilized a more subtle manipulation of context that is not related to the overall narrative of the experience, but rather on more general environmental cues. More specifically, the context in which rigid body dynamics of objects were demonstrated, affected their coherence. Although the phenomenon we investigated in this paper is a very particular case of coherence, we believe it can perhaps inspire future solutions for manipulating VR users’ expectations using perceptual cues, and thus, preserving PSI.
4.2 Limitations and suggestions for future work
Our investigation regarding cat conditions 2A and 2B was somewhat limited because we were motivated to keep the study design as similar as possible to earlier data collection related to the humanoid conditions 1A and 1B. For example, the height from which the tabs fell was rather low in this study, which might have affected how easy it was for participants to make physics judgments. In addition, there are no separate hypotheses to realism and expectations or additional exploratory questionnaires concerning object trajectories similar to earlier studies that focused only on one scale (Pouke et al., 2020; Pouke et al., 2021). A future study could utilize a design that is more similar to the aforementioned studies, and could then collect more data regarding either only the scaled-down or normal condition, specifically.
Alternatively, a future study could investigate the effect of virtual characters in a single study with same pool of participants. One limitation regarding our exploratory analysis is that Studies A and B had different samples, which limits the evidence we can draw from analyzing the results together. A follow-up experiment could seek further evidence by using same participants for collecting data regarding both virtual characters.
In addition, a future design could utilize a larger height for dropping the objects, and perhaps a different perspective. The virtual environment in which the cat operates could resemble, for example, a regular house environment instead of the minimalist room that was dictated by the previous designs. Furthermore, using multiple trials (instead of only one animation sequence of five interactions) could be beneficial to examine further whether the judgments stay random when observing the cat for a longer period of time or if the participants would eventually begin to favor one model against the other.
As self-scaling by manipulating virtual height and IPD was a poor predictor for perceived physics realism, it could be that our findings are not specific to VR, but could be observed in non-immersive screen-based applications as well. This is a potential target for a future study.
Finally, Unreal Engine changed its internal physics engine when transitioning from 4 x to 5.0, which introduces a potential confound between studies 1 and 2. However, we believe that the perceptual differences introduced by the experimental manipulation of gravity far outweigh potential perceivable differences in rigid body dynamics simulation between the two physics systems in the context of our studies.
5 Conclusion
We reported the results of two studies investigating the effect of scale cues and virtual characters on physics coherence in virtual environments. In Study A, participants observed a humanoid robot scaled five times smaller demonstrate two models of rigid body dynamics, a realistically approximated model (True Physics) as well a model where gravity was five times weaker (Movie Physics). The participants observed these models twice: while they were at normal scale, as well as when participants themselves were scaled five times smaller similarly to the robot. Study B was otherwise similar, but the virtual character demonstrating the physics models was a regular-sized cat instead. We predicted that in both studies participants would pick True Physics at normal scale and Movie Physics while scaled-down. What we found instead, was that the identity of the virtual characters was a significantly better predictor for participants’ choice of physics model instead of their own scale. In Study A, participants chose Movie Physics significantly more often compared to True Physics at both scales. In Study B, the responses were mixed almost even at both scales. According to our exploratory analyses, the participants were roughly 8 times more likely to favor True Physics when physics were demonstrated by the virtual cat, according to odds ratios estimated by logistic mixed-effects models. Based on the findings in this study, as well those in previous literature (Langbehn et al., 2016; Pouke et al., 2022), we suggest that virtual characters can act as strong scale cues for VR users when making judgements regarding coherence of rigid body dynamics.
Data availability statement
The raw data supporting the conclusions of this article will be made available by the authors, without undue reservation.
Ethics statement
The studies involving humans were approved by The Ethics Committee of Human Sciences of University of Oulu. The studies were conducted in accordance with the local legislation and institutional requirements. The participants provided their written informed consent to participate in this study.
Author contributions
MP and SL devised the overall research scope. MP and EC carried out the experimental design. MP and SP developed the experimental applications in Study A and Study B, respectively. Data was collected by SP and AC. The article was written by MP, EC, and TO. All authors contributed to the article and approved the submitted version.
Funding
The author(s) declare that financial support was received for the research, authorship, and/or publication of this article. This work was supported by the Academy of Finland projects PIXIE 331822, PERCEPT 322637, SRC of Academy of Finland project COMBAT 293389, Business Finland project HUMOR 3656/31/2019, and the European Research Council project ILLUSIVE 101020977.
