- Academic Medical Center, Department of Psychiatry, University of Amsterdam, Amsterdam, Netherlands
Background: Virtual reality (VR) has been previously shown as a means to mitigate acute pain. The critical parameters involved in the clinical efficacy of mitigating acute pain from different perspectives remains unknown. This study attempted to further deconstruct the critical parameters involved in mitigating acute pain by investigating whether affective and physiological responses to painful stimuli differed between a first and a third person perspective in virtual reality.
Methods: Two conditions were compared in a repeated-measures within subject study design for 17 healthy participants: First person perspective (i.e., where participants experienced their bodies from an anatomical and egocentric perspective) and third person perspective (i.e., where participants experienced their bodies from an anatomical perspective from across the room). The participants received noxious electrical stimulation at pseudorandom intervals and anatomical locations during both conditions. Physiological stress responses were measured by means of electrocardiography (ECG) and impedance cardiography (ICG). Subjective scores measuring tension, pain, anger, and fear were reported after every block sequence.
Results: There were no significant differences in physiological stress responses between conditions. However, the participants reported significantly higher tension during the third person condition.
Conclusion: Relative to a third person perspective, there are no distinct physiological benefits to inducing a first person perspective to mitigate physiological stress responses to acute pain in healthy individuals. However, there may be additional clinical benefits for doing so in specific clinical populations that have shown to benefit from relaxation techniques. Further research is needed in order to refine the clinical utility of different perspectives during virtual reality immersions that serve to act as a non-pharmacological analgesic during acute pain.
Introduction
Pain can be defined as an unpleasant sensory and emotional experience associated with, or resembling that associated with, actual or potential tissue damage (IASP, 2017). Currently, acute pain is largely relieved using pain-medication, however, these analgesics also have unwanted side effects which often requires additional, complicated, considerations for their administration (Alford et al., 2006). In order to better address these concerns, there has recently been an increased demand for non-invasive methods that have the potential to be self-administered. One candidate that is being increasingly explored in acute pain research is virtual reality. To date, a common class of techniques used to mitigate the experience of acute pain is by inducing a body illusion.
A body illusion can be defined as any event where an individual’s physical body has been perceptually modified in order to gain some further understanding of their own-body perception. The ability to modulate acute pain sensations have been explored in several different classes of body illusions; most commonly in body distortion (Mancini et al., 2011; Martini et al., 2013; Romano and Maravita, 2014; Matamala-Gomez et al., 2020) and body ownership (Longo et al., 2009; Hänsell et al., 2011; Martini et al., 2015) paradigms using both virtuality technologies and non-virtual (ex. mirrors) instruments. The use of virtuality technologies is becoming more ubiquitous in body illusion research due to the enhanced ability of being able to manipulate information in ways that are otherwise not possible. An example of such an advantage can be seen in another emerging class of body illusions known as out of body experiences (OBE), whereby the perceived location of the self is separated from the actual location of the physical self (Ehrsson, 2007; Lenggenhager et al., 2007; Blom et al., 2014; Pfeiffer et al., 2014). These illusions involve the individual viewing a third person perspective of themselves from a first person perspective.
Body illusions, regardless of class, are induced and sustained through the use of multisensory signal integration. The dynamics between this multisensory interplay of manipulating the sense of body ownership, agency, and the experience of acute pain still remains unclear for several reasons. From a more fundamental stance, it is still not clear what role perspective plays in facilitating both the affective and physiological responses to a noxious stimulus. Moreover, there is still a lack of clarity surrounding the understanding of autonomic responses to experimentally induced painful stimulation. Results from a previous review that sought to clarify the physiological component of the acute pain response to experimentally induced painful stimulation identified several important suggestions for future research which included how cognitive and affective constructs are under-investigated and warrant more attention and that studies should include measures that specifically assess both sympathetic and parasympathetic responses (Kyle and McNeil, 2014).
