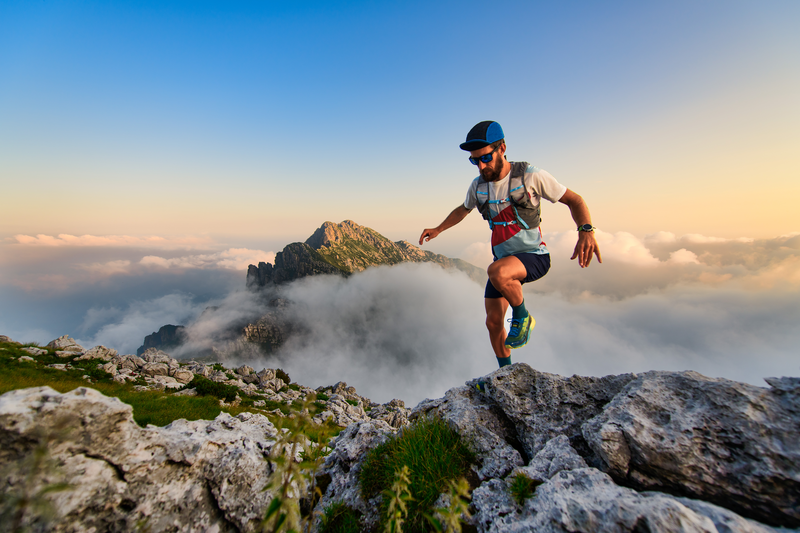
94% of researchers rate our articles as excellent or good
Learn more about the work of our research integrity team to safeguard the quality of each article we publish.
Find out more
ORIGINAL RESEARCH article
Front. Virtual Real. , 09 August 2022
Sec. Haptics
Volume 3 - 2022 | https://doi.org/10.3389/frvir.2022.930848
This article is part of the Research Topic New Materials and Technologies for Haptics that Enhance VR and AR View all 4 articles
Various methods for inducing an illusory force sensation to present a sense of force to users in energy-saving and space-saving systems have been proposed. One of them is the illusion of force sensation induced by cutaneous sensory stimulation. In this study, we hypothesized and empirically verified that lateral skin stretch alone on the face can induce an illusory force sensation in the direction of the stretch. We focused on the anterior temporal and cheekbone regions, in which the cushion part of the head-mounted display contacts the skin, and applied skin stretches of different intensities to these regions, envisioning a force presentation device built into the head-mounted display. Head rotations of approximately 40 and 50 degrees were generated by skin stretches in the anterior temporal and cheekbone regions, respectively, confirming the illusory force sensation in the direction of rotation. We confirmed a positive correlation between the head-turning angle and the amount of skin deformation. The intensity of the illusory force sensation can be controlled by changing the amount of lateral skin deformation; this may be applied to the development of a new force presentation head-mounted device.
Illusory force sensation has been actively researched as a tool to present force in an energy-saving and space-saving manner. Presenting visual stimuli is the most common method of generating an illusory force sensation. For example, the sense of weight can be altered by changing the texture or motion of an object (Lécuyer, 2009). However, the generation of this visual illusory force sensation requires gazing at the object, and it is difficult to provide force information about an object outside the field of vision. By contrast, presenting an illusory force sensation via cutaneous sensation makes it possible to present force information about an object being touched outside the visual field.
Many studies have reported that lateral skin deformation generates an illusory force sensation using cutaneous sensory stimulation. Minamizawa et al. (2007) proposed a method in which a motor is placed on the dorsal surface of the finger and a belt shears the skin of the finger pad. They confirmed that the shear stress exerted on the finger pad generates an illusory-gravity sensation.
Amemiya et al. (2005) showed that grasping an asymmetrically vibrating object creates the illusion of traction. They reported that the vibration frequency is related to the Meissner corpuscle, which detects slipping, and the Ruffini ending, which detects lateral skin deformation (Amemiya and Gomi, 2014). Tanabe et al. (2017) reported that asymmetric vibration in the tangential direction (skin shear direction) produces a more comprehensible illusory force sensation than asymmetric vibration in the direction normal to the skin (compression direction). These studies confirm that lateral skin deformation is important in generating force illusions, especially in the hand and fingertips.
