- 1Department of Computer Science, The College of New Jersey, Ewing, NJ, United States
- 2Department of Computer Sciences and Electrical Engineering, Marshall University, Huntington, WV, United States
- 3Department of Computer Science, University of Texas at San Antonio, San Antonio, TX, United States
Many users have shown increased postural instability while using Head-Mounted Displays (HMDs) as HMDs block their real-world vision. People with balance impairments are especially more affected by this as they depend more on their visual cues to maintain their balance. In addition, balance is a good indication of cybersickness according to postural instability theory. In this research, we have investigated how to use additional visual cues to improve postural stability. Through conducting one user study in Virtual Reality (VR) and Augmented Reality (AR), we have studied the effect of a Static Rest Frame (SRF) on postural stability in persons with balance impairments due to Multiple Sclerosis (MS). Results indicate that an SRF significantly improves postural stability in VR and AR for users with MS. Based on these results, we propose guidelines for designing more accessible VR and AR systems for persons with balance impairments.
1 Introduction
Many consumer-level head-mounted displays (HMDs) are currently available and/or in development used by a diverse population, including users with balance impairments. Previous research has shown that HMDs (e.g., the Oculus, 2020) can potentially hinder the balance of users with balance impairments while standing or walking as HMDs blocks peripheral vision (Guo et al., 2014). However, there is not enough research targeted to solve this problem. We aim to improve the postural stability in Virtual Reality (VR) and Augmented Reality (AR) for persons with balance impairments in this research.
Many rehabilitation programs use VR to improve balance for people with balance impairments (Sveistrup et al., 2003; Fulk, 2005; Lozano-Quilis et al., 2013; Nilsagård et al., 2013). However, VR that uses HMDs often negatively affects the balance of users with balance impairments. Therefore, HMDs are not popular in rehabilitation programs. Instead, projectors or large screens are mostly used as display media in these programs. Previous research shows that HMDs are more immersive than projectors and users may experience a higher presence (Moreno and Mayer, 2002). Hypothetically, HMDs could engage participants more effectively. Therefore, the imbalance problems of users with balance impairments while wearing HMDs need to be addressed.
Previous research shows that users have decreased postural stability in an immersive Virtual Environment (VE) that uses an HMD. Samaraweera et al. (Samaraweera et al., 2015) reported that participants have increased near falls and stumbles in VR. In other researches, positive effects of SRF were observed to improve presence (Prothero, 1998) and depth perception (Jones et al., 2013) and reduce cybersickness (Prothero, 1998; Chang E et al., 2013; LaViola, 2000). Based on these previous researches, we hypothesize that a Static Rest Frame (SRF), a subtle yet effective visual feedback, can improve postural stability. Baram et al. successfully demonstrated the positive effect of visual feedback on improving gait (i.e., walking patterns) for persons with balance impairments (Baram and Miller, 2006). Their research used a virtual grid on the floor to show that an additional visual cue, rendered from the perspective of the users’ view, improved gait in persons with Multiple Sclerosis (MS). The reason can be that persons with vestibular (i.e., balance perception in the inner ear) impairments and neuropathy (e.g., numbness) depend more on their visual feedback to maintain balance (Corporaal et al., 2013). Therefore, additional visual cues may improve a user’s postural stability. Being inspired by the previous work on the perceptual benefits of SRFs, our first study investigates how an SRF in an HMD affects postural stability for persons with balance impairments in VR.
To ensure undisturbed interaction in the VR, we wanted to use an SRF that minimally blocks the VE. Therefore, our SRF consists of five small static frames (one cross-hair in the middle and four L-shaped frames in four corners). Figure 1 shows the static positioning of the SRF regardless of participants’ left or right rotation. Figure 1 also shows a dodgeball game used to analyze the effects of an SRF on the postural stability of persons with balance impairments.
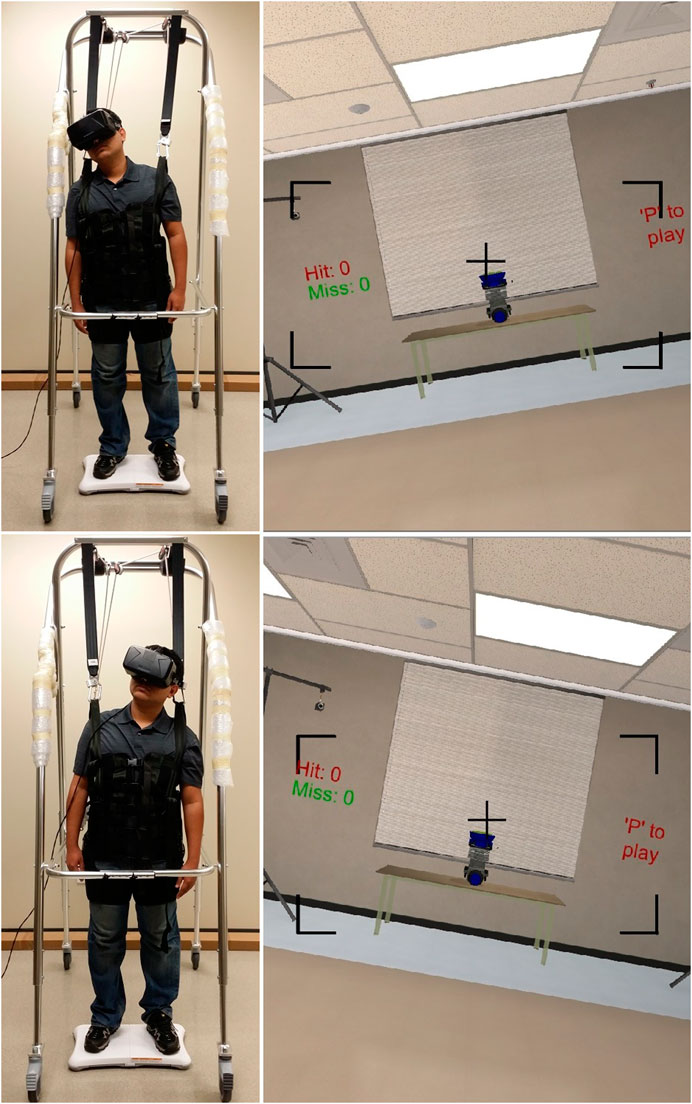
FIGURE 1. A participant playing the dodge ball game and his view in VR with a static rest frame as he shifts his balance to the right or left. The figure shows an author who is posing as a participant. He gave consent to publish this image.
In this article, we presented two studies to improve postural stability in VR and AR for persons with balance impairments, such as users with MS (see Section 3.2.1). The results of the VR study were published in a previous conference (Ferdous et al., 2016). The AR study data and results and the comparison between AR and VR study data are the unique contributions of this article. The VR study and results are also described in this article to provide the whole context. That is, Section 4 extends the published work described in Section 3. For the first study, we recruited seven users without impairment and seven users with balance impairments caused by MS to examine the effect of SRF in VR. We analyzed their Quiet Stance Balance (QSB) and Functional Balance (FB) while in the VE. QSB is defined as standing balance on a stable support surface. It is inspired by the Romberg test (Khasnis and Gokula, 2003). In the Romberg test, participants have to stand still with their eyes closed, feet together, and arms at the side while their balances are measured. FB is inspired by the multidirectional reach test (Newton, 2001). In a multidirectional reach test, a participant has to reach the front and back and lean side to side to their maximum reach with his feet flat on the floor. In our study, we only investigated participants’ FB for leaning side to side, which was proven to be an effective means of balance training (Bisson et al., 2007). Our first study results suggest that participants with balance impairments have a significantly improved balance in VR, while there is an SRF in the VE.
