- 1Health and Rehabilitation Sciences, Western University, London, ON, Canada
- 2Bone and Joint Institute, Western University, London, ON, Canada
- 3Computer Science, Western University, London, ON, Canada
- 4Kinesiology, Western University, London, ON, Canada
- 5School of Physical Therapy, Western University, London, ON, Canada
Purpose: Investigations of causal theories of neck pain (NP) following motor vehicle crashes (MVC) has been difficult, as simulation is limited. Thus, we sought to evaluate tolerability to a novel virtual reality (VR)-based road collision simulator and screen for adverse reactions.
Materials and Methods: Cross-sectional study. 25 healthy participants were exposed to a novel VR-based rear-end MVC with a small perturbation (0.2 g). The Simulator Sickness Questionnaire (SSQ) and Presence Questionnaire (PQ) were measured post-exposure and adverse reactions were recorded.
Results: The system was well tolerated with no adverse reactions, however one participant reported NP the following day not lasting longer than 48 h. Participants reported low levels of simulator sickness (mean SSQ = 23.49 ± 21.98, range = 0.00 to 89.76; max score = 235.62), while presence (mean PQ = 91.04 ± 14.08, range = 54.00 to 112.00; max score = 133), was lower than literature recommendations.
Conclusion: A VR-based road collision simulator can be safely used to explore the phenomenon of a motor vehicle crashes under controlled circumstances. Future work is needed to optimize the virtual reality environment and to investigate the effects of crash parameters.
Introduction
Evaluation of symptomology (e.g., neck pain) following a motor vehicle crash (MVC), has been historically difficult, though previous investigations have been able to ‘trick’ participants into believing that they have been involved in a MVC (Castro et al., 2001). However, it could be argued that this type of experimental set-up (i.e., blinded car that had been previously impacted), may not have been immersive enough to truly replicate the experience of a MVC. Accordingly, Virtual Reality (VR) has emerged as a technology through which otherwise dangerous situations such as MVCs can be simulated without the risk of biomechanical injury causing tissue damage (Lukacs and Salim, 2018). VR is usually achieved through the combination of a head-mounted display (HMD), head/limb tracking hardware, and a powerful computer to create an immersive three-dimensional environment (Sharar et al., 2008). The creation of an immersive three-dimensional virtual environment can help to create a sense of presence in said environment, which has been defined as the psychological sense of ‘being there’ (Slater et al., 1995). Traditionally, the sense of presence is amplified by enhanced virtual interactivity, greater visual vividness, and multi-sensory input while it is diminished by feelings of simulator sickness and an awareness of the apparatus being used (Lombard and Ditton, 1997; Riches et al., 2019).
We have created an immersive Virtual Reality (VR)-based road traffic collision simulator that also interfaces with a programmable six degrees of freedom robotic platform. While other forms of driving simulators exist, (Beck et al., 2007; Morley et al., 2016; Pietra et al., 2021), ours is the first to our knowledge where a virtual collision is the main intent whereby the participant is situated as a passenger. Also, while previous research has demonstrated the effects of placebo car crashes in lab settings (Castro et al., 2001), ours was specifically created to immerse participants in a scenario designed to imitate the settings of a real-world MVC and elicit feelings of presence. A VR environment that is designed to mimic emotional or stressful environments (i.e., MVC) may elicit greater feelings of presence, and thus may be more appropriate to explore the sensation of involvement in a MVC than traditional laboratory settings (Riva et al., 2007). MVC’s are considered to be a unique stressor in adult life, that can lead to feelings of sudden chaos and a fear of safety, all of which may act as a catalyst toward the development of symptoms like neck pain following a MVC (Walton and Elliott, 2017).
Thus, the purpose of this study was to define the capabilities of our new VR road collision simulator, explore its usability and tolerability, and to examine the development of any adverse reactions (i.e., reports of neck pain longer than 48 h, vomiting, inability to tolerate VR, disorientation). Exploration of development of symptoms following exposure to the VR platform was also deemed relevant as previous research has highlighted the possibility of symptom development following exposure to a placebo car crash (Castro et al., 2001).
Materials and methods
This was a cross-sectional exploratory study with a pre-to-post design.
Participants
Healthy university-level participants were recruited from the community via email and word of mouth between October 2020 and January 2021. Exclusion criteria were recent neck pain, headache, concussion, cardiovascular instability (e.g., heart disease, high or low blood pressure), were actively undergoing cancer treatment, neurological or systemic conditions that affect balance or postural control (e.g., Benign paroxysmal positional vertigo), migraines, visual pathology (e.g., saccades), technophobia, and claustrophobia. This study was approved by a Health Sciences Research Ethics Review Board prior to participation in the study and all participants provided written informed consent prior to participation.
