- 1School of Behavioural and Social Sciences, Brescia University College, London, ON, Canada
- 2Department of Psychology, Western University, London, ON, Canada
The ability to create an accurate mental survey representation, or cognitive map, when moving through an environment varies widely across individuals, and we are still trying to understand the origins of these individual differences. Non-immersive virtual environments used to test for cognitive map accuracy in the laboratory have shown sex differences with a performance advantage for men in some studies but not others. When sex differences are demonstrated, it is unclear whether women’s performance generalizes to familiar and unfamiliar real-world environments. In Experiment 1, 98 participants explored the virtual environment Silcton and afterwards estimated directions between the landmarks in Silcton and arranged landmarks found in Silcton on a map. In addition, they reported frequently visited real-world locations and then estimated directions between them and drew a map of the locations. Men were more accurate on tests of Silcton than women were, although there was no difference between sexes for accuracy with real-world locations. Within sexes, women were more accurate with the real-world locations than Silcton, while men showed the opposite pattern. In Experiment 2, 21 women were tested with Silcton and their familiar real-world locations as in Experiment 1 but were also walked through an unfamiliar real-world area on campus and completed direction estimation and map drawing tests for the new environment. Overall, women were more accurate with the two real-world environments than Silcton, with some evidence that accuracy with the new real-world environment was more accurate than the familiar real-world locations. Overall, women’s ability to create a cognitive map of a virtual environment in the laboratory does not seem to be indicative of their ability to do the same in the real world, and care should be taken when generalizing lab results with virtual environments.
1 Introduction
When travelling in a new place, individuals create mental representations of the environment to help guide their way. The most flexible and powerful of these representations is a survey representation, or cognitive map, which is an orientation-independent representation of landmark configurations that allows a traveler to improvise during a journey via short-cuts and detours (Tolman, 1948; O’Keefe and Nadel, 1978). On the other hand, relying only on memory for the sequence of landmarks along a route does not allow the same on-the-fly flexibility, because the traveler’s knowledge cannot extrapolate their spatial position beyond the specific route (Siegel and White, 1975). Thus, cognitive maps confer a distinct navigation advantage, although their existence is not without controversy (Bennett, 1996; Warren et al., 2017; Weisberg and Newcombe, 2018).
Theoretical proposals about the development of cognitive maps within individuals have been offered by Siegel and White (1975) and Montello (1998). Both models describe the acquisition of learning about landmarks, routes, and creating a map, but the sequence of learning and the final outcome differ. In Siegel and White’s model, individuals pass through stages that start with identifying and learning the names of individual landmarks. In the next stage, landmarks are associated with turns and remembered as a sequence along routes, and in the final stage, those routes are integrated into a single cognitive map. While the elements of learning are similar in Montello’s proposal (i.e., landmarks, routes, cognitive map), the sequence is not fixed and individual differences are considered. Montello suggested that some individuals may learn about landmarks and routes while simultaneously building a map, instead of in a stepwise fashion, and may do so from very early in the exploration process. Furthermore, Montello proposed that even with equal exposure to the environment, individuals will vary in the rate of acquiring these elements, and that not everyone will create a cognitive map representation and instead would rely on more or less accurate route and landmark information.
It is now clear that the kind of variation in cognitive map creation proposed by Montello (1998) occurs when people learn the spatial properties of both real-world environments (Ishikawa and Montello, 2006; Schinazi et al., 2013) and non-immersive virtual environments (i.e., virtual environments presented on a desktop computer monitor, rather than immersive virtual environments presented through a wearable headset; Weisberg and Newcombe, 2016; Youngson et al., 2019). For instance, Ishikawa and Montello (2006) introduced a route integration method of testing the accuracy of cognitive maps in a real-world setting that has now also been implemented virtually. Participants in route integration studies learn the names of landmarks along two separate routes in an environment and are then transported along one or more additional routes that serve to connect the routes with the previously learned landmarks. In order to test an individual’s mental spatial representation of the environment, participants are positioned at each landmark and are asked to point in the direction of each of the other landmarks (sometimes called a judgement of relative direction task). Pointing accurately to landmarks on the same route is taken as an indicator of good route knowledge, while the ability to accurately point to landmarks on the other route is taken as an indicator that the routes have been integrated into a mental cognitive map. Pointing accurately to an unseen landmark on another route can be thought of as a proxy for the ability to take a shortcut to the landmark from the current position, a hallmark of cognitive maps. Other tests including map drawing or arranging landmarks on a blank map have also been used as a second indicator of cognitive map accuracy. Broad individual differences in cognitive map use in a real-world environment were shown by Ishikawa and Montello, and similar methods in the virtual environment Silcton indicate that only a portion of participants create a cognitive map, while others remember specific routes and some have only weak knowledge of both routes and a cognitive map (Weisberg and Newcombe, 2016; Galati et al., 2018; Youngson et al., 2019). Thus, the variation in cognitive map ability suggested by Montello has been supported, and there is some evidence that differences in working memory, specific spatial skills, and personality factors contribute to these differences (Blacker et al., 2017; see Weisberg and Newcombe, 2018 for a review).
In addition to cognitive and personality factors, sex differences in cognitive map accuracy showing an advantage for men have been reported using Silcton and other virtual environments, but conclusions are not clear-cut (Castelli et al., 2008; Weisberg and Newcombe, 2016; Boone et al., 2018; Youngson et al., 2019). For instance, there is some indication that the male advantage in virtual environments might be accounted for in part by gaming and computer experience (Richardson et al., 2011; Nowak et al., 2015) or spatial perspective-taking skills (Youngson et al., 2019, Experiment 1). Conversely, some studies using the Silcton virtual environment show no sex differences (Weisberg et al., 2014; Nazareth et al., 2018; Youngson et al., 2019, Experiment 2; Pagkratidou et al., 2020). Overall, sex differences in cognitive map accuracy indicating women form less accurate cognitive maps than men seem to be fleeting yet are undeniably present in some studies.
It may be tempting to generalize from the results with virtual environments that women not only form weaker cognitive maps than men do in virtual reality (at least sometimes), but that they also form weaker cognitive maps than men do when navigating in their daily real-world lives. However, it is unclear whether women’s weaker performance in virtual reality means they fall into Montello’s (1998) category of people who never form cognitive maps in any environment, or whether they form such representations but at a different rate than men do, perhaps a rate that is not optimized in virtual studies. Multiple cues useful for navigation are impoverished in virtual reality compared to the real world, including auditory cues, kinesthetic feedback, and optic flow, and it could be that women rely more on these cues for navigation and building spatial mental representations than men do. In the current experiments, we were first interested in whether the accuracy of women’s cognitive maps of Silcton was related to the accuracy of their cognitive maps of their everyday, familiar, real-world environment, and whether there would be sex differences in these effects (Experiment 1). Second, we were also interested in comparing the accuracy of women’s cognitive maps of Silcton and their everyday familiar environment, plus an unfamiliar, real-world environment, and so we collected a second data set to explore these comparisons (Experiment 2).
