- 1German Society for Tissue Transplantation (DGFG) gGmbH, Hannover, Germany
- 2Klinik für Plastische Chirurgie, Hand- und Verbrennungschirurgie, Rhein-Maas Klinikum GmbH, Würselen, Germany
The human amniotic membrane (hAM) is the inner layer of the placenta and plays protective and nutritional roles for the fetus during pregnancy. It contains multiple growth factors and proteins that mediate unique regenerative properties and enhance wound healing in tissue regeneration. Due to these characteristics hAM has been successfully utilized in ophthalmology for many decades. This material has also found application in a variety of additional therapeutic areas. Particularly noteworthy are the extraordinary effects in the healing of chronic wounds and in the treatment of burns. But hAM has also been used successfully in gynecology, oral medicine, and plastic surgery and as a scaffold for in vitro cell culture approaches. This review aims to summarize the different graft preparation, preservation and storage techniques that are used and to present advantages and disadvantages of these methods. It shows the characteristics of the hAM according to the processing and storage methods used. The paper provides an overview of the currently mainly used application areas and raises new application possibilities. In addition, further preparation types like extracts, homogenates, and the resulting treatment alternatives are described.
1. Introduction
The human amniotic membrane (hAM) is the inner layer of the placenta and plays protective and nutritional roles for the fetus during pregnancy. The hAM is a relatively simple tissue consisting of an epithelium and a stroma which in turn can be divided in the basement membrane and a compact, fibroblast and spongy layer, respectively (Figure 1). Its characteristics and properties make it ideal for use in many medical fields. For example, no or low amounts of HLA antigens (A, B, C, DR) are expressed by the cellular components (3, 4), so that hAM can be described as non-immunogenic (5, 6), and it is not rejected after transplantation (7–9), even after experimental xenotransplantation (10). This property is not too surprising since the human amniotic membrane is located during pregnancy between two individuals with their divergent immune systems (11). Antimicrobial (12), anti-inflammatory, and antiangiogenic properties have been demonstrated (13–16). The anti-fibrotic activity helps to prevent scarring, another important property that has made the use of amniotic membrane valuable in medical therapy (17–19).
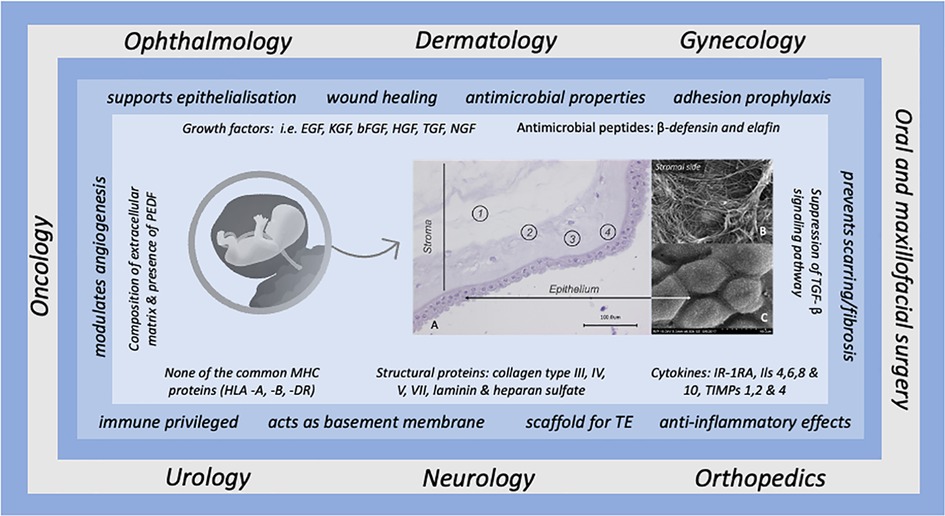
Figure 1. Overview of the most common applications of human amniotic membrane in conjunction with the beneficial effects attributed to it, based on the underlying biological factors. The histological illustration (left, A) shows the morphology of the hAM, the numbers indicate the individual structural elements of the hAM. 1 = Spongy layer, 2 = Fibroblast layer, 3 = Compact layer, 4 = Basement membrane. SEM images (right) display the surfaces (B) stromal side, (C) epithelial side at 5000x magnification (from Pogozhykh et al. (1). Pigment epithelial-derived factor (PEDF) is a non-inhibitory serpin with neuroprotective and antiangiogenic action (Tombran-Tink) (2)
In addition, amniotic membrane stimulates cell migration and proliferation, which are crucial for wound healing. Growth promotion is mainly mediated by growth factors such as epidermal growth factor (EGF), keratinocyte growth factor (KGF), hepatocyte growth factor (HGF) and fibroblast Growth Factor (bFGF) (20).
Due to its diverse characteristics hAM has been successfully utilized in ophthalmology for many decades, but also found application in additional therapeutic areas, such as healing of chronic wounds and in the treatment of burns, as well as in gynecology, oral medicine, and plastic surgery. A recent review provides a comprehensive overview of the different therapeutic targets and the mechanisms of action involved (21). Figure 1 summarizes the most described functions of hAM in connection to their potential mode of action in different fields of medical applications (22, 23).
To utilize amniotic membrane for these therapeutic purposes without risk and with the most benefit for the patient it is necessary to apply the best possible ways of collection, processing and storage while preserving their necessary effective structures. The review aims to give an overview of the possible processing methods involved in human amniotic membrane preparation, storage, preservation, and the available data regarding the characteristics of the membrane prepared by each technique.
2. Preparation and preservation techniques of hAM
The human placenta is typically obtained during a planned cesarean delivery under sterile surgical conditions. The donors provide their informed consent and are tested for various infectious diseases (e.g., HIV, HCV, HBV, Treponema pallidum). This ensures a low risk of disease transmission, a low bioburden and a minimal risk of contamination right from the start. Usually, this is followed by cooled transport to a specialized tissue bank, where the placenta is processed.
Prior to processing, the tissue is examined for specific conditions that would exclude hAM donation following the European guide to the quality and safety of tissues and cells for human application (24), such as premature rupturing of membranes, malformation of the fetus, or presence of endometritis or meconium ileus. Irrespective of the further procedure, the preparation starts with cleaning the placenta of adherent blood residues by rinsing with physiological solution. Then, the amniotic membrane is separated from the placenta and the chorionic part.
Depending on how the hAM is stored eventually, incubation in antibiotic-containing solution follows for varying lengths of time, commonly at least 1 h and up to 24 h. Most processing methods use a carrier material, e.g., a nitrocellulose membrane, in the further course to keep the thin hAM manageable. In this way different sizes can be cut depending on the purpose for which the hAM is to be used. For ophthalmological applications, pieces of 2 cm × 2 cm are mostly sufficient; in dermatological wound healing, the areas must be larger (25).
The further procedure is determined by the type of preservation that is to be applied. Table 1 provides an overview about the main techniques that are used: fresh or deep frozen, cryopreserved, and dried, with heat-dried, air-dried and freeze-dried/lyophilized being common. Glycerol-preserved hAM can also be found, as well as mixed forms, some of which are patented and therefore not disclosed.
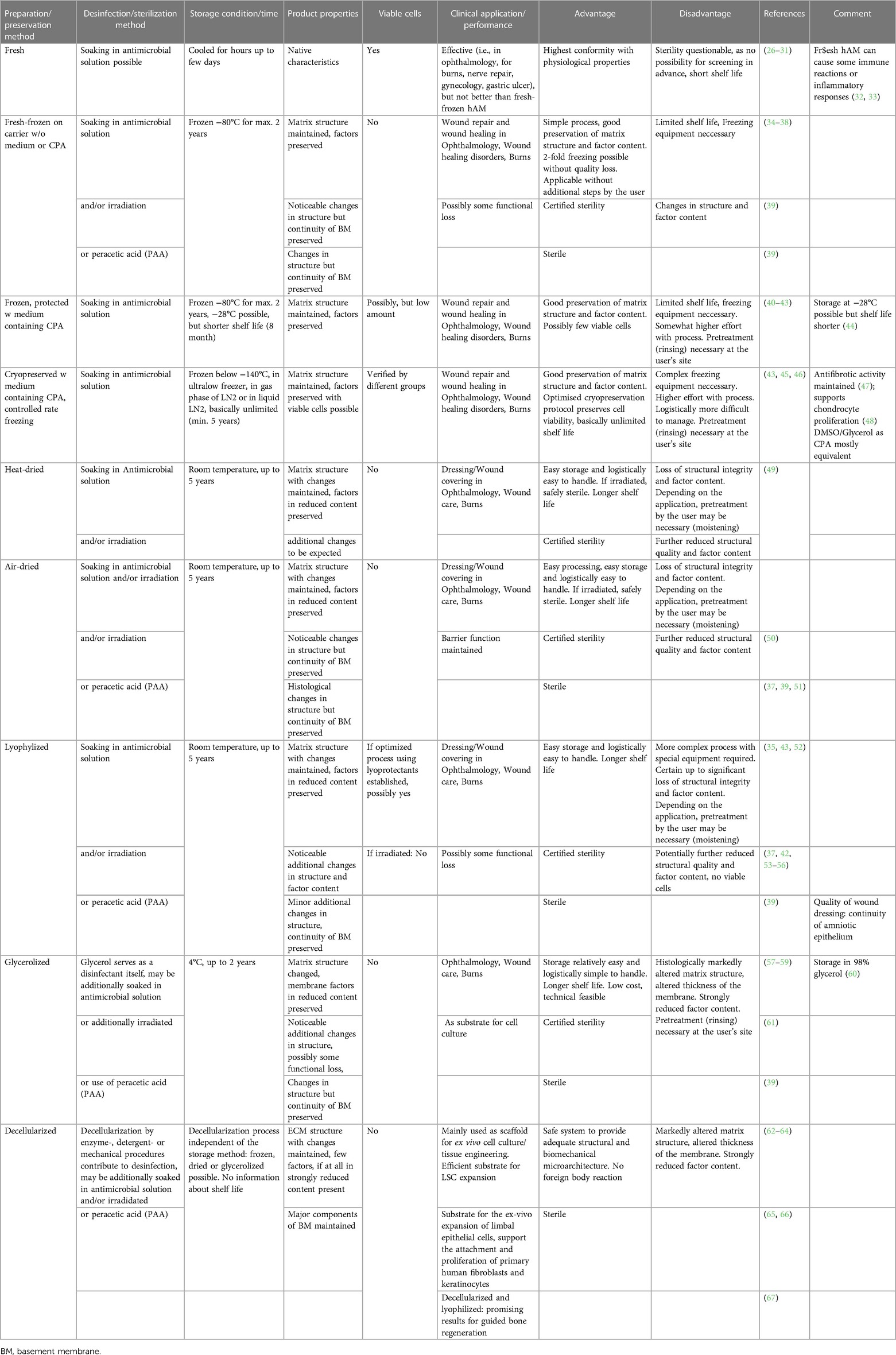
Table 1. Overview about the main techniques that are used to prepare and sterilize human amniotic membrane for medical application.
