- Department of Psychiatry and Psychotherapy, University of Bonn, Bonn, Germany
Supernumerary phantom limb (SPL) is a rare neuropsychiatric syndrome, under which patients perceive more limbs than they anatomically possess (e.g., two instead of only one right arm). Remarkably, the concurrent limb percepts can thereby differ in respect to their primarily experienced modality, for instance, that one kinesthetic and one moving limb are experienced. Although SPLs are well-documented after various neuropsychiatric conditions, their neurocognitive pathomechanisms remain elusive. Therefore, an experimental paradigm by which such aberrant body misperceptions could be transiently induced and systematically investigated in healthy participants would be helpful. Inspired by the virtual hand illusion, we developed a virtual supernumerary limb illusion (SLI) version, in which two embodiable virtual hands are presented to the participant via a head-mounted display. One virtual hand is thereby presented medially and the other laterally aside the participant's hidden real hand. Using this general setup, we examined the inducibility of a SLI in three consecutive experiments: Experiment 1 explored by which induction type (visuotactile congruency, visuothermal congruency, and visuomotor congruency) SLI experiences can be induced most robustly, Experiment 2 explored whether SLIs can be induced by a combination of these induction types, and Experiment 3 explored how visuoproprioceptive congruency influences the inducibility of a SLI. Sense of ownership toward the virtual hands was systematically assessed by means of experience sampling and by an implicit electrodermal embodiment measure. Results reveal a robust effect of stronger sense of ownership toward the medially than laterally presented virtual hand, while only a subgroup of participants (on average, across experiments: ~ 25%) reported concomitant sense of ownership toward both virtual hands (i.e., a full-blown SLI experience). The highest SLI responder rate (~63%) was observed for the combined application of visuotactile and visuothermal congruency, whereas a combined application of visuothermal and visuoproprioceptive congruency induced the lowest SLI responder rate (~4%). In conclusion, our study demonstrates that although under specific experimental conditions, a majority of participants can be made to experience a SLI, under most conditions, sense of ownership is only experienced toward one virtual hand. This indicates that multiple type-identical limb percepts are avoided to be co-instantiated by our brain, wherever possible.
Introduction
Under normal healthy conditions, we usually have no problems in correctly identifying our own body parts and we therefore unreservedly trust our bodily sensations. However, various clinical conditions exist under which the perception of our own body can be heavily distorted [for reviews, see; Synofzik et al. (2008), Braun et al. (2018)]. A particularly bizarre neuropsychiatric syndrome, under which patients perceive more limbs than they physically possess, is the supernumerary phantom limb (SPL) [for a review, see Cipriani et al. (2011)]. That is, these patients, for instance, perceive two right arms, whereas in reality, they only have one physical right arm.
While SPLs have been documented after stroke (Halligan et al., 1993; Srivastava et al., 2008; Khateb et al., 2009; Cipriani et al., 2011; Yoo et al., 2011), closed head injury (Rogers and Franzen, 1992), spinal cord injury (Curt et al., 2011), and during epileptic seizures (Millonig et al., 2011), their neurocognitive pathomechanisms remain unclear. Given that one essential mechanism of limb localization relies, among others, on the integration of efferent motor and afferent somatosensory signals, some consensus exists (Staub et al., 2006; Srivastava et al., 2008) that SPLs might result from a failure to integrate these two sources of information. In order to resolve the hereby emerging discrepancy, the brain assumes and instantiates a supernumerary limb. Another observation is that SPLs occur more often after right-hemispheric than left-hemispheric stroke. A systematic literature search by Srivastava et al. (2008), for instance, revealed that from the 21 stroke-induced SPL cases hitherto reported, 18 SPL cases were caused by a right-hemispheric stroke. Why this is the case has not been clarified yet, but Srivastava et al. (2008) allude to the particular involvement of the right hemisphere into monitoring somatic states and representations. Rogers and Franzen (1992), in turn, suggest that SPLs have at least partly also a “psychiatric etiology rather than a purely neurological foundation” (p. 469). To support their thesis, Rogers and Franzen (1992) presented a case report of a closed-head injury patient whose SPL experience was accompanied by a strong delusional conviction that his SPL perceived truly existed and was not just a pseudo-hallucination.
Considering the rarity of SPLs and the concomitant multitude of attempts to explain them, an experimental paradigm would be helpful with which SPLs could be transiently induced and systematically investigated in healthy participants. Even if such experimental paradigm would fail to accurately imitate the phenomenology of pathologically induced SPLs, the possibility of inducing SPLs experimentally would also be valuable for the experimental investigation of bodily self-awareness. In particular, it would allow addressing questions of how plastic and flexible one's own bodily self is and which sensory properties and top-down factors configure one's body matrix.
One possibility to induce SPL-like experiences is given by the supernumerary limb illusion (SLI). The SLI is a derivate of the famous rubber hand illusion [RHI; Botvinick and Cohen (1998)] and was introduced by Ehrsson (2009). In the original setting, the experimenter placed two anatomically aligned right artificial hands on a table in front of a participant, while the participant kept his or her real right hand hidden below the table. Next, the experimenter stroked both artificial hands and the participant's real hand for 60 to 120 s, either synchronously or asynchronously depending on the condition, before stabbing one of the two artificial hands with a syringe. While recording the participant's electrodermal activity (EDA), this procedure was repeated 12 times, pricking the syringe three times into each artificial hand for each condition. In the synchronous stroking condition, a stronger phasic EDA increase relative to the syringe applications was observed compared to the asynchronous stroking condition. Remarkably, these EDA differences thereby existed, regardless of whether the syringe was pricked into the more medial or more lateral artificial hand. Moreover, Ehrsson described that most of his participants reported “sensing the paintbrushes on both rubber hands” (Ehrsson, 2009, p. 310) and, in addition, a concomitant sense of ownership (SoO)—that is, a feeling of “mineness” and body-belonging—toward both artificial hands. For these observations, no systematic questionnaire data were, however, provided in the study.
Following up this pioneering study, Ehrsson's group conducted a further SLI study (Guterstam et al., 2011), in which they also acquired systematic questionnaire data. The experimental setup was slightly rearranged by using only one artificial hand and placing the participant's real hand directly laterally aside this artificial hand, such that both hands were in sight of the participant. As the authors of the study summarized, the experiment identified four factors that appear crucial to induce a SLI: First, the artificial hand needs to have the same hand laterality as the participant's real hand. Second, the object that is stroked must be an artificial hand and cannot be a non-hand-shaped object (i.e., an artificial foot). Third, the artificial hand needs to be placed in anatomical alignment (i.e., in an anatomically congruent position and posture with respect to the participant's real hand and body). Fourth, the stroking between the artificial hand and the participant's real hand must be synchronous.
Another SLI study (Folegatti et al., 2012) focused on spatial constraints that may restrict the inducibility of a SLI. For this purpose, the experimenters of the study placed two artificial hands side by side and medially to the hidden real hand of the participant on the table. An important finding in the present context was that the occurrence of a SLI (i.e., concomitant SoO toward both artificial hands) could not be induced in any experimental condition. Instead, if both artificial hands and the participant's real hand were stroked in synchrony, SoO was exclusively reported toward the more-medially positioned artificial hand. Hence, the authors concluded that both artificial hands must be equidistant to the participant's real hand in order to elicit a SLI.
A more active SLI setting was invented by Newport et al. (2010) using virtual reality (VR) technology. In this scenario, the participant saw a real-time video of his or her own moving left hand, which was actively stroking a brush. The video thereby overlaid the view onto the participant's real hand and showed two copies of it. In equidistance to the participant's real hand, the two virtual hands were thereby presented next to each other from a visuospatial perspective as if both hands originated from the participant's left shoulder. One important finding was that if both virtual hands were played in synchrony with the participant's real hand's stroking movements, SoO was reported for both virtual hands, whereas if only one virtual hand moved synchronously with the participant's own hand, SoO was exclusively reported for that virtual hand.
While the mentioned studies provide important evidence that SPL-like experiences might also be invocable in healthy participants, many research questions remain unaddressed. First, while the studies of Ehrsson (2009) and Guterstam et al. (2011) indicate that a SLI can be induced by visuotactile synchrony, Newport's (2010) study provides evidence that a SLI might also be inducible by visuomotor synchrony. What remains unanswered, however, is which induction type is most effective for inducing a SLI. While for the classical RHI, some empirical evidence exists for stronger SoO ratings under visuotactile synchrony than visuomotor synchrony (Dummer et al., 2009), other studies could not replicate this effect (Riemer et al., 2013; Kalckert and Ehrsson, 2014) (for a summary of findings, see Kalckert and Ehrsson, 2014). For the virtual hand illusion (VHI; i.e., the virtual derivate of the RHI), in turn, visuomotor synchrony turns out to be a quite potent VHI induction method (for a review, see Kilteni et al., 2015) thanks to new motion-tracking technologies that allow the provision of a rich and highly reliable visuomotor feedback. Hence, it remains uncertain which induction type is most potent in inducing a VR-based SLI. Second, the clinical SPL literature suggests a large variety in terms of the experiential character of SPLs [for a review and discussion, see Khateb et al. (2009)]. Many SPL patients, for instance, report that their two concurrent limb percepts differ in respect to which sensory modalities are predominantly experienced. This raises the question whether modality-specific SLIs might also be induced. This implies, for example, whether one tactile and one concomitant motoric hand percept may be experienced. Third, little is known about the spatial constraints for inducing a SLI. Although Folegatti et al.'s (2012) study provides the important finding that no SLI might be induced if both artificial hands are placed medially aside the participant's real hand, other spatial aspects remain uninvestigated. In particular, it remains to be examined how SoO is distributed to either artificial hand, if one artificial hand is placed medially aside and the other hand laterally aside the participant's real hand, or if one artificial hand is placed at the exact position of the participant's real hand and the other aside. Fourth, although three of the mentioned studies (Ehrsson, 2009; Newport et al., 2010; Guterstam et al., 2011) report successful SLI inductions, it appears that SLIs could only be induced in a small subsample of participants. Exact SLI responder rates (i.e., the percentage of participants reaching a pre-defined illusion criterion), have, however, not yet been reported.
Therefore, the aim of the present study was to complement the previous SLI work and to address these unresolved research questions. Inspired by the “virtual hand illusion” (Slater et al., 2008), we therefore implemented a VR-based SLI paradigm, in which the participant's real hand position and movements are translated to two concomitantly presented and realistically looking virtual hands, which the participant observes through a head-mounted display. Using this general setup, we examined the inducibility of a SLI in three consecutive VR experiments. Whereas, Experiment 1 examined by which induction type SLI experiences can be induced most robustly, Experiments 2 and 3 examined whether SLIs are also inducible by a combination of multiple induction types, so that SoO for one virtual hand is, for instance, induced by visuotactile congruency, whereas SoO for the other virtual hand is induced by visuomotor congruency. To systematically assess the participant's SoO experiences, we combined subjective and EDA measures and also evaluated SLI responder rates and limb-specific SoO values.
