- 1Learning in Virtual Environments Lab, Department of Electrical Engineering and Computer Science, Vanderbilt University, Nashville, TN, United States
- 2Verizon Wireless, Nashville, TN, United States
Spatial perception in immersive virtual environments, particularly regarding distance perception, is a well-studied topic in virtual reality literature. Distance compression, or the underestimation of distances, is and has been historically prevalent in all virtual reality systems. The problem of distance compression still remains open, but recent advancements have shown that as systems have developed, the level of distance compression has decreased. Here, we add evidence to this trend by beginning the assessment of distance compression in the HTC Vive Pro. To our knowledge, there are no archival results that report any findings about distance compression in this system. Using a familiar paradigm for studying distance compression in virtual reality hardware, we asked users to blind walk to a target object placed in a virtual environment and assessed their judgments based on those distances. We find that distance compression in the HTC Vive Pro mirrors that of the HTC Vive. Our results are not particularly surprising, considering the nature of the differences between the two systems, but they lend credence to the finding that resolution does not affect distance compression. More extensive study should be performed to reinforce these results.
Introduction
Scientific literature has demonstrated that people have the ability to accurately judge the distance between themselves and objects resting on a ground plane at distances in action space (up to 20 m away) (Thompson et al., 2004). However, this ability does not translate when one is viewing a simulated immersive virtual environment (IVE) that is mediated through a head-mounted display (HMD), and people tend to underestimate the distances between themselves and objects. This phenomenon is called distance compression. Distance compression in IVEs is common and widely reported in head-mounted displays (Renner et al., 2013; Creem-Regehr et al., 2015). For some time, the underlying cause of distance compression has not been understood, but some recent advancements have revealed some contributing factors to this issue (Buck et al., 2018; Masnadi et al., 2021). While distance compression still remains in virtual reality systems, it has been somewhat alleviated with the advent of newer commodity-level hardware. It is important to continue to understand distance compression in these systems; correct spatial perception is required to simulate realism in many different applications such as those used for medical and military training, architectural design, and educational simulations (Fox et al., 2009).
There is an expanse of literature documenting distance compression in various HMDs over the past 30 years, of which Renner et al. (2013) provide a thorough review. Distance compression findings have a history of mixed results; Witmer and Sadowski (1998) suggested the cause to be binocular disparity or poor pictorial depth cues, Thompson et al. (2004) eliminated the graphical quality of the environment as a cause, and Young et al. (2014) found systems with dissimilar mechanical qualities (i.e., weight, field of view (FOV) and resolution) to have different levels of compression. Recent work by Buck et al. (2018) pursued the findings from Young et al. (2014) and produced results that added further credibility to the notion that distance compression is affected by the mechanical factors of an HMD, specifically weight and FOV. Results also pointed to a reduction in distance compression in HMDs that have been released in more recent years—the HTC Vive and Oculus Rift Consumer Version 1 being those HMDs. Kelly et al. (2017) found the HTC Vive to produce less distance compression than older systems, and additionally, Li et al. (2018) and Masnadi et al. (2021) have both found that systems with a greater FOV elicit less distance compression.
Prior to most recent literature, however, findings have been mixed regarding the effect mechanical factors have on distance compression (Creem-Regehr et al. (2005); Jones et al. (2012); Willemsen et al. (2009)). When simulating an HMD, Knapp and Loomis (2004), Willemsen et al. (2009) and Grechkin et al. (2010) did not find results that suggested FOV or weight would have an effect on distance compression in an HMD. It is easy to speculate that the results of this body of work are not similar to our own since the authors tested these factors in a real environment instead of a virtual one. Thus, we press forward with the study of distance compression in the HTC Vive Pro to add further results to the literature with the expectation that, given that the HTC Vive and HTC Vive Pro have same weight and FOV, we will shed light on whether resolution has an effect on distance compression.
Here, we expand upon previous work by Buck et al. (2018) by assessing the performance of the HTC Vive Pro to determine the amount of compression in that system. In that work, mechanical factors of different HMDs (both old and current hardware) were evaluated and compared, and it was found that FOV and weight affected distance compression in each system. One of the key contributions of Buck et al. was the finding that FOV played a role in distance compression, as this factor had been discounted previously and had not been assessed in a commodity level system (Knapp and Loomis (2004)). The standard HTC Vive was tested, and it produced less distance compression than systems which tended to have a greater weight and FOV. Buck et al. also verified a finding of Thompson et al. (2004) that weight was a factor in distance compression.