2 Experiment 1
In Experiment 1, we compared the accuracy of women’s and men’s cognitive map representations of the virtual environment Silcton with the mental representations they held of their familiar everyday environment. We also measured participants’ spatial perspective taking skills, as we have found those to account for some of the sex differences in Silcton in a prior study (Youngson et al., 2019, Experiment 1). If performance in Silcton is an accurate indicator of women’s ability to create cognitive maps of their familiar real-world surroundings, we should see similar performance in the Silcton environment and the real-world home environment tests. In addition, if the sex differences seen with Silcton are an accurate indicator of differences in the real world, we should see that men show greater cognitive map accuracy than women in both environments. If, however, women’s performance in Silcton is due to the limited cues available in non-immersive virtual reality and/or if the rate of learning is not optimal in Silcton, we should see better cognitive map accuracy with familiar real-world locations. Finally, if the sex differences seen with Silcton are a function of testing in virtual reality and not indicative of everyday spatial mental representations, we should see that while men may perform better than women in Silcton, performance should be equivalent between sexes with real-world locations.
2.1 Method
2.1.1 Participants
An a priori power analysis using G*Power (Faul et al., 2007) was conducted. For a mixed ANOVA with 2 (environment: Silcton, real-world) within-subjects and 2 (sex: male, female) between-subjects conditions, 90 participants were required to detect a between-subjects main effect with a medium effect size (Cohen’s f = 0.25) and power of 0.80 (the within-subjects main effect and the interaction required fewer subjects for power of 0.80). Ninety-eight participants (48 self-identifying as women, 50 self-identifying as men) between the ages of 19 and 46 years (M = 20.00, SD = 3.97) completed the study. All participants were recruited through research participation pools at Western University and Brescia University College and received course credit for participation. All aspects of the study were approved by the Non-Medical Research Ethics Board at Western University and the Brescia Research Ethics Board at Brescia University College.
2.1.2 Materials and Procedure
Participants completed a combination of paper-and-pencil and computer-based tasks. All computer-based tasks were presented on a 15” Toshiba laptop running Windows 8.1 with a 64-bit Intel Core Processor at 2.40 GHz.
2.1.2.1 Demographic Questionnaire
The demographic questionnaire was completed with paper and pencil. Participants provided information on age, sex, year in university, how long they had resided in London, ON, and how often they played video games (rated on a scale of 0 = less than once per week to 4 = more than six times per week).
2.1.2.2 Spatial Orientation Test (SOT)
The SOT (Hegarty and Waller, 2004) measured spatial perspective-taking ability, and participants completed the 12 items with paper and pencil. Each item was presented on a separate page and showed an array of objects in the top half of the page. The same array was used for all 12 items. Participants were instructed to imagine they were standing at one object in the array while facing a second object, and to indicate the direction of a third object in the array on the bottom half of the sheet. The direction of the third object relative to the assumed heading was indicated by drawing a line from the center of a circle in the direction of the third object. Error in degrees was calculated as the absolute difference between the angle of the line drawn by the participant and the actual angle of the third object, and error scores were averaged across the items for each participant. Participants were given 5 min to complete the test. If a participant did not finish the 12 items within 5 min, average error score was calculated from the items that were completed.
2.1.2.3 Real-World Location Gathering
Using a pencil and a paper form, participants provided a minimum of four and maximum of 10 locations in London, ON that they visited frequently (e.g., home, grocery store, university building, etc.). They also rated how frequently they visited each location each week as either less than once per week, 1–2 times per week, 3–4 times per week, 5–6 times per week or more than six times per week. Afterwards, the researcher looked up each location using Google Maps and obtained the latitude and longitude coordinates for the location in order to score the real-world direction estimation and map tasks. The four locations most frequently visited by the participant were used as the locations for the direction estimation and sketch map tasks that followed.
2.1.2.4 Real-World Direction Estimation
This paper and pencil task was designed to be similar to the SOT (Hegarty and Waller, 2004) in terms of instructions to the participant (imagine you are at A, facing B, now indicate the direction of C), but the locations used were those most often visited by the participant as reported in the Real-World Location Gathering task. First, the researcher assigned each of the four most-frequently-visited locations a letter (A, B, C, or D). On each of the 24 items of the test, participants were instructed to imagine standing at one of the four locations (e.g., A—home) facing another location (e.g., B—grocery store) and to estimate the direction to a third target location (e.g., C—university classroom building). To facilitate this, each page of the test had a circle with a line from the center of the circle to the top of the circle. A letter at the center indicated the standing location, a letter at the top of the circle indicated the imagined heading, and the target location was indicated outside the circle at the top of the page. Participants were instructed to draw a line from the center standing position to correspond to the direction of the target from that heading. For buildings, participants were instructed to imagine standing at the front door. The accuracy of the participant’s heading was scored as the absolute error in degrees from the estimated direction to the actual direction. All locations served in each position an equal number of times across the 24 items.
2.1.2.5 Real-World Sketch Map
Next, participants drew a sketch map using paper and pencil of the four real-world locations used in the direction estimation task. The paper showed the outline of a square with “North” at the top and participants were instructed to draw the four locations within the square and label each one. Map accuracy was scored using Gardony Map Drawing Analyzer (Gardony et al., 2016). The software compared the map the participants drew to the actual latitude and longitude coordinates of the locations in the real-world and produced an R2 value using bidimensional regression, with values closer to 1.0 indicating greater concordance between the participant’s map and the actual map of locations.
2.1.2.6 Silcton Practice and Free Exploration
Next, participants explored the Silcton virtual environment (Figure 1; Weisberg et al., 2014) on the laptop using the arrow keys to move forward, backward and to the left or right. The mouse was used to look around in the environment. Participants first practiced moving in Silcton by moving around a statue in the roundabout in the center of the environment. Next, the researcher showed them an example of the floating diamonds that marked each to-be-remembered building. Once participants were comfortable with the controls, the exploration period started and lasted for a minimum of 10 and a maximum of 20 min. Participants traveled freely through Silcton and looked for and tried to remember the locations of eight buildings marked with a floating diamond. Participants were given a list of the eight target building names and marked off each one once it was located. They could end the exploration session when they felt they had found all the buildings and were confident in their locations, within the 10-min minimum and 20-min maximum constraints.