After discovering an SRF’s benefits to balance in consumer-level VR HMDs, we hypothesized that an SRF might also have similar benefits in the latest consumer-level augmented reality HMDs, such as the Microsoft HoloLens. AR HMDs do not block the periphery as the VR HMDs do. Therefore, the participants will get an additional cue from their peripheral vision to maintain balance in AR HMDs. We ran a follow-up study to determine SRF’s effect in AR. Additional visual feedback in augmented reality was useful in improving functional mobility for people with Parkinson’s disease (Kaminsky et al., 2007). There are augmented reality cueing devices available for improving gait in patients with Parkinson’s disease (Espay et al., 2010). Therefore, our result from the VR study and previous literature on using visual cues in augmented reality motivated us to investigate the effect of SRFs in AR. We found that the SRF improved balance in AR.
2 Background and Related Work
2.1 Balance and Multiple Sclerosis
People rely on three inputs for maintaining balance: proprioception, vestibular organ information, and the major contributing factor, which is vision (Hansson et al., 2010). When the vision is limited, postural sway is increased in people with MS more than people without MS (Van Emmerik et al., 2010). Moreover, when vision is compromised, people rely more on proprioceptive feedback, and proprioceptive impairments often affect balance in patients with MS (Rougier et al., 2007). Therefore, altering the major contributing factor (i.e., vision) can potentially affect balance for people with MS. This also opens the possibility of providing additional visual cues to improve balance, where visual information is altered or insufficient.
2.2 Virtual Reality and Rehabilitation
VR is becoming popular in the rehabilitation of balance impairments. Fulk et al. used VR to improve gait speed, gait endurance, and balance improvement (Fulk, 2005). Lozano-Quilis et al. developed REOVIEM, a system that uses VR and natural user interfaces for motor rehabilitation exercises (Lozano-Quilis et al., 2013). Nilsagård et al. proved the usability of Nintendo Wii games for balance and gait rehabilitation (Nilsagård et al., 2013). Sveistrup et al. showed a comparison of a VR-delivered exercise program to a conventional exercise program for the rehabilitation of shoulder joint range of motion in patients with chronic frozen shoulder and discussed the possibility of using VR in rehabilitation (Sveistrup et al., 2003).
2.3 Balance in Immersive VR
It is unknown what causes an imbalance in HMDs or how to mitigate these effects, but it is known that many users often experience an increased imbalance in immersive VR with HMDs. For example, for both persons without impairments and persons with balance impairments (Guo et al., 2014), Samaraweera et al. (Samaraweera et al., 2013) found that most users experienced reduced gait (i.e., walking patterns) performance; for example, they walked slower and took shorter strides in VR compared to the real environment. This could be indicative of more cautious behavior due to increased imbalance. Kennedy et al. showed that postural instability due to VR exposure is similar to alcohol-induced ataxia (Kennedy and Stanney, 1996). They developed an objective measurement of postural stability based on head movement to certify a VR system’s safety. Horlings et al. reported that VR causes an increase in postural sway in amplitude similar to that caused by closing the eyes (Horlings et al., 2009). The postural instability can linger even after the completion of VR exposure (Champney et al., 2007).
2.4 Cybersickness and Balance
The postural instability theory is a widely cited theory that describes postural instability as a cause of cybersickness (Riccio and Stoffregen, 1991). Riccio et al. categorized the environmental situations responsible for postural instability into four categories: low-frequency vibration, weightlessness, changing relationships between the gravitoinertial force vector and the surface of support, and altered specificity. LaViola et al. suggested altered specificity to be the cause of cybersickness (LaViola, 2000). In an altered specificity situation, Riccio et al. described that a person might exert muscular efforts to resist an optically specified acceleration. Moreover, overall body posture is strongly influenced by optical stimulation (Lee and Lishman, 1975). Persons with MS face more difficulty in muscular movement than persons without impairment (Bakshi, 2003) and have worse balance in VR. Thus, persons with MS may be more prone to cybersickness than persons without impairment.
Kennedy et al. developed the Simulator Sickness Questionnaire (SSQ) (Kennedy et al., 1993), which is widely used to measure cybersickness. SSQ measures cybersickness using three subscales:
2.5 SRF to Reduce Cybersickness
Prothero showed that SRFs could improve persons’ presence and reduce cybersickness in VEs (Prothero, 1998). He argued that humans have a strong perception of stationary things (e.g., we perceive the earth as stationary). He asserted that humans interpret relative motion to find if an object is moving. Therefore, humans need a rest frame to imply if the object in question is moving. In real life, the earth’s surface works as a rest frame, and we perceive relative motion with respect to the earth’s surface. Therefore, we hypothesize that additional static rest visual cues may aid in persons’ balance. Results from Prothero’s study support the hypothesis that the inclusion of an SRF improves presence and reduces cybersickness. His findings of improved presence motivated us to research the balance of persons in VEs.
Chang et al. demonstrated that an SRF consisting of one or several frames can be beneficial to lessen cybersickness indications (Chang E et al., 2013). They presented that the presence of an SRF changes one’s perception of the VE, and this change of perception may be the cause of reduced cybersickness. Therefore, with the change of perception, the SRF may impact the balance of a person.
2.6 SRF to Improve Depth Perception
Jones et al. showed that applying static white light at the periphery of the VE display improves a person’s ability to judge distance (Jones et al., 2013). The static light was also useful when a person estimates virtual space size using a visual scale task. Distance judgment ability or depth perception plays an essential role in a person’s balance (Lord, 2006). Therefore, the finding of Jones et al. in improving depth perception using a static light may also be applicable to improve balance. A similar effect may be achieved using an SRF as it is also static though it uses frames instead of lights.
All of the works described before in this section successfully used an SRF in different aspects of VR (e.g., presence, cybersickness, and depth perception). However, none of these previous researches focused on the balance of users in VR. Therefore, the success of previous researches leads us to the primary research goal of our first study, that is, investigating the effect of an SRF in VR on QSB and FB of users, primarily focusing on users with MS.
2.7 Augmented Reality and Rehabilitation
In general, showing additional visual cues in AR can help improve the mobility of people with mobility impairments. For example, AR was found to be useful in improving functional mobility for people with Parkinson’s disease (Kaminsky et al., 2007). The authors used virtual cueing spectacles to mimic kinesia paradoxa—a sudden, brief period of mobility under certain circumstances (Banou, 2015). Kaminsky et al. used spectacles that consist of a light-emitting diode that generates a series of horizontal lines, and the lights are reflected off a lens into the wearer’s eye. Other types of AR cueing devices have also been used to improve gait in people with Parkinson’s disease (Espay et al., 2010). These authors used visual cues that mimic a life-size virtual checkerboard-tiled floor. These checkerboards have a similar impact as earth-stationary cues (i.e., a real tiled floor) for improving gait. Rather than gait, our research specifically investigates balance in AR and the effects of SRFs.
3 Effect of Static Rest Frame in Virtual Reality
As the first step of our investigation, we focus on improving accessibility in VR. Inspired by the background works described in Section 2, we aimed to investigate our hypotheses with the help of a game we designed. The following subsections will describe our VR study in detail.