For fresh-frozen storage, the hAM is frozen on the carrier material at −80°C without further additives. Deep-frozen storage at −80°C is also possible: cryoprotected in a solution containing freeze-protective agents such as glycerol or dimethyl sulfoxide (DMSO), typically in a concentration of 5%–10% or in culture medium such as RPMI or physiological solutions without further protection. Samples can be stored for up to 2 years, but a freezer capable of holding temperatures of −80°C is required. Although it has been shown that storage at −20°C to −28°C is possible with a shorter storage time (44, 68). After thawing, tissues must be used within 6 h.
Glycerol or DMSO in this concentration are also used as cryoprotective agents (CPA) for cryopreservation of hAM. Cryopreservation, by definition, requires, in addition to using CPAs, a controlled freezing process by means of a controlled rate freezer to a deep-cold temperature (24). In addition to slow freezing, there is the possibility of vitrification, a process in which the aqueous phase is transformed directly to a glass phase (69). However, this has only been used experimentally so far. The final temperature is usually between −80°C to −140°C but can be as low as liquid nitrogen (LN2) temperature (−196°C). Subsequent storage should always be below the glass transition temperature of water, i.e., colder than −137°C to prevent further recrystallization that could lead to freezing damage. Ultralow freezers are used to reach about −152°C, alternatively storage is in the gas phase of LN2 at about −180°C or directly in LN2. In the liquid phase, however, it is necessary to seal the storage vessels so that no liquid nitrogen can penetrate, since it is only possible to produce sterile liquid nitrogen with great effort and there is otherwise a risk of contamination of the material. Cryopreservation allows longer storage. In principle, samples stored constantly below −137°C have an indefinite stability without further changes in quality, but usually 5 years shelf life is given for this condition. The disadvantage is the relatively complex and costly equipment needed for both the freezing process and long-term storage.
If the hAM is to be stored dry, it can be air-dried under a sterile class A workbench or heat-dried in an oven at about 40°C overnight after preparation. Alternatively, the tissue can be lyophilized, in which case a rapid freezing process to −80°C is followed by vacuum-drying in special devices. For this purpose, protective agents (LPA = lyoprotective agent) like trehalose or sucrose should be used (52, 56, 70), which on the one hand must protect against the damage caused by freezing, but additionally against the adverse effects of drying. Drying allows storage without special equipment at room temperature for a longer period which offers logistical advantages.
Glycerolized hAM is also used. For its production, the membrane is usually preserved in 85% glycerol and can thus be stored at 2°C–8°C. It has also been described that the glycerol concentration can be increased to 98% without compromising the clinical efficacy of hAM (60). The advantage is the simple storage with a relatively long shelf life of 2 years. However, grafts must be pretreated before use so that the patient is not affected by the glycerol.
Decellularization is a further option for processing hAM. Enzymes, detergents and/or mechanical procedures remove the cellular components. Although extracellular matrix structure is mainly preserved, it shows considerable changes (63). While ECM components like collagens I, II, IV, VI and VII, laminin-5, fibronectin, elastin and thrombospondin, remain present after most of the techniques, growth factors like TGF-a,-b1 and -b2 receptor, EGFR, KGF, bFGF, VEGF, and PDGF are only found when gentle procedures are used (43).
To make the use of hAM safe for the patient, it is essential to apply all possible measures that support sterility of the tissue. As described, this starts with the collection in a clean operation theatre and continues with the preparation under sterile conditions. While the use of cryogenic preservation procedures usually focuses on the use of antimicrobial substances, drying procedures are often followed by sterilization steps using irradiation with at least 25 kGy. Irradiation is also used for glycerolized hAM for sterilization purposes (71). In addition, glycerol itself has a disinfecting effect (59). Another possibility is the use of peracetic acid (PAA) for sterilization (39).
Most of the above-mentioned procedures are also used for hAM preparations which are commercially available, with the vast majority being freeze-dried variants. A comprehensive list of commercial hAM products can be found in a publication by Munoz-Torres et al. (21).
3. Characteristics of hAM in relation to the processing methods
Processing procedures have an impact on the properties of the human amniotic membrane (43).
The method used depends primarily on what impact on the biological material is desired or accepted. If for example the presence of viable cells in the hAM is irrelevant, stricter measures can be applied than if the vitality of the tissue is considered most important. Thus, the combination chosen must be weighed against which of the product's properties will ultimately be given the highest priority (Figure 2). For example, in ophthalmology the amniotic basement membrane facilitates migration and growth of epithelial cells, therefore promoting epithelialization. The avascular stroma of the hAM reduces fibrovascular ingrowth and abnormal neovascularization. And amniotic epithelium contains anti-inflammatory and growth factors beneficial to the treatment of inflammatory corneal diseases (72).
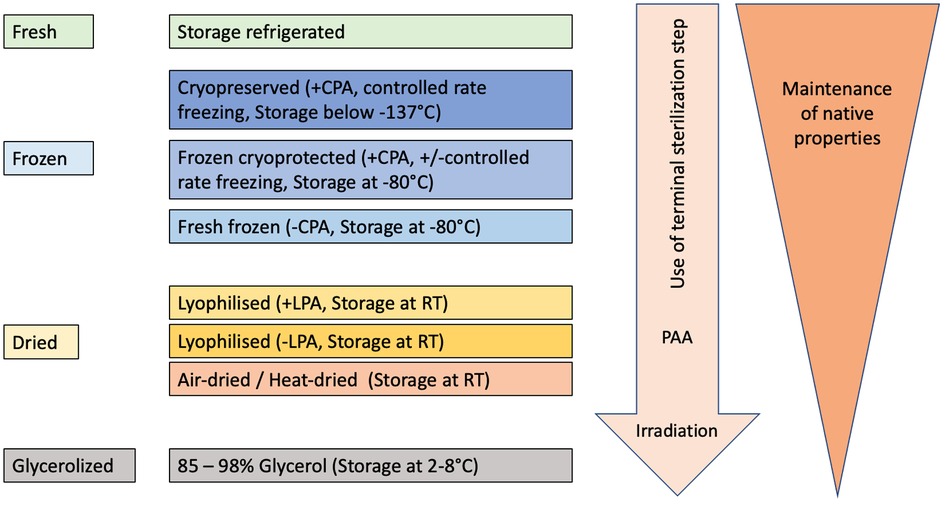
Figure 2. Schematic view of decreasing preservation of native properties of hAM depending on the degree of manipulation during processing, sterilization, and storage procedures.
Irrespective of the preparation and preservation processes the overall structural properties of the hAM are largely preserved. For example, the architecture of the basement membrane (BM) is maintained (39), which is considered to be the most important element for the application in ophthalmology (18). The hAM's basement membrane contains Type IV collagen, laminin 1, laminin 5, and collagen VII like the cornea BM, and these components are important for epithelial adhesion and growth (34).
Especially the freezing/cryopreservation procedures do not significantly alter the histological appearance and architectural properties of hAM (37, 73). Tan et al. (74) confirmed by histochemical staining that the cryopreservation process did not noticeably change the tissue architecture nor collagen and glycosaminoglycan density. In addition, important other structural components like high molecular weight hyaluronic acid or heavy chain-HA complex (i.e., the HC–HA/PTX3 complex) appear to be preserved better by cryopreservation than by drying (34, 75). Furthermore, it was shown that even after a second freezing process, the structural integrity of the hAM is maintained (1) with morphologically intact, nonviable cells. While hAM can be used directly after fresh freezing, it must first be rinsed after CPA containing freezing/cryopreservation processes to remove the substances. It cannot be ruled out that this may result in the loss of factors that were retained during the actual preservation process.
