- 1Department of Pediatrics, University of Nigeria Teaching Hospital, Enugu, Nigeria
- 2College of Medicine, University of Nigeria, Enugu, Nigeria
- 3Department of Epidemiology and Public Health, Swiss Tropical and Public Health Institute, Allschwil, Switzerland
- 4Department of Public Health, University of Basel, Basel, Switzerland
This narrative review aims to appraise the current evidence on using biomarkers of obstructive nephropathy to predict the onset and progression of chronic kidney disease (CKD) in infants with posterior urethral valves (PUV). PUV is the most frequently reported congenital anomaly of the kidney and urinary tract (CAKUT) associated with bladder outlet obstruction in male children. It contributes significantly to the CKD burden in childhood. Despite different approaches for its postnatal repair, evidence-based data still suggest a high risk of CKD and end-stage kidney disease (ESKD) later in childhood. In obstructive nephropathy, glomerular and tubulointerstitial lesions contribute to renal impairment. Although it may be difficult to predict these adverse renal outcomes in repaired PUV, detecting and monitoring future CKD appears enhanced using the combination of serum creatinine- or cystatin C-based estimated glomerular filtration rate (eGFR) and albuminuria. Given the drawbacks of these conventional biomarkers, there is a paradigm shift to novel biomarkers as tools for the early identification of glomerular and tubulointerstitial lesions seen in obstructive nephropathy. Most novel biomarkers are yet to be fully applied to routine clinical practice globally. Nevertheless, there is substantial evidence showing that they form part of the emerging diagnostics for obstructive nephropathy. From the reviewed studies, urine transforming growth factor-beta 1 (TGF-β1) is the most prominent biomarker among the novel biomarkers of obstructive nephropathy. However, other novel approaches like the machine learning (ML) model (a form of health-related artificial intelligence) and urodynamic parameters like bladder contractility index hold promise for PUV outcomes prediction (PUVOP). Because of the association of urine TGF-β1 with urine angiotensin level (a biomarker of the renin-angiotensin-aldosterone system [RAAS]), early angiotensin-converting enzyme inhibitor (ACEI) therapy in patients with PUV may potentially retard the progression of CKD and improve renal outcomes. Thus, future research directions will be to explore the role of ACEI as a pre-emptive treatment for poor renal outcomes in post valve-ablation patients and to conduct longitudinal studies that would properly demonstrate these biomarkers as predictors of these outcomes.
Introduction
Posterior urethral valves (PUV) are the most frequently reported congenital anomaly associated with bladder outlet obstruction in male children (1, 2). The anomaly contributes significantly to childhood chronic kidney disease (CKD) burden (3). Reported clinical factors that predict poor renal outcomes in patients with PUV consist of late age of presentation (after two years of age), delayed fulguration (intervention after one month) with high initial serum creatinine, failure of serum creatinine to return to physiologic level after one-month post-fulguration (4), and comorbidities like vesicoureteric reflux (VUR), urosepsis and renal dysplasia (5). Other authors also reported late primary valve ablation (intervention after the first year of life) as a risk factor for CKD (6).
Previous systematic reviews indicate high CKD or end-stage kidney disease (ESKD) risk even in postnatally-repaired cases of PUV (7, 8). Nevertheless, early diagnosis and surgical intervention remain the standard approach to its management. In some developed settings, prenatal ultrasound diagnosis and surgical intervention currently appear to be the norm (2, 9–11). In developing countries, the principal treatment challenges consist of late identification by caregivers and health professionals and delayed access to healthcare facilities (12, 13). Early diagnosis of PUV is crucial in mitigating the adverse renal outcomes associated with late identification. More importantly, diagnostic methods should be non-invasive, simple, and reliable enough to drive the early identification of renal dysfunction. PUV-associated obstructive nephropathy contributes to progressive deterioration of renal function, which may end up with ESKD.
Because of the tedious steps involved in obtaining measured glomerular filtration rate (mGFR), estimated glomerular filtration rate (eGFR) based on serum creatinine or cystatin C is widely utilized in assessing renal function. Several studies have shown the diagnostic accuracy of serum creatinine in predicting future renal outcomes in PUV patients after ablation. For instance, a recent study suggests that the severity of CKD can be predicted with precision using the nadir creatinine within six weeks of ablation (14). Another study reported that patients with post-ablation nadir creatinine above 0.85 mg/dl were at high risk for subsequent CKD (15). Elevated nadir creatinine in the first year of life is generally regarded as the best predictor of adverse renal outcomes in these patients (7). Furthermore, creatinine-based eGFR has been used with nadir creatinine to assess renal outcomes in postnatally-repaired PUV after two years of age; the authors noted an ESKD prevalence rate of 15% in these patients (16). Other investigators used eGFR after ≥ 5 years and > 6 years of follow-up and documented ESKD prevalence rates of 44% and 11.1%, respectively (17, 18). On the other hand, serum cystatin C is a reliable marker of GFR in patients with mildly-to-moderately deranged renal function; it has higher diagnostic accuracy than serum creatinine (19). Using both serum creatinine and cystatin C, however, has some disadvantages.
The accuracy of creatinine-based eGFR is limited by demographic factors that influence the endogenous production of creatinine, such as age, sex, race and body weight, and dietary intake (20). Secondly, serum creatinine is only elevated when renal function declines by about 50% (21). Besides, there is a 48-hour time lag between a sudden reduction in renal function and the increase in serum creatinine. Finally, an elevated serum creatinine level gives minimal information about the etiology and nature of the renal injury. Conversely, eGFR based on serum cystatin C appears more dependable because the biomarker is unaffected by age, sex, muscle mass, and body weight (22–24) and better reflects GFR than serum creatinine (25). Also, elevated serum cystatin C levels can indicate renal dysfunction early enough because of the biomarker’s metabolism, filtration, and reabsorption kinetics. However, body weight and lean mass are not correlated with serum cystatin C levels (26). Obesity affects serum levels; thus, formulas based on this biomarker overestimate GFR in higher body mass index (27). The cost of its clinical application is also a limiting factor to its conventional use.
In obstructive nephropathy, glomerular and tubulointerstitial lesions contribute to renal impairment. Although it may be difficult to predict these renal outcomes in repaired PUV, detecting and monitoring future CKD appears enhanced using the combination of eGFR based on serum creatinine or cystatin C and urine albumin (28). Albuminuria is the traditional biomarker of renal injury and precedes any reduction in GFR. But albuminuria can only occur following significant renal injury and not in tubulointerstitial disease. Given that cystatin C is physiologically undetectable in urine because of its complete tubular reabsorption, raised urine cystatin C level occurs in the tubulointerstitial lesion (29). Thus, urine cystatin C and other novel biomarkers have become a focus of scientific searchlight aimed at discovering alternative reliable tools for either predicting or diagnosing obstructive nephropathy related to PUV. Novel biomarkers are now well documented as useful tools in the early identification of glomerular and tubulointerstitial lesions seen in CKD (30). Although these biomarkers are more sensitive and specific than the traditional biomarkers (serum creatinine, serum cystatin C, and albuminuria), their diagnostic value for CKD appears augmented when used in combination or in panel form. In this narrative review, we aim to appraise the current evidence on using biomarkers of obstructive nephropathy to predict the onset and progression of CKD in patients with PUV.
Pathophysiology of obstructive nephropathy
As bladder outlet obstruction from PUV persists, this structural hindrance to normal urine flow (obstructive uropathy) will lead to sequential involvement of the upper urinary tract and renal parenchyma, leading to renal dysfunction (obstructive nephropathy). The intra-vesical pressure that builds up and the effect of the thickened bladder wall on the intramural portion of the ureter disrupts the functional integrity of the vesicoureteric valves, causing retrograde urine flow into the ureters. Besides ureteric hypertrophy, intra-ureteric pressures are elevated, resulting in hydro-ureters and hydronephrosis (Figure 1).
