- 1Cleveland Clinic Lerner Research Institute, Biomedical Engineering, Cleveland, OH, United States
- 2Case Western Reserve University School of Medicine, Cleveland, OH, United States
- 3Cleveland Clinic Center for Neurological Restoration, Neurological Institute, Cleveland, OH, United States
Introduction: The successful performance of instrumental activities of daily living (IADLs) is critical in maintaining independence for older adults. Traditional IADL questionnaires and performance-based assessments are time consuming, potentially unreliable, and fail to adequately consider the interplay between cognitive and motor performance in completing IADLs. The Cleveland Clinic Virtual Reality Shopping (CC-VRS) platform was developed to objectively quantify IADL performance through the characterization of cognitive, motor, and cognitive-motor function. The CC-VRS combines an immersive virtual grocery store with an omnidirectional treadmill to create a scenario in which the user physically navigates through a virtual environment. The primary aim of this project was to determine the known-group validity of the CC-VRS platform to characterize IADL performance in healthy older adults and young adults.
Methods: Twenty healthy young (n = 10) and older (n = 10) adults completed the Basic and Complex CC-VRS scenarios. Position data from VR trackers on the hands, waist, and feet were used to quantify motor performance. Cognitive and dual-task performance were automatically calculated by the application during specific shopping sub-tasks.
Results: Older adults exhibited significantly worse performance on multiple cognitive, motor, and dual-task outcomes of the CC-VRS (e. g., average walking speed, number of list activations, and stopping frequency).
Discussion: The CC-VRS successfully discriminated IADL performance between young and healthy older adults. The complex realistic environment of the CC-VRS, combined with simultaneous evaluation of motor and cognitive performance, has the potential to more accurately characterize IADL performance by identifying subtle functional deficits that may precede neurological disease.
1 Introduction
Basic activities of daily living include self-care tasks such as bathing and dressing. In contrast, instrumental activities of daily living (IADLs) are more complex activities necessary for independent, community living and include tasks such as medication management, financial management, transportation, food preparation, shopping, and housekeeping (Spector et al., 1987). Early identification of a decline in IADL function has been proposed as a potential prodromal marker for neurological disease (Amieva et al., 2008; Darweesh et al., 2017; Foubert-Samier et al., 2020). An IADL typically involves motor and cognitive components that are performed simultaneously (dual-task performance). Despite the dual-task nature of IADLs, the vast majority of work has focused on how changes in specific aspects of executive function impact IADL performance (Gold, 2012). Executive function (EF) is a complex domain that represents a wide range of cognitive processes including working memory, attention, planning, utilization of feedback, flexibility, and task switching (Chan et al., 2008). Executive impairment is associated with declines in personal independence and quality of life (Cahn-Weiner et al., 2002; Royall et al., 2007; Davis et al., 2010), and deficits in EF have been repeatedly linked to difficulty with IADLs (Bell-McGinty et al., 2002; Jefferson et al., 2006; Vaughan and Giovanello, 2010; Hughes et al., 2012).
The traditional approach to evaluating EF involves using neuropsychological tests (NPTs) aimed at measuring cognitive constructs. Although clinical tests are relatively easy to administer via paper and pencil or, in some cases computerization, their reliability and standardization are impacted by testing environment, administration procedures, and scoring inconsistencies. Furthermore, the ability of traditional tests of EF to identify IADL decline is highly variable; results from a meta-analysis indicated the total variance in IADL performance accounted for by cognitive predictors ranged from 0 to 80 percent, with an average of 21 percent predicted by all cognitive measures (Royall et al., 2007). The inconsistent relationship between NPT and IADL performance reflects a fundamental limitation in using traditional NPT tests: their failure to capture the contribution of motor and cognitive-motor functioning on successful IADL performance.
Burgess and others proposed a solution to address the gap between traditional NPTs and real-world performance by creating performance-based assessments in which an individual is evaluated in a real-world or laboratory simulated environment (Burgess et al., 2006). The Multiple Errands Test (MET) is a classic performance-based assessment that is completed in an actual physical supermarket and requires the purchasing of items (Shallice and Burgess, 1991). The MET provides a global evaluation, both motor and cognitive, of functional deficits compared to traditional NPTs that only provide information related to a specific EF construct (Rand, Basha-Abu Rukan et al., 2009). Unfortunately, limitations in scalability-including the time, resources, transportation, and organization required to administer the test in a quasi-controlled real-world environment, as well as the lack of control over the environment and other distractors-have prevented widespread clinical use of the MET (Bailey et al., 2010).
Virtual reality (VR) offers an opportunity to address the limitations of IADL assessment associated with traditional paper and pencil tests and the MET. Virtual environments can be standardized and provide an opportunity for participants to naturally interact with objects and respond to situations that mimic those encountered in daily life. In addition to offering a tightly controlled and simultaneously flexible environment, VR has the potential to objectively capture and quantify cognitive-motor functioning during IADL activities. Previous studies suggest the ability of VR platforms to consistently detect cognitive-motor impairments hinges on the technology used to create a fully immersive, ecologically valid, real-world experience (Janouch et al., 2018; Vickers et al., 2018; Nenna et al., 2021).
Several VR applications have leveraged virtual environments to create performance-based assessments similar to the MET (Parsons and McMahan, 2017; Corriveau Lecavalier et al., 2020); however, only a few have detected cognitive-motor impairments related to normal aging. The lack of significant findings from VR applications highlights the limited scope of many VR assessments as most include tasks that 1) focus on one component of executive function, e.g., memory recall (Plechata, et al., 2019), and/or 2) remove key components of traditional IADLs like walking and turning as users are assessed while seated (Parsons and McMahan, 2017). Comprehensive IADL assessments that include multiple components of cognitive, motor, and cognitive-motor outcomes acquired from freely moving participants can potentially improve the usability of IADL outcomes from VR applications to detect subtle declines in cognitive-motor function and trigger earlier interventions with greater preservation of function.
The Cleveland Clinic Virtual Reality Shopping (CC-VRS) platform was developed to provide an accurate and comprehensive assessment of IADL performance as it combines digital content with an omnidirectional treadmill to create a fully immersive environment to precisely measure real-world cognitive-motor performance (Alberts et al., 2022). The CC-VRS content and tasks were developed following a formal task analysis of grocery shopping, a traditional IADL, and each aspect of the task was identified as either motor, cognitive, or cognitive-motor (Alberts et al., 2022). Similar to virtual adaptations of the MET, participants are placed in a virtual grocery store with the goal of retrieving items from a list. Unique to the CC-VRS, participants physically navigate through the virtual store by walking, stopping, and turning on the omnidirectional treadmill. Data from virtual tracking devices on the user’s waist, feet, and hands are used to objectively quantify motor, cognitive, and cognitive-motor performance as they complete the shopping task.