Since the HTC Vive Pro is a newer version of the Vive headset with different properties, it offers an opportunity to test interesting factors not tested in the prior work mentioned (Buck et al. (2018); Kelly et al. (2017)). Namely, the Vive Pro has approximately the same weight and FOV characteristics as the Vive, but it has a significantly higher resolution. To our knowledge, there are no reported distance compression effects for this system, and so we believe it beneficial to assess and add to the current literature on distance perception in IVEs. We are not aware of prior studies that directly examine the effect of resolution on distance estimation in HMD-based virtual environments, but Thompson et al. (2004) found no effect of graphical quality on distance estimates in virtual environments and Langbehn et al. (2016) found no effect of visual blur on distance estimation. Thus, we hypothesize that we will find similar amounts of distance compression in the Vive Pro and the HTC Vive, as we do not believe that resolution will have a significant role in distance compression.
Methods
The same environment—both physical and virtual—as the one used in Buck et al. (2018) was used to assess distance perception in the HTC Vive Pro (Figure 1). The virtual environment was built using Vizard and consists of 3D planes textured with photographs from our campus to replicate an outdoor environment. The HTC Vive Pro has a resolution of 1,440 × 1,600 pixels per eye (2,880 × 1,600 pixels combined), the nominal FOV is 110° diagonally, the weight is 555 g and it has a refresh rate of 90 Hz. Twelve subjects (8 male, 4 female) were required to perform an indirect blind walking task. This was because distances displayed in the IVE were larger than our lab supports, and we wanted to replicate the method used in Buck et al. (2018) so that we could directly compare our results. Subjects wore the Vive Pro HMD throughout the study (Figure 2) and were allowed to adjust the fit and IPD of the HMD if necessary. Subjects were not instructed to make nor did they lens distance adjustments (i.e., they did not move the lenses further or closer to the eye); the lenses were left in their standard position. No subject had eyeglasses or eyelashes that interfered with the default position of the lens, and changing the default position of the lens would have altered the field of view of the device. A piece of tape on the lab floor denoted where subjects stood during the experiment, and subjects were not allowed to move freely throughout the IVE. A target (red hockey puck) was displayed in the IVE, and subjects were required to estimate the distance to that target. We chose a red hockey puck as the target because of its presence in classical literature on egocentric distance judgments made in the real world (Sinai et al. (1998); Wu et al. (2004)), and its use in prior distance judgment experiments in virtual environments Lin et al. (2011); Buck et al. (2018). After subjects were sure of their estimations, they were asked to close their eyes, remove the HMD and don a blindfold. They were then led into an adjacent hallway (walking approximately 3 m), and asked to blindly walk the distance that they had estimated. The adjacent hallway provided subjects with 70 m of unobstructed walking space. The target was displayed three separate times at 5, 7.5, and 10 m in a randomized order, resulting in 9 trials. Subjects completed two training trials at 6 and 8 m before beginning the actual experiment to familiarize themselves with the process. All walked distances for the trials were recorded.
Results
The measure that we used in processing our results captures the accuracy and precision at which subjects judged distance. Training trials were not included in our analyses.
We calculated the ratio of the judged distance to true distance (which we also refer to as constant error):
A repeated measures ANOVA with within subjects factors of distance and trial was run. Assumptions were checked and corrected for by SPSS. Results show a main effect of distance (F (2, 44) = 7.269, p = 0.013) and no effect of trial. A post hoc Tukey HSD test showed significant differences between 5 and 7.5, 5 1m and 10 m, but no difference between 7.5 and 10 m. The Vive Pro has an average constant error of 0.55 (SD = 0.133), 0.61 (SD = 0.186), and 0.65 (SD = 0.227) at 5, 7.5, and 10 m respectively. The average constant error across all trials in the Vive Pro is 0.60. These results demonstrate that subjects underestimated distances differently at each distance (except between 7.5 and 10 m) and experienced distance compression at all distances.