3.1 Hypotheses
The goal of this research is to improve the postural stability in VR and AR for persons with balance impairments. We broke down our research goal into two parts and conducted two studies: a VR study and an AR study. As a part of the VR study, we need to analyze each person’s QSB and FB in VR and find out the effect of the SRF on balance. Based on the known advantages of SRFs from the literature, the following hypotheses are to investigate the effects of SRFs on balance in VR:
H1: An SRF will significantly improve balance in VR for users with balance impairments.
H2: The balance of persons with balance impairment will be more affected by VE than that of persons without balance impairments.
H3: Persons with balance impairment experience different levels of severity of cybersickness compared to persons without impairments.
3.2 Methods
3.2.1 Participants and Selection Criteria
For the first study, we recruited seven participants without impairment and seven participants with MS to investigate the effect of the SRF while in VR on their QSB and FB. All participants were informed of the study procedure, and they provided written consent in accordance with the Institutional Review Board of the University of Texas at San Antonio (UTSA IRB #14–095). The experimental group consisted of persons with MS, and the control group was comprised of persons without any balance impairment. The participants without impairment came from a similar demographic background and had similar height and weight to the participants with MS. Every participant had a normal or corrected to normal vision. To keep the level of disability for the persons with MS homogeneous, we recruited persons who had an Expanded Disability Status Scale (EDSS) (Kurtzke, 1983) between 3 and 4.5. EDSS is measured from 0 to 10, where 0 means normal neurological state and 10 means death due to MS. Any person with severely blurred vision, vestibular diseases (non-MS related), psychiatric disorders, cognitive impairment, or cardiovascular and respiratory disorders were excluded from the study. Table 1 shows the mean (SD) age, EDSS, and other detailed information about the participants.
3.2.2 Study Conditions
QSB and FB in VR are the conditions that we examined in this study. QSB data were assessed with the SRF (QSB-SRF) and without the SRF (QSB-NoSRF) in the VE (see Section 3.2.4). FB data were also measured with the SRF (FB-SRF) and without the SRF (FB-NoSRF). The order of the subconditions (with or without the SRF) was counterbalanced and assigned randomly among the participants. In addition to these conditions, there were two baseline conditions in our study: Eyes Open (EO) balance and Eyes Closed (EC) balance. We compared the data from QSB-SRF and QSB-NoSRF conditions with the baseline conditions. Berg Balance Scale (Berg et al., 1989) and Tinetti Performance-Oriented Mobility Assessment (Tinetti, 1986) showed that EO and EC are effective measurements of balance.
We developed a virtual representation of the physical room where the experiment took place. Participants experienced the virtual representation in QSB-SRF and QSB-NoSRF conditions while their balances were being measured. In FB-SRF and FB-NoSRF conditions, they played a VR game where a virtual bowling machine shot tennis balls toward their heads and they dodged the balls by moving their head from side to side.
3.2.3 System Description
We designed a system with a Nintendo Wii Fit Balance Board to measure the participants’ balance in different study conditions as described earlier (see Section 3.2.2). Many studies showed the validity and reliability of the Wii balance board as an effective tool to measure balance (Clark et al., 2010; Chang WD et al., 2013). We supported all the participants with a harness attached to a partial weight-bearing support system to prevent them from sudden falls as half of our participants have mobility impairments. Both the harness and the suspension system were from (Kaye Products Inc, 2020).
The VR system was designed using Unity 5, a multiplatform game development system from Unity Technologies. The fully immersive VEs were rendered using an Oculus Rift DK2, an HMD developed by Oculus VR. The Oculus Rift DK2 has a resolution of 960 × 1,080 pixels per eye with a refresh rate of 60 Hz and a 100° field of view (FoV). Microsoft Kinect 2V tracked the movement of the participants using the depth sensor. Kinect 2V has a depth resolution of 512 × 424 pixels with a 30 Hz refresh rate and 70 × 60 FoV.
A high-performance computer generated the VEs and recorded the data of the experiment. The system was equipped with Intel Core i7 processor (2.50 GHz), 16 GB DDR3 RAM, NVIDIA GeForce GTX 860M graphics card with 2 GB of dedicated video memory, and a Windows 8.1 Pro operating system.
3.2.4 Virtual Environment
We developed a VE that was a virtual representation of the room where the experiment took place. According to previous studies, participants feel a higher presence in a VE if the VE is similar to the surrounding physical room (Bouchard et al., 2006). Figure 2 shows the VE with the SRF. In our VR system, participants moved their upper body to move an avatar’s upper body in the VE. Participants’ joints from the hips up were tracked by a Kinect depth sensor, and the avatar’s respective joints replicated the participants’ movement. As the participant’s lower body remained in a fixed position during the game and the Kinect joint data for the lower body were less accurate, the lower body from the hips down was not tracked. Figure 3 shows a participant’s view when he looks down to see his body and extends his arm to experience the responsiveness of the avatar’s hand movement.
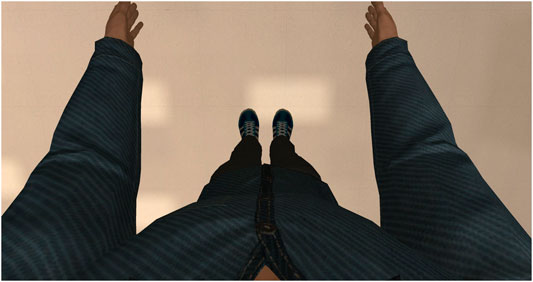
FIGURE 3. Participants’ view of their avatar when they look down and extend their arms to experience responsiveness of the avatar arms with the movement of their arms.
In FB-SRF and FB-NoSRF conditions, participants played a dodgeball game. There was a virtual bowling machine that shot virtual tennis balls toward a participant’s head. Participants lean on left or right while maintaining their balance to avoid the balls from hitting them in the face. The virtual bowling machine shot one ball every 1.5 s, and the ball took 1 s to travel from the bowling machine to the participant’s head. The game determined the position of the participant’s head using Kinect at the time of the shooting and shot the ball in the direction of the participant’s head. As the participant just dodged the previous ball by leaning on one side (left or right), the next ball will go toward that side (left or right). Therefore, the participant had to move to the other side to dodge the next ball. To break this rhythm, after shooting ten balls, the virtual machine paused for 1 s before shooting another set of ten balls. Participants heard different auditory feedback based on hit or miss. Figure 1 shows a participant playing the game. The game showed the scores (e.g., number of hits and misses) and information about the game on the wall of the virtual room. The total duration of the game was 120 balls. We used tennis balls that were well-textured, randomly rotated around their own axis, and illuminated using spotlights. This was done to facilitate depth perception in the game. A real-time reflection probe in the VE also increased depth perception by changing the lighting of the floor with the movement of participants. Participants played the game in two conditions, with the SRF and without the SRF. Figure 4 shows a virtual bowling machine, which is shooting tennis balls in a VE without the SRF condition. The frame rate of the game was approximately 60 FPS.
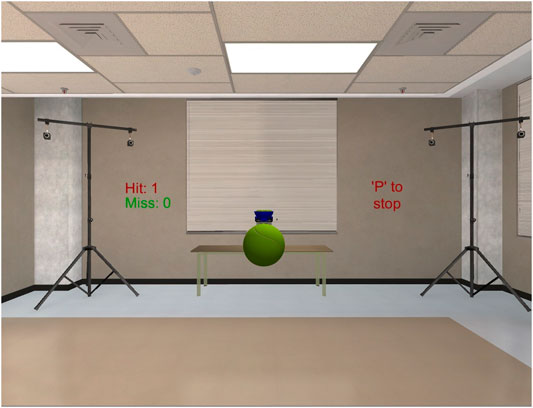
FIGURE 4. Bowling machine is shooting balls toward the head of the participants. This VE does not have the SRF.
