- 1Psychology, School for Social Sciences, University of Westminster, London, United Kingdom
- 2KITE-Toronto Rehabilitation Institute, University Health Network, Toronto, ON, Canada
- 3Department of Psychology, Ryerson University, Toronto, ON, Canada
Background: The introduction of new visual technologies increases the risk of visually induced motion sickness (VIMS). The aim was to evaluate the 6-item Visually Induced Motion Sickness Susceptibility Questionnaire (VIMSSQ; also known as the VIMSSQ-short) and other predictors for individual susceptibility to VIMS.
Methods: Healthy participants (10M + 20F), mean age 22.9 (SD 5.0) years, viewed a 360° panoramic city scene projected in the visual equivalent to the situation of rotating about an axis tilted from the vertical. The scene rotated at 0.2 Hz (72° s−1), with a ‘wobble’ produced by superimposed 18° tilt on the rotational axis, with a field of view of 83.5°. Exposure was 10 min or until moderate nausea was reported. Simulator Sickness Questionnaire (SSQ) was the index of VIMS. Predictors/correlates were VIMSSQ, Motion Sickness Susceptibility Questionnaire (MSSQ), migraine (scale), syncope, Social & Work Impact of Dizziness (SWID), sleep quality/disturbance, personality (“Big Five” TIPI), a prior multisensory Stepping-Vection test, and vection during exposure.
Results: The VIMSSQ had good scale reliability (Cronbach’s alpha = 0.84) and correlated significantly with the SSQ (r = 0.58). Higher MSSQ, migraine, syncope, and SWID also correlated significantly with SSQ. Other variables had no significant relationships with SSQ. Regression models showed that the VIMSSQ predicted 34% of the individual variation of VIMS, increasing to 56% as MSSQ, migraine, syncope, and SWID were incorporated as additional predictors.
Conclusion: The VIMSSQ is a useful adjunct to the MSSQ in predicting VIMS. Other predictors included migraine, syncope, and SWID. No significant relationship was observed between vection and VIMS.
Introduction
Visually induced motion sickness (VIMS) is a phenomenon similar to traditional motion sickness that is often observed in users of visual technologies such as simulators or Virtual Reality (VR) glasses. The widespread introduction of new visual technologies increases the risk of VIMS to the general population. The primary signs and symptoms of traditional motion sickness are nausea and vomiting, together with a host of other related symptoms including stomach awareness, sweating and facial pallor (sometimes called “cold sweating”), increased salivation, sensations of bodily warmth, dizziness, drowsiness (also denoted as the “sopite syndrome”), sometimes headache, loss of appetite, and increased sensitivity to odors (Reason and Brand, 1975). As opposed to traditional motion sickness due to whole-body accelerative stimuli such as during ship motion, the occurrence of oculomotor and central symptoms is relatively higher in VIMS where the provoking stimulus is of visual nature such as in simulators and VR systems. For example, headache is provoked more by visual than real motion, despite the fact that real motion is twice as provocative as equivalent visual motion in terms of nausea potential (Bijveld et al., 2008).
Individuals vary widely in their motion sickness susceptibility, and some general characteristics appear to influence individual differences in motion sickness susceptibility. A caveat is that most of the evidence concerns real motion stimuli rather than purely visual stimuli. For instance, twin studies on motion sickness suggest that a large proportion of variation in susceptibility is accounted for by genetic factors, with heritability estimates of 55–70% (Reavley et al., 2006). Multiple genes are involved and 35 single-nucleotide polymorphisms associated with motion sickness susceptibility have been identified (Hromatka et al., 2015). Age is another important factor, with infants and very young children being seemingly immune to motion sickness, while motion sickness susceptibility begins from around 6–7 years of age (Reason and Brand, 1975) and peaks around 9–10 years (Turner and Griffin, 1999). There is a subsequent decline of susceptibility during the teenage years towards adulthood around 20 years, doubtlessly reflecting habituation. However, for VIMS specifically, a second peak in susceptibility has been reported later in life, with older adults sometimes experiencing more VIMS than younger adults (Brooks et al., 2010; Keshavarz et al., 2018). Biological sex seems to play a role as well, as women appear somewhat more susceptible to motion sickness than men, although this is a much weaker effect than age (Kennedy et al., 1995). This increased susceptibility is likely to be objective and not subjective because women also vomit more than men as a response to motion stimuli; surveys of passengers at sea indicate a five-to-three female-to-male risk ratio for vomiting (Lawther and Griffin, 1988). With regard to VIMS, women have sometimes (but not always) been found to be more susceptible to visual stimulation (Flanagan et al., 2005; Klosterhalfen et al., 2006), and for VR headsets nonfit of interpupillary distance in females may contribute (Stanney et al., 2020). There is some evidence that several preexisting medical conditions that have an impact on quality of life are associated with raised motion sickness susceptibility, including dizziness (Bronstein et al., 2010; Golding and Patel, 2017), proneness to syncope (i.e., feeling of faintness; Bosser et al., 2006), worse sleep quality (Kaplan et al., 2017), and personality factors such as trait anxiety or neuroticism (although often weak effects; Reason and Brand, 1975). Some special groups have reduced or heightened risk: Individuals who have complete bilateral loss of labyrinthine (vestibular apparatus) function are largely immune to motion sickness (Kennedy et al., 1968; Cheung et al., 1991). But this may not be true under all circumstances, since there is evidence that a small minority of bilateral labyrinthine defective individuals are still susceptible to motion sickness provoked by visual stimuli designed to induce self-motion (vection) during pseudo-Coriolis stimulation (i.e., pitching head movements in a rotating visual field) (Johnson et al., 1999). However, it cannot be excluded that some residual vestibular peripheral function remained. Additionally, certain groups of patients with vestibular pathology and vertigo can be especially sensitive to any type of motion. For instance, patients with Meniere’s disease or with vestibular migraine are especially susceptible to motion sickness (Bronstein et al., 2020). Migraineurs (nonvestibular migraine) report greater susceptibility to motion sickness provoked by real physical motion and provoked by visual stimuli (Golding and Patel, 2017). There may be individual variation among migraineurs as to their relative degree of sensitivity to these two classes of stimuli (Drummond, 2005).