Acknowledgments
We wish to thank all our participants and cats, Ursula and Lucy.
Conflict of interest
The authors declare that the research was conducted in the absence of any commercial or financial relationships that could be construed as a potential conflict of interest.
Publisher’s note
All claims expressed in this article are solely those of the authors and do not necessarily represent those of their affiliated organizations, or those of the publisher, the editors and the reviewers. Any product that may be evaluated in this article, or claim that may be made by its manufacturer, is not guaranteed or endorsed by the publisher.
Footnotes
1The comparatively larger randomness in trajectories could have resulted from the newer Unreal Engine version utilizing the new Chaos system in place of Nvidia PhysX, however, we have no direct evidence of this.
References
Banakou, D., Groten, R., and Slater, M. (2013). Illusory ownership of a virtual child body causes overestimation of object sizes and implicit attitude changes. Proc. Natl. Acad. Sci. 110, 12846–12851. doi:10.1073/pnas.1306779110
Bhargava, A., Solini, H., Lucaites, K., Bertrand, J. W., Robb, A., Pagano, C. C., et al. (2020). “Comparative evaluation of viewing and self-representation on passability affordances to a realistic sliding doorway in real and immersive virtual environments,” in 2020 IEEE Conference on Virtual Reality and 3D User Interfaces (VR), China, 22-26 March 2020 (IEEE), 519–528.
Billinghurst, M., Kato, H., and Poupyrev, I. (2001). The magicbook: a transitional ar interface. Comput. Graph. 25, 745–753. doi:10.1016/s0097-8493(01)00117-0
Brübach, L., Westermeier, F., Wienrich, C., and Latoschik, M. E. (2022). Breaking plausibility without breaking presence-evidence for the multi-layer nature of plausibility. IEEE Trans. Vis. Comput. Graph. 28, 2267–2276. doi:10.1109/tvcg.2022.3150496
Deng, Z., and Interrante, V. (2019). “Am i floating or not? sensitivity to eye height manipulations in hmd-based immersive virtual environments,” in Acm symposium on applied perception 2019, Spain, 19 - 20 September, 2019, 1–6.
Hofer, M., Hartmann, T., Eden, A., Ratan, R., and Hahn, L. (2020). The role of plausibility in the experience of spatial presence in virtual environments. Front. Virtual Real. 2. doi:10.3389/frvir.2020.00002
Jörges, B., and López-Moliner, J. (2017). Gravity as a strong prior: implications for perception and action. Front. Hum. Neurosci. 11, 203. doi:10.3389/fnhum.2017.00203
Kim, J., and Interrante, V. (2017). “Dwarf or giant: the influence of interpupillary distance and eye height on size perception in virtual environments,” in 27th International Conference on Artificial Reality and Telexistence, ICAT 2017 and the 22nd Eurographics Symposium on Virtual Environments, EGVE 2017, USA, November 22 - 24, 2017 (Eurographics Association), 153–160.
Kopper, R., Ni, T., Bowman, D. A., and Pinho, M. (2006). “Design and evaluation of navigation techniques for multiscale virtual environments,” in Ieee virtual reality conference (vr 2006) (USA: IEEE), 175–182.
Krekhov, A., Cmentowski, S., Emmerich, K., Masuch, M., and Krüger, J. (2018). “Gullivr: a walking-oriented technique for navigation in virtual reality games based on virtual body resizing,” in Proceedings of the 2018 Annual Symposium on Computer-Human Interaction in Play, Australia, October 28 - 31, 2018, 243–256.
Langbehn, E., Bruder, G., and Steinicke, F. (2016). “Scale matters! analysis of dominant scale estimation in the presence of conflicting cues in multi-scale collaborative virtual environments,” in 2016 IEEE symposium on 3D user interfaces (3DUI) (USA: IEEE), 211–220.
La Scaleia, B., Ceccarelli, F., Lacquaniti, F., and Zago, M. (2020). Visuomotor interactions and perceptual judgments in virtual reality simulating different levels of gravity. Front. Bioeng. Biotechnol. 8, 76. doi:10.3389/fbioe.2020.00076
Linkenauger, S. A., Ramenzoni, V., and Proffitt, D. R. (2010). Illusory shrinkage and growth: body-based rescaling affects the perception of size. Psychol. Sci. 21, 1318–1325. doi:10.1177/0956797610380700
Martin Calderon, C., and Barnett-Cowan, M. (2022). “The influence of body orientation relative to gravity on egocentric distance estimates in virtual reality,” in ACM Symposium on Applied Perception 2022, China, 22 - 23 September 2022, 1–13.