3.2.5 Study Procedure
The study procedure consisted of the following five consecutive steps.
3.2.5.1 Consent and Prestudy Questionnaire
A participant started the study by signing a consent form, filling out an Activities-Specific Balance Confidence (ABC) (Powell and Myers, 1995) and a SSQ.
3.2.5.2 Baseline Data Measurement
We measured two baseline balance data conditions in the study: EO and EC. Participants stood quietly on a Wii Fit Balance Board, gazed straight ahead with eyes open. Their balance data were measured for 40 s. The same process was repeated for the EC condition. Participants were supported by a harness the whole time. The order of the EO and EC was counterbalanced. Between the two conditions, participants were released from the harness, and they sat for at least 1 min to rest.
3.2.5.3 Quiet Stance Balance Measurement
The process for measuring the QSB is the same as the EO balance. Instead of observing the real room, participants observed the virtual representation of the room using an HMD. This process was repeated with and without the SRF, and their order is counterbalanced. In these conditions, their balance data were measured with the Wii balance board for 40 s. Again, they rested for at least 1 min between the conditions.
3.2.5.4 Functional Balance Measurement
To measure FB, we instructed the participants to play the virtual dodge ball game (see Section 3.2.2). First, there was a 1 min training mode for the game. The purpose of the game is to make the participants comfortable with the gameplay. Once they were comfortable with the game, data measurement began while they played the game for 3 min. This process was repeated with and without the SRF, and their order is counterbalanced. One-minute rest was given between the conditions.
3.2.5.5 Poststudy Questionnaire
Participants ended the study by filling out an SSQ followed by an ABC. They received a payment of $50 after that. The average duration of the whole study for a single participant was 45 min.
3.3 Metrics
3.3.1 Imbalance Count
Previous studies have shown that to get valid balance data from a Wii balance board, we should sample the data at 10 Hz (Salavati et al., 2009). Following this guideline, we obtained pressure data from four pressure sensors of the Wii balance board at a 10 Hz sample rate. Calculating the average of these pressure sensors’ data, we got the participants’ weight distribution data. As participants were instructed to maintain their balance, their weight in any sample is expected to be within three standard deviations from the mean, assuming that their weight data are normally distributed. Moreover, 99.7 percent of the data were within three standard deviations away from the mean in a normal distribution. Therefore, if the weight data in any sample are more than three standard deviations away from the mean, we consider the participant to be imbalanced. However, if two or more consecutive sample data points show imbalance (e.g., balance data are three standard deviations away in both samples from 10.1 s to 10.2 s), we count it as one
The total count of these imbalances gives us a measurement of a participant’s balance in VR. The following equation shows how to calculate an
The imbalances were counted for 150 s (from 15th second to 165th second). The first 15 s and last 15 s of each session were excluded from the result as data can be more error-prone at the beginning and the end of the study. Participants were fit enough to work for a full day and rested well between conditions. Therefore, the imbalance count was minimally affected by fatigue.
3.3.2 Center of Pressure Path
Center of Pressure (COP) was calculated from four weight sensors in the Wii board using the following equation developed by Young et al. (2011):
where
where
3.3.3 Simulator Sickness Questionnaire
Simulator Sickness Questionnaire (SSQ) is a sixteen-item questionnaire where each item asks about participants’ different physiological discomfort (Kennedy et al., 1993). Each item can be rated from
where
3.3.4 Activities-Specific Balance Confidence Scale
Activities-Specific Balance Confidence Scale (ABC) is a sixteen-item questionnaire where each item asks about participants’ confidence in doing a specific daily life activity (Powell and Myers, 1995). Each item can be rated from 0% to 100%, where 0% is
3.3.5 Hit Count (Game Performance)
Hit count denotes how many times any participant failed to dodge the ball while they were playing the game.
3.4 Statistical Analysis
We compared the FB-NoSRF data with the FB-SRF data of both groups. We also compared both groups’ QSB-SRF and QSB-NoSRF data with EO baseline data. For FB data, we only considered the data for 150 s (from 15th second to 165th second), whereas for QSB data, we considered the data for 30 s (from the 5th second to 35th second). All the statistical analyses were performed using IBM SPSS version 19. We used a Mixed Model ANOVA and then used one-tailed paired sample t-tests with p values adjusted with Bonferroni correction as needed for post hoc analysis of within-group comparisons. For between-group comparisons, we used independent sample t-tests.
3.5 Results
3.5.1 Imbalance Count
We ran a paired sample t-test between the Imbalance Count of FB-SRF and FB-NoSRF conditions for both participants with MS and participants without impairments. For participants with MS, the result shows a significantly improved balance in FB-SRF (M = 11.28, SD = 2.62) to FB-NoSRF (M = 14.57, SD = 2.99) condition; t(6) = 2.02, p = 0.045. However, for participants without impairment, there is no significant difference between FB-SRF (M = 14.57, SD = 4.85) and FB-NoSRF (M = 12.42, SD = 3.90) conditions; t(6) = 1.205, p = 0.137. It is worth mentioning that the imbalance count of participants without impairment is not negligible as all of them are elderly persons, and postural instability increases with age (Abrahamova and Hlavacka, 2008).
Figure 5 shows the change of imbalance count of participants with MS in FB-SRF and FB-NoSRF conditions. Most of the participants with MS showed improvement in their balance in the FB-SRF condition.
3.5.2 Center of Pressure Path
We ran an independent sample t-test on participants’ QSB-NoSRF COP path and baseline EO COP path. The between-group results show that participants with MS (M = 36.83, SD = 16.79) do not have a significantly different EO COP path compared to the participants without impairment (M = 24.37, SD = 6.63) (t(12) = 1.82, p = 0.093). However, participants with MS (M = 42.87, SD = 21.56) have a significantly more deficient QSB-NoSRF than that of participants without impairment (M = 23.69, SD = 5.93) (t(12) = 2.27, p = 0.043).
Figure 6 shows that the mean COP path of QSB-NoSRF is larger in participants with MS than that of participants without impairment. However, the mean COP path from EO to QSB-NoSRF increased more for the participants with MS compared to the participants without impairment.
3.5.3 Simulator Sickness Questionnaire
We ran an independent sample t-test on different
3.5.4 ABC Questionnaire
For participants with MS, the paired sample t-test shows a significant improvement from preexposure ABC (M = 67.27, SD = 19.57) to postexposure ABC score (M = 70.71, SD = 19.93) (t(6) = 2.81, p = 0.015). However, for participants without impairment, we did not notice any significant difference from preexposure ABC (M = 96.25, SD = 3.49) to postexposure ABC score (M = 96.07, SD = 3.65) (t(6) = 1.55, p = 0.086).
3.5.5 Hit Count (Game Performance)
Paired sample t-test between the hit count with the SRF and without the SRF in the VE shows that hit counts do not have a significant difference between with the SRF and without the SRF conditions for both participants with MS (t(6) = 0.927, p = 0.39) and participants without impairment (t(6) = 0.717, p = 0.5). Table 3 shows the mean (SD) and the significance level of hit count with the SRF and without the SRF conditions for participants with MS and participants without impairment.