Currently, one of the major competing theories attempting to explain the analgesic potential of virtual reality postulates that virtual reality is able to effectively distract an individual from noxious stimuli (Hoffman et al., 2000; Hoffman et al., 2001; Schneider et al., 2004; Gershon et al., 2004; Hoffman et al., 2011). Furthermore, it has been hypothesized that the more attention demanding the virtual immersion is, the better it is for reducing pain (Lier et al., 2020). The purpose of this study aims to further deconstruct the basis of body illusions by further examining how perspective contributes to the affective and physiological experience of acute pain. In order to test this, we compared a first and third person perspective of the self during virtual immersion while administering noxious electrical stimuli. In the first person condition, there was visuomotor congruency between the visual information of the participants’ virtual embodiment and their bodily movements which they were asked to attend to (Figure 1A). However in the third person condition, the participants still experienced their bodily movements from an anatomical perspective but visually perceived themselves from a front facing profile from across the room. Therefore in this condition, there was incongruent, conflicting, visuomotor information between the visual information of their virtual embodiment and their bodily movements which they were asked to attend to (Figure 1B). One of the main differences with the extant literature on the effect of interaction in virtual environments (VE) is that we did not provide any visual cues in our VEs that could provide expectancy effects that could interfere or interact with the perspective, as previous literature has shown that environmental interaction compared to no interaction is significantly more analgesic (Lier et al., 2020). In line with the theory of attentional demand (Hoffman et al., 2019), we hypothesized that the incongruent visuomotor information experienced during the third person perspective would demand an increase in attentional capacity and therefore further distract the individuals from the painful stimuli, resulting in a relatively lower physiological stress response and lower subjective ratings compared to the first person perspective.
Methods
Participants
We recruited 17 healthy adults to participate in this research. Testing took place in the Psychiatry Department in the Amsterdam University Medical Centre (Amterdam UMC) (Amsterdam, Netherlands). Participants were included on the basis of having no previous DSM-V diagnosis of a mental disorder and no previous neurological diagnosis or complaints of having chronic pain or somatosensory hypersensitivity. This study was reviewed and approved by the medical ethical committee of the Amsterdam UMC. The participants provided their written informed consent in order to participate in this study. This research received no specific grant from any funding agency in the public, commercial, or not-for-profit sectors.
Virtual Environment and Equipment
The VE was presented on an HMD (Oculus Rift DK2) from both a first person and fixed third person perspective. The VE in the first person perspective depicted a video image of what the participants saw from their natural perspective, which consisted of them sitting in a chair in an empty room with white walls and a dark floor. The VE in the third person perspective depicted the individuals’ front facing profile as they sat on a chair in an empty room with white walls and a dark floor. The minimalistic design of these VEs allowed us to better study the effects of perspective on distraction by removing unnecessary environmental objects and interactions that could have further impacted the participants’ experience. In both conditions, the participants saw their movements from an anatomical reference. The VEs were created from a video feed which came from a stereoscopic camera (Zed Mini) that was mounted either on the front of the HMD or on a camera tripod in front of the individual. The VE ran within a game engine (Unity 3D).
Physiological Measurements
We measured three physiological indicators during the exposure to assess the participants’ stress response via autonomic nervous system (ANS) activity by means of electrocardiography (ECG) and impedance cardiography (ICG) using an ambulatory physiological recording device (VU-AMS, VU University). We adhered to the operational use of the device according to the manual (Vrije Universiteit, 2019). Our signals of interest included heart rate (HR), respiratory sinus arrhythmia (RSA), and pre-ejection period (PEP). HR is an indicator of combined parasympathetic and sympathetic control (Akselrod et al., 1981). RSA is a naturally occurring variation in HR during a respiration cycle and has been shown to be a reliable way to assess parasympathetic control (Katona and Jih, 1975). PEP is the time interval between electrical stimulation of the ventricles and the opening of the aortic values, which measures sympathetic control of cardiac activity (Newlin and Levenson, 1979; Berntson et al., 2004). VUDAMS software version 4.0 was used to pre-process the physiological data as described in the manual (Vrije Universiteit, 2019). After the data was visually inspected and manually corrected by an independent researcher, we could derive the inter beat interval (IBI) times series and respiration signals from the ECG and ICG signals. The different periods of the script were labeled using the visual interface within the DAMS environment. Heart rate (HR) was attained directly from the IBI time series. Respiratory sinus arrhythmia (RSA) was obtained by combining the reparation signals with the IBI time series using the peak valley method. For our analysis, we are reporting the “RSA-zero” mean variable, meaning the RSA value was automatically set to be zero for breaths that the software detected as having an invalid RSA. In order to be as conservative as possible, we discarded a dataset if more than 50% of the data was missing. This could be due to hardware malfunction or being manually set to missing because the researchers and independent researcher collectively agreed that a segment of data could not be reliably analyzed. Pre-ejection period (PEP) was calculated as the time from the onset of the Q-wave in the ECG to the opening of the aortic valves, selected by the B-point in the ICG signal.