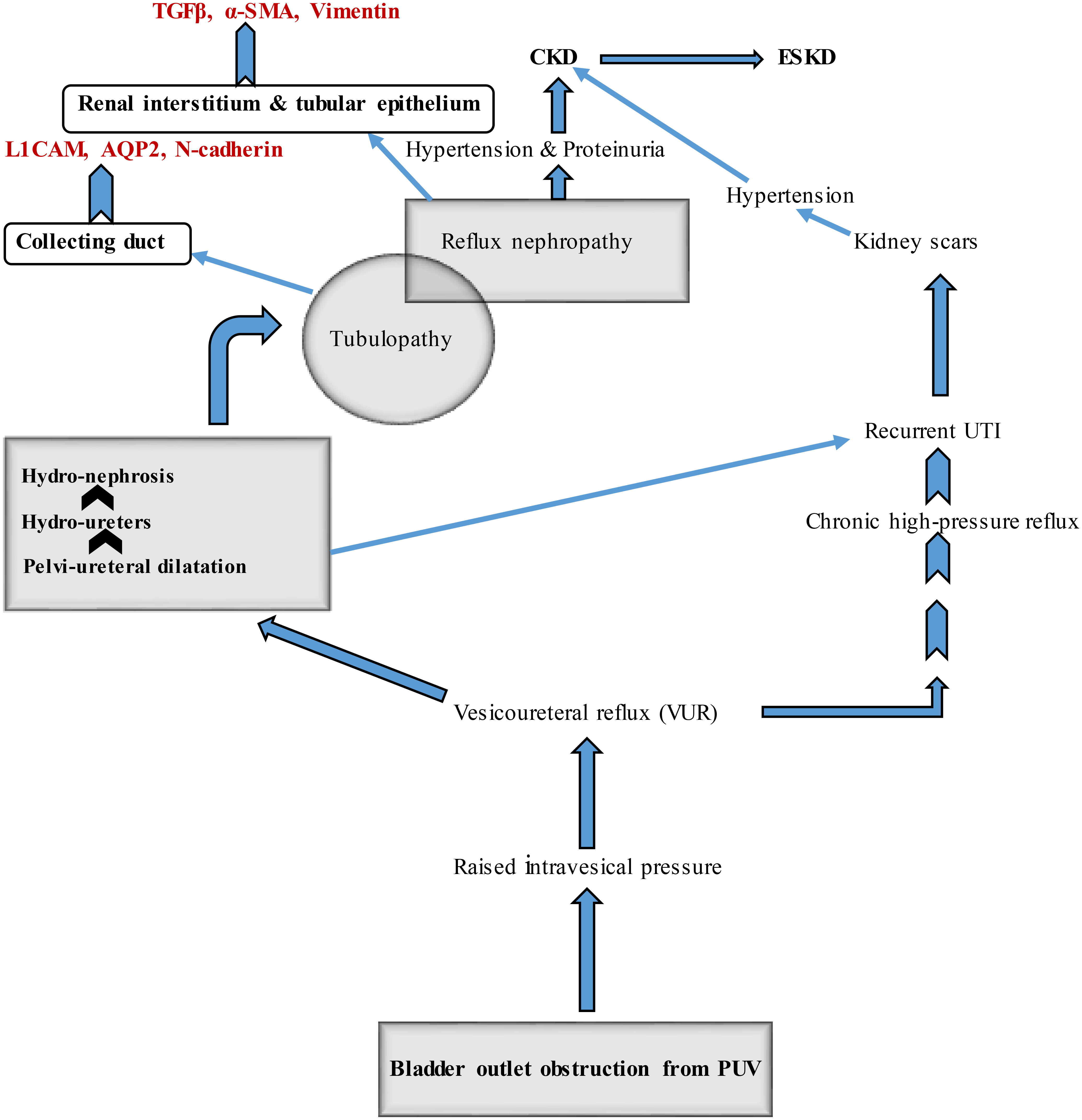
Figure 1 Pathophysiologic mechanisms involved in PUV-related obstructive uropathy and nephropathy with sites of expression of some candidate biomarkers. CKD, chronic kidney disease ESKD, end-stage kidney disease PUV, posterior urethral valve UTI, urinary tract infection TGFβ, transforming growth factor-beta α-SMA, α-smooth muscle actin L1CAM, L1 cell adhesion molecule AQP2, aquaporin-2.
Subsequently, dilatation of the collecting ducts and distal tubules is accompanied by urinary stasis, predisposing to recurrent urosepsis. Urinary stasis, recurrent urosepsis, and high intraluminal pressure synergistically damage the renal parenchyma leading to kidney scars. Hypertension and proteinuria resulting from obstructive nephropathy contribute to the onset of CKD. Renal scarring is also a cause of hypertension. Glomerular hyperfiltration is mechanistically associated with proteinuria. On the other hand, obstructive uropathy results in significant tubular injury (tubulopathy). Poor urinary concentration (hyposthenuria) and inadequate urinary acidification are the significant sequelae that may manifest as polyuria, nocturia, and metabolic acidosis.
The sequence of events related to uncorrected PUV shows that tubulopathy precedes glomerulopathy (the hallmark of CKD). Thus, novel biomarkers associated with tubular and glomerular injuries may play a role in predicting obstructive nephropathy in patients with congenital lower urinary tract obstruction (LUTO). More importantly, novel urine biomarkers can serve as tools for diagnosis and prognostication. Their utility in various forms of CKD has been extensively documented (31–34). For instance, some investigators had previously reported differential expression of candidate proteins in the tissues of obstructed and non-obstructed fetal kidneys using fetal non-human primate model of unilateral ureteric obstruction that focused on the role of medullary collecting duct changes (35); the epithelial-mesenchymal transition (EMT) of obstructed fetal collecting duct epithelium, associated with expression of these biomarkers, was subsequently demonstrated in human subjects (36). They noted increased expression of N-cadherin, L1 cell adhesion molecule (L1CAM), and aquaporin-2 (AQP2) from the collecting duct, as well as mesenchymal proteins like vimentin and α-smooth muscle actin (α-SMA) from the renal interstitium, and fibrosis-linked cytokines like TGFβ from the tubular epithelium and adjacent renal interstitium (Figure 1). Increased expression of vimentin and α-SMA mirrors EMT and recruitment of myofibroblasts, respectively. These processes mainly occur in renal fibrosis that follows obstructive nephropathy. Notably, elevated urine TGF-β1 has been demonstrated by other investigators as a biomarker of obstructive uropathy in children (37).
Novel biomarkers of obstructive nephropathy
As previously mentioned, comorbid prognostic factors may determine the future renal function in patients with PUV. Firstly, some studies indicate that subsequent renal outcomes are related to bladder dysfunction (17, 38–40). Patients with PUV develop fibrosis of the detrusor muscle leading to reduced bladder compliance and instability; poor bladder emptying, urinary stasis, and recurrent urinary tract infection (UTI) are the consequences (41). Because bladder dysfunction is associated with long-term morbidity despite valve ablation or fulguration, some authors recommend early commencement of anticholinergic therapy and time-determined voiding to manage the bladder dysfunction in these patients (42). Also, other authors had previously reported a bladder-dysfunction prevalence rate of as high as 75% despite a successful primary valve ablation (43). It is not surprising that a recent study has recommended that the bladder contractility index from a well-conducted urodynamic study could serve as a reliable tool for prognostication in PUV (44). Secondly, VUR is recognized as a significant prognostic factor in children with PUV as its persistence is associated with a poor renal outcome (45). However, there is no unanimity yet on its prognostic role based on the discordant findings from most studies (17, 39, 46, 47). Finally, postnatal comorbidity such as renal dysplasia/hypoplasia has been reported to predict future renal outcomes in PUV (5, 48, 49). Activating the renin-angiotensin-aldosterone-system (RAAS) is most likely to play a fundamental role as the pathogenic pathway. This hypothesis is supported by the fact that initial and subsequent plasma renin activity (PRA) levels constitute a significant predictor of final renal outcome in PUV (46).