The VISION system
The VISION (Virtual Interface for Stress-Trauma Interactions through Open World Navigation) system is comprised of a HTC Vive (HTC Corporation, Xindian City, Taipei) head mounted VR display (HMD), noise cancelling headphones, and a Mikrolar R3000 (Mikrolar Inc., Hampton, NH, United States) robotic platform (Figure 1). The virtual environment these hardware peripherals interface with depicts a simulated car ride through a city, and the virtual reality environment was developed in the Unity game engine (Unity Technologies, San Francisco, CA, United States) (Figure 1). The visuals of this simulation (targeted at 30–40 frames per second) are delivered by the onboard screens of the HMD which also tracks the participant’s head position via two fixed infra-red base stations, allowing the participant to examine 360 degrees of their surroundings from the passenger seat of the moving vehicle. To maintain a heightened sense of immersion, the simulation’s audio is delivered by a set of noise-cancelling headphones such the volume of all external audio sources is reduced, further emphasizing the sounds of the virtual environment. The simulation is designed such that the movements of the participant’s virtual car are synchronized with a robotic platform which can be controlled along six programmable degrees of freedom: the x-,y-, and z-planes as well as yaw, pitch and roll. Prior to the collision, the robotic platform will simulate the feeling of acceleration and deceleration by modulating its yaw, pitch, and roll to match that of the virtual car. Instructions provided to the robot were designed to not accelerate beyond 1 g for any of the crash scenarios. On pilot testing we determined that for the rear crash scenario the peak accelerations at the head were no greater than 0.2 g. It should also be noted that the cervical spine regularly experiences such accelerations (1 g) in daily life without provoking symptoms (Allen et al., 1994; Castro et al., 2001).
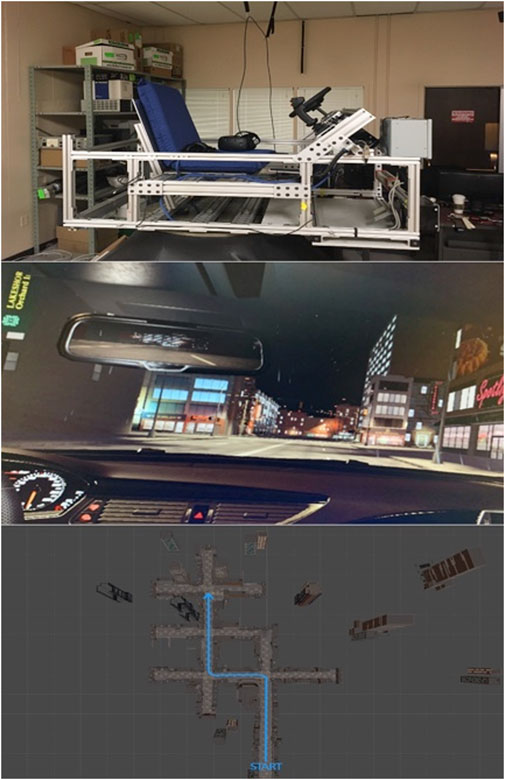
FIGURE 1. VISION Platform, virtual display of participant while using VISION platform and route taken by virtual car during crash route.
The VISION system is capable of multiple different crash scenarios, through manipulation of the following crash parameters: the principal direction of impact (rear-end, side-impact, or front-end), time of day (night or dusk), weather (rain or clear skies), seat position (front passenger, or right/left rear passenger), audio selection (no music or various musical selections), whether or not the glass of the car shatters upon impact, and whether or not the participant receives audio indication that a collision is to take place (e.g., lights and horn of colliding vehicle). For this initial exploration of tolerability to the system, participants were exposed to a low amplitude rear-end collision (0.2 g) at night under clear weather while they sat in the front right passenger seat with no music playing. A night setting was selected for the time of day to help hide any lower fidelity graphical assets. The windshield glass of the virtual car was set to shatter and the participant received an audio indication that a crash was imminent via a simulated car horn played through the earphones. These parameters were selected as they were thought to be the least provocative in nature yet mirror those in rear-end MVCs, for which development of whiplash injuries has been reported to be the most common (Berglund et al., 2003; Dabbour et al., 2020).