Another known illusory force sensation that uses cutaneous sensory stimulation is the hanger reflex, which creates the illusion of force in the direction of rotation of the head and extremities (Figure 1) (Sato et al., 2009). The head can be rotated as much as 60 degrees because of this illusion, and it has been used in the treatment of spastic cervical dystonia (Asahi et al., 2020). As this phenomenon is associated with relatively large body movements, it has a wide range of applications. It is reportedly caused by the compression of two opposing points of the head, such as the anterior and posterior temporal regions. In contrast, several studies have reported the importance of lateral skin deformation caused by compression (Sato et al., 2014; Miyakami et al., 2018). Kon et al. (2018) explained that in their device for controlling the head hanger reflex, the two opposing points are compressed by an inflated air bag, and the resulting frame movement causes skin shear, thereby reproducing and controlling the phenomenon (Figure 2 left). However, the effect of skin shear has not been considered separately from the compression because these devices inevitably involve compression.
FIGURE 1. Hanger reflex (Sato et al., 2009).
FIGURE 2. Force components of the hanger reflex (Kon et al., 2018) and our skin stretch method.
This study confirms the illusory force sensation in the direction of rotation of the head by skin shear stimulation on the face only. Based on several reports that lateral skin deformation is associated with the generation of an illusory force sensation, we hypothesized that lateral skin deformation alone can generate the illusory force sensation and verified this hypothesis empirically. We used tape and rubber bands as a method of generating only shear stimuli to the skin. This is a simplified version of the method of Hamdan et al. (2019); however, the stimuli are limited to skin shear, and pressure is minimized. It is also similar to the original hanger reflex in that it produces an illusory force sensation in the direction of rotation of a body part (Figure 2 right). Therefore, we refer to the known stimulation site of the hanger reflex as the body part to be stimulated. In this study, we confirmed that the head rotation phenomenon can be generated when only lateral skin deformation stimuli are applied to the anterior temporal regions. We also confirmed that this phenomenon can be generated at the cheekbone regions. As both the anterior temporal and cheekbone regions are in contact with the inner surface of the head-mounted display (HMD), it is easy to incorporate our method into the HMD.
This study makes the following two main contributions.
• We show that a new illusory force sensation is generated by lateral skin deformation.
• The correlation between the amount of skin shear deformation and the intensity of the illusory force sensation is determined, providing basic knowledge for the application of this phenomenon.
In this section, we describe related studies on force presentation and skin shear stimulus presentation to the head and discuss their respective contributions.
External forces applied to the head present directional information directly from the actuator to the user (Gugenheimer et al., 2016; Liu et al., 2020). Costes and Lecuyer (2021) connected a grounded robotic arm to an HMD to apply a highly flexible force to the head. However, the robot arm was large and grounded to the environment, limiting the workspace. Hoppe et al. (2021) proposed a non-grounded force presentation device that did not limit the workspace by attaching a propeller mechanism to the HMD. However, the loudness of the sound generated during the drive was approximately 100 dB, requiring the use of hearing protection or noise-canceling headphones.
Galvanic vestibular stimulation (GVS) and electrical muscle stimulation (EMS) methods are used in studies of head actuation by electrical stimulation. GVS induces a sense of acceleration by presenting electrical stimulation to the human head (Sra et al., 2019; Aoyama et al., 2017; Teo et al., 2020). The sense of acceleration is generated by attaching electrodes around the ears, which enables the presentation of inertial and other forces and the generation of motion.
Tanaka et al. (2022) reported that EMS stimulation of the neck muscles causes the head rotation in the yaw (left-right) and pitch (up-down) axes. They showed that combining head rotation with EMS and various VR/AR applications can enable new interactions.
The GVS and EMS system can be easily integrated into a variety of devices because of its small size. However, the sensation has been associated with symptoms of discomfort in some healthy users (Utz et al., 2011; Tanaka et al., 2022).
The hanger reflex generates motion that is sufficiently strong to move the body and has been proposed for a wide range of applications, including medical care, gait navigation, and virtual reality (VR) (Kon et al., 2018; Asahi et al., 2018; Kon et al., 2017).
