- 1Department of Veterans Affairs, Reno, NV, United States
- 2Palo Alto Institute for Research, Palo Alto, CA, United States
- 3VA Palo Alto Health Care System, Palo Alto, CA, United States
- 4Anesthesia and Pain Medicine, University of Washington School of Medicine, Seattle, WA, United States
- 5Department of Computer Science, King Abdulaziz University, Jeddah, Saudi Arabia
- 6Department of Mechanical Engineering HPL, University of Washington, Seattle, WA, United States
- 7Rehabilitation Services, VA Palo Alto Health Care System, Palo Alto, CA, United States
- 8Neurosurgery, Stanford University School of Medicine, Palo Alto, CA, United States
- 9Department of Psychology, University of Washington, Seattle, WA, United States
In Iraq and Afghanistan over 75% of the combat casualties suffered by U.S. troops have involved explosive devices. Improvements in body armor and advances in military medicine have significantly reduced the number of combat-related fatalities, but have greatly increased the number of U.S. active component personnel suffering painful trauma injuries. Unfortunately, so far, advances in pharmacologic analgesia pain medications have not kept pace with advances in survivability. For many active component personnel and Veterans, pain is a top health complaint from patients. The opioid epidemic has increased the urgency of developing powerful non-pharmacologic approaches for the management of pain. Immersive VR is proving to be a powerful non-opioid pain management technique for acute pain. However, the cost and usability limitations of pre-2016 VR clinical products resulted in limited treatment adoption rates for clinical use. In recent years, VR technology has become increasingly immersive, portable, and miniaturized, requiring minimal technical expertise to operate, and low-cost, factors that are likely contributing to the recent increase in the clinical use of VR analgesia. VR is greatly benefitting from a growing string of major technological breakthroughs and VR treatment improvements that will likely continue to increase the effectiveness and suitability of VR analgesia for military and VA patients. Regarding acute pain, we propose that the next revision to the current Tactical Combat Casualty Care guidelines consider including VR as an effective and hemodynamically safe approach to the current management of acute trauma pain in military personnel during medical procedures. With recent miniaturization and ruggedization, VR can potentially be used closer to the battlefield in the future. Beyond distraction, innovative VR therapy techniques designed to help reduce chronic pain are discussed. Recent breakthroughs in the mass production of inexpensive, highly immersive lightweight stand alone VR systems and augmented reality systems increase the potential for widespread dissemination of VR analgesia for acute and potentially for chronic pain. For example, the U.S. military recently purchased 22 billion dollar’s worth of Microsoft Hololens mixed reality systems (e.g., for training). Expanded research and development of VR analgesia customized for the unique needs of military and VA patients is recommended.
Introduction
The mental and physical demands of deployment readiness training, combat, and other military duties place soldiers at an increased risk of acute and chronic pain (Bader et al., 2018; Reif et al., 2018). In one study, more than 50% of active component (AC) soldiers reported at least one non-combat injury in 2017, 70% of which were physical overuse-related non-combat musculo-skeletal injuries (Molloy et al., 2020). In Iraq and Afghanistan over 75% of the combat casualties suffered by U.S. troops have involved Explosive Devices (Belmont, Schoenfeld and Goodman, 2010). These include enemy roadside bombs that explode up into the U.S. soldiers Humvees, rocket propelled grenades, and suicide bombers. Improvised Explosive Devices (IEDs) often lead to blast injuries of U.S. troops, resulting in lost limbs, traumatic brain injury, severe burns, and severe psychological problems such as PTSD and depression. Advanced body armor, more heavily armored military vehicles, and improvements in military medicine have helped reduce fatalities, but with large increases in injured survivors. Snipers targeting U.S. forces often injure a limb without hitting a major organ, leading to severe and painful but survivable bullet wounds (e.g., limb amputations).
Controlling acute procedural pain during physical and occupational therapy (PT/OT) is a widespread medical problem. Repetitive excessive acute pain, such as during daily wound care, and PT/OT contributes to sensitization and increased anticipatory pain (Gardner et al., 2017) and is a factor in delayed wound healing (Upton et al., 2012). For active component soldiers scheduled to re-deploy after recovery, the higher physical standards for active component soldiers returning to duty often involves more aggressive and painful rehabilitation therapy. Furthermore, military patients often have polytrauma, e.g., one or often several physical injuries combined with traumatic brain injury and/or symptoms of post-traumatic stress disorder, which can exacerbate pain during rehab exercises (e.g., headaches and flashbacks, in addition to severe burns).
Pharmacologic analgesia for acute pain. Post-surgical pain, and persistent post-trauma pain are risk factors for the onset of chronic pain (McGreevy et al., 2011). Persistent pain is associated with changes to nociceptive pathways in the central and peripheral nervous system that lead to pain sensitization and the transition from acute to chronic pain (Fregroso et al., 2019; Glare et al., 2019). The provision of adequate pain relief is considered a fundamental human right (Kharasch and Brunt, 2016), and the effectiveness of opioids has made them the most commonly administered drug for analgesia and supplementary sedation during the perioperative period (Casserly and Alexander, 2017). Furthermore, opioids have long been considered a critical part of the “balanced anesthesia” concept (Egan, 2019). Unfortunately, opioid side effects (e.g., constipation, urinary retention, delirium, disordered sleep (Garimella and Cellini, 2013; Rogers et al., 2013; Morina et al., 2015; Gupta et al., 2020), get worse with higher opioid doses, limiting dose increases. In addition, undermedication, and large individual differences in response to opioids and other pain medications, limit the effectiveness of pain management strategies based solely on pharmacologic analgesia. As a result, for a wide range of medical procedures, moderate to severe pain and anxiety during medical procedures is common (Correll et al., 2014; Shoar et al., 2012; Hoffman et al., 2019;2020a,b; Maani et al., 2011a,b).
Prescription opioids have been the leading cause of death by overdose, reflecting 68% of all U.S. drug overdose deaths (Wilson et al., 2020). Of note, surgical patients, even those that only require minor outpatient procedures, are at an increased risk of chronic opioid use or abuse (Sun et al., 2016). Patients innocently initially take the pills to control their pain, but some become addicted and inappropriately continue to take pills beyond when they should wean off.
The military has long been interested in nurturing the development of powerful new non-pharmacologic acute pain management techniques that do not cloud the soldiers' decision process and that support deployment readiness (Cleeland et al., 2003; Tanielian and Farmer, 2019).
Immersive virtual reality (VR), typically associated with the entertainment and video gaming industry, is increasingly also being applied as a therapeutic modality (Bailenson, 2018). For example, immersive virtual reality is proving to be an unusually effective non-pharmacologic analgesic that can be used in addition to traditional pain medications to help reduce acute pain, such as during painful medical procedures. The breakthrough findings that immersive VR can serve as a non-drug analgesic were first introduced in the late 1990s treating patients during burn wound care and physical therapy range of motion exercises (Hoffman et al., 2000a,b; Hoffman 1998).
Adjunctive VR has been shown to reduce the pain of patients with very large severe burns (covering more than 1/3rd of the patient’s body) who remain conscious during burn wound cleaning sessions in the ICU tank room. Compared to No VR, patients getting wound care reported a 40% reduction in pain intensity during virtual reality, and VR continued to be effective when used again during several separate/repeated daily wound cleaning/debridement sessions (Hoffman et al., 2019; 2020). A growing number of studies on the application of VR analgesia have replicated the VR analgesia results in burn patients and during a wide range of other painful medical procedures (Atzori et al., 2018a; Carrougher et al., 2009; Indovina et al., 2018; Garrett et al., 2014; Hoffman et al., 2011; Malloy and Ming, 2010; Morris et al., 2009; Hoffman et al., 2000a,b; Hoffman, 1998; Trost, 2021a). Furthermore, VR has been shown to support rehabilitation in clinical settings and to encourage at-home adjustive treatment adherence (Tran et al., 2021; Hoffman, Boe et al., 2020).
Despite the promising results of clinical applications of VR as a new acute pain control technique, initial adoption rates in clinical practice were slow before 2016, primarily due to the high cost and the cumbersome nature of VR systems (but also bureaucratic billing issues).
Beginning in 2016, the new “Oculus” generation of VR first began to become commercially available, and since then, VR from a number of major tech companies has undergone a dramatic punctuated equilibrium of advancements during the 5 years between 2016 and 2021, with further major advances on the near horizon. In 2021, in a very competitive new VR helmet market, the Quest2 (Quest2 Pro, and Quest 3) is an inexpensive, lightweight, and highly portable, untethered, self contained, stand alone VR helmet that delivers high-quality crisp graphics that are remarkably immersive and can be viewed using VR helmets without the need for any computers. The Oculus Quest2, Quest2 Pro and Quest3 include optical hand tracking/cyber-hand technology already integrated. The cost of this highly immersive Quest2 VR system in 2021 is currently $299 per helmet, 1/1000 the cost of a comparable helmet in 2013 of $36,500 for one helmet (e.g., expensive pre-Oculus military VR goggles used successfully by Hoffman et al. (2019)). The lightweight VIVE Focus3 and Ultra lightweight, untethered, portable, 100 degree FOV immersive VR eyeglasses called VIVE Flow are now available for purchase from VIVE.com. Other manufacturers are also reducing the size and weight of VR goggles/immersive VR eyeglasses.
Emerging evidence is pointing towards continued rapid evolution of increasingly more immersive but affordable VR systems that will be able to further amplify the potency of adjunctive VR distraction for acute pain, and VR therapy for persistent pain (Al-Ghamdi et al., 2020; Wender et al., 2009; Hoffman et al., 2006; Hoffman, 2021; Keefe et al., 2012). Acute pain laboratory studies have helped isolate factors that increase analgesic effectiveness. Goggles that stimulate peripheral vision are more effective at reducing pain than narrower field of view goggles (Hoffman et al., 2006b), and interactive worlds such as SnowWorld (see Figure 1) are more effective than passive versions of the same worlds (Wender et al., 2009). Several recent studies have shown that immersive add-ons such as interactive eye tracking (Al Ghamdi et al., 2020); and interactive avatar cyber-hands (see Figure 2) (Hoffman, 2021), significantly increase VR analgesia for acute pain. Unlike opioid-based analgesia, carefully designed adjunctive VR pain distraction treatments customized for patients (e.g., designed to minimize simulator sickness) had little or no side effects, even with more immersive VR systems, i.e., higher (more immersive, more effective) doses of VR distraction. And there is likely considerable room for further increasing the effectiveness of VR analgesia, as the technology and knowledge about VR analgesia advances.
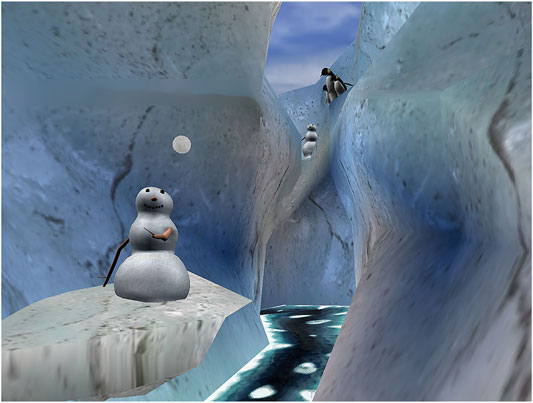
FIGURE 1. A still shot of what patients see during burn wound care and physical therapy. SnowWorld is the first virtual world custom designed for pain control. Image by Ari Hollander and Howard Rose, copyright Hunter Hoffman, www.vrpain.com.
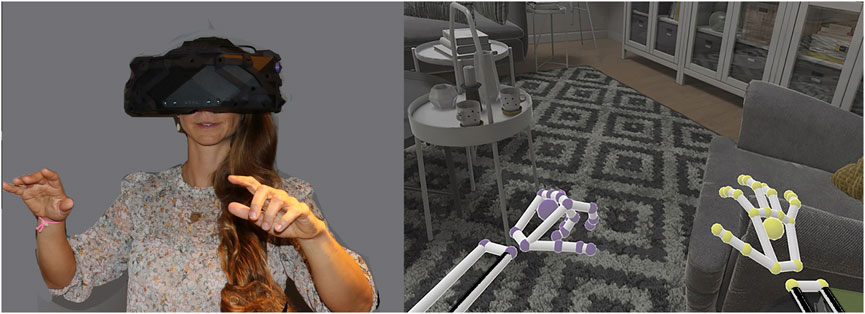
FIGURE 2. A person interacting with virtual objects in a virtual living room, using embodied cyberhands, in a recent Mayday Fund supported study (Hoffman, 2021). Copyright Hunter Hoffman, www.vrpain.com, living room demo came with the high resolution, extra wide (180 degree) field of view XTAL helmet by VRgineering.com.