3.6 Discussion
3.6.1 Effect of SRF on Balance
Results from
3.6.2 Balance Comparison Between the Virtual and Real World
Our results from the
3.6.3 Cybersickness
We did not find any significant differences in SSQ result other than for postexposure disorientation. The participants with MS had a little high preexposure SSQ (34.19 out of 235.62). It is not surprising since they are older adults (average age 51) with MS. For all the participants, the postexposure SSQ is very close to preexposure SSQ, and the difference is nonsignificant. The reason behind this could be that the SRF helps to reduce cybersickness in VR (Prothero, 1998; Chang E et al., 2013). In half of the time of the exposure, the VE had an SRF and did not generate any cybersickness. Therefore, this study setup was not suitable to find the effect of MS on cybersickness, and we cannot accept hypothesis 3. We plan to investigate hypothesis three in the future with a VE that induces more cybersickness by producing more sensory conflicts.
3.6.4 Confidence in Balance from VR Game
We have an interesting finding of the significant improvement of confidence in balance, based on the results from the ABC questionnaire. Note that this improvement results from playing the VR game only for 7–8 min. This is a very short time for actual improvement in balance. This raises the question of whether their confidence in balance is actually improved or this is just a temporary improvement after playing the game. A VR game with 30 min duration has been shown to be effective in improving short-term balance for persons with MS (Kalron and Frid, 2012). However, the minimum time required for an improvement in short-term balance is still unknown. We plan to investigate this in the future.
3.6.5 Effect of SRF on Game Performance
We did not find any significant difference in the hit counts between with the SRF and without the SRF conditions for both participants with MS and participants without impairment. This suggests that adding an SRF does not affect the game performance significantly.
4 Effect of Static Rest Frame in Augmented Reality
After getting positive results from our SRF in the VR study, we conducted a follow-up study to investigate the effects of an SRF in AR. The following subsections describe our AR study in detail.
4.1 Hypotheses
The goal of this study is to investigate the effect of an SRF on balance in AR. Based on the results we obtained from the previous VR study and the known advantages of SRFs from the literature, the following hypotheses are to investigate the effects of SRFs on balance in AR:
H1: An SRF will significantly improve balance in AR for users with balance impairments.
H2: Users with balance impairment will have a better balance in AR than VR.
H3: Persons with balance impairment experience less cybersickness in AR than VR.
4.2 Methods
4.2.1 Participants and Selection Criteria
We recruited the same seven participants with MS that we recruited for the VR study described in Section 3.2.1. However, the AR study was done after the VR study, and some of the participants had a slightly increased EDSS (Kurtzke, 1983) score. Therefore, the EDSS range was from 4.0 to 6.0. No participant had any problem in participating in the study, and nobody reported any fatigue. Table 4 shows the mean, standard deviation (SD), weight, EDSS, and other detailed information about the participants.
4.2.2 Study Conditions
The study conditions were similar to the VR study described in Section 3.2.2. The only difference is that in QSB and FB conditions, the virtual model of the physical room is removed from the scene as participants can see the physical room in the AR simulation. The virtual bowling machine (see Section 3.2.4) was present in the environment.
4.2.3 System Description
To keep the studies comparable, we used the same hardware that we used for balance measurement, Nintendo Wii Fit Balance Board. We also used the same harness that we used in the earlier study to support the participants. The game was designed using the same version of Unity. The only difference was instead of Oculus Rift DK2, we used (Microsoft, 2020) to provide the augmented reality experience. Microsoft HoloLens has a 30 × 17.5° FoV (Kreylos, 2017). It has a refresh rate of 60 Hz and a maximum supported resolution of 1,268 × 720 pixels per eye. The same configuration computer was used to record the data.
4.2.4 Augmented Environment
The augmented environment is similar to the VE described in Section 3.2.4. However, as the participant can see the physical room, the virtual model of the room was not a part of the augmented environment. There was no avatar in the augmented environment as participants can see their own bodies in AR. In short, the only augmented components were the bowling machine parts, the scores, and the SRF (depending on the study conditions). Figure 7 shows the augmented environment with the SRF. It may appear that the FoV in Figure 7 is much smaller than the FoV in Figure 2. For the VR study (Figure 2), the picture was taken from a screenshot from Unity, and for the AR study (Figure 7), the picture was a screenshot from a video recording using HoloLens. However, the ball machine’s distance, size, and ball’s speed were the same if looked through the headsets.
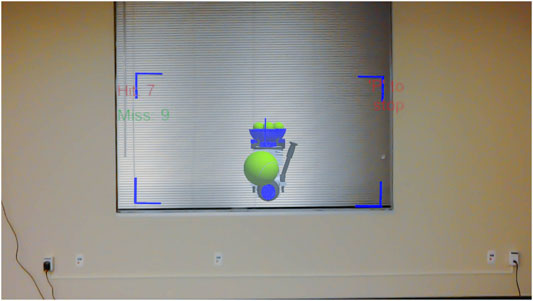
FIGURE 7. Bowling machine is shooting balls toward the head of the participants. This augmented environment has SRF.
In FB conditions, participants played the same dodge ball game where they dodge virtual tennis balls coming toward their heads. Figure 8 shows a participant playing the game in AR. The size and speed of the balls, the distance from where the balls are coming from, the interval between two balls, and the pause between sets of ten balls were the same as in the VR study. The scores were shown in a similar fashion to the previous study. The audio feedback for hit or miss was similar to the VR study. Figure 8 shows the virtual machine shooting virtual tennis balls in AR.
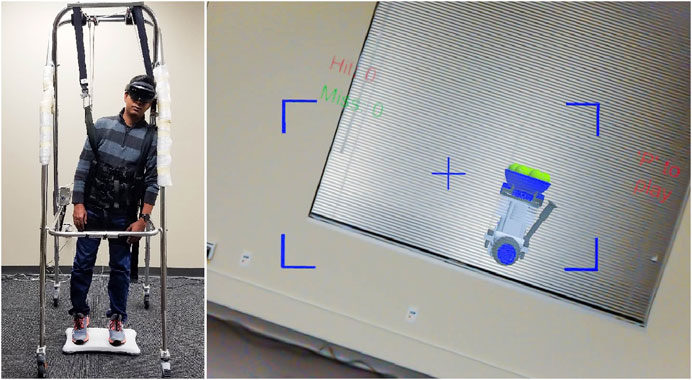
FIGURE 8. A participant playing the dodge ball game and his view in the AR with an SRF as he shifts his balance to the left. The figure shows an author who is posing as a participant. He gave consent to publish this image.
4.2.5 Study Procedure
The study procedure for the AR study was identical to the VR study with the following five steps:
1. Consent and Prestudy Questionnaire
2. Baseline Data Measurement
3. Quiet Stance Balance Measurement
4. Functional Balance Measurement
5. Poststudy Questionnaire
Please see Section 3.2.5 for more details.
4.3 Metrics
We have used four metrics that are the same as the metrics we used for the VR study. Please see Section 3.3 for more details. The metrics that are the same as the VR study are as follows:
1. Imbalance Count
2. Center of Pressure path
3. Simulator Sickness Questionnaire
4. Activities-Specific Balance Confidence Scale
In addition to these metrics mentioned above, we have added the following metrics to compare the impact of VR and AR on balance:
5. Impact Ratio: Impact Ratio is the quotient between QSB and EO balance. It is motivated by Romberg ratio, where the quotient between EO and EC balance is taken as a measurement of visual dependency of balance (Kalron, 2017). For our study, we have taken the quotient between QSB-NoSRF and EO balance to normalize the effect of EO balance on QSB-NoSRF. The equation for calculating the impact ratio is as follows:
4.4 Statistical Analysis
We compared the FB-NoSRF data of participants with MS with FB-SRF data. We also compared their QSB-NoSRF and QSB-SRF data with their baseline EO data. Furthermore, to compare between studies (VR vs. AR), we compared the impact ratio, SSQ, and ABC data. All the statistical analyses were performed using IBM SPSS version 19. We used a Mixed Model ANOVA and then used one-tailed paired sample t-tests with p values adjusted with Bonferroni correction as needed for post hoc analysis of within-group comparisons. For between-study comparisons, we used independent sample t-tests.