The primary aim of this preliminary project was to determine the known-group validity of the CC-VRS platform to characterize IADL performance in healthy older adults and young adults. It was hypothesized that older adults would exhibit declines in CC-VRS IADL performance measures that reflect specific aspects of motor, cognitive, and cognitive-motor declines compared to young adults. The capability to comprehensively evaluate IADL function and discriminate between older and younger adults could aid in the eventual early identification of deficits that may suggest an underlying neurological deficit that is not detectable using traditional NPT or performance-based IADL assessments. Finally, considering the novelty of the CC-VRS, we sought to evaluate older and young adult user acceptance of the platform through the Simulator Sickness Questionnaire (SSQ) (Kennedy and Berbaum, 1993) and System Usability Scale (SUS) (Lewis and Sauro, 2009).
2 Materials and methods
2.1 Participants
Ten young adults (average age 23.0 years) and ten healthy older adults (average age 70.1 years) participated in this study. All participants were free of neurological disease, including dementia and mild cognitive impairment, and based on self-report were able to ambulate independently for at least 20 min. The experimental protocol was approved by the Cleveland Clinic Institutional Review Board, and all participants provided written informed consent prior to study participation.
All participants completed questionnaires regarding prior experience with grocery shopping and immersive VR, as well as the Lawton IADL scale (Lawton and Brody, 1969). Global cognitive functioning was assessed using the Montreal Cognitive Assessment (MoCA), a common screening tool for mild cognitive impairment which evaluates short term and working memory, visuospatial skills, executive function, attention, and language skills (Nasreddine et al., 2005).
2.2 CC-VRS platform
2.2.1 Hardware and set up
Details of the CC-VRS platform have been described in detail previously (Alberts et al., 2022). Briefly, the CC-VRS, shown in Figure 1, delivers a fully immersive grocery store environment to the user via a head-mounted VR display (HMD, Valve Index). Speakers embedded within the HMD provide ambient grocery store noises and announcements to enhance immersion. Participants physically navigate through the store via natural locomotion on an omnidirectional treadmill (Infinadeck, Rocklin, California) while secured in a non-weight-bearing harness for safety. Handheld VR controllers (Valve Index) are used to view the shopping list and interact with any of the 480 unique items on the shelves throughout the store. In addition to the controllers, VR tracking devices (Vive HTC) are affixed to the lower back and feet to provide upper and lower extremity kinematic data during task performance.
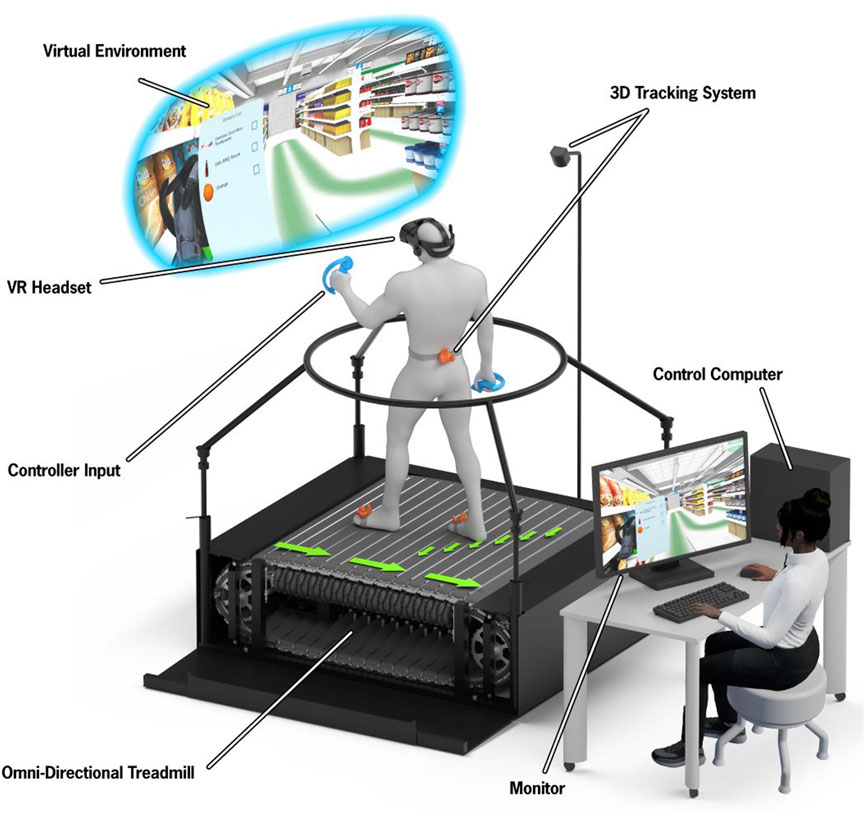
FIGURE 1. A depiction of the CC-VRS hardware setup. The participant wears a VR headset, hand controllers, and foot trackers. The participant is secured via a non-weight bearing harness system. Study staff are provided a first-person view from the participant’s headset, displayed on a computer screen. (Adapted from Alberts et al., 2022 with permission)
2.2.2 Rules of CC-VRS
In the CC-VRS, participants follow a designated 200 m route through a large virtual grocery store while searching for five items on the shopping list. The route begins at the entrance to the store and ends at the register, passing all designated items on the list. Deviations from the route are not permitted; participants cannot backtrack for missed items. Participants are instructed to complete the shopping task as quickly and as efficiently as possible while minimizing the number of errors (i.e., missing items, selecting incorrect items, or collisions with elements of the environment). The shopping list is activated via a button on the left controller. Participants are instructed to refer to the list as often as necessary and are informed that the number and duration of list activations will be recorded. Items are selected by reaching out and grasping the virtual object using the trigger button of the left or right controller. The item is then released into a virtual shopping cart positioned in front of the avatar. Items are not automatically eliminated from the shopping list when placed in the cart; however, participants may choose to utilize the optional checkboxes on the list to keep track of items selected. Figure 2 contains screenshots of the CC-VRS application and a top-down view of the designated route through the store.
