- Affordance Perception-Action Laboratory, School of Kinesiology, University of Minnesota, Minneapolis, MN, United States
Motion sickness is common in virtual environments. The risk of motion sickness varies widely between individuals and across situations. The subjective experience of motion sickness often is preceded by distinctive patterns of movement in the control of head and body posture. Previous research has documented reliable sex differences in the kinematics of postural activity, as well as reliable differences in postural activity between participants who were in control of a virtual vehicle and participants who were not. We asked whether postural precursors of motion sickness would simultaneously be influenced by individual and situational factors. We analyzed movement of the head and torso while seated participants were exposed to a driving video game presented through a head-mounted display. Half of the participants were women, and half were men. Using a yoked-control design, half of the participants controlled the virtual vehicle (Drivers), whereas half watched previously recorded vehicle trajectories (Passengers). The maximum exposure duration was 15 min, but participants were instructed to discontinue participation immediately if they experienced any symptoms of motion sickness, however mild. We analyzed movement kinematics not only in terms of sex and vehicle control but also in terms of participants who did or did not report motion sickness. Movement differed between Drivers and Passengers, in terms of both the spatial magnitude and multifractality of movement. The spatial magnitude of movement was simultaneously influenced by sex (men vs. women) and vehicle control (Drivers vs. Passengers). In addition, in statistically significant interactions, we identified postural precursors of motion sickness that differed between Drivers and Passengers and, separately, between Drivers and Passengers as a function of sex. The results are consistent with a prediction of the postural instability theory of motion sickness etiology and shed new light on the multifactorial origins of postural precursors of motion sickness in virtual environments.
Introduction
Among users of interactive technologies, motion sickness is widely reported. For head-mounted displays (HMDs), this type of motion sickness is often referred to as cybersickness. Typically, the risk of motion sickness is greater during applications that feature virtual locomotion (i.e., movement of the observer relative to a virtual world) and is less common in applications that do not include virtual locomotion (e.g., Bruder et al., 2012; Munafo et al., 2017; Nilsson et al., 2018).
A common example of virtual locomotion is virtual driving. In many cases, users control virtual vehicles: they are drivers. In other cases, users merely observe the motion of virtual vehicles; in effect, they are passengers. Both physical and virtual vehicles are associated with the Driver–Passenger effect, in which the risk of motion sickness typically is greater for passengers than for drivers (e.g., Rolnick and Lubow, 1991; Dong et al., 2011). In this article, we report the final component of a larger study of sex differences in the driver–passenger effect in HMDs. Earlier reports presented data on the incidence and severity of motion sickness (Curry et al., 2020a) and on standing body sway prior to HMD exposure (Curry et al., 2020b). In the present article, our focus was on seated postural activity during exposure to a virtual vehicle presented through an HMD.
Postural Precursors of Motion Sickness During Exposure
The postural instability theory of motion sickness predicts that the quantitative kinematics of postural activity will differ between persons who state that they are motion sick and persons who state that they are not motion sick, and that these differences should exist before the onset of motion sickness (Riccio and Stoffregen, 1991). In the empirical literature, this prediction has been operationalized in terms of relations between quantitative measures of postural activity (i.e., continuous variables) and the incidence of motion sickness. In most tests, motion sickness incidence has been a dichotomous variable, with individual participants being classified as being either well or sick. Several studies have investigated the kinematics of postural activity during exposure to potentially nauseogenic motion. Most have used a method in which participants were instructed to discontinue immediately if they experienced any symptoms of motion sickness, however mild. This instruction is given repeatedly (e.g., during the consent process and before each exposure trial). In addition, participants are informed that they may discontinue participation at any time for any reason, and that there is no penalty for early discontinuation. These aspects of the design remove motivation for false positives (i.e., feigning motion sickness as an excuse to discontinue) and ensure that all postural data precede the onset of any subjective symptoms of motion sickness (e.g., Stoffregen and Smart, 1998; Dong et al., 2011; Stoffregen et al., 2017).
Using this method, researchers have identified postural precursors of visually induced motion sickness in laboratory devices (e.g., Stoffregen et al., 2010; Koslucher et al., 2014, 2016a; Li et al., 2018; Walter et al., 2019), in desktop virtual environments (e.g., Stoffregen et al., 2008, 2017; Dong et al., 2011; Chang et al., 2017), in handheld devices (Stoffregen et al., 2014), in projection video systems (e.g., Villard et al., 2008; Palmisano et al., 2018), and in HMDs (e.g., Merhi et al., 2007).
During exposure to virtual environments, postural activity evolves; that is, it changes over time. This effect has been documented in a wide variety of studies (e.g., Stanney et al., 1998; Stoffregen et al., 2010; Koslucher et al., 2016a). In a logically distinct effect, some studies have identified statistically significant interactions between the duration of virtual environment (VE) exposure and the subsequent development of motion sickness (e.g., Villard et al., 2008; Stoffregen et al., 2010, 2014; Koslucher et al., 2016a). We expected to replicate these empirical effects.
Sex Differences in Postural Precursors of Motion Sickness
A common observation is that susceptibility to motion sickness differs between the sexes. In both field research and in the laboratory, women typically are more susceptible than men (e.g., Lawther and Griffin, 1988; Koslucher et al., 2015). Separately, both laboratory and population studies have found that the kinematics of standing body sway differ between the sexes (e.g., Era et al., 2006; Kim et al., 2010). Recent research has revealed that these two effects are related; that is, that postural precursors of motion sickness are different for women and men, with differences that often are qualitative. Several studies have found sex-specific postural precursors of motion sickness in standing body sway prior to exposure to any motion stimuli (e.g., Koslucher et al., 2016a; Munafo et al., 2017; Curry et al., 2020b). Koslucher et al. (2016a) found this to be the case during exposure to nauseogenic motion. In the present study, we conducted the first assessment of possible sex differences in postural precursors of motion sickness during seated exposure to virtual locomotion in an HMD.
Postural Precursors and the Driver–Passenger Effect
Arcioni et al. (2018; see also Risi and Palmisano, 2019) exposed participants to a virtual environment through an HMD. All participants controlled their own motion within the virtual environment. The authors measured standing body sway before HMD exposure, and in these data, they identified postural precursors of (subsequent) motion sickness. Arcioni et al. and Risi and Palmisano included both women and men, but the authors did not analyze for possible sex differences in postural precursors of motion sickness. Munafo et al. (2017) compared women and men, but measured postural activity only prior to exposure to the virtual environment. In addition, in their study, all participants controlled virtual locomotion.