A rapid estimate of an individual’s susceptibility to traditional motion sickness can be made using the Motion Sickness Susceptibility Questionnaires (sometimes called Motion History Questionnaires). One of the best validated is the MSSQ (Golding, 2006). The MSSQ was developed mainly to predict the risk of motion sickness to real motion (e.g., translational motion, cross-coupled motion, seasickness, airsickness, etc.) although it was also validated to predict sickness provoked by a visual-vestibular conflict simulator. During the MSSQ development phase a range of items concerning provocative visual stimuli were tested in the item bank. However, as noted in the paper, “…excluded were visual/optokinetic items (Cinerama, Virtual Reality, etc.) … but could become important in the future.” (Golding, 2006). In the intervening years, the importance of visual stimuli as a source of motion sickness has grown considerably. Therefore, work was undertaken to develop a questionnaire which might improve the predictive power for VIMS (Golding and Keshavarz, 2017). The prototype Visually Induced Motion Sickness Susceptibility Questionnaire (VIMSSQ) was 67 items long (Keshavarz et al., 2019), and, in the present study, this was reduced to short form consisting of six items.
The primary aim of this experiment was to evaluate the predictive efficiency of the new 6-item Visually Induced Motion Sickness Susceptibility Questionnaire (VIMSSQ) for individual susceptibility to VIMS. The Simulator Sickness Questionnaire (SSQ) of Kennedy et al. (1993) was employed as the metric of VIMS. Additional aims were to evaluate other questionnaire predictors (including MSSQ, migraine, dizziness, syncope, trait anxiety) for individual susceptibility to VIMS and, lastly, to investigate the possibility that individual differences in multisensory recalibration (the “Stepping-Vection test”) might prove of some use as a possible predictor.
Methods
Participants
Participants were healthy unpaid volunteers with normal or corrected-to-normal vision, had intact vestibular function, and were not on any current medication. They were fully briefed, gave informed consent, and were free to withdraw at any time. Ethical approval was granted by the Psychology Ethics Committee of the University of Westminster, London. Thirty participants (20 females, 10 males) with a mean age of 22.9 years (SD = 5.0 years) were recruited. They were all undergraduate and postgraduate students, their gender ratio reflecting the greater numbers of females in the University. Their susceptibility to motion sickness was assessed using the MSSQ (Golding, 2006), and their percentile scores (M = 48.5 ± 36.0%) indicated that the sample was similar in overall susceptibility to the population norm, which is 50% by definition.
Questionnaires and Other Measurements
Questionnaires at Baseline
A variety of different questionnaires were administered at baseline prior to the visual stimuli in order to test their efficacy for predicting VIMS susceptibility.
(1) The short form of the VIMSSQ—a 6-item short version of the VIMSSQ (Golding and Keshavarz, 2017; Keshavarz et al., 2019)—was developed to capture one’s susceptibility to VIMS and was designed with the expectancy that it would be used in conjunction with the MSSQ as a supplement for circumstances when VIMS is anticipated. The VIMSSQ-short inquires about the frequency of five different symptoms (nausea, headache, fatigue, dizziness, and eye-strain) and also possible consequent avoidance, when using a variety of visual devices and displays (e.g., smartphone, movie theatre, video games, tablets, and Virtual Reality glasses). Items are scored 0 (Never) to 3 (Often). A total scale score is formed by the addition of all items giving a maximum possible range for the VIMSSQ total scale score of minimum of 0 to maximum of 18. Higher scores indicate a stronger susceptibility to VIMS. The VIMSSQ is shown in Table 1.
(2) The short form of the MSSQ (Golding, 2006) was used to assess the participants’ susceptibility to motion sickness. The MSSQ inquires about the participants’ previous experiences of motion sickness when using nine different modes of transportation (e.g., boat, car, bus, and plane) or amusement rides (e.g., funfair rides). Participants have to rate each item on a scale from 0 (never got motion sick) to 3 (often got motion sick). They can also indicate if they never used or experienced the respective item. The MSSQ has two sections, one asking about childhood experiences before the age of 12 (MSSQ Child) and one asking about experiences during adulthood over the last 10 years (MSSQ Adult). A raw score of the whole MSSQ scale can be calculated and translated into percentile scores based on the population norms reported in Golding (2006). Higher scores indicate a stronger susceptibility to motion sickness.
(3) The Migraine Screen Questionnaire (Lainez et al., 2010) consists of five items that are rated on a binary scale (yes, no) in order to measure the participants’ tendency to experience migraines. Items include, for instance, the person’s experience of frequent or intense headaches and duration of those. A total score can be calculated by summing together the value of each item (max. score = 5). Higher scores indicate a greater likelihood of migraines.
(4) The Social Life and Work Impact of Dizziness questionnaire (SWID) measures the negative impact of dizziness on everyday activities (Bronstein et al., 2010). The SWID consists of a set of four social, travel, family, and work-related questions and has been previously validated in patient and control samples. Again, higher scores indicate greater probability of being affected by dizziness.
(5) A single-item syncope question was added to measure the participants’ tendency to experience vasovagal syncope (Golding and Patel, 2017). Participants had to indicate how often they experience the feeling of faintness (e.g., if stressed, in pain, or sighting blood), with higher scores indicating more frequent syncope. This single-item question was adapted from Bosser et al. (2006).
(6) A Sleep Quality questionnaire (Yu et al., 2012) was added to measure the participants’ general sleep quality. This questionnaire measures sleeping patterns in the past 7 days and consists of 16 items each rated on five-point scale not at all to very much, such as My sleep was restless, I had problems during the day because of poor sleep, etc. Higher scores indicate a worse sleep quality.
(7) The Ten Item Personality Inventory (TIPI; Gosling et al., 2003) is a brief measure of the Big Five Personality Factors and was used to investigate the relationship between the personality factors extraversion, agreeableness, conscientiousness, emotional stability, and openness to experience. Participants rate their level of agreement with 10 statements (e.g., I see myself as extraverted/enthusiastic) on a scale from 1 (strongly disagree) to 7 (strongly agree).
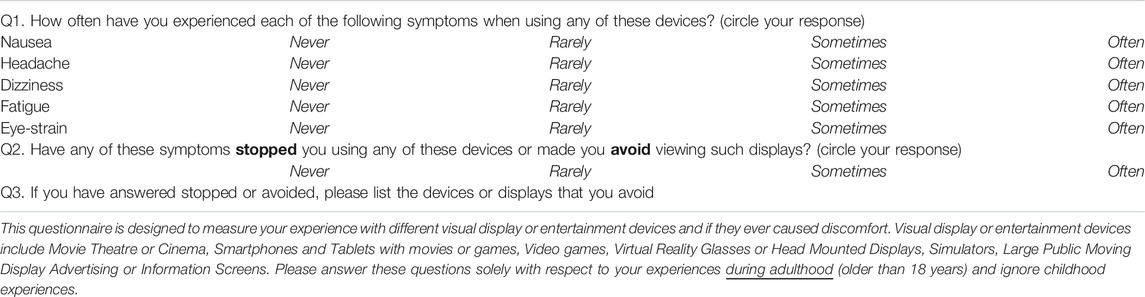
TABLE 1. Visually induced motion sickness susceptibility questionnaire (VIMSSQ). Also known as the VIMSSQ-short.