Mechanism of Action
Although a number of studies have found that VR reduces acute pain, Hoffman (2021) is one of the first studies to test the mechanism of how VR works. In the first acute pain study to test whether avatars can enhance VR analgesia for acute pain (see Figure 2), Hoffman (2021) found that picking up a virtual object with their cyberhand increased participants illusion of “being there” in the computer-generated world, increased pain reduction, and reduced accuracy on an attention demanding task. This pattern of results implicates an attentional (distraction) mechanism for how VR reduces pain (Hoffman, 2021, see also; Birnie et al., 2017; Gold, Belmont and Thomas 2007).
Neuroimaging studies have explored what is happening in the brain when VR reduces acute pain. During a study by Hoffman et al. (2004), healthy civilian volunteers reported feeling considerable pain during brief thermal pain stimuli (at a safe and tolerable temperature). During functional magnetic resonance imaging (fMRI), participants’ brains showed significant pain-related brain activity during the No VR condition (Figure 3, No Virtual Reality). As shown in Figure 3, during VR, these same participants reported significant reductions in pain, and during VR, fMRI brain scans found significant reductions in metabolic activity in brain regions typically associated with the perception of acute pain - the thalamus, primary and secondary somatosensory cortex, anterior cingulate cortex, and insula (see Figure 3). In other words, VR significantly reduced pain-related brain activity.
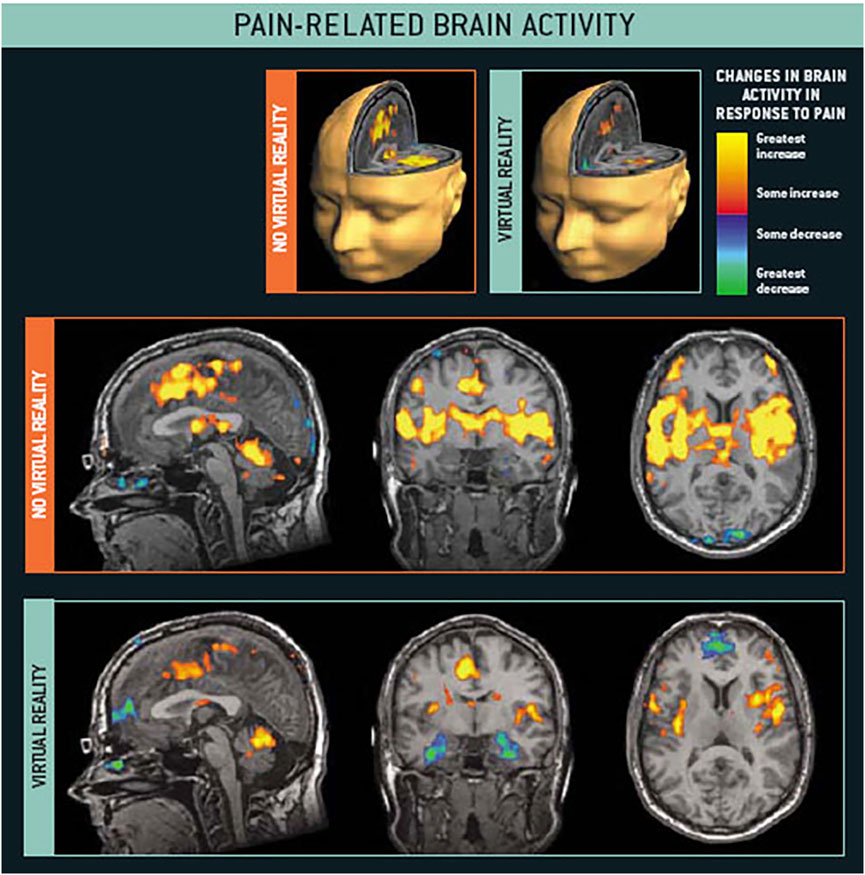
FIGURE 3. fMRI brain scans show intense pain related brain activity during no VR, and significant reductions in pain-related brain activity during VR. Hoffman et al., 2004. Images by Todd Richards and Aric Bills, copyright Hunter Hoffman, www.vrpain.com.
Furthermore, in a followup fMRI study published 3 years later, the amount of pain reduction during VR alone was comparable to the analgesia from a moderate dose of the opiate hydromorphone, and the adjunctive combination of VR and opioids was the most effective pain relief treatment (Hoffman et al., 2007).
Brain scan studies are beginning to increase our understanding of the neurobiological mechanism(s) of immersive VR and the interplay of VR with cortical activation (Li et al., 2011), an important area of future research. Although laboratory pain studies with healthy volunteers are very informative, more clinical pain brain scan research on active component and Veteran patients experiencing pain with and without VR is needed. Traditional fMRI brain scans measuring acute pain-related brain activity require the participant to be physically present in the brain scanner tube during the pain. So in the studies by Hoffman et al., 2004; 2006a; 2007, healthy volunteers received brief thermal pain stimuli during an fMRI scan. Participants received “No VR” during part of the scan and “YES VR” during fMRI during the other part of the same brain scan, treatment order randomized. Measuring clinical pain-related brain activity of severe burn patients during burn wound care was not possible using traditional fMRI. During fMRI scans, the participant’s body is positioned deep inside a huge tube, and everyone but the patient must leave the room during the scan. No metal objects are allowed in the room, so it would be challenging if not impossible to measure pain and pain-related brain activity of a severe burn patients during burn wound care in a traditional fMRI scanner.
But we WERE recently able to measure patients’ clinical pain during burn wound care, using Single Photon Emission. Computed Tomography (SPECT) imaging. With the SPECT technique, the patient receives an injection during the painful burn wound cleaning/debridement procedure (Bermo et al., 2021). The tracers in the injection go to the parts of the brain that are metabolically active at the time of injection (e.g., during painful wound care). The burn patients who volunteered to get SPECT brain scans did not need to be in the scanner at the time of injection. The patterns of pain-related brain activity during wound care were temporarily “frozen in time”. In the study by Bermo et al. (2020), the patient received their usual painful burn wound care/scrubbing in the wound care room, with their usual wound care nurse. Patients received an injection of the tracer, but no scan during wound care. After the wound care was over and their burn wounds were rebandaged, the patients were then taken downstairs to the SPECT Scanner, and the image of the brain activity at the time of the injection (during wound care), frozen in time by the injected tracer, was captured by the scanner. Although the SPECT scanning technique involves the use of short acting radioisotopes, the ability to freeze the patterns of brain activity at one time point, and image them at another time (e.g., up to 2 hours after the painful event), has a lot of potential for increasing our understanding of clinical acute and chronic pain of actual patients during painful wound care or other medical events (e.g., headaches). Another related example is epileptic seizures. Traditional fMRI brain scans require that the patients hold very still during the scan, deep inside the magnet tube. With SPECT, you can give the patient an injection during the seizure, and later go to the scanner to collect the image from the patient’s brain, after they are no longer having a seizure. This can help locate the epicenter of the epileptic activity during the recent seizure.
In addition to the studies of burn patients, and analog laboratory pain studies, the analgesic benefits of VR distraction for acute pain have also been reported in patients with blunt force trauma, venipuncture of cancer patients, dental procedures, urological endoscopic surgeries, minor orthopedic surgery procedures, and pain during physical therapy range of motion skin stretching exercises (Hoffman et al., 2020; Trost et al., 2021a; Garrett et al., 2014; Wiederhold et al., 2007). In some cases VR may also help reduce the total dose of opioids used during medical procedures (Firoozabadi et al., 2020; Kipping et al., 2012; Lew et al., 2020), with one study reporting a 39% reduction in opioid medication for patients who received virtual reality during burn wound care (McSherry et al., 2018). Furthermore, in some instances, conducting minor surgical procedures in the outpatient clinic using VR in combination with oral opioids (see Figure 4), can replace the use of much higher dose intravenous (IV) sedation/anesthesia when the same surgical procedure is performed in the operating room (Firoozabadi et al., 2020). Recent reports highlight the safety and efficacy of VR distraction for the management of acute pain during painful medical procedures (Le May et al., 2021; Hoffman et al., 2019; 2020).
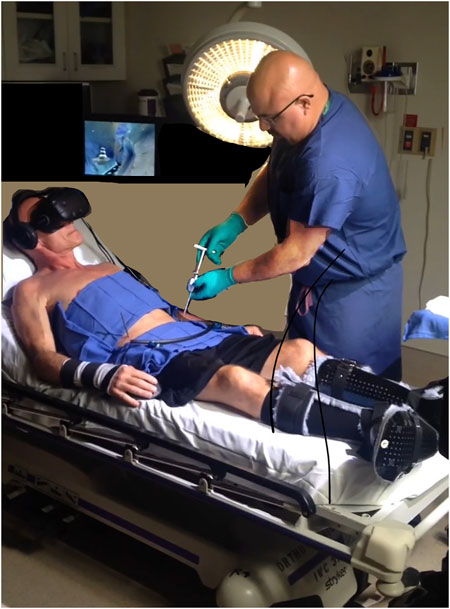
FIGURE 4. VR analgesia may encourage patients to get minor surgical procedures performed in the outpatient clinic with analgesia instead of in the Operating Room with Anesthesia/sedation (much larger medication doses in the OR). Firoozabadi et al., 2020. Photo and copyright Hunter Hoffman, www.vrpain.com.
Use of VR Distraction for Active Component Soldiers
The first use of VR analgesia for military patient populations involved two separate case studies of soldiers with combat-related burn injuries (Maani et al., 2008; Maani et al., 2011a) and a small, randomized control repeated measures study of 12 soldiers with combat-related burn injuries from improvised explosive devices (IED). The soldiers were injured during a combat deployment to Iraq and Afghanistan as part of Operation Enduring Freedom (OEF) and Operation Iraqi Freedom (OIF) (Maani et al., 2011b, see Figure 5). The findings from Maani et al., 2011b showed significant reductions in 1) Time spent thinking about pain, 2) Pain unpleasantness, 3) Worst pain, and an 8 fold increase in 4) Fun during wound care (See Figure 6).
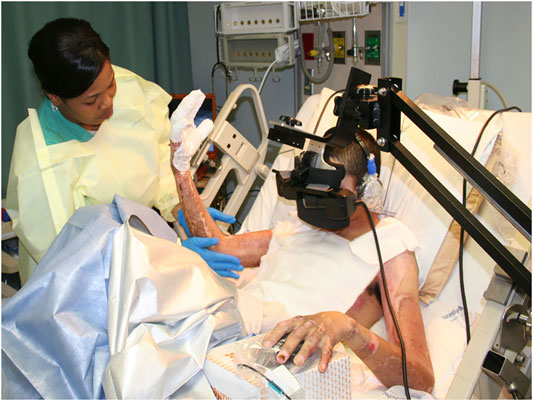
FIGURE 5. A soldier with a combat-related burn injury during burn wound debridement while in VR. A custom robot-like arm goggle holder suspended the VR goggles near the patients face, so he did not have to wear a VR helmet on his head, which had burns. Image and copyright Hunter Hoffman, www.vrpain.com.
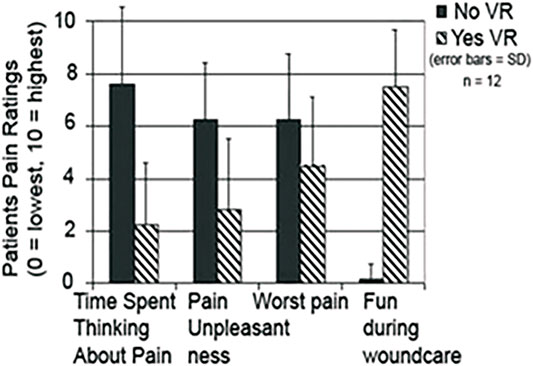
FIGURE 6. Soldiers with IED combat related burn injuries reported large reductions in acute pain during burn wound care with adjunctive VR vs. standard of care, (Maani et al., 2011b). Image and copyrights Hunter Hoffman, www.vrpain.com.