Dong et al. (2011) examined the Driver–Passenger effect in virtual vehicles as presented to seated participants through a desktop video monitor. Using a yoked-control design (cf. Rolnick and Lubow, 1991), one member of each pair of participants (the Driver) drove a virtual vehicle (i.e., played the driving video game), while their performance was recorded. This recording was replayed and viewed by the other member of the pair (the Passenger). This design ensured that visual motion stimuli were identical for the two members of each pair: exposure to the game differed only in that one participant controlled the virtual vehicle, whereas the other did not. The results revealed that the incidence of motion sickness was greater among Passengers than among Drivers, consistent with the Driver–Passenger effect. Dong et al. also recorded the kinematics of the head and torso as seated participants were exposed to the video game. Patterns of postural activity were found to differ between Drivers and Passengers and, separately, between participants who later reported motion sickness, and those who did not. In the present study, we asked new questions about relations between postural precursors of motion sickness, the Driver–Passenger effect, and sex differences.
The Present Study
The present study was modeled on Dong et al. (2011), in terms of our focus on head and torso movement of seated participants during exposure to a driving video game, either as drivers or as passengers. Like Dong et al., we used a yoked-control design in which one member of each pair of participants played a driving game (i.e., drove a virtual automobile). A recording of that performance was viewed (in a separate session) by the other member of the pair. Thus, the two members of each pair were exposed to identical vehicle trajectories, but the risk of behavioral contagion was minimized. The present study differed from Dong et al. in several respects. First, we used a different driving video game. Second, the game was presented through an HMD, rather than being presented through a desktop interface. Third, we crossed our manipulation of vehicle control (i.e., Drivers vs. Passengers) with a manipulation of sex: half of our participants were men, whereas half were women. Independent measures of motion sickness incidence and symptom severity from our sample were reported by Curry et al. (2020a), who found that the incidence of motion sickness did not differ between Drivers and Passengers or between women and men. That is, they did not replicate either the classical Driver–Passenger effect or commonly reported sex differences in susceptibility. The study by Curry et al. (2020a) was the first assessment of the Driver–Passenger effect in an HMD, as well as being the first study of sex differences in the control of virtual vehicles. It is possible that unique characteristics of HMDs may minimize the Driver–Passenger effect, while the dynamics of virtual vehicles may tend to suppress sex differences in the incidence of motion sickness (for a discussion, see Curry et al. (2020a). In the present study, we investigated the kinematics of head and torso movement as seated participants were exposed to the driving video game in the study by Curry et al. (2020a). Previous studies have found differences in postural precursors of motion sickness between groups (e.g., people with vs. without experience driving physical vehicles) even when groups did not differ in motion sickness incidence or severity (e.g., Stoffregen et al., 2017).
Postural activity typically changes over time during exposure to virtual environments, and postural precursors of motion sickness often vary as a function of exposure duration (e.g., Dong et al., 2011; Chang et al., 2017; Stoffregen et al., 2017). Following these studies, we separated data on postural kinematics into three non-overlapping Time Windows, which allowed us to evaluate possible changes in postural activity as a function of exposure duration.
We predicted that postural activity would differ between Drivers and Passengers and between women and men. Our primary prediction was that differences in postural precursors of motion sickness between Drivers and Passengers would, themselves, be modulated by sex. Within these interactions, we did not make predictions about specific contrasts. For this reason, we do not report post-hoc contrasts on statistically significant effects.
Method
Participants
Curry et al. (2020a) reported data on 79 participants. Some of those participants were not included in the present study (see the Results section for details). The present analysis included data from 65 individuals (32 women and 33 men), who participated in exchange for course credit. Participants ranged in age from 18 to 36 years (mean = 21.55 years, SD = 3.04 years), in height from 1.51 to 1.94 m (mean = 1.73 m, SD = 0.10 m), and in weight from 47.63 to 104.33 kg (mean = 72.19 kg, SD = 12.22 kg). The research protocol (STUDY00001875) was approved in advance by the IRB of the University of Minnesota.
Apparatus
Participants wore the Oculus Rift CV1. The device comprised a lightweight (0.360 kg) headset that completely covered the field of view. The headset included separate displays for each eye, each with 1,080 × 1,020 resolution, yielding a 100° horizontal field of view. A lens located in front of each display rendered display content at optical infinity.
We used a magnetic tracking system (Fastrak; Polhemus, Colchester, VT) to record postural activity. Sensors were worn at the head and torso (as described below), and each was sampled at 60 Hz. For each sensor, we collected data on movement in the anterior–posterior (AP) and mediolateral (ML) axes.
Procedure
We obtained informed consent from each participant. We informed participants that they could discontinue at any time, for any reason, without penalty. Following previous studies (e.g., Stoffregen and Smart, 1998; Merhi et al., 2007; Stoffregen et al., 2008, 2010; Dong et al., 2011; Koslucher et al., 2015), we used independent assessments of the incidence of motion sickness and the severity of symptoms (for details, see Curry et al., 2020a). To assess motion sickness incidence, participants answered a forced-choice, yes/no question, Are you motion sick? Participants were instructed (both verbally and on the consent form) to discontinue the experiment immediately if they experienced any motion sickness symptoms, however mild. After completion of the consent process, we conducted a pre-exposure assessment of motion sickness incidence and severity, after which we measured standing body sway while participants performed some simple visual tasks, as reported by Curry et al. (2020b).
Following our assessment of standing posture, participants sat on a stool that did not rotate and had no wheels and were fitted with a sensor from the magnetic tracking system, which was attached, using cloth medical tape, between the shoulder blades, at the base of the neck. Another sensor was attached to the Oculus headset. Participants donned the Oculus headset and were exposed to Assetto Corsa, a commercial driving game. Each Driver drove a Ferrari 458 Italia on the Highlands Long Track (Figure 1). Details of the driving game were reported in Curry et al. (2020a). During exposure to the video game, we used a between-participants, yoked-control design, with individual Passengers yoked to individual Drivers. Participant pairs were sex-matched: men with men and women with women. Participants played or viewed the game for up to 15 min. Data on head and torso motion were collected continuously. Additional details of the yoked-control procedure are reported in Curry et al. (2020a).
After completing the 15-min game exposure, or after discontinuation (whichever came first), we again assessed motion sickness incidence and severity. Participants who answered yes to the forced-choice, yes/no question, Are you motion sick? were assigned to the sick group. All others were assigned to the well group.