Rating Scales and Questionnaires During and Immediately After Exposure to the Visual Stimulus
The severity of VIMS was measured in two ways. First, immediately after cessation of the visual stimulus, the Simulator Sickness Questionnaire (SSQ; Kennedy et al., 1993) was employed as the primary metric of VIMS. The SSQ contains 16 symptoms (e.g., general discomfort, fatigue, nausea, and stomach awareness) and the intensity of each item is scored on a 4-point Likert scale (0 = not at all, 1 = mild, 2 = moderate, and 3 = severe). Although three subscores (disorientation, oculomotor, and nausea) and a total score can be produced using specific weighting procedures suggested by the authors of the SSQ, this involves some items being counted twice whereas others are counted only once. Other researchers have failed to find any three-subscale-factor solution but find a clear 2-factor solution. Importantly no double scoring items are evident, i.e., no cross-loadings (e.g., Bouchard et al., 2007). Consequently, we decided to generate a simple, single overall score by summing the scores for each item for the purposes of simplicity. This has proved a useful approach in our previous experiments (Golding et al., 2012) and also in ship motion surveys in the Southern Ocean (Besnard et al., 2019). This has the advantage of capturing the greatest amount of data concerning level of motion sickness in a single variable, for subsequent analyses.
Second, the Sickness Rating scale (SR), a quick self-rating of motion sickness, was used to track the development of motion sickness on a minute-by-minute basis during stimulus exposures. The SR has been validated across a wide range of real and virtual provocative motion sickness environments (Golding et al., 2012). Participants indicate their level of sickness by choosing a score from 6-point scale (1 = no symptoms; 2 = initial symptoms of motion sickness but no nausea; 3 = mild nausea; 4 = moderate nausea; 5 = severe nausea and/or retching; 6 = vomiting). Initial symptoms commonly associated with motion sickness that do not include nausea (SR = 2) can include those commonly associated with motion sickness, including stomach awareness, feelings of bodily warmth, sweating, changes in salivation, and unusual tastes in the mouth. The SR scale was used to track development of motion sickness on a minute-by-minute basis during stimulus exposures. This was to ensure for ethical and safety reasons that the stimulus was stopped immediately if any participant reported moderate nausea and did not experience further adverse consequences. Although not the aim of this experiment, the SR scale also enables an immediate comparison of VIMS with “traditional motion sickness,” since this may well be of interest to workers coming from other areas of research on seasickness, airsickness, Zero-G, etc.
In addition, participants’ experiences of self-motion (vection) were measured after stimulus exposure. That is, participants had to report their level of vection by indicating the percentage of time that they experienced vection during stimulus exposure and its qualitative characteristics (e.g., constant, increasing, decreasing, or varying vection) (Golding et al., 2012).
The Stepping-Vection Test
The Stepping-Vection test was developed using pilot experiments to produce a shortened and reliable modification of an experimental procedure reported by Moss and Muth (2015). The study by Moss and Muth originated in the observation that perceptions of body orientation influence the planning of simple movements, enabling any necessary recalibration factors to be incorporated to ensure the accuracy of movements (Cohn et al., 2000). Based on this, the rationale of the Stepping-Vection test used in the present study was that, after exposure to wide field visual stimulus rotating in yaw, a participant stepping on the spot but now blindfolded will tend to rotate in the opposite direction to the previous visual stimulus, e.g., if the visual stimulus rotates to the left, vection is typically experienced in the opposite direction (to the right). After cessation of the visual stimulus and in the absence of any visual orientation cues (blindfolded), the subsequent direction of whole-body rotation when stepping on the spot will then also be in the same direction as the sensation of vection if experienced (turning rightwards). The visual stimulus used here for the Stepping-Vection test was the same as for the visual stimulus to provoke motion sickness (see next section), but importantly without the superimposed tilt wobble and with a very brief stimulus exposure of only 60 s. Immediately following cessation of this visual stimulus, any sickness was rated on the SR scale as a check, followed by the stepping part of the task. This required the participant to stand on a grid with their feet close together and start walking on the spot for 30 s with their eyes blindfolded and arms crossed over their chest. The instruction to the participants was simply to step on the spot while they were blindfolded. They were not instructed to follow the direction of the previous visual stimulus. Participants were then instructed to stop and remain in final standing position at the end of the task. Participants wore sound blocking headphones to remove any possible auditory spatial orientation cues during this task. The amount of degrees turned and the distance travelled or drifted (if any) from the starting grid were recorded.
Stimuli and Apparatus
The Visual (Optokinetic) Stimulus
A 360o digital photographic panorama of a scene on Westminster bridge over the River Thames in London (see Figure 1) was used as a visual scene. The scene had been chosen to be universally familiar for participants and to contain numerous cues including Big Ben, Houses of Parliament, the London Eye, river, pedestrians, cars, pavement road signs, buildings, and a highly contrasted sky. The scene was rotated through 360o (as if the camera were turning in yaw in a complete circle) for 10 min. The visual scene was projected to be viewed as though the participant was rotating at 72o/s about the long axis of his/her body, tilted from the Earth Vertical by 18° of tilt. This produced a repetition frequency of the visual features of the 360o scene at 0.2 Hz and at the same time an apparent cyclical movement of the horizon reference with an apparent upward and then downward movement of the horizon reference, again at 0.2 Hz. This latter effect we refer to as an apparent “wobble” of the scene, and this has been developed and proven to enhance nauseogenicity (Golding et al., 2009; 2012). The repetition cycle frequency is the same as is known to be maximal for inducing motion sickness by real motion in land, sea, and air environments as well as by visually induced apparent motion (Golding et al., 2009; Diels et al., 2013). This visual stimulus has been compared to equivalent real whole-body Off Vertical Axis Rotation (OVAR) at same 0.2 Hz frequency, 18° tilt, 10 min exposure. This visual stimulus is approximately half as strong in terms of induced motion sickness as exposure to real whole-body motion OVAR equivalent in frequency, tilt, and time duration in the same participants (Bijveld et al., 2008).