Despite the direct correlation between renal-structural injury and renal dysfunction in patients with obstructive nephropathy, there are few investigations that can predict those who will progress to CKD and ESKD. Besides imaging studies for prenatal and postnatal diagnosis of PUV, evaluating the extent of renal injury in these patients appears more cumbersome. Thus, several researchers have assessed the utilization of urine-based surrogate markers of renal damage as indicators of obstructive nephropathy (37, 50–53).
In a cohort study involving twenty-seven prevalent case patients with PUV (aged 1-18 years) and twenty age-matched controls, the investigators estimated the urinary excretion of candidate biomarkers (using whole-urine and urine exosome samples). They tried to correlate their urinary levels with GFR (37). They also evaluated the performance of these biomarkers as tests for reduced GFR using receiver-operating characteristic (ROC) curve and regression analyses. Interestingly, the median urine protein-to-creatinine ratio was higher in PUV patients than in controls and indirectly correlated with renal function. Furthermore, a significant reduction in AQP2 excretion and an increase in TGFβ and L1CAM excretion in whole urine occurred. Whole-urine TGFβ, L1CAM, and urine protein-to-creatinine ratio exhibited the best performance as tests for reduced eGFR, with areas under ROC curves of 0.788, 0.795, and 0.814, respectively. Specifically, higher TGF-β1 levels were associated with reduced GFR in the PUV patients based on linear regression analysis. This observation is in tandem with the findings of other authors who evaluated the usefulness of TGFβ as a surrogate marker for differentiating obstructive uropathy from non-obstructive uropathy in children (50, 52), although another study showed no correlation between urinary TGF-β1 excretion and eGFR (51). Additionally, it was noted that urine TGF-β1 levels directly correlated with proteinuria and urine α1-microglobulin in children with obstructive uropathy (50). TGF-β1 belongs to the TGFβ superfamily of cytokines and modulates cell growth, proliferation, differentiation, and apoptosis. The biomarker is the major fibrogenic growth factor or profibrotic inflammatory mediator encoded by the TGFB1 gene in man (54). On the other hand, urine α1-microglobulin is a radical scavenger as it protects cells and tissues against free hemoglobin-or oxidative stress-induced injury (55). This biomarker has also been used to indicate proteinuria (56). Therefore, the positive correlation of urine TGF-β1 with urine α1-microglobulin (as a surrogate marker of proteinuria) further underscores the ability of the former to indicate glomerular lesions arising from PUV-related obstructive nephropathy.
To further demonstrate increased renal expression of TGF-β1 in obstructive uropathy, another group of investigators examined fourteen children with PUV and sixteen healthy children without PUV who served as controls (57). They measured the total urine TGF-β1 level, expressed as a ratio to urine creatinine level. Although their results showed significantly greater excretion of the candidate biomarker in PUV patients’ urine than in their healthy controls, there was no correlation between urine TGF-β1 excretion and eGFR. However, the observation that TGF-β1 did not correlate with eGFR is at variance with the previous report that showed a direct relationship between urine TGFβ and reduced GFR (37). Despite this disparity in findings, the authors concluded that TGF-β1 might contribute to progressive CKD in patients with PUV: given its pathogenetic role in renal fibrosis (57).
Again, other researchers conducted a study of thirty patients with PUV in whom they estimated the pre-operative (pre-ablation) and post-operative (post-ablation) urinary cytokines such as TGF-β1 and tumor necrosis factor-α (TNF-α), interleukin-6 (IL-6) levels and microalbuminuria (52). They also sought to investigate the therapeutic role of angiotensin-converting enzyme inhibitors (ACEI) on adverse renal outcomes. Whereas urinary TGF-β1, TNF-α, and microalbumin levels were elevated in these patients, their post-operative levels declined significantly and continued for one year. Notably, the introduction of ACEI resulted in a substantial decline in urine TGF-β1 and microalbuminuria and improvement in GFR. Their findings also indicate the potential benefits of ACEI in retarding renal insufficiency in patients with PUV.
In a more recent prospective cohort study, the authors compared the changes in pre-ablation and post-ablation levels of urine TGF-β1 and monocyte chemoattractant protein-1 (MCP-1) in twenty children with PUV with the same parameters in their age-matched controls without the renal disease (53). Regarding the patients with PUV, the levels of these biomarkers declined after valve ablation. The differences in the pre-ablation and post-ablation median urine MCP-1 levels were significant on the follow-up timelines of the first, third, and sixth months after surgery. On the other hand, the authors found that the post-ablation median urine TGF-β1 level significantly reduced only six months after surgery. MCP-1 has multiple extra-renal production sites (monocytes, macrophages, and dendritic cells) and is grouped as a biomarker of inflammation and a glomerular biomarker. It is not surprising that any relief in the obstructive lesion of the urinary tract will easily affect its urinary level.
The sources and potential uses of the biomarkers have been highlighted in Table 1. The novel biomarkers proven as predictors of obstructive nephropathy comprise TGF-β1, L1CAM, AQP2, N-cadherin, TNF-α, IL-6, MCP-1, vimentin, and α-SMA. Among these listed biomarkers, TGF-β1 stands out as the most consistent predictor of renal injury and renal insufficiency as it is the commonest isoform of the TGFβ family in the immune system. It is a major pleiotropic cytokine with strong immuno-regulatory characteristics (33). This pro-inflammatory and fibrogenic cytokine has also been used in predicting disease progression in HIV infection and diabetic nephropathy (58).
Improving prediction of obstructive nephropathy: A look at some tubular biomarkers
Novel biomarkers are important in tracing the trajectory of CKD in patients with obstructive uropathy. Fortunately, the measurement of urine biomarkers is non-invasive and straightforward. Given that tubulopathy and glomerulopathy are the significant structural lesions in obstructive nephropathy, urine tubular biomarkers should form part of the biomarker panel for predicting this adverse renal outcome.
Firstly, urine cystatin C is a typical example of such tubular biomarkers. Cystatin C is produced by all nucleated cells in the body. It is freely filtered by the glomerulus and reabsorbed by the tubules, where it is almost wholly catabolized. The un-catabolized fraction is subsequently excreted in the urine. Thus, cystatin C breakdown is decreased in renal tubular diseases with an accompanying increase in urinary excretion. In an assay of freshly obtained urine samples of fifty-two patients with renal tubular disease, forty-seven patients with glomerular disease, and sixty normal controls, the investigators found that the mean urine cystatin C levels of the patients with tubulopathy were significantly higher than the levels in the controls and the patients with glomerulopathy (29). Additionally, serum and urine cystatin C have been reported as early predictors of diabetic nephropathy in type 2 diabetes mellitus (59), whereas urine cystatin C predicted the progression of diabetic kidney disease (60). Because of its precision in predicting tubular dysfunction in nephropathies, the urine cystatin C assay is an adjunct to the standard biomarker panel for screening renal diseases.