4.5 Results
4.5.1. Imbalance Count
We ran a paired sample t-test and found out that there is a significant difference in imbalance count for FB-NoSRF (M = 13.71, SD = 6.77) and FB-SRF conditions (M = 10.28, SD = 5.79); t(6) = 2.58, p = 0.02. Figure 9 shows the change of imbalance count of participants in FB-NoSRF and FB-SRF conditions. Most of the participants showed improvement in their balance with the presence of the SRF.
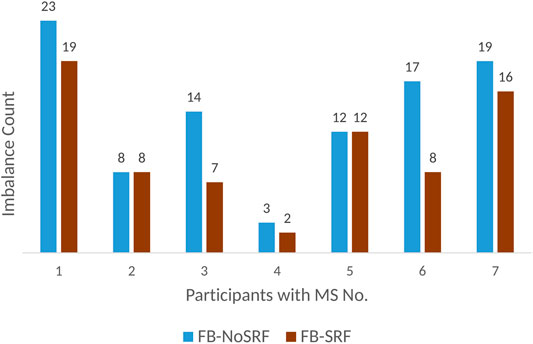
FIGURE 9. Imbalance count of participants with MS in FB-NoSRF and FB-SRF conditions in the AR study.
4.5.2 Center of Pressure Path
We ran a paired sample t-test and discovered no significant difference in COP path for QSB-NoSRF (M = 36.17, SD = 16.60) and EO (M = 44.26, SD = 16.60); t(6) = 1.502, p = 0.092. Similarly, we did not find any significant difference in paired sample t-test in COP path for QSB-SRF (M = 31.85, SD = 10.75) and EO (M = 44.26, SD = 16.60); t(6) = 1.481, p = 0.094. Figure 10 shows how mean COP path changes in different study conditions in augmented reality.
For the between-study comparison, we compared their impact ratio as we have conducted the study at two different times and the participants had different EO balances. The impact ratio normalizes the effect of EO balance on QSB. We conducted a paired sample t-test between the impact ratio of VR and AR study and discovered significant differences in impact ratio between VR (M = 1.18, SD = 0.29) and AR (M = 0.85, SD = 0.25) study; t(6) = 2.5, p = 0.023. Figure 11 shows the comparison of the impact ratio of participants between VR and AR study.
4.5.3 Simulator Sickness Questionnaire
We ran a paired sample t-test on different SSQ subscales and total SSQ scores with before and after AR study data. We also ran an independent sample t-test between VR study and AR study data. However, there is no significant difference in any of the subscales or total SSQ scores in any test.
4.5.4 ABC Questionnaire
We ran a paired sample t-test on ABC score with before and after AR study data. We also ran an independent sample t-test between VR study and AR study data. However, there is no significant difference in ABC score within or between study data.
4.6. Discussion
4.6.1. Effect of SRF on Balance
Statistical results from imbalance count also suggest that SRF is beneficial to improve balance in AR as well as in VR. Therefore, we can accept hypothesis 1: a SRF will significantly improve balance in AR for users with balance impairments. The reason may be similar to the reason for balance improvement in VR that a person needs an SRF to make proper judgments of object movement (Prothero, 1998).
4.6.2 Balance Comparison Between Augmented and Virtual Reality
Unlike VR, participants’ peripheral vision is not blocked in AR. Therefore, they can see the real world as well as the ball machine in the scene. The ball machine provides extra visual feedback to maintain their balance. The reason may be that vision is the most contributing factor in balance and AR is not blocking the periphery (Hansson et al., 2010). Moreover, optical see-through AR does not introduce latency for the real objects. QSB-SRF is also smaller than EO for all six of the participants who showed decreased COP path in QSB. However, there were no significant differences found in this comparison, possibly due to our small sample size. Future studies with larger samples are needed to investigate this.
There is a significant difference observed between the impact ratio of VR and AR. Therefore, we can accept hypothesis 2: users with balance impairment will have a better balance in AR than VR. The reason could be the presence of peripheral vision feedback from the real world in AR—vision is the most contributing factor in balance (Hansson et al., 2010). Unlike VR, participants’ peripheral vision is not blocked in AR. Therefore, they can see the real world as well as the ball machine in the scene. Their balances were not affected by the presence of the ball machine. Their balance is further improved in the QSB-SRF condition. Therefore, it is possible that the ball machine and the SRF provided them with more references that they already had in the real world to improve their balance.
4.6.3 Cybersickness
There were no significant differences observed in any of the subscales or the total SSQ score between VR and AR conditions. One reason can be the VE was simple, and there were not many changes in the visual information. Another reason can be that the duration of the VR exposure was short, and it was under the recommended time of 20 min (Kennedy et al., 2000). Therefore, for the lack of enough evidence, we cannot remark on cybersickness, and we cannot accept hypothesis 3: persons with balance impairment experience less cybersickness in AR than VR.
4.7 Study Limitations
There are some limitations to our study. There is a difference in the FoV between Oculus and HoloLens (see Section 3.2.3 and Section 4.2.3 for more details). The reason for our limitations is that there was no optical see-through AR display available that had a comparable FoV to the popular VR HMDs. In the future, similar studies can be conducted with video see-through HMDs that have been improving recently.
Another limitation of our study is that all of the participants with MS were females. The reason for this is that MS is three times more common in women than in men (MS-Society, 2020). We can expect to see similar results if we had male participants since postural stability does not depend on gender (Hageman et al., 1995).
We have some nonsignificant results, especially in SSQ. This can possibly be due to the small sample size. However, the main objective of this study is focused on postural stability, not cybersickness. Since our sample size achieved significant results for postural stability comparison, we did not increase our sample size. Another reason can be that SSQ is a subjective measurement and often can be affected by personal preference. That is, two persons with similar cybersickness may report differently in their SSQ questionnaire. We plan to include objective measures (e.g., heart rate variability (Zużewicz et al., 2011) and galvanic skin response (Kennedy et al., 2010)), in addition to SSQ, to have a comprehensive set of metrics for cybersickness analysis in our future studies.
5 Conclusion
The objective of our research is to improve the postural stability in VR and AR for users with balance impairments. To obtain a feasible solution, we investigated the effect of an SRF on the QSB and the FB of persons with MS in VR and AR. Our results suggest that the inclusion of an SRF will improve the balance for persons with balance impairments, while it has no significant impact on the balance of persons without impairment. Therefore, an option for adding an SRF will make VR that uses fully immersive HMDs more accessible. Our results show both VR and AR benefit from an SRF.
In the future, we plan to investigate how different types of visual feedback can affect balance and gait in VR and AR. The scope of this study was to analyze standing balance and functional balance while standing, as many of the rehabilitation games (e.g., Wii games) involve playing while you are standing in the same place. However, some games involve real walking. In the future, we also plan to investigate the effect of SRF on persons’ gait and how it differs in persons with MS. As the SRF blocks a subtle portion of the environment, it may have some effect on presence. Thus, we also plan to investigate the SRF’s impact on presence in the future. There are many VR-based rehabilitation exercises that are proved to be improving balance (Fulk, 2005; Nilsagård et al., 2013). It would be interesting to study in the future if adding an SRF in the VE can make VR rehabilitation more effective by reducing rehabilitation time.