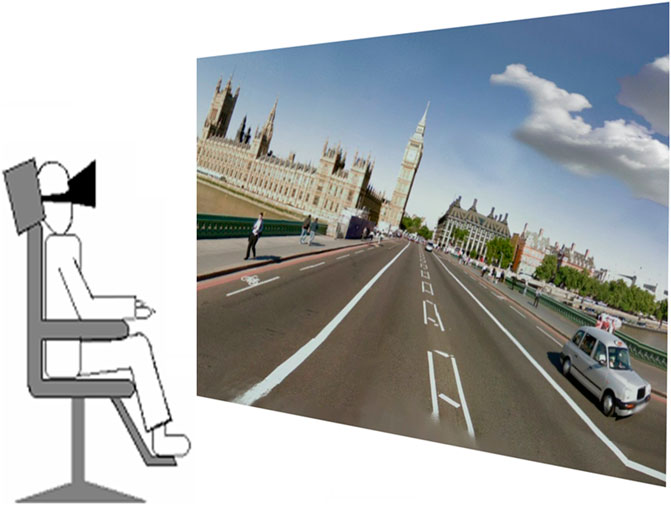
FIGURE 1. Schematic of the visual stimulus: Rotating scene of complete 360° panorama; frequency 0.2 Hz (72 deg/s); “wobble” 18° axial tilt; field of view with mask to 83.5° with circular restriction (Golding et al., 2012).
Apparatus and Laboratory Setting
The panoramic scene was projected to fill a 2 m × 2 m screen which displayed a 90° segment at any given moment in time. The display had a pixel resolution of 1024 × 768 at a refresh rate of 60 Hz. A comfortable supportive chair was positioned centrally in front of the screen such that, when seated, the distance between the participant’s eyes and the screen would be 1.12 m. The armchair in which the participant sat back provided a comfortable head support for the participant but the head was not physically restrained. The participant wore a lightweight face mask mounted with a cone through which the subject viewed the screen to restrict the field of view to 83.5° to exclude peripheral vision of stationary cues of the laboratory. The cone gave a circular perimeter to the field of view.
Procedure
Participants attended the laboratory for a single session in the afternoon. They were given a familiarization briefing and then completed the baseline questionnaires. They then completed the Stepping-Vection test. This was followed by the exposure to visual (optokinetic) stimulus, recovery, and debriefing. The direction of rotation of the visual scenes was counter-balanced between participants, the same direction being used for the Stepping-Vection test and visual (optokinetic) stimulus within each participant.
The level of sickness was rated every minute during optokinetic stimulation using the SR scale. Stimulus exposures were for 10 min or until moderate nausea (SR = 4) was reported. The participants continued to rate their level of sickness during recovery at 1, 2, 3, 4, 5, 10, 15, and 30 min after optokinetic stimulation stopped. Recovery monitoring was necessary for ethical as well as research reasons.
Immediately after the visual stimulation, participants filled out the primary metric of VIMS and the SSQ, and vection was rated. The visual stimulation at 72° per second raised the possibility that participants could experience some optokinetic after-nystagmus (OKAN) which typically may last 10–60 s after stimulation. However, no participants commented on this occurring subjectively nor as a problem and it did not interfere with their completion of the questionnaire after stimulus.
Statistical Analysis
Results were analyzed using SPSS V25.0. Descriptive analysis, reliability analysis, correlations (Pearson and nonparametric), exploratory factor analysis, and multiple linear regressions were employed. Where statistical tests could be directional, the significances were 2-tailed.
Results
General
Descriptive results for baseline variables are shown in Table 2. Comparative benchmark data for these variables are shown in an additional column to the right. The VIMSSQ is a recent scale and consequently there are no published population norms. The MSSQ (Golding, 2006) percentile scores indicated that the sample was similar in overall susceptibility to the population norms. The scores for the other variables, migraine (Lainez et al., 2010), syncope (Golding and Patel, 2017), SWID (Bronstein et al., 2010), sleep quality (Yu et al., 2012), and all “Big Five” personality scales (Gosling et al., 2003) were similar to published norms.
VIMS ratings during the visual stimulus experiment as well as descriptive data for the Stepping-Vection test are shown in Table 3. The reported sickness levels achieved were equivalent to those found in previous experiments using the same visual stimulus (Golding et al., 2012). Poststimulus sickness ratings followed an approximate exponential decline to full subjective recovery in most participants by 5 min and in all by 10 min. For the Stepping-Vection test, the angle turned was in the expected direction, with reversing direction between subjects according to the counter-balanced direction of stimulus rotation. No significant differences in the degrees of rotation or in the amount of drift from the starting grid showed with respect to motion direction of the stimulus (left vs. right). The Stepping-Vection test did not provoke any motion sickness.
Correlations
The VIMSSQ had good scale reliability (Cronbach’s alpha = 0.84). Bivariate correlations between the SSQ and the other baseline variables are shown in Table 4. Stronger VIMS (measured by SSQ) was significantly associated with higher scores in the VIMSSQ, MSSQ, migraine, syncope, and SWID measures. However, the SWID just failed significance when reexamined using nonparametric correlation. There was a tendency for worse sleep quality to be associated with VIMS (measured by SSQ). The association with stepping distance moved was not significant when retested using nonparametric correlation and inspection of the scatterplot revealed that a few outliers were causing any association. All other correlations were low and not significant.
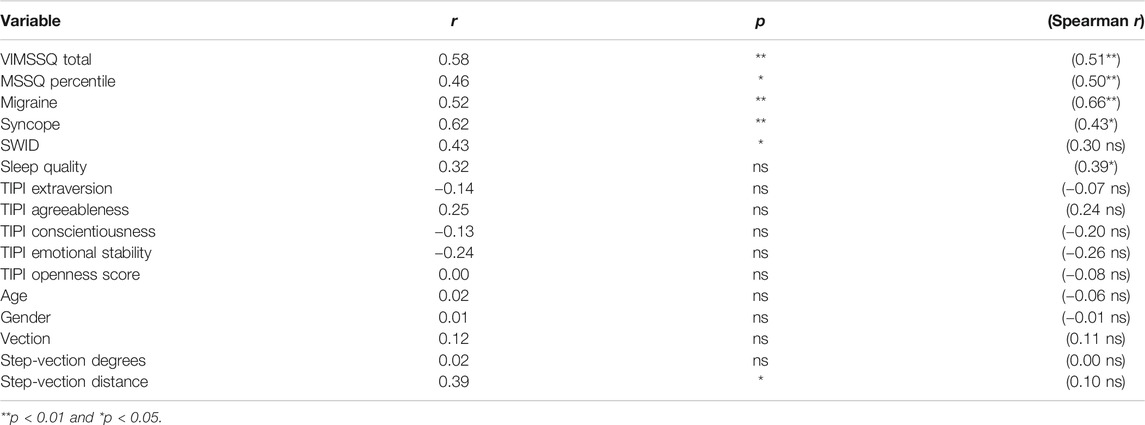
TABLE 4. Correlations of VIMSSQ and other variables with the Simulator Sickness Questionnaire (SSQ) as the metric of visually induced motion sickness after exposure to the visual stimulus video.