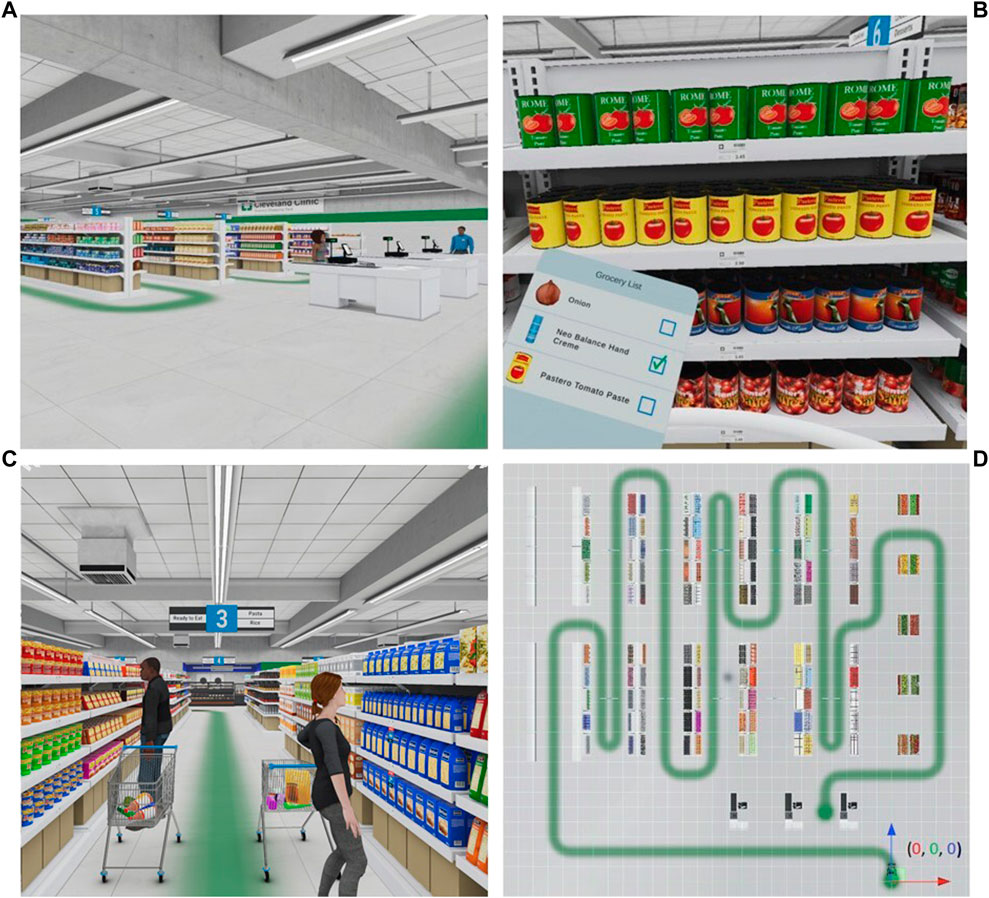
FIGURE 2. Screenshots of CC-VRS platform depicting (A) first-person view of the store, (B) shopping list and corresponding items, (C) first-person view of narrowed aisle avoidance task in Complex scenario, (D) top-down map view of 200 m route through virtual store.
2.2.3 CC-VRS protocol
Participants were introduced to the omnidirectional treadmill and given time to acclimate to the treadmill before donning the HMD. Study staff adjusted the HMD to ensure a clear visual display and secure fit. All participants completed a 5-min tutorial to ensure understanding of the task and demonstrate proficiency in completing necessary task components (e.g., shopping list activation, retrieving an item, etc.). In a single testing session lasting approximately 1 hour, each participant completed two CC-VRS scenarios in a randomized order: Basic and Complex. Both scenarios are governed by the same rules and use an identical route through the store. The Basic scenario requires the user to retrieve five items from their list. The Complex scenario has a different five-item shopping list and increased cognitive and motor demands. To evaluate working memory, the Complex scenario begins with an overhead store announcement of five words, and participants are instructed that they will be asked to recall these words following task completion. Additionally, in the Complex scenario, the shopping list contains one sale item that evaluates financial decision-making, and participants are instructed to choose the more cost-effective option (e.g., choosing between 8 oz. of ketchup for $1.00 or 16 oz. for $1.50). Precision of motor control is increased via the placement of additional shoppers and stocking carts in two locations to effectively narrow the aisle.
2.2.4 CC-VRS outcome measures
The primary outcomes to characterize cognitive, motor, and cognitive-motor performance during Basic and Complex scenarios are provided in Table 1.
2.3 User experience questionnaires
All participants completed the SSQ before and immediately after completing the CC-VRS. The SSQ is used to assess cybersickness; participants score 16 symptoms on a four-point scale. The SSQ produces a weighted total score (maximum 235.6) as well as additional weighted sub-scores for nausea, disorientation, and oculomotor symptoms with a higher score indicating greater sickness symptoms (Kennedy and Berbaum, 1993). Usability was evaluated using the SUS, a 10-item scale that measures ease-of-use, global satisfaction, and learnability of a system (maximum score 100; higher score indicates greater usability) (Lewis and Sauro, 2009).
2.4 Statistical analysis
Demographic variables were summarized by mean (standard deviation) for numerical variables and by number (percentage) for categorical variables. All CC-VRS outcomes were summarized by median (interquartile range). Non-parametric Wilcoxon Rank Sum tests were used to evaluate differences in CC-VRS metrics between participant groups. The same approach was used to evaluate differences between groups in SSQ and SUS scores. All analyses were performed using RStudio (version 4.2.2).
3 Results
3.1 Participants
Participant demographics are provided in Table 2. With the exception of age, there were no significant differences in demographic variables. In each group, 80% of participants identified as the primary grocery shopper of their household, and the average grocery shopping frequency was 3.5 times per month for young adults vs. 7.2 times per month for older adults (p = 0.073). The majority of participants in each group reported no prior experience with VR, and only one young adult participant had extensive (>3 exposures) experience with VR prior to the study. None of the participants had ever walked on an omnidirectional treadmill prior to the study. There was no significant difference in MoCA scores between young and older adults, and Lawton IADL scores for both groups were identical.
3.2 CC-VRS performance
All participants completed both the Basic and Complex CC-VRS scenarios. Outcomes for both scenarios are provided in Table 3.