Analysis of Head and Torso Movement
Postural activity can be characterized in terms of spatial magnitude (i.e., spatial structure), but it can also be characterized in terms of temporal dynamics (i.e., temporal structure). Recent years have seen the development of a wide array of dependent variables that assess different aspects of the temporal dynamics of the kinematics of human movement. Many widely used parameters are derived from general physical processes and do not have an a priori or intrinsic relation to animate movement. For example, stabilogram diffusion analysis (e.g., Collins and De Luca, 1993) is derived from models of the movement of gas molecules and has no intrinsic relation to the physical structure of the body. One relatively new parameter is the multifractality of movement. Several scholars have argued that multifractality may be a fundamental property of animate movement, and that, as such, measures of multifractality may be more meaningful than measures of other aspects of temporal dynamics (Kelty-Stephen et al., 2013; Palatinus et al., 2014). Several studies have documented the existence of multifractality in standing body sway (Thurner et al., 2000; Shimizu et al., 2002; Ihlen et al., 2013; Munafo et al., 2016). Other studies have shown that postural precursors of motion sickness can occur in the multifractality of postural activity (e.g., Koslucher et al., 2016a; Munafo et al., 2017; Curry et al., 2020b).
We conducted separate evaluations of the spatial magnitude and multifractality of movement. We evaluated the spatial magnitude of postural activity in terms of positional variability, which we defined operationally as the standard deviation of position. We used multifractal detrended fluctuation analysis, or MF-DFA, to evaluate the multifractality of postural activity (e.g., Kantelhardt et al., 2002; Ihlen et al., 2013; Munafo et al., 2016). MF-DFA is an extension of detrended fluctuation analysis, or DFA (Lin et al., 2008). MF-DFA has been used in the assessment of postural sway in a variety of contexts (e.g., Munafo et al., 2016). Detrended fluctuation analysis assumes that fluctuations in a time series are homogeneous (Ihlen and Vereijken, 2010), but this assumption typical is not met in data on human movement: multifractal fluctuations are interdependent and heterogeneous. The heterogeneous nature of multifractal fluctuations can be revealed in the range of the singularity exponent, h(q) (Ihlen, 2012). The width of this range is an index of the degree (or amount) of multifractality in a time series. The range of h(q) values is known as the singularity spectrum or the spectrum. The wider the spectrum, the more multifractal is the movement (Kelty-Stephen et al., 2013). For each trial, we conducted inferential statistics on the width of the singularity spectrum. We obtained the width of the spectrum using open source code for MATLAB (MFDFA1; Ihlen, 2012). Following Munafo et al. (2016), we selected a minimum scaling range of 16 data points with 19 evenly spaced increasing segment sizes to a maximum of the length of the time series. This range was the same for each time series.
Exposure duration varied between participants, as reflected in variations in discontinuation time, and in the fact that some participants completed the 15-min protocol. We conducted separate repeated measures ANOVAs on positional variability and the width of the multifractal spectrum. For each ANOVA, the factors were Time Windows (W1, W2, W3), Segment (head vs. torso), Body Axis (AP vs. ML), Sex (women vs. men), Control (drivers vs. passengers), and Sickness Groups (well vs. sick). Time Windows, Segment, and Body Axis were within-participants factors, whereas Sex, Control, and Sickness Groups were between-participants factors.
Results
As reported by Curry et al. (2020a), the overall incidence of motion sickness was 43% (34/79). Data on symptom severity were also reported by Curry et al. (2020a).
We excluded the kinematic data from three participants (one well, two sick) because of technological difficulties. Of the remaining 32 participants in the sick group, 11 discontinued after <6 min of game play. For this reason, these three participants were excluded from movement analysis. For the remaining 21 participants in the sick group, the mean exposure to the game was 620.64 ± 190.01 s. Following Chang et al. (2017), we defined time windows for the well groups based on the mean exposure time of participants in the sick group. Accordingly, Window 1 comprised the first 120 s of game play, Window 2 ran from 251 to 371 s, and Window 3 ran from 501 to 621 s.
Positional Variability
The results are summarized in Table 1, which details Factors, F-values, p-values, and values of partial η2. For positional variability, the main effect of Segments was significant. Positional variability for the head (M = 1.17 cm, SE = 0.08 cm) was greater than that for the torso (M = 0.76 cm, SE = 0.06 cm). The main effect of Time Windows was significant (Window 1 mean = 1.06 cm, SE = 0.07 cm; Window 2 mean = 0.91 cm, SE = 0.08 cm; Window 3 mean = 0.93 cm, SE = 0.07 cm).
There were several significant interactions involving the Time Windows factor. A stand-alone effect was the significant Segment × Time Windows interaction. As shown in Figure 2, motion of the head and torso changed differently over time (i.e., across Time Windows). For the torso, changes across Time Windows were not significant. The Body Axis × Time Windows interaction was significant. This interaction was subsumed in two higher-order interactions. The Body Axis × Time Windows × Control × Sex interaction was significant (Figure 3). In addition, the Body Axis × Time Windows × Control × Sickness Groups interaction was significant (Figure 4).
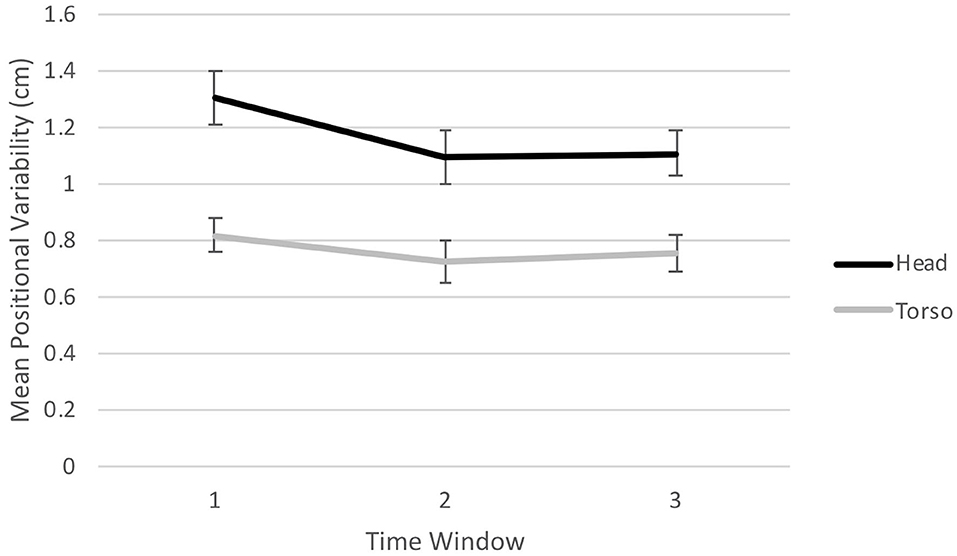
Figure 2. Positional variability, illustrating the statistically significant interaction between Body Segment (head, torso) and Time Windows.