Secondly, urine α1-microglobulin (previously mentioned as a surrogate marker of proteinuria) shares similar kinetic properties with cystatin C. Both pass through the physiologic processes of glomerular filtration and tubular reabsorption. More importantly, urine α1-microglobulin level was increased in type 2 diabetes-mellitus patients with normoalbuminuria before the onset of microalbuminuria, making the tubular biomarker an earlier predictor of CKD in diabetic nephropathy (61, 62). This diagnostic property may equally apply to obstructive nephropathy.
Thirdly, urine neutrophil gelatinase-associated lipocalin (NGAL) is an established tubular biomarker expressed by tubular epithelial cells following tubulointerstitial injury (63). The biomarker levels showed a significant negative correlation with serum creatinine levels and eGFR (64, 65) and predicted the worsening of CKD (66–69). Besides, several studies have reported that urine NGAL levels were elevated in different forms of CKD (65–70).
Finally, urine levels of N-acetyl-β-D glucosaminidase (NAG) have been noted as predictors of early and late stages of CKD related to diabetes mellitus (71–73). The urine level of this tubular biomarker has also been adjudged reliable in detecting tubular injury related to proteinuria seen in the early stage of specific subtypes of idiopathic glomerulonephritis (71). These findings suggest that urine NAG is a predictor of the onset and progression of CKD. Interestingly, the baseline urine levels of this biomarker either predicted microalbuminuria and macroalbuminuria (72) or preceded microalbuminuria in diabetes mellitus patients (73). Thus, NAG also appears to be an early predictor of glomerulopathy. These published data about tubular biomarkers emphasize their ability to predict tubulopathy and glomerulopathy in other types of CKD. When these biomarkers are used in synergy with the established biomarkers of obstructive nephropathy, the detection of renal injury and dysfunction associated with PUV may be improved.
Enhanced prediction of obstructive nephropathy: Novel approaches and proposed panels of biomarkers
Obstructive nephropathy is an evolving disease where renal injury continues even after valve ablation or fulguration interventions. It is trite to mention that chronic obstruction due to PUV triggers tubulointerstitial injury arising from the activation of distinct pathophysiologic pathways. As the tubulointerstitial injury progresses, chronic renal injury comprising tubular atrophy, inflammatory cell infiltration, and interstitial fibrosis occurs. The timing of surgical intervention appears crucial in predicting long-term adverse renal outcomes. Thus, three novel biomarkers - urine epidermal growth factor (EGF)/MCP-1 ratio, urine NGAL, and urine kidney injury molecule-1 (KIM-1) - have been proposed as useful early biomarkers of progressive renal injury and could have a potential role in predicting long-term renal outcome in PUV (74). Reports show that ureteral obstruction leads to a significantly increased MCP-1 expression and a decreased EGF expression by renal tubular cells (75, 76). Whereas renal expression and urine excretion of MCP-1 are linked to tubular injury and the degree of monocyte infiltration, expression of EGF modulates tubular cell growth and tissue response to renal injury with a tubulointerstitial lesion (77, 78). Thus, a reduced urine EGF/MCP-1 ratio has been suggested as a marker of acute and chronic renal injury in obstructive nephropathy (79, 80). KIM-1 is expressed and released by injured epithelial cells of the proximal tubule, making urine KIM-1 a reliable biomarker of tubular injury (81). A study that assessed its role in obstructive nephropathy found a significantly higher urine KIM-1 in children with severe hydronephrosis secondary to ureteropelvic junction obstruction than in the control group (82).
Furthermore, new frontiers in the early prediction of renal outcomes in PUV have been explored using artificial intelligence (AI) in healthcare. Health-related AI applications potentially focus on diagnostics, personalized medicine, and patient monitoring using machine learning (ML) models. In a recent study based on this novel approach, some authors used ML to predict clinically relevant outcomes in PUV patients (83). They developed a ML model for predicting clinically relevant outcomes such as progression in CKD stage, initiation of kidney replacement therapy (KRT), and need for clean-intermittent catheterization in one hundred and three patients with PUV. Additionally, the authors evaluated model performance by concordance index (c-index) and externally validated it. Interestingly, they found that the ML model predicted CKD progression (c-index = 0.77; external C-index = 0.78), KRT (c-index = 0.95; external C-index = 0.89) and indicated clean-intermittent catheterization (c-index = 0.70; external C-index = 0.64), with all performing better than Cox proportional-hazards regression (83).
In a recent study, some urodynamic parameters (such as bladder-contractility index, bladder-outlet obstruction index, end-filling pressure, bladder compliance, and bladder-volume efficiency) were correlated with progression to late stages of CKD in PUV patients after valve fulguration (44). The authors applied Cox regression analysis to risk factors predicting the development of late stages of CKD. They found that bladder contractility index, end filling pressure, and bladder compliance were significantly associated with the study endpoint. Specifically, the ROC cut-off level for bladder contractility index predicting the primary endpoint showed a modest diagnostic performance, with a sensitivity of 78.2% and specificity of 62.5% (44). Thus, the bladder contractility index was adjudged a valuable tool for early detection of PUV patients at risk of progression to stages of CKD.
Nevertheless, the sensitivity and specificity of novel biomarkers for obstructive nephropathy may be enhanced when used as a panel. Combining biomarkers’ complimentary performance rather than a single biomarker’s performance in CKD diagnostics is essential for diagnostic precision. A recent longitudinal prospective study analyzed two serum renal biomarkers (serum creatinine and serum cystatin C) and six urinary renal biomarkers (NGAL, KIM-1, TGF-β1, retinol-binding protein [RBP], urine cystatin C and microalbuminuria) to determine detection of early renal injury in a cohort thirty seven infants with congenital urinary tract obstruction (84). The patients were categorized into three subgroups of those with unilateral hydro (uretero) nephrosis, bilateral hydro (uretero) nephrosis and LUTO who were compared with twenty four healthy infants. The authors found that best biomarker combination results for the three subgroups were obtained by matching RBP with TGF-ß1 or KIM-1 and NGAL with cystatin C, with a sensitivity and specificity of about 92.4% and 92.8%, respectively. A panel of these biomarkers (RBP, NGAL, KIM-1, TGF-β1, and cystatin C) were therefore recommended as highly efficient in predicting renal injury in surgically-amenable congenital obstructive uropathy (84). Similarly, serum NGAL and KIM-1 have been adjudged useful in potentially guiding the decision for surgical intervention in infants with antenatal hydronephrosis due to congenital obstructive uropathy (85).
Based on the present review, we therefore propose a panel of biomarkers which can potentially enhance the early diagnosis of obstructive nephropathy (Figure 2). Given that TGF-β1 appears to be the most consistent urinary biomarker of obstructive nephropathy, it can be augmented by serum/urine cystatin C, urine α1-microglobulin, urine NAG and urine NGAL. Whereas elevated urine TGF-β1 is an indicator of obstructive uropathy, it also correlates directly with urine α1-microglobulin (a surrogate marker of proteinuria). Increased serum cystatin C level would suggest a deterioration in glomerular filtration, while increased urine cystatin C level would signify a tubulopathy. These renal outcomes constitute the significant sequelae of PUV in children. Besides the role of NGAL as a predictor of a tubulointerstitial lesion, the indirect correlation of its urinary level with eGFR suggests its potential precision in detecting obstructive nephropathy. Finally, inclusion of NAG in the panel is predicated on the fact that it is an early predictor of tubulopathy and glomerulopathy. We excluded urinary cytokine (TNF-α, IL-6) levels from this proposed panel because they were not dependable as a screening test for clinically significant nephrouropathies (53). One study rather revealed an improved diagnostic performance of TNF-α for the early detection of concurrent renal injury in patients with PUV when used together with urine TGF-β1 and microalbuminuria (51).