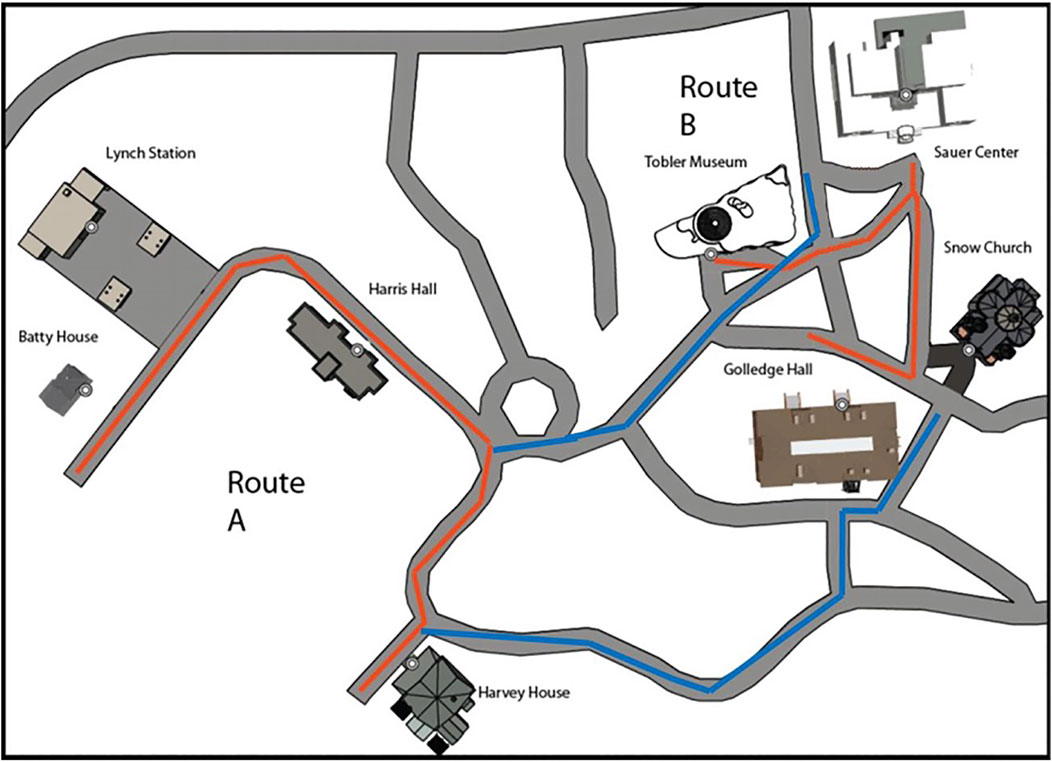
FIGURE 1. Overhead view of the Silcton environment including the two main routes A (in red or lighter gray that connects Batty House, Lynch Station, Harris Hall, and Harvey House) and B (in red or lighter gray that connects Tobler Museum, Sauer Center, Snow Church, and Golledge Hall) and the two connector routes (in blue or darker gray). From “Variations in Cognitive Maps: Understanding Individual Differences in Navigation,” by S. M. Weisberg, V. R. Schinazi, N. S. Newcombe, T. F. Shipley, and R. A. Epstein, 2014, Journal of Experimental Psychology: Learning, Memory, and Cognition, 40 (3), 671. Copyright 2013 by the American Psychological Association.
2.1.2.7 Silcton Direction Estimation
Immediately after completing the Silcton exploration task, participants completed the Onsite Direction Task from the Silcton software suite. On each of 56 trials, the participant was placed in front of the front door of one of the eight target buildings in Silcton, facing out. Words on the screen instructed them to point a crosshairs image in the direction of another of the eight buildings. The absolute difference between the angle indicated by the participant and the correct angle was recorded in the software on each trial. Each of the eight target buildings served as the standing location, and directions were estimated for the other seven buildings.
2.1.2.8 Silcton Map Building Task
After estimating directions in Silcton, participants completed a map building task (called Model Building in the Silcton software package). Using the mouse, participants dragged and dropped overhead views of the eight target buildings into position on a blank map. The accuracy of the participants’ building locations was determined in the Silcton software using bidimensional regression and produced an R2 value ranging from 0–1.0, with higher values indicating greater accuracy.
2.2 Results
All statistical analyses were performed using JASP verion 0.14.1 (JASP Team, 2020).
2.2.1 Sex × Environment Mixed ANOVAs
First, we conducted a 2 (Sex: men, women) × 2 (Environment: Silcton, real world) mixed ANOVA for the direction estimation error scores. There was a significant main effect of Sex with men (M = 37.93, SD = 15.80) showing overall lower estimation error (i.e., higher accuracy) than women (M = 56.03, SD = 11.78), F (1, 96) = 41.04, p < 0.001, η2p = 0.30, but the main effect of Environment (Silcton: M = 46.42, SD = 22.02; real world: M = 47.12, SD = 22.41) was not significant, F (1, 96) = 0.03, p = 0.87, η2p = 0.00. The Sex × Environment interaction was significant, F (1, 96) = 65.57, p < 0.001, η2p = 0.41. Analyses of the simple main effects of Sex at each level of Environment for the direction estimations revealed that men (M = 47.46, SD = 23.30) and women (M = 46.86, SD = 21.69) did not significantly differ in real-world direction estimation error, F (1, 96) = 0.02, p = 0.88, but for Silcton, men (M = 28.40, SD = 13.31) were significantly more accurate (i.e., showed lower error) than women (M = 65.19, SD = 10.53), F (1, 96) = 229.21, p < 0.001. Therefore, men estimated directions between buildings in Silcton more accurately than women, but there was no difference between sexes for real-world locations that the participants provided themselves. Figure 2 shows the means, standard errors, and individual scores for men and women in both environments.
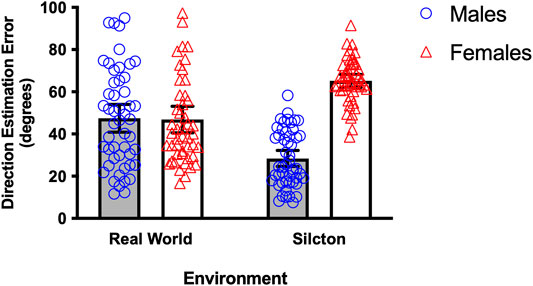
FIGURE 2. Direction estimation error means and individual scores for women and men estimating directions between landmarks in Silcton and the real-world locations they provided in Experiment 1. Horizontal scatter of the individual scores corresponds to the number of scores at that value (wider = more scores). Error bars show 95% confidence intervals.
Next, we conducted a similar 2 (Sex: men, women) × 2 (Environment: Silcton, real world) mixed ANOVA for the map creation scores, and these data can be seen in Figure 3. There was a significant main effect of Environment, F (1, 96) = 21.49, p < 0.001, η2p = 0.18, indicating that maps created of real-world locations (M = 0.71, SD = 0.26) were more accurate than those created of Silcton (M = 0.54, SD = 0.30). In addition, there was a significant main effect of Sex, F (1, 96) = 4.69, p = 0.03, η2p = 0.05, with men (M = 0.67, SD = 0.26) creating more accurate maps overall than women (M = 0.58, SD = 0.26). The Sex × Environment interaction was also significant, F (1, 96) = 15.67, p < 0.001, η2p = 0.14. Simple main effects analyses of sex for each environment showed that men were more accurate at building maps of Silcton than women were (men: M = 0.66, SD = 0.26; women: M = 0.43, SD = 0.29), F (1, 96) = 17.23, p < 0.001, but there was no difference between sexes (men: M = 0.68, SD = 0.25; women: M = 0.74, SD = 0.26) for maps drawn of familiar locations in the real world, F (1, 96) = 1.30, p = 0.26.
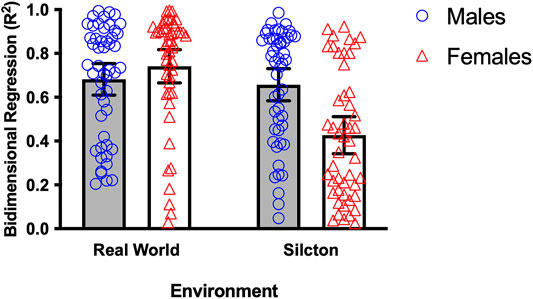
FIGURE 3. Bidimensional regression means and individual scores for women and men creating maps of Silcton and the real-world locations the participants provided in Experiment 1. Horizontal scatter of the individual scores corresponds to the number of scores at that value (wider = more scores). Error bars show 95% confidence intervals.