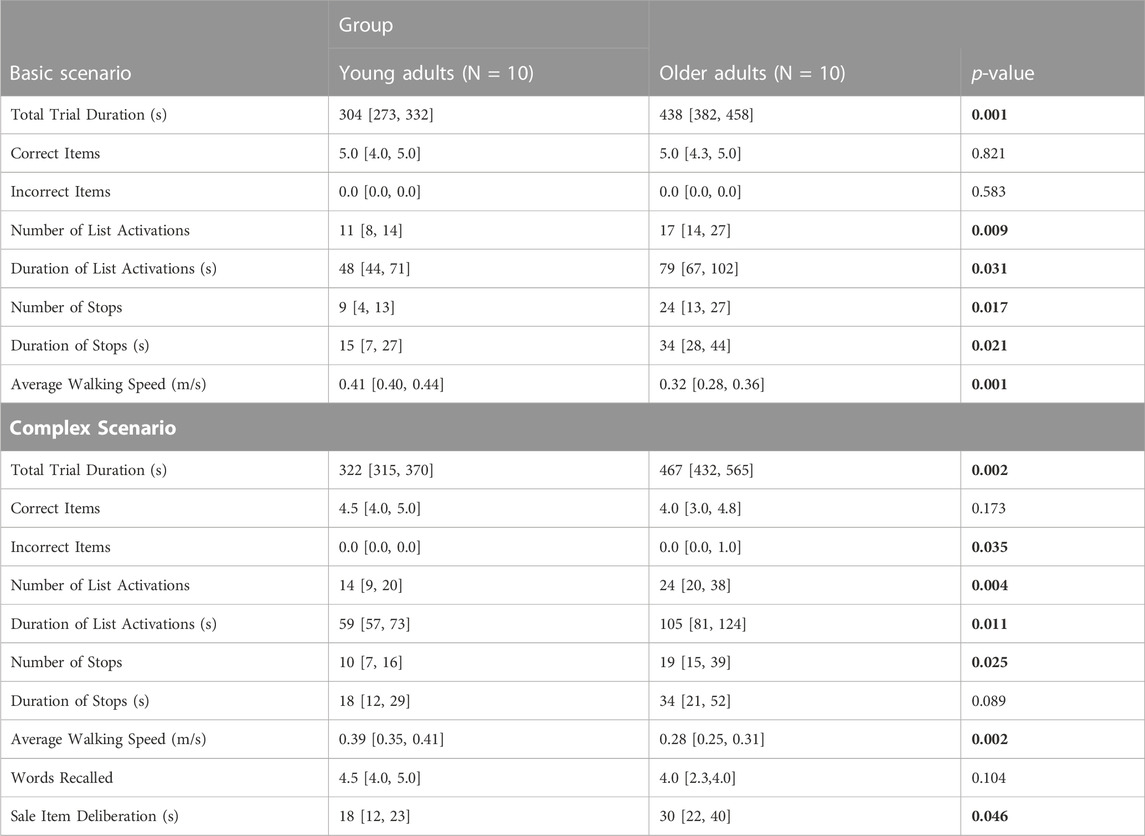
TABLE 3. Results of Basic and Complex CC-VRS scenarios by group: Results shown as median [quartile 1, quartile 3]. p-values based on Wilcoxon rank sum test. Significant differences bolded (p < 0.05).
3.2.1 Basic scenario
The cumulative distance traveled from a representative Basic CC-VRS trial from one young and one older adult participant are shown in Figure 3. The young adult completed the trial in less time (344 vs. 421 s), had fewer list views (7 vs. 15 count) and walked faster (0.40 vs. 0.32 m/s).
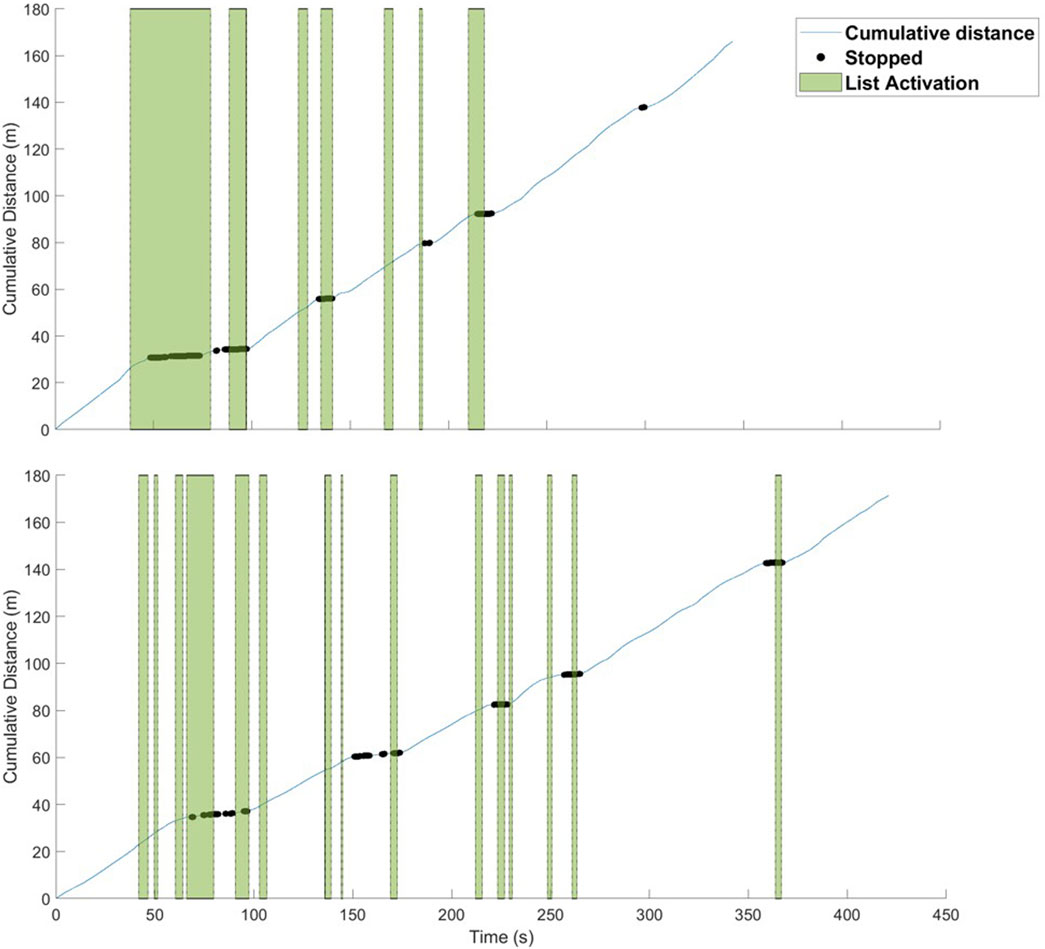
FIGURE 3. Cumulative distance for one representative trial for a young (upper figure) and older adult (lower figure) participant. The blue trace represents the distance traveled at each time point in the trial with intervals with little to no slope marked to indicate periods where the participant stopped. Vertical green bars indicate time points when the participant activated the list. The young adult completed the trial in less time (344 vs. 421 s), had fewer list views (7 vs. 15 count) and walked faster (0.40 vs. 0.32 m/s).