However, differences appear in the thickness of the final product: while after drying the tissue is 20–30 μm thick, hAM thickness varied between 45 and 50 μm after glycerol preservation due to liquid deposit in the membrane (39).
Biomechanical characteristics of hAM described by Dadkhah-Tehrani et al. (23) seem also to be well preserved by freezing (1, 36): Tensile strength and Young's modulus were not influenced by frozen storage methods regardless of whether a CPA (glycerol) was used or the membrane was frozen without additives. This does not change even if the material is only stored at −28°C instead of −80°C (44). However, these parameters are significantly altered when the material is dried (76). This factor should be considered since elasticity, in addition to practical considerations during surgery, could also influence the adherence, proliferation and phenotype of cells (77).
It should be considered that mechanical properties also differ due to the placental region from which the hAM originates, since placental hAM was shown to be significantly stronger and more stretchable than their peripheral counterparts (78).
While the structure of the membrane is mostly preserved by the various processes, the effects on the protein level are more significant. Although there are already major interindividual differences in the amount and occurrence of individual factors isolated from hAM from different donor placentas (79, 80), the preservation techniques additionally change their concentration significantly (35, 53, 81, 82). Again, freezing processes do not have as much effect overall as drying or lyophilization methods (42, 83). However, optimization of the drying process using lyoprotective agents such as trehalose or raffinose can reduce the changes (52). It must be mentioned that different factors were examined in each of the various studies. Together with the circumstance of the above mentioned interindividual differences and additionally the different processing methods, consequently the results are not directly comparable. The largely consistent statement in literature is that the losses of the various factors investigated are lower in freezing than in drying processes or in glycerol. It is also uniformly described that final sterilization steps, especially by high irradiation doses, but also when PAA is used, have a negative effect on the respective proteins/cytokines under consideration (51). A combination of the procedures further reduces the levels (53). Moreover, it can be assumed from the individual studies that the diverse growth factors and cytokines are differently susceptible to the influences of the various methods due to the individual sensitivity of proteins to external conditions such as temperature and hydration status. For example, while TGF-ß levels seem to be relatively unaffected by the different treatment methods, EGF concentration was found to be significantly reduced, especially by irradiation (53).
The most drastic changes after preparation and preservation procedures are found in the presence of living cells in the final product. For all those techniques that are suboptimal for cell survival it cannot be assumed that viable cells are present to a significant amount following the procedure. This is especially true for the uncontrolled freezing procedures without CPA and the drying methods (84). For glycerol storage, it has also been shown that viability of cells is not preserved (58, 81).
In contrast with respect to the viability of the existing cells an optimized cryopreservation strategy with CPA and adjusted freezing rates is preferable. Up to 82% viable cells could be achieved (85). Differences occur in the final storage temperature where the highest survival rate was achieved at −196°C by Hettiarachchi et al. (45) but only 13%–18% reported by Hennerbichler et al. (58) with this procedure. Storage at −80°C, on the other hand, already considerably reduces the proportion of vital cells with increasing losses over storage time, as recrystallization processes continue to take place at this temperature, which affect the integrity of the cells. Conversely, the preservation of vital cells also has an influence on the factor content, since living cells continue to produce proteins. For example, the preparation method affects the angiogenic factor (AF) profile via the cell vitality (81). Nevertheless, the relevance of vital cells for clinical outcome has not yet been clarified (86). The multitude of published positive healing results achieved with material in which no living cells were present show that, as a rule, the vitality of the cells does not play a decisive role. Adds et al. (26) showed that application of fresh membrane, presumably with vital cells, did not achieve re-epithelialization of the cornea better than cryopreserved hAM. A similar conclusion was reached by Fenelon et al. (67), who found no difference between cryopreserved and fresh hAM for guided bone regeneration. In fact, the few adverse reactions described occurred when fresh membrane was used (32, 33), so it could be suggested that viable cells may not be advantageous for the most commonly used applications at present.
As described before, the highest priority must be given to the safety of the application to the treated patient. To achieve a sterile product, various sterilization processes are used.
Numerous preparation methods apply an antibiosis step to avoid contamination of the hAM with microorganisms. For rinsing with antimicrobial substances mostly streptomycin/penicillin mixtures in combination with the antimycotic amphotericin B are used (73). Recently, some antibiotics like gentamicin and ciprofloxacin have been shown to cause changes in the ultrastructure of the membrane (87) even though these do not seem to have any effect on clinical efficiency. However, rinsing will always affect the soluble protein content. It can therefore be expected that the duration of antibiosis will also reduce the quantity of proteins and thus alter the factor profile of the hAM.
It is agreed that the use of antimicrobial substances cannot be considered a terminal sterilization measure. Gamma irradiation or by electron-beam is most commonly used for this purpose. However, it is consistently reported that this type of treatment exerts the strongest influence on the properties of the amniotic membrane. This includes both the structure and the biomechanical characteristics associated with it. In addition, the proteins present in the membrane are significantly affected. Living cells cannot be detected after these procedures (88). In addition, the changes triggered by the irradiation add up to those already caused by the preparation and/or preservation process. However, it is described that the original antimicrobial properties of hAM (89) are maintained even after higher doses of gamma irradiation (90). The same authors describe a change in pH after irradiation, which could be particularly significant for the treatment of chronic wounds (91, 92). Nevertheless, the result is a guaranteed sterile product. This may be favorable especially for the use in open wounds (90).
In any case and independent of the way of processing the hAM will be subjected to a final product control for sterility before it can be released for use.
4. Currently predominantly used application areas for hAM
In this section the most prominent indications for hAM utilization are presented. Due to its special properties, new applications for hAM are constantly being developed, although the focus is increasingly shifting to the cells that can be isolated from birth associated tissues like amniotic membrane or umbilical cord (93).
4.1. Ophthalmology
Since 1940, when de Rötth (94) described the first amniotic membrane transplantation (AMT) to cover conjunctival defects, this therapy has become a routine procedure in ophthalmology. Thus, these application fields are described comprehensively:
• ocular surface disturbances caused by physical or chemical injuries, infections or systemic disorders may cause scarring of the conjunctiva or result in persistent ocular inflammation
• corneal ulceration with progressive thinning, descemetocoele and/or corneal perforation
• symptomatic bullous keratopathy, corneal disorders with associated limbal stem cell deficiency
• recurrence-free pterygium surgery
• other ocular surface diseases with large portions of bare sclera, such as dysplasia, tumors, scars and symblepharon
• corneoscleral melts and perforations
• in glaucoma treatment to reduce scarring in filtering surgery
[for detailed review see among others (18, 72, 95, 94)].
Among this the reconstruction of the corneal surface is one of the most common aims for AMT. In the majority of published studies, the amniotic membrane is used as a patch and is fixed to the ocular surface so that the corneal epithelium can grow over it.
In the case of deeper injuries or ulcers, inlay techniques are also used, whereby one or more membranes are placed in the cavity and, if necessary, covered with another hAM as an overlay.
There is controversy about the necessary orientation of the membrane; however, the chorionic/stromal side is usually placed toward the surface. It is assumed that the hAM acts mainly as a guide rail and basement membrane. This would explain why those different preparation, storage, and sterilization procedures, which have a profound influence on the factor content but preserve the matrix structure seem to have no negative effect on the clinical efficacy for healing corneal surface defects. Many clinical data are available for this application, which were achieved with hAM prepared according to all conceivable procedures.
For a long time, fixation on the corneal surface was done via a suture. Thus, most applications were limited to the one-time coverage of a defect. More recently, constructs like PROKERA® (97) and AmnioClip-plus (98, 99) have become available which allow the suture-free placing of the membrane on the ocular surface. For the use of these systems, data on the successful treatment of pathologies like Steven-Johnson Syndrome, toxic epidermal necrolysis or dry eye syndrome among others is existing (100–102). Here the guide rail function does not seem to play a major role. It can be hypothesized that the factors stored in the membrane trigger the clinical effect. In both systems, a freeze-storage procedure is used, so that it can be assumed that sufficient cytokines and growth factors are preserved in their native effectiveness.
With the development of cell therapy, hAM can be also used as a carrier for ex vivo pre-culturing of limbal stem cells (LSC) for reverse transplantation in limbal stem cell deficiency (LSCD) (103–105). For this purpose, it seems to be sufficient or even advantageous if the membrane merely forms the extracellular matrix for the cells. Therefore, the products that are more intensely processed can also be used. Decellularized or deepithelialized (denuded) hAM, for example, have proved successful for such applications as well as for tissue engineering (62, 69) but the preparation method to favor is the subject of controversy (43, 65, 106–108).
4.2. Dermatology
The use of amniotic membrane in dermatology began as early as 1910 when AMT was described as a skin substitute by Davis (109). A little later, Sabella and Stern (110, 111) used hAM to treat burns. Since then, AMT has become firmly established in dermatology and the number of clinical reports has been growing steadily (112, 113).