In addition to examining the correlations of variables with the SSQ, the full correlation matrix was scrutinized (for brevity not shown). Many of the variables which correlated with the SSQ also correlated with each other. An exploratory factor analysis was performed entering SSQ scores together with those variables which were significantly associated with the SSQ (i.e., VIMSSQ, MSSQ, migraine, syncope, and SWID; see Table 4). This revealed only a single factor on which each variable loaded highly and accounted for 52% of the total variance. This implies the existence of a single underlying latent variable encompassing VIMS (measured by SSQ) together with sickness susceptibility, migraine, dizziness, and autonomic reactivity exemplified by syncope.
Multiple Linear Regression Predictor Models
A series of regression models were examined to predict visually induced motion sickness as measured by the SSQ score. Three examples are shown in Figure 2. Since the primary aim of this experiment was to investigate the predictive power of the 6-item VIMSSQ, the first scatterplot simply illustrates the prediction of SSQ by VIMSSQ (R2 = 0.34, p = 0.001). The next adds the MSSQ as an additional predictor in multiple linear regression and it can be observed how some outliers are immediately pulled in (adjusted R2 = 0.36, p = 0.001). The final illustrative model entered all variables which were significant correlates of SSQ (see Table 4) used as predictors: VIMSSQ, MSSQ-pcn, migraine, syncope, and SWID (adjusted R2 = 0.56, p < 0.001). This demonstrated how prediction efficiency increased as more predictor variables were added to the multiple linear regression models (see Figure 2). It should be noted that, depending on which combination of predictor variables was entered, the loadings of predictors would vary. This is due to collinearity between the predictor variables themselves (see comment at the end of the previous section ‘Correlations’). As a consistency check concerning the possible effects of multicollinearity in the preceding multiple regression analysis, we performed an exploratory factor analysis on all predictor variables which were used in the multiple regression model to predict the SSQ scores (i.e., predictors VIMSSQ, MSSQ-pcn, migraine, syncope, and SWID). A one-factorial solution was found, which accounted for 49.3% of the variance. Factor scores were then computed and outputted. We then used these factor scores in a regression model to predict the SSQ scores. The correlation between the factor scores and SSQ was r = 0.73 (R2 = 0.54, p < 0.001). This solution was very similar to the final multiple linear regression scatterplot shown above, both in terms of the amount of variance of SSQ predicted (54% vs. 56%) and also in the pattern of scatter of the individual datapoints.
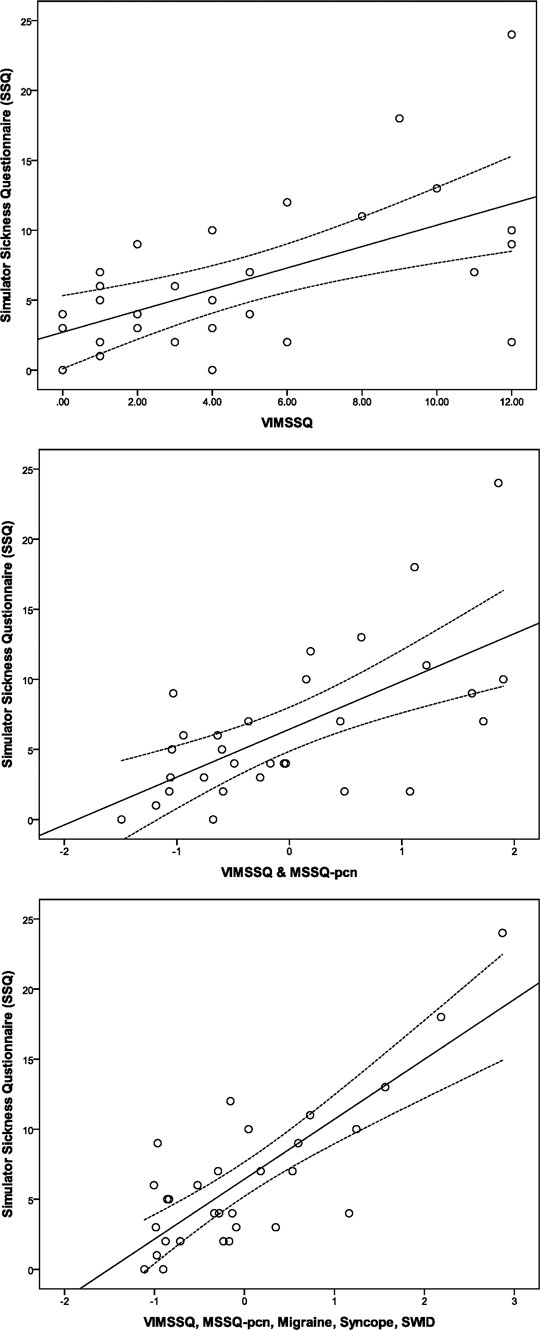
FIGURE 2. Prediction of visually induced motion sickness (VIMS) as measured by the SSQ, showing how prediction efficiency increases as more predictor variables are added to the multiple linear regression models. Top using as predictor: VIMSSQ only (R2 = 0.34); middle using: VIMSSQ and MSSQ percentile (pcn) (adjusted R2 = 0.36); bottom using: VIMSSQ, MSSQ percentile (pcn), migraine, syncope, and SWID (adjusted R2 = 0.56). The fitted regression line is shown in each scatterplot together with the 95%CI dashed lines on either side.
Discussion
The Prediction of VIMS
The main aim of the present study was to investigate the effectiveness of a short questionnaire, the 6-item Visually Induced Sickness Susceptibility Questionnaire (VIMSSQ), to predict individual susceptibility to VIMS when administered prior to exposure to a moving panoramic visual scene known to elicit VIMS. Higher scores on the VIMSSQ significantly predicted VIMS as measured by the SSQ (Kennedy et al., 1993). The VIMSSQ predicted approximately one-third of the individual variation in VIMS.