Currently, devices that reproduce the hanger reflex reproduce the pressure applied to two opposing points on the head. However, the devices are rather large as they require a hard outer frame around the body to maintain the vertical force during the pressure presentation.
It has been reported that the direction of the rotational motion of the hanger reflex is determined by the direction of the lateral skin deformation (Sato et al., 2014) and that there is a correlation between lateral skin deformation and the intensity of the illusion in a single-user study (Miyakami et al., 2018). These reports suggest that shear deformation of the skin significantly contributes to the generation of the illusion. However, the effects of skin shear stimulus changes with the illusory force intensity at the same stimulus point and the correlation between the physical lateral skin deformation and head rotation angle have not been clarified.
Several studies have proposed applications for skin shear stimulation, typically as a directional cue for navigation (Hamdan et al., 2019; Preechayasomboon et al., 2020; Clark et al., 2018; Colella et al., 2019; Kuang et al., 2022). Wang et al. (2019) demonstrated a variety of applications by applying skin shear stimuli to six points on the face. Their study is similar to our present study in that it applied skin shear stimuli to the face, including the anterior temporal and cheekbone regions. However, it aimed only at presenting cutaneous sensations and did not generate force sensations or motion, such as the hanger reflex.
In this experiment, we investigated the head rotation generation phenomenon when skin shear stimulation using tape was applied to the face. The stimulated body parts were the anterior temporal and cheekbone regions, which are in contact with the inner surface of the HMD when it is worn. For each stimulus condition, we evaluated the subjective force intensity using questionnaires and measured the physical rotation angle.
Before investigating the head rotation phenomenon by skin traction stimulation, we first investigated whether the head hanger reflex and skin traction stimulation produced head rotation.
For the head hanger reflex, the long side of a wire hanger was attached to the anterior temporal region, the stimulation site where the head rotation phenomenon has been observed. Skin traction was applied to the anterior temporal and cheekbone regions, respectively, using the same experimental device used in the experiment described below in combination with Kinesio tape and rubber bands. Although the traction intensity was not measured, the experimenter visually confirmed that the skin was sheared and deformed by the traction stimulus.
To reduce the experimental time, the experimenter visually observed the subject’s head rotation. The subjects were also asked to answer Yes or No to whether they felt a sense of force in the direction of rotation to confirm the generation of an illusory force.
Thirteen male participants (23–27 years old) participated in the study. The experimenter confirmed that three out of the 13 participants experienced head rotation due to the head hanger reflex but did not experience head rotation due to the traction stimulus. Because head rotation occurred in both cases for the rest of the participants, we conducted the main experiment with the remaining 10 participants.
This study was reviewed and approved by the ethics committee of the University of Electro Communications (No. 21074). All participants provided written informed consent to participate.
The participants were the aforementioned 10 men (23–27 years old). Skin shear stimulation was induced using tape and rubber bands. The tape used was Kinesio tape, which was also used in the skin shear deformation technique by Hamdan et al. (2019) using a shape memory alloy. This tape has high elasticity, and it deforms in accordance with the skin deformation that occurs during skin traction stimulation. The tape size was 2 × 2 cm, which was the size at which the phenomenon occurred most frequently in our preliminary survey (Figure 3). A rubber band was placed in the center of the nonadhesive side of the tape, and a 1 cm wide piece of tape was glued over it to secure it in place. A 0.5 × 0.5 cm piece of retroreflective tape was applied to each of the four corners of the tape to measure skin deformation.
FIGURE 3. The 2 × 2 cm Kinesio tape used in the experiment. Retroreflective tapes were attached to the four corners.
There were four conditions for traction force strength (50 gf, 100 gf, 150 gf, and 200 gf), and two conditions for stimulation site (the anterior temporal and cheekbone regions), for eight conditions in total. The participants were instructed to close their eyes during the measurement of the head rotation angle and not to resist the perceived force sensation. To measure the head angle, a retroreflective marker was attached to the top of the head, and the head rotation angle was measured using a motion capture system (Optitrack Trio, Natural Point, Inc.) (Figure 4).