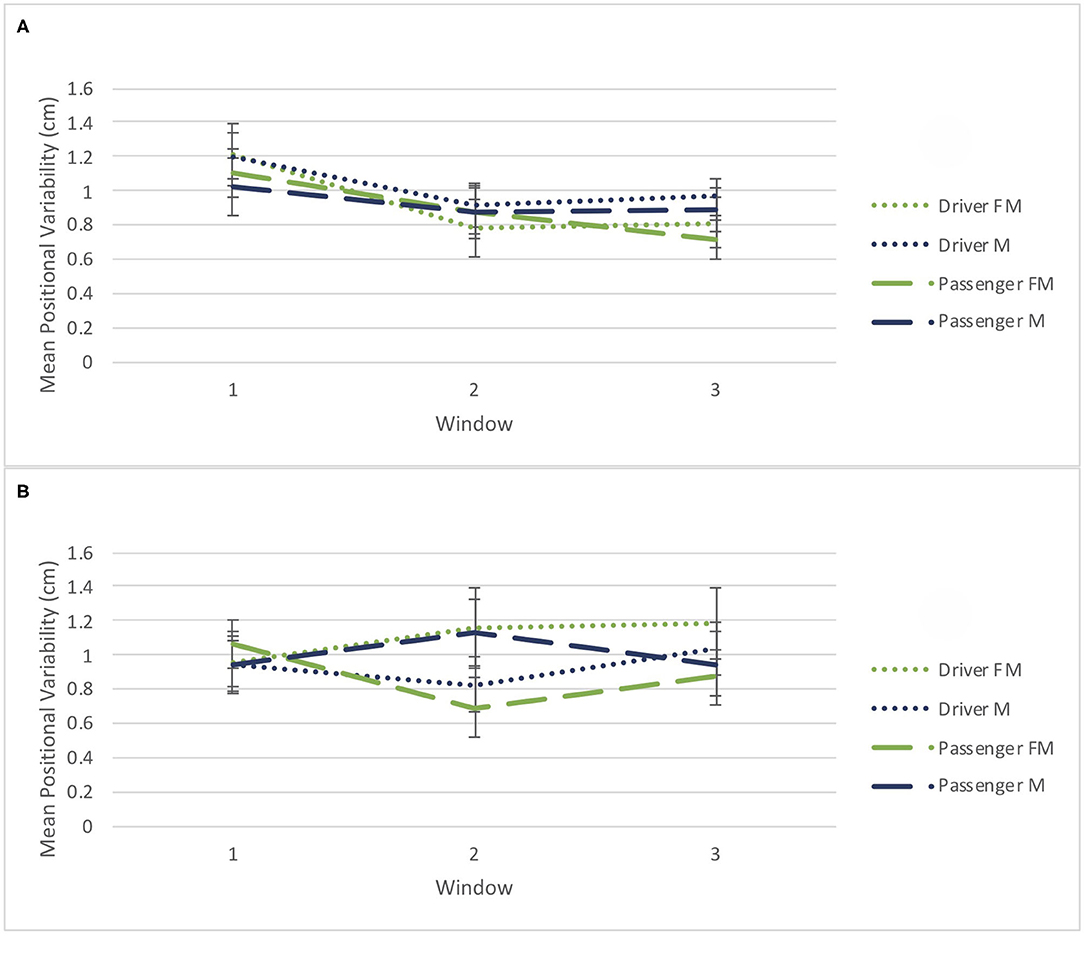
Figure 3. Positional variability, illustrating the statistically significant interaction between Body Axis (anterior–posterior, mediolateral), Sex, Control (drivers, passengers), and Time Windows. (A) Movement in the mediolateral axis. (B) Movement in the anterior–posterior axis.
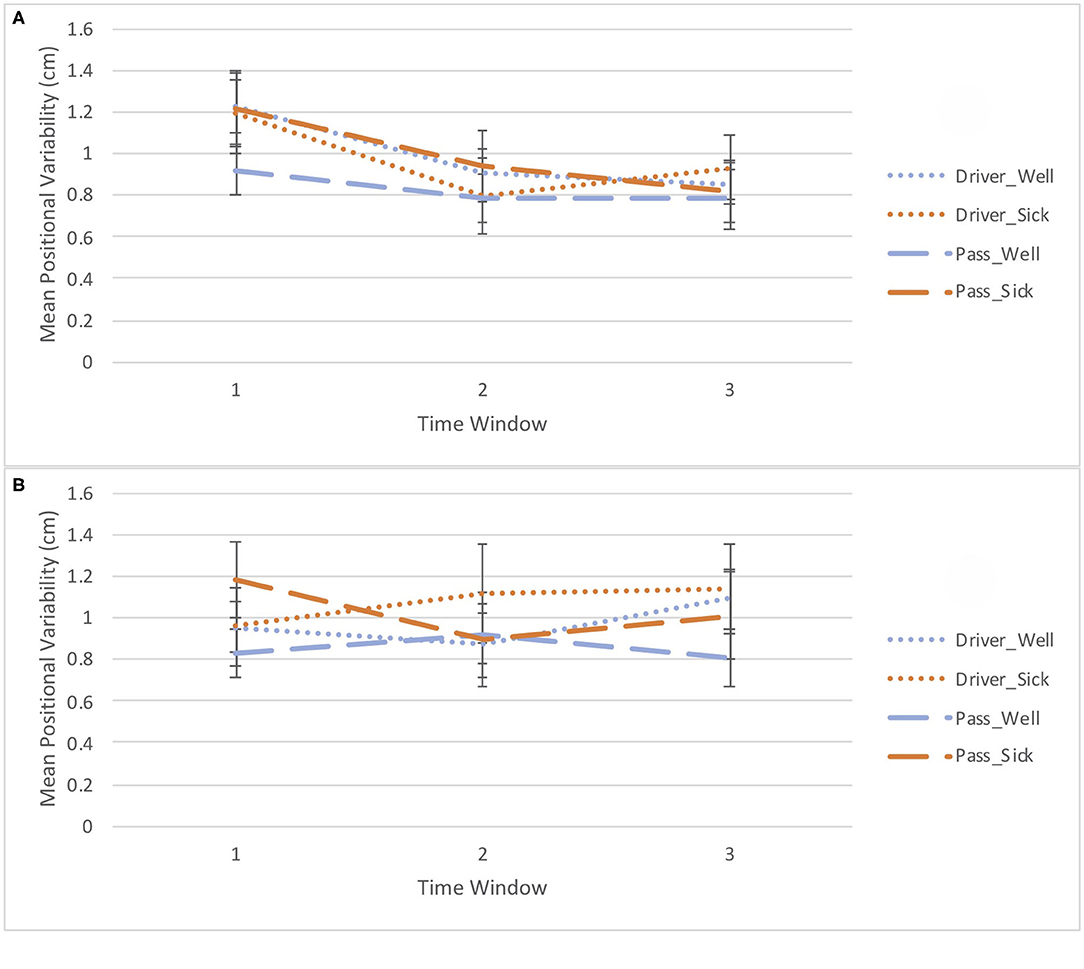
Figure 4. Positional variability, illustrating the statistically significant interaction between Body Axis (anterior–posterior, mediolateral), Control (drivers, passengers), Time Windows, and Sickness Groups. (A) Movement in the mediolateral axis. (B) Movement in the anterior–posterior axis.