In dermatology hAM is used to treat chronic wounds that do poorly or not respond to other treatments, e.g., chronic ulcers of the lower leg or diabetic foot. Impaired wound healing is particularly common in patients with diabetes and is associated with serious complications such as ulceration, infection, and gangrene. Diabetic foot ulcers (DFU) can lead to costly complications like hospitalization, amputation, and increased mortality. Standard treatments (Standard of Care, SOC) for DFU and other chronic wounds often need to be supplemented with additional therapies to stimulate healing of recalcitrant wounds. For this objective, the application of amniotic membrane has already proven its benefit in many cases (114–120). Although cryopreserved hAM has also been used successfully in this regard (85, 121), most reports refer to dehydrated material, for which the actual manufacturing process used is usually not described. However, meta-analysis showed (113, 122) that the dehydrated material achieved statistically significant better wound closure than SOC alone (70%–97% healing with dhACM within 4–12 weeks compared to 15%–32% with SOC). Therefore, similar as in ophthalmology, the provision of the tissue as a scaffold for the growth of the patient's own cells can be seen as one mechanism of action, since it seems, that all hAM products with a well-preserved structure like dehydrated membrane are suitable. These types of products are listed by the FDA as “wound covering” or “dressings” to distinguish them from active agents.
More recently, research has also investigated other mechanisms of action of hAM in healing of chronic wounds. As could be expected, it appears that various growth factors and signaling molecules also interfere with the healing mechanism (123, 124). In this regard, it could be advantageous to use hAM material in which the protein profile with the corresponding factors is preserved. This was demonstrated for all freezing procedures.
Human amniotic membrane, frozen at −20°C to −80°C or cryopreserved via controlled process and stored at −180°C has also been successfully used to heal fistulas of different etiology (125–128). Because inflammatory processes play a major role in the persistence of fistulas (129), immunomodulatory properties of the hAM (130) seem to contribute to the modification of the inflammatory condition so that wound healing becomes possible (131).
Since immunomodulatory properties are primarily mediated by factors such as interleukin-10 (IL-10), transforming growth factor-b (TGF-b), hepatocyte growth factor (HGF), or prostaglandin E2 (PGE2), the success in the use of cryopreserved membrane may be due to the fact that freezing procedures sufficiently conserve these factors compared to dehydration (51).
The use of hAM has also been proven in the treatment of burns. It has been useful as a temporary epidermal substitute for covering wounds in the treatment of second-degree burns, and especially in children (49, 132, 133). Pain reduction, early wound drying and significantly accelerated wound healing with epithelialization are described as particular advantages (134–137). Additional positive effects seem to be a reduction of microorganisms, which is especially important in burns, as well as the promotion of neoangiogenesis (138). In addition to its use as a temporary skin substitute, the amniotic membrane is useable as a wound dressing for split-thickness skin-graft donor sites. Here the benefits are the improvement of the aesthetic result and the reduction of scarring. Basement membrane formation is accelerated, wound secretion is reduced and there is less itching, which increases patient satisfaction (139). The mechanism of action for these applications appears to be primarily based, again, on scaffold and basement membrane properties. Therapeutic success is described with all hAM products, regardless of how they were processed and sterilized (140–142). The antimicrobial property of particular interest for wound healing seems to be preserved by freezing as well as lyophilization (143).
In addition, a variety of approaches for this field of activity are described, in which hAM is used as a matrix for regenerative tissue engineering, including combined constructs (23, 41, 69).
4.3. Oral and maxillofacial surgery
4.3.1. Amniotic membrane-assisted tissue regeneration in periodontal and oral surgery
Due to demographic change and the desire for a high quality of life into old age, dental health is increasingly becoming a focus of the population. In this context, dentistry is confronted with the widespread disease periodontitis and the associated long-term preservation of the natural chewing function. The reconstruction of the jawbone lost due to infection and the surrounding periodontium with its mucosa are fundamental measures for preserving the patient's own teeth. In the context of preparing an implant bed for reconstructions, the application of resorbable or non-resorbable membranes as a guide structure for functional tissue regeneration with simultaneous exclusion of inferior tissue is of central importance. Systematic reviews and meta-analyses have shown that guided tissue regeneration (GTR) procedures result in a significantly greater gain of attached gingiva than surgical procedures without the use of membranes (144, 145). At this point, hAM offers itself as an allogeneic biomaterial for the use of a natural GTR and guided bone regeneration (GBR) membrane. Its antimicrobial properties as well as its lack of immunogenicity offer infection prophylaxis in a physiologically bacterially contaminated surgical site (7, 146, 147). Furthermore, it fulfills important requirements for use as a barrier membrane due to its high tensile stability and anti-adhesive effect (148, 149). Previous studies on the use of hAM in periodontal and oral surgery confirm these results (150–153).
In hAM transplantation for GBR, not only a barrier function but also an induction of bone growth and regeneration could be demonstrated in both animal models and patients (154, 155). Furthermore, in a randomized controlled trial, hAM was shown to be equivalent to collagen membrane in GTR in covering gingival recessions (156). A reduction in periodontitis-induced gingival pockets and coverage of dental recessions were also successfully confirmed (157). Studies show excellent results for the use of hAM in the context of preprosthetic surgical procedures such as vestibuloplasty (158). The suitability as an intraoral wound dressing after local tissue excisions confirms the wide range of applications of hAM in periodontal and oral surgery (159, 160). Due to its intrinsic material properties, hAM represents an attractive alternative to established biomaterials and is able to demonstrate an alternative to 3rd generation drug- and growth factor-coated membranes [Kesting et al. (161), Review].
Other therapeutic options in this area include the management of medication-related osteonecrosis of the jaw (162–164) and mucosal defects, root coverage of gingival recession, and oronasal fistulae management (86).
4.3.2. Adhesion prophylaxis in orbital fracture reconstruction
The human orbit has a volume of about 30 cm3, of which about a quarter is occupied by the bulb (165). It is formed by seven bones and has a bony thickness of only 0.3 mm in the area of the lamina papyracea. Frequently, orbital involvement occurs in the context of other midface fractures (>40%) (166). The clinical symptoms of an orbital fracture are manifold. An absolute emergency is the retrobulbar hematoma with the risk of permanent blindness. Surgical reconstruction of orbital fractures within the first 48 h after trauma significantly reduces the likelihood of persistent symptoms (167, 168). The goal of surgical orbital reconstruction is the unrestricted restoration of ocular function. The rate of persistent postoperative discomfort varies significantly in the literature from 0.53%–81% and regularly requires corrective surgery (169, 170). Known reasons for a limited postoperative result are on the one hand the fracture size, but also the choice of reconstruction material. In particular, the use of titanium implants and resorbable PDS®1 sheets is controversial regarding the induction of scarring adhesions.
The use of hAM is considered an innovative procedure for the therapy of orbital adhesions and strabismus (OAS) correction (171, 172) and has shown significant success both in animal experiments and in patients for adhesion prophylaxis of secondary motility disorders (149, 173, 174). Other properties such as pain modulating, angiogenic and anti-inflammatory effects make hAM an extremely promising biomaterial in anti-adhesive and reconstructive orbital surgery. The use of hAM can therefore also be helpful when using orbital implants and ocular prostheses after enucleation (175).
In view of the established use of the amniotic membrane in ophthalmology, a permanent extension of the indication of the amniotic membrane into the field of oral and maxillofacial surgery with a focus on the prophylaxis of the OAS after orbital fractures should be considered.
4.4. Gynecology
There are two main fields in gynecological applications of hAM: Asherman syndrome (intrauterine adhesions, IUA) and vaginoplasties. Intrauterine adhesions (IUAs) are a benign uterine disorder that results in intrauterine adhesions and scarring. The principle for the application of hAM to IUA is the use of a biologically active mechanical separator after hysteroscopic adhesiolysis (176). The reports show that fresh hAM graft improved the clinical outcome and reduced the recurrence of adhesion reformation (29, 177, 178) while meta-analyses of studies showed that dried or freeze-dried hAM increased menstrual blood volume but failed to improve the rates of intrauterine adhesion recurrence, pregnancy or spontaneous abortion (179–181).
Recent efforts are directed toward the use of human amniotic mesenchymal stromal cells for the management of IUAs by promoting endometrial regeneration and repair (182). The results of this investigation suggest that the divergent results of the above-mentioned studies are due to the use of differently pretreated products. For a successful treatment of IUAs with reduced recurrence rates active cells seem to be advantageous. Therefore, such membranes would be recommended for this application, which support presence of vital cells, e.g., via a controlled cryopreservation procedure.
Further, in various techniques of vaginoplasty, the use of hAM can be considered a safe and simple procedure with good functional results (183). The successful application of hAM in this field was already described in 1979 (184). Since then, various other groups have used this material with good clinical outcome (185–192). As both fresh and chemically processed and sterilized freeze-dried hAM were used, it can be assumed that the results are again based on the matrix effect of the membrane.