Pain Stimuli
Four electrodes from a stimulation device (ENERGY Light) were positioned on the participants’ legs. Electrodes were placed above the knee on the right leg, below the knee on the right leg, above the knee on the left leg, and below the knee on the left leg. Before beginning the test, each of the individual current intensities were adjusted on an individual-to-individual as well as a region-by-region basis to be experienced as moderately painful. This was determined by gradually increasing the intensity of the current until the individual reported a moderate level of discomfort. These stimuli were administered remotely, with the researcher being out of view from the participant, and silently. This approach helped us to mitigate any potential effects due to stimulus expectancy.
Verbal Likert Scale
Subjective levels of pain were obtained by administering a verbal Likert scale (VLS). The range for the scaled questions was from 1–5 with (1 not at all—5 extreme). The scaled questions addressed how tense they were, how much pain they were in, how fearful they were, and how angry they were.
Procedure
This study consisted of two conditions, a first person perspective and a third person perspective, using a repeated measures within-subjects design. For a complete overview of the experimental design, please see Figure 2, which has been adopted and modified from the method section of previous research (Turbyne et al., 2021). Before starting either condition, the participants underwent a relaxation phase which presented a series of five different neutral images taken from a validated database (Lang et al., 2008) which were adopted for use in an HMD at a rate of one stimulus per minute. We synchronized the onset of the physiological recordings with the presentation of these images in order to prevent false detections, which established the baseline for our analysis. This phase was directly followed by an immersion phase which allowed the participants to acclimate themselves within the virtual environment in which they were encouraged to explore their bodies by looking at themselves and moving their limbs while being seated. This phase lasted for a total of 5 min. After this phase we initiated either the first or third person condition. We presented a total of three sequences per condition, each of which contained four blocks that corresponded to an anatomical region. The anatomical regions were: A) above the left knee, B) below the left knee, C) above the right knee, and D) below the right knee. Each block within every sequence was pseudo-randomly presented, such that each block was represented in that sequence and that no block could not be repeated back-to-back either in that sequence or the subsequent sequence. The inter-sequence interval was set to 2 min, which allowed us to mitigate any potential carry over effects from the previous sequence, as well as allow us ample time to administer a VLS. Each block contained 6 stimuli, with each stimulus lasting 0.2 ms. The inter-stimulus interval was set to 20 s, which allowed us to obtain a clear and analyzable physiological signal. After completing the first condition, the participants removed the HMD before beginning the subsequent condition. The inter-condition interval was variable amongst subjects, with a maximum period of 30 min. The purpose of the inter-condition interval was to ensure that the participant did not experience any simulation sickness before beginning the subsequent condition. The conditions were counterbalanced between participants. The verbal explanation to the participants prior to starting each condition was the following: “During this condition, I will stimulate different areas of your legs. Please try to visually focus on yourself as I do this. You are allowed to move around freely while remaining seated”.
Statistical Analysis
We conducted a generalized estimating equation (GEE) analysis to examine the between condition differences in physiological measurements from baseline to pain provocation (ΔHR, ΔRSA, ΔPEP) as well as VLS scores. GEE models can be used to analyze repeated measures data with binary, ordinal, or continuous outcomes (Zeger et al., 1988; Liang and Zeger, 1993). For our GEE analysis we selected an exchangeable correlation structure. The model’s fixed effects consisted of main effects for stimulus region and perspective, as well as their interaction. For the physiological parameters ∆HR, ∆RSA, and ∆PEP, a Gaussian link function was specified. For the ordinal VLS scores, a logistic function was used. SPSS software version 26 was used to perform the GEE analysis. We Bonferroni corrected the obtained p-values for the number of dependent variables included in the repeated-measures GEE models; we multiplied p-values by three for the physiological indicators and by four for the VLS data.
Results
Physiological data was excluded for six participants due to large sections of missing data that was found to be caused by the electrodes losing contact sporadically throughout the experiment, leaving 11 subjects for analysis. As shown in Table 1, the GEE analysis revealed that perspective and stimulus region were not statistically significant predictors for ΔPEP [(perspective (Wald χ = 1.115, df = 1, p = 0.291)] [stimulus region (Wald χ = 3.692, df = 3, p = 0.297)] [perspective*stimulus region (Wald χ = 5.294, df = 3, p = 0.152)] or ΔRSA [[perspective (Wald χ = 1.873, df = 1, p = 0.171)] [stimulus region (Wald χ = 7.170, df = 3, p = 0.067)] [perspective*stimulus region (Wald χ = 4.216, df = 3, p = 0.239)]. However, for ΔHR, while there were no significant main effects for perspective [Wald χ = 3.115, df = 1, p = 0.078] or an interaction effect between perspective and stimulus region [Wald χ = 2.291, df = 3, p = 0.514], there was a significant main effect for stimulus region (Wald χ = 16.909, df = 3, p = 0.001, Bonferroni-corrected p = 0.003) (Figure 3). The main effect of perspective for ∆HR, while not significant, was approaching significance. In order to test if we had enough power, we performed a post-hoc sensitivity analysis using G*Power for repeated measures with the following settings: alpha = 0.0166, power = 0.80, sample size = 11, number of measurements = 12, and a correlation among repeated measures = 0.357. We were able to detect only large effects (Cohen’s d = 0.65).