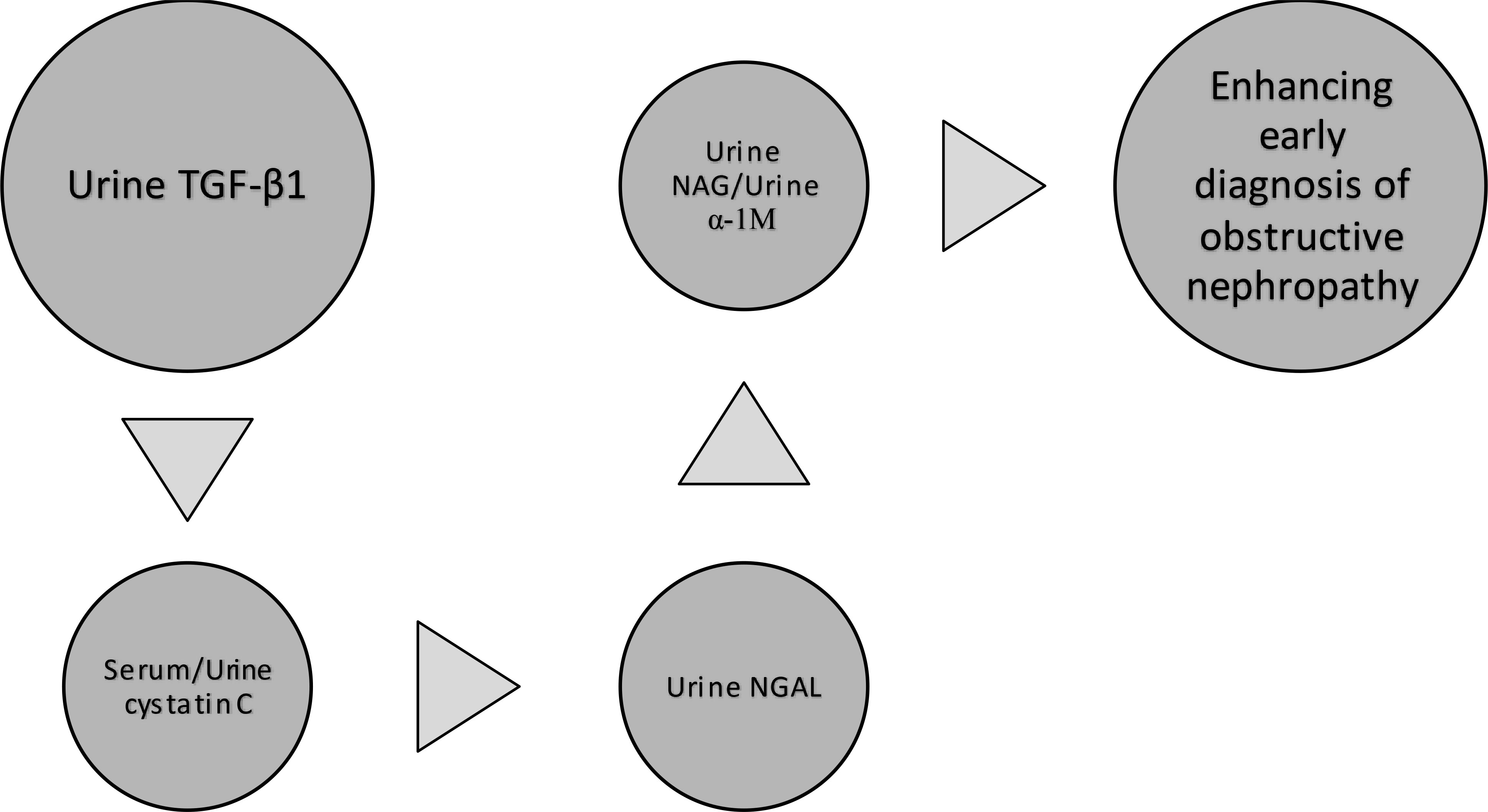
Figure 2 Proposed panel of biomarkers to enhance early diagnosis of obstructive nephropathy. TGF-β1, transforming growth factor-beta 1 NGAL, neutrophil gelatinase-associated lipocalin NAG, N-acetyl-β-D glucosaminidase α-1 M, α1-microglobulin.
Conclusion
Although most novel biomarkers are yet to be fully applied to routine clinical practice globally, there is substantial evidence showing that they form part of the emerging diagnostics for PUV-related CKD. Biomarkers of obstructive nephropathy are now well documented. Published reports indicate that raised urine levels of these biomarkers are associated with adverse renal outcomes in PUV. Additionally, their increased urine levels correlate well with reduced eGFR and microalbuminuria (a marker of glomerular injury). Urine TGF-β1 is the most prominent and consistent among the biomarkers of obstructive nephropathy. However, other novel approaches like the ML model (a form of health-related AI) and urodynamic parameters like bladder contractility index hold promise for PUV outcomes prediction (PUVOP). Because of the association of urine TGF-β1 with urine angiotensin level (a biomarker of the RAAS), early ACEI therapy in patients with PUV may potentially retard the progression of renal injury and improve renal outcomes. Thus, we suggest that further research directions should include conducting longitudinal studies that would properly demonstrate these biomarkers as predictors of future adverse renal outcomes. Secondly, it is important to explore the role of ACEI as a pre-emptive treatment for these outcomes in post valve- ablation patients.
Author contributions
SU conceived the topic, searched the literature, and wrote the initial draft. CO, NM, and IE searched the literature and criticized the initial draft. All authors read and approved the final draft.
Conflict of interest
The authors declare that the research was conducted in the absence of any commercial or financial relationships that could be construed as a potential conflict of interest.
Publisher’s note
All claims expressed in this article are solely those of the authors and do not necessarily represent those of their affiliated organizations, or those of the publisher, the editors and the reviewers. Any product that may be evaluated in this article, or claim that may be made by its manufacturer, is not guaranteed or endorsed by the publisher.