Experimental protocol
Prior to their visit with the research team, each participant completed a study-specific demographic questionnaire indicating their sex and age. For the hour before the study, each participant was advised to refrain from any eating, drinking, or physical activity. After having their cervical spines screened, and 5 minutes of quiet sitting, each subject sat in the VISION simulator (Figure 1) during a non-crash route for 5 minutes to become acclimatized to being in a virtual reality simulation and to judge the immersion of the simulator. This non-crash route involved sitting in the front right passenger of a virtual car with it making four turns around a city block with no crash. Seat height of each participant was optimized to their respective height based on personal preference, the time of day was set to night, and no in-simulator music was playing. Each subject was allowed to survey their virtual environment as much as they wished during the virtual drive. Following the 5-min acclimatization period during the non-crash route, subjects were given a minute of rest prior to the next simulation. This rest period consisted of removal of the VR-headset, but participants remained seated in the robotic platform. Immediately following the non-crash simulation, each participant was informed that the subsequent simulation would include a virtual car crash (Figure 1) but were not told when or where (i.e., rear-end collision) it would transpire. Each participant was then exposed to the low-amplitude rear end collision through the VISION platform, that occurred after approximately 1 min of simulated riding in the virtual car. During the crash the robot delivered a small anterior perturbation (0.2 g) to the participant through the car seat to further the immersion of the simulation. Afterwards, the participants dismounted from the simulator, and were observed for 5 minutes to monitor for the presence of any immediate adverse reactions.
Sense of presence and simulator sickness
After this period each participant completed the Presence Questionnaire (PQ), (Witmer and Singer, 1998; UQO Cyberpsychology Lab, 2004), and the Simulator Sickness Questionnaire (SSQ) (Kennedy et al., 1993) to gather initial feedback regarding the immersion of the VISION simulator and to capture any adverse reactions. The PQ has 24 items from 1 to 7 on a Likert scale designed to measure the degree to which a participant feels immersed or present in a virtual simulation. Each item from the Presence Questionnaire is summed with higher scores representing greater immersion (UQO Cyberpsychology Lab, 2004). The individual items from the PQ can also be summed to produce summary scores in the following categories: Realism, Possibility to act, Quality of interface, Possibility to examine, and self-evaluation of performance (UQO Cyberpsychology Lab, 2004). For the present study, questions 20 to 24 were pertinent to sounds and haptic and such were excluded from the study as they were deemed not immediately relevant before study commencement. The 19-item version of the PQ with a maximum score of 133 was selected over the original 32-item questionnaire due in part to its shortened length and good internal consistency (Witmer and Singer, 1998; UQO Cyberpsychology Lab, 2004).
The SSQ is a 16-item survey with responses ranked from 0 to 3 points (Kennedy et al., 1993). The 16 symptoms are placed into three categories (Oculomotor, Disorientation, and Nausea) with unique weights attached and summed to produce a total score, with higher scores indicating a greater degree of nausea with a maximum score of 235.62 (Kennedy et al., 1993; Balk et al, 2013). Total scores above 20 have been suggested to indicate ‘sufficient discomfort’ (Webb et al., 2009). Each participant was instructed to follow-up with the research team should they develop any adverse reactions that lasted beyond 48 h following the protocol. Usability and tolerability were assessed via interpretation of the participant’s scores on both the PQ and SSQ.
Data analysis
Descriptive statistics, including mean, standard deviation and range, were calculated for participant demographics. Data were visualized via box-and-whisker plots and outliers were identified through visual (in reference to interquartile range) and statistical tests of normality. The majority of the measures were non-parametric in nature (Kolmogorov-Smirnov tests). Individual items from the PQ and SSQ were critically examined independently to determine the usability of the VISION platform and to ascertain areas for improvement. SSQ items were recorded as a percentage of the total sample to understand incidence for each of the scores (i.e., none, slight, moderate and severe). As the PQ items do not have a descriptor for each part of the scale, individual items were examined descriptively (mean ± standard deviation). Adverse reactions were recorded throughout the protocol as incident counts of reported neck pain longer than 48 h, immediate vomiting, inability to tolerate the simulator, and self-reported disorientation post-protocol.
Sample size calculation
As this research project was exploratory in nature, no formal sample size calculation was performed, though a small sample of as little as 20 participants has been suggested as appropriate for exploratory studies (Daniel, 2012). A sample of 25 participants was deemed adequate to estimate tolerance to the VISION simulator in otherwise healthy young adults and to identify potential areas for improvement while minimizing risk of exposure to this potentially provocative scenario.
Results
Participants
25 healthy participants (16 male, 9 female) with no recent (3 months) significant trauma or injury that required medical care participated in the study. The mean age of the group was 27.3 ± 4.1 years. All subjects successfully completed all aspects of the protocol and there were no verbal reports of nausea, inability to tolerate VR, and complete disorientation in response to the virtual simulation. There were no reports of neck pain immediately after the simulation. One subject did report neck pain on the day immediately after the virtual simulation, but their symptoms only lasted 1 day. No medical follow-up was required.