Methods Across Experiments
Participants
Twenty-four healthy subjects (three females, four left-handed, at an average age of ~25.167, SD = 7.966 years) participated in the study after giving their written informed consent. Participants were between 19 and 46 years of age, had corrected-to-normal or normal vision and reported no presence or history of a neurological or psychiatric disorder. All subjects received a monetary compensation of 20€ for their participation. The experiment was approved by the local medical ethics committee of the University Bonn (protocol number: 040–19). Due to technical malfunctioning, two participants did not fully complete Experiment 3, so that here the data could only be partially evaluated.
General Procedure
The study was carried out in the VR laboratory of the University Hospital of Bonn. In total, the study lasted around 60 min and consisted of three consecutive VR experiments. Participants were first informed about the purpose and content of the study and then gave their informed consent. Next, they filled out a lab-internal demographic questionnaire, while the experimenter prepared them for the VR experiments and concomitant neurophysiological recordings. Afterwards, participants were accustomed to the virtual testing environment, in which they first underwent a VR-familiarizing instruction phase, before the three actual VR experiments started. Upon completion of the VR experiments, participants were asked to fill out a motion-sickness questionnaire and the expense allowance form.
Apparatus and Virtual Environment
The experimental apparatus and virtual environment used are depicted in Figure 1. Throughout the three experiments, participants sat in front of a 1 × 1 m table in the middle of a 3.70 × 2.65 m VR-play area and placed both arms onto the table. The left hand became equipped with several neurophysiological sensors (for details see section EDA Analysis and Virtual Syringe Application), while the right hand remained cable-free and became subject of the SLI manipulations. Participants immersed into the virtual testing environment by means of the head-mounted display (HMD) HTC VIVE Business (HTC Corporation, Taoyuan City, Taiwan). This HMD has a 1,080 × 1,200 image resolution per eye, a 110-degree field of view, and a screen refresh rate of 90 Hz. Wearing this HMD, the participants immersed into a virtual recreation of our experiment room that contained the same overall furniture as the real experiment room. By means of positional tracking, the physical and virtual room were thereby spatially mapped, such that the participant's viewing angle in real space corresponded to the HMD's viewing angle in the virtual space. That is, as in the real world, the participants found themselves situated in the virtual world in front of a 1 × 1 m virtual table. The virtual environment was created within Unity 3D 2018.3.4f1 (Unity Technologies, San Francisco, CA, USA) and its interaction logic was coded in C#. Each experimental condition was implemented as a separate Unity scene, and a scene controller script coordinated the iterative invocation of the distinctive scenes. Some 3D objects of the virtual room were self-created by means of Blender 2.79b (Stichting Blender Foundation, Amsterdam, Netherlands), others were purchased.
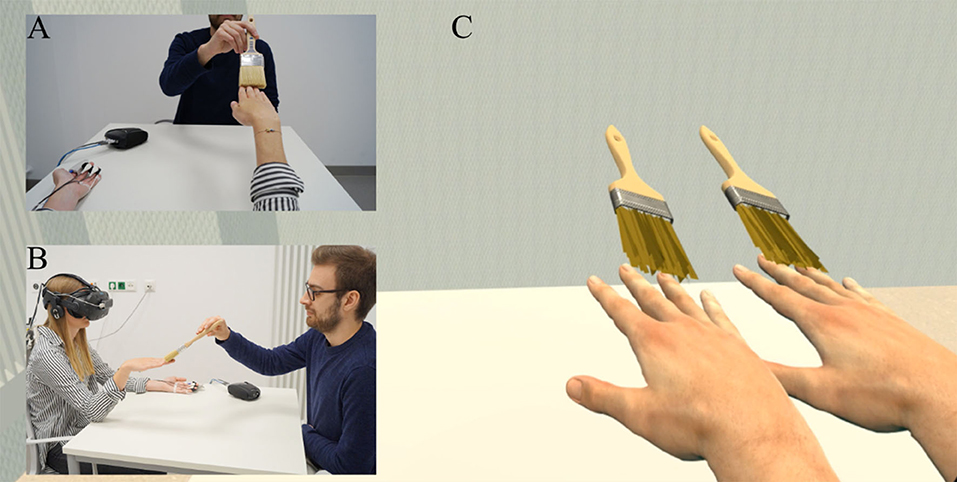
Figure 1. Experimental apparatus. (A) First-person perspective of participant in the real world. Wearing a head-mounted display (HMD), participants sat in front of a table and held their right hand in front. The experimenter was sitting at the opposite of the table, from where he was, depending on the condition, stroking or thermostimulating the participant's real hand. (B) Lateral view onto the experimental setup. (C) First-person perspective of the participant in the virtual world (HMD view). Data privacy remark: The persons shown on the figure agreed with the publication of this figure.
Throughout the experiments, two virtual right hands were concomitantly shown to the participant. Both virtual hands were presented side by side in a 3D orientation, as if both virtual hands were originating from the participant's own right shoulder. The virtual hand that was closer to the participant's body midsagittal line was referred to as the medial virtual hand (MVH), whereas the virtual hand that was more distant to the participant's midsagittal line was called lateral virtual hand (LVH). Both the MVH and LVH looked identical and were based on a realistically looking, white-colored, and average-sized 3D human hand model out of the “Leap Motion Realistic Hands” collection (available over Unity's asset store). The relative positions of both virtual hands and their postures were real-time coupled to the participant's real hand. That is, whenever the participants moved their biological right hand in the real space, the two virtual hands always moved correspondingly and without noticeable delay in the virtual space. Technically, this motion-tracking of the participants' real right hand was realized by the LeapMotion system (Leap Motion Incorporation, San Francisco, CA, USA). This system consists of a small USB device that is mounted on top of the HMD and allows the tracking of hand movements in real time using optical sensors and infrared light (Bachmann et al., 2018). Software functionalities are provided as a ready-to-use software development kit (SDK) for Unity 3D (https://developer.leapmotion.com/unity; accessed 13.03.20). Using this SDK, we tracked the participant's right hand real-time positions and movements and translated them to the two rigged virtual hand models. The participant's left hand, in turn, was not motion-tracked and not represented in the virtual environment throughout the experiment.
Instruction Phase
Before the actual experiments began, an instruction phase was preceded, during which the participants became familiarized with the virtual environment. First, the participants were taught on how to conduct artifact-free virtual limb movements. To this end, they were shown some prototypical motor movements (e.g., slow grasping movement) that are easily recognizable by the Leap Motion system and known to not cause visual distortions. Next, after the participants had learnt to conduct artifact-free virtual hand movements, they were taught in how to use our VR-embedded user interface (UI) that was later used for experience sampling (details below). As illustrated in Figure 2A, the UI was directly displayed within the virtual environment as a semi-transparent overlay appearing around 80 cm in front of the participant. The participants could easily control the UI by mere gesture control. To this end, the participants just hold their virtual index finger (during experience sampling, only one virtual right hand was blended in) on the desired answer button until it turned green after 2 s and confirmed their answer by holding their virtual index finger on a confirm button for another 2 s.
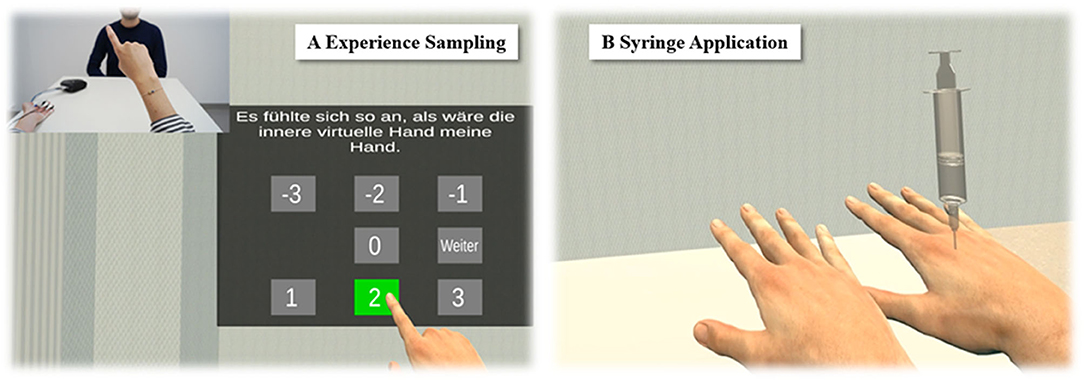
Figure 2. Explicit and implicit assessment of SLI experiences. (A) Experience sampling. To explicitly assess the participants' SLI experiences without having them to leave the virtual environment, a user interface showed up after each trial, by means of which participants rated their phenomenal experiences during the just finished trial. (B) Syringe application. To implicitly assess the participants' level of embodiment toward the virtual hands, a virtual syringe was injected into either virtual hand after each trial from Experiment 1.
Experience Sampling
Throughout experiments, a UI appeared at the end of each trial in order to survey the participants about their phenomenal experiences with the just finished trial. The UI thereby guided the participants through a small SLI questionnaire (see Table 1) that was adopted from previous studies (Kalckert and Ehrsson, 2012; Braun et al., 2014) and remained identical throughout the three experiments. Each item was separately displayed in a pseudorandomized order and the participants had to indicate their level of agreement to the currently displayed item on a seven-point Likert scale that varied from −3 (“totally disagree”) to +3 (“totally agree”). One item related to SoO toward the MVH (Q1: “It felt like the inner virtual was my hand”), another item to SoO toward the LVH (Q2: “It felt like the outer virtual hand was my hand”), and a third item to concomitant SoO toward both virtual hands (Q3: “It felt like I had two right hands”). Moreover, a SoO control item (Q4: “It felt like my right hand was disappearing, like I didn't have a right hand anymore”) was entailed in the questionnaire, which was illusion-related, but did not specifically capture the phenomenal experience of SoO. Hence, in the case of a successful SLI induction, we expected that the SoO items should have high affirmative ratings, whereas the SoO control item should not be specifically affected by our experimental manipulations. Besides these SoO-related items, the questionnaire also entailed some sense of agency (SoA)-related questions, which were, however, not the subject of the present study, and will be reported elsewhere. As in former studies (Kalckert and Ehrsson, 2012; Braun et al., 2014, 2016), the illusion threshold to confirm a successful SoO induction toward one or both virtual hands was set to ≥1.
For the statistical analyses, we calculated several average scores for each participant, each experimental condition, and each induction type: SoO values toward the MVH (i.e., the average response to Q1 across trials), SoO values toward the LVH (i.e., the average response to Q2 across trials), SLI values (i.e., the average response to Q3 across trials), and SoO control values (i.e., the average response to Q4 across trials). Moreover, for each just-mentioned average score, we calculated the belonging illusion-responder rate, which we defined as the percentage of participants that indicated a SoO value above the defined illusion threshold for the respective average score.
Experiment 1
Experiment 1 investigated by which induction type SLI experiences can be induced most robustly. For this purpose, three induction types that are typically used within RHI experiments were compared: visuotactile congruency, visuothermal congruency, and visuomotor congruency.