We were also interested in whether performance with the virtual environment was an accurate reflection of performance in the real world for each sex. For instance, inspection of Figure 3 suggests that women’s scores on the Silcton map creation task were lower than their real-world map scores, whereas men’s scores appear similar. Therefore, we conducted the alternate set of simple main effects analyses of environment for each sex. For the map creation tasks, men did not show a significant difference between maps created of Silcton (M = 0.66, SD = 0.26) and the real-world locations (M = 0.68, SD = 0.25), F (1, 96) = 0.23, p = 0.63. Women, however, were significantly better at creating maps of the real-world locations (M = 0.74, SD = 0.26) than of Silcton (M = 0.43, SD = 0.29), F (1, 96) = 36.19, p < 0.001. For the direction estimation tasks, women were significantly more accurate (i.e., they showed lower error) at estimating directions between locations in the real world (M = 46.86, SD = 21.69) than in Silcton (M = 65.19, SD = 10.53), F (1, 96) = 30.88, p < 0.001. Men also showed a significant difference on direction estimation between environments, F (1, 96) = 34.79, p < 0.001, but the pattern of means was opposite to women’s: Men were significantly more accurate estimating directions of locations in Silcton (M = 28.40, SD = 13.31) than locations in the real world (M = 47.46, SD = 23.30).
2.2.2 Pearson Correlations
In order to assess the associations of sex, spatial perspective taking on the SOT, gaming frequency, and time living in the current city with accuracy on the Silcton and real-world direction estimation and map building tasks, Pearson correlations were conducted and are shown in Table 1, along with means and standard deviations for all variables. As can be seen in Table 1, the length of time participants had lived in the city and gaming frequency were not significantly associated with any other variables. In addition, SOT score was significantly correlated with direction estimation performance for both Silcton and real-world locations, and it was significantly associated with the accuracy of maps created of Silcton, but not of real-world locations. Next, and as would be expected, performance on the Silcton tasks (direction estimation and map creation) were significantly correlated, as was performance on the corresponding tasks with real-world locations. Across environments, only Silcton map creation was significantly correlated with real-world direction estimation. Finally, sex showed significant correlations indicating an advantage for men with Silcton direction estimation, SOT scores, and Silcton map creation.
Given that women seemed to have relatively weaker performance on the Silcton tasks, the relationship of video game playing and the Silcton tasks was investigated further. Specifically, the lack of correlations between the frequency of playing video games and Silcton assessments may differ for each sex, so additional correlations were conducted to confirm that the lack of a correlation was true for both sexes. For men, there was no significant correlation between the frequency of video game use (M = 1.54, SD = 1.33) and Silcton direction estimation, r (48) = −0.16, p = 0.26, and no significant correlation between video gaming and Silcton map creation, r (48) = 0.01, p = 0.97. For women, however, the correlation between video game playing (M = 1.44, SD = 1.64) and Silcton map creation was significant, r (46) = 0.30, p = 0.04, and the correlation of video games with Silcton direction estimation approached significance, r (46) = 0.24, p = 0.095.
2.2.3 Mediation and Linear Regression
To investigate the association of both sex and SOT scores with performance on the two Silcton tasks, an exploratory mediation analysis was conducted to determine whether the effect of sex on the Silcton tasks was fully or partially mediated by perspective taking. The mediation was performed using the mediation feature of the JASP (JASP Team, 2020) structural equation modeling add-in with 95% confidence intervals estimated using a bias-corrected bootstrap procedure with 1,000 replications. The predictor variable was sex, the outcome variables were Silcton direction estimation and Silcton map creation, and the mediating variable was SOT score. The analysis showed a significant indirect effect of sex on Silcton map creation performance with SOT score as a mediator, b = −0.06, SE = 0.03, 95%CI = (−0.13, −0.01), p = 0.03. In addition, the direct effect of sex on Silcton map creation (i.e., with SOT held constant) was significant, b = −0.17, SE = 0.06, 95%CI = (−0.30, −0.04), p = 0.003, indicating that the effect of sex on Silcton map creation was partially, but not fully, mediated by perspective taking on the SOT. For the Silcton onsite pointing outcome variable, the indirect effect of sex with SOT as a mediator only approached significance, b = 2.22, SE = 1.77, 95%CI = (0.07, 5.14), p = 0.06, while the direct effect of sex on Silcton onsite pointing was significant b = 34.57, SE = 2.59, 95%CI = (29.73, 38.73), p < 0.001. It should also be noted that linear regression showed that SOT scores alone were a significant predictor of Silcton direction estimation performance, β = 0.39, p < 0.001, and the model accounted for a significant proportion of variance in Silcton direction estimation error scores, R2 = 0.20, F (1, 96) = 24.19, p < 0.001. Therefore, the effect of sex on Silcton direction estimation performance was not mediated by SOT scores, but SOT scores on their own did predict Silcton direction estimation scores.
Finally, we used linear regression to investigate whether SOT scores were a significant predictor of direction estimation for real-world locations. SOT scores were a significant predictor of real-world direction estimation scores, β = 0.31, p = 0.002, and the model accounted for a significant proportion of variance in real-world direction estimation error scores, R2 = 0.10, F (1, 96) = 10.12, p = 0.002.
2.3 Discussion
In Experiment 1, we replicated previous studies demonstrating an advantage for men on tasks that assess cognitive map accuracy of items in a virtual environment but showed that for cognitive maps of familiar real-world locations, there were no sex differences. Spatial perspective-taking skill was also important for direction estimation and map creation based on a virtual environment, and direction estimation of familiar real-world locations. For women, playing video games was associated with one of the Silcton assessments. Furthermore, perspective taking partially mediated the sex differences in direction estimation between locations in the virtual environment, providing a potential mechanism for those differences.
Our participants’ cognitive map accuracy for virtual environment locations was not a strong indication of the accuracy of their cognitive maps of familiar real-world environments. Women performed worse on the tasks based on the virtual environment than those based on familiar real-world locations. On the other hand, men either showed equivalent accuracy for the two environments (for map creation) or were more accurate in the virtual environment than for real-world locations (direction estimation). This suggests that testing spatial mental representations based on virtual environments explored in the lab may be underestimating women’s accuracy in “real life” and, for some tasks, it may overestimate men’s accuracy.
Importantly, our tasks differed not only in their virtual vs. real-world nature, but also in the degree of familiarity participants had with each environment. This means it is not entirely clear whether performance differences across environments was due to the virtual or real-world nature of the locations or to the novelty of Silcton and the familiarity of the locations participants provided. Montello (1998) proposed that increasing exposure to an environment should lead to a more accurate mental representation that would be more map-like. It could be that our participants were so familiar with the locations they reported to us that everyone’s performance appeared bolstered when compared to a novel virtual environment.
There were also some important differences between the way the direction estimation and map tasks were carried out, with pencil and paper responses for the real-world tasks and computerized tasks for Silcton. In particular, the direction estimation task for Silcton placed the participant back in the environment, while the real-world task was done away from the locations provided by the participant. In addition, participants freely explored Silcton rather than being constrained to specific routes as in typical route integration studies. In Experiment 2, we attempted to address these limitations.