Several significant interactions did not include the Time Windows factor. The Segment × Control interaction was significant, as was the Body Axis × Segment interaction was significant. In addition, the Body Axis × Segment × Sex × Sickness Groups interaction was significant. These interactions were subsumed in a statistically significant 5-way interaction between Body Axis, Segment, Control, Sex, and Sickness Groups (Figure 5). There were no other significant differences.
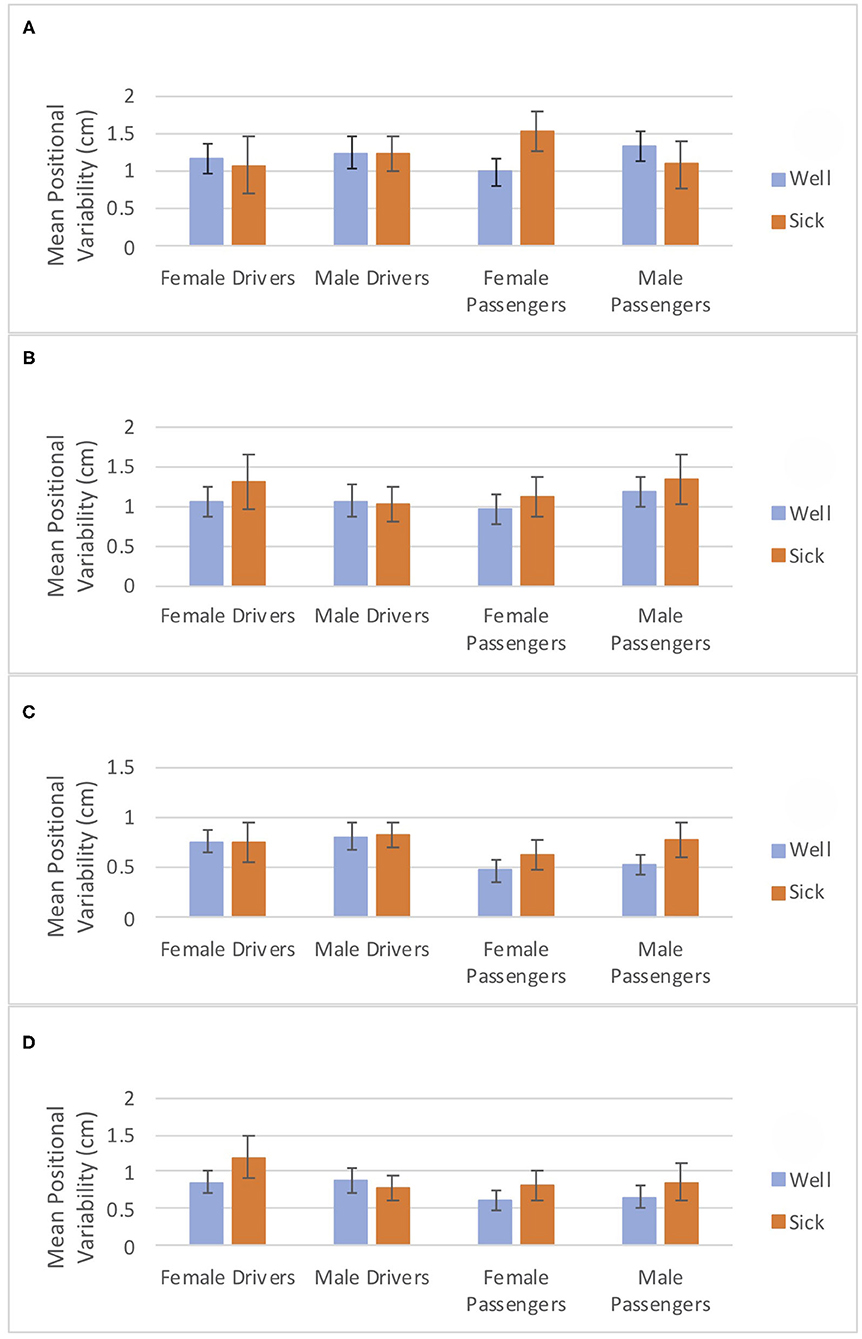
Figure 5. Positional variability, illustrating the statistically significant interaction between Body Axis (anterior–posterior, mediolateral), Segment (Head, Torso), Sex, Control (drivers, passengers), and Sickness Groups. (A) Head movement in the mediolateral axis. (B) Head movement in the anterior–posterior axis. (C) Torso movement in the mediolateral axis. (D) Torso movement in the anterior–posterior axis.
Width of the Multifractal Spectrum
The results are summarized in Table 1. For the width of the multifractal spectrum, the main effect of Control was significant. The multifractal spectrum was wider among Passengers (M = 0.36, SE = 0.02) than among Drivers (M = 0.30, SE = 0.02). In addition, the Body Axis × Segment interaction was significant (head AP M = 0.31, SE = 0.01; head ML M = 0.32, SE = 0.014; torso AP M = 0.36, SE = 0.02; torso ML M = 0.32, SE = 0.02). There were no other significant effects.
Discussion
We exposed seated participants to a virtual vehicle in a driving video game that was presented through an HMD. We covaried sex (women vs. men) control of the virtual vehicle (drivers vs. passengers) and motion sickness status (well vs. sick, as reported by Curry et al., 2020a). In the present study, we examined movement of the head and torso during game exposure. We found several effects that were independent of motion sickness status. Some of these replicated common findings in the literature, whereas others were novel. The principal result of the study was our identification of postural precursors of motion sickness. Two statistically significant interactions revealed that postural precursors of motion sickness differed between drivers and passengers and between women and men. We discuss these results in turn.
Movement Independent of Motion Sickness
The main effect of Segment was significant for the positional variability of postural activity, but this effect was subsumed in the significant Segment × Time Windows interaction (Figure 2). The nature of the interaction was unusual, in that movement of both the head and torso declined across Time Windows. This pattern contrasts with previous studies, in which postural activity has tended to increase over time (e.g., Merhi et al., 2007; Villard et al., 2008; Dong et al., 2011). The Segment × Body Axis interaction was also significant for the width of the multifractal spectrum. That is, relations between body segments and body axes influenced the orthogonal variables of positional variability and movement multifractality. A similar effect was reported by Walter et al. (2019) who exposed standing participants to oscillation of the visual environment along the line of sight.