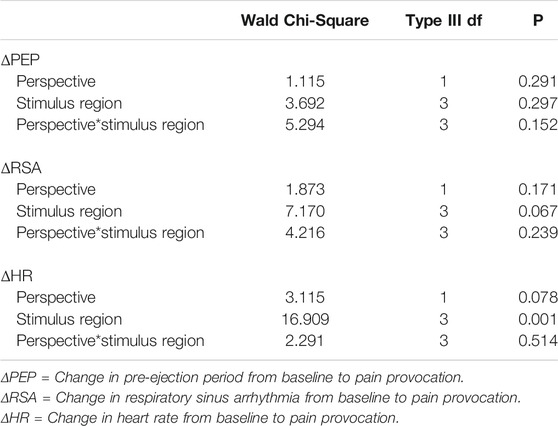
TABLE 1. Results of exchangeable correlation generalized estimating equation for physiological measurements from baseline to pain provocation.
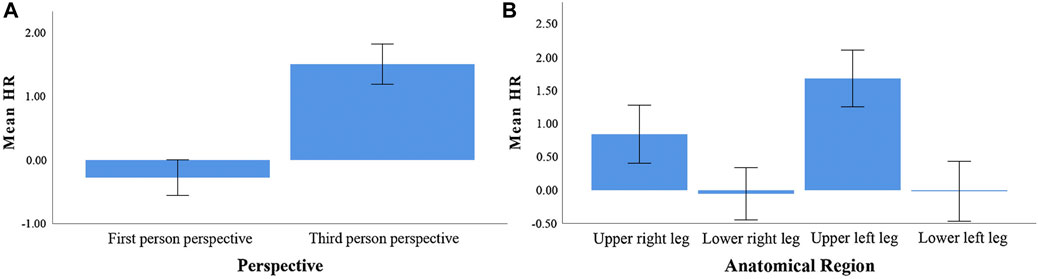
FIGURE 3. Mean HR for perspective (A) shows that third person perspective scores higher, which did not reach significance (p = 0.078). Anatomical region (B) showed a main effect with higher HR for upper stimulation region, which was significant (p = 0.001). Error bars are 95% CI.
The mean ratings for the individual questionnaire items are shown in Figure 4. When comparing means for VLS scores between conditions, pain, tension, anxiety, and anger were 2.0 (SD = 0.935), 1.82 (SD = 1.158), 1.48 (SD = 0.972), and 1.67 (SD = 1.051) during the first person condition and 2.12 (SD = 0.927), 2.18 (SD = 1.103), 1.61 (SD = 0.788), 1.55 (SD = 0.794) during the third person condition, respectively. As shown in Table 2, perspective and stimulus region were not statistically significant predictors for pain [perspective (Wald χ = 0.271, df = 1, p = 0.602)] [stimulus region (Wald χ = 2.409, df = 1, p = 0.121)] [perspective*stimulus region (Wald χ = 2.042, df = 1, p = 0.153)], anxiety [perspective (Wald χ = 1.682, df = 1, p = 0.195)] [stimulus region (Wald χ = 0.006, df = 1, p = 0.938)] [perspective*stimulus region (Wald χ = 0.147, df = 1, p = 0.702)], or anger [perspective (Wald χ = 0.085, df = 1, p = 0.771)] [stimulus region (Wald χ = 0.618, df = 1, p = 0.432)] [perspective*stimulus region (Wald χ = 0.621, df = 1, p = 0.431)]. However, for tension while there were no significant main effects for stimulus region (Wald χ = 0.025, df = 1, p = 0.873) or an interaction effect between perspective and stimulus region [Wald χ = 1.073, df = 1, p = 0.300], there was a significant main effect for perspective [Wald χ] = 6.449, df = 1, p = 0.011, Bonferroni-corrected p = 0.044).