McIntyre, J., Zago, M., Berthoz, A., and Lacquaniti, F. (2001). Does the brain model Newton’s laws? Nat. Neurosci. 4, 693–694. doi:10.1038/89477
Mijatović, A., La Scaleia, B., Mercuri, N., Lacquaniti, F., and Zago, M. (2014). Familiar trajectories facilitate the interpretation of physical forces when intercepting a moving target. Exp. brain Res. 232, 3803–3811. doi:10.1007/s00221-014-4050-6
Millet, G., Lécuyer, A., Burkhardt, J.-M., Haliyo, D. S., and Régnier, S. (2008). “Improving perception and understanding of nanoscale phenomena using haptics and visual analogy,” in International Conference on Human Haptic Sensing and Touch Enabled Computer Applications, Germany, 2022. 22-25 May (Springer), 847–856.
Morfoisse, T., Altamira, G. H., Angelini, L., Clément, G., Beraneck, M., McIntyre, J., et al. (2024). Modality-independent effect of gravity in shaping the internal representation of 3d space for visual and haptic object perception. J. Neurosci. 44, e2457202023. doi:10.1523/jneurosci.2457-20.2023
Peillard, E., Thebaud, T., Normand, J.-M., Argelaguet, F., Moreau, G., and Lécuyer, A. (2019). “Virtual objects look farther on the sides: the anisotropy of distance perception in virtual reality,” in 2019 IEEE Conference on Virtual Reality and 3D User Interfaces (VR), China, 23-27 March 2019 (IEEE), 227–236.
Piumsomboon, T., Lee, G. A., Ens, B., Thomas, B. H., and Billinghurst, M. (2018a). Superman vs giant: a study on spatial perception for a multi-scale mixed reality flying telepresence interface. IEEE Trans. Vis. Comput. Graph. 24, 2974–2982. doi:10.1109/tvcg.2018.2868594
Piumsomboon, T., Lee, G. A., Hart, J. D., Ens, B., Lindeman, R. W., Thomas, B. H., et al. (2018b). “Mini-me: an adaptive avatar for mixed reality remote collaboration,” in Proceedings of the 2018 CHI conference on human factors in computing systems, China, April 21 - 26, 2018, 1–13.
Pouke, M., Center, E. G., Chambers, A. P., Pouke, S., Ojala, T., and Lavalle, S. M. (2022). The body scaling effect and its impact on physics plausibility. Front. Virtual Real. 3. doi:10.3389/frvir.2022.869603
Pouke, M., Mimnaugh, K. J., Chambers, A. P., Ojala, T., and LaValle, S. M. (2021). The plausibility paradox for resized users in virtual environments. Front. Virtual Real. 2, 48. doi:10.3389/frvir.2021.655744
Pouke, M., Mimnaugh, K. J., Ojala, T., and LaValle, S. M. (2020). “The plausibility paradox for scaled-down users in virtual environments,” in 2020 IEEE Conference on Virtual Reality and 3D User Interfaces (VR), USA, 22-26 March 2020 (IEEE), 913–921.