4.5. Urology
Every year, thousands of surgical procedures are performed to replace or repair ureters, urinary bladders or urethrae that are damaged through disease or trauma. In principle, the hAM is also suitable for this purpose (193), but it has been shown that the biomechanical properties of the thin membrane might not be sufficient for this intention. Therefore, the approaches in this area are focused on the use of composite material with chorion or other adjuvants (194–196), which shows promising results. An overview for such regenerative approaches is given by Nejad et al. (197).
Human amniotic membrane can improve tissue regeneration and functional outcome after radical prostatectomy (RP) due to the growth factors and unique immune tolerance. Preliminary studies showed the potential value of hAM in the reconstruction of the urinary tract and nerve protection during RP (198). Their results showed that dehydrated hAM could be considered as a suitable scaffold for faster improving vesicoureteral anastomosis (VUA) healing. Another clinical study was developed in 2020 by Barski et al. (199) to evaluate the efficacy and safety of hAM placed around the neurovascular bundle during RP for the treatment of the localized prostate cancer.
These applications are closely related to the use of hAM in Neurology below.
4.6. Neurology and neurosurgery
Multiple investigations—most of them, however, still in animal models—have highlighted hAMs role in preventing recurrence of perineural adhesions, reducing fibrosis, accelerating nerve repair, and improving nerve function. Thus, the amniotic membrane has ideal properties for treating peripheral nerve injuries (28, 41, 200). The authors conclude that the best would be a freeze-dried tissue containing the amnion and chorion layers in order to preserve all its growth factors and facilitate its handling and storage in the operating room. Another approach in this direction is to support the natural properties of the amniotic membrane by electrospun polycaprolactone (PCL) fibers (201). In particular for neurosurgery, several studies have shown its value for dural repair in both cranial and spine surgeries (202).
4.7. Orthopedics
Human amniotic membrane has been evaluated for the repair of tendon and ligament, attenuation of cartilage and joint space diseases, prevention of scarring and adhesion formation in spinal fusion procedures. It can prevent tendon adhesions after injury and reconstruction (41, 203). Products from all different processing methods are used with equal success. Furthermore, hAM as an injectable material is utilized to improve healing or manage pain in osteoarthritis (204, 205). For example, decellularized and dehydrated human amniotic/chorionic membrane is micronized and injected for knee osteoarthritis, chronic tendinosis or arthropathy (206–211). However, the particle size seems to play a role (212). For cartilage repair unchanged hypothermically stored membrane was used. The success of the treatment seems to depend on living cells in the product (213). Approaches that use cells or exosomes/extracellular vesicles (EVs) derived from them support that interpretation (21, 197, 214).
Over the last 20 years, there has been increasing interest in bone regeneration using hAM. Numerous reports show that hAM has a positive effect on bone healing as already mentioned in the oral surgery part. However, there is no consensus regarding the optimal usage strategies (215) as all kinds of preparation methods are used. Direct comparison between fresh, frozen, and decellularized freeze-dried membrane showed that decellularized and lyophilized hAM performed significantly better (67). This result would suggest that bone regeneration requires mainly the matrix of the membrane, rather than the content of growth factors. In contrast, studies are also known in which cells isolated from the amniotic membrane (amniotic-derived epithelial cells (AECs), and amniotic mesenchymal stromal cells (AMSCs)) associated with an appropriate scaffold seem to be ideal candidates for tissue engineering strategies applied to bone healing (41, 216).
4.8. Oncology
Human amniotic membrane has also generated increasing interest for applications in oncology (217, 218). Pro-apoptotic, anti-angiogenic as well as cell-cycle arrest, and immune-regulatory properties (219, 220) make the material a promising candidate for anticancer therapy. One approach is using the membrane as a matrix to line a cavity formed after surgery to prevent tumor regrowth by acting as a physical barrier and providing anti-angiogenic properties (221–224). However, most reports refer to the use of the factors present in the membrane or the cells that produce them (225, 226). Therefore, also the use of conditioned medium for the therapy of breast cancer or hepatocarcimoma cells (227, 228), of hAM homogenate in a model of bladder cancer (229) or extracts (218, 225) is described. The mode of action in these cases could be based on exosomes or EVs. Cryopreservation does not alter the anti-cancer activity of hAM (230), but the mode of homogenization seems to have an impact (229).
However, all results, especially if cells like placental mesenchymal stroma cells (MSCs) are used, must be considered with caution, as the opposite effects have also been described. For example, angiogenic properties may influence regrowth of tumors (130, 231–233). Since angiogenic factors appear to be reduced by freezing processes (81) it could therefore be useful to apply such processing methods for this purpose in order to make greater use of the anti-carcinogenic properties of hAM while avoiding opposite effects.
5. Further preparation types and application fields
The applications of human amniotic membrane are constantly expanding, so that further reports can be found, e.g., on the implantation of hAM on pancreatic anastomosis after pancreaticoduodenectomy (234), for palatal epithelial-connective tissue reconstruction (235) or for the treatment of open myelomeningocele (MMC) and lipomeningocele (LMC) (236). The hAM is used to improve the post-tonsillectomy recovery by reducing post-operative pain and bleeding and promoting the wound healing process (237) and in airway reconstruction following chondrosarcoma resection (238). Another approach is the treatment of liver fibrosis. For this purpose, the anti-fibrotic properties of hAM are useful, which were retained even after freezing (47).
As mentioned above for the individual application areas, in recent times newer application focus on more manipulated derivatives from hAM like extracts, homogenates or exosomes/EVs (239). Moreover, the membrane is used for tissue engineering purposes, i.e., combined with other materials such as hydrogel or fibers of different origin (23, 69, 240). For these purposes, often decellularized material is used, which can be produced by means of different techniques (43). A newer study investigates the effect of an injectable hydrogel generated from a decellularized amniotic membrane (dhAM-gel) on preventing the development of an intrauterine adhesion (241).
Some recent reports demonstrate the feasibility of producing vascular grafts by various techniques involving hAM for example by weaving yarn from the membrane or combine it with electrospun polycaprolactone (PCL)/silk fibroin (SF) (245, 246, 244). For this purpose, decellularized material is used, as the structural components are important.
To prepare an extract from human amniotic membrane (hAME), the membrane is usually obtained and purified as described above. The clean fresh, cryopreserved or dried hAM is then grounded generally using cryogenic temperature (LN2). The micronization can be done via mortars or e.g., cryo-mills, followed by an extraction, e.g., with physiological solution. After centrifugation, supernatants may be sterilized by filtering through a 0.2-μm pore size membrane (245, 246). Studies have shown that hAME used as eye drops (AMEED), supports proliferation and differentiation of corneal epithelial cells, enhances epithelial wound healing, and inhibits corneal neovascularization by supporting in vivo cultivation of limbal stem cells (244–249) whereby the extract is as efficient as AMT (250). Promising results are achieved for treatment of dry eye syndrome and Graft-versus-Host disease (251–253). The hAME can provide a new therapeutic strategy to modulate the bone density or calcification during bone regeneration by modifying osteogenic efficacy (254).
Alternatively, homogenates are used which in principle are prepared in the same way as extracts but without a centrifugation step. No sterile filtration step can be performed here, so sterility must be achieved prior to the processing by antibiotic treatment or by irradiation.
Homogenates of fresh and cryopreserved hAM show a strong antimicrobial effect, but it is important to choose the right storage condition to preserve this activity (224).
By processing into extracts or homogenates, the matrix structure is no longer preserved. However, the various process steps also have an influence on the product. For example, it has been demonstrated that the type of pretreating (frozen or freeze-dried) or grinding (pulverization vs. homogenization) changes the factor content (245, 255). Furthermore, the protein content seems lower in suspension/extracts in contrast to homogenates (256, 257) and could undergo an additional reduction by irradiation, but the remaining amount seems sufficient to achieve the desired healing effect, i.e., on human corneal epithelial cells (258, 259).
Injectable hAM particulates are successfully used for Osteoarthritis (260, 261) and the detrimental effect of homogenate on bladder cancer cells shows a promising option in oncologic therapy (229).
6. Discussion
For more than 100 years, birth-associated tissues such as human amniotic membrane have been used for clinical purposes. Despite early healing successes, the application could not initially find its way into routine use. It was only with the introduction of newer preparation and storage methods that guarantee a safe product that amniotic membrane transplantation became established in clinical practice in many medical disciplines. The aim of the present work is to summarize the most common processing and storage methods and applications currently in use, and to link these to the preferred properties of hAM.
Following our expectation, the literature has shown that all processes have an impact on the qualities of the finally used membrane. However, the described procedures and their influence on the properties of hAM are difficult to compare because on the one hand, the research groups used different methods for firstly the processing and preservation, secondly the factor/protein isolation and analysis and thirdly for evaluation and presentation of the results. Additionally, no uniform nomenclature is used: authors use identical terms for different procedures. In addition, the complete procedure is not always described. For example, it is usually left open whether fresh-frozen process without CPA is carried out in medium or liquid (e.g., buffer or salt solution) or dry. The term cryopreservation is used by most authors to describe all freezing procedures, independent of the freezing process performed, the added media/CPA used and the final storage temperature. The described changes in hAM properties due to the processing and preservation procedures can therefore not be assessed reliably or coherently. For example, Allen et al. (52) conclude that the optimized lyophilization process they used causes less damage to the tissue than cryopreservation. However, the process they describe for cryopreservation is an uncontrolled freezing in saline solution without protective measures, so any changes found as a result cannot be unexpected.