The secondary aim was to examine other possible predictors for VIMS. Significant baseline predictors for VIMS included higher scores on the MSSQ, greater scores on the migraine screening scale, greater susceptibility to syncope, and higher scores on the SWID. These additional variables, when combined with the VIMSSQ in a multivariate model, significantly improved the overall predictive power. However, this increase in predictive power was not as large as what might be expected, since all these variables significantly correlated with each other and with the VIMSSQ itself. The consequent collinearity produced redundancy. Indeed, exploratory factor analysis of all these variables, together with the SSQ as the measure of sickness, revealed only a single factor. This implies the existence of a single underlying latent variable encompassing VIMS together with motion sickness susceptibility, migraine, dizziness, and autonomic reactivity exemplified by syncope. Such a finding is reminiscent of what has been found in large surveys of the general population and patients experiencing vestibular disorders which produce vertigo (Golding and Patel, 2017). To explain this, it has been proposed that there is an underlying set of risk factors which distribute with increasing strength throughout the general population up into what is then termed the ‘clinical population’ for vestibular related disorders such as Visual Vertigo (Peverall and Golding, 2017) and Persistent Perceptual Postural Dizziness (PPPD) (Bronstein et al., 2020; Powell et al., 2020).
A number of variables measured at baseline failed as predictors. The Stepping-Vection test was a much shortened modification of an experiment reported by Moss and Muth (2015). This modification was successful in that it reliably reproduced the expected movement bias in recalibration of multisensory integration observed by Moss and Muth (2015). However, the modified test failed to be useful in predicting susceptibility to VIMS. It might be argued that the shortening of the test to less than 2 min in total from the original 20 min of optokinetic exposure and 10 replications of a 45 s stepping task (total around half an hour or more) may have been the reason for failure to be a predictor. However, if the test were to be reinstated to the original duration, then it would be of no practical utility, since it would then be quicker to perform the actual visual exposure to elicit VIMS. Consequently, although of great theoretical interest, it seems at this point that this test has limited development potential as a predictor of VIMS susceptibility.
Age and, to a lesser extent, sex (gender) are known to influence motion sickness susceptibility. The failure of age to significantly correlate is unsurprising in the context of the present experiment. This is because the age range of the young adult participants was very narrow, with the consequent restriction of range statistical effect. The failure to find a sex effect might be due to various reasons: the sample size of N = 30 was relatively small to detect an effect of sex and the sample was unbalanced in sex ratio, which further reduced the test power. Additionally, it has been suggested that sex effects are more contradictory for VIMS than for classical motion sickness susceptibility, at least as understood so far given the number of studies at present (Saredakis et al., 2020). There was a trend for worse sleep quality to be associated with higher levels of VIMS, but this failed significance. It may be that effects of sleep deprivation can only be reliably observed when the amount of sleep deprivation is much stronger, similar to the study of Kaplan et al. (2017), which used sleep deprivation as an actual intervention against low frequency real motion. There were no significant relationships observed between any of the ‘Big Five’ personality factors and VIMS. The most likely relationship with greater motion sickness susceptibility is with trait anxiety or neuroticism, but these are weak (Reason and Brand, 1975) and are usually observed only in large studies (e.g., Paillard et al., 2013), perhaps because under those conditions enough highly anxious individuals can be tested.
The symptom scores and amount of time vection was experienced were similar in this experiment to a previous study using the same visual stimulus (Golding et al., 2012). Notably, no correlation was observed between vection and VIMS, and there was not any evidence that the quality of vection, for example increasing, decreasing, or changing, had any relationship to VIMS. Indeed, one notable feature of vection is that this illusion can onset and then vanish within seconds, whereas motion sickness usually builds up more slowly over time. This lack of relationship was also noted in our previous studies using this type of visual stimulus (Bijveld et al., 2008; Golding et al., 2009; Golding et al., 2012). Although some studies have found relationships between vection and VIMS (e.g., Nooij et al., 2017), the literature is contradictory with many failures to show such relationships (Lawson, 2014; Kuiper et al., 2019). A thorough discussion on the topic can be found in Keshavarz et al. (2015). Vection may play a role in VIMS but the relationship between them is not one-to-one and appears not to be directly causal in any obvious fashion. The explanation may be that vection is a conscious illusory perception, presumably happening at a cortical level in the brain. By contrast the visual-vestibular mismatches or conflicts provoking motion sickness are doubtless occurring at the brainstem–cerebellar level (Oman and Cullen, 2014) and may not be directly accessible to conscious perception. This may explain the lack of reliable association between vection and VIMS.
Limitations and Future Outlook
This study had some limitations. Although the stimulus used to provoke VIMS was well validated and reliable, it is important to note that it was only one type of stimulus. The effectiveness of the short 6-item VIMSSQ has to be shown for other settings such as driving simulators or VR. With Head Mounted Displays (HMDs) and virtual environments there are also other factors including eye-head coordination such as update lags, accommodation-vergence conflicts, flicker, etc. The prototype 67-item VIMSSQ did demonstrate predictive power for VIMS elicited by a driving simulator task (Keshavarz et al., 2019). Nevertheless, the 6-item VIMSSQ needs to be evaluated on a variety of stimuli capable of eliciting VIMS to demonstrate its general utility. One limitation of the present study was the small sample size of n = 30, which will require follow-up studies with larger sample size to strengthen our initial findings. Another limitation was that the participants in this study were healthy, fit young adults. The generalizability of predictive power of the VIMSSQ to the older population is necessary. This is because people become more visually dependent with increasing age as they reweight the three main sensory inputs used for balance and orientation. The reweighting is usually away from vestibular and proprioceptive inputs (which often become less reliably accurate with ageing) to greater dependence of visual inputs (Pavlou and Newham, 2013). Again, older adults may have had less experience with new visual technologies. Both these factors may increase susceptibility to VIMS. At the same time, an opposing factor comes into play, that overall motion sickness susceptibility to physical motion is known to decline with age (with individual variation) (Paillard et al., 2013). Consequently, the predictive efficiency of the VIMSSQ needs to be tested for the older population. Some room for optimism is provided by Keshavarz et al. (2019) study, which included both younger and older adults. This showed that the 67-item prototype VIMSSQ could predict VIMS with relatively small differences in predictive power across the age span.