Similar results were evident in the cohort analysis comparing data from the young and older adult groups. Specifically, young adults and older adults performed differently on the Basic CC-VRS for all outcome measures except number of correct and incorrect items gathered, which were similar across groups. Overall, older adults completed the Basic CC-VRS significantly slower than young adults (438 vs. 304 s respectively, p = 0.001). Older adults walked slower through the store than young adults (0.32 vs. 0.41 m/s, p = 0.001).
From a cognitive perspective, older adults activated the shopping list over 50 percent more frequently than young adults (17 vs. 11 activations, p = 0.009). Cognitive-motor performance of older adults was also worse, as they made more stops to check the grocery list (24 vs. 9 stops, p = 0.017). Total stopping time for older adults was more than twice that of young adults (34 vs. 15 s, p = 0.021).
3.2.2 Complex scenario
Similar to the Basic CC-VRS, older adults took significantly greater time to complete the Complex scenario compared to young adults (467 vs. 322 s, p = 0.002). The number of incorrect items differed significantly between groups (p = 0.035); 40 percent of older adults selected at least one incorrect item, and no young adult selected any incorrect items.
Compared to younger adults, older adults activated the shopping list more frequently (24 vs. 14 activations, p = 0.004) and for a longer total duration (105 vs. 59 s, p = 0.011). Although the difference in total stop duration between groups did not reach statistical significance (34 vs. 18 s, p = 0.089), the number of stops was greater for older than young adults (19 vs. 10 stops, p = 0.025).
In the Complex CC-VRS, both older and young adults recalled a similar number of words presented at the beginning of shopping session (4.0 vs. 4.5 words, p = 0.104). Regarding the sale item price discrimination task, six older adults and seven young adults made the correct selection; however, the average time to make the sale item selection was longer for older adults compared to young adults (30 vs. 18 s, p = 0.046).
3.3 User sickness and experience
All participants completed the SSQ before and after completing the CC-VRS. Post-test scores were subtracted from baseline pre-test scores for analysis. There was no significant difference between groups for SSQ total score (median scores = 4 [IQR: 0, 24] for older adults, 0 [IQR: 0, 3] for young adults, p = 0.166). There were no differences between groups in sub-scores for nausea (p = 0.060), disorientation (p = 0.078), or oculomotor symptoms (p = 0.815).
User experience was evaluated using the SUS. The CC-VRS received a median score of 79 [IQR: 68, 88] from all participants, and there was no significant difference in SUS scores between participant groups (older adult median = 80 [IQR: 64, 89], young adult median = 79 [IQR: 71, 86], p = 0.939). The SUS score for the CC-VRS ranged from 58 to 100 across all participants.
4 Discussion
4.1 Discussion
The aim of this project was to compare healthy young and older adult IADL performance using the immersive CC-VRS platform. Initial data indicate young and older adults can complete the CC-VRS with minimal symptoms of VR sickness, and outcomes from CC-VRS successfully discriminate young and older adult IADL performance. The CC-VRS platform is the first performance-based IADL assessment that combines an immersive VR environment with an omnidirectional treadmill to facilitate the objective quantification of the important components of successful IADL function: cognitive, motor, and dual-task performance.
Generally, outcomes from the Basic and Complex CC-VRS scenarios discriminated between healthy older and young adults. Although both groups were successful in following instructions and gathering the items designated by the shopping list, overall performance of the task differed between young and older adults across most measures. Differences in the aforementioned metrics reveal declines in aspects of cognition, motor function, and cognitive-motor dual-task function in older adults compared to younger. Older adults referenced the shopping list more frequently and for longer duration than young adults. List activation behaviors may represent a component of working memory and short-term recall, and CC-VRS results are consistent with the established relationship between healthy aging and declines in memory systems, particularly working memory (Luo and Craik, 2008). Another aspect of cognition, the sale price comparison task was designed to evaluate financial decision-making and processing speed by requiring mental financial math to select the more cost-efficient item. Compared to young adults, older adults took almost 70% longer to choose between sale items. The price comparison task of the CC-VRS may capture more subtle age-related changes in financial decision-making and processing speed, both of which have been identified as leading indicators of overall cognitive function and markers of longitudinal trajectories of IADL decline and cognitive aging (Salthouse, 1996; Snowdon et al., 1996; Finkel and Pedersen, 2004; Peres et al., 2008).
From a motor perspective, it is well-established that older adults walk slower than young adults (Bohannon et al., 1996). Therefore, a group effect on walking speed through the grocery store was expected and found in both scenarios. However, the number of stops while shopping, a measure of dual-task performance, indicated that older adults had more frequent stops compared to younger adults. Older adults generally moved slower through the store, stopped more frequently, and remained stopped for longer than young adults. While young adults were able to successfully walk while simultaneously referencing the shopping list and searching the shelves for the designated items, older adults appeared to prioritize completion of the cognitive task at the expense of reduced gait speed or coming to a complete stop altogether. It is well-documented that performance of tasks requiring simultaneous cognitive and motor functions declines with age (Park and Reuter-Lorenz, 2009; Alexander et al., 2012). Even seemingly automated motor tasks such as gait and postural control require attentional resources, particularly with advances in age (Huxhold et al., 2006; Nobrega-Sousa et al., 2020). Historically, there has been an effort to tease apart the effects of cognitive and motor declines on changes in IADL performance due to aging. More frequent stopping to check the shopping list underscores the importance of evaluating cognitive and motor function simultaneously as both are important and reflect the true nature of IADL performance.
Considering the known impact of dual-task conditions on cognitive and motor performance in older adults, the CC-VRS offers a distinct advantage over traditional tests of EF that evaluate a single cognitive domain at a time and are performed in a sterile clinical environment, IADL questionnaires that rely on self-report to quantify performance, and current performance-based assessments.
The older and young adults included in this study were similar with respect to global cognitive function as measured by the MoCA and IADL performance as measured by the Lawton IADL scale. However, differences between young and older adults in CC-VRS duration, list activation behaviors, stopping behaviors, and walking speed suggest the CC-VRS can capture subtle declines in cognitive and dual-task performance that may indicate an impending problem in IADL performance. The continuum between healthy aging, MCI, and dementia is vast. Current approaches to IADL assessment, both questionnaire and performance-based, lack the precision to measure subtle, but potentially informative, changes that can more precisely identify one’s position on the continuum of function. Early identification of IADL decline could trigger additional medical care such as referrals to neurology or geriatric specialties and rehabilitative services such as physical, occupational, or speech therapy. Further, if subtle declines in IADL function precede a diagnosis of PD or AD by 5–10 years (Amieva et al., 2008; Darweesh et al., 2017; Foubert-Samier et al., 2020), cognitive, motor, and dual-task performance must be measured with a level of precision that is commensurate with the potential degree of change. While some have claimed that prodromal neurological disease is characterized by a “silent phase,” we contend more precise and comprehensive measures of potential dysfunction need to be utilized to detect changes during this clinically silent phase.