FIGURE 4. A participant wearing the experimental device. (Fixation devices are shown in red frames).
The following procedure was used to determine the stimulus position for the experiment. The experimenter instructed the participants to wear a wire hanger such that its long side was in contact with their right anterior temporal region and confirm the occurrence of the hanger reflex. Upon confirmation, a mark was placed on the skin at the position where pressure was applied by the long edge of the wire hanger (Figure 5A). Next, the participants were instructed to wear the HMD in a position where they could clearly see the image, and a mark was made at the intersection of the HMD’s contact area with the skin and the perpendicular line from the mark made in the procedure using the wire hanger (green dot in Figure 5B). Tapes were attached to the marked positions (Figure 5C), and they were pulled by the rubber. The traction force was measured by a triaxial force sensor (DSA-03A, TecGihan) embedded in the fixation devices of the rubber band attached with Velcro tape (Figure 6). The traction force was adjusted by changing the position of the fixation device, allowing an error of ±10 gf.
FIGURE 5. Procedure for determining the position of the tapes: (A) Use hanger reflex to determine the position along the horizontal axis. (B) Put on an HMD and determine the vertical position of the two stimulation sites. (C) Apply the tape to each stimulation site.
Participants wore the experimental device on their heads and sat in a position where their heads could be tracked by the optical motion capture system. The experimenter presented stimuli at a specified force using the aforementioned preparation. During the preparation, the participants were instructed to keep their face forward after the stimulus was applied, even if they felt a force sensation. Subsequently, the participants sheared the skin of the stimulation site using their hands in the direction opposite to the direction of traction to cancel adaptation, and the measurement of the head rotation angle started after releasing the hands. At this time, the participants were instructed to close their eyes and not resist the felt force. After the participants considered that the head rotation was finished, the rotation angle was recorded as the final angle of the head rotation. The participants were then asked to rate the perceived force on a 7-point Likert scale, with 1 being “no force felt” and 7 being “force felt very strongly”. The correlation between the subjective force intensity and the head rotation angle was observed to determine whether the head rotation angle is a quantified physical quantity of subjective force intensity. After the response, the experimenter removed the rubber band from the stimulating fixation device, placed it on the stimulating fixation device again under different conditions, and repeated the stimulation. A total of eight trials were conducted, one trial for each condition. The order of the conditions was randomized.
Figure 7 shows the experimental results for the correlation analysis of the participant’s head rotation angle and subjective force intensity. The vertical and horizontal axes are the head rotation angle and subjective force intensity, respectively. Spearman’s rank correlation analysis revealed a high positive correlation between the head rotation angle and perceived force intensity (rs = 0.796, p < 0.01). This confirms that the head rotation angle can be treated as a quantification of subjective force intensity. Consequently, we use the head rotation angle in the following discussion.
Figure 8 shows the experimental results of the participant’s head rotation angle when the traction stimulus was applied to the anterior temporal and cheekbone regions. The vertical axis represents the participant’s head rotation angle (positive is in the right rotation direction), and the horizontal axis represents the stimulus intensity condition. A two-way repeated measures ANOVA was conducted to confirm the difference in head rotation angle between the stimulus intensity conditions. The results confirmed that the main effect of the stimulus intensity condition was significant (F(3, 27) = 18.639, MSE = 97.235) and that the main effect of the stimulus position condition was also significant (F(1, 9) = 13.294, MSE = 271.872). No interaction effects between stimulus intensity and stimulus position were identified. Multiple comparisons (Bonferroni method) were performed between stimulus sites to ascertain differences in the head rotation angle for each stimulus site. The results of the multiple comparisons showed significant differences between the 50 gf and other stimulus intensity conditions.
FIGURE 8. Head rotation angle during the anterior part of the temporal head stimulation (***: p < 0.001, *: p < 0.05).
This experiment and analysis aimed to determine the correlation between the amount of skin shear deformation and the angle of head rotation. For this purpose, we measured the skin deformation when the skin shear stimulation using tape was applied to the anterior temporal and cheekbone regions. We then confirmed the correlation between the amount of physical skin deformation and the head rotation angle obtained in the previous experiment.