In addition to using it in the hospital, VR has potential as a field analgesic that does not impair cognition or decision-making in the way that pharmacological agents can (van Steenbergen et al., 2019).
For a number of reasons, injured soldiers are frequently undermedicated at the point of injury (e.g, on the battlefield), and during medical evacuation (Malchow and Black, 2008). For example, soldiers frequently report acute trauma pain and are often hemodynamically unstable shortly after a severe combat injury. Opioids can further increase hemodynamic instability in some patients (Gordon et al., 2004), a serious medical concern.
Nonpharmacologic VR may prove valuable as a novel analgesic technique that could be used closer to the battlefield, e.g., on the battle field, and/or during transport from the battlefield to a medical facility. At present, limited research is available on the tolerability or effectiveness of VR analgesia during medical transportation requiring flight, but occasional breaks from VR are likely to minimize nausea (Weech et al., 2019). In addition, calm, non-nauseogenic VR worlds such as SnowWorld (Hoffman et al., 2019), are specifically designed to minimize motion sickness. However, until recently the lack of commercially available, lightweight, wide field of view, high-tech VR helmets suitable for widespread military use for VR analgesia has been a barrier to widespread clinical adoption of this promising new acute pain analgesia treatment into everyday military use. Stand alone helmets (e.g., Quest2/Quest2 Pro/Quest3 or HTC VIVE Focus 3 helmet), or the new ultra lightweight and highly portable (100 degrees FOV) VIVE Flow immersive VR eyeglasses, are may prove especially useful. https://www.vive.com/us/product/vive-flow/overview/
Although very little is known about using augmented reality for pain distraction, the use of see through augmented reality goggles may prove valuable on the battlefield and/or during Medivac. Similarly 22 billion dollars worth of Microsoft Hololen goggles have reportedly been purchased and 120,000 ruggedized and customized for military versions of the Hololens are coming into circulation in the U.S. military during the next 5 years https://www.forbes.com/sites/moorinsights/2021/04/06/why-microsoft-won-the-22-billion-army-hololens-2-ar-deal/?sh=6fb6067a5d43. In addition to see-through augmented reality mode, Hololens goggles may be used in occlusive mode, to achieve a portable, moderately immersive VR distraction experience for injured patients during medivac (although there are patent filings suggesting that the Hololens 3 will have much wider field of view, the Hololens 2 currently has approximately 50 degrees field of view as of 2021, compared to approximately > 100 degree FOV for the fully immersive untethered ultra lightweight VIVE FLOW VR helmet or Quest2/Quest2 Pro/Quest3). The blinder on the VIVE FLOW and the Quest helmets can easily be removed, allowing patients to see the real ground via peripheral vision, and be more aware of their real world surroundings while in VR. Keeping partial view of the physical horizon in the real world helps reduce simulator sickness.
The Defense Advanced Research Projects Agency (DARPA) and the Department of Defense (DOD) have played and continue to play a critical role in the development of the internet, fighter pilot flight training simulators, surgical simulators (Satava et al., 2020), immersive virtual reality (IVR) and augmented reality technologies (AVR) for the training of combat and non-combat military personnel (Mowery and Simcoe, 2002). Simulators are also being used to train units in Tactical Combat Casualty Care (TCCC).
The landmark report on the occurrence of combat fatalities occurring during pre-hospital care and the U.S. Department of Defense (DoD) and the Defense Health Agency (DHA) Joint Trauma System’s Tactical Combat Casualty Care (TCCC) protocol (Butler et al., 2018) have led to “…unprecedented decreases in preventable combat death in military units that have trained all of their members in TCCC” (Butler, 2017 p. e1563). A recent review of the TCCC recommendations highlights hemorrhage and resuscitation control as one of the top 3 recommendations in the care of combat-injured patients to reduce combat fatalities further. Reducing opioids (with VR) could potentially reduce opioid induced hemodynamic instability, reducing the risk of hemorrhage. In light of the growing evidence supporting the efficacy of VR as a non-drug analgesic for acute pain, we propose that the TCCC consider adding VR distraction to the TCCC guidelines/recommendations for treating acute pain.
Chronic Pain in Veterans
Compared to civilians, in Veterans, chronic pain is often more severe and is frequently a function of polytrauma and is accompanied by complex medical and psychiatric issues (Nahin, 2017; O’Malley et al., 2020; Sandbrink et al., 2020). Chronic pain often begins with musculoskeletal and spinal cord injuries, military combat, blasts, and explosions, burns, amputations, vehicle accidents, and/or traumatic brain injury. The wide range of etiologies of chronic pain present significant challenges for treatment (Benavides et al., 2021; Zoas, 2021). Some treatment approaches may work for some chronic pain patient populations but not others. There is not a singular approach that benefits everyone. Sometimes it’s a combination of approaches that work. Virtual Reality is so intuitive that even patients who are new to technology are often surprisingly receptive to using virtual reality. And recent research shows that a positive experience in VR made veterans more open to trying other non-pharmalogic treatments that they had previously not been willing to try (Rawlins et al., 2021).
Chronic pain is a life-altering condition for service members and veterans that significantly affects patient’s quality of life, sleep quality, anxiety, stress, depression (Skadberg et al., 2020; Burrowes et al., 2021) and difficulty working (Katz, 2002). The management of chronic pain in veterans often requires a multidisciplinary approach that includes collaboration between various types of providers (pain, anesthesia, psychiatry, and rehabilitation), patient, and caregiver. Left untreated, such pain is debilitating, resulting in pathological anatomical and functional alterations in the brain, which can have long-term physical and psychological consequences (Zhang et al., 2020; Wang et al., 2021).
Due in part to their demonstrable efficacy in treating acute pain, opioids are currently also the cornerstone of pharmacologic pain control for persistent pain in the United States. Postoperatively, opioids are widely prescribed to treat chronic pain, especially in the United States (Alexander et al., 2019). More than 70% of surgical patients in America filled opioid prescriptions, compared to 11% in Sweden (Ladha et al., 2019).
Until recently, there has been an increased use of opioids to treat chronic pain. But this increase in prescriptions has been accompanied by an epidemic of opioid-related adverse events (Rosenberg, 2018, see https://www.cdc.gov/drugoverdose/deaths/index.html). To treat and manage pain, while also countering substance abuse, the Office of Veterans Affairs (VA) has focused on increasing the use of innovative and alternative biopsychosocial treatment strategies, such as the Interdisciplinary Pain Management Centers (IPMC). The IPMC provides military members with a combination of traditional face to face therapies, including coping skills through mindfulness, cognitive-behavioral therapies, tai chi, yoga, meditation, massage therapy, healthy eating and sleeping habits, and acupuncture, in addition to the more standard medication management and physical therapy (Nguyen et al., 2020). Such integrated approaches to pain management have yielded a reduction in service members diagnosed with opioid use disorder in recent years. However, more powerful non-drug analgesics are greatly needed (Crofford, 2015), especially as the medical community continues to seek new alternatives to opioids as a pain control technique for chronic pain. Immersive VR may help enhance the effectiveness of some of the abovementioned non-pharmacologic treatments, as well as brand new techniques unique to VR.
Using Virtual Reality Avatar Therapy to Reduce Chronic Pain
Some types of chronic pain (e.g., Chronic Regional Pain Syndrome, CRPS) typically develop in an arm, hand, leg or foot, or lower back after an acute trauma (e.g., after injury or after surgery, or after a stroke) and are characterized by persistent pain (e.g., burning), and in some cases, greatly exaggerated and debilitating sensitivity to pain. Although the pathogenesis of chronic pain is still poorly understood, central sensitization, by which nociceptive input can trigger increased excitability of neurons in central nociceptive pathways, is likely a relevant mechanism in some types of chronic pain (Bruehl and Warner, 2010; Woolf, 2011). In addition to severe pain, patients with chronic pain also suffer from significant impairments in function and impaired psychological well being. There is currently no known cure for chronic pain. Treatment remains exceptionally difficult (Harden et al., 2013). Many commonly used interventions are invasive (e.g., nerve blocks, spinal cord stimulators, opioid analgesics), have significant side effects, or have not rigorously been shown to be efficacious (Kingery, 1997; Perez, Kwakkel, Zuurmond, De Lange, and management, 2001). Chronic pain is one of the leading causes of hospital visits, and chronic pain causes an enormous economic burden in the United States in annual hospital costs and lost income/reduced productivity. Thus, a new low cost, non-drug analgesia technique that can help patients achieve long term reductions in persistent chronic pain levels (during their everyday lives when patients are not in VR) would be a highly valuable, cost-saving and humanitarian medical advance with important clinical implications.
The Challenges of Treating Chronic Pain
Because moving or touching the hand or limb affected by chronic pain can be extremely painful, patients often avoid using their hypersensitive injured limb or body part (e.g., lower back). Chronic pain patients often develop a psychological avoidance, phobia/fear of movement, and disuse can lead to muscle atrophy, loss of strength, and reduced functionality (permanently reduced range of motion). As a result of disuse, skin, bones and muscles may begin to deteriorate, and the patient may experience tightening of their hands and feet, and in some cases the patient’s fingers or toes may contract into a fixed position.
As an example of maladaptive neuroplasticity, if disused long enough, the part of the brain cortex that controls the body part affected by chronic pain (e.g., the patient’s hand) can shrink from disuse, and the patient can develop a distorted homunculus. For example, amputees who develop phantom limb pain sometimes show distortions in their cortical maps. And indeed the theories behind why mirror therapy (and VR avatar therapy) may help some chronic pain patients (e.g., CRPS) is based in part on encouraging results from phantom limb patients, which is also a challenging chronic pain disease. For some phantom limb patients, mirror therapy helped make their phantom limb and phantom limb pain disappear and helped normalize their distorted homunculus (Ramachandran and Ramachandran, 1996; Chan et al., 2007).
As summarized by Moseley (2004, p 192), “Shrinkage of the cortical representation of the affected limb in the primary somatosensory cortex (Juottonen et al., 2002) and disrupted body schema (Schwoebel et al., 2001) have both been observed in CRPS1 patients, and also in amputees with phantom limb pain and post-stroke patients” (Coslett, 1998; Flor et al., 1995). According to Moseley (2004, p 192–193), “in both amputees with phantom limb pain and in stroke patients, a primary goal is to activate cortical areas that subserve the effected limb, which leads to symptomatic and functional improvements (Liepert et al., 2000; Flor et al., 2001) and which in turn correlate with cortical reorganization (Kopp et al., 1999; Flor et al., 2001).”
The Innovative Use of Mirror Therapy for Treating Chronic Pain
Mirror therapy uses a mirror to help trick the brain of chronic pain patients. The patient positions a mirror such that when the patient moves their healthy limb, their brain perceives the mirror reflection as the limb that is affected by chronic pain (except healthy looking and moving without pain instead of the stump that remains hidden from the patients view during mirror therapy). Research shows that for patients with phantom limb pain and some other chronic pain syndromes (e.g., Complex Regional Pain Syndrome, or CRPS), this type of therapy (mirror therapy) helps improve function and reduce pain (Smart et al., 2016; Méndez-Rebolledo et al., 2017; Taylor et al., 2021).
Mirror-therapy is inexpensive, simple and widely available, but compliance with homeworks is a major factor for improvement (Kuys et al., 2012). Unfortunately, patients often find mirror therapy difficult to self-administer correctly, repetitive (Moseley, 2008), and some may find it boring, which may reduce compliance with low tech mirror therapy homework assignments. We predict that mirror therapy is also less attention grabbing than interactive immersive avatar VR therapy, so the patients may experience more flair up pain while doing physical/occupation therapy via home based mirror therapy. Feeling increased pain during mirror therapy movement exercises could discourage the patient’s cooperation with their healthcare providers (e.g., reducing compliance with homeworks), reducing long term therapeutic outcome.