Many digital performance-based assessments similar to the MET have been created to harness the value of assessing IADLs to measure cognitive decline; however, while on the continuum of VR, they are not truly immersive (Borgnis et al., 2022). The Virtual MET (VMET) and the Virtual Reality Functional Capacity Assessment Tool (VRFCAT) are two examples of non-immersive VR applications, where the user is seated and uses a joystick to move an avatar through a virtual environment on a 2D screen. Immersive VR systems include a HMD which surround users in computer-generated simulations with 3D video and audio elements (Borgnis et al., 2022). Although an improvement from pen and paper, and from first generation non-immersive VR applications, many of these non-immersive VR assessments are limited in accurately assessing IADL function as they rely on utilizing a joystick to move an avatar through the virtual environment. Functional gait, specifically turning, is an important component in most IADLs as humans perform approximately 71 turns per hour when performing daily activities (Mancini 2015). The CC-VRS allows users to freely walk and turn while quantifying cognitive (sale price), motor (gait speed), or cognitive-motor (number of stops) function separately. With this approach, subtle declines in the cognitive and motor domains associated with aging can be precisely identified and potentially targeted with appropriate therapies and interventions to preserve more function.
By combining VR technology, an omnidirectional treadmill, and objective biomechanical outcomes to precisely quantify IADL function, the CC-VRS represents an important shift in the evaluation of older adults and potential for transition to neurological disease. The current project was largely a feasibility study to determine if older adults could complete an immersive VR assessment and if the selected cognitive, motor, and cognitive-motor outcomes could differentiate healthy young and older adults. Importantly, individuals of all ages, with little to no prior VR experience, were able to complete the entire CC-VRS protocol in less than an hour. Few participants experienced mild nausea, and overall symptom scores were minimal in both groups. Users rated the usability of the CC-VRS as 79/100 on the System Usability Scale. For comparison, the top ten popular mobile phone and tablet applications received an average score of 77.7/100 (Kortum P 2015). Several projects with the CC-VRS platform are in progress. The CC-VRS is being used to understand how neurological disease, in particular, Parkinson’s disease impacts IADL performance and potentially identify scenarios that trigger freezing of gait episodes. Results from this ongoing project will aid in treatment of gait dysfunction in Parkinson’s disease and provide additional context to performance of healthy older adults. The second project, a larger study of 400 older adults has been initiated to determine the feasibility of integrating a hybrid of the Basic and Complex CC-VRS scenarios, requiring less than 15 min to complete, into routine clinical care of older adults in the annual visit to their primary care provider. The fundamental goal of this project is to determine the feasibility of clinical integration and secondarily serve as a screen which could be used to trigger referral to a neurological specialist. It is envisioned that subtle declines in performance would trigger further monitoring or referral to specialty services such as neurology or rehabilitation. The clinical version of the CC-VRS was reduced slightly in terms of the distance walked in the store through the elimination of 180° turns. Important factors in scaling the CC-VRS will be the meaningfulness of outcomes to providers and patients and thoughtful integration of the technology into the clinical workflow. Regarding the latter, the CC-VRS was developed from the onset with clinical integration as a goal using the Develop with Clinical Intent model that we have recently published (Alberts, et al., 2023).
4.2 Limitations
A sample size of 20 young and older adults is relatively small, and all conclusions regarding the precise effects of age on IADL performance must be weighed appropriately. Multiple larger studies are on-going and planned to better understand the relationship between CC-VRS performance and potential neurological disease.
The use of an omnidirectional walking platform facilitates the immersion of the user into a VR environment while simultaneously facilitating the collection of biomechanical outcomes related to gait. It is appreciated that the cost to replicate the current experimental paradigm may limit clinical adoption today. However, the cost of VR headsets and omnidirectional treadmills continues to decline, and it is envisioned that this platform could be integrated into clinical care. The cost of an omnidirectional treadmill is ∼$10,000-$50,000 USD, similar to many rehabilitation treadmills (i.e., body-weight support treadmills and treadmills with projection or interactive screens). Despite these limitations, the results of this project provide initial evidence that immersive VR, such as the CC-VRS, can be used by older adults and can identify subtle deficits in IADL performance.
4.3 Conclusion
The CC-VRS platform combines an immersive virtual grocery store with an omnidirectional treadmill to deliver a performance-based assessment of IADL function. In this preliminary project, motor, cognitive, and dual-task outcomes of the CC-VRS successfully discriminated between healthy older and young adults, whereas traditional evaluations of global cognitive function and IADL performance did not capture differences between groups. By evaluating EF and IADL performance under complex dual-task conditions, the CC-VRS may identify subtle deficits in functional ability that are not quantified by traditional NPTs in a clinical setting. Furthermore, the CC-VRS was generally well-tolerated and rated high in usability among users of all ages, and additional ongoing projects will determine the feasibility of clinical integration of a modified CC-VRS platform into routine clinical care of older adults.
Data availability statement
The raw data supporting the conclusion of this article will be made available by the authors, without undue reservation.
Ethics statement
The project was approved by the Cleveland Clinic Institutional Review Board, and was conducted in accordance with the local legislation and institutional requirements. The participants provided their written informed consent to participate in this study.
Author contributions
ML: Conceptualization, Data curation, Formal Analysis, Investigation, Methodology, Project administration, Software, Supervision, Validation, Visualization, Writing–original draft, Writing–review and editing. CW: Data curation, Investigation, Methodology, Project administration, Software, Validation, Writing–review and editing. KS: Conceptualization, Software, Visualization, Writing–review and editing. LS: Conceptualization, Software, Visualization, Writing–review and editing. KO: Formal Analysis, Validation, Visualization, Writing–review and editing. KH: Formal Analysis, Validation, Visualization, Writing–review and editing. MM: Conceptualization, Formal Analysis, Funding acquisition, Investigation, Methodology, Supervision, Writing–review and editing. AR: Conceptualization, Data curation, Funding acquisition, Investigation, Methodology, Supervision, Writing–review and editing. JA: Conceptualization, Funding acquisition, Investigation, Resources, Writing–review and editing.
Funding
The author(s) declare financial support was received for the research, authorship, and/or publication of this article. This work was supported by the Michael J. Fox Foundation for Parkinson’s Research (MJFF-020020) and the Edward and Barbara Bell Family Chair.