Conclusion
The results of this study indicate that the short version of the VIMSSQ can successfully predict around a third of the individual variation in VIMS. At six items it is short and very quick to complete. To increase predictive power, it is probably best used in conjunction with the MSSQ. Researchers may also wish to consider adding other possible predictors such as migraine and a measure of autonomic reactivity such as syncope.
Data Availability Statement
The raw data supporting the conclusions of this article will be made available by the authors, without undue reservation.
Ethics Statement
The studies involving human participants were reviewed and approved by the Psychology Ethics Committee, University of Westminster, London. The patients/participants provided their written informed consent to participate in this study.
Author Contributions
JG and BK conceived the idea for the study. JG, BK, and AR wrote the manuscript. JG, BK, and AR analyzed and interpreted the data. AR and JG carried out the experiments. AR recruited participants.
Conflict of Interest
The authors declare that the research was conducted in the absence of any commercial or financial relationships that could be construed as a potential conflict of interest.
Supplementary Material
The Supplementary Material for this article can be found online at: https://www.frontiersin.org/articles/10.3389/frvir.2021.576871/full#supplementary-material.
References
Besnard, S., Bois, J., Golding, J., Hitier, M., and Vogt, J. (2019). “SICKVEST program: survey of astrolab's passengers.” in Congress on motion sickness, July 7–10, 2019, akureyri, Iceland. Available at: https://www.motionsickness.is.
Bijveld, M. M., Bronstein, A. M., Golding, J. F., and Gresty, M. A. (2008). Nauseogenicity of off-vertical axis rotation vs. equivalent visual motion. Aviat Space Environ. Med. 79, 661–665. doi:10.3357/asem.2241.2008
Bosser, G., Caillet, G., Gauchard, G., Marçon, F., and Perrin, P. (2006). Relation between motion sickness susceptibility and vasovagal syncope susceptibility. Brain Res. Bull. 68, 217–226. doi:10.1016/j.brainresbull.2005.05.031
Bouchard, S., Robillard, G., and Renaud, P. (2007). Revising the factor structure of the simulator sickness questionnaire. The 2007 Annual Review of CyberTherapy and Telemedicine. 5, 128–137.
Bronstein, A. M., Golding, J. F., Gresty, M. A., Mandalà, M., Nuti, D., Shetye, A., and Silove, Y. (2010). The social impact of dizziness in London and Siena. J. Neurol. 257, 183–190. doi:10.1007/s00415-009-5287-z
Bronstein, A. M., Golding, J. F., and Gresty, M. A. (2020). Visual vertigo, motion sickness, and disorientation in vehicles. Semin. Neurol. 40, 116–129. doi:10.1055/s-0040-1701653
Brooks, J. O., Goodenough, R. R., Crisler, M. C., Klein, N. D., Alley, R. L., Koon, B. L., Logan, W. C., Ogle, J. H., Tyrrell, R. A., and Wills, R. F. (2010). Simulator sickness during driving simulation studies. Accid. Anal. Prev. 42 (3), 788–796. doi:10.1016/j.aap.2009.04.013
Cheung, B. S., Howard, I. P., and Money, K. E. (1991). Visually-induced sickness in normal and bilaterally labyrinthine-defective subjects. Aviat Space Environ. Med. 62 (6), 527–531.
Cohn, J. V., DiZio, P., and Lackner, J. R. (2000). Reaching during virtual rotation: context specific compensations for expected coriolis forces. J. Neurophysiol. 83, 3230–3240. doi:10.1152/jn.2000.83.6.3230
Diels, C., and Howarth, P. A. (2013). Frequency characteristics of visually induced motion sickness. Hum. Factors. 55, 595–604. doi:10.1177/0018720812469046
Drummond, P. D. (2005). Triggers of motion sickness in migraine sufferers. Headache 45, 653–656. doi:10.1111/j.1526-4610.2005.05132.x
Flanagan, M. B., May, J. G., and Dobie, T. G. (2005). Sex differences in tolerance to visually-induced motion sickness. Aviat Space Environ. Med. 76, 642–646.
Golding, J. F., Arun, S., Wortley, E., Wotton-Hamrioui, K., Cousins, S., and Gresty, M. A. (2009). Off-vertical axis rotation of the visual field and nauseogenicity. Aviat Space Environ. Med. 80, 516–521. doi:10.3357/asem.2433.2009
Golding, J. F., Doolan, K., Acharya, A., Tribak, M., and Gresty, M. A. (2012). Cognitive cues and visually induced motion sickness. Aviat Space Environ. Med. 83, 477–482. doi:10.3357/asem.3095.2012
Golding, J. F., and Keshavarz, B. (2017). “Predictors of visually induced motion sickness susceptibility.” in 6th international VIMS conference, Toronto, Canada, November 16–17, 2017. Available at: https://www.mive.ca/vims2017.
Golding, J. F., and Patel, M. (2017). Meniere's, migraine, and motion sickness. Acta Otolaryngol. 137, 495–502. doi:10.1080/00016489.2016.1255775
Golding, J. F. (2006). Predicting individual differences in motion sickness susceptibility by questionnaire. Pers. Indiv. Differ. 41, 237–248. doi:10.1016/j.paid.2006.01.012
Gosling, S. D., Rentfrow, P. J., and Swann, W. B. (2003). A very brief measure of the Big-Five personality domains. J. Res. Pers. 37, 504–528. doi:10.1016/s0092-6566(03)00046-1
Hromatka, B. S., Tung, J. Y., Kiefer, A. K., Do, C. B., Hinds, D. A., and Eriksson, N. (2015). Genetic variants associated with motion sickness point to roles for inner ear development, neurological processes and glucose homeostasis. Hum. Mol. Genet. 24, 2700–2708. doi:10.1093/hmg/ddv028
Johnson, W. H., Sunahara, F. A., and Landolt, J. P. (1999). Importance of the vestibular system in visually induced nausea and self-vection. J. Vestib. Res. 9, 83–87.
Kaplan, J., Ventura, J., Bakshi, A., Pierobon, A., Lackner, J. R., and DiZio, P. (2017). The influence of sleep deprivation and oscillating motion on sleepiness, motion sickness, and cognitive and motor performance. Auton. Neurosci. 202, 86–96. doi:10.1016/j.autneu.2016.08.019
Kennedy, R. S., Graybiel, A., McDonough, R. C., and Beckwith, F. D. (1968). Symptomatology under storm conditions in the north Atlantic in control subjects and in persons with bilateral labyrinthine defects. Acta Otolaryngol. 66 (1–6), 533–540. doi:10.3109/00016486809126317
Kennedy, R. S., Lanham, D. S., Massey, C. J., and Drexler, J. M. (1995). Gender differences in simulator sickness incidence : implications for military virtual reality systems. SAFE J. 25, 69–76.