3 Experiment 2
In Experiment 2, we added a novel real-world environment to the virtual Silcton and familiar real-world conditions to begin to tease apart whether familiarity or presentation modality of the locations was the critical factor in performance differences in Experiment 1. In particular, we were interested in whether women’s weaker performance on spatial tasks of Silcton was due to the unfamiliarity of Silcton, suggesting weaker cognitive mapping ability in a new environment, or the virtual nature of Silcton, suggesting that the issue was learning in a non-immersive, virtual reality modality. We tested a small sample of women both in the lab and outdoors in summer and fall 2019 before weather conditions, and then the COVID-19 pandemic, halted testing. In addition, a new building was erected at the outdoor testing site which substantially altered the environment by mid-2020. Therefore, further testing in this area was not possible, and we report the data in Experiment 2 as an exploratory investigation to motivate and inform further research, and a chance to replicate some of the findings from Experiment 1.
We predicted that if women’s relatively weak performance with Silcton in Experiment 1 was due to difficulty learning a new environment, we should see similar weak performance in tests based on the novel real-world environment, i.e., both should be significantly worse than the familiar environment. On the other hand, if weak performance with Silcton in Experiment 1 was due to the virtual nature of the environment and not its novelty, we should see performance with the novel real-world environment be similar to the familiar real-world environment, with weaker performance on virtual Silcton tasks.
3.1 Method
3.1.1 Participants
Twenty-one women who ranged in age from 18 to 38 years (M = 24.19, SD = 5.27) were recruited from the Brescia University College and Western University communities. All participants completed all aspects of the study and received monetary remuneration. The study was approved by the Non-Medical Research Ethics Board at Western University and the Research Ethics Boards at Brescia University College and Huron University College. Participants were tested in summer and fall 2019, before the study was discontinued.
3.1.2 Materials and Procedure
3.1.2.1 Demographic Questionnaire and SOT
Participants completed the demographic questionnaire from Experiment 1 with the addition of questions assessing their familiarity with the Huron campus: Are you familiar with Huron’s campus? If participants responded “yes”, they rated their level of familiarity with the campus (How familiar are you with Huron’s campus?, with responses on a 5-point scale from Not at all familiar to Extremely familiar, and Overall, how frequently do you go to this location?, with responses on a 5-point scale from Less than once per week to More than six times per week). The SOT was also completed as in Experiment 1.
3.1.2.2 Novel Real-World Exploration
After completing the demographic questionnaire and the SOT, participants were led outside by the experimenter to explore a nearby area of campus that students from the recruitment population typically would rarely visit, Huron University College. Figure 4 shows a map of the testing area. After completing both the demographic questionnaire and the SOT in a room at Brescia University College, participants walked with the researcher to Huron along Western Rd to the starting location on the path next to the Dining Hall (1), indicated by the pink circle in Figure 4. The researcher explained to the participant that they would walk around Huron and would be shown eight buildings by the researcher. The participant’s task would be to try to remember the names and locations of the eight buildings indicated by the researcher. Participants were led first along route A and back, and four buildings were pointed out along the way: Dining Hall (1), O’Neill Ridley Hall (9), Chapel (13), and Lucas Alumni House (16). Once back at the starting location, they were led along the connector route and then onto route B, where they were shown four more buildings: Southwest Residence (3), Young House (20), Brough House (19), and Henderson House (18). All buildings had a clear sign showing the building’s name, which the researcher pointed to.
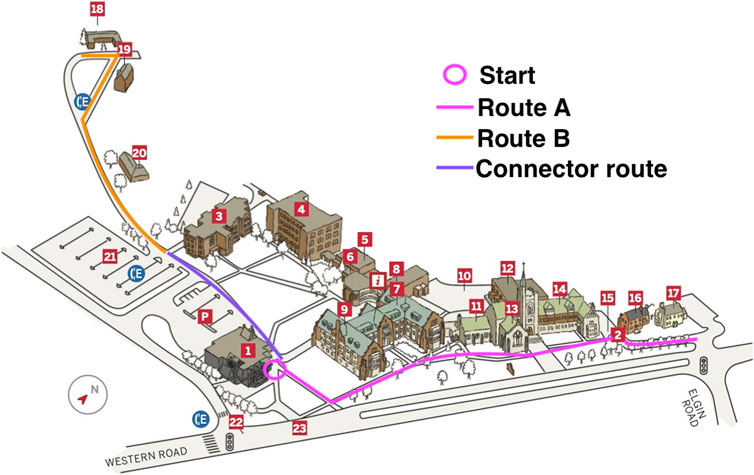
FIGURE 4. Map of the Huron University College campus showing the starting point, routes A and B, and the connector route. Buildings 3, 20, 19, and 18 were landmarks on route A, and buildings 1, 9, 13, and 16 were landmarks on route B. Adapted from Huron’s Campus Map, by Huron University College, 2021 (https://huronatwestern.ca/form-centre/hurons-campus-map/). Copyright 2021 by Huron University College. Adapted with permission.
3.1.2.3 Novel Real-World Direction Estimation and Map
After completing route B, the researcher and the participant went to the Huron library (12) via a new path to complete the rest of the tasks. First, they completed a paper-and-pencil direction estimation task, similar in format to the familiar real-world direction estimation task from Experiment 1, that used the eight Huron campus buildings. Each page consisted of a circle with a building name in the middle where the participant was to imagine standing, and a building at the top of the circle, which the participant was to imagine facing. Participants were asked to draw lines indicating the directions of the six other buildings from this imagined heading. Each building was in the center of the circle once and at the top once over 16 trials. After the novel real-world direction estimation task, participants drew a sketch map of the eight Huron buildings. Both tasks were scored in the same way as the real-world direction estimation and map tasks from Experiment 1.
3.1.2.4 Familiar Real-World and Silcton Tasks
After the Huron tasks, participants completed the familiar real-world tasks (location gathering, direction estimation, sketch map) from Experiment 1 and modified Silcton tasks (route exploration, direction estimation, map creation). First, instead of freely exploring Silcton as in Experiment 1, participants traveled in Silcton using the “route learning” function, where they explored each of two separate routes, labelled A and B in Figure 1 (each route had four of the eight target buildings) and then the connecting routes (route C and D). Participants traveled from the start of each route to the route’s end and then back to the start and were given as much time as they needed to do so. Second, the Silcton direction estimation task was changed to make it more similar to the real-world direction estimation tasks. Instead of the onsite direction estimation task as described in Experiment 1, participants completed the “offsite pointing” direction estimation task in the Silcton software suite, which was outside the environment but still on the laptop. Participants in this task were shown a circle with one of the target buildings at the top and one at the centre. They were instructed to imagine they were standing at the building in the centre of the circle, facing the building at the top of the circle, and to drag and drop images of the other six buildings into their correct relative locations around the edge of the circle. They completed eight circles, with each building presented as both the top and the centre building once each. While not identical in format, this made the Silcton direction estimation task more comparable to the real-world direction estimation task. Finally, they sketched a map of Silcton with paper and pencil, as they had for the novel and familiar real-world locations, instead of dragging and dropping overhead views of the buildings into position, as in Experiment 1.
3.2 Results
All statistical analyses for Experiment 2 were performed using JASP version 0.14.1 (JASP Team, 2020).