Simulator sickness and sense of presence
Results for the Simulator Sickness Questionnaire and Presence Questionnaire and their subscales (mean ± standard deviation, range) are presented in Table 1. In terms of Simulator Sickness, the mean total score was 23.49 ± 21.98 (0.00–89.76) out of a possible 235.62 indicating sufficient discomfort. The majority of participants indicated none to slight for each item of the SSQ items with twelve participants reporting moderate severity of a number of symptoms including nausea and fatigue, and only one participant indicating severe symptoms (eye strain) (Table 2). Mean PQ score was 91.04 ± 14.08 (54.00–112.00) out of a possible 133. Participants rated the ability to control events (mean 2.04 out of 7.00), environmental responsiveness (mean 3.40 out of 7.00) least favorably compared to the remainder of the PQ items (mean 5.00 out of 7.00; Table 3).
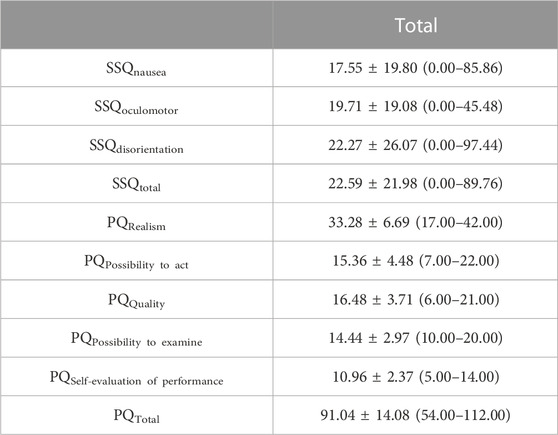
TABLE 1. Simulator Sickness Questionnaire and Presence Questionnaire results post simulation including subscales. Data are presented as mean ± standard deviation with range in brackets.
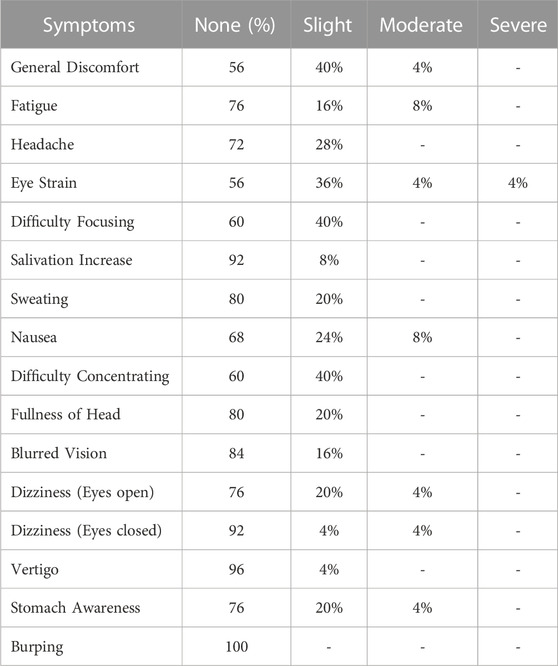
TABLE 2. Simulator Sickness Questionnaire individual items analysis. Data are presented as percentages reported by total sample.
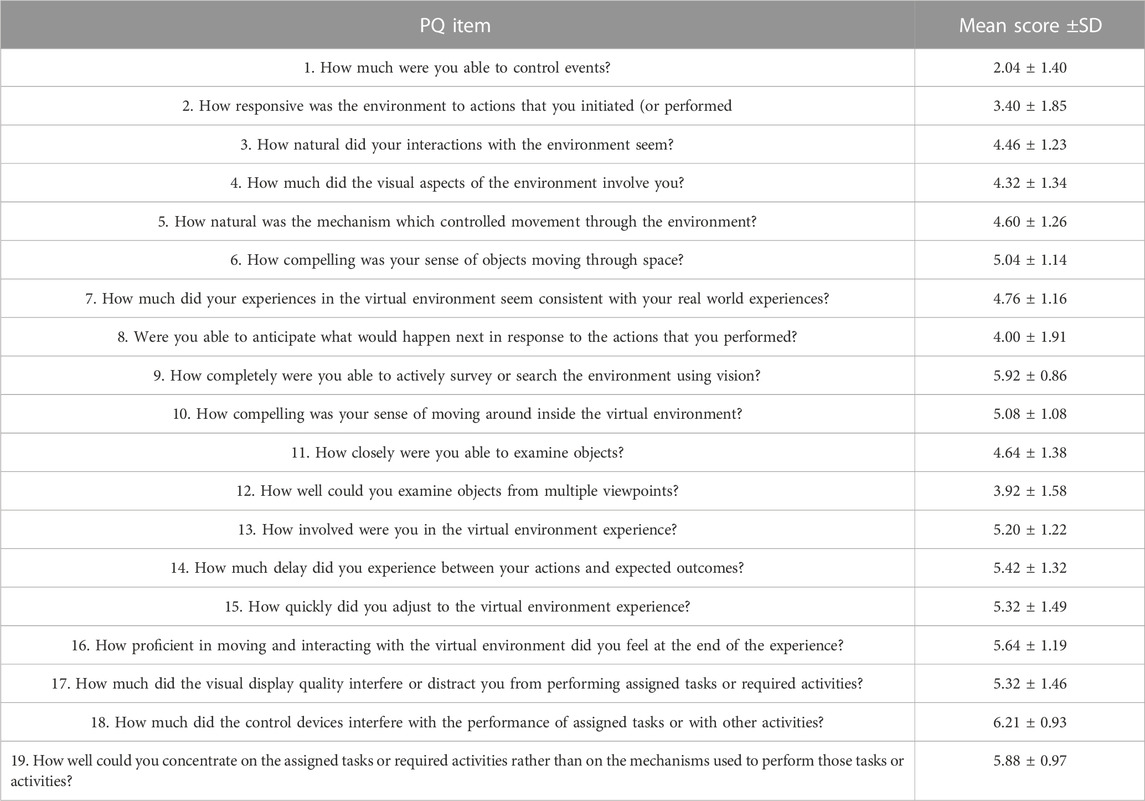
TABLE 3. Presence Questionnaire individual items analysis. Items were rated from 1 to 7, with higher scores representing increased immersion; items 14, 17, and 18 are reversed.
Discussion
The purpose of this study was to explore initial user reactions to a novel virtual reality-based road collision simulator for empirical investigation into potential mechanisms of symptomology following a MVC. We also sought to screen for the presence of any adverse reactions in reaction to the VISION simulator. Our study demonstrated that exposure to a VR-based MVC was well tolerated by most participants, although one participant reported neck pain the following day, which did not last longer than 24 h. The genesis of this work was derived from a criticism regarding previous controllable lab-based motor vehicle crashes, in that the experimental set-up is obviously one that is not placed in a real-world setting (Brault et al., 1998; Castro et al., 2001; Barrett et al., 2021). As a result, VR and a controllable robot was used to help create an immersive experience to mimic the experience of a MVC, without the potential for tissue damage. The benefit of our novel platform is that many different crash parameters can be selected for empirical study, and those that are found to be of the highest relevance can be further developed. While the many settings of the VISION platform have yet to be tested, our goal was to define its capabilities and explore its usability and tolerability in otherwise healthy subjects.