Similarly, for liquid derivatives of hAM, the terms extract and homogenate are used interchangeably, although the preparation of the two formulations is different. This also applies to suspension and conditioned medium.
The terms viability and vitality are also not used uniformly. Some authors seem to use it to refer to the remaining activity in the sense of the effectiveness of the hAM rather than referring to living cells. At the same time, studies show that vital cells do not necessarily need to be present for effectiveness of the treatment (86) unless a high factor content is essential, as living cells continue to produce proteins after transplantation (85).
In summary, all procedures presented in this review have influence on the properties and hence the possible way of usage of the amniotic membrane. Prior to a clinical application it should be carefully evaluated which properties of hAM are needed, such as matrix or guide rail functions, or factor release and immunomodulating activities by cells, respectively.
The huge number of publications shows that amniotic membrane has proven its effect and benefit in various fields of clinical applications, making it an essential treatment option for patient care.
Author contributions
NH, AS and MB designed the study and wrote the manuscript. H-OR revised all versions of the manuscript. All authors contributed to the article and approved the submitted version.
Acknowledgments
The authors thank F. Gindraux, F.J. Nicolás and X. Lafarge (members of COST action CA17116) for encouragement and fruitful discussion. This work contributes to the COST Action CA17116 International Network for Translating Research on Perinatal Derivatives into Therapeutic Approaches (SPRINT), supported by COST (European Cooperation in Science and Technology).
Conflict of interest
The authors declare that the research was conducted in the absence of any commercial or financial relationships that could be construed as a potential conflict of interest.
Publisher's note
All claims expressed in this article are solely those of the authors and do not necessarily represent those of their affiliated organizations, or those of the publisher, the editors and the reviewers. Any product that may be evaluated in this article, or claim that may be made by its manufacturer, is not guaranteed or endorsed by the publisher.
Footnote
1PDS® sheets consist of resorbable polydioxanone, which is completely replaced by the patient's own body tissue after 180 days.
References
1. Pogozhykh O, Hofmann N, Gryshkov O, von Kaisenberg C, Mueller M, Glasmacher B, et al. Repeated freezing procedures preserve structural and functional properties of amniotic membrane for application in ophthalmology. Int J Mol Sci. (2020) 21:4029. doi: 10.3390/ijms21114029
2. Tombran-Tink J. The neuroprotective and angiogenesis inhibitory serpin, PEDF: new insights into phylogeny, function, and signaling. Front Biosci. (2005) 10:2131. doi: 10.2741/1686
3. Banas RA, Trumpower C, Bentlejewski C, Marshall V, Sing G, Zeevi A. Immunogenicity and immunomodulatory effects of amnion-derived multipotent progenitor cells. Hum Immunol. (2008) 69:321–8. doi: 10.1016/j.humimm.2008.04.007
4. Liu Q-W, Huang Q-M, Wu H-Y, Zuo G-S-L, Gu H-C, Deng K-Y, et al. Characteristics and therapeutic potential of human amnion-derived stem cells. Int J Mol Sci. (2021) 22:970. doi: 10.3390/ijms22020970
5. Insausti CL, Blanquer M, Bleda P, Iniesta P, Majado MJ, Castellanos G, et al. The amniotic membrane as a source of stem cells. Histol Histopathol. (2010) 25:91–8. doi: 10.14670/HH-25.91
6. Miki T. A rational strategy for the use of amniotic epithelial stem cell therapy for liver diseases. Stem Cells Transl Med. (2016) 5:405–9. doi: 10.5966/sctm.2015-0304
7. Akle CA, Adinolfi M, Welsh KI, Leibowitz S, McColl I. Immunogenicity of human amniotic epithelial cells after transplantation into volunteers. Lancet. (1981) 2:1003–5. doi: 10.1016/S0140-6736(81)91212-5
8. Adinolfi M, Akle CA, McColl I, Fensom AH, Tansley L, Connolly P, et al. Expression of HLA antigens, beta 2-microglobulin and enzymes by human amniotic epithelial cells. Nature. (1982) 295:325–7. doi: 10.1038/295325a0
9. Miki T, Strom SC. Amnion-derived pluripotent/multipotent stem cells. Stem Cell Rev. (2006) 2:133–42. doi: 10.1007/s12015-006-0020-0
10. Kubo M, Sonoda Y, Muramatsu R, Usui M. Immunogenicity of human amniotic membrane in experimental Xenotransplantation. Invest Ophthalmol Vis Sci. (2001) 42:1539–46.11381058
11. Hunt JS, Fishback JL. Amniochorion: immunologic aspects–a review. Am J Reprod Immunol. (1989) 21:114–8. doi: 10.1111/j.1600-0897.1989.tb01014.x
12. Robson MC, Krizek TJ. The effect of human amniotic membranes on the bacteria population of infected rat burns. Ann Surg. (1973) 177:144–9. doi: 10.1097/00000658-197302000-00003
13. Hao Y, Ma DH, Hwang DG, Kim WS, Zhang F. Identification of antiangiogenic and antiinflammatory proteins in human amniotic membrane. Cornea. (2000) 19:348–52. doi: 10.1097/00003226-200005000-00018
14. Solomon A, Rosenblatt M, Monroy D, Ji Z, Pflugfelder S, Tseng S. Suppression of interleukin 1α and interleukin 1β in human limbal epithelial cells cultured on the amniotic membrane stromal matrix. Br J Ophthalmol. (2001) 85:444–9. doi: 10.1136/bjo.85.4.444
15. Shimmura S, Shimazaki J, Ohashi Y, Tsubota K. Antiinflammatory effects of amniotic membrane transplantation in ocular surface disorders. Cornea. (2001) 20:408–13. doi: 10.1097/00003226-200105000-00015
16. Shao C, Sima J, Zhang SX, Jin J, Reinach P, Wang Z, et al. Suppression of corneal neovascularization by PEDF release from human amniotic membranes. Invest Ophthalmol Vis Sci. (2004) 45:1758–62. doi: 10.1167/iovs.03-0882
17. Dua HS, Azuara-Blanco A. Amniotic membrane transplantation. Br J Ophthalmol. (1999) 83:748–52. doi: 10.1136/bjo.83.6.748
18. Kruse FE, Meller D. Amniotic membrane transplantation for reconstruction of the ocular surface. Ophthalmologe. (2001) 98:801–10. doi: 10.1007/s003470170055
19. Meller D, Pauklin M, Thomasen H, Westekemper H, Steuhl K-P. Amniotic membrane transplantation in the human eye. Dtsch Arztebl Int. (2011) 108:243–8. doi: 10.3238/arztebl.2011.0243
20. Koizumi NJ, Inatomi TJ, Sotozono CJ, Fullwood NJ, Quantock AJ, Kinoshita S. Growth factor mRNA and protein in preserved human amniotic membrane. Curr Eye Res. (2000) 20:173–7. doi: 10.1076/0271-3683(200003)2031-9FT173
21. Munoz-Torres JR, Martínez-González SB, Lozano-Luján AD, Martínez-Vázquez MC, Velasco-Elizondo P, Garza-Veloz I, et al. Biological properties and surgical applications of the human amniotic membrane. Front Bioeng Biotechnol. (2023) 10:1–20. doi: 10.3389/fbioe.2022.1067480
22. Mamede AC, Carvalho MJ, Abrantes AM, Laranjo M, Maia CJ, Botelho MF. Amniotic membrane: from structure and functions to clinical applications. Cell Tissue Res. (2012) 349:447–58. doi: 10.1007/s00441-012-1424-6
23. Dadkhah Tehrani F, Firouzeh A, Shabani I, Shabani A. A review on modifications of amniotic membrane for biomedical applications. Front Bioeng Biotechnol. (2020) 8:606982. doi: 10.3389/fbioe.2020.606982
24. EDQM-Guide. Guide to the quality and safety of tissues and cells for human application. 5th ed. Strasbourg: European Directorate for the Quality of Medicines & HealthCare of the Council of Europe (EDQM) (2022).
25. Laranjo M. Preservation of amniotic membrane. In: Mamede AC, Botelho MF, editors. Amniotic Membrane Origin, Characterization and Medical Applications. Dordrecht: Springer Netherlands (2015). p. 209–30.