3.2.1 Video Games, SOT Scores, and Familiarity With the Huron Campus
Ten of the 21 women tested reported playing video games. Five of those participants reported playing less than once per week, three reported playing 1–2 times per week, one reported playing 5–6 times per week, and one reported playing more than six times per week. Video games were not correlated with performance on Silcton measures [direction estimation: r (19) = 0.12, p = 0.61; map creation: r (19) = 0.27, p = 0.23] or familiar real-world location measures [direction estimation: r (19) = −0.34, p = 0.13; map creation: r (19) = 0.01, p = 0.97]. Video games were correlated with maps created of the novel real-world locations, r (19) = −0.45, p = 0.04, but not direction estimations based on those locations, r (19) = 0.01, p = 0.67. These correlation results must be considered only suggestive, given the small number of participants in this study.
Women’s performance on the SOT (M = 38.19, SD = 23.23) was better in this sample (i.e., error was lower) than women’s performance in Experiment 1 (M = 45.57, SD = 28.75).
Only three of the 21 participants in the study reported being at all familiar with Huron’s campus. Of those three participants, all reported that they were only “slightly familiar” with the campus (2 on the 1–5 scale). For frequency of visits to Huron, two of the three reported going to Huron less than once per week, and one reported going to Huron 1–2 times per week. Therefore, none of our participants had extensive knowledge of Huron, and most had never visited the campus before. We included the data of the three participants who had some experience with Huron.
3.2.2 Direction Estimation Across the Novel Real-World, Familiar Real-World, and Silcton Environments
Figure 5 shows the means, 95% confidence intervals, and individual participant scores for the three environments. Due to the low sample size and consequent low power to detect a medium effect with a traditional frequentist repeated-measures ANOVA test (0.59), we compared performance for the three environments using nonparametric frequentist and Bayesian statistics. First, using Friedman’s Test, we found a significant difference in direction estimation error across the familiar real-world (M = 53.00, SD = 25.95), novel real-world (M = 37.04, SD = 13.66), and Silcton virtual environments (M = 75.62, SD = 7.19), χ2 (2) = 24.67, p < 0.001. Posthoc pairwise comparisons using a Bonferroni-corrected Conover test showed that there was not a significant difference in direction estimation error between participants’ performance with familiar real-world locations and the novel real-world locations, t (40) = 2.01, p = 0.16. However, direction estimation using familiar real-world locations was significantly lower (i.e., more accurate) than direction estimation error for Silcton buildings, t (40) = 2.93, p = 0.02. Finally, direction estimation of the novel real-world environment buildings was more accurate than Silcton direction estimations, t (40) = 4.94, p < 0.001. Therefore, participants were significantly worse at estimating directions based on a virtual world than either a familiar or new real-world environment.
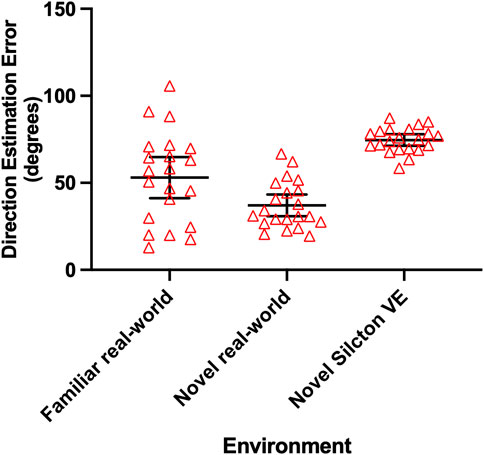
FIGURE 5. Direction estimation error in degrees for all participants across the three environments in Experiment 2. Centre lines show means and error lines indicated 95% confidence intervals. Triangles show individual scores within each condition. Horizontal scatter of the scores corresponds to the number of scores at that value (wider = more scores).
A Bayesian repeated measures ANOVA using default priors was also conducted to determine the likelihood of the null hypothesis that no differences in direction estimation performance existed across environments versus the likelihood of differences in direction estimation performance across environments. The Bayes factor, BF10 = 8.849e + 6, indicated strong evidence that direction estimation differed across environments. Follow-up posthoc pairwise comparisons showed moderate evidence for a difference between familiar real-world estimates and novel real-world estimates, BF10 = 7.56. There was strong evidence for a difference between familiar real-world estimates and Silcton estimates, BF10 = 14.60, and also for a difference between novel real-world estimates and Silcton estimates, BF10 = 2.987e + 7. Thus, the Bayesian findings largely parallel the non-parametric analysis results but suggest more evidence of a difference between the real-world environments.
3.2.3 Sketch Maps Across the Novel Real-World, Familiar Real-World, and Silcton Environments
Sketch maps were analyzed using the Gardonay Map Drawing Analyzer (Gardony et al., 2016) as in Experiment 1. Figure 6 shows the means, 95% confidence intervals, and individual participant scores for the sketch maps based on the three environments. Using Friedman’s test, we found a significant difference in sketch map accuracy for the familiar real-world locations (M = 0.78, SD = 0.23), the novel real-world locations (M = 0.76, SD = 0.14) and the Silcton virtual environment locations (M = 0.42, SD = 0.18), χ2 (2) = 21.24, p < 0.001. Bonferroni-corrected Conover posthoc tests showed no significant difference in accuracy between the familiar and novel real-world maps, t (40) = 0.78, p = 1.0, but the familiar real-world maps were more accurate than Silcton maps, t (40) = 4.32, p < 0.001, and the novel real-world maps were also significantly more accurate than the Silcton maps, t (40) = 3.55, p = 0.003. Therefore, participants were similarly accurate at sketching maps of real-world locations, whether familiar or new to them, but maps based on locations from a virtual environment were less accurate.
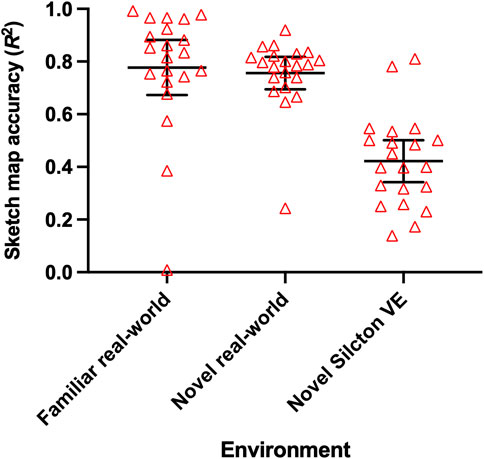
FIGURE 6. Sketch map accuracy (R2) across the three conditions in Experiment 2. Centre lines show means and the error bars show 95% confidence intervals. Triangles indicate individual scores, and the degree of horizonal scatter of the scores reflects the number of scores at that value.
To investigate map construction, a Bayesian analysis tested the likelihood of the null hypothesis (no differences across environments) versus the hypothesis that map accuracy differed across environments. The Bayes factor, BF10 = 1.951e + 7, indicated strong evidence of a difference across environments. Posthoc pairwise comparisons showed moderate evidence of no difference between familiar real-world and novel real-world maps, BF10 = 0.24, while evidence for differences between familiar real-world maps and Silcton maps was very strong, BF10 = 24,255.85, and evidence for differences between novel real-world maps and Silcton was also very strong, BF10 = 31,534.31.
3.3 Discussion
In this small study, we replicated the results of Experiment 1, as women’s performance on real-world tasks was better than on Silcton tasks. In addition, women performed as well or even slightly better when a real-world environment was novel versus familiar. This pattern suggests that it is the virtual nature of Silcton that is the driver of weaker performance, rather than an inability to learn the layout of a new environment per se.