Acknowledgments
We would like to thank Elm Park Labs (Detroit, MI) for their assistance in building the VR environment and Evelyn Thoman for her assistance with project execution.
Conflict of interest
JA, AR, and ML have authored intellectual property associated with the virtual reality software which has been licensed to a medical software company.
The remaining authors declare that the research was conducted in the absence of any commercial or financial relationships that could be construed as a potential conflict of interest.
Publisher’s note
All claims expressed in this article are solely those of the authors and do not necessarily represent those of their affiliated organizations, or those of the publisher, the editors and the reviewers. Any product that may be evaluated in this article, or claim that may be made by its manufacturer, is not guaranteed or endorsed by the publisher.
References
Alberts, J. L., Mcgrath, M., Miller Koop, M., Waltz, C., Scelina, L., Scelina, K., et al. (2022). The immersive Cleveland clinic virtual reality shopping platform for the assessment of instrumental activities of daily living. J. Vis. Exp., doi:10.3791/63978
Alberts, J. L., Shuaib, U., Fernandez, H., Walter, B. L., Schindler, D., Miller Koop, M., et al. (2023). The Parkinson’s Disease waiting room of the future: measurements, not magazines. Front. Neurol. 14, 1212113. doi:10.3389/fneur.2023.1212113
Alexander, G. E., Ryan, L., Bowers, D., Foster, T. C., Bizon, J. L., Geldmacher, D. S., et al. (2012). Characterizing cognitive aging in humans with links to animal models. Front. Aging Neurosci. 4, 21. doi:10.3389/fnagi.2012.00021
Amieva, H., Le Goff, M., Millet, X., Orgogozo, J. M., Peres, K., Barberger-Gateau, P., et al. (2008). Prodromal Alzheimer's disease: successive emergence of the clinical symptoms. Ann. Neurol. 64, 492–498. doi:10.1002/ana.21509
Bailey, P. E., Henry, J. D., Rendell, P. G., Phillips, L. H., and Kliegel, M. (2010). Dismantling the "age-prospective memory paradox": the classic laboratory paradigm simulated in a naturalistic setting. Q. J. Exp. Psychol. (Hove) 63, 646–652. doi:10.1080/17470210903521797
Bell-Mcginty, S., Podell, K., Franzen, M., Baird, A. D., and Williams, M. J. (2002). Standard measures of executive function in predicting instrumental activities of daily living in older adults. Int. J. Geriatr. Psychiatry 17, 828–834. doi:10.1002/gps.646
Bohannon, R. W., Andrews, A. W., and Thomas, M. W. (1996). Walking speed: reference values and correlates for older adults. J. Orthop. Sports Phys. Ther. 24, 86–90. doi:10.2519/jospt.1996.24.2.86
Borgnis, F., Baglio, F., Pedroli, E., Rossetto, F., Uccellatore, L., Oliveira, J. A. G., et al. (2022). Available virtual reality-based tools for executive functions: a systematic review. Front. Psychol. 13, 833136. doi:10.3389/fpsyg.2022.833136
Burgess, P. W., Alderman, N., Forbes, C., Costello, A., Coates, L. M., Dawson, D. R., et al. (2006). The case for the development and use of "ecologically valid" measures of executive function in experimental and clinical neuropsychology. J. Int. Neuropsychol. Soc. 12, 194–209. doi:10.1017/s1355617706060310
Cahn-Weiner, D. A., Boyle, P. A., and Malloy, P. F. (2002). Tests of executive function predict instrumental activities of daily living in community-dwelling older individuals. Appl. Neuropsychol. 9, 187–191. doi:10.1207/s15324826an0903_8
Chan, R. C., Shum, D., Toulopoulou, T., and Chen, E. Y. (2008). Assessment of executive functions: review of instruments and identification of critical issues. Arch. Clin. Neuropsychol. 23, 201–216. doi:10.1016/j.acn.2007.08.010
Corriveau Lecavalier, N., Ouellet, E., Boller, B., and Belleville, S. (2020). Use of immersive virtual reality to assess episodic memory: a validation study in older adults. Neuropsychol. Rehabil. 30, 462–480. doi:10.1080/09602011.2018.1477684
Darweesh, S. K., Verlinden, V. J., Stricker, B. H., Hofman, A., Koudstaal, P. J., and Ikram, M. A. (2017). Trajectories of prediagnostic functioning in Parkinson's disease. Brain 140, 429–441. doi:10.1093/brain/aww291
Davis, J. C., Marra, C. A., Najafzadeh, M., and Liu-Ambrose, T. (2010). The independent contribution of executive functions to health related quality of life in older women. BMC Geriatr. 10, 16. doi:10.1186/1471-2318-10-16
Finkel, D., and Pedersen, N. L. (2004). Processing speed and longitudinal trajectories of change for cognitive abilities: the Swedish Adoption/Twin Study of Aging. Aging Neuropsychology Cognition 11, 325–345. doi:10.1080/13825580490511152
Foubert-Samier, A., Helmer, C., Le Goff, M., Guillet, F., Proust-Lima, C., Jacqmin-Gadda, H., et al. (2020). Cognitive and functional changes in prediagnostic phase of Parkinson disease: a population-based study. Park. Relat. Disord. 79, 40–46. doi:10.1016/j.parkreldis.2020.08.022
Gold, D. A. (2012). An examination of instrumental activities of daily living assessment in older adults and mild cognitive impairment. J. Clin. Exp. Neuropsychol. 34, 11–34. doi:10.1080/13803395.2011.614598
Hughes, T. F., Chang, C. C., Bilt, J. V., Snitz, B. E., and Ganguli, M. (2012). Mild cognitive deficits and everyday functioning among older adults in the community: the Monongahela-Youghiogheny Healthy Aging Team study. Am. J. Geriatr. Psychiatry 20, 836–844. doi:10.1097/jgp.0b013e3182423961
Huxhold, O., Li, S. C., Schmiedek, F., and Lindenberger, U. (2006). Dual-tasking postural control: aging and the effects of cognitive demand in conjunction with focus of attention. Brain Res. Bull. 69, 294–305. doi:10.1016/j.brainresbull.2006.01.002
Janouch, C., Drescher, U., Wechsler, K., Haeger, M., Bock, O., and Voelcker-Rehage, C. (2018). Cognitive-motor interference in an ecologically valid street crossing scenario. Front. Psychol. 9, 602. doi:10.3389/fpsyg.2018.00602
Jefferson, A. L., Paul, R. H., Ozonoff, A., and Cohen, R. A. (2006). Evaluating elements of executive functioning as predictors of instrumental activities of daily living (IADLs). Archives Clin. Neuropsychology 21, 311–320. doi:10.1016/j.acn.2006.03.007
Kennedy, R. S., Berbaum, K. S., and Lilienthal, M. G. (1993). Simulator sickness questionnaire: an enhanced method for quantifying simulator sickness. Int. J. Aviat. Psychol. 3, 203–220. doi:10.1207/s15327108ijap0303_3
Kortum P, S. M., and Sorber, M. (2015). Measuring the usability of mobile applications for phones and tablets. J. Hum. Comput. Interact. 31, 518–529. doi:10.1080/10447318.2015.1064658
Lawton, M. P., and Brody, E. M. (1969). Assessment of older people: self-maintaining and instrumental activities of daily living. Gerontologist 9, 179–186. doi:10.1093/geront/9.3_part_1.179
Lewis, J. R., and Sauro, J. (2009). “The factor structure of the system usability scale,” in Human centered design, 2009. Editor M. KUROSU (Berlin, Heidelberg: Springer Berlin Heidelberg), 94–103.