In order to determine whether distance compression in the HTC Vive Pro is similar to that found in HTC Vive, we ran an independent samples t-test over the constant error with a grouping variable of device (HTC Vive and HTC Vive Pro). There was a significant difference between the HTC Vive (M = 0.729, SD = 0.083) and the HTC Vive Pro (M = 0.650, SD = 0.224) when the target was placed at 10m; t (22) = 1.127, p = 0.008, but no other significant differences were found. We next ran Bayes Factor analyses. Bayes factors provide support for the null hypothesis through an odds ratio1 We use the method developed by Rouder et al. (2009), which takes sample size into account and adjusts for power. Prior odds were set to 1, which favors neither the null nor the alternative. Comparing the constant error between devices at 5 m gives a Jeffrey-Zellner-Siow (JZS) Bayes factor of 2.58 in favor of the null hypothesis. Comparing the constant error between devices at 7.5 m gives a JZS Bayes factor of 2.92 in favor of the null hypothesis. And finally, comparing the constant error between devices at 10 m gives a JZS Bayes factor of 2.07 in favor of the null hypothesis. Thus, it is important to note the similarity between these results and those found from the HTC Vive in Buck et al. (2018). The HTC Vive had an average constant error of 0.60 (SD = 0.123), 0.65 (SD = 0.112), and 0.73 (SD = 0.083) at 5, 7.5, and 10 m respectively. The average constant error across all trials in the HTC Vive found in that work was 0.66. The ratios of judged distance to true distance for both the HTC Vive Pro and HTC Vive can be found and compared in Figure 3.
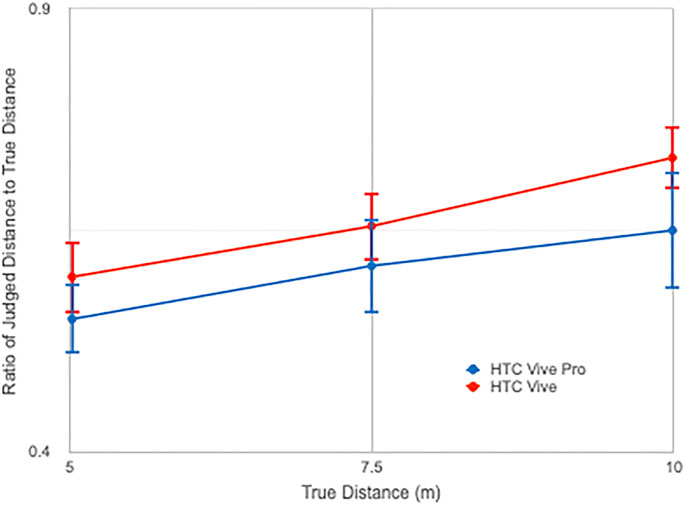
FIGURE 3. The ratio of the judged distance to true distance for each distance tested in both the Vive Pro and Vive HMDs.
Discussion
Ultimately, this work sought to measure the amount of distance compression in the HTC Vive Pro. From a mechanical standpoint, these findings are interesting considering the specs of the HTC Vive in relation to the HTC Vive Pro. These systems only differ in resolution, with the HTC Vive having a resolution of 2,160 × 1,200 and the HTC Vive Pro having a resolution of 2,880 × 1,600. Since these systems performed similarly, this is further evidence that resolution does not affect distance compression, as was found by Willemsen and Gooch (2002) and Thompson et al. (2004). However, we must consider that in older works, the type of estimation task (i.e., blind walking, triangulation, verbal estimates, etc.) affects distance estimates (Peer and Ponto (2017)), and have been shown to affect distance estimates made in environments with different graphical fidelity (Renner et al. (2013)).
Spatial resolution, or where the pixel density and field of view are taken into account to describe how resolution is experienced in virtual reality, is worth mentioning in light of this work. It is known that the lenses in HMDs tend to cause pixels to be distributed unevenly across the visual field, thus resulting in degraded visual acuity (Fidopiastis et al. (2005); Peer and Ponto (2020)). Older systems, such as those used in Wright (1995), have a similar spatial resolution to newer systems. These results might suggest that the visual acuity achieved in systems may not have an effect on distance judgments.