This analysis was performed with the same 10 participants as the previous experiment to apply it to the experimental data in the previous section. The position of the tapes and the strength of the shear force were the same as in the previous experiment, and measurements were conducted in the same eight conditions. Three trials were conducted for each stimulus condition, for a total of 24 trials. The four corner positions of the tapes were measured using an optical motion capture system (Optitrack Trio, Natural Point, Inc., accuracy 0.5 mm), and the center positions of the tapes were calculated to obtain skin deformation. During the measurement, the face was placed on a fixation device and the participants were instructed not to move (Figure 9).
The average of the three measurements of the skin deformation for each stimulus condition was used for correlation analysis.
Figure 10 shows the results of the correlation analysis between the participant’s head rotation angle obtained in the previous experiment and the amount of skin deformation obtained in this measurement. Pearson’s product-rate correlation analysis showed a moderate positive correlation between head rotation angle and skin deformation (r = 0.575, p < 0.01).
The respective average values of head rotation angle and skin deformation for each stimulus intensity and stimulus position were plotted on the same graph. It was observed that the traction force above 100 gf did not significantly change the amount of skin deformation, as well as head rotation angle, compared to the 100 gf traction force.
The experimental results in Figure 8 show head rotation of approximately 40 degrees in the anterior temporal region and 50 degrees in the cheekbone region. Compared with the result of correlation analysis between the angle of head rotation and subjective force intensity in Figure 7, these rotation angles correspond to 3–5 in subjective force intensity, meaning that the participant felt a slight to moderate sense of force sensation. Therefore, we consider that the illusory force perception in the direction of rotation was generated when only the lateral skin deformation was applied.
Because the head rotation angle changed between stimulus intensities, we theorize that the illusory force intensity can also be controlled by controlling the traction intensity using an actuator. However, whereas significant differences in the head rotation angle between stimulus intensities of 50 gf and other stimulus intensities were observed, no significant differences were observed between stimulus conditions of 100 gf or higher. We consider that there are two possibilities. One is that the illusory force sensation perceived by the participant reached its maximum and saturated. The other possibility is that there was little difference in the amount of skin deformation between the stimulus conditions of 100 gf or higher. Miyakami et al. (2018) reported a correlation between the amount of skin deformation and the intensity of the illusory force sensation, and a similar relationship may be observed in this phenomenon.
An analysis of the head rotation angle and skin deformation (Figure 10) confirmed a positive correlation. It was also observed that the head rotation angle above 100 gf and the amount of skin deformation did not change significantly under the respective stimulus position conditions of the anterior temporal and cheekbone regions. This leads us to believe that the head rotation angle did not change significantly at head rotation angles greater than 100 gf because there was not much change in skin deformation between stimulation conditions above 100 gf.
We believe that the proposed head rotation phenomenon caused by facial skin shear deformation can be incorporated into HMDs to improve the immersive experience of VR.
Tanaka et al. (2022) proposed VR situations using head rotation, for example, when punching in a VR scenario, subject comments confirm that the head rotation of the user being punched improves the immersion of the experience. Kon et al. (2018) also proposed various VR situations that use head rotation, such as an application to support exploration by head rotation in the direction of an object when exploring objects in a VR space. Overall, although the current head rotation is only one degree of freedom of rotation, there are many situations where it can be used to improve the user experience, including immersion, in applications that use head rotation in VR environments, such as boxing and object exploration assistance.
Currently, the skin shear deformation required to generate the head rotation phenomenon is presented using a combination of rubber bands and tape. However, this method cannot dynamically change the traction force, making it difficult to apply to VR using HMDs.
Therefore, we believe that an HMD-integrated force presentation device that enables force-sensing presentation in the direction of rotation can be realized by using a dynamic skin stretching technology with skin traction using a motor, as proposed by Chasm and Masque (Preechayasomboon et al., 2020; Wang et al., 2019), to reproduce the head rotation phenomenon.
This study has several limitations. First, owing to the design of the size of the rubber band fixation device used, it was difficult to present a traction force of less than 50 gf. To confirm whether it is possible to control the illusory force sensation with higher resolution, it is necessary to measure the head rotation angle under stimulus conditions from 0 to 50 gf.