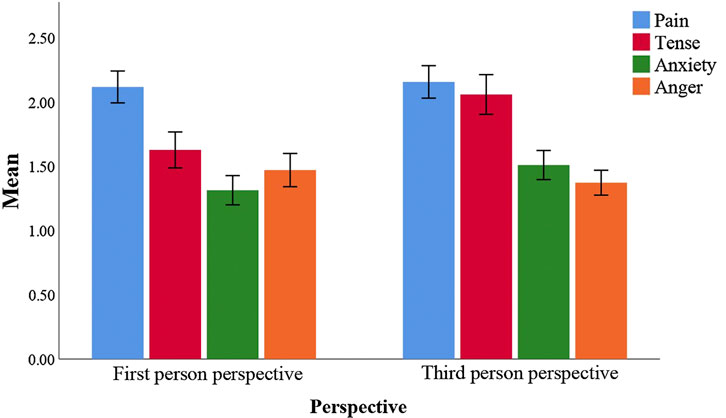
FIGURE 4. Mean VLS scores for the first person (left) and third person (right) conditions. Error bars are 95% CI.
Discussion
The goal of this study was to investigate whether perspective can differentially effect the affective and physiological responses to acute pain in virtual reality. This was investigated by comparing a modified body representation from a third person perspective, which consisted of incongruent visuomotor information between the visual information of the participants’ virtual embodiment and their bodily movements, with an unmodified body representation from a first person perspective. We hypothesized that the third person condition would elicit a relatively lower physiological stress response and subjective report scores compared to the first person condition.
The results from our exploratory analyses showed no differences in physiological stress responses between perspectives or any interaction effects between perspectives and stimulus regions. However, we did find a significant main effect for stimulus region in ∆HR. This measure alone is not informative enough to be able to determine whether or not there is a stress response or what the magnitude of a potential stress response may be. HR is used more-so as a secondary measure to determine whether or not there is a stress response due to the fact that it is both a sensitive measure and that it only reflects the combined sympathetic and parasympathetic activity. Because we were unable to observe any significance for either PEP or RSA, this leaves us unable to determine what this difference in HR actually reflects. Arguably, this effect could be stronger if we added repetitions, per subject, to the data in order to increase the power, although this would likely be ineffective due to the fact that our within-effect already has eight repeats, being four repeats per condition times both perspectives. Adding additional repetitions would have also expanded the total length of the experiment, including setup time, to over 4 h which is not feasible from a practical stance, while also inducing trend effects from such a lengthy recording. In terms of affective responses, participants indicated low mean subjective scores for both conditions. However, individuals experienced significantly more tension in the third-person perspective compared to the first-person perspective. These results highlight the complex relationship of how specific states of emotion experienced during acute pain influence the underlying physiology of its perception. Evidence from a systematic review has shown how relaxation can benefit pain outcomes (Kwekkeboom and Gretarsdottir, 2006). Furthermore, previous meta-analytic evidence suggests that relaxation techniques are effectively able to reduce pain in cancer patients with acute pain (Luebbert et al., 2001). Therefore, while reduced tension had no effect in the healthy population we tested, the use of a first person perspective may be more advantageous to mitigate acute pain in certain clinical populations that have been found to further benefit from relaxation techniques.
In the current research we were unable to observe whether tension, i.e., mental stress, affected how the individuals visually attended to each condition. Previous research has shown that focused attention can mitigate pain (Roelofs et al., 2004). This could be further explored through the use of eye tracking during immersion, as previous research has found that eye tracking can be used to assess involuntary attentional consequences of pain (Schmidt et al., 2018). Therefore, future research with a similar design should seek to use virtuality headsets with integrated eye tracking in order to better determine the relationship between perspective, tension/relaxation, and the physiological stress response to acute pain.
Another explanation for why we did not find any significant effects of perspective for physiological stress responses could be due to our noxious stimulus selection and administration. While previous research has suggested that both heat and electrical noxious stimuli have been found to be comparable to each other in terms of their efficacy to elicit an acute pain response (Jiang et al., 2019) individual differences towards different types of painful stimuli will invariably occur. In our paradigm, four out of the seventeen participants had to max out the stimulation intensity of the device in order to achieve a self-reported moderate level of pain. It is reasonable to suggest that, due to individual differences, people naturally separate themselves into being relatively higher and lower responders to specific types of noxious stimulation. In order to better understand the potential analgesic effects of perspective, researchers should seek to administer tailored stimuli on an individual-to-individual basis. By integrating different types of noxious stimuli with different intensity levels into single research designs, researchers can begin to foster a classification system specific to different perspectives.