Recent work on distance judgments made in virtual environments have found that distance estimates continue to be more accurate in newer systems. Feldstein et al. (2020) found distance estimates to be equally accurate in a real and a virtual environment using an Oculus Rift DK2. The environment in this work provided users with a motion tracked avatar and a matched photorealistic rendering of the real environment used. The inclusion of a realistic avatar would have perhaps increased the accuracy of estimates in our own work (Mohler et al. (2008); Phillips et al. (2010)). The fact that we did not have a real world environment is also a limitation of our work, even though the accuracy of the distance judgments made in our work is substantially different than what is normally found in the real world (Thompson et al. (2004)). Additional work has found the physical environment to have an effect on distance judgments (Zhang et al. (2021)), which should be explored further and could have potentially impacted our own results.
While the HTC Vive and HTC Vive Pro are still considered commodity level systems, it would be interesting to look at other systems that have been released recently such as the Oculus Quest 2 and the Varjo XR-3. The Oculus Quest 2 is lighter than the Vive HMDs, and the Varjo XR-3 boasts the widest field of view of any widely available XR HMD. Additionally, augmented reality systems like the HoloLens face the same issue and need further study, although the underlying mechanisms causing underestimation are likely different (Gagnon et al. (2021); Peillard et al. (2020)).
Data Availability Statement
The raw data supporting the conclusions of this article will be made available by the authors, without undue reservation.
Ethics Statement
The studies involving human participants were reviewed and approved by the Vanderbilt University Institutional Review Board. The patients/participants provided their written informed consent to participate in this study.
Author Contributions
LB wrote the article, developed the VR software for the experiment, ran study participants and data analysis. RP ran study participants and helped with data analysis and figures. BB came up with the experimental idea and edited the article.
Funding
This work is supported under a grant issued by the Office of Naval Research (N00014-18-1-2964).
Conflict of Interest
RP was employed by Verizon Wireless.
The remaining authors declare that the research was conducted in the absence of any commercial or financial relationships that could be construed as a potential conflict of interest.
Publisher’s Note
All claims expressed in this article are solely those of the authors and do not necessarily represent those of their affiliated organizations, or those of the publisher, the editors and the reviewers. Any product that may be evaluated in this article, or claim that may be made by its manufacturer, is not guaranteed or endorsed by the publisher.
Footnotes
1An online calculator for Bayes factor analyses can be found at http://pcl.missouri.edu/bayesfactor.
References
Buck, L. E., Young, M. K., and Bodenheimer, B. (2018). A Comparison of Distance Estimation in HMD-Based Virtual Environments with Different HMD-Based Conditions. ACM Trans. Appl. Percept. 15, 1–15. doi:10.1145/3196885
Creem-Regehr, S. H., Stefanucci, J. K., and Thompson, W. B. (2015). “Perceiving Absolute Scale in Virtual Environments: How Theory and Application Have Mutually Informed the Role of Body-Based Perception,” in Psychology of Learning and Motivation. Editor B. H. ROSS Vol 62 of Psychology of Learning and Motivation (Academic Press), 195–224. doi:10.1016/bs.plm.2014.09.006
Creem-Regehr, S. H., Willemsen, P., Gooch, A. A., and Thompson, W. B. (2005). The Influence of Restricted Viewing Conditions on Egocentric Distance Perception: Implications for Real and Virtual Indoor Environments. Perception 34, 191–204. doi:10.1068/p5144
Feldstein, I. T., Kölsch, F. M., and Konrad, R. (2020). Egocentric Distance Perception: A Comparative Study Investigating Differences between Real and Virtual Environments. Perception 49, 940–967. doi:10.1177/0301006620951997
Fidopiastis, C., Fuhrman, C., Meyer, C., and Rolland, J. (2005). Methodology for the Iterative Evaluation of Prototype Head-Mounted Displays in Virtual Environments: Visual Acuity Metrics. Presence: Teleoperators. Virtual Environ. 14, 550–562. doi:10.1162/105474605774918697
Fox, J., Arena, D., and Bailenson, J. N. (2009). Virtual Reality. J. Media Psychol. 21, 95–113. doi:10.1027/1864-1105.21.3.95
Gagnon, H. C., Rosales, C. S., Mileris, R., Stefanucci, J. K., Creem-Regehr, S. H., and Bodenheimer, R. E. (2021). Estimating Distances in Action Space in Augmented Reality. ACM Trans. Appl. Percept. 18, 1–16. doi:10.1145/3449067
Grechkin, T. Y., Nguyen, T. D., Plumert, J. M., Cremer, J. F., and Kearney, J. K. (2010). How Does Presentation Method and Measurement Protocol Affect Distance Estimation in Real and Virtual Environments? ACM Trans. Appl. Perception (Tap) 7, 26. doi:10.1145/1823738.1823744
Jones, J. A., Suma, E. A., Krum, D. M., and Bolas, M. (2012). “Comparability of Narrow and Wide Field-Of-View Head-Mounted Displays for Medium-Field Distance Judgments,” in Proceedings of the ACM Symposium on Applied Perception (ACM), Los Angeles, CA, August 3–4, 2012, 119.