Second, a 7-point Likert scale was used in this study to quantify the subjective force intensity. Although this questionnaire method was used in this study to shorten the experimental time, it is preferable to use a ratio scale when conducting correlation analysis with continuous variables such as the head rotation angle. We plan to quantify subjective force intensity in the future by using a magnitude estimation method that can be measured on a ratio scale.
Third, we presented a stimulus of traction with a rubber band on a tape attached to the participant’s skin. Apparently, the direction of the stimulus was limited to the tangential direction only, and we believe that this setup minimizes pressure (vertical force) against the skin. However, we did not directly measure the vertical force against the skin. Therefore, we plan to measure the vertical force using a pressure sensor or similar device.
Finally, the selection of subjects for the experiment is a limitation. The subjects in this experiment were only relatively young men, which is not common in human perception studies. Since different populations may perceive illusions differently, we need to recruit women in our next step.
This study confirmed the generation of an illusory force sensation by skin shear stimulation on the face and showed that it is possible to control its intensity. Traction stimuli of different intensities were presented. Consequently, a head rotation phenomenon of approximately 40 degrees in the anterior temporal region and approximately 50 degrees in the cheekbone region was generated, confirming an illusory force sensation in the direction of rotation. The head rotation angle was also changed by changing the stimulation intensity, confirming the controllability of the illusory force sensation intensity. The results of correlation analysis between the amount of skin shear deformation measured during traction stimulation and the angle of head rotation confirmed a positive correlation in both the anterior temporal and the cheekbone regions, suggesting that the amount of skin deformation is important for the generation of this phenomenon. Although the importance of lateral skin deformation has been discussed in previous studies, this is the first time that a correlation between skin deformation and head rotation angle has been confirmed in a situation where only lateral skin deformation of different intensities was applied at the same site.
The following are possible applications of the illusory force sensation presentation using this method. The first is to incorporate a mechanism that reproduces skin shear deformation into the HMD. Since both the anterior temporal and cheek regions are in contact with the inner surface of the HMD, it is considered to be easily incorporated into the HMD. Second, a mechanism that reproduces skin shear deformation could be incorporated into respiratory masks. Recent change of society due to the COVID-19 pandemic requires that people wear masks daily. The illusory force sensation using skin shear stimulation to the cheek region could be used as a navigation interface built into (and hidden by) the mask.
In future, we will develop a system that can control skin traction strength with high resolution using motors, shape memory alloys, or other actuators and investigate in detail the generation of illusory force sensation when the amount of lateral skin deformation is further changed.
The raw data supporting the conclusion of this article will be made available by the authors, without undue reservation.
The studies involving human participants were reviewed and approved by the ethics committee of the University of Electro Communications (No. 21074). The participants provided their written informed consent to participate in this study.
MM developed the software setup, implemented the experiments, and analyzed the results with AT. HK provided guidance on various aspects, such as the study design and development of the measurement system. All authors have made substantial direct and intellectual contributions to the article and have approved the submitted version.
This research was supported by JSPS KAKENHI (Grant Number JP20K20627) and JST SPRING (Grant302 Number JPMJSP2131).
The authors would like to thank all the participants who participated in the study for their time and comments that provided a way to improve their work.
The authors declare that the research was conducted in the absence of any commercial or financial relationships that could be construed as a potential conflict of interest.
All claims expressed in this article are solely those of the authors and do not necessarily represent those of their affiliated organizations, or those of the publisher, the editors and the reviewers. Any product that may be evaluated in this article, or claim that may be made by its manufacturer, is not guaranteed or endorsed by the publisher.
Amemiya, T., Ando, H., and Maeda, T. (2005). “Virtual force display: Direction guidance using asymmetric acceleration via periodic translational motion,” in First Joint Eurohaptics Conference and Symposium on Haptic Interfaces for Virtual Environment and Teleoperator Systems. World Haptics Conference (Pisa, Italy: IEEE), 619–622.