26. Adds PJ, Hunt CJ, Dart JK. Amniotic membrane grafts, “fresh” or frozen? A clinical and in vitro comparison. Br J Ophthalmol. (2001) 85:905–7. doi: 10.1136/bjo.85.8.905
27. Ganatra MA, Durrani KM. Method of obtaining and preparation of fresh human amniotic membrane for clinical use. J Pak Med Assoc. (1996) 46:126–8.8991368
28. Zhang Z-Y, Yang J, Fan Z-H, Wang D-L, Wang Y-Y, Zhang T, et al. Fresh human amniotic membrane effectively promotes the repair of injured common peroneal nerve. Neural Regen Res. (2019) 14:2199–208. doi: 10.4103/1673-5374.262596
29. Wu C, Dong Y, Li Y, Liu H. The efficacy of amniotic membrane-mediated sequential double-barrier therapy for the treatment of postoperative intrauterine adhesions. Medicine. (2021) 100:e25416. doi: 10.1097/MD.0000000000025416
30. Farghali HA, AbdElKader NA, Khattab MS, AbuBakr HO. Novel approach to gastric mucosal defect repair using fresh amniotic membrane allograft in dogs (experimental study). Stem Cell Res Ther. (2017) 8:235. doi: 10.1186/s13287-017-0682-3
31. Gündüz K, Uçakhan OO, Kanpolat A, Günalp I. Nonpreserved human amniotic membrane transplantation for conjunctival reconstruction after excision of extensive ocular surface neoplasia. Eye. (2006) 20:351–7. doi: 10.1038/sj.eye.6701890
32. Wang M, Yoshida A, Kawashima H, Ishizaki M, Takahashi H, Hori J. Immunogenicity and antigenicity of allogeneic amniotic epithelial transplants grafted to the cornea, conjunctiva, and anterior chamber. Invest Ophthalmol Vis Sci. (2006) 47:1522–32. doi: 10.1167/iovs.05-0787
33. Gholipourmalekabadi M, Farhadihosseinabadi B, Faraji M, Nourani MR. How preparation and preservation procedures affect the properties of amniotic membrane? How safe are the procedures? Burns. (2020) 46:1254–71. doi: 10.1016/j.burns.2019.07.005
34. Tighe S, Mead OG, Lee A, Tseng SCG. Basic science review of birth tissue uses in ophthalmology. Taiwan J Ophthalmol. (2020) 10:3–12. doi: 10.4103/tjo.tjo_4_20
35. McQuilling JP, Vines JB, Kimmerling KA, Mowry KC. Proteomic comparison of amnion and chorion and evaluation of the effects of processing on placental membranes. Wounds. (2017) 29:E36–40.28682294
36. Wagner M, Walter P, Salla S, Johnen S, Plange N, Rütten S, et al. Cryopreservation of amniotic membrane with and without glycerol additive. Graefes Arch Clin Exp Ophthalmol. (2018) 256:1117–26. doi: 10.1007/s00417-018-3973-1
37. von Versen-Hoeynck F, Steinfeld AP, Becker J, Hermel M, Rath W, Hesselbarth U. Sterilization and preservation influence the biophysical properties of human amnion grafts. Biologicals. (2008) 36:248–55. doi: 10.1016/j.biologicals.2008.02.001
38. Lamon M, Bertolin M, Trojan D, Spagnol L, Donisi PM, Camposampiero D, et al. Cryopreservation of human amniotic membrane for ocular surface reconstruction: a comparison between protocols. Cell Tissue Bank. (2022) 23:851–61. doi: 10.1007/s10561-022-10002-y
39. Von Versen-Höynck F, Syring C, Bachmann S, Möller DE. The influence of different preservation and sterilisation steps on the histological properties of amnion allografts–light and scanning electron microscopic studies. Cell Tissue Bank. (2004) 5:45–56. doi: 10.1023/B:CATB.0000022276.47180.96
40. Mermet I, Pottier N, Sainthillier JM, Malugani C, Cairey-Remonnay S, Maddens S, et al. Use of amniotic membrane transplantation in the treatment of venous leg ulcers. Wound Repair Regen. (2007) 15:459–64. doi: 10.1111/j.1524-475X.2007.00252.x
41. Fénelon M, Catros S, Meyer C, Fricain J-C, Obert L, Auber F, et al. Applications of human amniotic membrane for tissue engineering. Membranes. (2021) 11:387. doi: 10.3390/membranes11060387
42. Rodríguez-Ares MT, López-Valladares MJ, Touriño R, Vieites B, Gude F, Silva MT, et al. Effects of lyophilization on human amniotic membrane. Acta Ophthalmol. (2009) 87:396–403. doi: 10.1111/j.1755-3768.2008.01261.x
43. Riau AK, Beuerman RW, Lim LS, Mehta JS. Preservation, sterilization and de-epithelialization of human amniotic membrane for use in ocular surface reconstruction. Biomaterials. (2010) 31:216–25. doi: 10.1016/j.biomaterials.2009.09.034
44. Witt J, Grumm L, Salla S, Geerling G, Menzel-Severing J. Cryopreservation in a standard freezer: -28°C as alternative storage temperature for amniotic membrane transplantation. J Clin Med. (2022) 11:1109. doi: 10.3390/jcm11041109
45. Hettiarachchi D, Dissanayake VHW, Goonasekera HWW. Optimizing amniotic membrane tissue banking protocols for ophthalmic use. Cell Tissue Bank. (2016) 17:387–97. doi: 10.1007/s10561-016-9568-3
46. Rama P, Giannini R, Bruni A, Gatto C, Tiso R, Ponzin D. Further evaluation of amniotic membrane banking for transplantation in ocular surface diseases. Cell Tissue Bank. (2001) 2:155–63. doi: 10.1023/A:1020158206073
47. Ricci E, Vanosi G, Lindenmair A, Hennerbichler S, Peterbauer-Scherb A, Wolbank S, et al. Anti-fibrotic effects of fresh and cryopreserved human amniotic membrane in a rat liver fibrosis model. Cell Tissue Bank. (2013) 14:475–88. doi: 10.1007/s10561-012-9337-x
48. Díaz-Prado S, Rendal-Vázquez ME, Muiños-López E, Hermida-Gómez T, Rodríguez-Cabarcos M, Fuentes-Boquete I, et al. Potential use of the human amniotic membrane as a scaffold in human articular cartilage repair. Cell Tissue Bank. (2010) 11:183–95. doi: 10.1007/s10561-009-9144-1
49. Mostaque AK, Rahman KBMA. Comparisons of the effects of biological membrane (amnion) and silver sulfadiazine in the management of burn wounds in children. J Burn Care Res. (2011) 32:200–9. doi: 10.1097/BCR.0b013e31820aad94
50. Singh R, Chacharkar MP. Dried gamma-irradiated amniotic membrane as dressing in burn wound care. J Tissue Viability. (2011) 20:49–54. doi: 10.1016/j.jtv.2010.06.001
51. Thomasen H, Pauklin M, Steuhl K-P, Meller D. Comparison of cryopreserved and air-dried human amniotic membrane for ophthalmologic applications. Graefes Arch Clin Exp Ophthalmol. (2009) 247:1691–700. doi: 10.1007/s00417-009-1162-y
52. Allen CL, Clare G, Stewart EA, Branch MJ, McIntosh OD, Dadhwal M, et al. Augmented dried versus cryopreserved amniotic membrane as an ocular surface dressing. PLoS One. (2013) 8:1–15. doi: 10.1371/journal.pone.0078441
53. Paolin A, Trojan D, Leonardi A, Mellone S, Volpe A, Orlandi A, et al. Cytokine expression and ultrastructural alterations in fresh-frozen, freeze-dried and γ-irradiated human amniotic membranes. Cell Tissue Bank. (2016) 17:399–406. doi: 10.1007/s10561-016-9553-x
54. Nakamura T, Yoshitani M, Rigby H, Fullwood NJ, Ito W, Inatomi T, et al. Sterilized, freeze-dried amniotic membrane: a useful substrate for ocular surface reconstruction. Invest Ophthalmol Vis Sci. (2004) 45:93–9. doi: 10.1167/iovs.03-0752
55. Nakamura T, Inatomi T, Sekiyama E, Ang LPK, Yokoi N, Kinoshita S. Novel clinical application of sterilized, freeze-dried amniotic membrane to treat patients with pterygium. Acta Ophthalmol Scand. (2006) 84:401–5. doi: 10.1111/j.1600-0420.2006.00667.x
56. Nakamura T, Sekiyama E, Takaoka M, Bentley AJ, Yokoi N, Fullwood NJ, et al. The use of trehalose-treated freeze-dried amniotic membrane for ocular surface reconstruction. Biomaterials. (2008) 29:3729–37. doi: 10.1016/j.biomaterials.2008.05.023
57. Kim JC, Tseng SC. Transplantation of preserved human amniotic membrane for surface reconstruction in severely damaged rabbit corneas. Cornea. (1995) 14:473–84.8536460
58. Hennerbichler S, Reichl B, Pleiner D, Gabriel C, Eibl J, Redl H. The influence of various storage conditions on cell viability in amniotic membrane. Cell Tissue Bank. (2007) 8:1–8. doi: 10.1007/s10561-006-9002-3
59. Maral T, Borman H, Arslan H, Demirhan B, Akinbingol G, Haberal M. Effectiveness of human amnion preserved long-term in glycerol as a temporary biological dressing. Burns. (1999) 25:625–35. doi: 10.1016/s0305-4179(99)00072-8