Researchers have begun exploring ways to use immersive virtual reality embodiment (aka avatar VR) to treat chronic pain. Although this line of research is in the early stages, preliminary results are encouraging (Matamala-Gomez et al., 2019). Several studies to date have shown significant short term reductions in chronic pain during avatar VR. In many of these early studies, the avatars were static. For example, the Chronic Regional Pain Syndrome (CRPS) patient laid their real arm on a real table such that when they put on the VR helmet, the virtual arm they saw laying on a virtual table in the virtual world was co-located with their real arm. Researchers then manipulated the size, shape, color and transparency of the virtual body part, and measured how those manipulations affected the patients chronic pain ratings during the VR sessions (Matamala-Gomez et al., 2019; Matamala-Gomez et al., 2019; Matamala-Gomez et al., 2020). In another study using static avatars to treat chronic pain, the stationary avatar pulsed with each of the patient’s heartbeats. Seeing their avatar light up slightly each time the patient’s heart beat helped reduce chronic pain in CRPS patients, while the patient was in VR (Solcà et al., 2018). According to Solcà et al. (2018) multisensory integration (e.g., a pulsing avatar) is key to giving patients a compelling illusion of ownership of the avatar.
Giving patients with paralyzed legs the illusion of control over virtual limbs (i.e., virtual walking), can help reduce neuropathic pain in patients with spinal cord injuries (Austin and Siddal, 2019; Trost, et al., 2021b). As reviewed by Trost, et al. (2021a), most acute pain studies are using VR as a distraction, whereas for most studies exploring the use of VR for treating chronic pain, distraction is not the target of the treatment. Instead, when used to treat chronic pain, “virtual reality is used as an adjunct to deliver psychological coping skills (e.g., cognitive behavioral therapy), improve mood, promote movement such as …increased lumbar flexion among back pain sufferers with fear of movement.” (Trost, 2021a, p. 327).
Although the preliminary results using VR analgesia to treat chronic pain are encouraging, as noted by Trost, et al., 2021a, p 327, regarding VR chronic pain studies, “…most of the studies to date do not meet criteria for “high-quality evidence” or RCT status”.
Dynamic avatars controlled by optical hand tracking technology have only recently become widely commercially available, and affordable, a major technological development by the new multi-billion dollar VR helmet companies. We predict that dynamic avatars (e.g., cyberhands) can be designed to be unusually potent for reducing chronic pain and improving long term outcome instead of just short term improvements. While wearing a VR helmet, the patient sees two computer generated virtual hands (a right cyberhand and a left cyberhand) in the virtual world. Patients cannot see their real hands, because the helmet blocks their view of the real world. Miniature cameras embedded in the VR helmet track the patients real hand locations and movements, and the virtual hands (cyberhands) seen by the patient in VR, mimic their real hand movements in real time. Patients can use their real hands and fingers to reach out their cyberhand in VR and interact with virtual objects in the computer-generated world via their avatar cyberhands (see Figure 2).
Compared to static avatars, this illusion of ownership of the virtual body known as embodiment, (e.g., these cyberhands are my hands) is much more compelling and convincing with dynamic avatars (e.g., cyberhands).
Hoffman (2021) found that interacting with virtual objects via embodied cyberhands and movable cyberfingers made VR significantly more effective at reducing acute pain while the person was in VR (Hoffman, 2021).
VR distraction can also temporarily reduce chronic pain (Wiederhold et al., 2014; Rawlins et al., 2021) and we predict may temporarily reduce chronic pain during PT/OT sessions. And that may help increase patient’s compliance with the therapists. While the direct analgesic benefits of VR distraction may be fairly short lived, what patients do while in VR may have lasting long term benefits, e.g., therapeutic movements that will help activate their atrophied homunculus, and/or learning a mindfulness coping skill while in VR (e.g., controlled breathing), that they can use to reduce their anxiety in the real world when they are not wearing a VR helmet. In addition, Cognitive Behavioral Therapy delivered via VR treatments can help correct misconceptions the patients have (e.g., teaching them the counterintuitive notion that movement may increase their pain in the short term and the day after, but may help reduce their chronic pain in the long term, Keefe et al., 2012), Based on our recent laboratory study of the effect of cyberhands on VR analgesia for acute pain (Hoffman, 2021, see Figure 2), we predict that avatar VR treatment for chronic pain patients will be unusually attention grabbing and will non-pharmacologically reduce chronic procedural pain during physical/occupational therapy and will make movements of the affected limb less painful during physical/occupational therapy. In addition, during VR, patients will be able to use their hands/cyberhands to interact with virtual objects and perform simple tasks requiring manual dexterity. These interactions with objects in the virtual world will help motivate patients to physically move their affected limb. We predict that dynamic VR avatar therapy will improve both short term and long term pain scores and functional status (i.e., long term reductions in chronic pain, improved limb range of motion in the real world), and Avatar VR therapy will have long term psychological benefits (reduced depression, reduced negative emotions, reduced centralized pain sensitivity, reduced fear of movement, and/or improved quality of life).
Dynamic Immersive Avatar VR (cyberhands) is designed to stimulate the parts of the patients’ brain associated with the chronic pain affected limb. As mentioned earlier, this is a logic similar to the logic of treating chronic pain in amputees who have also stopped using their affected (e.g, amputated) hand, which is in pain (their phantom limb). In other words, the rationale for why VR avatar therapy might work is based on the same logic as using mirror therapy for phantom limb patients.
We predict that dynamic avatar VR will promote exercise in the affected limb to more intensely engage neural pathways in a way designed to stimulate the part of the patient’s brain that controls the affected limb (the motor cortex, and also the contralateral primary somatosensory cortex).
VR Mindfulness
Chronic pain is associated with greater levels of stress, and depression, which results in significant reductions in patients’ quality of life and leads to substantial direct and indirect healthcare costs (Skadberg, Moore, and Elledge, 2020). Stressful events, depression and other negative psychological influences can exacerbate chronic pain. A substantial body of research supports the efficacy of traditional mindfulness-based stress reduction (MBSR) for the reduction of stress and anxiety that can help reduce chronic pain (Kabat-Zinn 1982, 1990; Kabat-Zinn et al., 1985). Traditional mindfulness practice is known to be associated with an increase in positive emotions, a reduction in negative emotions, improved emotion regulation, and reduced stress. However, the accompanying training (typically in person, in groups, at scheduled times) and a commitment to a regular practice that MBSR approaches require is a frequent adoption barrier (Flett et al., 2019).
During mindfulness VR, using Mindfulness RiverWorld (see Figure 7), patients listen to instructions that teach mindfulness skills by observing sights and sounds. In pilot studies, VR mindfulness skills training reduced negative emotions and increased positive emotions in patients with borderline personality disorder (Nararro-Haro et al., 2016), paralysis following spinal cord injury (Flores et al., 2018), and severe burns (Gomez et al., 2017). In a randomized controlled study, patients with Generalized Anxiety Disorder who received traditional mindfulness + VR Mindfulness, had significantly higher completion rates (fewer dropouts) than patients who received traditional mindfulness only (Navarro-Haro et al., 2019).
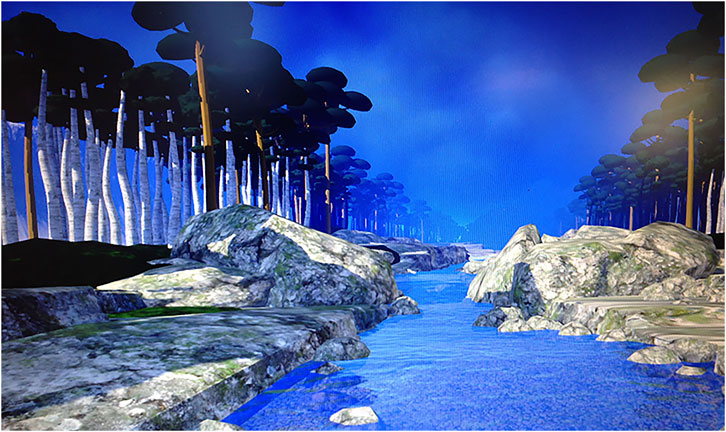
FIGURE 7. In Mindfulness RiverWorld, patients while in a natural and calming virtual world, patients listen to instructions that teach mindfulness skills. Image by bigenvironments.com, copyright Hunter Hoffman, www.vrpain.com.
Additional Treatments
In addition to its application for acute, brief, and chronic pain management, VR is increasingly being used for the treatment of the frequently accompanying psychiatric challenges that are commonplace post-deployment such as anxiety and depression. And reducing PTSD remains a core mandate of the DoD and the VA (Rizzo and Shilling, 2017). Therapeutic VR is showing promise for the treatment of these common co-occurring psychiatric symptoms.
VR graded exposure therapy (VR-GET) has shown particular promise for PTSD (Difede and Hoffman, 2002; Difede et al., 2014; McLay et al., 2014; Rothbaum et al., 2014; Rizzo and Shilling, 2017; Norr et al., 2018; Gramlich et al., 2021) a condition that affects between 9 and 30% of Veterans (Neria, 2021).
Trypanophobia or fear of needles is a common phobia that can cause unnecessary distress, anxiety, and rumination, may in some cases require physical restraint during the procedure (e.g, Atzori et al., 2018b). Avoidance of a simple venipuncture procedure can prevent potentially lifesaving medical care, particularly vaccinations. VR distraction has shown efficacy for the temporary management of pain, fear and distress associated with venipuncture using VR distraction (e.g, SnowWorld) in a range of populations - children, adults with autism spectrum disorders, and cancer patients (Atzori, 2018b; Gold and Mahrer, 2018; Chan et al., 2019; Love and Love., 2021). Alternatively, instead of using VR for distraction to temporarily get the patient through the venipuncture, permanently reducing the persons fear of needles can often be achieved in a few cognitive behavioral therapy sessions involving exposure therapy (e.g., VR exposure therapy in “Virtual Needle World”), guided by a clinical therapist.
Future Directions
In the future, patients may find home-based physical therapy homework exercises more interesting and fun using networked avatar VR enhanced PT/OT homework sessions. Information collected from the patient by the VR system can be used to keep track of how often patients are doing their physical therapy or mindfulness exercises, and to measure and give them feedback about their improvements over time. Networked multiplayer VR (e.g., Wendrich et al., 2016) allows therapists and patients to meet in a common shared virtual environment, and “buddy systems” where pairs of patients can support each other during VR sessions during their treatment. VR is becoming an established research and treatment option, and the rapid advances in VR technology, continued reduction in hardware and software costs, and the application of VR to a growing number of medical conditions, suggests that if results warrant, its use for chronic pain reduction will likely increase. The familiarity of Veterans with VR makes it a readily adoptable modality in the treatment of the complex physical and psychiatric disorders typical of this population (Kramer et al., 2010). For example, there is recent evidence that Veterans who briefly try VR distraction are impressed by the temporary reduction in pain they experience while in VR (i.e, VR distraction), and become more receptive/open minded to trying other non-pharmacologic analgesia treatments that might give them short term breaks from their pain while in VR (Rawlins et al., 2021) as well as long term solutions to their chronic pain discussed above (VR Mindfulness skills training, VR CBT, and VR Neuroscience pain education).
VR is readily incorporated into telehealth and telepsychiatry initiatives to offer access to healthcare to remote locations (e.g., patients living in rural communities) and as a cost-saving solution (Miner et al., 2016; Pierce et al., 2020; Sampaio et al., 2021a,b). Emerging reports point to the readiness of clinicians to add VR to their highly effective telehealth and telepsychology practice, particularly during the global pandemic (Sampaio et al., 2021a,b). The new generation of VR systems supports avatars (e.g., cyberhands and soon wireless full body tracking, full avatar bodies, face tracking and eye tracking), and the addition of “embodied” presence is expected to enhance efficacy, as it enhances the patient’s experience, increases patient’s sense of “physical control” of their body (albeit a virtual body). Avatar VR may be useful for some chronic pain patients (Matamala-Gomez, Diaz et al., 2019) and also for a wide range of acutely painful medical procedures (Hoffman, 2021).