It should also be mentioned that while our results may be due to a small sample size, they may also be due to the subtlety of our manipulation. The only physiological indicator that was approaching significance for a main of effect of perspective was ∆HR and we were only able to establish large effect sizes in our sensitivity analysis. In a typical sense, this is insufficient, as it is desirable to also be able to detect other effect sizes. Contrastingly, clinical utility is contingent on being able to detect large effect sizes. It is possible that the absence of a difference in perspective could be due to allowing participants to freely initiate the movements of their own body while remaining seated. Because of this, individuals tended to move less over the duration of the experiment which may have diminished the strength of the manipulation by reducing the frequency of body ownership updates on body representation. Therefore, future research should seek to examine whether a stronger physiological/affective response can be observed if participants initiate body movements more frequently, which can be tested by having the participants perform pre-specified movements at specific time intervals.
Finally, we did not explicitly evaluate body ownership via subjective reporting (i.e., do you believe that this body is your own?). While our main intention was to assess the effect of perspective, which by definition implies modifying body representation, we are unable to conclusively determine whether or not our observations were due to perspective alone or if they were also influenced by the possibility that certain individuals felt that they did not own the body they were perceiving. As such, future research that seeks to further disentangle the effects of perspective on acute pain should include measures that directly assess body ownership.
Conclusion
There are no significant differences between perspectives or anatomical regions for physiological stress responses to noxious electrical stimulation that could be observed in the current study. Despite this, participants were significantly more tense during the third person perspective. Our findings reveal that there are no clinical benefits for modifying a healthy individual’s perspective during the experience of acute pain. However, there may still be clinical benefits for modifying an individuals perspective for specific patient populations that have been found to benefit from relaxation techniques. Our findings outline specific considerations that future research should consider in order to more fully understand the extent to which perspective may influence affective and physiological responses to acute pain in virtual reality pain reduction paradigms.
Data Availability Statement
The raw data supporting the conclusions of this article will be made available by the authors upon reasonable request.
Ethics Statement
The studies involving human participants were reviewed and approved by METC Amsterdam UMC. The patients/participants provided their written informed consent to participate in this study.
Author Contributions
CT: Conception and design, data acquisition, data analysis, interpretation of the data, drafting the original article, editing, revision. PK: Revising original article for important intellectual content. DS: Data analysis, interpretation of the data. DD: Final approval of the version published, accountable for the article to ensure that all questions regarding the accuracy and/or integrity of the study are investigated and resolved.
Conflict of Interest
The authors declare that the research was conducted in the absence of any commercial or financial relationships that could be construed as a potential conflict of interest.
References
Akselrod, S., Gordon, D., Ubel, F., Shannon, D., Berger, A., and Cohen, R. (1981). Power Spectrum Analysis of Heart Rate Fluctuation: a Quantitative Probe of Beat-To-Beat Cardiovascular Control. Science 213 (4504), 220–222. doi:10.1126/science.6166045
Alford, D. P., Compton, P., and Samet, J. H. (2006). Acute Pain Management for Patients Receiving Maintenance Methadone or Buprenorphine Therapy. Ann. Intern. Med. 144 (2), 127–134. doi:10.7326/0003-4819-144-2-200601170-00010