Methods
Study Design
The study design for Experiment 1 is depicted in Figure 3. Every induction type represented one experimental condition, and for each condition, participants performed two trials. The condition order and the trial order were counterbalanced across participants. Apart from the induction type applied, all trials were identically structured, regardless of the condition they were affiliated to: First, in order to make participants aware of the beginning of the new trial, a 10-s countdown was blended in overlaying the view onto the virtual experiment room. Next, the virtual experiment room and two virtual right hands were displayed, one hand 14 cm medially aside the participant real hand's position and the other 14 cm laterally aside. Hence, the distance between the MVH and participant's real hand equaled the distance between the LVH and participant's real hand. As soon as the two virtual hands were shown, the condition-dependent SLI induction phase started.
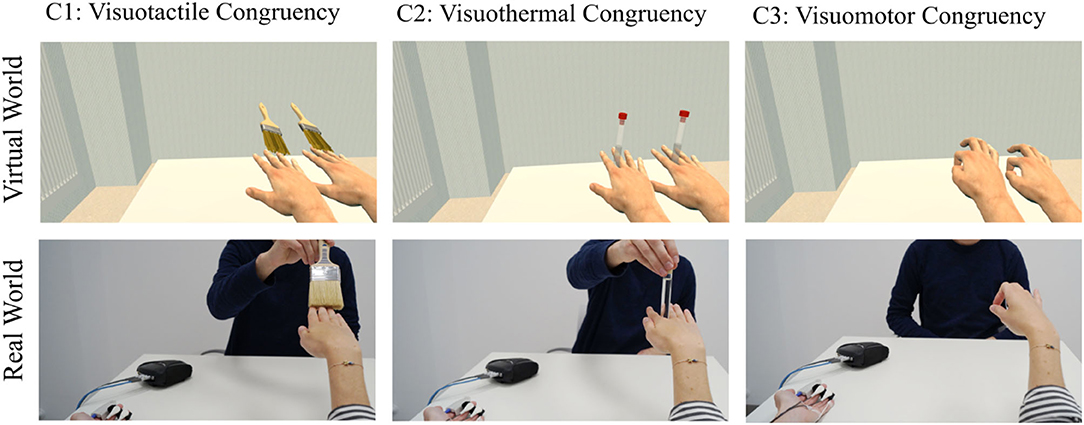
Figure 3. Study design of Experiment 1. Three different SLI induction types were compared. While in Condition 1, the two virtual hands were stroked in synchrony to the stroking of the participant's real hand, in Condition 2, all three hands appeared to be synchronously thermostimulated. In Condition 3, the two virtual hands realistically imitated the participant's momentary real hand's movements.
For the trials of the visuotactile condition, both virtual hands as well as the real hand were synchronously stroked with a brush (Figure 3, left panels). To this end, two virtual brushes were shown synchronously stroking either virtual hands in a 0.5-Hz regular rhythm, while the experimenter stroked the participant's real hand with a real brush in the same rhythm. To synchronize the stroking patterns, the experimenter followed the virtual scene on a computer screen and adjusted his stroking to the seen stroking. For the visuothermal condition (Figure 3, middle panels), a test tube filled with warm water was stuck between the real index and middle fingers of the participant, while each virtual hand also held a test tube between its index and middle finger. Lastly, for the visuomotor condition (Figure 3, right panels), participants conducted grasping movements with their own hand, while the two virtual hands mirrored these movements in real time. The SLI induction phase lasted 60 s. Immediately after its end, a virtual syringe appeared in the scene (Figure 2B) which was slowly pricked into one of the two virtual hands. For each condition, the virtual syringe stabbed once into the MVH, and once into the LVH, whereby the order of the syringe injection was counterbalanced across all participants. Finally, after the syringe application, the UI appeared, by which the participants reported their phenomenal experiences for the present trial.
EDA Analysis and Virtual Syringe Application
Following previous RHI studies (Ocklenburg et al., 2011; Alimardani et al., 2013, 2014; Braun et al., 2016), EDA was recorded during the syringe applications and used as an implicit measure for virtual hand embodiment. In line with the previous studies, we expected that if the virtual hands were experienced as part of the own body and not just as some external object, the syringe applications would induce a physiologically measurable fear response. Furthermore, we assumed that the deflection of this transient EDA increase would be indicative for the level of virtual hand embodiment. Hardware-wise, the participant's EDA was acquired using the Nexus 10 device (Mind Media BV, Herten, Netherlands), which connects to the computer via Bluetooth. Two skin conductance sensors were attached to the middle and ring finger of the left hand. To avoid recording artifacts, participants had to rest the left hand on the table throughout the experiment. Software-wise, the continuous EDA signal was digitized with a sampling frequency of 256 Hz using a self-adapted SDK provided by the manufacturer that allows recording EDA data via LabStreamingLayer (LSL; https://github.com/sccn/labstreaminglayer). For the offline EDA data analysis, the EDA data were imported into Matlab R2018a and analyzed by the toolbox LEDALAB v3.4.9 (Benedek and Kaernbach, 2010a,b). This toolbox allows conducting a continuous decomposition analysis (CDA) by which an EDA signal may be decomposed into its tonic and phasic EDA parts (Benedek and Kaernbach, 2010a). For each participant, the resulting phasic EDA part was retained and z-scored by calculating the mean and SD across all data samples and subtracting this mean from each data sample and dividing the resulting value by the SD. Next, the z-scored phasic EDA part was segmented from −2 s to +12 s relative to the start of the syringe applications. For the statistical analysis, mean phasic EDA responses were separately extracted for each condition of Experiment 1 and each virtual hand. To this end, we calculated the mean amplitude for the +5 s to +11 s time interval, relative to the syringe applications.
Statistical Analyses
Experiment 1 included six dependent variables (SLI values, SLI responder rates, MVH-related SoO, LVH-related SoO, MVH-related EDA responses, and LVH-related EDA responses) and one control variable (SoO control values), from which all further statistics were derived. Three major statistical analyses were conducted:
First, to ensure that our experimental manipulations only affected the core phenomenology of virtual hand embodiment and not some more generic illusion effect, pairwise comparisons between SLI values and SoO control values were separately carried out for each condition. We thereby reasoned that in case of a successful virtual hand embodiment, SLI values would be significantly higher than SoO control values, since only SLI values should be affected by our experimental manipulation, but not the SoO control values.
Second, to investigate the differences in SLI strength between the three induction types, a one-way repeated measures ANOVA was conducted on the SLI values of the three compared experimental conditions. In case that the ANOVA was significant, this effect was followed up by planned pairwise comparisons (post hoc t-tests) for each possible condition pair (i.e., visuotactile congruency vs. visuothermal congruency, visuotactile congruency vs. visuomotor congruency, and visuothermal congruency vs. visuomotor congruency). The same type of ANOVA was also conducted on the hand-specific EDA response values.
Third, to explore potential SoO differences toward the MVH and LVH, which might be interacting with the induction types applied, we conducted an additional 3 × 2 repeated measures ANOVAs with the factor Hand Side (MVH vs. LVH) and the factor Induction (visuotactile, visuothermal, and visuomotor congruency) on the MVH- and LVH-related SoO values.
Throughout the paper, effect sizes are reported by Cohen's d for the t-tests and partial η2 for the ANOVAs.
Results
Questionnaire Data
Questionnaire data for Experiment 1 is depicted in Figure 4. SLI values (i.e., concomitant SoO toward both hands) are depicted in green, whereas exclusive SoO values toward the MVH are shown in blue, exclusive SoO values toward the LVH in red, and control questions in orange. Regarding SLI values, the a priori defined illusion criterion (+1) was reached in 25% of the participants for the visuotactile condition (M = −0.646, SD = 1.850), ~8% for the visuothermal condition (M = −1.396, SD = 1.452), and ~46% for the visuomotor condition (M = −0.083, SD = 2.062). SoO control values, in turn, were clearly below the illusion criterion for each participant and each condition (on average, across conditions: M = −2.368, SD = 0.912), confirming the illusion specificity of Experiment 1. The planned tests between the SLI values and SoO control values were significant for the visuotactile condition [t(23) = 3.622; p < 0.001; d = 0.921], visuothermal condition [t(23) = 2.567; p = 0.017; d = 0.644], and visuomotor condition [t(23) = 5.300; p < 0.001; d = 1.254].
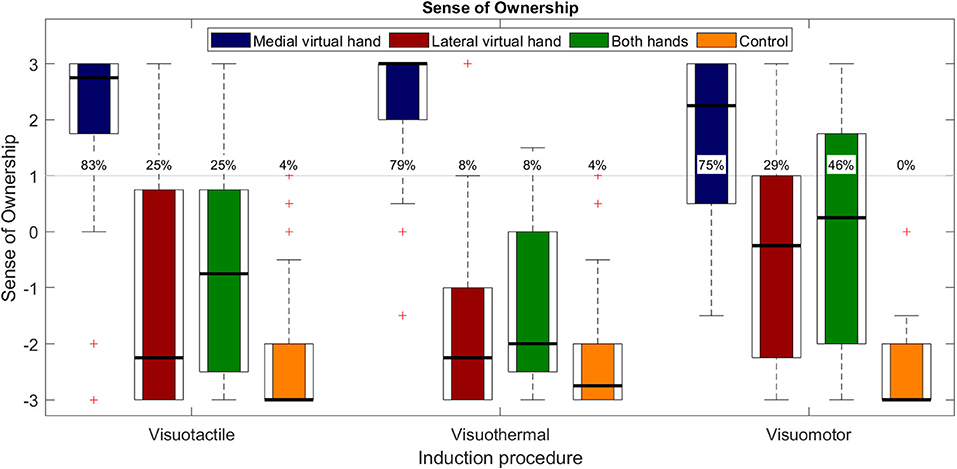
Figure 4. Questionnaire results for Experiment 1. Boxplots with medians (black lines), IQR (box edges), and outliers (whiskers). The SoO values toward the MVH are depicted in blue, the SoO values toward the LVH are depicted in red, the SLI values (i.e., concomitant SoO toward both virtual hands) are depicted in green, and the SLI control values are depicted in yellow. The gray line indicates the pre-defined illusion (+1) threshold and the percentages specify the illusion responder rates for each operationalized phenomenal target property for N = 24 subjects.
A one-way repeated measures ANOVA on SLI scores revealed a significant main effect for induction [F(2,46) = 5.449; p = 0.008; η2 = 0.192] that was followed up by our planned pairwise comparisons. Planned pairwise comparisons were conducted for each induction pair. Significantly stronger SLI values were reported for the visuomotor condition as compared to the visuothermal condition [t(23) = −2.922, p = 0.008; d = 0.696]. Moreover, a trend effect for stronger SLI values was found in the visuotactile condition compared to the visuothermal condition [t(23) = 2.055; p = 0.051; d = 0.444], while no significant difference emerged between the visuotactile and visuomotor condition [t(23) = −1.490; p = 0.150; d = 0.287].
Regarding SoO toward the MVH and LVH, strong affirmative SoO ratings were given for the MVH conditions (on average, across conditions: M = 1.958, SD = 0.989; illusion-responder rate: ~79%), whereas rather low SoO ratings were given for the LVH conditions (on average, across conditions: M = −1.104, SD = 1.367; illusion-responder rate: ~21%). This graphical impression was also confirmed by our 3 × 2 repeated measures ANOVA that revealed a main effect of hand side [F(1,23) = 65.867; p < 0.001; η2 = 0.741], in that, across conditions, stronger SoO was reported for the MVH than LVH. Besides that, the ANOVA also revealed a trend for a main effect of induction [F(2,46) = 3.162; p = 0.052; η2 = 0.121], in line with the abovementioned condition effect for the SLI values, and no interaction effect [F(2,46) = 2.168; p = 0.126; η2 = 0.086].