References
1. Nasir AA, Ameh EA, Abdur-Rahman LO, Adeniran JO, Abraham MK. Posterior urethral valve. World J Pediatr (2011) 7:205–16. doi: 10.1007/s12519-011-0289-1
2. Hodges SJ, Patel B, McLorie G, Atala A. Posterior urethral valves. Sci World J (2009) 9:1119–26. doi: 10.1100/tsw.2009.127
3. Alsaywid BS, Mohammed AF, Jbril SM, Bahashwan M, Mukharesh L, Al Khashan M. Renal outcome among children with posterior urethral valve: When to worry? Urol Ann (2021) 13:30–5. doi: 10.4103/UA.UA_112_19
4. Velhal R, Jain A, Nayan A, Patwardhan S, Patil B. Impact of surgical intervention on progression to end-stage renal disease in patients with posterior urethral valve. Afr J Urol (2021) 27:158. doi: 10.1186/s12301-021-00261-8
5. Farhat W, McLorie G, Capolicchio G, Khoury A, Bägli D, Merguerian PA. Outcomes of primary valve ablation versus urinary tract diversion in patients with posterior urethral valves. Urology (2000) 56:653−7. doi: 10.1016/s0090-4295(00)00784-6
6. Sarhan O, El−Ghoneimi A, Dawaba M, Ghaly A, Vesaigrain Y. Early versus late primary valve ablation: Does it affect the prognosis in children with posterior urethral valves. J Pediatr Urol (2008) 4(S1):S68. doi: 10.1016/j.jpurol.2008.01.157
7. Hennus PM, van der Heijden GJ, Bosch JL, de Jong TP, de Kort LM. A systematic review on renal and bladder dysfunction after endoscopic treatment of infravesical obstruction in boys. PLoS One (2012) 7:e44663. doi: 10.1371/journal.pone.0044663
8. Uwaezuoke SN, Muoneke UV, Mbanefo NR. Future risks of chronic kidney disease and end-stage kidney disease in infants with postnatally-repaired posterior urethral valve: A systematic review. J Integr Nephrol Androl (2020) 7:23–33. doi: 10.4103/jina.jina_9_20
9. Sharma S, Joshi M, Gupta DK, Abraham M, Mathur P, Mahajan JK, et al. Consensus on the management of posterior urethral valves from antenatal period to puberty. J Indian Assoc Pediatr Surg (2019) 24:4–14. doi: 10.4103/jiaps.JIAPS_148_18
10. Holmes N, Harrison MR, Baskin LS. Fetal surgery for posterior urethral valves: long-term postnatal outcomes. Pediatrics (2001) 108:E7. doi: 10.1542/peds.108.1.e7
11. Sananes N, Cruz-Martinez R, Favre R, Ordorica-Flores R, Moog R, Zaloszy A, et al. Two-year outcomes after diagnostic and therapeutic fetal cystoscopy for lower urinary tract obstruction. Prenat Diagn (2016) 36:297–303. doi: 10.1002/pd.4771
12. Uwaezuoke SN, Odetunde OI, Okafor HU. The significance of abnormal urine stream in the male infant: A survey of mothers’ knowledge in a south-east Nigerian city and implications for preventive nephrology. J Adv Med Med Res (2015) 9:1–9. doi: 10.9734/BJMMR/2015/19165
13. Okafor HU, Ekenze SO, Uwaezuoke SN. Posterior urethral valves: Determinants of outcome in a developing country. J Paed Child Health (2013) 49:115–9. doi: 10.1111/JPC.12091
14. Wu CQ, Blum ES, Patil D, Shin HS, Smith EA. Predicting childhood chronic kidney disease severity in infants with posterior urethral valve: A critical analysis of creatinine values in the first year of life. Pediatr Nephrol (2021) 37:1339–45. doi: 10.1007/s00467-021-05271-w
15. Coleman R, King T, Nicoara CD, Bader M, McCarthy L, Chandran H, et al. Nadir creatinine in posterior urethral valves: How high is low enough? J Pediatr Urol (2015) 11:356.e1–5. doi: 10.1016/j.jpurol.2015.06.008
16. Coquillette M, Lee RS, Pagni SE, Cataltepe S, Stein DR. Renal outcomes of neonates with early presentation of posterior urethral valves: A 10−year single-center experience. J Perinatol (2020) 40:112−7. doi: 10.1038/s41372-019-0489-4
17. Lopez Pereira P, Espinosa L, Martinez Urrutina MJ, Lobato R, Navarro M, Jaureguizar E. Posterior urethral valves: Prognostic factors. BJU Int (2003) 91:687−90. doi: 10.1046/j.1464-410x.2003.04178.x
18. Tambo FF, Tolefac PN, Ngowe MN, Ze Minkande J, Mbouche L, Guemkam G, et al. Posterior urethral valves: 10 years audit of epidemiologic, diagnostic, and therapeutic aspects in yaoundé gynaeco−obstetric and pediatric hospital. BMC Urol (2018) 18:46. doi: 10.1186/s12894-018-0364-1
19. Hojs R, Bevc S, Ekart R, Gorenjak M, Puklavec L. Serum cystatin c as an endogenous marker of renal function in patients with mild to moderate impairment of kidney function. Nephrol Dial Transplant (2006) 21:1855–62. doi: 10.1093/ndt/gfl073
20. Earley A, Miskulin D, Lamb EJ, Levey AS, Uhlig K. Estimating equations for glomerular filtration rate in the era of creatinine standardization: A systematic review. Ann Intern Med (2012) 156:785–95. doi: 10.7326/0003-4819-156-6-201203200-00391
21. Coca SG, Yalavarthy R, Concato J, Parikh CR. Biomarkers for the diagnosis and risk stratification of acute kidney injury: A systematic review. Kidney Int (2008) 73:1008–16. doi: 10.1038/sj.ki.5002729
22. Dharnidharka VR, Kwon C, Stevens G. Serum cystatin c is superior to serum creatinine as a marker of kidney function: A meta-analysis. Am J Kidney Dis (2002) 40:221–6. doi: 10.1053/ajkd.2002.34487
23. Shimizu-Tokiwa A, Kobata M, Io H, Kobayashi N, Shou I, Funabiki K, et al. Serum cystatin c is a more sensitive marker of glomerular function than serum creatinine. Nephron (2002) 92:224–6. doi: 10.1159/000064453
24. Hoek FJ, Kempermen FAW, Krediet RT. A comparison between cystatin c, plasma creatinine, and the cockcroft and gault for the estimation of glomerular filtration rate. Nephrol Dial Transplant (2003) 18:2024–31. doi: 10.1093/ndt/gfg349
25. Shlipak MG, Matsushita K, Ärnlöv J, Inker LA, Katz R, Polkinghorneet KR, et al. Cystatin c versus creatinine in determining risk based on kidney function. N Engl J Med (2013) 369:932–43. doi: 10.1056/NEJMoa1214234
26. Baxmann AC, Ahmed MS, Marques NC, Menon VB, Pereira AB, Kirsztajn GM, et al. Influence of muscle mass and physical activity on serum and urinary creatinine and serum cystatin c. Clin J Am Soc Nephrol (2008) 3:348–54. doi: 10.2215/CJN.02870707
27. Vupputuri S, Fox CS, Coresh J, Woodward M, Muntner P. Differential estimation of CKD using creatinine versus cystatin c-based estimating equations by category of body mass index. Am J Kidney Dis (2009) 53:993–1001. doi: 10.1053/j.ajkd.2008.12.043
28. Peralta CA, Shlipak MG, Judd S, Cushman M, McClellan W, Zakai NA, et al. Detection of chronic kidney disease with creatinine, cystatin c, and urine albumin-to-creatinine ratio and associations with progression to end-stage renal disease and mortality. JAMA (2011) 305:1545–52. doi: 10.1001/jama.2011.468
29. Conti M, Moutereau S, Zater M, Lallali K, Durrbach A, Manivet P, et al. Urinary cystatin c as a specific marker of tubular dysfunction. Clin Chem Lab Med (2006) 44:288–91. doi: 10.1515/CCLM.2006.050
30. Uwaezuoke SN, Ayuk AC, Muoneke UV, Mbanefo NR. Chronic kidney disease in children: Novel biomarkers as predictors of disease. Saudi J Kidney Dis Transplant (2018) 29:775–84. doi: 10.4103/1319-2442.239657
31. Uwaezuoke SN. The role of novel biomarkers in childhood idiopathic nephrotic syndrome: A narrative review of published evidence. Int J Nephrol Renovasc Dis (2017) 10:123–8. doi: 10.2147/IJNRD.S131869
32. Uwaezuoke SN. The role of novel biomarkers in predicting diabetic nephropathy: A review. Int J Nephrol Renovasc Dis (2017) 10:221–31. doi: 10.2147/IJNRD.S143186
33. Trnka P, Ivanova L, Hiatt MJ, Matsell DG. Urinary biomarkers in obstructive nephropathy. Clin J Am Soc Nephrol (2012) 7:1567–75. doi: 10.2215/CJN.09640911
34. Lopez-Giacoman S, Madero M. Biomarkers in chronic kidney disease: from kidney function to kidney damage. World J Nephrol (2015) 4:57–73. doi: 10.5527/wjn.v4.i1.57
35. Butt MJ, Tarantal AF, Jimenez DF, Matsell DG. Collecting duct epithelial-mesenchymal transition in fetal urinary tract obstruction. Kidney Int (2007) 72:936–44. doi: 10.1038/sj.ki.5002457
36. Trnka P, Hiatt MJ, Ivanova L, Tarantal AF, Matsell DG. Phenotypic transition of the collecting duct epithelium in congenital urinary tract obstruction. J BioMed Biotechnol (2010) 2010:696034. doi: 10.1155/2010/696034
37. Zieg J, Blahova K, Seeman T, Bronsky J, Dvorakova H, Pechova M, et al. Urinary transforming growth factor-β1 in children with obstructive uropathy. Nephrol (Carlton) (2011) 16:595–8. doi: 10.1111/j.1440-1797.2011.01459.x
38. Puri A, Bhatnagar V, Grover VP, Agarwala S, Mitra DK. Urodynamics-based evidence for the beneficial effect of imipramine on valve bladders in children. Eur J Pediatr Surg (2005) 15:347–53. doi: 10.1055/s-2005-865842
39. Lal R, Bhatnagar V, Mitra DK. Long−term prognosis of renal function in boys treated for posterior urethral valves. Eur J Pediatr Surg (1999) 9:307−1. doi: 10.1055/s-2008-1072270
40. Ghanem MA, Wolffenbuttel KP, De Vylder A, Nijman RJ. Long−term bladder dysfunction and renal function in boys with posterior urethral valves based on urodynamic findings. J Urol (2004) 171:2409−12. doi: 10.1097/01.ju.0000127762.95045.93
41. Aitken KJ, Bägli DJ. The bladder extracellular matrix. part I: Architecture, development, and disease. Nat Rev Urol (2009) 6:596–611. doi: 10.1038/nrurol.2009.201
42. De Gennaro M, Capitanucci ML, Capozza N, Caione P, Mosiello G, Silveri M. Detrusor hypocontractility in children with posterior urethral valves arises before puberty. Br J Urol (1998) 81(Suppl 3):81–5. doi: 10.1046/j.1464-410x.1998.00014.x
43. Parkhouse HF, Woodhouse CR. Long−term status of patients with posterior urethral valves. Urol Clin North Am (1990) 17:373−8.