Kelly, J. W., Cherep, L. A., and Siegel, Z. D. (2017). Perceived Space in the Htc Vive. ACM Trans. Appl. Percept. 15 (2), 1–16. doi:10.1145/3106155
Knapp, J. M., and Loomis, J. M. (2004). Limited Field of View of Head-Mounted Displays Is Not the Cause of Distance Underestimation in Virtual Environments. Presence: Teleoperators. Virtual Environ. 13, 572–577. doi:10.1162/1054746042545238
Langbehn, E., Raupp, T., Bruder, G., Steinicke, F., Bolte, B., and Lappe, M. (2016). “Visual Blur in Immersive Virtual Environments: Does Depth of Field or Motion Blur Affect Distance and Speed Estimation?” in Proceedings of the 22nd ACM Conference on Virtual Reality Software and Technology, Munich, Germany, November 2019, 241–250. doi:10.1145/2993369.2993379
Li, B., Walker, J., and Kuhl, S. A. (2018). The Effects of Peripheral Vision and Light Stimulation on Distance Judgments through Hmds. ACM Trans. Appl. Percept. 15 (12), 1–14. doi:10.1145/3165286
Lin, Q., Xie, X., Erdemir, A., Narasimham, G., McNamara, T. P., Rieser, J., et al. (2011). “Egocentric Distance Perception in Real and HMD-Based Virtual Environments,” in APGV ’11:Proceedings of the ACM SIGGRAPH Symposium on Applied Perception in Graphics and Visualization, Toulouse, France, August 27–28, 2011 (New York, NY, USA: Association for Computing Machinery), 75–82. doi:10.1145/2077451.2077465
Masnadi, S., Pfeil, K. P., Sera-Josef, J. V. T., and LaViola, J. J. (2021). “Field of View Effect on Distance Perception in Virtual Reality,” in IEEE Conference on Virtual Reality and 3D User Interfaces Abstracts and Workshops (VRW), Lisbon, Portugal, March 27–April 1, 2021, 542–543. doi:10.1109/vrw52623.2021.00153
Mohler, B. J., Bülthoff, H. H., Thompson, W. B., and Creem-Regehr, S. H. (2008). “A Full-Body Avatar Improves Egocentric Distance Judgments in an Immersive Virtual Environment,” in Proceedings of the 5th Symposium on Applied Perception in Graphics and Visualization, Los Angeles, CA, August 9–10, 2008, 194.
Peer, A., and Ponto, K. (2017). “Evaluating Perceived Distance Measures in Room-Scale Spaces Using Consumer-Grade Head Mounted Displays,” in 2017 IEEE symposium on 3d user interfaces (3dui), Los Angeles, CA, March 18–19, 2017 (IEEE), 83–86.
Peer, A., and Ponto, K. (2020). “Measuring Visual Acuity and Stereo Accuracy as Mediated by Immersive Displays,” in 2020 IEEE Conference on Virtual Reality and 3D User Interfaces Abstracts and Workshops (VRW), Atlanta, GA, March 22–26, 2020 (IEEE), 219–223.
Peillard, E., Itoh, Y., Moreau, G., Normand, J.-M., Lécuyer, A., and Argelaguet, F. (2020). “Can Retinal Projection Displays Improve Spatial Perception in Augmented Reality,” in 2020 IEEE International Symposium on Mixed and Augmented Reality (ISMAR), Porto de Galinhas, Brazil, November 9–13, 2020 (IEEE), 80–89.