Phasic EDA Responses
Phasic EDA responses are shown in Figure 5. The three subplots represent the three different conditions and the blue EDA time curves and bars represent the MVH conditions, while the red time curves and bars represent the LVH conditions. In all three conditions, an increase in phasic EDA was observable shortly after the syringe injection into either virtual hand. The strongest EDA increase was found in the MVH-visuotactile condition (M = 1.370; SD = 1.333) and the weakest was found in the LVH-visuotactile condition (M = 0.563; SD = 0.864). A 3 × 2 repeated measures ANOVA revealed a significant main effect for hand side [F(1,23) = 12.903; p = 0.002; η2 = 0.359], but no main effect for induction [F(2,46) = 0.033; p = 0.968; η2 = 0.001] and no interaction effect [F(2,46) = 2.057; p = 0.139; η2 = 0.082]. The effect of hand side consisted in significantly stronger phasic EDA responses for the MVH than LVH conditions.
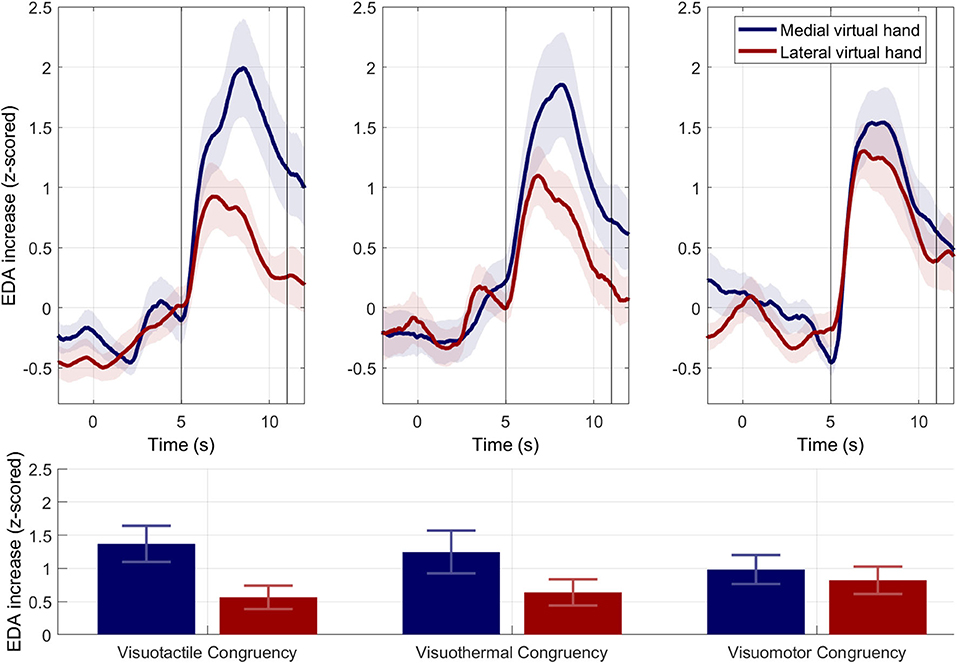
Figure 5. Electrodermal results for Experiment 1. Phasic EDA responses. Upper panels. Mean phasic EDA responses (±SEMs) across subjects for the MVH (in blue) and LVH (in red) during syringe injections. Time point zero indicates the beginning of the syringe application. Lower panel. Mean phasic EDA responses (±SEMs) for the extracted time interval between 5 and 11 s. Across conditions, mean phasic EDA responses were significantly stronger for the syringe injections applied to the MVH than LVH.
Associations Between SoO and Phasic EDA Responses
Pearson correlations without Bonferroni adjustments between SoO values and phasic EDA responses are depicted in Table 2. A high (r = 0.465; p = 0.022) positive correlation was found between SoO values and EDA responses in the visuotactile condition for the MVH. No further significant associations were found for any remaining comparison.
Experiment 2
Experiment 2 followed up our hypothesis that SLIs might be also inducible by a combination of induction types, such that SoO for one virtual hand is induced by one induction type and SoO for the remaining virtual hand is induced by another.
Methods
Study Design
To address our hypothesis, we compared three different induction type combinations (Figure 6): visuotactile–visuothermal congruency, visuothermal–visuomotor congruency, and visuotactile–visuomotor congruency. As in Experiment 1, every induction type combination represented one condition, participants performed two trials per condition, and the condition order was counterbalanced across participants. Likewise, apart from the induction type combination applied, the overall trial structure was identical for each trial and almost identical to the trial structure of Experiment 1. The only difference was that the syringe application procedure was spared due to expected habituation effects. Each induction type combination was realized by combining the described procedures for the individual induction types. As an example, for the visuotactile–visuothermal condition (cf. Figure 6, left panels), the previously described procedure for the visuotactile induction was combined with the visuothermal induction method. More specifically, the participant's real right hand was concomitantly stroked and thermostimulated, while in the virtual environment, one virtual hand was visually stroked and the other was visually thermostimulated. Across both trials, it was counterbalanced which virtual hand was visually stroked and which was visually thermostimulated. The same logic of combining the two induction types was also applied to the remaining conditions.
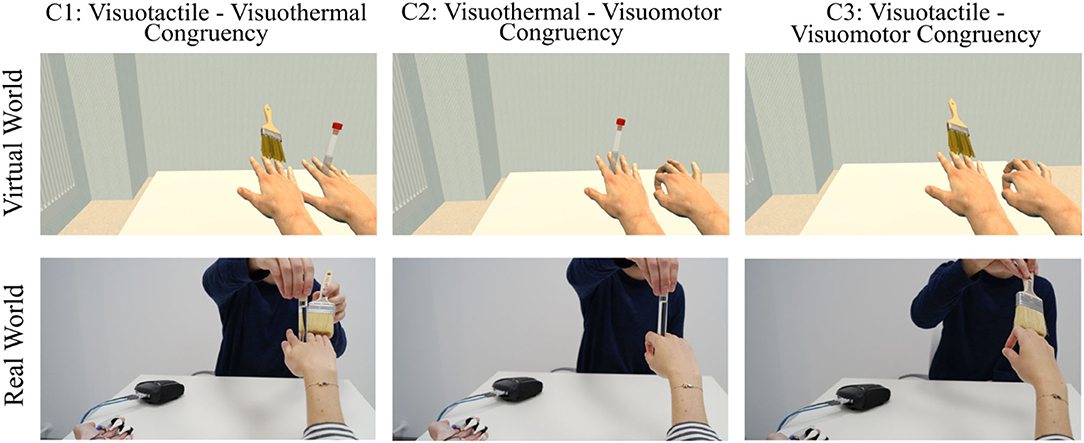
Figure 6. Study design of Experiment 2. Three different SLI induction-type combinations were compared. For Condition 1, for example, the visuotactile induction was combined with the visuothermal induction, in that the participant's real right hand was concomitantly stroked and thermostimulated, while in the virtual environment, one virtual hand was visually stroked and the other was visually thermostimulated.
Statistical Analyses
With the exception of EDA values, Experiment 2 included the same dependent variables and control variables as Experiment 1. Moreover, the conducted statistical analyses were quite similar: First, pairwise comparisons between SLI values and SoO control values were separately carried out for each condition, in order to validate that our experimental manipulations only affected the core phenomenology of virtual hand embodiment. Next, to compare the SLI strengths between the three induction type combinations applied, a one-way repeated measures ANOVA was conducted on the SLI values of the three conditions compared. In case that the ANOVA was significant, this effect was followed up by planned pairwise comparisons (post hoc t-tests) for each possible condition pair. Finally, to explore how potential SoO differences toward the MVH and LVH might be interacting with the induction types applied, we separately conducted 2 × 2 repeated measures ANOVAs with the factor Hand Side and the factor Induction (first induction type, second induction type) for each experimental condition (i.e., each induction type combination).
Results
SLI values for each induction type combination of Experiment 2 are depicted in Figure 7. SLI values (in green) above the a priori defined illusion criterion (+1) were reported in ~63% of the participants for the visuotactile–visuothermal condition (M = 0.854, SD = 1.948), 25% for the visuothermal–visuomotor condition (M = −0.771, SD = 1.950), and ~29% for the visuotactile–visuomotor condition (M = −0.188, SD = 1.614). SoO control values, in turn, were clearly disaffirmative in all participants for all three experimental conditions (on average, across conditions: M = −2.392, SD = 0.915, responder rate: ~3%), confirming the illusion specificity of Experiment 2. Planned paired t-tests between the SLI values and SoO control values were significant for the visuotactile–visuothermal condition [t(23) = 6.046; p < 0.001; d = 1.405], visuothermal–visuomotor condition [t(23) = 3.124; p = 0.005; d = 0.847], and visuotactile–visuomotor condition [t(23) = 6.194; p < 0.001; d = 1.345].
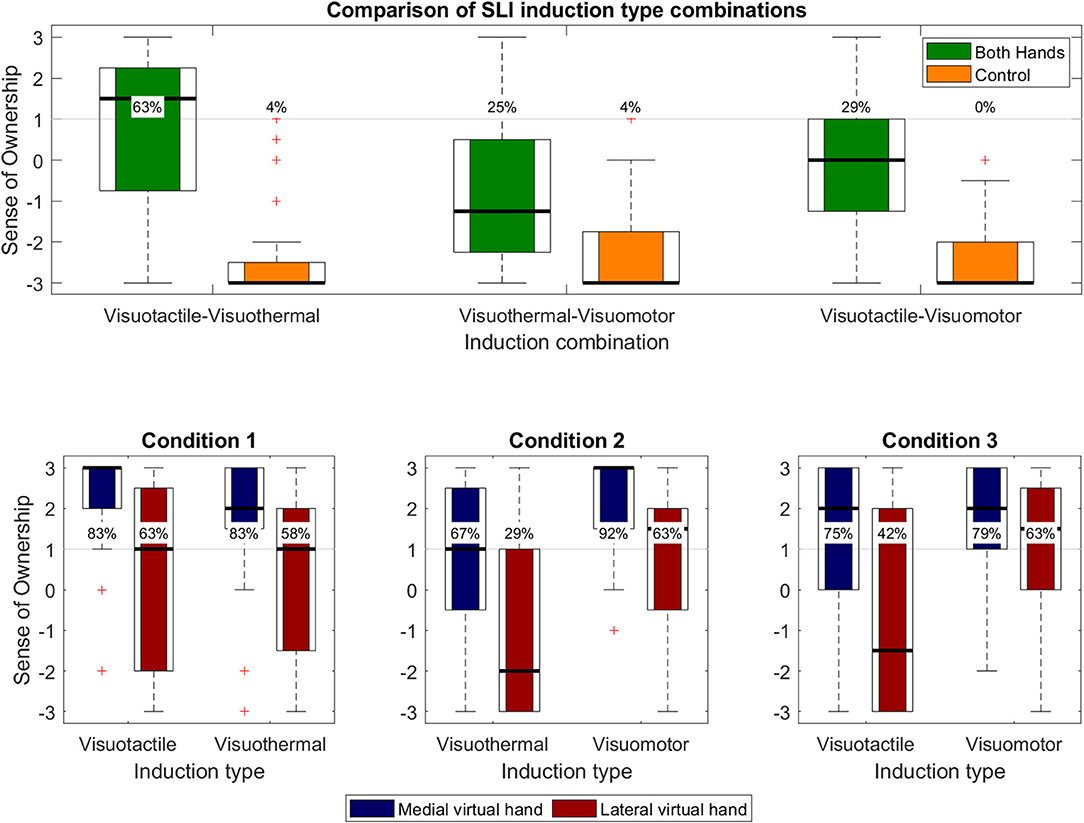
Figure 7. Questionnaire results of Experiment 2. Upper panel. Overall SLI values (in green) and SLI control values (in orange). Percentages specify the illusion responder rates for each operationalized construct. Lower panels. Each panel represents one condition and separately shows the limb-specific SoO values toward the MVH (in red) and LVH (in blue) for both applied induction types.