Still, there are significant limitations to interpretation of these data. First, the sample size was small and likely did not capture the full range of women’s performance on the direction estimation and map-drawing tasks in the population. Second, it could be that our novel environment was too easy because the target locations and/or the routes were too close, or that even though the area was unfamiliar to participants, it was near familiar areas that allowed them to keep track of their orientation, potentially resulting in a ceiling effect on the novel real-world tasks. Nonetheless, it should be noted that variation in scores is still present on the novel real-world tasks. Finally, a fully crossed design that could truly tease apart familiarity of the environment and modality of the environment presentation (virtual or real life) would include a familiar area presented virtually, and future studies should include this condition where possible. Overall, these data offer a starting point for further investigation of women’s performance on spatial tasks in the lab versus in the real world.
4 General Discussion
Experiment 1 tested the accuracy of the mental cognitive maps men and women formed of familiar locations and of the novel Silcton virtual environment. Women’s cognitive maps of familiar locations were more accurate than the cognitive maps they created of Silcton, while men either showed no difference in accuracy across environments or were more accurate with the virtual world. When comparing across sexes it was observed that men’s cognitive maps of Silcton were more accurate than women’s, but men and women were equally accurate at estimating directions and creating maps of real-world locations with which they were familiar. This pattern suggests that performance with Silcton in the lab for women was not indicative of the accuracy of their everyday spatial mental representations. In Experiment 2, we conducted a small study with women to determine whether women’s weaker performance with Silcton in Experiment 1 was due to the virtual nature of Silcton or its unfamiliarity. The small sample size of this study requires that its results be interpreted with caution, but it replicated the pattern of findings in women in Experiment 1 and also suggests that women can form cognitive maps of locations in a real-world unfamiliar environment that are as accurate as their cognitive maps of familiar real-world locations.
Both studies indicate that women’s performance was weaker when creating a cognitive map of a virtual environment than when creating cognitive maps of both familiar and unfamiliar real-world environments. Experience with video games, and therefore, these types of 3D virtual worlds, seems like an obvious potential explanation, although findings from the two studies are mixed, with some evidence showing women’s video game play associated with better accuracy on Silcton tasks in Experiment 1, but no indication of a similar relationship in Experiment 2. We did not ask follow-up questions about the types of games our participants played, but the immersive, 3-dimensional nature of Silcton is most like the environments in action video games, although simplified and without the fast pace. If experience playing video games confers an advantage in creating a mental representation of Silcton, the most likely genre of game is action. Indeed, in a meta-analysis of studies investigating the effect of action video game play on cognition in both cross-sectional (habitual game play) and intervention (training on action video games versus non-action games) designs, Bediou et al. (2018) found that action video games had a positive effect on multiple areas of cognition, with some of the strongest effects on aspects of spatial cognition, like mental rotation and spatial working memory tasks. In one of those studies, Cherney (2008) found that women’s mental rotation performance improved more than men’s after training with an action video game. Importantly, studies included in Bediou et al. largely addressed spatial cognition in terms of mental rotation ability, which Hegarty and Waller (2004) showed can be dissociated from the skill of spatial perspective taking, an important skill for cognitive map accuracy. Whether training women with action video games would benefit cognitive map accuracy in virtual or real-world environments is to our knowledge untested.
There is also the possibility that women’s cognitive maps of virtual worlds are lower in accuracy due to differences in the reliance on non-visual, kinesthetic cues. One of the common criticisms of virtual testing is that it eliminates movement and other cues such as sound that are normally combined with visual cues during navigation. This could be addressed by comparing performance with head-mounted virtual reality displays with non-immersive desktop VR, although VR sickness is a concern with head-mounted displays (Chang et al., 2020) and could be confounded with performance. Another way to target the effect of a virtual modality would be to construct a virtual environment identical to a real-world environment, as in Schmelter et al. (2009), who interestingly found no significant difference in accuracy of maps drawn of a maze experienced virtually or in real life, and also no sex differences.
An important consideration for training women to form more accurate spatial mental representations of virtual worlds is the functional utility of such skills. Sex differences in our study were non-existent when participants were asked about familiar areas, so it does not appear that the everyday spatial mental representations women hold of their environment are in any particular need of improvement. The importance of being able to quickly form an accurate cognitive map of a virtual world depends on the role virtual environments will (and do) play in everyday life. A more targeted approach that focuses on both women and men whose spatial mental representations of their familiar locations is weak may be a more meaningful intervention that helps more people navigate in the real world.
If we do decide that navigating virtual environments is important enough to implement interventions to train women to form more accurate cognitive maps of such spaces, our data suggest that spatial perspective taking would be a worthwhile skill to target. Perspective-taking partially mediated the effect of sex on Silcton map creation, and on its own accounted for 20% of the variance in Silcton direction estimation. It seems reasonable that training with action video games could improve perspective taking, although currently, mental rotation is commonly used as the targeted skill (Bediou et al., 2018). In addition, the range of games that could be useful for such training is expanding, as Dale et al. (2020) have noted that traditionally “non-action” games increasingly incorporate aspects of action games into their design. Nonetheless, it remains to be seen how virtual reality will be integrated across our daily lives, and whether training women to maintain their position in the space of such environments is necessary.
Data Availability Statement
The datasets presented in these studies can be found at: https://osf.io/2ds7z/.
Ethics Statement
These studies were approved by the Brescia Research Ethics Board at Brescia Univeristy College, the Non-Medical Research Ethics Board at Western University, and Study 2 was also approved by the Huron Research Ethics Board at Huron University College. Participants provided written informed consent to participate in the studies.
Author Contributions
JS, CC, and NY conceived the experiments, CC and NY tested participants, JS conducted analyses and wrote the manuscript.
Funding
Funding for these projects was provided by a Discovery Grant from the Natural Sciences and Engineering Research Council (NSERC) to JS.
Conflict of Interest
The authors declare that the research was conducted in the absence of any commercial or financial relationships that could be construed as a potential conflict of interest.
Publisher’s Note
All claims expressed in this article are solely those of the authors and do not necessarily represent those of their affiliated organizations, or those of the publisher, the editors and the reviewers. Any product that may be evaluated in this article, or claim that may be made by its manufacturer, is not guaranteed or endorsed by the publisher.
Acknowledgments
We thank Huron University College for access to their campus to conduct the study. CC is now at the Department of Psychology, University of British Columbia, Vancouver, Canada.