Phillips, L., Ries, B., Kaeding, M., and Interrante, V. (2010). “Avatar Self-Embodiment Enhances Distance Perception Accuracy in Non-photorealistic Immersive Virtual Environments,” in 2010 IEEE virtual reality conference (VR), Boston, MA, March 20–24, 2010 (IEEE), 115–1148.
Renner, R. S., Velichkovsky, B. M., and Helmert, J. R. (2013). The Perception of Egocentric Distances in Virtual Environments — A Review. ACM Comput. Surv. 46 (23), 1–23. doi:10.1145/2543581.2543590
Rouder, J. N., Speckman, P. L., Sun, D., Morey, R. D., and Iverson, G. (2009). Bayesian T Tests for Accepting and Rejecting the Null Hypothesis. Psychon. Bull. Rev. 16, 225–237. doi:10.3758/pbr.16.2.225
Sinai, M. J., Ooi, T. L., and He, Z. J. (1998). Terrain Influences the Accurate Judgement of Distance. Nature 395, 497–500. doi:10.1038/26747
Thompson, W. B., Willemsen, P., Gooch, A. A., Creem-Regehr, S. H., Loomis, J. M., and Beall, A. C. (2004). Does the Quality of the Computer Graphics Matter when Judging Distances in Visually Immersive Environments? Presence: Teleoperators. Virtual Environ. 13, 560–571. doi:10.1162/1054746042545292
Willemsen, P., Colton, M. B., Creem-Regehr, S. H., and Thompson, W. B. (2009). The Effects of Head-Mounted Display Mechanical Properties and Field of View on Distance Judgments in Virtual Environments. ACM Trans. Appl. Percept. 6 (8), 1–14. doi:10.1145/1498700.1498702
Willemsen, P., and Gooch, A. A. (2002). “Perceived Egocentric Distances in Real, Image-Based, and Traditional Virtual Environments,” in Proceedings IEEE Virtual Reality 2002 (IEEE), Orlando, FL, March 24–28, 2002, 275–276.
Witmer, B. G., and Sadowski, W. J. (1998). Nonvisually Guided Locomotion to a Previously Viewed Target in Real and Virtual Environments. Hum. Factors 40, 478–488. doi:10.1518/001872098779591340
Wright, R. H. (1995). Virtual Reality Psychophysics: Forward and Lateral Distance, Height, and Speed Perceptions with a Wide-Angle Helmet Display. Tech.report., 1025. Alexandria Va: Army Research Institute For The Behavioral And Social Sciences.
Wu, B., Ooi, T. L., and He, Z. J. (2004). Perceiving Distance Accurately by a Directional Process of Integrating Ground Information. Nature 428, 73–77. doi:10.1038/nature02350
Young, M. K., Gaylor, G. B., Andrus, S. M., and Bodenheimer, B. (2014). “A Comparison of Two Cost-Differentiated Virtual Reality Systems for Perception and Action Tasks,” in Proceedings of the ACM Symposium on Applied Perception (ACM), Vancouver, BC, August 8–9, 2014, 83–90.
Keywords: virtual reality, perception, distance compression, head-mounted displays, human-computer interaction
Citation: Buck L, Paris R and Bodenheimer B (2021) Distance Compression in the HTC Vive Pro: A Quick Revisitation of Resolution. Front. Virtual Real. 2:728667. doi: 10.3389/frvir.2021.728667
Received: 21 June 2021; Accepted: 17 November 2021;
Published: 07 December 2021.
Edited by:
J. Adam Jones, University of Mississippi, United StatesReviewed by:
Kyle John Johnsen, University of Georgia, United StatesKevin Ponto, University of Wisconsin-Madison, United States
Copyright © 2021 Buck, Paris and Bodenheimer. This is an open-access article distributed under the terms of the Creative Commons Attribution License (CC BY). The use, distribution or reproduction in other forums is permitted, provided the original author(s) and the copyright owner(s) are credited and that the original publication in this journal is cited, in accordance with accepted academic practice. No use, distribution or reproduction is permitted which does not comply with these terms.
*Correspondence: Lauren Buck, lauren.e.buck.1@vanderbilt.edu