Pouke, M., Uotila, E., Center, E. G., Timperi, K. G., Chambers, A. P., Ojala, T., et al. (2024). Adaptation to simulated hypergravity in a virtual reality throwing task. ACM Trans. Appl. Percept. 21, 1–23. doi:10.1145/3643849
Renner, R. S., Velichkovsky, B. M., and Helmert, J. R. (2013). The perception of egocentric distances in virtual environments-a review. ACM Comput. Surv. (CSUR) 46, 1–40. doi:10.1145/2543581.2543590
Senot, P., Zago, M., Lacquaniti, F., and McIntyre, J. (2005). Anticipating the effects of gravity when intercepting moving objects: differentiating up and down based on nonvisual cues. J. Neurophysiology 94, 4471–4480. doi:10.1152/jn.00527.2005
Sitti, M. (2007). Microscale and nanoscale robotics systems [grand challenges of robotics]. IEEE Robotics Automation Mag. 14, 53–60. doi:10.1109/mra.2007.339606
Skarbez, R., Brooks, F. P., and Whitton, M. C. (2017). A survey of presence and related concepts. ACM Comput. Surv. (CSUR) 50, 1–39. doi:10.1145/3134301
Skarbez, R., Brooks, F. P., and Whitton, M. C. (2020). Immersion and coherence: research agenda and early results. IEEE Trans. Vis. Comput. Graph. 27, 3839–3850. doi:10.1109/tvcg.2020.2983701
Slater, M. (2009). Place illusion and plausibility can lead to realistic behaviour in immersive virtual environments. Philosophical Trans. R. Soc. B Biol. Sci. 364, 3549–3557. doi:10.1098/rstb.2009.0138
Slater, M., Banakou, D., Beacco, A., Gallego, J., Macia-Varela, F., and Oliva, R. (2022). A separate reality: an update on place illusion and plausibility in virtual reality. Virtual Real 3, 914392. doi:10.3389/frvir.2022.914392
Slater, M., Usoh, M., and Steed, A. (1994). Depth of presence in virtual environments. Presence Teleoperators Virtual Environ. 3, 130–144. doi:10.1162/pres.1994.3.2.130
Stanney, K., Fidopiastis, C., and Foster, L. (2020). Virtual reality is sexist: but it does not have to be. Front. Robotics AI 7 (4), 4. doi:10.3389/frobt.2020.00004
Ullman, T. D., Spelke, E., Battaglia, P., and Tenenbaum, J. B. (2017). Mind games: game engines as an architecture for intuitive physics. Trends cognitive Sci. 21, 649–665. doi:10.1016/j.tics.2017.05.012
Usoh, M., Catena, E., Arman, S., and Slater, M. (2000). Using presence questionnaires in reality. Presence 9, 497–503. doi:10.1162/105474600566989
Van Der Hoort, B., and Ehrsson, H. H. (2016). Illusions of having small or large invisible bodies influence visual perception of object size. Sci. Rep. 6, 34530–34539. doi:10.1038/srep34530
Van Der Hoort, B., Guterstam, A., and Ehrsson, H. H. (2011). Being barbie: the size of one’s own body determines the perceived size of the world. PloS one 6, e20195. doi:10.1371/journal.pone.0020195
Weber, S., Mast, F. W., and Weibel, D. (2020). Body size illusions influence perceived size of objects: a validation of previous research in virtual reality. Virtual Real. 24, 385–397. doi:10.1007/s10055-019-00402-z
Yao, H.-y., and Hayward, V. (2006). “An experiment on length perception with a virtual rolling stone,” in Proc. Eurohaptics, 325–330.
Zhang, J., Dong, Z., Lindeman, R., and Piumsomboon, T. (2020a). Spatial scale perception for design tasks in virtual reality. Symposium Spatial User Interact., 1–3. doi:10.1145/3385959.3422697
Zhang, J., Piumsomboon, T., Dong, Z., Bai, X., Hoermann, S., and Lindeman, R. (2020b). Exploring spatial scale perception in immersive virtual reality for risk assessment in interior design. Ext. Abstr. 2020 CHI Conf. Hum. Factors Comput. Syst., 1–8. doi:10.1145/3334480.3382876
Zhang, X., and Furnas, G. W. (2005). mcves: using cross-scale collaboration to support user interaction with multiscale structures. Presence 14, 31–46. doi:10.1162/1054746053890288
Keywords: virtual reality, physics, plausibility, coherence, gravity perception
Citation: Pouke M, Pouke S, Center EG, Chambers AP, Ojala T and LaValle SM (2024) The cat is out of the bag: the effect of virtual characters and scale cues on physics coherence. Front. Virtual Real. 5:1250618. doi: 10.3389/frvir.2024.1250618
Received: 30 June 2023; Accepted: 22 July 2024;
Published: 06 August 2024.
Edited by:
Angus Antley, Independent Researcher, Redmond, United StatesReviewed by:
David Lindlbauer, Carnegie Mellon University, United StatesVitor Coluci, State University of Campinas, Brazil
Jhasmani Tito Cruz, State University of Campinas, Brazil, in collaboration with reviewer VC
Copyright © 2024 Pouke, Pouke, Center, Chambers, Ojala and LaValle. This is an open-access article distributed under the terms of the Creative Commons Attribution License (CC BY). The use, distribution or reproduction in other forums is permitted, provided the original author(s) and the copyright owner(s) are credited and that the original publication in this journal is cited, in accordance with accepted academic practice. No use, distribution or reproduction is permitted which does not comply with these terms.
*Correspondence: Matti Pouke, bWF0dGkucG91a2VAb3VsdS5maQ==