For positional variability, the Body Axis × Time Windows interaction was significant; however, this interaction was subsumed in the significant Body Axis × Sex × Control × Time Windows interaction (Figure 3). Sex differences are a common feature of the kinematics of standing body sway (e.g., Era et al., 2006; Kim et al., 2010). In the present study, participants were seated, which made it possible for us to evaluate the possibility that there might be sex differences in the control of seated postural sway. We are not aware of any previous research on sex differences in seated postural activity. Accordingly, the effect illustrated in Figure 3 appears to be novel.
The main effect of Control was significant for the width of the multifractal spectrum, confirming our prediction. The multifractal spectrum was wider (that is, postural activity exhibited a greater degree of multifractality) for Passengers than for Drivers. Differences in postural activity between seated Drivers and Passengers in virtual vehicles have been reported in previous studies in which virtual vehicles were presented via a desktop monitor. Dong et al. (2011) found that postural activity of Drivers and Passengers differed in terms of both the positional variability and temporal dynamics of the head and the torso. A similar effect has been reported for seated participants who controlled the gait of a virtual avatar vs. participants who merely watched recorded locomotion of the avatar (Chen et al., 2012). Chen et al. also found control-related differences in the positional variability of the torso and the temporal dynamics of the head. That movement might differ between Drivers and Passengers is not surprising. Because Drivers control the virtual vehicle, their postural adjustments related to vehicle motion can be anticipatory. For Passengers, postural adjustments for motion of the virtual vehicle must be compensatory (Dong et al.; Stoffregen et al., 2017).
Postural Precursors of Motion Sickness
We identified postural precursors of motion sickness in the positional variability of the head and torso. One such effect was a statistically significant Body Axis × Time Windows × Control × Sickness Groups interaction (Figure 4). This interaction reveals that the temporal evolution of postural precursors of motion sickness differed between Drivers and Passengers. This finding is novel. Dong et al. (2011) found that the temporal evolution of movement differed over time (i.e., across Time Windows) between Drivers and Passengers. In a separate effect, they found that the temporal evolution of movement differed between the well and sick groups; however, they found no evidence of any interaction between these factors. In the present study, our novel identification of this interaction may be related to the fact that our driving game was presented via an HMD, whereas in Dong et al., the driving video game was presented on a desktop monitor.
Our primary prediction was that there would be statistically significant interactions that would include the factors Sickness Groups, Sex, and Control. This prediction was confirmed in the statistically significant Body Axis × Segment × Control × Sex × Sickness Groups interaction (Figure 5). This effect reveals, for the first time, that sex can interact with vehicle control in determining postural precursors of motion sickness.
To summarize, in two statistically significant interactions, postural precursors of motion sickness differed between Drivers and Passengers (Figures 4, 5). In one of these interactions, postural precursors of motion sickness that differed between Drivers and Passengers also differed between women and men (Figure 5). Several studies have identified sex differences in postural precursors of motion sickness (Koslucher et al., 2016a,b; Munafo et al., 2017), but this is the first demonstration that sex differences in postural precursors of motion sickness can differ between drivers and passengers. These effects confirm a prediction of the postural instability theory of motion sickness (Riccio and Stoffregen, 1991) that the kinematics of movement should differ between individuals who (later) report motion sickness and those who do not, and that these differences should exist before the onset of any subjective symptoms of motion sickness. The postural instability theory predicts that any factor that influences the control of posture can modulate postural precursors of motion sickness. The present results demonstrate that such individual differences can be situational, or task related (i.e., Drivers vs. Passengers; cf. Slobounov and Newell, 1994; Stoffregen et al., 1999), or structural (i.e., women vs. men). Our results are consistent with broader developments in the study of human movement, such as the claim that the subtle kinematics of movement may be unique to each individual (e.g., Slowinski et al., 2016). Other theories of motion sickness etiology (e.g., Reason, 1978; Oman, 1982) make no predictions about how postural precursors of motion sickness might be modulated by either situational or structural factors.
Interpupillary Distance: Cause or Correlate?
The Oculus Rift system fits persons with interpupillary distance (IPD) in the range 58–71 mm. Most adults fall within this range; however, 30% of adult women have IPD <59 mm (Stanney et al., 2020). Stanney et al. (2020) found that cybersickness was correlated with the “goodness” of IPD fit. However, based on this correlational finding, they did not claim that IPD played a causal role in cybersickness. If IPD were a causal factor in motion sickness among HMD users, then we would expect to see higher rates of sickness among populations that tend to have smaller IPD. One such population is children, who often are enthusiastic users of HMD systems. Thus, if motion sickness is caused by inappropriate matching between HMD design capabilities and users' IPD, then we would expect that HMD-related motion sickness would be especially common among children. We know of no evidence for differential rates of HMD-related sickness between children and adults. There is also an issue of etiology. A correlation between IPD and motion sickness susceptibility does not, by itself, imply any particular etiological interpretation. On the one hand, the discrepancy might be interpreted as a source of sensory conflict, such that the correlation between IPD and cybersickness might have a causal link through the sensory conflict theory of motion sickness (Reason, 1978; Oman, 1982). However, an interpretation in terms of sensory conflict is not mandatory. Different causal linkages can be proposed. It might be, for example, that improper fit of HMD headsets can undermine stable control of the body, which is more likely to have a causal relation to cybersickness. We predict that correlations should be stronger between motion sickness and postural kinematics than between motion sickness and IPD.
Conclusion
We examined the postural activity of seated participants during exposure to a driving video game presented through an HMD. We covaried sex (women vs. men), vehicle control (Drivers vs. Passengers), and motion sickness status (as reported by Curry et al., 2020a). Analysis of the positional variability of head and torso movement revealed differences between Drivers and Passengers in the temporal evolution of postural precursors of motion sickness. In a separate effect, postural precursors of motion sickness that differed between Drivers and Passengers co-varied as a function of sex. These results are in agreement with the general hypothesis that motion sickness is preceded by patterns of postural activity that differ between individuals who (later) report motion sickness and those who do not. In addition, these results reveal that the nature of postural precursors of motion sickness can differ between the sexes and between Drivers and Passengers. In general, the results are consistent with predictions derived from the postural instability theory of motion sickness (Riccio and Stoffregen, 1991).
Data Availability Statement
The datasets presented in this study can be found in online repositories. The names of the repository/repositories and accession number(s) can be found below: https://doi.org/10.13020/a9w0-8k04.
Ethics Statement
The studies involving human participants were reviewed and approved by University of Minnesota IRB. The patients/participants provided their written informed consent to participate in this study.