Conclusion
We propose a revision to the current TCCC guidelines (see Montgomery et al., 2021) to include the addition of VR as an effective and hemodynamically safe approach to the current management of acute trauma pain in military personnel during medical procedures. Future research is needed on the feasibility of using VR acute pain distraction at point of injury, and during transport to emergency care (e.g., using lightweight VR goggles, or ultralightweight immersive eyeglasses, or Hololens mixed reality glasses). VR can also be used to support recovery from chronic pain by augmenting the efficacy of already established psychotherapeutic approaches such as CBT, exposure therapy, Dialectical Behavioral Therapy (Linehan, 1993), Mindfulness Based Stress Reduction (Kabat-Zinn, 1985) and other mindfulness based therapies. Furthermore, VR has been shown to support rehabilitation in clinical settings and to encourage at-home treatment adherence (Tran et al., 2021). Moreover, VR is a useful platform to provide interactive pain neuroscience education (PNE) about perioperative pain and expected recovery (Louw et al., 2020). In addition to psychosocial information about chronic pain, VR can enhance physical rehabilitation by providing brief analgesia during rehabilitation exercises, and the therapeutic physical movements may in turn help reduce chronic pain (Garcia et al., 2015; Keefe et al., 2012; Trost, 2021ab). Expanded research and development of VR analgesia customized for the unique needs of military and VA patients is recommended (Chan et al., 2018, Matamala-Gomez et al., 2019, Moseley, 2004, Perez et al., 2001, Siedlecka et al., 2014).
Author Contributions
All authors listed have made a substantial, direct, and intellectual contribution to the work and approved it for publication.
Funding
This project was funded in part by the Mayday Fund to HH. The project was funded by the Deanship of Scientific Research (DSR), King Abdulaziz University, Jeddah, Saudi Arabia under grant NO. (DF-582-611-1441) to WA.
Conflict of Interest
HH is Scientific Advisor to BehaVR.com.
The remaining authors declare that the research was conducted in the absence of any commercial or financial relationships that could be construed as a potential conflict of interest.
Publisher’s Note
All claims expressed in this article are solely those of the authors and do not necessarily represent those of their affiliated organizations, or those of the publisher, the editors and the reviewers. Any product that may be evaluated in this article, or claim that may be made by its manufacturer, is not guaranteed or endorsed by the publisher.
Acknowledgments
The authors, therefore, gratefully acknowledge DSR technical and financial support. We also thank the Headache Center of Excellence (HCOE) at VA Palo Alto Healthcare Systems for their support and thanks to Scott F. Shepherd 3rd, MD Emergency Colonel, Critical Care Air Transport Physician USAF, for helpful input.
References
Al-Ghamdi, N. A., Meyer, W. J., Atzori, B., Alhalabi, W., Seibel, C. C., Ullman, D., et al. (2020). Virtual Reality Analgesia with Interactive Eye Tracking during Brief Thermal Pain Stimuli: A Randomized Controlled Trial (Crossover Design). Front. Hum. Neurosci. 13, 467. PMID: 32038200; PMCID: PMC6990370. doi:10.3389/fnhum.2019.00467
Alexander, J. C., Patel, B., and Joshi, G. P. (2019). Perioperative Use of Opioids: Current Controversies and Concerns. Best Pract. Res. Clin. Anaesthesiology 33 (3), 341–351. Epub 2019 Jul 17. PMID: 31785719. doi:10.1016/j.bpa.2019.07.009
Atzori, B., Hoffman, H. G., Vagnoli, L., Patterson, D. R., Alhalabi, W., Messeri, A., et al. (2018b). Virtual Reality Analgesia during Venipuncture in Pediatric Patients with Onco-Hematological Diseases. Front. Psychol. 9, 2508. doi:10.3389/fpsyg.2018.02508
Atzori, B., Lauro Grotto, R., Giugni, A., Calabrò, M., Alhalabi, W., and Hoffman, H. G. (2018a). Virtual Reality Analgesia for Pediatric Dental Patients. Front. Psychol. 9, 2265. PMID: 30532720; PMCID: PMC6265341. doi:10.3389/fpsyg.2018.02265
Austin, P. D., and Siddall, P. J. (2019). Virtual Reality for the Treatment of Neuropathic Pain in People with Spinal Cord Injuries: a Scoping Review. J. Spinal Cord Med., 44, 1–11. doi:10.1080/10790268.2019.1575554
Bader, C. E., Giordano, N. A., McDonald, C. C., Meghani, S. H., and Polomano, R. C. (2018). Musculoskeletal Pain and Headache in the Active Duty Military Population: An Integrative Review. Worldviews Evidence-Based Nurs. 15 (4), 264–271. Epub 2018 Jun 29. PMID: 29957866. doi:10.1111/wvn.12301
Bailenson, J. N. (2018). Experience on Demand: What Virtual Reality Is, How it Works, and what it Can Do. New York, NY: W. W. Norton.
Belmont, P. J., Schoenfeld, A. J., and Goodman, G. (2010). Epidemiology of Combat Wounds in Operation Iraqi Freedom and Operation Enduring Freedom: Orthopaedic burden of Disease. J. Surg. Orthop. Adv. 19 (1), 2–7. PMID: 20370999.
Bermo, M., Saqr, M., Hoffman, H., Patterson, D., Sharar, S., Minoshima, S., et al. (2021). Utility of SPECT Functional Neuroimaging of Pain. Front. Psychiatry 12, 705242. doi:10.3389/fpsyt.2021.705242
Bermo, M. S., Patterson, D., Sharar, S. R., Hoffman, H., and Lewis, D. H. (2020). Virtual Reality to Relieve Pain in Burn Patients Undergoing Imaging and Treatment. Top. Magn. Reson. Imaging 29 (4), 203–208. PMID: 32511197. doi:10.1097/RMR.0000000000000248
Birnie, K. A., Chambers, C. T., and Spellman, C. M. (2017). Mechanisms of Distraction in Acute Pain Perception and Modulation. Pain 158 (6), 1012–1013. PMID: 28514252. doi:10.1097/j.pain.0000000000000913
Bruehl, S., and Warner, D. S. (2010). An Update on the Pathophysiology of Complex Regional Pain Syndrome. PAIN, 113 (3), 713–725. doi:10.1097/aln.0b013e3181e3db38
Burrowes, S. A. B., Goloubeva, O., Stafford, K., McArdle, P. F., Goyal, M., Peterlin, B. L., et al. (2021). Enhanced Mindfulness-Based Stress Reduction in Episodic Migraine-Effects on Sleep Quality, Anxiety, Stress, and Depression: a Secondary Analysis of a Randomized Clinical Trial. Pain, 1–9. doi:10.1097/j.pain.0000000000002372
Butler, F. K. (2017). Two Decades of Saving Lives on the Battlefield: Tactical Combat Casualty Care Turns 20. Mil. Med. 182 (3), e1563–e1568. doi:10.7205/MILMED-D-16-00214
Butler, Frank. K., Holcomb, John. B., Shackelford, Stacy., Barbabella, Sean., Bailey, Jeffrey. A., Baker, Jay. B., et al. (2018). Advanced Resuscitative Care in Tactical Combat Casualty Care: TCCC Guidelines Change 18-01. J. Spec. Oper. Med. 18 (4), 37–55. https://www.jsomonline.org/SharedScience/2018437Butler.pdf.
Carrougher, G. J., Hoffman, H. G., Nakamura, D., Lezotte, D., Soltani, M., Leahy, L., et al. (2009). The Effect of Virtual Reality on Pain and Range of Motion in Adults with Burn Injuries. J. Burn Care Res. 30 (5), 785–791. doi:10.1097/BCR.0b013e3181b485d3
Casserly, E., and Alexander, J. C. (2017). Perioperative Uses of Intravenous Opioids in Adults. Waltham, MA: UpToDate Inc. Available from: http://www.uptodate.com (Accessed August 21, 2019).
Chan, B. L., Witt, R., Charrow, A. P., Magee, A., Howard, R., Pasquina, P. F., et al. (2007). Mirror Therapy for Phantom Limb Pain. N. Engl. J. Med. 357 (21), 2206–2207. PMID: 18032777. doi:10.1056/NEJMc071927
Chan, E., Foster, S., Sambell, R., and Leong, P. (2018). Clinical Efficacy of Virtual Reality for Acute Procedural Pain Management: A Systematic Review and Meta-Analysis. PLoS One 13 (7), e0200987. PMID: 30052655; PMCID: PMC6063420. doi:10.1371/journal.pone.0200987
Chan, E., Hovenden, M., Ramage, E., Ling, N., Pham, J. H., Rahim, A., et al. (2019). Virtual Reality for Pediatric Needle Procedural Pain: Two Randomized Clinical Trials. J. Pediatr. 209, 160–167. doi:10.1016/j.jpeds.2019.02.034
Cleeland, C. S., Reyes-Gibby, C. C., Schall, M., Nolan, K., Paice, J., Rosenberg, J. M., et al. (2003). Rapid Improvement in Pain Management: the Veterans Health Administration and the Institute for Healthcare Improvement Collaborative. The Clin. J. Pain 19 (5), 298–305. doi:10.1097/00002508-200309000-00003
Correll, D. J., Vlassakov, K. V., and Kissin, I. (2014). No Evidence of Real Progress in Treatment of Acute Pain, 1993-2012: Scientometric Analysis. J. Pain Res. 7, 199–210. doi:10.2147/JPR.S60842
Crofford, L. J. (2015). Chronic Pain: Where the Body Meets the Brain. Trans. Am. Clin. Climatol. Assoc. 126, 167–183. PMID: 26330672; PMCID: PMC4530716.
Difede, J., Cukor, J., Wyka, K., Olden, M., Hoffman, H., Lee, F. S., et al. (2014). D-cycloserine Augmentation of Exposure Therapy for Post-Traumatic Stress Disorder: a Pilot Randomized Clinical Trial. Neuropsychopharmacol. 39, 1052–1058. doi:10.1038/npp.2013.317
Difede, J., and Hoffman, H. G. (2002). Virtual Reality Exposure Therapy for World Trade Center Post-Traumatic Stress Disorder: a Case Report. CyberPsychology Behav. 5 (6), 529–535. PMID: 12556115. doi:10.1089/109493102321018169
Egan, T. D. (2019). Are Opioids Indispensable for General Anaesthesia. Br. J. Anaesth. 122 (6), e127–e135. Epub 2019 Mar 28. PMID: 31104756. doi:10.1016/j.bja.2019.02.018
Firoozabadi, R., Elhaddad, M., Drever, S., Soltani, M., Githens, M., Kleweno, C. P., et al. (2020). Case Report: Virtual Reality Analgesia in an Opioid Sparing Orthopedic Outpatient Clinic Setting: A Case Study. Front. Virtual Real. 1, 553492. Epub 2020 Dec 14. PMID: 33585832; PMCID: PMC7877803. doi:10.3389/frvir.2020.55349210.3389/frvir.2020.553492
Flett, J. A. M., Hayne, H., Riordan, B. C., Thompson, L. M., and Conner, T. S. (2019). Mobile Mindfulness Meditation: a Randomised Controlled Trial of the Effect of Two Popular Apps on Mental Health. Mindfulness 10 (5), 863–876. doi:10.1007/s12671-018-1050-9
Flor, H., Denke, C., Schaefer, M., and Grüsser, S. (2001). Effect of Sensory Discrimination Training on Cortical Reorganisation and Phantom Limb Pain. The Lancet 357, 1763–1764. doi:10.1016/s0140-6736(00)04890-x
Flor, H., Elbert, T., Knecht, S., Wienbruch, C., Pantev, C., Birbaumers, N., et al. (1995). Phantom-limb Pain as a Perceptual Correlate of Cortical Reorganization Following Arm Amputation. Nature 375, 482–484. doi:10.1038/375482a0
Flores, A., Linehan, M. M., Todd, S. R., and Hoffman, H. G. (2018). The Use of Virtual Reality to Facilitate Mindfulness Skills Training in Dialectical Behavioral Therapy for Spinal Cord Injury: A Case Study. Front. Psychol. 9, 531. PMID: 29740365; PMCID: PMC5924802. doi:10.3389/fpsyg.2018.00531
Fregoso, G., Wang, A., Tseng, K., and Wang, J. (2019). Transition from Acute to Chronic Pain: Evaluating Risk for Chronic Postsurgical Pain. Pain Physician 22 (5), 479–488. PMID: 31561647.