One subject reported severe eye strain in reaction to the simulator; otherwise, the simulator was well tolerated across all other items of the SSQ. The mean SSQ score was 23.49 + 21.98, which based on previous research, indicates ‘sufficient discomfort’ (Webb et al., 2009). However, SSQ score interpretation has faced some contention as the cutoff score of 20.0 has been criticized as too strict in the context of non-aviation VR simulations, nor is it recommended to interpret scores compared to the maximum possible score (235.62) (Bimberg et al., 2020). That being understood, as most of the items on the SSQ were rated as ‘None’ or ‘Slight’, we are reasonably confident that we can conclude that the VISION platform was tolerated well enough. There were no adverse reactions recorded in response to the simulator, allowing us to conclude that the use of VR-based road collision simulator can be safely used to simulate rear-end MVCs in a controlled fashion.
One of the 25 participants (4%) in the present study reported neck pain following exposure to the novel VR-based MVC. We are confident in saying that this was not due to the perturbation delivered by the VISION platform, as the peak accelerations were magnitudes lower than those encountered in daily life. The peak accelerations of the VISION platform were also lower than that of those reported by Fice. (2019) who simulated laboratory based rear-end collisions without VR with peak accelerations of 2.1 g seemingly without incident (Fice, 2019). However, this finding of neck pain stands in stark contrast to the findings of Castro et al. (2001), who found that approximately 20% of their sample developed whiplash like symptoms in exposure to a placebo car crash (Castro et al., 2001). There could be multiple explanations for this finding. In our study, one explanation is that participants were aware that the VISION platform was a simulator, compared to the real-world objects were used to provide a placebo car crash in the study by (Castro et al., 2001). However, as VR has been previously used successfully to treat posttraumatic stress disorder following a MVC, (Beck et al., 2007; Wiederhold and Wiederhold, 2010), it appears that VR is able to replicate near-real virtual representations of traumatic scenarios (Trappey et al., 2021). While the present study was not able to effectively confirm the development of WAD in reaction to a VR-based MVC, it also is not able to refute the theory either. Future work is needed to clarify the development of WAD-like symptoms from simulated collisions.