Kennedy, R. S., Lane, N. E., Berbaum, K. S., and Lilienthal, M. G. (1993). Simulator sickness questionnaire: an enhanced method for quantifying simulator sickness. Int. J. Aviat. Psychol. 3 (3), 203–220. doi:10.1207/s15327108ijap0303-3
Keshavarz, B., Riecke, B. E., Hettinger, L. J., and Campos, J. L. (2015). Vection and visually induced motion sickness: how are they related? Front. Psychol. 6 (472), 1–11. doi:10.3389/fpsyg.2015.00472
Keshavarz, B., Saryazdi, R., Campos, J. L., and Golding, J. F. (2019). “Introducing the VIMSSQ: measuring susceptibility to visually induced motion sickness.” in HFES, human factors and ergonomics society annual meeting. Seattle, Washington, USA. Available at: http://www.hfes2019.org/.
Keshavarz, B., Ramkhalawansingh, R., Haycock, B., Shahab, S., and Campos, J. L. (2018). Comparing simulator sickness in younger and older adults during simulated driving under different multisensory conditions. Transport. Res. F Traffic Psychol. Behav. 54, 47–62. doi:10.1016/j.trf.2018.01.007
Klosterhalfen, S., Pan, F., Kellermann, S., and Enck, P. (2006). Gender and race as determinants of nausea induced by circular vection. Gend. Med., 3 (3), 236–242. doi:10.1016/s1550-8579(06)80211-1
Kuiper, O. X., Bos, J. E., and Diels, C. (2019). Vection does not necessitate visually induced motion sickness. Displays 58, 82–87. doi:10.1016/j.displa.2018.10.001
Láinez, M. J., Castillo, J., Domínguez, M., Palacios, G., Díaz, S., and Rejas, J. (2010). New uses of the migraine screen questionnaire (MS-Q): validation in the primary care setting and ability to detect hidden migraine. MS-Q in primary care. BMC Neurol. 10, 39. doi:10.1186/1471-2377-10-39
Lawson, B. (2014). “Motion sickness symptomatology and origins.” in Handbook of virtual environments: Design, implementation, and applications, Editors K. S. Hale, and K. M. Stanney, (Boca Raton, FL: CRC Press), 531–600.
Lawther, A., and Griffin, M. J. (1988). A survey of the occurrence of motion sickness amongst passengers at sea. Aviat Space Environ. Med., 59: 399–406.
Moss, J. D., and Muth, E. R. (2015). After-effects following circular vection exposure. Poster presented at the 86th annual meeting of the aerospace medical association, May 10-14, 2015, Orlando, Florida, USA.
Nooij, S. A., Pretto, P., Oberfeld, D., Hecht, H., and Bülthoff, H. H. (2017). Vection is the main contributor to motion sickness induced by visual yaw rotation: implications for conflict and eye movement theories. PloS One 12 (4), e0175305. doi:10.1371/journal.pone.0175305
Oman, C. M., and Cullen, K. E. (2014). Brainstem processing of vestibular sensory exafference: implications for motion sickness etiology. Exp. Brain Res. 232 (08), 2483–2492. doi:10.1007/s00221-014-3973-2
Paillard, A. C., Quarck, G., Paolino, F., Denise, P., Paolino, M., Golding, J. F., and Ghulyan-Bedikian, V. (2013). Motion sickness susceptibility in healthy subjects and vestibular patients: effects of gender, age and trait-anxiety. J. Vestib. Res. 23, 203–209. doi:10.3233/VES-130501
Pavlou, M., and Newham, D. (2013). “The principles of balance treatement and rehabilitation.” in: Oxford textbook of vertigo and imbalance. Editor A. M. Bronstein Chapter 28. (Oxford University Press, Oxford UK), 179–195.
Peverall, L., and Golding, J. F. (2017). Predictors of visual vertigo and dizziness in the general population. London, UK: 11th Meeting of the British Society of Neuro-Otology. St Thomas’ HospitalAvailable at: http://www.bsno.org.uk (Accessed October 6, 2017).
Powell, G., Derry-Sumner, H., Rajenderkumar, D., Rushton, S. K., and Sumner, P. (2020). Persistent postural perceptual dizziness is on a spectrum in the general population. Neurology 94, e1929–e10. doi:10.1212/WNL.0000000000009373
Reavley, C. M., Golding, J. F., Cherkas, L. F., Spector, T. D., and MacGregor, A. J. (2006). Genetic influences on motion sickness susceptibility in adult females: a classical twin study Aviat Space. Environ. Med. 77, 1148–1152.
Saredakis, D., Szpak, A., Birckhead, B., Keage, H. A. D., Rizzo, A., and Loetscher, T. (2020). Factors associated with virtual reality sickness in head-mounted displays: a systematic review and meta-analysis. Front. Hum. Neurosci. 14, 96. doi:10.3389/fnhum.2020.00096
Stanney, K., Fidopiastis, C., and Foster, L. (2020). Virtual reality is sexist: but it does not have to Be. Front. Robot. AI. 7 (4), 1–19. doi:10.3389/frobt.2020.00004
Turner, M., and Griffin, M. J. (1999). Motion sickness in public road transport: passenger behavior and susceptibility. Ergonomics 42, 444–461. doi:10.1080/001401399185586
Keywords: motion sickness (simulator sickness), migraines, optokinetic, vection, personality, anxiety, syncope, sleep
Citation: Golding JF, Rafiq A and Keshavarz B (2021) Predicting Individual Susceptibility to Visually Induced Motion Sickness by Questionnaire. Front. Virtual Real. 2:576871. doi: 10.3389/frvir.2021.576871
Received: 27 June 2020; Accepted: 07 January 2021;
Published: 26 February 2021.
Edited by:
Kay Marie Stanney, Design Interactive, United StatesReviewed by:
James Robert Lackner, Brandeis University, United StatesErnesto Ruiz, Design Interactive, United States
Copyright © 2021 Golding, Rafiq and Keshavarz. This is an open-access article distributed under the terms of the Creative Commons Attribution License (CC BY). The use, distribution or reproduction in other forums is permitted, provided the original author(s) and the copyright owner(s) are credited and that the original publication in this journal is cited, in accordance with accepted academic practice. No use, distribution or reproduction is permitted which does not comply with these terms.
*Correspondence: John F. Golding, goldinj@westminster.ac.uk