A one-way repeated measures ANOVA revealed a significant main effect [F(2,46) = 10.443; p < 0.001; η2 = 0.312] between the three experimental conditions, which was followed up by planned pairwise comparisons. The t-test between the visuotactile–visuothermal condition and visuothermal–visuomotor condition was significant [t(23) = 4.749; p < 0.001; d = 0.775], in that the combined induction of visuotactile congruency and visuothermal congruency induced stronger SLI experiences than the combined induction of visuothermal congruency and visuomotor congruency. Moreover, higher SLI values were also reported for the visuotactile–visuothermal condition as compared to the visuotactile–visuomotor condition [t(23) = 2.632; p = 0.015; d = 0.564], while no significant difference was found between the visuothermal–visuomotor condition and visuotactile–visuomotor condition [t(23) = −1.715; p = 0.100; d = −0.325].
Condition 1: Visuotactile Congruency vs. Visuothermal Congruency
A 2 × 2 repeated measures ANOVA revealed a significant main effect for hand side [F(1,23) = 15.751; p < 0.001; η2 = 0.406], but no main effect for induction [F(1,23) = 0.397; p = 0.535; η2 = 0.017] or an interaction effect [F(1,23) = 0.083; p = 0.776; η2 = 0.004]. The effect of hand side consisted in stronger SLI values for the MVH than LVH conditions.
Condition 2: Visuothermal Congruency vs. Visuomotor Congruency
A 2 × 2 repeated measures ANOVA revealed a significant main effect for hand side [F(1,23) = 14.804; p < 0.001; η2 = 0.392] as well as an induction main effect [F(1,23) = 14.842; p < 0.001; η2 = 0.392], but no interaction effect [F(1,23) = 1.381; p = 0.252; η2 = 0.057]. The effect of hand side consisted in stronger SLI values for the MVH than LVH conditions, whereas the induction effect consisted in stronger SoO values for the visuomotor than the visuothermal condition.
Condition 3: Visuotactile Congruency vs. Visuomotor Congruency
A 2 × 2 repeated measures ANOVA revealed a significant main effect for hand side [F(1,23) = 6.687; p = 0.017; η2 = 0.225], in that stronger SoO values were reported for the MVH than outer LVH conditions. No main effect for induction was found [F(1,23) = 2.920; p = 0.101; η2 = 0.113], but a trend interaction effect [F(1,23) = 4.123; p = 0.054; η2 = 0.152].
Experiment 3
Experiment 3 investigated spatial constraints for inducing a SLI. More specifically, it was asked how SoO is allocated to the virtual hands, if one virtual hand is presented at the participant's exact real hand location (i.e., a situation where there is full visuoproprioceptive congruency), but neither moved nor stimulated, whereas the other virtual hand is displayed medially or laterally aside, but moved or stimulated. The rationale behind this setup was that most clinical SPL cases take place against the backdrop of a limb paresis (i.e., the limb that is experientially instantiated twice has typically become paretic).
Methods
Study Design
Three different induction type combinations were compared (Figure 8): visuotactile–visuoproprioceptive congruency, visuothermal–visuoproprioceptive congruency, and visuomotor–visuoproprioceptive congruency. As in Experiments 1 and 2, every induction type combination represented one condition and the condition order was counterbalanced across participants. Again, there were two counterbalanced trials per condition. The two trials differed in that in one trial, the MVH matched the actual hand position of the participant and the LVH was stimulated or moved, whereas in the other trial, the LVH matched the participant's real hand position and the MVH was stimulated or moved. The trial structure of Experiment 3 was identical to the trial structure of Experiment 2.
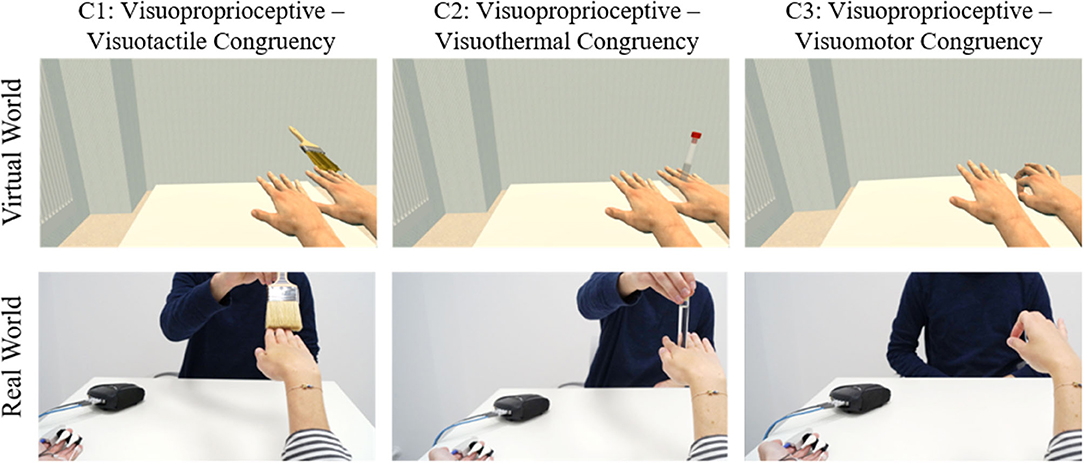
Figure 8. Study design of Experiment 3. Experiment 3 investigated how SoO is allocated to the virtual hands, if one virtual hand is presented at the participant's exact real hand location (i.e., a situation, where there is full visuoproprioceptive congruency), but not moved or stimulated, whereas the other virtual hand is displayed medially or laterally aside (as here illustrated), but moved or stimulated.
Statistical Analysis
Experiment 3 included the same dependent and control variables as Experiments 1 and 2, and the statistical analyses conducted were identical to Experiment 2.
Results
SLI values for each experimental condition of Experiment 3 are shown in Figure 9. SLI values above the a priori defined illusion criterion (+1) were reported in ~8% of the participants for the visuotactile–visuoproprioceptive condition (M = −1.091, SD = 1.525) and ~4% for the visuothermal–visuoproprioceptive condition (M = −1.864 SD = 1.125), while ~13% of the participants reported a SLI experience for the visuomotor–visuoproprioceptive condition (M = −1.000 SD = 1.485). SoO control values were clearly disaffirmative in all three experimental conditions (across conditions: M = −2.470, SD = 0.625). The planned t-tests between the SLI values and SoO control values were significant for the visuotactile–visuoproprioceptive condition [t(21) = 3.650; p = 0.001; d = 0.965], visuothermal–visuoproprioceptive condition [t(21) = 2.093; p = 0.049; d = 0.592], and visuomotor–visuoproprioceptive condition [t(22) = 4.860; p < 0.001; d = 1.037].
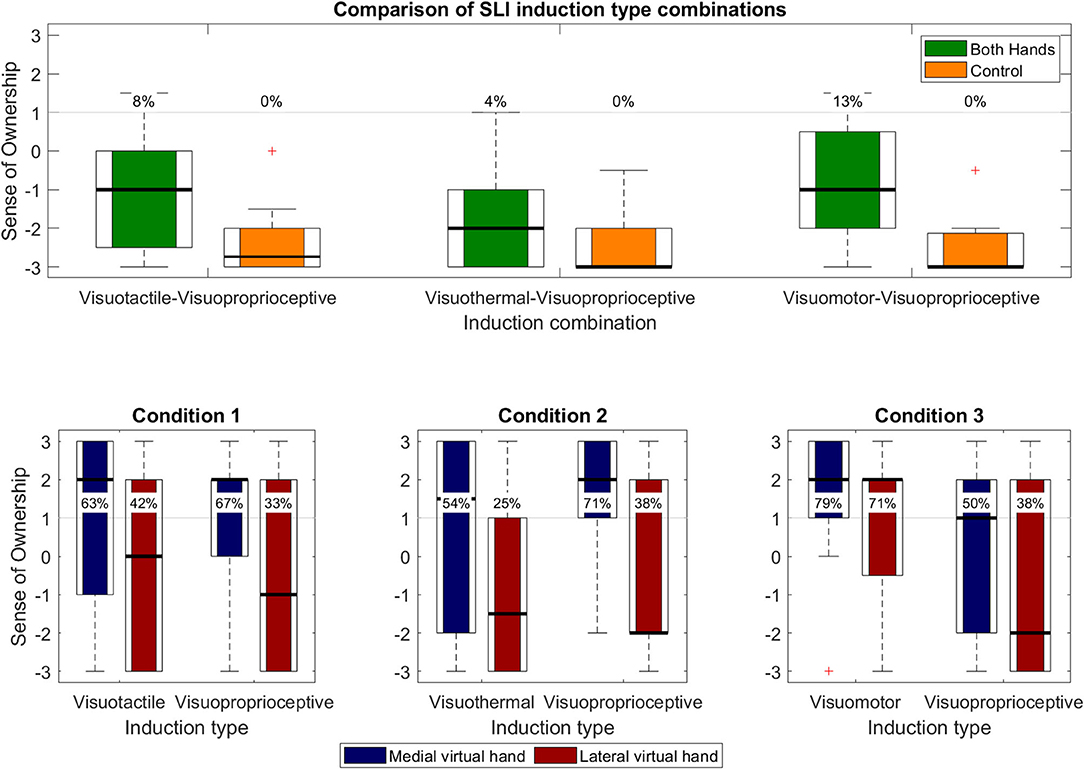
Figure 9. Questionnaire results of Experiment 3. Upper panel. Overall SLI values (i.e., concomitant SoO toward both virtual hands) and SLI control values. Percentages depict illusion responder rates for each operationalized construct. Lower panels. Each panel represents one of the three conditions and separately shows the limb-specific SoO values for that condition.