Amemiya, T., and Gomi, H. (2014). “Distinct pseudo-attraction force sensation by a thumb-sized vibrator that oscillates asymmetrically,” in International Conference on Human Haptic Sensing and Touch Enabled Computer Applications (Berlin, Germany: Springer), 88–95.
Aoyama, K., Higuchi, D., Sakurai, K., Maeda, T., and Ando, H. (2017). “Gvs ride: Providing a novel experience using a head mounted display and four-pole galvanic vestibular stimulation,” in ACM SIGGRAPH 2017 emerging technologies, 1–2.
Asahi, T., Nakamura, T., Sato, M., Kon, Y., Kajimoto, H., Sato, S., et al. (2020). The hanger reflex: An inexpensive and non-invasive therapeutic modality for dystonia and neurological disorders. Neurol. Med. Chir. (Tokyo). 60, 525–530. doi:10.2176/nmc.ra.2020-0156
Asahi, T., Sato, M., Nakamura, T., Kon, Y., Kajimoto, H., Oyama, G., et al. (2018). Pilot study of a device to induce the hanger reflex in patients with cervical dystonia. Neurol. medico-chirurgica 2018, 206–211.
Clark, J. P., Kim, S. Y., and O’Malley, M. K. (2018). “The rice haptic rocker: Comparing longitudinal and lateral upper-limb skin stretch perception,” in International Conference on Human Haptic Sensing and Touch Enabled Computer Applications (Berlin, Germany: Springer), 125–134.
Colella, N., Bianchi, M., Grioli, G., Bicchi, A., and Catalano, M. G. (2019). A novel skin-stretch haptic device for intuitive control of robotic prostheses and avatars. IEEE Robot. Autom. Lett. 4, 1572–1579. doi:10.1109/lra.2019.2896484
Costes, A., and Lécuyer, A. (2021). The “kinesthetic hmd”: Enhancing self-motion sensations in vr with head-based force feedback. arXiv Prepr. arXiv:2108.10196.
Gugenheimer, J., Wolf, D., Eiriksson, E. R., Maes, P., and Rukzio, E. (2016). “Gyrovr: Simulating inertia in virtual reality using head worn flywheels,” in Proceedings of the 29th Annual Symposium on User Interface Software and Technology, 227–232.
Hamdan, N. A. H., Wagner, A., Voelker, S., Steimle, J., and Borchers, J. (2019). “Springlets: Expressive, flexible and silent on-skin tactile interfaces,” in Proceedings of the 2019 CHI Conference on Human Factors in Computing Systems, 1–14.
Hoppe, M., Oskina, D., Schmidt, A., and Kosch, T. (2021). Odin’s helmet: A head-worn haptic feedback device to simulate g-forces on the human body in virtual reality. Proc. ACM Hum. Comput. Interact. 5, 1–15. doi:10.1145/3461734
Kon, Y., Nakamura, T., and Kajimoto, H. (2017). “Hangeron: A belt-type human walking controller using the hanger reflex haptic illusion,” in ACM SIGGRAPH 2017 emerging technologies, 1–2.
Kon, Y., Nakamura, T., Sakuraqi, R., Shlonolrl, H., Yem, V., and Kajirnoto, H. (2018). “Hangerover: Development of hmo-embedded haptic display using the hanger reflex and vr application,” in 2018 IEEE Conference on Virtual Reality and 3D User Interfaces (VR) (Tuebingen, Germany: IEEE), 765–766.
Kuang, L., Aggravi, M., Giordano, P., and Pacchierotti, C. (2022). “Wearable cutaneous device for applying position/location haptic feedback in navigation applications,” in HAPTICS 2022-IEEE Haptics symposium.
Lécuyer, A. (2009). Simulating haptic feedback using vision: A survey of research and applications of pseudo-haptic feedback. Presence Teleoperators Virtual Environ. 18, 39–53. doi:10.1162/pres.18.1.39
Liu, S. H., Yen, P. C., Mao, Y. H., Lin, Y. H., Chandra, E., Chen, M. Y., et al. (2020). Headblaster: A wearable approach to simulating motion perception using head-mounted air propulsion jets. ACM Trans. Graph. 39, 84. doi:10.1145/3386569.3392482
Minamizawa, K., Kajimoto, H., Kawakami, N., and Tachi, S. (2007). “A wearable haptic display to present the gravity sensation-preliminary observations and device design,” in Second Joint EuroHaptics Conference and Symposium on Haptic Interfaces for Virtual Environment and Teleoperator Systems (WHC’07) (Tsukuba, Japan: IEEE), 133–138.