Berntson, G. G., Lozano, D. L., Chen, Y.-J., and Cacioppo, J. T. (2004). Where to Q in PEP. Psychophysiology 41 (2), 333–337. doi:10.1111/j.1469-8986.2004.00156.x
Blom, K. J., Arroyo-Palacios, J., and Slater, M. (2014). The Effects of Rotating the Self Out of the Body in the Full Virtual Body Ownership Illusion. Perception 43 (4), 275–294. doi:10.1068/p7618
Ehrsson, H. H. (2007). The Experimental Induction of Out-Of-Body Experiences. Science 317 (5841), 1048. doi:10.1126/science.1142175
Gershon, J., Zimand, E., Pickering, M., Rothbaum, B. O., and Hodges, L. (2004). A Pilot and Feasibility Study of Virtual Reality as a Distraction for Children with Cancer. J. Am. Acad. Child Adolesc. Psychiatry 43 (10), 1243–1249. doi:10.1097/01.chi.0000135621.23145.05
Hänsell, A., Lenggenhagerl, B., Känell, R., Curatolol, M., and Blankel, O. (2011). Seeing and Identifying with a Virtual Body Decreases Pain Perception. Eur. J. pain 15 (8), 874–879. doi:10.1016/j.ejpain.2011.03.013
Hoffman, H. G., Chambers, G. T., Meyer, W. J., Arceneaux, L. L., Russell, W. J., Seibel, E. J., et al. (2011). Virtual Reality as an Adjunctive Non-pharmacologic Analgesic for Acute Burn Pain during Medical Procedures. Ann. Behav. Med. 41 (2), 183–191. doi:10.1007/s12160-010-9248-7
Hoffman, H. G., Doctor, J. N., Patterson, D. R., Carrougher, G. J., and Furness, T. A. (2000). Virtual Reality as an Adjunctive Pain Control during Burn Wound Care in Adolescent Patients. Pain 85 (1–2), 305–309. doi:10.1016/s0304-3959(99)00275-4
Hoffman, H. G., Garcia-Palacios, A., Patterson, D. R., Jensen, M., Furness, T., and Ammons, W. F. (2001). The Effectiveness of Virtual Reality for Dental Pain Control: A Case Study. CyberPsychology Behav. 4 (4), 527–535. doi:10.1089/109493101750527088
Hoffman, H. G., Meyer, W. J., Drever, S. A., Soltani, M., Atzori, B., Herrero, R., et al. (2019). “Virtual Reality Distraction to Help Control Acute Pain during Medical Procedures,” in Virtual Reality for Psychological and Neurocognitive Interventions. Virtual Reality Technologies for Health and Clinical Applications. Editors A. Rizzo, and S. Bouchard (New York, NY: Springer), 195–208. doi:10.1007/978-1-4939-9482-3_8
IASP (2017). IASP Terminology. Available at: https://www.iasp-pain.org/Education/Content.aspx?ItemNumber=1698#Pain (Accessed January 28, 2021).
Jiang, M., Mieronkoski, R., Syrjälä, E., Anzanpour, A., Terävä, V., Rahmani, A. M., et al. (2019). Acute Pain Intensity Monitoring with the Classification of Multiple Physiological Parameters. J. Clin. Monit. Comput. 33 (3), 493–507. doi:10.1007/s10877-018-0174-8
Katona, P. G., and Jih, F. (1975). Respiratory Sinus Arrhythmia: Noninvasive Measure of Parasympathetic Cardiac Control. J. Appl. Physiol. 39 (5), 801–805. doi:10.1152/jappl.1975.39.5.801
Kwekkeboom, K. L., and Gretarsdottir, E. (2006). Systematic Review of Relaxation Interventions for Pain. J. Nurs. Scholarship 38 (3), 269–277. doi:10.1111/j.1547-5069.2006.00113.x
Kyle, B. N., and McNeil, D. W. (2014). Autonomic Arousal and Experimentally Induced Pain: a Critical Review of the Literature. Pain Res. Manage. 19 (3), 159–167. doi:10.1155/2014/536859
Lang, P. J., Bradley, M. M., and Cuthbert, B. N. (2008). International Affective Picture System (IAPS): Affective Ratings of Pictures and Instruction Manual. Gainesville, FL: University of Florida.
Lenggenhager, B., Tadi, T., Metzinger, T., and Blanke, O. (2007). Video Ergo Sum: Manipulating Bodily Self-Consciousness. Science 317 (5841), 1096–1099. doi:10.1126/science.1143439
Liang, K. Y., and Zeger, S. L. (1993). Regression Analysis for Correlated Data. Annu. Rev. Public Health 14, 43–68. doi:10.1146/annurev.pu.14.050193.000355
Lier, E. J., Oosterman, J. M., Assmann, R., de Vries, M., and van Goor, H. (2020). The Effect of Virtual Reality on Evoked Potentials Following Painful Electrical Stimuli and Subjective Pain. Sci. Rep. 10. doi:10.1038/s41598-020-66035-4
Longo, M. R., Betti, V., Aglioti, S. M., and Haggard, P. (2009). Visually Induced Analgesia: Seeing the Body Reduces Pain. J. Neurosci. 29 (39), 12125–12130. doi:10.1523/jneurosci.3072-09.2009