While our PQ total score (68.4%) was lower than the clinically acceptable amount for VR-based driving simulators for VR-based exposure therapy (80%), we believe that with further refinement PQ scores will improve which may lead to higher incidences of self-reported neck pain and/or other symptoms (Walshe et al., 2005). Another possible explanation for the discrepancy between our findings and that of Castro and others is that the sample in our study was much more limited than (Castro et al., 2001). The psychological profile of our sample was limited to university students aged 18–35 and may not have included participants with a higher tendency for psychosomatic disorders who may be a higher risk for WAD-like symptoms from a simulated MVC without biomechanical potential for injury (Castro et al., 2001).
As the VISION platform is a prototype for simulation of MVCs, visual fidelity of the VR may be partially to blame for the lower-than-desired presence scores. There were occasional visual ‘skipping’ or ‘lag’ episodes where the framerate of the virtual display would drop to an unrealistic level (i.e., below 30 frames per second). These episodes may explain why subjects rated the responsiveness of the virtual environment lower on the PQ but are expected to improve as the framerate of the VISION platform is optimized. Given that industry standard recommendations for VR are to be at least 60 frames per second, further work is required to optimize the VISION platform to minimize any chance of related simulator sickness as a result (Wang et al., 2023).
Limitations
The sample size of this exploratory study was small and as such its conclusions must be interpreted with caution. However, this study sought to seek initial responses to a novel virtual car collision simulator and sought to explore initial reactions and the presence of adverse reactions. As such, we believe we were successful in this endeavor and that the results of the study will help to inform larger study designs to answer additional questions. Another limitation with this work is that we did not control for multiple comparisons as the work was preliminary in nature. However, we feel that due to the exploratory nature of the research the results still hold merit and invite future investigation. As mentioned earlier, we also did not control for subjects with previous virtual reality experience, nor did we control for participants who had been involved in previous car crashes. Both variables could influence the sense of presence and/or simulator sickness in response to the VISION platform. While this study explored the initial usability and tolerability of the VISION platform, formal interviews with future participants may also help to provide further feedback for optimization.
Additionally, there were rare occurrences of the base stations losing track of the participant’s head in 3-D space. As a result, the system had to be re-set to re-track the participant’s location. These tracking errors may have led to lower feeling of presence during the simulation. Again, with further refinement, it is expected that the incidence of these episodes should be greatly reduced. The framerate of the VISION system also requires optimization, as framerates were found to dip below 30 frames per second at times. Lower frame rates could partially explain feelings of simulator sickness. Participants were also made aware that a collision would occur which may have influenced their reaction to the simulator. The VISION platform also only allows for participants to be passengers as opposed to drivers of the virtual vehicle. Examination of the experience from the driver’s perspective is an area for future development, and the same hardware has been used for simulated forklift driving (Dickey et al., 2013). Lastly, we only used a small sub-set of the VISION platform’s settings. As such, it is unclear how the other settings (e.g., direction of impact, in-simulation music, awareness of impending collision) may impact stress, the sense of presence, and simulator sickness.
Conclusion
In conclusion, we have created a virtual-reality based road collision simulator that is capable of simulating MVCs under a controlled fashion for lab-based research. There were no adverse reactions, and all participants tolerated the VISION system well despite one participant reporting neck pain the following day. It appeared that the sense of presence may be negatively associated with simulator sickness. Sense of presence was not as high as we would have hoped, and opportunities for improvement include improvement of framerates to increase the responsiveness of the simulator, and better tracking of the HMD used in the simulator. Future research should examine the effects of combining simulator settings (e.g., front end collision with no audio warning) to explore other adverse reactions and the sense of presence.
Data availability statement
The raw data supporting the conclusions of this article will be made available by the authors, without undue reservation.
Ethics statement
The studies involving human participants were reviewed and approved by the Health Sciences Research Ethics Review Board at Western University. The patients/participants provided their written informed consent to participate in this study.
Author contributions
ML was responsible for study design, data collection, data analysis and manuscript preparation. MB assisted with study design and manuscript preparation. CWJM, JD, and DW all assisted with study design, data analysis, and provided feedback on the manuscript. All authors contributed to the article and approved the submitted version.