Author Contributions
CC, RL, and TS developed the study concept and design. CC, NP, and RL performed the testing and data collection. CC, NP, and TS contributed to the manuscript draft. RL contributed to the study concept and design. All authors approved the final version of the manuscript for submission and performed the data analysis and interpretation.
Funding
Elisheeva Savvateev was supported by the University of Minnesota Undergraduate Research Opportunities Program. CC was supported by the NRT grant for translational sensory science (NRT-1734815). TS was supported by NSF-1901423, CHS: Medium: Prediction, Early Detection, and Mitigation of Virtual Reality Simulator Sickness.
Conflict of Interest
The authors declare that the research was conducted in the absence of any commercial or financial relationships that could be construed as a potential conflict of interest.
Acknowledgments
We thank Elisheeva Savvateev for assistance in data collection and Dawn McBride for assistance with statistical inference.
References
Arcioni, B., Palmisano, S., Apthorp, D., and Kim, J. (2018). Postural stability predicts the likelihood of cybersickness in active HMD-based virtual reality. Displays 58, 3–11. doi: 10.1016/j.displa.2018.07.001
Bruder, G., Steinicke, F., Wieland, P., and Lappe, M. (2012). Tuning self-motion perception in virtual reality with visual illusions. IEEE Trans. Vis. Comput. Graph. 18, 1068–1078. doi: 10.1109/TVCG.2011.274
Chang, C.-H., Chen, F.-C., Kung, W.-C., and Stoffregen, T. A. (2017). Effects of physical driving experience on body movement and motion sickness during virtual driving. Aerospace Med. Hum. Perform. 88, 985–992. doi: 10.3357/AMHP.4893.2017
Chen, Y.-C., Dong, X., Chen, F.-C., and Stoffregen, T. A. (2012). Control of a virtual avatar influences postural activity and motion sickness. Ecol. Psychol. 24, 279–299. doi: 10.1080/10407413.2012.726181
Collins, J. J., and De Luca, C. J. (1993). Open-loop and closed-loop control of posture: a random-walk analysis of center-of-pressure trajectories. Exp. Brain Res. 95, 308–318. doi: 10.1007/BF00229788
Curry, C., Peterson, N. A., Li, R., and Stoffregen, T. A. (2020b). Postural precursors of motion sickness in head-mounted displays: drivers and passengers, women and men. Ergonomics 63, 1502–1511. doi: 10.1080/00140139.2020.1808713
Curry, C., Li, R., Peterson, N. A., and Stoffregen, T. A. (2020a). Cybersickness in virtual reality head-mounted displays: examining the influence of sex differences and vehicle control. Int. J. Hum. Comput. Interact. 36, 1161–1167. doi: 10.1080/10447318.2020.1726108
Dong, X., Yoshida, K., and Stoffregen, T. A. (2011). Control of a virtual vehicle influences postural activity and motion sickness. J. Exp. Psychol. Appl. 17, 128–138. doi: 10.1037/a0024097
Era, P., Sainio, P., Koskinen, S., Haavisto, P., Vaara, M., and Aromaa, A. (2006). Postural balance in a random sample of 7,979 subjects aged 30 years and over. Gerontology 52, 204–213. doi: 10.1159/000093652
Ihlen, E. A. (2012). Introduction to multifractal detrended fluctuation analysis in Matlab. Front. Physiol. 3:141. doi: 10.3389/fphys.2012.00141
Ihlen, E. A., Skjaeret, N., and Vereijken, B. (2013). The influence of center-of- mass movements on the variation in the structure of human postural sway. J. Biomech. 46, 484–490. doi: 10.1016/j.jbiomech.2012.10.016
Ihlen, E. A., and Vereijken, B. (2010). Interaction-dominant dynamics in human cognition: beyond 1/f α fluctuation. J. Exp. Psychol. 139, 436–463. doi: 10.1037/a0019098
Kantelhardt, J. W., Zschiegner, S. A., Koscielny-Bunde, E., Havlin, S., Bunde, A., and Stanley, H. E. (2002). Multifractal detrended fluctuation analysis of nonstationary time series. Phys. A 316, 87–114. doi: 10.1016/S0378-4371(02)01383-3
Kelty-Stephen, D. G., Palatinus, K., Saltzman, E., and Dixon, J. A. (2013). A tutorial on multifractality, cascades, and interactivity for empirical times series in ecological science. Ecol. Psychol. 25, 1–62. doi: 10.1080/10407413.2013.753804
Kim, J. W., Eom, G. M., Kim, C. S., Kim, D. H., Lee, J. H., Park, B. K., et al. (2010). Sex differences in the postural sway characteristics of young and elderly subjects during quiet natural standing. Geriatr. Gerontol. Int. 10, 191–198. doi: 10.1111/j.1447-0594.2009.00582.x
Koslucher, F. C., Haaland, E., Malsch, A., Webeler, J., and Stoffregen, T. A. (2015). Sex differences in the incidence of motion sickness induced by linear visual oscillation. Aerospace Med. Hum. Perform. 86, 787–793. doi: 10.3357/AMHP.4243.2015
Koslucher, F. C., Haaland, E., and Stoffregen, T. A. (2014). Body load and the postural precursors of motion sickness. Gait Posture 39, 606–610. doi: 10.1016/j.gaitpost.2013.09.016
Koslucher, F. C., Haaland, E., and Stoffregen, T. A. (2016b). Sex differences in visual performance and postural sway precede sex differences in visually induced motion sickness. Exp. Brain Res. 234, 313–322. doi: 10.1007/s00221-015-4462-y
Koslucher, F. C., Munafo, J., and Stoffregen, T. A. (2016a). Postural sway in men and women during nauseogenic motion of the illuminated environment. Exp. Brain Res. 234, 2709-2720. doi: 10.1007/s00221-016-4675-8
Lawther, A., and Griffin, M. J. (1988). A survey of the occurrence of motion sickness amongst passengers at sea. Aviat. Space Environ. Med. 59, 399–406.