44. Ansari MS, Nunia SK, Bansal A, Singh P, Sekhon V, Singh D, et al. Bladder contractility index in posterior urethral valve: A new marker for early prediction of progression to renal failure. J Pediatr Urol (2018) 14:162.e1−5. doi: 10.1016/j.jpurol.2017.09.029
45. Parkhouse HF, Barratt TM, Dillon MJ, Duffy PG, Fay J, Ransley PG, et al. Long-term outcome of boys with posterior urethral valves. Br J Urol (1988) 62:59–62. doi: 10.1111/j.1464-410x.1988.tb04267.x
46. Bhadoo D, Bajpai M, Panda SS. Posterior urethral valve: Prognostic factors and renal outcome. J Indian Assoc Pediatr Surg (2014) 19:133–7. doi: 10.4103/0971-9261.136459
47. Ansari MS, Gulia A, Srivastava A, Kapoor R. Risk factors for progression to end-stage renal disease in children with posterior urethral valves. J Pediatr Urol (2010) 6:261–4. doi: 10.1016/j.jpurol.2009.09.001
48. Roth KS, Carter WH Jr., Chan JC. Obstructive nephropathy in children: Long−term progression after relief of posterior urethral valve. Pediatrics (2001) 107:1004−10. doi: 10.1542/peds.107.5.1004
49. Uthup S, Binitha R, Geetha S, Hema R, Kailas L. A follow−up study of children with posterior urethral valve. Indian J Nephrol (2010) 20:72−5. doi: 10.4103/0971-4065.65298
50. MacRae Dell K, Hoffman BB, Leonard MB, Ziyadeh FN, Schulman SL. Increased urinary transforming growth factor-beta (1) excretion in children with posterior urethral valves. Urology (2000) 56:311–4. doi: 10.1016/s0090-4295(00)00591-4
51. Mandelia A, Bajpai M, Agarwala S, Gupta AK, Kumar R, Ali A. The role of urinary TGF-β₁, TNF-α, IL-6, and microalbuminuria for monitoring therapy in posterior urethral valves. Pediatr Nephrol (2013) 28:1991–2001. doi: 10.1007/s00467-013-2506-8
52. Vasconcelos MA, Bouzada MC, Silveira KD, Moura LR, Santos FF, Oliveira JM, et al. Urinary levels of TGF β-1 and of cytokines in patients with prenatally detected nephrouropathies. Pediatr Nephrol (2011) 26:739–47. doi: 10.1007/s00467-011-1802-4
53. Panigrahi P, Chowdhary S, Sharma SP, Kumar R, Agarwal N, Sharma SP. Role of urinary transforming growth factor beta-B1 and monocyte chemotactic protein-1 as prognostic biomarkers in posterior urethral valve. J Indian Assoc Pediatr Surg (2020) 25:219–24. doi: 10.4103/jiaps.JIAPS_104_19
54. Vaughn SP, Broussard S, Hall CR, Scott A, Blanton SH, Milunsky JM, et al. Confirmation of the mapping of the camurati-englemann locus to 19q13.2 and refinement to a 3.2-cM region. Genomics (2000) 66:119–21. doi: 10.1006/geno.2000.6192
55. Olsson MG, Allhorn M, Bulow L, Hansson SR, Ley D, Olsson ML, et al. Pathological conditions involving extracellular hemoglobin: Molecular mechanisms, clinical significance, and novel therapeutic opportunities for alpha-1-microglobulin. Antioxid Redox Signal (2012) 17:813–46. doi: 10.1089/ars.2011.4282
56. Åkerström B, Lögdberg L, Berggård T, Osmark P, Lindqvist A. Alpha-1-microglobulin: A yellow-brown lipocalin. Biochem Biophys Act (2000) 1482:172–84. doi: 10.1016/s0167-4838(00)00157-6
57. Yoshimura A, Wakabayashi Y, Mori T. Cellular and molecular basis for the regulation of inflammation by TGF-β. J Biochem (2010) 147:781–92. doi: 10.1093/jb/mvq043
58. Theron AJ, Anderson R, Rossouw TM, Steel HC. The role of transforming growth factor beta-1 in the progression of HIV/AIDS and development of non-AIDS-defining fibrotic disorders. Front Immunol (2017) 8 1461. doi: 10.3389/fimmu.2017
59. Jeon YK, Kim MR, Huh JE, Mok JY, Song SH, Kim SS, et al. Cystatin c as an early biomarker of nephropathy in patients with type 2 diabetes. J Korean Med Sci (2011) 26:258–63. doi: 10.3346/jkms.2011.26.2.258
60. Kim SS, Song SH, Kim IJ, Jeon YK, Kim BH, Kwak IS, et al. Urinary cystatin c and tubular proteinuria predict progression of diabetic nephropathy. Diabetes Care (2003) 36:656–61. doi: 10.2337/dc12-0849
61. Shore N, Khurshid R, Saleem M. Alpha-1 microglobulin: a marker for early detection of tubular disorders in diabetic nephropathy. J Ayub Med Coll Abbottabad (2010) 22:53–5.