Garcia-Palacios, A., Herrero, R., Vizcaíno, Y., Belmonte, M. A., Castilla, D., Molinari, G., et al. (2015). Integrating Virtual Reality with Activity Management for the Treatment of Fibromyalgia. Clin. J. Pain 31 (6), 564–572. PMID: 25551475. doi:10.1097/AJP.0000000000000196
Gardner, S. E., Abbott, L. I., Fiala, C. A., and Rakel, B. A. (2017). Factors Associated with High Pain Intensity during Wound Care Procedures: A Model. Wound Rep. Reg. 25 (4), 558–563. Epub 2017 Aug 14. PMID: 28805960; PMCID: PMC5831166. doi:10.1111/wrr.12553
Garimella, V., and Cellini, C. (2013). Postoperative Pain Control. Clin. Colon Rectal Surg. 26 (3), 191–196. PMID: 24436674; PMCID: PMC3747287. doi:10.1055/s-0033-1351138
Garrett, B., Taverner, T., Masinde, W., Gromala, D., Shaw, C., and Negraeff, M. (2014). A Rapid Evidence Assessment of Immersive Virtual Reality as an Adjunct Therapy in Acute Pain Management in Clinical Practice. Clin. J. Pain 30 (12), 1089–1098. PMID: 24535053. doi:10.1097/AJP.0000000000000064
Glare, P., Aubrey, K. R., and Myles, P. S. (2019). Transition from Acute to Chronic Pain after Surgery. The Lancet 393 (10180), 1537–1546. PMID: 30983589. doi:10.1016/S0140-6736(19)30352-6
Gold, J. I., Belmont, K. A., and Thomas, D. A. (2007). The Neurobiology of Virtual Reality Pain Attenuation. CyberPsychology Behav. 10 (4), 536–544. PMID: 17711362. doi:10.1089/cpb.2007.9993
Gold, J. I., and Mahrer, N. E. (2018). Is Virtual Reality Ready for Prime Time in the Medical Space? A Randomized Control Trial of Pediatric Virtual Reality for Acute Procedural Pain Management. J. Pediatr. Psychol. 43 (3), 266–275. PMID: 29053848. doi:10.1093/jpepsy/jsx129
Gomez, J., Hoffman, H. G., Bistricky, S. L., Gonzalez, M., Rosenberg, L., Sampaio, M., et al. (2017). The Use of Virtual Reality Facilitates Dialectical Behavior Therapy "Observing Sounds and Visuals" Mindfulness Skills Training Exercises for a Latino Patient with Severe Burns: A Case Study. Front. Psychol. 8, 1611. PMID: 28993747; PMCID: PMC5622494. doi:10.3389/fpsyg.2017.01611
Gordon, E. M., Myers, C., and Blumer, J. (2004). In Vitro evaluation of the Potential Role of Sulfite Radical in Morphine-Associated Histamine Release. BMC Pharmacol. 4, 21. PMID: 15469613; PMCID: PMC526189. doi:10.1186/1471-2210-4-21
Gramlich, M. A., Smolenski, D. J., Norr, A. M., Rothbaum, B. O., Rizzo, A. A., Andrasik, F., et al. (2021). Psychophysiology during Exposure to Trauma Memories: Comparative Effects of Virtual Reality and Imaginal Exposure for Posttraumatic Stress Disorder. Depress. Anxiety 38 (6), 626–638. Epub 2021 Mar 5. PMID: 33666322. doi:10.1002/da.23141
Gupta, S., Mohta, A., and Gottumukkala, V. (2020). Opioid-free Anesthesia-Caution for a One-Size-Fits-All Approach. Perioper. Med. (Lond) 9, 1–4. doi:10.1186/s13741-020-00147-3
Harden, R. N., Oaklander, A. L., Burton, A. W., Perez, R. S. G. M., Richardson, K., Swan, M., et al. (2013). Complex Regional Pain Syndrome: Practical Diagnostic and Treatment Guidelines, 4th Edition. Pain Med. 14 (2), 180–229. doi:10.1111/pme.12033
Hoffman, H. G., Doctor, J. N., Patterson, D. R., Carrougher, G. J., and Furness, T. A. (2000a). Virtual Reality as an Adjunctive Pain Control during Burn Wound Care in Adolescent Patients. Pain 85 (1-2), 305–309. PMID: 10692634. doi:10.1016/s0304-3959(99)00275-4
Hoffman, H. G., Boe, D. A., Rombokas, E., Khadra, C., LeMay, S., Meyer, W. J., et al. (2020a). Virtual Reality Hand Therapy: A New Tool for Nonopioid Analgesia for Acute Procedural Pain, Hand Rehabilitation, and VR Embodiment Therapy for Phantom Limb Pain. J. Hand Ther. 33 (2), 254–262. Epub 2020 May 30. PMID: 32482376; PMCID: PMC7719341. doi:10.1016/j.jht.2020.04.001
Hoffman, H. G., Chambers, G. T., Meyer, W. J., Arceneaux, L. L., Russell, W. J., Seibel, E. J., et al. (2011). Virtual Reality as an Adjunctive Non-pharmacologic Analgesic for Acute Burn Pain during Medical Procedures. Ann. Behav. Med. 41 (2), 183–191. PMID: 21264690; PMCID: PMC4465767. doi:10.1007/s12160-010-9248-7
Hoffman, H. G. (2021). Interacting with Virtual Objects via Embodied Avatar Hands Reduces Pain Intensity and Diverts Attention. Sci. Rep. 11, 10672. doi:10.1038/s41598-021-89526-4
Hoffman, H. G., Patterson, D. R., and Carrougher, G. J. (2000b). Use of Virtual Reality for Adjunctive Treatment of Adult Burn Pain during Physical Therapy: a Controlled Study. Clin. J. Pain 16 (3), 244–250. PMID: 11014398. doi:10.1097/00002508-200009000-00010
Hoffman, H. G., Patterson, D. R., Magula, J., Carrougher, G. J., Zeltzer, K., Dagadakis, S., et al. (2004a). Water-friendly Virtual Reality Pain Control during Wound Care. J. Clin. Psychol. 60 (2), 189–195. PMID: 14724926. doi:10.1002/jclp.10244
Hoffman, H. G., Patterson, D. R., Rodriguez, R. A., Peña, R., Beck, W., and Meyer, W. J. (2020b). Virtual Reality Analgesia for Children with Large Severe Burn Wounds during Burn Wound Debridement. Front. Virtual Real. 1, 602299. Epub 2020 Dec 10. PMID: 33585833; PMCID: PMC7880045. doi:10.3389/frvir.2020.602299
Hoffman, H. G., Richards, T. L., Bills, A. R., Van Oostrom, T., Magula, J., Seibel, E. J., et al. (2006a). Using fMRI to Study the Neural Correlates of Virtual Reality Analgesia. CNS Spectr. 11 (1), 45–51. PMID: 16400255. doi:10.1017/s1092852900024202
Hoffman, H. G., Richards, T. L., Coda, B., Bills, A. R., Blough, D., Richards, A. L., et al. (2004b). Modulation of thermal Pain-Related Brain Activity with Virtual Reality: Evidence from fMRI. Neuroreport 15, 1245–1248. doi:10.1097/01.wnr.0000127826.73576.91
Hoffman, H. G., Richards, T. L., Van Oostrom, T., Coda, B. A., Jensen, M. P., Blough, D. K., et al. (2007). The Analgesic Effects of Opioids and Immersive Virtual Reality Distraction: Evidence from Subjective and Functional Brain Imaging Assessments. Anesth. Analg. 105 (6), 1776–1783. PMID: 18042882. doi:10.1213/01.ane.0000270205.45146.db
Hoffman, H. G., Rodriguez, R. A., Gonzalez, M., Bernardy, M., Peña, R., Beck, W., et al. (2019). Immersive Virtual Reality as an Adjunctive Non-opioid Analgesic for Pre-dominantly Latin American Children with Large Severe Burn Wounds during Burn Wound Cleaning in the Intensive Care Unit: A Pilot Study. Front. Hum. Neurosci. 13, 262. PMID: 31440148; PMCID: PMC6694842. doi:10.3389/fnhum.2019.00262
Hoffman, H. G., Seibel, E. J., Richards, T. L., Furness, T. A., Patterson, D. R., and Sharar, S. R. (2006b). Virtual Reality Helmet Display Quality Influences the Magnitude of Virtual Reality Analgesia. The J. Pain 7 (11), 843–850. PMID: 17074626. doi:10.1016/j.jpain.2006.04.006
Hoffman, H. (1998). Virtual Reality: A New Tool for Interdisciplinary Psychology Research. CyberPsychology Behav. 1 (2), 195–200. doi:10.1089/cpb.1998.1.195
Indovina, P., Barone, D., Gallo, L., Chirico, A., De Pietro, G., and Giordano, A. (2018). Virtual Reality as a Distraction Intervention to Relieve Pain and Distress during Medical Procedures. Clin. J. Pain 34 (9), 858–877. PMID: 29485536. doi:10.1097/AJP.0000000000000599
Juottonen, K., Gockel, M., Silén, T., Hurri, H., Hari, R., and Forss, N. (2002). Altered central Sensorimotor Processing in Patients with Complex Regional Pain Syndrome. Pain 98, 315–323. doi:10.1016/s0304-3959(02)00119-7
Kabat-Zinn, J. (1990). Full Catastrophe Living: Using the Wisdom of Your Body and Mind to Face Stress, Pain and Illness. New York (NY): Dell Publishing Co.
Kabat-Zinn, J. (1982). An Outpatient Program in Behavioral Medicine for Chronic Pain Patients Based on the Practice of Mindfulness Meditation: Theoretical Considerations and Preliminary Results. Gen. Hosp. Psychiatry 4 (1), 33–47. doi:10.1016/0163-8343(82)90026-3
Kabat-Zinn, J., Lipworth, L., and Burney, R. (1985). The Clinical Use of Mindfulness Meditation for the Self-Regulation of Chronic Pain. J. Behav. Med. 8 (2), 163–190. PMID: 3897551. doi:10.1007/BF00845519
Katz, N. (2002). The Impact of Pain Management on Quality of Life. J. Pain Symptom Manage. 24 (1 Suppl. l), S38–S47. PMID: 12204486. doi:10.1016/s0885-3924(02)00411-6
Keefe, F. J., Huling, D. A., Coggins, M. J., Keefe, D. F., Rosenthal, Z. M., Herr, N. R., et al. (2012). Virtual Reality for Persistent Pain: a New Direction for Behavioral Pain Management. Pain 153 (11), 2163–2166. Epub 2012 Jul 4. PMID: 22770840; PMCID: PMC3472118. doi:10.1016/j.pain.2012.05.030
Kharasch, E. D., and Brunt, L. M. (2016). Perioperative Opioids and Public Health. Anesthesiology 124 (4), 960–965. PMID: 26808634. doi:10.1097/ALN.0000000000001012
Kingery, W. S. (1997). A Critical Review of Controlled Clinical Trials for Peripheral Neuropathic Pain and Complex Regional Pain Syndromes. Pain 73 (2), 123–139. PMID: 9415498. doi:10.1016/S0304-3959(97)00049-3
Kipping, B., Rodger, S., Miller, K., and Kimble, R. M. (2012). Virtual Reality for Acute Pain Reduction in Adolescents Undergoing Burn Wound Care: a Prospective Randomized Controlled Trial. Burns 38, 650–657. doi:10.1016/j.burns.2011.11.010
Kopp, B., Kunkel, A., Münickel, W., Villringer, K., Taub, E., and Flor, H. (1999). Plasticity in the Motor System Related to Therapy-Induced Improvement of Movement after Stroke. NeuroReport 10, 807–810. doi:10.1097/00001756-199903170-00026
Kramer, T. L., Pyne, J. M., Kimbrell, T. A., Savary, P. E., Smith, J. L., and Jegley, S. M. (2010). Clinician Perceptions of Virtual Reality to Assess and Treat Returning Veterans. Ps 61 (11), 1153–1156. PMID: 21041358. doi:10.1176/ps.2010.61.11.1153
Kuys, S. S., Edwards, T., and Morris, N. R. (2012). Effects and Adherence of Mirror Therapy in People with Chronic Upper Limb Hemiparesis: A Preliminary Study. ISRN Rehabil. 2012, 1–9. doi:10.5402/2012/926784
Ladha, K. S., Neuman, M. D., Broms, G., Bethell, J., Bateman, B. T., Wijeysundera, D. N., et al. (2019). Opioid Prescribing after Surgery in the United States, Canada, and Sweden. JAMA Netw. Open 2 (9), e1910734. PMID: 31483475; PMCID: PMC6727684. doi:10.1001/jamanetworkopen.2019.10734
Le May, S., Tsimicalis, A., Noel, M., Rainville, P., Khadra, C., Ballard, A., et al. (2021). Immersive Virtual Reality vs. Non‐immersive Distraction for Pain Management of Children during Bone Pins and Sutures Removal: A Randomized Clinical Trial Protocol. J. Adv. Nurs. 77 (1), 439–447. doi:10.1111/jan.14607
Lew, D., Bancila, L., Wang, K., Kim, S., Spiegel, B., Almario, C., et al. (2020). S0081 Virtual Reality Can Reduce Pain and Opioid Use in Sphincter of Oddi Dysfunction (SOD) Type III: A Prospective Pilot Study. Am. J. Gastroenterol. 115, S39–S40. doi:10.14309/01.ajg.0000702372.03529.33
Liepert, J., Bauder, H., Miltner, W. H. R., Taub, E., Weiller, C., and Weiller, C. (2000). Treatment-induced Cortical Reorganization after Stroke in Humans. Stroke 31, 1210–1216. doi:10.1161/01.str.31.6.1210
Linehan, M. M. (1993). Cognitive-Behavioral Treatment of Borderline Personality Disorder. New York: Guilford Press.