Data Availability Statement
The raw data supporting the conclusions of this article will be made available by the authors without undue reservation.
Author Contributions
SF is the primary author of this article. He designed, developed the system, ran human subject studies, analyzed the data, and wrote the manuscript. TC and IA worked on the development and testing of the system as well as running human subject studies. JQ is their supervisory author who helped to edit the manuscript.
Funding
This work was supported by grants from the National Science Foundation under Grant No. IIS-1350995.
Conflict of Interest
The authors declare that the research was conducted in the absence of any commercial or financial relationships that could be construed as a potential conflict of interest.
Acknowledgments
The content of this manuscript has been presented partially at the IEEE Symposium on 3D User Interfaces (3DUI) (Ferdous et al., 2016). The authors would also like to thank the participants in their studies.
Abbreviations
ABC, Activities-Specific Balance Confidence; AR, Augmented Reality; EC, Eyes Closed; EO, Eyes Open; EDSS, Expanded Disability Status Scale; FB, Functional Balance; QSB, Quiet Stance Balance; SRF, Static Rest Frame; SSQ, Simulator Sickness Questionnaire; VE, Virtual Environment; VR, Virtual Reality.
References
Abrahamová, D., and Hlavacka, F. (2008). Age-related changes of human balance during quiet stance. Physiol. Res. 57, 957.
Bakshi, R. (2003). Fatigue associated with multiple sclerosis: diagnosis, impact and management. Mult. Scler. 9, 219–227. doi:10.1191/1352458503ms904oa |
Banou, E. (2015). Kinesia paradoxa: a challenging Parkinson’s phenomenon for simulation. Adv. Exp. Med. Biol. 822, 165–177. doi:10.1007/978-3-319-08927-0_18 |
Baram, Y., and Miller, A. (2006). Virtual reality cues for improvement of gait in patients with multiple sclerosis. Neurology. 66, 178–181. doi:10.1212/01.wnl.0000194255.82542.6b |
Berg, K., Wood-Dauphine, S., Williams, J., and Gayton, D. (1989). Measuring balance in the elderly: preliminary development of an instrument. Physiother. Can. 41, 304–311. doi:10.3138/ptc.41.6.304
Bisson, E., Contant, B., Sveistrup, H., and Lajoie, Y. (2007). Functional balance and dual-task reaction times in older adults are improved by virtual reality and biofeedback training. Cyberpsychol. Behav. 10, 16–23. doi:10.1089/cpb.2006.9997 |
Bouchard, S., Dumoulin, S., Chartrand-Labonté, G., Robillard, G., and Renaud, P. (2006). “Perceived realism has a significant impact on presence,” in Proceedings of the 11th annual CyberTherapy 2006 conference: virtual healing—designing reality.
Champney, R. K., Stanney, K. M., Hash, P. A., Malone, L. C., Kennedy, R. S., and Compton, D. E. (2007). Recovery from virtual environment exposure: expected time course of symptoms and potential readaptation strategies. Hum. Factors. 49, 491–506. doi:10.1518/001872007X200120 |
Chang, E., Hwang, I., Jeon, H., Chun, Y., Kim, H. T., and Park, C. (2013). Effects of rest frames on cybersickness and oscillatory brain activity, 62–64.
Chang, W. D., Chang, W. Y., Lee, C. L., and Feng, C. Y. (2013). Validity and reliability of wii fit balance board for the assessment of balance of healthy young adults and the elderly. J. Phys. Ther. Sci. 25, 1251. doi:10.1589/jpts.25.1251
Clark, R. A., Bryant, A. L., Pua, Y., McCrory, P., Bennell, K., and Hunt, M. (2010). Validity and reliability of the nintendo wii balance board for assessment of standing balance. Gait Posture. 31, 307–310. doi:10.1016/j.gaitpost.2009.11.012 |
Corporaal, S. H., Gensicke, H., Kuhle, J., Kappos, L., Allum, J. H., and Yaldizli, Ö. (2013). Balance control in multiple sclerosis: correlations of trunk sway during stance and gait tests with disease severity. Gait Posture. 37, 55–60. doi:10.1016/j.gaitpost.2012.05.025 |
Espay, A. J., Baram, Y., Dwivedi, A. K., Shukla, R., Gartner, M., Gaines, L., et al. (2010). At-home training with closed-loop augmented-reality cueing device for improving gait in patients with Parkinson disease. J. Rehabil. Res. Dev. 47, 573. doi:10.1682/jrrd.2009.10.0165
Ferdous, S. M. S., Arafat, I. M., and Quarles, J. (2016). “Visual feedback to improve the accessibility of head-mounted displays for persons with balance impairments,” in 3D user interfaces (3DUI), 2016 IEEE symposium on (Greenville, SC, USA: IEEE), 121–128.
Fulk, G. D. (2005). Locomotor training and virtual reality-based balance training for an individual with multiple sclerosis: a case report. J. Neurol. Phys. Ther. 29, 34–42. doi:10.1097/01.npt.0000282260.59078.e4
Guo, R., Samaraweera, G., and Quarles, J. (2014). Mobility impaired users respond differently than healthy users in virtual environments. Comput. Animation Virtual Worlds. 26, 509–526. doi:10.1002/cav.1610
Hageman, P. A., Leibowitz, J. M., and Blanke, D. (1995). Age and gender effects on postural control measures. Arch. Phys. Med. Rehabil. 76, 961–965. doi:10.1016/s0003-9993(95)80075-1 |
Hansson, E. E., Beckman, A., and Håkansson, A. (2010). Effect of vision, proprioception, and the position of the vestibular organ on postural sway. Acta Otolaryngol. 130, 1358–1363. doi:10.3109/00016489.2010.498024 |
Horlings, C. G., Carpenter, M. G., Küng, U. M., Honegger, F., Wiederhold, B., and Allum, J. H. (2009). Influence of virtual reality on postural stability during movements of quiet stance. Neurosci. Lett. 451, 227–231. doi:10.1016/j.neulet.2008.12.057 |
Jones, J., Swan, J. E., and Bolas, M. (2013). Peripheral stimulation and its effect on perceived spatial scale in virtual environments. IEEE Trans. Visual. Comput. Graph. 19, 701–710. doi:10.1109/TVCG.2013.37
Kalron, A. (2017). The romberg ratio in people with multiple sclerosis. Gait Posture. 54, 209–213. doi:10.1016/j.gaitpost.2017.03.016 |
Kalron, A., and Frid, L. (2012). Nintendo wii virtual reality game improves short term balance capabilities in multiple sclerosis patients: a pilot quasi-experimental study. J. Phys. Ther. 5, 54–62.
Kaminsky, T. A., Dudgeon, B. J., Billingsley, F. F., Mitchell, P. H., and Weghorst, S. J. (2007). Virtual cues and functional mobility of people with Parkinson’s disease: a single-subject pilot study. J. Rehabil. Res. Dev. 44, 437. doi:10.1682/jrrd.2006.09.0109
Kaye products inc (2020). Kaye products. Available at: http://kayeproducts.com/. (Accessed November 11, 2020).