62. Hong CY, Hughes K, Chia KS, Ng V, Ling SL. Urinary alpha 1-microglobulin as a marker of nephropathy in type 2 diabetic Asian subjects in Singapore. Diabetes Care (2003) 26:338–42. doi: 10.2337/diacare.26.2.338
63. Mishra J, Mori K, Ma Q, Kelly C, Barasch J, Devarajan P. Neutrophil gelatinase-associated lipocalin: A novel early urinary biomarker for cisplatin nephrotoxicity. Am J Nephrol (2004) 24:307–15. doi: 10.1159/000078452
64. Ding H, He Y, Li K, Yang J, Li X, Lu R, et al. Urinary neutrophil gelatinase-associated lipocalin (NGAL) is an early biomarker for renal tubulointerstitial injury in IgA nephropathy. Clin Immunol (2007) 123:227–34. doi: 10.1016/j.clim.2007.01.010
65. Bolignano D, Coppolino G, Campo S, Aloisi C, Nicocia G, Frisina N, et al. Urinary neutrophil gelatinase-associated lipocalin (NGAL) is associated with severity of renal disease in proteinuric patients. Nephrol Dial Transplant (2008) 23:414–6. doi: 10.1093/ndt/gfm541
66. Bolignano D, Coppolino G, Campo S, Aloisi C, Nicocia G, Frisina N, et al. Neutrophil gelatinase-associated lipocalin in patients with autosomal-dominant polycystic kidney disease. Am J Nephrol (2007) 27:373–8. doi: 10.1159/000103912
67. Bhavsar NA, Köttgen A, Coresh J, Astor BC. Neutrophil gelatinase-associated lipocalin (NGAL) and kidney injury molecule 1 (KIM-1) as predictors of incident CKD stage 3: The atherosclerosis risk in communities (ARIC) study. Am J Kidney Dis (2012) 60:233–40. doi: 10.1053/j.ajkd.2012.02.336
68. Smith ER, Lee D, Cai MM, Tomlinson LA, Ford ML, McMahon LP, et al. Urinary neutrophil gelatinase-associated lipocalin may aid prediction of renal decline in patients with non-proteinuric stages 3 and 4 chronic kidney disease (CKD). Nephrol Dial Transplant (2013) 28:1569–79. doi: 10.1093/ndt/gfs586
69. Bolignano D, Lacquaniti A, Coppolino G, Donato V, Campo S, Fazio MR, et al. Neutrophil gelatinase-associated lipocalin (NGAL) and progression of chronic kidney disease. Clin J Am Soc Nephrol (2009) 4:337–44. doi: 10.2215/CJN.03530708
70. Kern EF, Erhard P, Sun W, Genuth S, Weiss MF. Early urinary markers of diabetic kidney disease: A nested case-control study from the diabetes control and complications trial (DCCT). Am J Kidney Dis (2010) 55:824–34. doi: 10.1053/j.ajkd.2009.11.009
71. Patel DN, Kaila K. Efficacy of urinary n-acetyl-β-D glucosaminidase to evaluate early renal tubular damage as a consequence of type 2 diabetes mellitus: A cross-sectional study. Int J Diabetes Dev Ctries (2015) 35(Suppl 3):449–57. doi: 10.1007/s13410-015-0404-2
72. Jones AP, Lock S, Griffiths KD. Urinary n-acetyl-β-D glucosaminidase activity in type 1 diabetes mellitus. Ann Clin Biochem (2006) 21:142–8. doi: 10.1177/000456329503200104
73. Bazzi C, Petrini C, Rizza V, Arrigo G, Napodano P, Paparella M, et al. Urinary n-acetyl-β-D glucosaminidase excretion is a marker of tubular cell dysfunction and predictor of outcome in primary glomerulonephritis. Nephrol Dial Transplant (2002) 17:1890–6. doi: 10.1093/ndt/17.11.1890
74. Lucarelli G, Mancini V, Galleggiante V, Rutigliano M, Vavallo A, Battaglia M, et al. Emerging urinary markers of renal injury in obstructive nephropathy. BioMed Res Int (2014) 2014:7:303298. doi: 10.1155/2014/303298
75. Rüster C, Wolf G. Renin-angiotensin-aldosterone system and progression of renal disease. J Am Soc Nephrol (2006) 17:2985–91. doi: 10.1681/ASN.2006040356
76. Felsen D, Schulsinger D, Gross SS, Kim FY, Marion D, Vaughan ED Jr. Renal hemodynamic and ureteral pressure changes in response to ureteral obstruction: The role of nitric oxide. J Urol (2003) 169:373–6. doi: 10.1097/01.ju.0000039820.60560.74
77. Humes HD, Cieslinski DA, Coimbra TM, Messana JM, Galvao C. Epidermal growth factor enhances renal tubule cell regeneration and repair and accelerates the recovery of renal function in post-ischemic acute renal failure. J Clin Invest (1989) 84:1757–61. doi: 10.1172/JCI114359
78. Kennedy WA II, Buttyan R, Garcia-Montes E, Olsson CA, Sawczuk IS. Epidermal growth factor suppresses renal tubular apoptosis following ureteral obstruction. Urology (1997) 49:973–80. doi: 10.1016/s0090-4295(97)00101-5
79. Grandaliano G, Gesualdo L, Bartoli F, Ranieri E, Monno R, Leggio A, et al. MCP-1, and EGF renal expression and urine excretion in human congenital obstructive nephropathy. Kidney Int (2000) 58:182–92. doi: 10.1046/j.1523-1755.2000.00153.x
80. Torres DD, Rossini M, Manno C, Mattace-Raso F, D'Altri C, Ranieri E, et al. The ratio of epidermal growth factor to monocyte chemotactic peptide-1 in the urine predicts renal prognosis in IgA nephropathy. Kidney Int (2008) 73(3):327–33. doi: 10.1038/sj.ki.5002621
81. Lim AI, Tang SCW, Lai KN, Leung JCK. Kidney injury molecule-1: more than just an injury marker of tubular epithelial cells? J Cell Physiol (2013) 228:917–24. doi: 10.1002/jcp.24267
82. Wasilewska A, Taranta-Janusz K, Debek W, Zoch-Zwierz W, Kuroczycka-Saniutycz E. KIM-1 and NGAL: New markers of obstructive nephropathy. Pediatr Nephrol (2011) 26:579–86. doi: 10.1007/s00467-011-1773-5
83. Kwong JC, Khondker A, Kim JK, Chua M, Keefe DT, Dos Santos J, et al. Posterior urethral valves outcomes prediction (PUVOP): A machine learning tool to predict clinically relevant outcomes in boys with posterior urethral valves. Pediatr Nephrol (2022) 37:1067–74. doi: 10.1007/s00467-021-05321-3
84. Kostic D, Dos Santos Beozzo GPN, do Couto SB, Kato AHT, Lima L, Palmeira P, et al. First-year profile of biomarkers for early detection of renal injury in infants with congenital urinary tract obstruction. Pediatr Nephrol (2019) 34:1117–28. doi: 10.1007/s00467-019-4195-4
Keywords: biomarkers of renal function, biomarkers of renal injury, chronic kidney disease, endstage kidney disease, obstructive nephropathy, posterior urethral valve
Citation: Uwaezuoke SN, Odimegwu CL, Mbanefo NR and Eze IC (2022) Posterior urethral valve in children: Using novel biomarkers as an early predictive tool for the onset and progression of chronic kidney disease. Front. Urol. 2:904452. doi: 10.3389/fruro.2022.904452
Received: 25 March 2022; Accepted: 01 August 2022;
Published: 26 August 2022.
Edited by:
Sachit Anand, Kokilaben Dhirubhai Ambani Hospital and Medical Research Institute, IndiaCopyright © 2022 Uwaezuoke, Odimegwu, Mbanefo and Eze. This is an open-access article distributed under the terms of the Creative Commons Attribution License (CC BY). The use, distribution or reproduction in other forums is permitted, provided the original author(s) and the copyright owner(s) are credited and that the original publication in this journal is cited, in accordance with accepted academic practice. No use, distribution or reproduction is permitted which does not comply with these terms.
*Correspondence: Samuel N. Uwaezuoke, samuel.uwaezuoke@unn.edu.ng