Louw, A., Rico, D., Langerwerf, L., Maiers, N., Diener, I., and Cox, T. (2020). Preoperative Pain Neuroscience Education for Shoulder Surgery: A Case Series. South Afr. J. Physiother. 76 (1), 1417. PMID: 32935067; PMCID: PMC7479411. doi:10.4102/sajp.v76i1.1417
Love, A. S., and Love, R. J. (2021). Considering Needle Phobia Among Adult Patients during Mass COVID-19 Vaccinations. J. Prim. Care Community Health 12, 21501327211007393. doi:10.1177/21501327211007393
Maani, C., Hoffman, H. G., DeSocio, P. A., Morrow, M., Galin, C., Magula, J., et al. (2008). Pain Control during Wound Care for Combat-Related Burn Injuries Using Custom Articulated Arm Mounted Virtual Reality Goggles. J. Cyber Ther. Rehabil. 1 (2), 193–198.
Maani, C. V., Hoffman, H. G., Fowler, M., Maiers, A. J., Gaylord, K. M., and Desocio, P. A. (2011a). Combining Ketamine and Virtual Reality Pain Control during Severe Burn Wound Care: One Military and One Civilian Patient. Pain Med. 12 (4), 673–678. Epub 2011 Apr 11. PMID: 21481162; PMCID: PMC4460983. doi:10.1111/j.1526-4637.2011.01091.x
Maani, C. V., Hoffman, H. G., Morrow, M., Maiers, A., Gaylord, K., McGhee, L. L., et al. (2011b). Virtual Reality Pain Control during Burn Wound Debridement of Combat-Related Burn Injuries Using Robot-like Arm Mounted VR Goggles. J. Trauma 71 (1 Suppl. l), S125–S130. PMID: 21795888; PMCID: PMC4460976. doi:10.1097/TA.0b013e31822192e2
Malchow, R. J., and Black, I. H. (2008). The Evolution of Pain Management in the Critically Ill Trauma Patient: Emerging Concepts from the Global War on Terrorism. Crit. Care Med. 36 (7 Suppl. l), S346–S357. PMID: 18594262. doi:10.1097/CCM.0b013e31817e2fc9
Malloy, K. M., and Milling, L. S. (2010). The Effectiveness of Virtual Reality Distraction for Pain Reduction: a Systematic Review. Clin. Psychol. Rev. 30 (8), 1011–1018. Epub 2010 Jul 13. PMID: 20691523. doi:10.1016/j.cpr.2010.07.001
Matamala-Gomez, M., Diaz Gonzalez, A. M., Slater, M., and Sanchez-Vives, M. V. (2019). Decreasing Pain Ratings in Chronic Arm Pain through Changing a Virtual Body: Different Strategies for Different Pain Types. J. Pain 20 (6), 685–697. Epub 2018 Dec 16. PMID: 30562584. doi:10.1016/j.jpain.2018.12.001
Matamala-Gomez, M., Donegan, T., Bottiroli, S., Sandrini, G., Sanchez-Vives, M. V., and Tassorelli, C. (2019). Immersive Virtual Reality and Virtual Embodiment for Pain Relief. Front. Hum. Neurosci. 13, 279. doi:10.3389/fnhum.2019.00279
Matamala-Gomez, M., Nierula, B., Donegan, T., Slater, M., and Sanchez-Vives, M. V. (2020). Manipulating the Perceived Shape and Color of a Virtual Limb Can Modulate Pain Responses. Jcm 9 (2), 291. doi:10.3390/jcm9020291
McGreevy, K., Bottros, M. M., and Raja, S. N. (2011). Preventing Chronic Pain Following Acute Pain: Risk Factors, Preventive Strategies, and Their Efficacy. Eur. J. Pain Suppl. 5 (2), 365–376. doi:10.1016/j.eujps.2011.08.013
McLay, R., Ram, V., Murphy, J., Spira, J., Wood, D. P., Wiederhold, M. D., et al. (2014). Effect of Virtual Reality PTSD Treatment on Mood and Neurocognitive Outcomes. Cyberpsychology, Behav. Soc. Networking 17 (7), 439–446. doi:10.1089/cyber.2013.0383
McSherry, T., Atterbury, M., Gartner, S., Helmold, E., Searles, D. M., and Schulman, C. (2017). Randomized, Crossover Study of Immersive Virtual Reality to Decrease Opioid Use during Painful Wound Care Procedures in Adults. J. Burn Care Res. 39 (2), 1–285. PMID: 28570305. doi:10.1097/BCR.0000000000000589
Méndez-Rebolledo, G., Gatica-Rojas, V., Torres-Cueco, R., Albornoz-Verdugo, M., and Guzmán-Muñoz, E. (2017). Update on the Effects of Graded Motor Imagery and Mirror Therapy on Complex Regional Pain Syndrome Type 1: A Systematic Review. Bmr 30 (3), 441–449. PMID: 27858687. doi:10.3233/BMR-150500
Miner, A., Kuhn, E., Hoffman, J. E., Owen, J. E., Ruzek, J. I., and Taylor, C. B. (2016). Feasibility, Acceptability, and Potential Efficacy of the PTSD Coach App: A Pilot Randomized Controlled Trial with Community Trauma Survivors. Psychol. Trauma Theor. Res. Pract. Pol. 8 (3), 384–392. Epub 2016 Jan 25. PMID: 27046668. doi:10.1037/tra0000092
Molloy, J. M., Pendergrass, T. L., Lee, I. E., Chervak, M. C., Hauret, K. G., and Rhon, D. I. (2020). Musculoskeletal Injuries and United States Army Readiness Part I: Overview of Injuries and Their Strategic Impact. Mil. Med. 185 (9-10), e1461–e1471. PMID: 32175566. doi:10.1093/milmed/usaa027
Montgomery, H. R., Drew, B., and Butler, F. K. (2021). Summary of Recent Changes to the TCCC Guidelines 14 April 2021. J. Spec. Oper. Med. 21 (2), 120–121. PMID: 34105137.
Morina, N., Ijntema, H., Meyerbröker, K., and Emmelkamp, P. M. G. (2015). Can Virtual Reality Exposure Therapy Gains Be Generalized to Real-Life? A Meta-Analysis of Studies Applying Behavioral Assessments. Behav. Res. Ther. 74, 18–24. Epub 2015 Aug 31. PMID: 26355646. doi:10.1016/j.brat.2015.08.010
Morris, L. D., Louw, Q. A., and Grimmer-Somers, K. (2009). The Effectiveness of Virtual Reality on Reducing Pain and Anxiety in Burn Injury Patients. Clin. J. Pain 25 (9), 815–826. PMID: 19851164. doi:10.1097/AJP.0b013e3181aaa909
Moseley, G. L., Gallace, A., and Spence, C. (2008). Is Mirror Therapy All it Is Cracked up to Be? Current Evidence and Future Directions. Pain 138, 7–10. doi:10.1016/j.pain.2008.06.026
Moseley, L. G. (2004). Graded Motor Imagery Is Effective for Long-Standing Complex Regional Pain Syndrome: a Randomised Controlled Trial. Pain 108 (1-2), 192–198. PMID: 15109523. doi:10.1016/j.pain.2004.01.006
Mowery, D. C., and Simcoe, T. (2002). Is the Internet a US Invention?—An Economic and Technological History of Computer Networking. Res. Pol. 31 (8-9), 1369–1387. doi:10.1016/s0048-7333(02)00069-0
Nahin, R. L. (2017). Severe Pain in Veterans: The Effect of Age and Sex, and Comparisons with the General Population. J. Pain 18 (3), 247–254. doi:10.1016/j.jpain.2016.10.021
Nararro-Haro, M. V., Hoffman, H. G., Garcia-Palacios, A., Sampaio, M., Alhalabi, W., Hall, K., et al. (2016). The Use of Virtual Reality to Facilitate Mindfulness Skills Training in Dialectical Behavioral Therapy for Borderline Personality Disorder: a Case Study. Front. Psychol. 7, 1573. doi:10.3389/fpsyg.2016.01573
Navarro-Haro, M. V., Modrego-Alarcón, M., Hoffman, H. G., López-Montoyo, A., Navarro-Gil, M., Montero-Marin, J., et al. (2019). Evaluation of a Mindfulness-Based Intervention with and without Virtual Reality Dialectical Behavior Therapy Mindfulness Skills Training for the Treatment of Generalized Anxiety Disorder in Primary Care: A Pilot Study. Front. Psychol. 10, 55. doi:10.3389/fpsyg.2019.00055
Neria, Y. (2021). Functional Neuroimaging in PTSD: From Discovery of Underlying Mechanisms to Addressing Diagnostic Heterogeneity. Ajp 178 (2), 128–135. PMID: 33517750. doi:10.1176/appi.ajp.2020.20121727
Nguyen, K. T., Beauchamp, D. W., Lovett, U., Tillman, D., Janze, A., Ruiz, A., et al. (2020). Evaluation of a Functional Restoration Program at Fort Bliss Interdisciplinary Pain Management Clinic. Mil. Med. 185 (11-12), e2097–103. doi:10.1093/milmed/usaa200
Norr, A. M., Smolenski, D. J., Katz, A. C., Rizzo, A. A., Rothbaum, B. O., Difede, J., et al. (2018). Virtual Reality Exposure versus Prolonged Exposure for PTSD: Which Treatment for Whom. Depress. Anxiety 35 (6), 523–529. Epub 2018 May 7. PMID: 29734488. doi:10.1002/da.22751
O’Malley, K. A., Vinson, L., Pless Kaiser, A., Sager, Z., and Hinrichs, K. (2020). Mental Health and Aging Veterans: How the Veterans’ Health Administration Meets the Needs of Aging Veterans. Public Pol. Aging Rep. 30 (1), 19–23.