Kennedy, R. S., Drexler, J., and Kennedy, R. C. (2010). Research in visually induced motion sickness. Appl. Ergon. 41, 494–503. doi:10.1016/j.apergo.2009.11.006 |
Kennedy, R. S., and Stanney, K. M. (1996). Postural instability induced by virtual reality exposure: development of a certification protocol. Int. J. Hum. Comput. Interact. 8, 25–47. doi:10.1080/10447319609526139
Kennedy, R. S., Lane, N. E., Berbaum, K. S., and Lilienthal, M. G. (1993). Simulator sickness questionnaire: an enhanced method for quantifying simulator sickness. Int. J. Aviat. Psychol. 3, 203–220. doi:10.1207/s15327108ijap0303_3
Kennedy, R. S., Stanney, K. M., and Dunlap, W. P. (2000). Duration and exposure to virtual environments: sickness curves during and across sessions. Presence Teleoperators Virtual Environ. 9, 463–472. doi:10.1162/105474600566952
Kenney, R. S., Berbaum, K. S., and Lilienthal, M. G. (1997). Disorientation and postural ataxia following flight simulation. Aviat Space Environ. Med. 68, 13–17.
Kurtzke, J. F. (1983). Rating neurologic impairment in multiple sclerosis: an expanded disability status scale (EDSS). Neurology. 33, 1444–1452. doi:10.1212/wnl.33.11.1444 |
LaViola, J. J. (2000). A discussion of cybersickness in virtual environments. ACM SIGCHI Bull. 32, 47–56. 10.1145/333329.333344
Lee, D., and Lishman, J. (1975). Visual proprioceptive control of stance. J. Hum. Mov. Stud. 1, 87–95.
Lord, S. R. (2006). Visual risk factors for falls in older people. Age Ageing. 35 (Suppl. 2), ii42–ii45. doi:10.1093/ageing/afl085 |
Lozano-Quilis, J., Gil-Gomez, H., Gil-Gómez, J., Albiol-Perez, S., Palacios, G., Fardoum, H. M., et al. (2013). “Virtual reality system for multiple sclerosis rehabilitation using kinect,” in Pervasive computing technologies for healthcare (PervasiveHealth), 2013 7th international conference on (Venice, Italy: IEEE), 366–369.
Microsoft (2020). Microsoft hololens—mixed reality technology for business. Available at: https://www.microsoft.com/en-us/hololens (Accessed November 11, 2020).
Moreno, R., and Mayer, R. E. (2002). Learning science in virtual reality multimedia environments: role of methods and media. J. Educ. Psychol. 94, 598–610. doi:10.1037/0022-0663.94.3.598
Ms-Society (2020). Who gets ms? — national multiple sclerosis society. Available at: https://www.nationalmssociety.org/What-is-MS/Who-Gets-MS 11 (Accessed November 11, 2020), [Dataset].
Newton, R. A. (2001). Validity of the multi-directional reach test: a practical measure for limits of stability in older adults. J. Gerontol. A Biol. Sci. Med. Sci. 56, M248–M252. doi:10.1093/gerona/56.4.m248
Nilsagård, Y. E., Forsberg, A. S., and von Koch, L. (2013). Balance exercise for persons with multiple sclerosis using wii games: a randomised, controlled multi-centre study. Multiple Scler. J. 19, 209–216. doi:10.1177/1352458512450088
Oculus (2020). Oculus rift: Vr headset for vr ready pcs—oculus. Available at: http://www.oculusvr.com/rift (Accessed November 11, 2020).
Powell, L. E., and Myers, A. M. (1995). The activities-specific balance confidence (abc) scale. J. Gerontol. A Biol. Sci. Med. Sci. 50A, M28–M34. doi:10.1093/gerona/50a.1.m28
Prothero, J. D. (1998). The role of rest frames in vection, presence and motion sickness. Doctoral dissertation. University of Washington, CUMINCAD.
Riccio, G. E., and Stoffregen, T. A. (1991). An ecological theory of motion sickness and postural instability. Ecol. Psychol. 3, 195–240. doi:10.1207/s15326969eco0303_2
Rougier, P., Faucher, M., Cantalloube, S., Lamotte, D., Vinti, M., and Thoumie, P. (2007). How proprioceptive impairments affect quiet standing in patients with multiple sclerosis. Somatosens. Mot. Res. 24, 41–51. doi:10.1080/08990220701318148 |
Salavati, M., Hadian, M. R., Mazaheri, M., Negahban, H., Ebrahimi, I., Talebian, S., et al. (2009). Test-retest reliability [corrected] of center of pressure measures of postural stability during quiet standing in a group with musculoskeletal disorders consisting of low back pain, anterior cruciate ligament injury and functional ankle instability. Gait Posture. 29, 460–464. doi:10.1016/j.gaitpost.2008.11.016 |
Samaraweera, G., Guo, R., and Quarles, J. (2015). “Applying latency to half of a self-avatar’s body to change real walking patterns,” in Proceedings of IEEE symposium on virtual reality (VR) (Arles, Camargue, Provence, France: IEEE), 89–96.
Samaraweera, G., Guo, R., and Quarles, J. (2013). “Latency and avatars in virtual environments and the effects on gait for persons with mobility impairments,” in 3D user interfaces (3DUI), 2013 IEEE symposium on (Orlando, FL, USA: IEEE), 23–30.
Sveistrup, H., McComas, J., Thornton, M., Marshall, S., Finestone, H., McCormick, A., et al. (2003). Experimental studies of virtual reality-delivered compared to conventional exercise programs for rehabilitation. Cyberpsychol. Behav. 6, 245–249. doi:10.1089/109493103322011524 |
Tinetti, M. E. (1986). Performance-oriented assessment of mobility problems in elderly patients. J. Am. Geriatr. Soc. 34, 119–126. doi:10.1111/j.1532-5415.1986.tb05480.x
Van Emmerik, R., Remelius, J., Johnson, M., Chung, L., and Kent-Braun, J. (2010). Postural control in women with multiple sclerosis: effects of task, vision and symptomatic fatigue. Gait Posture. 32, 608–614. doi:10.1016/j.gaitpost.2010.09.002 |
Young, W., Ferguson, S., Brault, S., and Craig, C. (2011). Assessing and training standing balance in older adults: a novel approach using the ‘Nintendo Wii’ Balance Board. Gait Posture. 33, 303–305. doi:10.1016/j.gaitpost.2010.10.089 |
Keywords: balance, postural stability, accessibility, multiple sclerosis, cybersickness, virtual reality, augmented reality, head-mounted display
Citation: Shahnewaz Ferdous SM, Chowdhury TI, Arafat IM and Quarles J (2021) Static Rest Frame to Improve Postural Stability in Virtual and Augmented Reality. Front. Virtual Real. 1:582169. doi: 10.3389/frvir.2020.582169
Received: 10 July 2020; Accepted: 21 December 2020;
Published: 23 February 2021.
Edited by:
Kay Marie Stanney, Design Interactive, United StatesReviewed by:
Roberto Champney, Louisiana State University, United StatesMark Billinghurst, University of South Australia, Australia
Copyright © 2021 Shahnewaz Ferdous, Chowdhury, Arafat and Quarles. This is an open-access article distributed under the terms of the Creative Commons Attribution License (CC BY). The use, distribution or reproduction in other forums is permitted, provided the original author(s) and the copyright owner(s) are credited and that the original publication in this journal is cited, in accordance with accepted academic practice. No use, distribution or reproduction is permitted which does not comply with these terms.
*Correspondence: Sharif Mohammad Shahnewaz Ferdous, c2hhcmlmLnNoYWhuZXdhekB0Y25qLmVkdQ==