References
Bediou, B., Adams, D. M., Mayer, R. E., Tipton, E., Green, C. S., and Bavelier, D. (2018). Meta-Analysis of Action Video Game Impact on Perceptual, Attentional, and Cognitive Skills. Psychol. Bull. 144 (1), 77–110. doi:10.1037/bul0000130
Bennett, A. T. (1996). Do Animals Have Cognitive Maps? J. Exp. Biol. 199, 219–224. doi:10.1242/jeb.199.1.219
Blacker, K. J., Weisberg, S. M., Newcombe, N. S., and Courtney, S. M. (2017). Keeping Track of Where We are: Spatial Working Memory in Navigation. Vis. Cogn. 25 (7-8), 691–702. doi:10.1080/13506285.2017.1322652
Boone, A. P., Gong, X., and Hegarty, M. (2018). Sex Differences in Navigation Strategy and Efficiency. Mem. Cogn. 46 (6), 909–922. doi:10.3758/s13421-018-0811-y
Castelli, L., Latini Corazzini, L., and Geminiani, G. C. (2008). Spatial Navigation in Large-Scale Virtual Environments: Gender Differences in Survey Tasks. Comput. Hum. Behav. 24 (4), 1643–1667. doi:10.1016/j.chb.2007.06.005
Chang, E., Kim, H. T., and Yoo, B. (2020). Virtual Reality Sickness: A Review of Causes and Measurements. Int. J. Hum-Comput. Int. 36 (17), 1658–1682. doi:10.1080/10447318.2020.1778351
Cherney, I. D. (2008). Mom, Let Me Play More Computer Games: They Improve My Mental Rotation Skills. Sex. Roles 59 (11-12), 776–786. doi:10.1007/s11199-008-9498-z
Dale, G., Joessel, A., Bavelier, D., and Green, C. S. (2020). A New Look at the Cognitive Neuroscience of Video Game Play. Ann. N.Y. Acad. Sci. 1464 (1), 192–203. doi:10.1111/nyas.14295
Faul, F., Erdfelder, E., Lang, A.-G., and Buchner, A. (2007). G*Power 3: A Flexible Statistical Power Analysis Program for the Social, Behavioral, and Biomedical Sciences. Behav. Res. Methods 39 (2), 175–191. doi:10.3758/bf03193146
Galati, A., Weisberg, S. M., Newcombe, N. S., and Avraamides, M. N. (2018). When Gestures Show Us the Way: Co-Thought Gestures Selectively Facilitate Navigation and Spatial Memory. Spatial Cogn. Comput. 18 (1), 1–30. doi:10.1080/13875868.2017.1332064
Gardony, A. L., Taylor, H. A., and Brunyé, T. T. (2016). Gardony Map Drawing Analyzer: Software for Quantitative Analysis of Sketch Maps. Behav. Res. 48 (1), 151–177. doi:10.3758/s13428-014-0556-x
Hegarty, M., and Waller, D. (2004). A Dissociation Between Mental Rotation and Perspective-Taking Spatial Abilities. Intelligence 32 (2), 175–191. doi:10.1016/j.intell.2003.12.001
Ishikawa, T., and Montello, D. (2006). Spatial Knowledge Acquisition from Direct Experience in the Environment: Individual Differences in the Development of Metric Knowledge and the Integration of Separately Learned Places. Cogn. Psychol. 52, 93–129. doi:10.1016/j.cogpsych.2005.08.003
JASP Team (2020). JASP (Version 0.14.1). Computer Software. Available at https://jasp-stats.org/.
Montello, D. R. (1998). “A New Framework for Understanding the Acquisition of Spatial Knowledge in Large-Scale Environments,” in Spatial and Temporal Reasoning in Geographic Information Systems. New York, NY: Oxford University Press, 143–154.
Nazareth, A., Weisberg, S. M., Margulis, K., and Newcombe, N. S. (2018). Charting the Development of Cognitive Mapping. J. Exp. Child Psychol. 170, 86–106. doi:10.1016/j.jecp.2018.01.009
Nowak, N. T., Murali, A., and Driscoll, I. (2015). Factors Related to Sex Differences in Navigating a Computerized Maze. J. Environ. Psychol. 43, 136–144. doi:10.1016/j.jenvp.2015.06.007
O'Keefe, J., and Nadel, L. (1978). The Hippocampus as a Cognitive Map. Oxford, New York: Clarendon Press.
Pagkratidou, M., Galati, A., and Avraamides, M. (2020). Do Environmental Characteristics Predict Spatial Memory About Unfamiliar Environments? Spatial Cognition Comput. 20 (1), 1–32. doi:10.1080/13875868.2019.1676248
Richardson, A. E., Powers, M. E., and Bousquet, L. G. (2011). Video Game Experience Predicts Virtual, but Not Real Navigation Performance. Comput. Hum. Behav. 27 (1), 552–560. doi:10.1016/j.chb.2010.10.003
Schinazi, V. R., Nardi, D., Newcombe, N. S., Shipley, T. F., and Epstein, R. A. (2013). Hippocampal Size Predicts Rapid Learning of a Cognitive Map in Humans. Hippocampus 23 (6), 515–528. doi:10.1002/hipo.22111
Schmelter, A., Jansen, P., and Heil, M. (2009). Empirical Evaluation of Virtual Environment Technology as an Experimental Tool in Developmental Spatial Cognition Research. Eur. J. Cognitive Psychol. 21 (5), 724–739. doi:10.1080/09541440802426465
Siegel, A. W., and White, S. H. (1975). The Development of Spatial Representations of Large-Scale Environments. Adv. Child Dev. Behav. 10, 9–55. doi:10.1016/S0065-2407(08)60007-5
Tolman, E. C. (1948). Cognitive Maps in Rats and Men. Psychol. Rev. 55, 189–208. doi:10.1037/h0061626
Warren, W. H., Rothman, D. B., Schnapp, B. H., and Ericson, J. D. (2017). Wormholes in Virtual Space: From Cognitive Maps to Cognitive Graphs. Cognition 166, 152–163. doi:10.1016/j.cognition.2017.05.020
Weisberg, S. M., and Newcombe, N. S. (2016). How Do (Some) People Make a Cognitive Map? Routes, Places, and Working Memory. J. Exp. Psychol. Learn. Mem. Cognition 42 (5), 768–785. doi:10.1037/xlm0000200
Weisberg, S. M., and Newcombe, N. S. (2018). Cognitive Maps: Some People Make Them, Some People Struggle. Curr. Dir. Psychol. Sci. 27 (4), 220–226. doi:10.1177/0963721417744521
Weisberg, S. M., Schinazi, V. R., Newcombe, N. S., Shipley, T. F., and Epstein, R. A. (2014). Variations in Cognitive Maps: Understanding Individual Differences in Navigation. J. Exp. Psychol. Learn. Mem. Cognition 40, 669–682. doi:10.1037/a0035261
Keywords: spatial cognition, cognitive map, virtual environment, navigation, sex differences
Citation: Cocquyt CM, Youngson N and Sutton JE (2022) Sex Differences and Cognitive Maps: Studies in the Lab don’t Always Reflect Cognitive Map Accuracy in Everyday Life. Front. Virtual Real. 3:896081. doi: 10.3389/frvir.2022.896081
Received: 14 March 2022; Accepted: 11 April 2022;
Published: 24 May 2022.
Edited by:
Victoria D. Chamizo, University of Barcelona, SpainReviewed by:
Petra Jansen, University of Regensburg, GermanyMartina Rahe, University of Koblenz-Landau, Germany
Copyright © 2022 Cocquyt, Youngson and Sutton. This is an open-access article distributed under the terms of the Creative Commons Attribution License (CC BY). The use, distribution or reproduction in other forums is permitted, provided the original author(s) and the copyright owner(s) are credited and that the original publication in this journal is cited, in accordance with accepted academic practice. No use, distribution or reproduction is permitted which does not comply with these terms.
*Correspondence: Jennifer E. Sutton, jennifer.sutton@uwo.ca