A one-way repeated measures ANOVA revealed a significant main effect [F(2, 42) = 6.045; p = 0.005; η2 = 0.224] between the three experimental conditions, which was followed up by planned pairwise comparisons. The t-test between the visuotactile–visuoproprioceptive condition and visuothermal–visuoproprioceptive condition was significant [t(21) = 2.966; p = 0.007; d = 0.560], in that stronger SLI values were reported for the visuotactile–visuoproprioceptive condition than the visuothermal–visuoproprioceptive condition. Moreover, higher SLI values were also reported for the visuomotor–visuoproprioceptive condition as compared to the visuothermal–visuoproprioceptive condition [t(21) = −3.112; p = 0.005; d = 0.621], while no significant difference was found between the visuotactile–visuoproprioceptive condition and visuomotor–visuoproprioceptive condition [t(21) = −0.324; p < 0.749; d = 0.060].
Condition 1: Visuotactile Congruency vs. Visuoproprioceptive Congruency
A 2 × 2 repeated measures ANOVA revealed a significant main effect for hand side [F(1,21) = 8.984; p = 0.007; η2 = 0.300], but no main effect for induction [F(1,21) = 0.001; p = 0.971; η2 = 0.000] or an interaction effect [F(1,21) = 2.072; p = 0.165; η2 = 0.090]. The effect of hand side consisted in stronger SLI values for the MVH than LVH conditions.
Condition 2: Visuothermal Congruency vs. Visuoproprioceptive Congruency
A 2 × 2 repeated measures ANOVA revealed a significant main effect for hand side [F(1,21) = 9.925; p = 0.005; η2 = 0.321], but no main effect for induction [F(1,21) = 1.214; p = 0.283; η2 = 0.055] or an interaction effect [F(1,21) = 2.646; p = 0.119; η2 = 0.112]. The effect of hand side consisted in stronger SLI values for the MVH than LVH conditions.
Condition 3: Visuomotor Congruency vs. Visuoproprioceptive Congruency
A 2 × 2 repeated measures ANOVA revealed a significant main effect for hand side [F(1,22) = 5.217; p < 0.032; η2 = 0.192] and for induction [F(1,22) = 4.939; p = 0.037; η2 = 0.183], but no interaction effect [F(1,22) = 0.107; p = 0.747; η2 = 0.005]. The effect of hand side consisted in stronger SLI values for the MVH than LVH conditions, whereas the induction effect consisted in stronger SoO values for the visuomotor than visuoproprioceptive congruency condition.
Comparison of SLI Responder Rates Across Experiments
Besides experiment-specific calculations, we also descriptively compared the different experimental conditions across experiments. A summarizing comparison of SLI responder rates across experiments is given in Figure 10. This comparison should, however, be cautiously interpreted, given that only the order of conditions within each experiment was counterbalanced, but not the experiment order. The highest SLI responder rate was reported for the combined induction of visuotactile congruency and visuothermal congruency (~63%) and the lowest for the combined induction of visuoproprioceptive and visuothermal congruency (4%). The average SLI responder rate across conditions was ~25%.
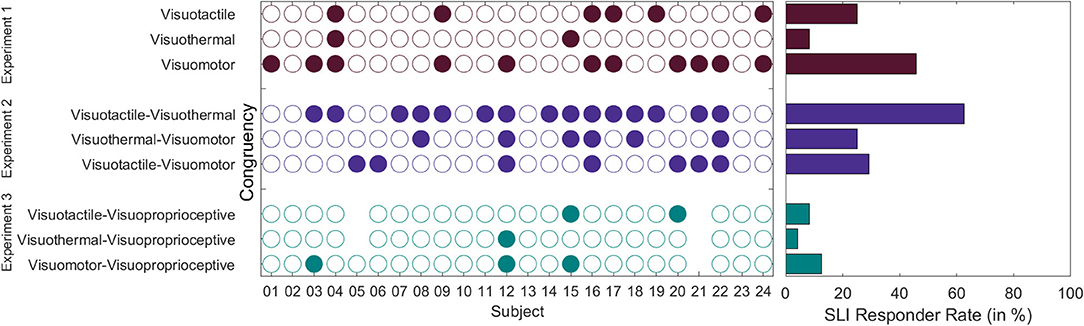
Figure 10. SLI responder rates across experiments. Left panel. Individual listing in which experimental conditions the participants experienced a SLI. Right panel. SLI responder rates (in %) across participants. SLI responder rates should be compared with caution, given that only the condition order within experiments, but not the experiment order was counterbalanced across participants.
Moreover, we investigated whether there was a “SLI pre-disposition” in that SLI occurrences were not randomly distributed between participants, but that those participants experiencing a SLI in one condition were also more likely to experience a SLI in a further condition. To this end, we calculated the SLI agreement level between experimental conditions by means of Fleiss's Kappa (Fleiss, 1971). Each condition was thereby regarded as a separate rating instrument for judging the presence or absence of a SLI. The result is that some participants appear to be more susceptible toward a SLI experience than others. That is, there was “slight” inter-conditional agreement (K = 0.098; Z = 2.766; p = 0.006) in that SLI experiences were not randomly distributed between participants, but those participants experiencing a SLI in one condition were also more likely to experience a SLI in a further condition.
Discussion
The aim of the present study was to complement previous SLI studies in respect to which induction types suit best for inducing a SLI (Experiment 1), whether a SLI can also be induced by a combined application of multiple induction types (Experiment 2), and how visuoproprioceptive congruency affects the invocability of a SLI (Experiment 3). In brief, our study reveals three major results: First, SLI experiences appear to be only invocable in a minority of participants. Second, the majority of participants, instead, only report SoO toward the more medially presented MVH. This midsagittal line effect even occurs if the LVH is placed at the participant's exact real hand position. Third, SLI experiences can also be induced by a combined application of multiple SLI induction types, especially if one virtual hand is visually stroked and the other is visually thermostimulated. In the following, these findings will be recapitulated in detail and possible explanations for these findings will be discussed.
SLI Vividness Depends on Applied Induction Type
An important finding from Experiment 1 is that our different induction types induced different SLI levels. More specifically, we found a significant effect for stronger SLI ratings after visuomotor congruency than visuothermal congruency, as well as a trend effect for stronger SLI ratings after visuotactile congruency than visuothermal congruency. In the absence of any existing RHI or SLI studies directly comparing the visuothermal induction type to the two other induction types [but see Trojan et al. (2018), for a successful RHI induction by visuothermal congruency], we conclude that inducing a SLI by visuothermal congruency is less efficient than inducing it by visuotactile or visuomotor congruency.
For the comparison between visuotactile congruency and visuomotor congruency, we found, however, no SLI differences. This null finding is in line with those former RHI studies that also revealed no differences between both induction types (Riemer et al., 2013; Kalckert and Ehrsson, 2014), while it contradicts Dummer et al. (2009) findings of stronger SoO after visuotactile congruency than visuomotor congruency. As pointed out by Kalckert and Ehrsson (2014), the results by Dummer et al. (2009) are, however, derived from a between-group comparison and thus may potentially also be due to some pre-existing group difference. Moreover, it remains questionable in how far these former RHI results are directly comparable to the present SLI findings, given that the present visuomotor feedback implementation was based on motion-tracking technology and thus supposedly richer in sensory detail than Dummer et al. (2009) analogous visuomotor feedback implementation. Therefore, our own interpretation is that both induction types are similarly potent (suitable) in inducing a SLI, although subtle differences may potentially exist and warrant further investigation.
Full-Blown SLIs Occur Rather Seldom
As observable by the SLI responder rates (cf. Figure 10), the induction of a full-blown SLI experience was seldom. In fact, across all experiments and conditions, the average SLI responder rate was just ~25%. This low SLI responder rate, however, is not just a specialty of the present study, but in line with previous SLI results (Newport et al., 2010; Guterstam et al., 2011; Folegatti et al., 2012). Although, unfortunately, none of the previous SLI studies presented their SLI responder rates, the reported SLI values are on average rather low. Whereas, in Folegatti et al. (2012) study average SLI values (item 6) were at maximum 2.5 on a 15-point Likert scale and in Guterstam et al. (2011) study at maximum around 5 on a 10-point Likert scale (items S5 and S6), slightly higher average SLI values (items 7 and 8) were only reported in Newport et al. (2010) study with a maximum around 5 on a 7-point Likert scale. These SLI values were, nevertheless, still low compared to the typically reported SoO values for the classical RHI.
Hence, it seems that either the correct setup to robustly generate vivid SLIs has not yet been found, or the neurocognitive instantiation of two competing hand percepts is a seldom representational state that is avoided to be instantiated by the brain, wherever possible. Our suspicion is that the latter is the case. Support for this view comes from the widespread evidence within the RHI literature that SoO instantiation depends not only on intermodal synchrony but also on transsituationally stable, internal body maps. In fact, various spatial, anatomical, textural, and postural constraints have been identified that a perceptual object needs to fulfill in order to become experienceable as part of the own body (for a review, see Braun et al., 2018). For instance, a RHI is typically not-invocable, if a non-hand-shaped object is used instead of an artificial hand (Haans et al., 2008; Tsakiris et al., 2010) or if the artificial hand is not sufficiently anatomically aligned with the participant's real hand (Ehrsson et al., 2004; Kalckert and Ehrsson, 2012, 2014; Braun et al., 2014, 2016). Hence, it appears reasonable to assume that the presumed internal body maps also entail an anatomical constraint that multiple type-identical limbs are avoided to be co-instantiated, wherever possible. That such an anatomical constraint exists appears not merely reasonable, given that the body is usually configured this way, but there is also empirical evidence for a “neuromatrix” that appears to pre-define the neurocognitive representation of our body as a whole (Melzack, 1990).
Stronger SoO Becomes Attributed Toward the MVH
A recurring finding in our study is that stronger limb ownership was reported toward the MVH than LVH. That is, throughout experimental conditions, the participants experienced the medially presented virtual hand as more “mine” and more body-belonging than the laterally presented virtual hand. Remarkably, this effect not only occurred in Experiments 1 and 2, where both virtual hands were shown in exact equidistance to the participant's real hand, but as well in those Experiment 3 trials where the LVH exactly matched the participant's real hand position. That is, even under conditions where the LVH was presented at the exact participant's real hand position and the MVH medially aside, the participants indicated stronger SoO for the medially displaced virtual hand than for the correctly positioned (but non-stimulated) virtual hand.
In line with this phenomenological finding of higher SoO toward the MVH than LVH was also our EDA result. To recap, we recorded the participant's phasic EDA responses during the syringe applications and used these responses as an implicit measure for virtual hand embodiment (Ocklenburg et al., 2011; Alimardani et al., 2013, 2014; Braun et al., 2016). The stronger a virtual hand was felt like part of their own body, so our reasoning, the stronger would be the EDA fear response, if this virtual hand gets threatened. Our results reveal that while phasic EDA responses relative to the syringe application occurred for both virtual hands, the significantly stronger EDA responses were observed for the MVH conditions. Hence, besides the phenomenological evidence, there is also electrodermal evidence for stronger embodiment of the MVH than LVH.
While, to our knowledge, the present study is the first to demonstrate this “midsagittal line effect” in a systematic manner, it appears that the effect per se has already been registered before, but not further considered. It is noteworthy that both Guterstam et al. (2011) and Folegatti et al. (2012) used a SLI setup where the artificial hands were always placed medially and never laterally aside the participant's real hand. As clarified by Guterstam et al. (2011), it was no coincidence that the SLI setup was arranged in this way, but based on the piloting observation that the SLI robustness was stronger if the artificial hand was placed medially and not laterally aside.