Miyakami, M., Kon, Y., Nakamura, T., and Kajimoto, H. (2018). “Optimization of the hanger reflex (i): Examining the correlation between skin deformation and illusion intensity,” in International Conference on Human Haptic Sensing and Touch Enabled Computer Applications (Berlin, Germany: Springer), 36–48.
Preechayasomboon, P., Israr, A., and Samad, M. (2020). “Chasm: A screw based expressive compact haptic actuator,” in Proceedings of the 2020 CHI Conference on Human Factors in Computing Systems, 1–13.
Sato, M., Matsue, R., Hashimoto, Y., and Kajimoto, H. (2009). “Development of a head rotation interface by using hanger reflex,” in RO-MAN 2009-The 18th IEEE International Symposium on Robot and Human Interactive Communication (Toyama, Japan: IEEE), 534–538.
Sato, M., Nakamura, T., and Kajimoto, H. (2014). “Movement and pseudo-haptics induced by skin lateral deformation in hanger reflex,” in SIG TeleXistence 5th workshop.
Sra, M., Jain, A., and Maes, P. (2019). “Adding proprioceptive feedback to virtual reality experiences using galvanic vestibular stimulation,” in Proceedings of the 2019 CHI Conference on Human Factors in Computing Systems, 1–14.
Tanabe, T., Yano, H., and Iwata, H. (2017). Evaluation of the perceptual characteristics of a force induced by asymmetric vibrations. IEEE Trans. Haptics 11, 220–231. doi:10.1109/toh.2017.2743717
Tanaka, Y., Nishida, J., and Lopes, P. (2022). “Electrical head actuation: Enabling interactive systems to directly manipulate head orientation,” in CHI Conference on Human Factors in Computing Systems, 1–15.
Teo, T., Nakamura, F., Sugimoto, M., Verhulst, A., Lee, G. A., Billinghurst, M., et al. (2020). “Feel it: Using proprioceptive and haptic feedback for interaction with virtual embodiment,” in ACM SIGGRAPH 2020 emerging technologies, 1–2.
Utz, K. S., Korluss, K., Schmidt, L., Rosenthal, A., Oppenländer, K., Keller, I., et al. (2011). Minor adverse effects of galvanic vestibular stimulation in persons with stroke and healthy individuals. Brain Inj. 25, 1058–1069. doi:10.3109/02699052.2011.607789
Keywords: illusory force sensation, lateral skin stretch, head rotation, haptics, virtual reality
Citation: Miyakami M, Takahashi A and Kajimoto H (2022) Head rotation and illusory force sensation by lateral skin stretch on the face. Front. Virtual Real. 3:930848. doi: 10.3389/frvir.2022.930848
Received: 28 April 2022; Accepted: 28 June 2022;
Published: 09 August 2022.
Edited by:
Luca Turchet, University of Trento, ItalyReviewed by:
Seongkook Heo, University of Virginia, United StatesCopyright © 2022 Miyakami, Takahashi and Kajimoto. This is an open-access article distributed under the terms of the Creative Commons Attribution License (CC BY). The use, distribution or reproduction in other forums is permitted, provided the original author(s) and the copyright owner(s) are credited and that the original publication in this journal is cited, in accordance with accepted academic practice. No use, distribution or reproduction is permitted which does not comply with these terms.
*Correspondence: Masahiro Miyakami, bWl5YWthbWlAa2FqaS1sYWIuanA=
Disclaimer: All claims expressed in this article are solely those of the authors and do not necessarily represent those of their affiliated organizations, or those of the publisher, the editors and the reviewers. Any product that may be evaluated in this article or claim that may be made by its manufacturer is not guaranteed or endorsed by the publisher.
Research integrity at Frontiers
Learn more about the work of our research integrity team to safeguard the quality of each article we publish.