Li, R., Walter, H., Curry, C., Rath, R., Peterson, N., and Stoffregen, T. A. (2018). Postural time-to-contact as a precursor of visually induced motion sickness. Exp. Brain Res. 236, 1631-1641. doi: 10.1007/s00221-018-5246-y
Lin, D., Seol, H., Nussbaum, M. A., and Madigan, M. L. (2008). Reliability of COP-based postural sway measures and age-related differences. Gait Posture 28, 337–342. doi: 10.1016/j.gaitpost.2008.01.005
Merhi, O., Faugloire, E., Flanagan, M., and Stoffregen, T. A. (2007). Motion sickness, console video games, and head mounted displays. Hum. Factors 49, 920–934. doi: 10.1518/001872007X230262
Munafo, J., Curry, C., Wade, M. G., and Stoffregen, T. A. (2016). The distance of visual targets affects the spatial magnitude and multifractal scaling of standing body sway in younger and older adults. Exp. Brain Res. 234, 2721–2730. doi: 10.1007/s00221-016-4676-7
Munafo, J., Diedrick, M., and Stoffregen, T. A. (2017). The virtual reality head-mounted display Oculus Rift induces motion sickness and is sexist in its effects. Exp. Brain Res. 235, 889–901. doi: 10.1007/s00221-016-4846-7
Nilsson, N. C., Peck, T., Bruder, G., Hodgson, E., Serafin, S., Whitton, M., et al. (2018). “15 years of research on redirected walking in immersive virtual environments,” in IEEE Computer Graphics and Applications (New York, NY). doi: 10.1109/MCG.2018.111125628
Oman, C. M. (1982). A heuristic mathematical model for the dynamics of sensory conflict and motion sickness. Acta Oto-Laryngol. Suppl. 392:44. doi: 10.3109/00016488209108197
Palatinus, Z., Kelty-Stephen, D., Kinsella-Shaw, J., Carello, C., and Turvey, M. (2014). Haptic perceptual intent in quiet standing affects multifractal scaling of postural fluctuations. J. Exp. Psychol. 40, 1808–1818. doi: 10.1037/a0037247
Palmisano, S., Arcioni, B., and Stapley, P. J. (2018). Predicting vection and visually induced motion sickness based on spontaneous postural activity. Exp. Brain Res. 236, 315–329. doi: 10.1007/s00221-017-5130-1
Reason, J. T. (1978). Motion sickness adaptation: a neural mismatch model. J. R. Soc. Med. 71, 819–829. doi: 10.1177/014107687807101109
Riccio, G. E., and Stoffregen, T. A. (1991). An ecological theory of motion sickness and postural instability. Ecol. Psychol. 3, 195–240. doi: 10.1207/s15326969eco0303_2
Risi, D., and Palmisano, S. (2019). Effects of postural stability, active control, exposure duration and repeated exposures on HMD induced cybersickness. Displays 60, 9–17. doi: 10.1016/j.displa.2019.08.003
Rolnick, A., and Lubow, R. E. (1991). Why is the driver rarely motion sick? The role of controllability in motion sickness. Ergonomics 34, 867–879. doi: 10.1080/00140139108964831
Shimizu, Y., Thurner, S., and Ehrenberger, K. (2002). Multifractal spectra as a measure of complexity in human posture. Fractals 10, 103–116. doi: 10.1142/S0218348X02001130
Slobounov, S., and Newell, K. M. (1994). Postural dynamics as a function of skill level and task constraints. Gait Posture 2, 85–93. doi: 10.1016/0966-6362(94)90097-3
Slowinski, P., Zhai, C., Alderisio, F., Salesse, R., Gueugnon, M., Marin, L., et al. (2016). Dynamic similarity promotes interpersonal coordination in joint action. J. R. Soc. Interface 13:20151093 doi: 10.1098/rsif.2015.1093
Stanney, K., Fidopiastis, C., and Foster, L. (2020). Virtual reality is sexist: But it does not have to be. Front. Robotics AI 7:4. doi: 10.3389/frobt.2020.00004
Stanney, K., Salvendy, G., Deisinger, J., DiZio, P., Ellis, S., Ellision, J., et al. (1998). Aftereffects and sense of presence in virtual environments: formulation of a research and development agenda. Report sponsored by the Life Sciences Division at NASA Headquarters. Int. J. Human Comput. Interact. 10, 135–187. doi: 10.1207/s15327590ijhc1002_3
Stoffregen, T. A., Chang, C.-H., Chen, F.-C., and Zeng, W.-J. (2017). Effects of decades of physical driving on body movement and motion sickness during virtual driving. PLoS ONE 12:e0187120. doi: 10.1371/journal.pone.0187120
Stoffregen, T. A., Chen, Y.-C., and Koslucher, F. C. (2014). Motion control, motion sickness, and the postural dynamics of mobile devices. Exp. Brain Res. 232, 1389-1397. doi: 10.1007/s00221-014-3859-3
Stoffregen, T. A., Faugloire, E., Yoshida, K., Flanagan, M., and Merhi, O. (2008). Motion sickness and postural sway in console video games. Hum. Factors 50, 322–331. doi: 10.1518/001872008X250755
Stoffregen, T. A., and Smart, L. J. (1998). Postural instability precedes motion sickness. Brain Res. Bull. 47, 437–448. doi: 10.1016/S0361-9230(98)00102-6
Stoffregen, T. A., Smart, L. J., Bardy, B. G., and Pagulayan, R. J. (1999). Postural stabilization of looking. J. Exp. Psychol. 25, 1641–1658. doi: 10.1037/0096-1523.25.6.1641
Stoffregen, T. A., Yoshida, K., Villard, S., Scibora, L., and Bardy, B. G. (2010). Stance width influences postural stability and motion sickness. Ecol. Psychol. 22, 169–191. doi: 10.1080/10407413.2010.496645
Thurner, S., Mittermaier, C., Hanel, R., and Ehrenberger, K. (2000). Scaling-violation phenomena and fractality in the human posture control system. Phys. Rev. E 62, 4018–4024. doi: 10.1103/PhysRevE.62.4018
Villard, S., Flanagan, M. B., Albanese, G., and Stoffregen, T. A. (2008). Postural instability and motion sickness in a virtual moving room. Hum. Factors 50, 332–345. doi: 10.1518/001872008X250728
Keywords: motion sickness, cybersickness, virtual reality, head-mounted display, posture, sex differences
Citation: Curry C, Peterson N, Li R and Stoffregen TA (2020) Postural Activity During Use of a Head-Mounted Display: Sex Differences in the “Driver–Passenger” Effect. Front. Virtual Real. 1:581132. doi: 10.3389/frvir.2020.581132
Received: 07 July 2020; Accepted: 23 November 2020;
Published: 23 December 2020.
Edited by:
Stephen Palmisano, University of Wollongong, AustraliaReviewed by:
Lihan Chen, Peking University, ChinaJoanna Hale, University College London, United Kingdom
Copyright © 2020 Curry, Peterson, Li and Stoffregen. This is an open-access article distributed under the terms of the Creative Commons Attribution License (CC BY). The use, distribution or reproduction in other forums is permitted, provided the original author(s) and the copyright owner(s) are credited and that the original publication in this journal is cited, in accordance with accepted academic practice. No use, distribution or reproduction is permitted which does not comply with these terms.
*Correspondence: Thomas A. Stoffregen, tas@umn.edu