Funding
ML was supported in part by a Transdisciplinary Training Award from the Bone and Joint Institute at Western University, Canada. This work was also supported by a Catalyst Grant from the Bone and Joint Institute at Western University, Canada awarded to DW and JD. Funding for the open access publication fees were received from a Western Strategic Support for NSERC Success awarded to DW.
Acknowledgments
This work was conducted as part of the PhD thesis for ML at Western University.
Conflict of interest
The authors declare that the research was conducted in the absence of any commercial or financial relationships that could be construed as a potential conflict of interest.
Publisher’s note
All claims expressed in this article are solely those of the authors and do not necessarily represent those of their affiliated organizations, or those of the publisher, the editors and the reviewers. Any product that may be evaluated in this article, or claim that may be made by its manufacturer, is not guaranteed or endorsed by the publisher.
References
Allen, M. E., Weir-Jones, L., Motiuk, D. R., Flewin, K. R., Goring, R. D., Kobetitch, R., et al. (1994). Acceleration perturbations of daily living. Spine 19 (11), 1285–1290. doi:10.1097/00007632-199405310-00017
Balk, S. A., Bertola, M. A., and Inman, V. W. (2013). “Simulator sickness questionnaire: Twenty years later” In: Proceedings of the Seventh International Driving Symposium on Human Factors in Driver Assessment Bolton, June 17-20, 2013, 257–263.
Barrett, J. M., Fewster, K. M., and Callaghan, J. P. (2021). Model-aided design of a rear-impact collision testing system for in vivo investigations. J. biomechanical Eng. 143 (9), 094502. doi:10.1115/1.4050909
Beck, J. G., Palyo, S. A., Winer, E. H., Schwagler, B. E., and Ang, E. J. (2007). Virtual reality exposure therapy for PTSD symptoms after a road accident: An uncontrolled case series. Behav. Ther. 38 (1), 39–48. doi:10.1016/j.beth.2006.04.001
Berglund, A., Alfredsson, L., Jensen, I., Bodin, L., and Nygren, Å. (2003). Occupant- and crash-related factors associated with the risk of whiplash injury. Ann. Epidemiol. 13 (1), 66–72. doi:10.1016/s1047-2797(02)00252-1
Bimberg, P., Weissker, T., and Kulik, A. (2020). “On the usage of the simulator sickness questionnaire for virtual reality research,” in Proceedings - 2020 IEEE Conference on Virtual Reality and 3D User Interfaces, VRW 2020 (Atlanta, GA: Institute of Electrical and Electronics Engineers Inc), 464–467.
Brault, J. R., Wheeler, J. B., Siegmund, G. P., and Brault, E. J. (1998). Clinical response of human subjects to rear-end automobile collisions. Arch. Phys. Med. Rehabil. 79, 72–80. doi:10.1016/s0003-9993(98)90212-x
Castro, W. H. M., Meyer, S. J., Becke, M. E. R., Nentwig, C. G., Hein, M. F., Ercan, B. I., et al. (2001). No stress - no whiplash? Int. J. Leg. Med. 114 (6), 316–322. doi:10.1007/s004140000193
Dabbour, E., Dabbour, O., and Martinez, A. A. (2020). Temporal stability of the factors related to the severity of drivers’ injuries in rear-end collisions. Accid. analysis Prev. 142, 105562. doi:10.1016/j.aap.2020.105562
Daniel, J. (2012). “Choosing the size of the sample,” in Sampling essentials: Practical guidelines for making sampling choices, 236–253.
Dickey, J. P., Eger, T. R., Frayne, R. J., Delgado, G. P., and Ji, X. (2013). Research using virtual reality: Mobile machinery safety in the 21st century. Miner. 2013 3 (2), 145–164. doi:10.3390/min3020145
Fice, J. B. (2019). “Neuromechanics of neck muscles: Implication for whiplash,”. Doctoral dissertation (Vancouver, BC: University of British Columbia).
Kennedy, R. S., Lane, N. E., Berbaum, K. S., and Lilienthal, M. G. (1993). Simulator sickness questionnaire: An enhanced method for quantifying simulator sickness. Int. J. Aviat. Psychol. 3 (3), 203–220. doi:10.1207/s15327108ijap0303_3
Lombard, M., and Ditton, T. (1997). At the heart of it all: The concept of presence. J. Computer-Mediated Commun. 3, 0. doi:10.1111/j.1083-6101.1997.tb00072.x
Lukacs, M., and Salim, S. (2018). Exploring immersive technologies: The potential for innovation in whiplash research. Health Sci. Inq. 9, 69–70. doi:10.29173/hsi276
Morley, D. C., Lawrence, G., and Smith, S. (2016). “Virtual reality user experience as a deterrent for smartphone use while driving,” in ACM International Conference Proceeding Series.