Perez, R. S. G. M., Kwakkel, G., Zuurmond, W. W. A., De Lange, J. J., and management, s. (2001). Treatment of Reflex Sympathetic Dystrophy (CRPS Type 1). J. Pain Symptom Manage. 21 (6), 511–526. doi:10.1016/s0885-3924(01)00282-2
Pierce, B. S., Perrin, P. B., Tyler, C. M., McKee, G. B., and Watson, J. D. (2020). The COVID-19 Telepsychology Revolution: A National Study of Pandemic-Based Changes in U.S. Mental Health Care Delivery. Am. Psychol. 76 (1), 14–25. doi:10.1037/amp0000722
Ramachandran, V. S., and Rogers-Ramachandran, D. (1996). Synaesthesia in Phantom Limbs Induced with Mirrors. Proc. Biol. Sci. 263 (263), 377–386. doi:10.1098/rspb.1996.0058
Rawlins, C. R., Veigulis, Z., Hebert, C., Curtin, C., and Osborne, T. F. (2021). Effect of Immersive Virtual Reality on Pain and Anxiety at a Veterans Affairs Health Care Facility. Front. Virtual Real. 2, 719681. doi:10.3389/frvir.2021.719681
Reif, S., Adams, R. S., Ritter, G. A., Williams, T. V., and Larson, M. J. (2018). Prevalence of Pain Diagnoses and Burden of Pain Among Active Duty Soldiers, FY2012. Mil. Med. 183 (9-10), e330–e337. PMID: 29547946; PMCID: PMC6115865. doi:10.1093/milmed/usx200
Rizzo, A. S., and Shilling, R. (2017). Clinical Virtual Reality Tools to advance the Prevention, Assessment, and Treatment of PTSD. Eur. J. Psychotraumatology 8 (Suppl. 5), 1414560. PMID: 29372007; PMCID: PMC5774399. doi:10.1080/20008198.2017.1414560
Rogers, E., Mehta, S., Shengelia, R., and Reid, M. C. (2013). Four Strategies for Managing Opioid-Induced Side Effects in Older Adults. Clin. Geriatr. 21 (4). Available at: http://www.consultant360.com/articles/four-strategies-managing-opioid-induced-side-effects-older-adults.
Rosenberg, J. M., Bilka, B. M., Wilson, S. M., and Spevak, C. (2018). Opioid Therapy for Chronic Pain: Overview of the 2017 US Department of Veterans Affairs and US Department of Defense Clinical Practice Guideline. Pain Med. 19 (5), 928–941. PMID :29025128. doi:10.1093/pm/pnx203
Rothbaum, B. O., Price, M., Jovanovic, T., Norrholm, S. D., Gerardi, M., Dunlop, B., et al. (2014). A Randomized, Double-Blind Evaluation Of d-Cycloserine or Alprazolam Combined with Virtual Reality Exposure Therapy for Posttraumatic Stress Disorder in Iraq and Afghanistan War Veterans. Ajp 171 (6), 640–648. PMID: 24743802; PMCID: PMC4115813. doi:10.1176/appi.ajp.2014.13121625
Sampaio, M., Haro, M. V., De Sousa, B., Melo, W. V., and Hoffman, H. G. (2021a). Therapists Make the Switch to Telepsychology to Safely Continue Treating Their Patients during the COVID-19 Pandemic, Virtual Reality Telepsychology May Be Next 1, 1–27. doi:10.3389/frvir.2020.576421
Sampaio, M., Navarro Haro, M. V., Wilks, C., De Sousa, B., Garcia-Palacios, A., and Hoffman, H. G. (2021b). Spanish-speaking Therapists Increasingly Switch to Telepsychology during COVID-19: Networked Virtual Reality May Be Next. Telemed. e-Health 27, 919–928. Epub ahead of print. PMID: 34182825. doi:10.1089/tmj.2021.0124
Sandbrink, F., Oliva, E. M., McMullen, T. L., Aylor, A. R., Harvey, M. A., Christopher, M. L., et al. (2020). Opioid Prescribing and Opioid Risk Mitigation Strategies in the Veterans Health Administration. J. Gen. Intern. Med. 35 (Suppl. 3), 927–934. Epub 2020 Nov 16. PMID: 33196968; PMCID: PMC7728840. doi:10.1007/s11606-020-06258-3
Satava, R. M., Stefanidis, D., Levy, J. S., Smith, R., Martin, J. R., Monfared, S., et al. (2020). Proving the Effectiveness of the Fundamentals of Robotic Surgery (FRS) Skills Curriculum. Ann. Surg. 272 (2), 384–392. PMID: 32675553. doi:10.1097/SLA.0000000000003220
Schwoebel, J., Friedman, R., Duda, N., and Coslett, H. B. (2001). Pain and the Body Schema: Evidence for Peripheral Effects on Mental Representations of Movement. Brain 124, 2098–2104. doi:10.1093/brain/124.10.2098
Shoar, S., Esmaeili, S., and Safari, S. (2012). Pain Management after Surgery: a Brief Review. Anesth. Pain 1 (3), 184–186. Epub 2012 Jan 1. PMID: 24904790; PMCID: PMC4018688. doi:10.5812/kowsar.22287523.3443
Siedlecka, M., Klimza, A., Łukowska, M., and Wierzchoń, M. (2014). Rubber Hand Illusion Reduces Discomfort Caused by Cold Stimulus. PLoS One 9, e109909. doi:10.1371/journal.pone.0109909
Skadberg, R. M., Moore, T. M., and Elledge, L. C. (2020). A 20-year Study of the Bidirectional Relationship between Anxious and Depressive Symptomology and Pain Medication Usage. Pain Manage. 10 (1), 13–22. Epub 2020 Jan 30. PMID: 31999222. doi:10.2217/pmt-2019-0037
Smart, K. M., Wand, B. M., and O'Connell, N. E. (2016). Physiotherapy for Pain and Disability in Adults with Complex Regional Pain Syndrome (CRPS) Types I and II. Cochrane Database Syst. Rev. 2, CD010853. PMID: 26905470. doi:10.1002/14651858.CD010853.pub2
Solcà, M., Ronchi, R., Bello-Ruiz, J., Schmidlin, T., Herbelin, B., Luthi, F., et al. (2018). Heartbeat-enhanced Immersive Virtual Reality to Treat Complex Regional Pain Syndrome. Neurology 91 (5), e479–e489. Epub 2018 Jul 6. PMID: 29980635. doi:10.1212/WNL.0000000000005905
Sun, E. C., Darnall, B. D., Baker, L. C., and Mackey, S. (2016). Incidence of and Risk Factors for Chronic Opioid Use Among Opioid-Naive Patients in the Postoperative Period. JAMA Intern. Med. 176 (9), 1286–1293. doi:10.1001/jamainternmed.2016.3298
Tanielian, T., and Farmer, C. (2019). The US Military Health System: Promoting Readiness and Providing Health Care. Health Aff. 38 (8), 1259–1267. PMID: 31381396. doi:10.1377/hlthaff.2019.00239
Taylor, S.-S., Noor, N., Urits, I., Paladini, A., Sadhu, M. S., Gibb, C., et al. (2021). Complex Regional Pain Syndrome: A Comprehensive Review. Pain Ther. doi:10.1007/s40122-021-00279-4
Tran, J. E., Fowler, C. A., Delikat, J., Kaplan, H., Merzier, M. M., Schlesinger, M. R., et al. (2021). Immersive Virtual Reality to Improve Outcomes in Veterans with Stroke: Protocol for a Single-Arm Pilot Study. JMIR Res. Protoc. 10 (5), e26133. doi:10.2196/26133
Trost, Z., Anam, M., Seward, J., Shum, C., Rumble, D., Sturgeon, J., et al. (2021a). Immersive Interactive Virtual Walking Reduces Neuropathic Pain in Spinal Cord Injury: Findings from a Preliminary Investigation of Feasibility and Clinical Efficacy. PAIN, 1–12. doi:10.1097/j.pain.0000000000002348
Trost, Z., France, C., Anam, M., and Shum, C. (2021b). Virtual Reality Approaches to Pain: toward a State of the Science. PAIN 162, 325–331. doi:10.1097/j.pain.0000000000002060
Upton, D., Solowiej, K., Hender, C., and Woo, K. Y. (2012). Stress and Pain Associated with Dressing Change in Patients with Chronic Wounds, 56,58 passim. J. Wound Care 21 (2), 53–61. PMID: 22584524. doi:10.12968/jowc.2012.21.2.53
van Steenbergen, H., Eikemo, M., and Leknes, S. (2019). The Role of the Opioid System in Decision Making and Cognitive Control: A Review. Cogn. Affect Behav. Neurosci. 19 (3), 435–458. PMID: 30963411; PMCID: PMC6599188. doi:10.3758/s13415-019-00710-6
Wang, X., Ali, N., and Lin, C.-l. G. (2021). Emerging Role of Glutamate in the Pathophysiology and Therapeutics of Gulf War Illness. Life Sci. 280, 119609. Epub 2021 May 13. PMID: 33991547. doi:10.1016/j.lfs.2021.119609
Weech, S., Kenny, S., and Barnett-Cowan, M. (2019). Presence and Cybersickness in Virtual Reality Are Negatively Related: a Review. Front. Psychol. 10, 158. doi:10.3389/fpsyg.2019.00158
Wender, R., Hoffman, H. G., Hunner, H. H., Seibel, E. J., Patterson, D. R., and Sharar, S. R. (2009). Interactivity Influences the Magnitude of Virtual Reality Analgesia. J. Cyber Ther. Rehabil. 2 (1), 27–33. PMID: 20390047; PMCID: PMC2853033.
Wendrich, R. E., Chambers, K., Al-Halabi, W., Seibel, E. J., Grevenstuk, O., Ullman, D., et al. (2016). “Hybrid Design Tools in a Social Virtual Reality Using Networked Oculus Rift: A Feasibility Study in Remote Real-Time Interaction,” in Proceedings of the ASME 2016 International Design Engineering Technical Conferences & Computers and Information in Engineering Conference IDETC/CIE, North Carolina, USA, 2016 August (Charlotte), 21–24.
Wiederhold, B. K., Gao, K., Sulea, C., and Wiederhold, M. D. (2014). Virtual Reality as a Distraction Technique in Chronic Pain Patients. Cyberpsychology, Behav. Soc. Networking 17 (6), 346–352. PMID: 24892196; PMCID: PMC4043365. doi:10.1089/cyber.2014.0207
Wiederhold, M. D., and Wiederhold, B. K. (2007). Virtual Reality and Interactive Simulation for Pain Distraction: Table 1. Pain Med. 8 (3), S182–S188. doi:10.1111/j.1526-4637.2007.00381.x
Wilson, N., Kariisa, M., Seth, P., Smith, H., and Davis, N. L. (2020). Drug and Opioid-Involved Overdose Deaths - United States, 2017-2018. MMWR Morb. Mortal. Wkly. Rep. 69 (11), 290–297. doi:10.15585/mmwr.mm6911a4external.icon
Woolf, C. J. (2011). Central Sensitization: Implications for the Diagnosis and Treatment of Pain. PAIN, 152 (3), S2–S15. doi:10.1016/j.pain.2010.09.030
Zhang, Y., Avery, T., Vakhtin, A. A., Mathersul, D. C., Tranvinh, E., Wintermark, M., et al. (2020). Brainstem Atrophy in Gulf War Illness. Neurotoxicology 78, 71–79. doi:10.1016/j.neuro.2020.02.006
Keywords: virtual reality, opioids, analgesia, chronic pain, military, veterans
Citation: Peterson BN, Hitching R, Howard L, Zhu K, Fontenot MR, Alhalabi W, Seibel A, Harris OA, Madrigal E, Adamson MM and Hoffman HG (2021) Immersive Virtual Reality: A Safe, Scalable, Non-opioid Analgesic for Military and Veteran Patients. Front. Virtual Real. 2:742290. doi: 10.3389/frvir.2021.742290
Received: 16 July 2021; Accepted: 21 October 2021;
Published: 30 November 2021.
Edited by:
Albert Rizzo, University of Southern California, United StatesReviewed by:
Katarzyna Wyka, City University of New York, United StatesVangelis Lympouridis, University of Southern California, United States
Copyright © 2021 Peterson, Hitching, Howard, Zhu, Fontenot, Alhalabi, Seibel, Harris, Madrigal, Adamson and Hoffman. This is an open-access article distributed under the terms of the Creative Commons Attribution License (CC BY). The use, distribution or reproduction in other forums is permitted, provided the original author(s) and the copyright owner(s) are credited and that the original publication in this journal is cited, in accordance with accepted academic practice. No use, distribution or reproduction is permitted which does not comply with these terms.
*Correspondence: Maheen Mausoof Adamson, bWFkYW1zb25Ac3RhbmZvcmQuZWR1; Hunter G. Hoffman, aHVudGhvZmY5QGdtYWlsLmNvbQ==
†These authors share first authorship