Taking these findings together, it appears that this effect is quite robust. This raises the question why and how this effect comes about. The only difference that we can see between the positioning of the MVH and LVH is that the MVH position is more proximate to the participant's midsagittal line than the LVH position. Therefore, our own suspicion is that besides all the already identified RHI/SLI constraints, a further “spatial proximity” constraint exists: The closer an object is located to a person's body midsagittal line, the more likely the person is about to experience this object as part of his or her own body. From a predictive coding perspective [see, e.g., Limanowski and Blankenburg (2013), Apps and Tsakiris (2014), Limanowski (2014), Tsakiris (2016)], according to which “one's body is processed in a probabilistic manner as the most likely to be “me”” (Tsakiris, 2016, p. 8), such SoO attribution mechanism appears conceivable: The smaller the distance between a physical object and the body midsagittal line, the higher might be the probability that this physical object is part of the own physical body. An alternative explanation might be a simple attention effect, given that the MVH appeared in a more salient position (i.e., within the center of the participant's visual field) than the LVH. That is, the MVH perhaps just attracted more attention than the LVH. Future studies are necessary to disentangle the reasons for the observed midsagittal line effect.
SLI Experiences Can Also Be Induced by a Combined Application of Multiple Induction Types
Another important finding from Experiment 2 is that SLI experiences can also be induced by a combined application of multiple SLI induction types, such that one virtual hand is shown to be visually stimulated by one induction type and the other virtual hand by another induction type. In particular, this applies to the combined induction of visuotactile–visuothermal congruency, which obtained the highest SLI responder rate (~63%) throughout all three experiments. The other induction type combinations were, however, also possible and reveal SLI responder rates that are comparable to our ~25% average SLI responder rate. The finding that SLIs can also be induced by a combined application of multiple SLI induction types, is consistent with the clinical observation that the competitively perceived SPLs may differ in respect to their primarily experienced modality (cf. e.g., Khateb et al., 2009). That is, that, for instance, one right hand is felt being touched, while another competing right hand is seen and felt moving. A potential neurocognitive explanation for this might lay in the multiple body maps the brain possesses. As known from the literature, the brain does not (only) possess one polymodal map of the body as a whole, but it possesses multiple, modality-specific body maps, such as the famous distinction between a motor homunculus and sensory homunculus (Penfield and Boldrey, 1937). Our suspicion is that modality-specific SPLs become instantiated whenever the modality-specific sensory maps cannot be integrated anymore and therefore begin to independently instantiate modality-specific limbs. The reason for this decoupling might thereby be seen as etiologically different for the clinical and experimental SPL instantiation. In the clinical SPL case, this decoupling might be for instance resulting from an axonal dissection between the different sensory maps, while in the experimental case, the competing SPLs could result in the perceptual infusibility of two equally embodiable, modality-specific limb percepts.
Why the combined induction of visuotactile–visuothermal congruency induced by far the strongest SLI responder rates, as compared to the other induction type combinations, remains speculative. One potential explanation might be that both involved induction types do not provoke a constant updating of proprioceptive information—unlike the visuomotor congruency induction type—and thus less proprioceptive accuracy exists as to where the participant's real hand is actually currently located. Consequently, in the absence of a clear hand location expectancy, but concomitant presence of two spatially apart and equally embodiable hand percepts, the brain gives up its anatomical constraint of avoiding co-instantiating type-identical limbs and resolves its multisensory conflict by attributing SoO toward both virtual hands. An alternative explanation might be that the SLI responder rates were just strongest for the visuotactile–visuothermal induction type combination, because the other induction type combinations induced stronger multisensory conflicts, given that they always involved a visuomotor stimulation. The reasoning for this assumption would be that if the visuomotor induction type gets combined with the visuotactile or visuothermal induction type, a sensory conflict arises in two accounts: First, the participant sees one virtual hand being static, while he or she is actually momentarily moving his or her real hand. Second, the strokes or thermostimulations are applied on a moving real hand, but seen on a static virtual hand.
Study Limitations and Future Directions
One limitation of our study is that besides our implicit EDA measure for virtual hand embodiment, our study solely relied on self-rating data. This is problematic in so far as introspective data are often confounded by various response biases such as confirmation bias (Nickerson, 1998), acquiescence (Paulhus, 1991), or social desirability (Nederhof, 1985). In particular, this also applies to introspective data derived from RHI or other body-transfer illusion studies. Two recent works, for instance, suggest that the RHI appears to be (partly) driven by imaginative suggestibility (Lush et al., 2019, non-peer-reviewed pre-print) and demand characteristics (Lush, 2020) that, according to Lush et al. (2019), cannot be controlled for by the currently established RHI control questions. Hence, although in the present study we attempted to mitigate response biases by inserting the typically used control questions, pseudo-randomizing the order of questions, and defining illusion criteria and aggregating across multiple trials, we cannot rule out that there were also some response biases in the current study. For future studies, it would be helpful if some objective SLI measures could be developed besides introspective data.
A first step toward an objective SLI measure has been undertaken by Newport et al. (2010) by inserting a pointing task into their SLI setting [as an adaptation of Kammers et al. (2006) reaching task]. In an elegant manner, this task allows one to also assess functional levels of embodiment over the virtual hands (i.e., which of the two virtual hands is actually used, when it comes to reaching an object). In brief, after each SLI trial, both virtual hands abruptly disappeared and instead a white dot emerged, after which the participants should reach with their hand. The point hereby emerged in front of the just disappeared virtual hands and in sagittal alignment to the participants' real hand position, such that it was equidistant to either virtual hand. One interesting result was that if the MVH moved synchronously with the participants' real hands' movements, participants showed a lateralized reaching error (i.e., they misreached the target point to the body-distant side), whereas if the LVH moved synchronously with the participants' real hands' movements, participants showed a medialized reaching error (i.e., they misreached the target point to the midsagittal side). If both virtual hands, in turn, moved synchronously with the participants' real hands' movements, most participants again showed a lateralized reaching error. Hence, as the authors conclude, it seems that “while multiple limbs can be incorporated into the body image, the body schema can accommodate only one” (Newport et al., 2010, p. 385). In retrospect, it would have been interesting to include such a reaching task in our SLI study. One interesting aspect would have been, whether our modality-specific SoO inductions would have had an impact on the pointing task. That is, whether the virtual hand showing visuomotor congruency, for instance, would have had more strongly influenced the grasping movement than the virtual hand showing visuotactile or visuothermal congruency. Likewise, it would have been interesting to find out whether the midsagittal line preference, seen for the introspective data, would have also been reflected in the pointing task (in fact, Newport's data indicate such effect).
Another issue that was not addressed in this study was the qualitative character of the instantiated limb percepts. That is, whether for instance, one virtual hand was distinctively felt to be thermostimulated and the other virtual hand to be distinctively stroked, or whether there was a perceptual fusion for either competing limb percepts. While our anecdotical observation is that participants indeed experienced modality-specific limbs, we did not investigate this question in a systematic manner.
Furthermore, regarding the comparison of SLI responder rates across experiments, we cannot dismiss the possibility of some confounding time on task effects, given that the three experiments were conducted in the same order for every participant. The reason why we decided for a fixed experiment order was that participants should first get familiarized with each induction method in isolation (Experiment 1), before they would then get confronted with combinations of them (Experiment 2 and 3). In hindsight, however, this consideration was injudicious and a counterbalancing of blocks would have been the better option. On the one hand, a potential confounding factor could, for instance, be a familiarization effect, in that participants got used to the SLI over time. Consequently, they could then have indicated higher SoO/SLI values for the lastly presented conditions than for the earlier presented conditions. On the other hand, fatigue effects may have mitigated the strength of the illusion in the later stages of the study. Therefore, future investigations are necessary to also compare the respective induction type combinations in a more counterbalanced design.
Another limitation of the present study is the rather low sample size (N = 24), which was due to practical constraints (the study acquisition took place within the scope of a bachelor thesis). Instead of carrying out a power analysis, we based our sample size on the former reported SLI studies that used similar (Guterstam et al., 2011) or lower samples sizes (Ehrsson, 2009; Newport et al., 2010; Folegatti et al., 2012) within their individual experiments. However, a post hoc sensitivity analysis on our used three-level one-factorial RM ANOVAS revealed a chance of 80% (α = 0.05) to detect an effect around f = 0.26, or larger. Translated into Cohen's d, this relates to a medium effect size of d = 0.52, and more moderate effects with a lower effect size might not have been detectable by our study.
Moreover, it should be noted that the virtual movement patterns of the visuotactile and visuothermal stimulation were not perfectly temporally aligned with the real stimuli but subjectively syntonized by the experimenter. The reason for this is that during experiment creation, we failed to technically implement a positional real-time tracking of the brush and test tube that was precise enough to real-time mirror the experimenter's stroking/thermostimulating behavior. Given this technical constraint, we believe that subjectively synchronizing with a jitter-free pre-recorded animation was the preferable option. Hereby, both stimuli objects were presented at a constant and slow-paced rate, such that the visual estimate of the experimenter was probably plausibly matching enough.
Conclusion
In conclusion, our study demonstrates that although under specific experimental conditions, a majority of participants can be made to experience a SLI, under most conditions, SoO is only experienced toward one virtual hand: the virtual hand that is more medial to the participant's midsagittal body line. This finding supports the view that a “neuromatrix” exists that pre-defines our bodily self-representation and avoids the co-instantiation of multiple type-identical limb percepts, wherever possible.
Data Availability Statement
The raw data supporting the conclusions of this article will be made available by the authors, without undue reservation.
Ethics Statement
The studies involving human participants were reviewed and approved by Medical Ethics Committee of the University of Bonn. The patients/participants provided their written informed consent to participate in this study. Written informed consent was obtained from the individuals for the publication of any potentially identifiable images or data included in this article.
Author Contributions
AB designed the experiment under the supervision of NB and collected the data. AB and NB analyzed the data. NB, KK, and AB wrote major parts of the manuscript. AP, SL, and AB contributed to, reviewed, and edited the manuscript. All authors contributed to the article and approved the submitted version.
Conflict of Interest
The authors declare that the research was conducted in the absence of any commercial or financial relationships that could be construed as a potential conflict of interest.
Acknowledgments
This research article is a reworked excerpt from a non-published bachelor thesis by AB and was funded by budgets from the University of Bonn. No third party funding was involved. We acknowledge the support of Prof. Dr. Reinhard Klein.