Pietra, A., Vazquez Rull, M., Etzi, R., Gallace, A., Scurati, G. W., Ferrise, F., et al. (2021). Promoting eco-driving behavior through multisensory stimulation: A preliminary study on the use of visual and haptic feedback in a virtual reality driving simulator. Virtual Real. 1, 945–959. doi:10.1007/s10055-021-00499-1
Riches, S., Elghany, S., Garety, P., Rus-Calafell, M., and Valmaggia, L. (2019). Factors affecting sense of presence in a virtual reality social environment: A qualitative study. Cyberpsychology, Behav. Soc. Netw. 22 (4), 288–292. doi:10.1089/cyber.2018.0128
Riva, G., Mantovani, F., Capideville, C. S., Preziosa, A., Morganti, F., Villani, D., et al. (2007). Affective interactions using virtual reality: The link between presence and emotions. Cyberpsychology Behav. 10 (1), 45–56. doi:10.1089/cpb.2006.9993
Sharar, S. R., Miller, W., Teeley, A., Soltani, M., Hoffman, H. G., Jensen, M. P., et al. (2008). Applications of virtual reality for pain management in burn-injured patients. Expert Rev. Neurother. 8 (11), 1667–1674. doi:10.1586/14737175.8.11.1667
Slater, M., Usoh, M., and Steed, A. (1995). Taking steps: The influence of a walking technique on presence in virtual reality. ACM Trans. Computer-Human Interact. (TOCHI) 2 (3), 201–219. doi:10.1145/210079.210084
Trappey, A., Trappey, C. V., Chang, C. M., Tsai, M. C., Kuo, R. R. T., and Lin, A. P. C. (2021). Virtual reality exposure therapy for driving phobia disorder (2): System refinement and verification. Appl. Sci. Switz. 11 (1), 347–362. doi:10.3390/app11010347
UQO Cyberpsychology Lab (2004). Cyberpsy. Revised WS Questionnaire [online]. Available at: http://w3.uqo.ca/cyberpsy/index.php/documents-utiles/.
Walshe, D., Lewis, E., O’Sullivan, K., and Kim, S. I. (2005). Virtually driving: Are the driving environments “real enough” for exposure therapy with accident victims? An explorative study. Cyberpsychology Behav. 8 (6), 532–537. doi:10.1089/cpb.2005.8.532
Walton, D. M., and Elliott, J. M. (2017). An integrated model of chronic whiplash-associated disorder. J. Orthop. Sports Phys. Ther. 47 (7), 462–471. doi:10.2519/jospt.2017.7455
Wang, J., Shi, R., Zheng, W., Xie, W., Kao, D., and Liang, H.-N. (2023). Effect of frame rate on user experience, performance, and simulator sickness in virtual reality. IEEE Trans. Vis. Comput. Graph. (01), 1–11. doi:10.1109/tvcg.2023.3247057
Webb, C. M., Bass, J. M., Johnson, D. M., Kelley, A. M., Martin, C. R., and Wildzunas, R. M. (2009). Simulator sickness in a helicopter flight training school. Aviat. Space Environ. Med. 80 (6), 541–545. doi:10.3357/asem.2454.2009
Wiederhold, B. K., and Wiederhold, M. D. (2010). Virtual reality treatment of posttraumatic stress disorder due to motor vehicle accident. Cyberpsychology, Behav. Soc. Netw. 13 (1), 21–27. doi:10.1089/cyber.2009.0394
Keywords: simulator sickness, stress, virtual reality, whiplash associated disorder, presence, pain
Citation: Lukacs MJ, Babin M, Dickey JP, Melling CWJ and Walton DM (2023) Development and tolerability of a novel virtual- and proprioception-based car crash simulator as a new research tool in motor vehicle trauma research. Front. Virtual Real. 4:891423. doi: 10.3389/frvir.2023.891423
Received: 07 March 2022; Accepted: 20 March 2023;
Published: 31 March 2023.
Edited by:
Jaehyun Park, Incheon National University, Republic of KoreaReviewed by:
Jože Guna, University of Ljubljana, SloveniaDiego Vilela Monteiro, ESIEA University, France
Copyright © 2023 Lukacs, Babin, Dickey, Melling and Walton. This is an open-access article distributed under the terms of the Creative Commons Attribution License (CC BY). The use, distribution or reproduction in other forums is permitted, provided the original author(s) and the copyright owner(s) are credited and that the original publication in this journal is cited, in accordance with accepted academic practice. No use, distribution or reproduction is permitted which does not comply with these terms.
*Correspondence: Michael J. Lukacs, bWx1a2FjczJAdXdvLmNh