Luebbert, K., Dahme, B., and Hasenbring, M. (2001). The Effectiveness of Relaxation Training in Reducing Treatment-Related Symptoms and Improving Emotional Adjustment in Acute Non-surgical Cancer Treatment: a Meta-Analytical Review. Psycho-oncology 10 (6), 490–502. doi:10.1002/pon.537
Mancini, F., Longo, M. R., Kammers, M. P. M., and Haggard, P. (2011). Visual Distortion of Body Size Modulates Pain Perception. Psychol. Sci. 22 (3), 325–330. doi:10.1177/0956797611398496
Martini, M., Kilteni, K., Maselli, A., and Sanchez-Vives, M. V. (2015). The Body Fades Away: Investigating the Effects of Transparency of an Embodied Virtual Body on Pain Threshold and Body Ownership. Scientific Rep. 5, 13948. doi:10.1038/srep13948
Martini, M., Pérez Marcos, D., and Sanchez-Vives, M. V. (2013). What Color Is My Arm? Changes in Skin Color of an Embodied Virtual Arm Modulates Pain Threshold. Front. Hum. Neurosci. 7, 438. doi:10.3389/fnhum.2013.00438
Matamala-Gomez, M., Nierula, B., Donegan, T., Slater, M., and Sanchez-Vives, M. V. (2020). Manipulating the Perceived Shape and Color of a Virtual Limb Can Modulate Pain Responses. J. Clin. Med. 9 (2), 291. doi:10.3390/jcm9020291
Newlin, D. B., and Levenson, R. W. (1979). Pre-ejection Period: Measuring Beta-Adrenergic Influences upon the Heart. Psychophysiology 16 (6), 546–552. doi:10.1111/j.1469-8986.1979.tb01519.x
Pfeiffer, C., Schmutz, V., and Blanke, O. (2014). Visuospatial Viewpoint Manipulation during Full-Body Illusion Modulates Subjective First-Person Perspective. Exp. Brain Res. 232 (12), 4021–4033. doi:10.1007/s00221-014-4080-0
Roelofs, J., Peters, M. L., van der Zijden, M., and Vlaeyen, J. W. S. (2004). Does Fear of Pain Moderate the Effects of Sensory Focusing and Distraction on Cold Pressor Pain in Pain-free Individuals? The J. Pain 5 (5), 250–256. doi:10.1016/j.jpain.2004.04.001
Romano, D., and Maravita, A. (2014). The Visual Size of One׳s Own Hand Modulates Pain Anticipation and Perception. Neuropsychologia 57, 93–100. doi:10.1016/j.neuropsychologia.2014.03.002
Schmidt, K., Gamer, M., Forkmann, K., and Bingel, U. (2018). Pain Affects Visual Orientation: an Eye-Tracking Study. The J. pain 19 (2), 135–145. doi:10.1016/j.jpain.2017.09.005
Schneider, S. M., Prince-Paul, M., Allen, M. J., Silverman, P., and Talaba, D. (2004). January). Virtual Reality as a Distraction Intervention for Women Receiving Chemotherapy. Oncol. Nurs. Forum 31 (No. 1). 81. doi:10.1188/04.onf.81-88
Turbyne, C., Koning, P. d., Zantvoord, J., and Denys, D. (2021). Body Integrity Identity Disorder Using Augmented Reality: a Symptom Reduction Study. BMJ Case Rep. 14 (1), e238554, doi:10.1136/bcr-2020-238554
Vrije Universiteit (2019). Data Analysis and Management Software (DAMS) for the Vrije Universiteit Ambulatory Monitoring System (VU-AMS). Available at: http://www.vu-ams.nl/fileadmin/user_upload/manuals/VU-DAMS_manual_v1.5.pdf (Accessed June 24, 2019).
Keywords: virtual reality, first person perspective, third person perspective, affective psychophysiology, acute pain analgesia
Citation: Turbyne C, de Koning P, Smit D and Denys D (2021) Affective and Physiological Responses During Acute Pain in Virtual Reality: The Effect of First-Person Versus Third-Person Perspective. Front. Virtual Real. 2:694511. doi: 10.3389/frvir.2021.694511
Received: 13 April 2021; Accepted: 23 June 2021;
Published: 21 July 2021.
Edited by:
Jordi Vallverdu, Universitat Autònoma de Barcelona, SpainReviewed by:
Aryabrata Basu, Emory University, United StatesLynne Sneddon, University of Gothenburg, Sweden
Copyright © 2021 Turbyne, de Koning, Smit and Denys. This is an open-access article distributed under the terms of the Creative Commons Attribution License (CC BY). The use, distribution or reproduction in other forums is permitted, provided the original author(s) and the copyright owner(s) are credited and that the original publication in this journal is cited, in accordance with accepted academic practice. No use, distribution or reproduction is permitted which does not comply with these terms.
*Correspondence: Collin Turbyne, c.a.turbyne@amsterdamumc.nl