References
Alimardani, M., Nishio, S., and Ishiguro, H. (2013). Humanlike robot hands controlled by brain activity arouse illusion of ownership in operators. Sci. Rep. 3, 1–5. doi: 10.1038/srep02396
Alimardani, M., Nishio, S., and Ishiguro, H. (2014). Effect of biased feedback on motor imagery learning in BCI-teleoperation system. Front. Syst. Neurosci. 8:52. doi: 10.3389/fnsys.2014.00052
Apps, M. A. J., and Tsakiris, M. (2014). The free-energy self: A predictive coding account of self-recognition. Neurosci. Biobehav. Rev. 41, 85–97. doi: 10.1016/j.neubiorev.2013.01.029
Bachmann, D., Weichert, F., and Rinkenauer, G. (2018). Review of three-dimensional human-computer interaction with focus on the leap motion controller. Sensors 18:2194. doi: 10.3390/s18072194
Benedek, M., and Kaernbach, C. (2010a). A continuous measure of phasic electrodermal activity. J. Neurosci. Methods 190, 80–91. doi: 10.1016/j.jneumeth.2010.04.028
Benedek, M., and Kaernbach, C. (2010b). Decomposition of skin conductance data by means of nonnegative deconvolution. Psychophysiology 47, 647–658. doi: 10.1111/j.1469-8986.2009.00972.x
Botvinick, M., and Cohen, J. (1998). Rubber hands “feel” touch that eyes see. Nature 391:756. doi: 10.1038/35784
Braun, N., Debener, S., Spychala, N., Bongartz, E., Sorös, P., Müller, H. H. O., et al. (2018). The senses of agency and ownership: a review. Front. Psychol. 9:535. doi: 10.3389/fpsyg.2018.00535
Braun, N., Emkes, R., Thorne, J. D., and Debener, S. (2016). Embodied neurofeedback with an anthropomorphic robotic hand. Sci. Rep. 6, 1–13. doi: 10.1038/srep37696
Braun, N., Thorne, J. D., Hildebrandt, H., and Debener, S. (2014). Interplay of agency and ownership: the intentional binding and rubber hand illusion paradigm combined. PLoS ONE 9:e111967. doi: 10.1371/journal.pone.0111967
Cipriani, G., Picchi, L., Vedovello, M., Nuti, A., and Di Fiorino, M. (2011). The phantom and the supernumerary phantom limb: historical review and new case. Neurosci. Bull. 27, 359–365. doi: 10.1007/s12264-011-1737-6
Curt, A., Yengue, C. N., Hilti, L. M., and Brugger, P. (2011). Supernumerary phantom limbs in spinal cord injury. Spinal Cord 49, 588–595. doi: 10.1038/sc.2010.143
Dummer, T., Picot-Annand, A., Neal, T., and Moore, C. (2009). Movement and the rubber hand illusion. Perception 38, 271–280. doi: 10.1068/p5921
Ehrsson, H. H. (2009). How many arms make a pair? Perceptual illusion of having an additional limb. Perception 38, 310–312. doi: 10.1068/p6304
Ehrsson, H. H., Spence, C., and Passingham, R. E. (2004). That's my hand! Activity in premotor cortex reflects feeling of ownership of a limb. Science 305, 875–877. doi: 10.1126/science.1097011
Fleiss, J. L. (1971). Measuring nominal scale agreement among many raters. Psychol. Bull. 76, 378–382. doi: 10.1037/h0031619
Folegatti, A., Farnè, A., Salemme, R., and de Vignemont, F. (2012). The rubber hand illusion: two's a company, but three's a crowd. Conscious. Cogn. 21, 799–812. doi: 10.1016/j.concog.2012.02.008
Guterstam, A., Petkova, V. I., and Ehrsson, H. H. (2011). The illusion of owning a third arm. PLoS ONE 6:e17208. doi: 10.1371/journal.pone.0017208
Haans, A., IJsselsteijn, W. A., and de Kort, Y. A. W. (2008). The effect of similarities in skin texture and hand shape on perceived ownership of a fake limb. Body Image 5, 389–394. doi: 10.1016/j.bodyim.2008.04.003
Halligan, P. W., Marshall, J. C., and Wade, D. T. (1993). Three arms: a case study of supernumerary phantom limb after right hemisphere stroke. J. Neurol. Neurosurg. Psychiatry 56, 159–166. doi: 10.1136/jnnp.56.2.159
Kalckert, A., and Ehrsson, H. H. (2012). Moving a rubber hand that feels like your own: a dissociation of ownership and agency. Front. Hum. Neurosci. 6:40. doi: 10.3389/fnhum.2012.00040
Kalckert, A., and Ehrsson, H. H. (2014). The moving rubber hand illusion revisited: comparing movements and visuotactile stimulation to induce illusory ownership. Conscious. Cogn. 26, 117–132. doi: 10.1016/j.concog.2014.02.003
Kammers, M. P. M., van der Ham, I. J. M., and Dijkerman, H. C. (2006). Dissociating body representations in healthy individuals: differential effects of a kinaesthetic illusion on perception and action. Neuropsychologia 44, 2430–2436. doi: 10.1016/j.neuropsychologia.2006.04.009
Khateb, A., Simon, S. R., Dieguez, S., Lazeyras, F., Momjian-Mayor, I., Blanke, O., et al. (2009). Seeing the phantom: a functional magnetic resonance imaging study of a supernumerary phantom limb. Ann. Neurol. 65, 698–705. doi: 10.1002/ana.21647
Kilteni, K., Maselli, A., Kording, K. P., and Slater, M. (2015). Over my fake body: body ownership illusions for studying the multisensory basis of own-body perception. Front. Hum. Neurosci. 9:141. doi: 10.3389/fnhum.2015.00141
Limanowski, J. (2014). What can body ownership illusions tell us about minimal phenomenal selfhood? Front. Hum. Neurosci. 8:946. doi: 10.3389/fnhum.2014.00946
Limanowski, J., and Blankenburg, F. (2013). Minimal self-models and the free energy principle. Front. Hum. Neurosci. 7:547. doi: 10.3389/fnhum.2013.00547
Lush, P. (2020). Demand characteristics confound the rubber hand illusion. Collabra Psychol. 6:22. doi: 10.1525/collabra.325
Lush, P., Botan, V., Scott, R. B., Seth, A., Ward, J., and Dienes, Z. (2019). Phenomenological control: response to imaginative suggestion predicts measures of mirror touch synaesthesia, vicarious pain and the rubber hand illusion. PsyArxiv preprint. doi: 10.31234/osf.io/82jav
Melzack, R. (1990). Phantom limbs and the concept of a neuromatrix. Trends Neurosci.13, 88–92. doi: 10.1016/0166-2236(90)90179-E
Millonig, A., Bodner, T., Donnemiller, E., Wolf, E., and Unterberger, I. (2011). Supernumerary phantom limb as a rare symptom of epileptic seizures—case report and literature review. Epilepsia 52, e97–e100. doi: 10.1111/j.1528-1167.2011.03156.x
Nederhof, A. J. (1985). Methods of coping with social desirability bias: a review. Eur. J. Soc. Psychol. 15, 263–280. doi: 10.1002/ejsp.2420150303
Newport, R., Pearce, R., and Preston, C. (2010). Fake hands in action: embodiment and control of supernumerary limbs. Exp. Brain Res. 204, 385–395. doi: 10.1007/s00221-009-2104-y
Nickerson, R. S. (1998). Confirmation bias: a ubiquitous phenomenon in many guises. Rev. General Psychol. 2, 175–220. doi: 10.1037/1089-2680.2.2.175
Ocklenburg, S., Rüther, N., Peterburs, J., Pinnow, M., and Güntürkün, O. (2011). Laterality in the rubber hand illusion. Laterality 16, 174–187. doi: 10.1080/13576500903483515
Paulhus, D. L. (1991). “Measurement and Control of Response Bias,” in Measures of Personality and Social Psychological Attitudes (New York, NY: Academic Press), 17–59. doi: 10.1016/b978-0-12-590241-0.50006-x
Penfield, W., and Boldrey, E. (1937). Somatic motor and sensory representation in the cerebral cortex of man as studied by electrical stimulation. Brain 60, 389–443. doi: 10.1093/brain/60.4.389
Riemer, M., Kleinbohl, D., Holzl, R., and Trojan, J. (2013). Action and perception in the rubber hand illusion. Exp. Brain Res. 229, 383–393. doi: 10.1007/s00221-012-3374-3
Rogers, M. J. C., and Franzen, M. D. (1992). Delusional reduplication following closed-head injury. Brain Inj. 6, 469–476. doi: 10.3109/02699059209008142
Slater, M., Perez-Marcos, D., Ehrsson, H. H., and Sanchez-Vives, M. V. (2008). Towards a digital body: the virtual arm illusion. Front. Hum. Neurosci. 2:6. doi: 10.3389/neuro.09.006.2008
Srivastava, A., Taly, A. B., Gupta, A., Murali, T., Noone, M. L., Thirthahalli, J., et al. (2008). Stroke with supernumerary phantom limb: case study, review of literature and pathogenesis. Acta Neuropsychiatr. 20, 256–264. doi: 10.1111/j.1601-5215.2008.00294.x
Staub, F., Bogousslavsky, J., Maeder, P., Maeder-Ingvar, M., Fornari, E., Ghika, J., et al. (2006). Intentional motor phantom limb syndrome. Neurology 67, 2140–2146. doi: 10.1212/01.wnl.0000249135.78905.75
Synofzik, M., Vosgerau, G., and Newen, A. (2008). I move, therefore i am: a new theoretical framework to investigate agency and ownership. Conscious. Cogn. 17, 411–424. doi: 10.1016/j.concog.2008.03.008
Trojan, J., Fuchs, X., Speth, S. L., and Diers, M. (2018). The rubber hand illusion induced by visual-thermal stimulation. Sci. Rep. 8, 1–9. doi: 10.1038/s41598-018-29860-2
Tsakiris, M. (2016). The multisensory basis of the self: from body to identity to others. Q. J. Exp. Psychol. 70, 597–609. doi: 10.1080/17470218.2016.1181768
Tsakiris, M., Carpenter, L., James, D., and Fotopoulou, A. (2010). Hands only illusion: multisensory integration elicits sense of ownership for body parts but not for non-corporeal objects. Exp. Brain Res. 204, 343–352. doi: 10.1007/s00221-009-2039-3
Keywords: supernumerary phantom limb, supernumerary limb illusion, virtual hand illusion, rubber hand illusion, EDA
Citation: Braun N, Berisha A, Anders D, Kannen K, Lux S and Philipsen A (2020) Experimental Inducibility of Supernumerary Phantom Limbs: A Series of Virtual Reality Experiments. Front. Virtual Real. 1:12. doi: 10.3389/frvir.2020.00012
Received: 08 April 2020; Accepted: 31 July 2020;
Published: 16 October 2020.
Edited by:
Michael Madary, University of the Pacific, United StatesReviewed by:
Harry Farmer, University of Greenwich, United KingdomAntonella Maselli, Italian National Research Council, Italy
Copyright © 2020 Braun, Berisha, Anders, Kannen, Lux and Philipsen. This is an open-access article distributed under the terms of the Creative Commons Attribution License (CC BY). The use, distribution or reproduction in other forums is permitted, provided the original author(s) and the copyright owner(s) are credited and that the original publication in this journal is cited, in accordance with accepted academic practice. No use, distribution or reproduction is permitted which does not comply with these terms.
*Correspondence: Niclas Braun, bmljbGFzLmJyYXVuJiN4MDAwNDA7dWtib25uLmRl
†These authors share last authorship