Luo, L., and Craik, F. I. (2008). Aging and memory: a cognitive approach. Can. J. Psychiatry 53, 346–353. doi:10.1177/070674370805300603
Mancini, M., El-Gohary, M., Pearson, S., McNames, J., Schlueter, H., Nutt, J. G., et al. (2015). Continuous monitoring of turning in Parkinson's disease: Rehabilitation potential. NeuroRehabilitation 37(37), 3–10. doi:10.3233/NRE-151236
Nasreddine, Z. S., Phillips, N. A., Bedirian, V., Charbonneau, S., Whitehead, V., Collin, I., et al. (2005). The Montreal Cognitive Assessment, MoCA: a brief screening tool for mild cognitive impairment. J. Am. Geriatr. Soc. 53, 695–699. doi:10.1111/j.1532-5415.2005.53221.x
Nenna, F., Do, C. T., Protzak, J., and Gramann, K. (2021). Alteration of brain dynamics during dual-task overground walking. Eur. J. Neurosci. 54, 8158–8174. doi:10.1111/ejn.14956
Nobrega-Sousa, P., Gobbi, L. T. B., Orcioli-Silva, D., Conceicao, N. R. D., Beretta, V. S., and Vitorio, R. (2020). Prefrontal cortex activity during walking: effects of aging and associations with gait and executive function. Neurorehabil Neural Repair 34, 915–924. doi:10.1177/1545968320953824
Park, D. C., and Reuter-Lorenz, P. (2009). The adaptive brain: aging and neurocognitive scaffolding. Annu. Rev. Psychol. 60, 173–196. doi:10.1146/annurev.psych.59.103006.093656
Parsons, T. D., and Mcmahan, T. (2017). An initial validation of the virtual environment grocery store. J. Neurosci. Methods 291, 13–19. doi:10.1016/j.jneumeth.2017.07.027
Peres, K., Helmer, C., Amieva, H., Orgogozo, J. M., Rouch, I., Dartigues, J. F., et al. (2008). Natural history of decline in instrumental activities of daily living performance over the 10 years preceding the clinical diagnosis of dementia: a prospective population-based study. J. Am. Geriatr. Soc. 56, 37–44. doi:10.1111/j.1532-5415.2007.01499.x
Plechata, A., Sahula, V., Fayette, D., and Fajnerova, I. (2019). Age-related differences with immersive and non-immersive virtual reality in memory assessment. Front. Psychol. 10, 1330. doi:10.3389/fpsyg.2019.01330
Rand, D., Basha-Abu Rukan, S., Weiss, P. L., and Katz, N. (2009). Validation of the Virtual MET as an assessment tool for executive functions. Neuropsychol. Rehabil. 19, 583–602. doi:10.1080/09602010802469074
Royall, D. R., Lauterbach, E. C., Kaufer, D., Malloy, P., Coburn, K. L., Black, K. J., et al. (2007). The cognitive correlates of functional status: a review from the Committee on Research of the American Neuropsychiatric Association. J. Neuropsychiatry Clin. Neurosci. 19, 249–265. doi:10.1176/jnp.2007.19.3.249
Salthouse, T. A. (1996). The processing-speed theory of adult age differences in cognition. Psychol. Rev. 103, 403–428. doi:10.1037/0033-295x.103.3.403
Shallice, T., and Burgess, P. W. (1991). Deficits in strategy application following frontal lobe damage in man. Brain 114 (2), 727–741. doi:10.1093/brain/114.2.727
Snowdon, D. A., Kemper, S. J., Mortimer, J. A., Greiner, L. H., Wekstein, D. R., and Markesbery, W. R. (1996). Linguistic ability in early life and cognitive function and Alzheimer's disease in late life. Findings from the Nun Study. JAMA 275, 528–532. doi:10.1001/jama.275.7.528
Spector, W. D., Katz, S., Murphy, J. B., and Fulton, J. P. (1987). The hierarchical relationship between activities of daily living and instrumental activities of daily living. J. Chronic Dis. 40, 481–489. doi:10.1016/0021-9681(87)90004-x
Vaughan, L., and Giovanello, K. (2010). Executive function in daily life: age-related influences of executive processes on instrumental activities of daily living. Psychol. Aging 25, 343–355. doi:10.1037/a0017729
Keywords: virtual reality, digital health, technology, aging, instrumental activities of daily living (IADL), omnidirectional treadmill
Citation: Lewis MM, Waltz C, Scelina K, Scelina L, Owen K, Hastilow K, Miller Koop M, Rosenfeldt AB and Alberts JL (2023) Older adults exhibit declines in instrumental activities of daily living during a virtual grocery shopping task. Front. Virtual Real. 4:1261096. doi: 10.3389/frvir.2023.1261096
Received: 18 July 2023; Accepted: 10 November 2023;
Published: 29 November 2023.
Edited by:
Miao Wang, Beihang University, ChinaReviewed by:
Mark Ettenhofer, University of California, San Diego, United StatesWeiya Chen, Huazhong University of Science and Technology, China
Copyright © 2023 Lewis, Waltz, Scelina, Scelina, Owen, Hastilow, Miller Koop, Rosenfeldt and Alberts. This is an open-access article distributed under the terms of the Creative Commons Attribution License (CC BY). The use, distribution or reproduction in other forums is permitted, provided the original author(s) and the copyright owner(s) are credited and that the original publication in this journal is cited, in accordance with accepted academic practice. No use, distribution or reproduction is permitted which does not comply with these terms.
*Correspondence: Jay L. Alberts, albertj@ccf.org