60. Zidan SM, Eleowa SA, Nasef MA, Abd-Almoktader MA, Elbatawy AM, Borhamy AG, et al. Maximizing the safety of glycerol preserved human amniotic membrane as a biological dressing. Burns. (2015) 41:1498–503. doi: 10.1016/j.burns.2015.03.009
61. Ab Hamid SS, Zahari NK, Yusof N, Hassan A. Scanning electron microscopic assessment on surface morphology of preserved human amniotic membrane after gamma sterilisation. Cell Tissue Bank. (2014) 15:15–24. doi: 10.1007/s10561-012-9353-x
62. Figueiredo GS, Bojic S, Rooney P, Wilshaw S-P, Connon CJ, Gouveia RM, et al. Gamma-irradiated human amniotic membrane decellularised with sodium dodecyl sulfate is a more efficient substrate for the ex vivo expansion of limbal stem cells. Acta Biomater. (2017) 61:124–33. doi: 10.1016/j.actbio.2017.07.041
63. Trosan P, Smeringaiova I, Brejchova K, Bednar J, Benada O, Kofronova O, et al. The enzymatic de-epithelialization technique determines denuded amniotic membrane integrity and viability of harvested epithelial cells. PLoS One. (2018) 13:e0194820. doi: 10.1371/journal.pone.0194820
64. Lakkireddy C, Vishwakarma SK, Raju N, Ahmed SI, Bardia A, Khan MA, et al. Fabrication of decellularized amnion and chorion scaffolds to develop bioengineered cell-laden constructs. Cell Mol Bioeng. (2021) 15:137–50. doi: 10.1007/s12195-021-00707-7
65. Shortt AJ, Secker GA, Lomas RJ, Wilshaw SP, Kearney JN, Tuft SJ, et al. The effect of amniotic membrane preparation method on its ability to serve as a substrate for the ex-vivo expansion of limbal epithelial cells. Biomaterials. (2009) 30:1056–65. doi: 10.1016/j.biomaterials.2008.10.048
66. Wilshaw S-P, Kearney J, Fisher J, Ingham E. Biocompatibility and potential of acellular human amniotic membrane to support the attachment and proliferation of allogeneic cells. Tissue Eng Part A. (2008) 14:463–72. doi: 10.1089/tea.2007.0145
67. Fenelon M, Etchebarne M, Siadous R, Grémare A, Durand M, Sentilhes L, et al. Assessment of fresh and preserved amniotic membrane for guided bone regeneration in mice. J Biomed Mater Res A. (2020) 108:2044–56. doi: 10.1002/jbm.a.36964
68. Ashraf NN, Siyal NA, Sultan S, Adhi MI. Comparison of efficacy of storage of amniotic membrane at -20 and -80 degrees centigrade. J Coll Physicians Surg Pak. (2015) 25:264–7.25899191
69. Leal-Marin S, Kern T, Hofmann N, Pogozhykh O, Framme C, Börgel M, et al. Human amniotic membrane: a review on tissue engineering, application, and storage. J Biomed Mater Res B Appl Biomater. (2021) 109:1198–215. doi: 10.1002/jbm.b.34782
70. Wolkers WF, Oldenhof H. Principles underlying cryopreservation and freeze-drying of cells and tissues. Methods Mol Biol. (2021) 2180:3–25. doi: 10.1007/978-1-0716-0783-1_1
71. Paggiaro AO, Mathor MB, Teodoro WR, Isaac C, Capelozzi VL, Gemperli R. Evaluation of radiosterilized glyercerolated amniotic membranes as a substrate for cultured human epithelial cells. Organogenesis. (2020) 16:27–41. doi: 10.1080/15476278.2020.1723366
72. Dekaris I, Gabrić N. Preparation and preservation of amniotic membrane. Dev Ophthalmol. (2009) 43:97–104. doi: 10.1159/000223842
73. Jirsova K, Jones GLA. Amniotic membrane in ophthalmology: properties, preparation, storage and indications for grafting-a review. Cell Tissue Bank. (2017) 18:193–204. doi: 10.1007/s10561-017-9618-5
74. Tan EK, Cooke M, Mandrycky C, Mahabole M, He H, O'Connell J, et al. Structural and biological comparison of cryopreserved and fresh amniotic membrane tissues. J Biomater Tissue Eng. (2014) 4:379–88. doi: 10.1166/jbt.2014.1180
75. Cooke M, Tan EK, Mandrycky C, He H, O’Connell J, Tseng SCG. Comparison of cryopreserved amniotic membrane and umbilical cord tissue with dehydrated amniotic membrane/chorion tissue. J Wound Care. (2014) 23:465–74. doi: 10.12968/jowc.2014.23.10.465
76. Chuck RS, Graff JM, Bryant MR, Sweet PM. Biomechanical characterization of human amniotic membrane preparations for ocular surface reconstruction. Ophthalmic Res. (2004) 36:341–8. doi: 10.1159/000081637
77. Skardal A, Mack D, Atala A, Soker S. Substrate elasticity controls cell proliferation, surface marker expression and motile phenotype in amniotic fluid-derived stem cells. J Mech Behav Biomed Mater. (2013) 17:307–16. doi: 10.1016/j.jmbbm.2012.10.001
78. Grémare A, Jean-Gilles S, Musqui P, Magnan L, Torres Y, Fénelon M, et al. Cartography of the mechanical properties of the human amniotic membrane. J Mech Behav Biomed Mater. (2019) 99:18–26. doi: 10.1016/j.jmbbm.2019.07.007
79. Weidinger A, Poženel L, Wolbank S, Banerjee A. Sub-regional differences of the human amniotic membrane and their potential impact on tissue regeneration application. Front Bioeng Biotechnol. (2021) 8:613804. doi: 10.3389/fbioe.2020.613804
80. López-Valladares MJ, Teresa Rodríguez-Ares M, Touriño R, Gude F, Teresa Silva M, Couceiro J. Donor age and gestational age influence on growth factor levels in human amniotic membrane. Acta Ophthalmol. (2010) 88:e211–216. doi: 10.1111/j.1755-3768.2010.01908.x
81. Wolbank S, Hildner F, Redl H, van Griensven M, Gabriel C, Hennerbichler S. Impact of human amniotic membrane preparation on release of angiogenic factors. J Tissue Eng Regen Med. (2009) 3:651–4. doi: 10.1002/term.207
82. Hopkinson A, McIntosh RS, Shanmuganathan V, Tighe PJ, Dua HS. Proteomic analysis of amniotic membrane prepared for human transplantation: characterization of proteins and clinical implications. J Proteome Res. (2006) 5:2226–35. doi: 10.1021/pr050425q
83. Kim TG, Do Ki K, Lee M-K, So J-W, Chung SK, Kang J. Comparison of cytokine expression and ultrastructural alterations in fresh-frozen and dried electron beam-irradiated human amniotic membrane and chorion. Cell Tissue Bank. (2019) 20:163–72. doi: 10.1007/s10561-019-09748-9
84. Becktell L, Matuska AM, Hon S, Delco ML, Cole BJ, Begum L, et al. Proteomic analysis and cell viability of nine amnion, chorion, umbilical cord, and amniotic fluid-derived products. Cartilage. (2021) 13:495S–507S. doi: 10.1177/1947603520976767
85. Duan-Arnold Y, Gyurdieva A, Johnson A, Uveges TE, Jacobstein DA, Danilkovitch A. Retention of endogenous viable cells enhances the anti-inflammatory activity of cryopreserved amnion. Adv Wound Care. (2015) 4:523–33. doi: 10.1089/wound.2015.0636
86. Gaudet C, Solecki L, Mathéaud B, Odet S, Meyer C, Louvrier A, et al. Questions about residual cell viability in cryopreserved human amniotic membrane and its impact on clinical applications. Biomedicines. (2022) 10:2456. doi: 10.3390/biomedicines10102456
87. Cavalu S, Roiu G, Pop O, Heredea DAP, Costea TO, Costea CF. Nano-scale modifications of amniotic membrane induced by UV and antibiotic treatment: histological, AFM and FTIR spectroscopy evidence. Materials. (2021) 14:863. doi: 10.3390/ma14040863
88. Nemr W, Bashandy AS, Araby E, Khamiss O. Biological activity alterations of human amniotic membrane pre and post irradiation tissue banking. Pak J Biol Sci. (2016) 19:289–98. doi: 10.3923/pjbs.2016.289.298
89. Kjaergaard N, Hein M, Hyttel L, Helmig RB, Schønheyder HC, Uldbjerg N, et al. Antibacterial properties of human amnion and chorion in vitro. Eur J Obstet Gynecol Reprod Biol. (2001) 94:224–9. doi: 10.1016/s0301-2115(00)00345-6
90. Bashandy AS, Khalaf MA, AbdElAl MS, ALGhamry AA. Changes in the functional characteristics of amniotic membrane after gamma irradiation. IJAR. (2016) 4:1189–95. doi: 10.21474/IJAR01/368
91. Dissemond J, Witthoff M, Brauns TC, Haberer D, Goos M. PH values in chronic wounds. Evaluation during modern wound therapy. Hautarzt. (2003) 54:959–65. doi: 10.1007/s00105-003-0554-x
92. Dissemond J. (The importance of pH for wound healing) die bedeutung des pH-wertes für die wundheilung. HARTMANN WundForum. (2006) 1:15–9.
93. Gindraux F, Hofmann N, Agudo-Barriuso M, Antica M, Couto PS, Dubus M, et al. Perinatal derivatives application: identifying possibilities for clinical use. Front Bioeng Biotechnol. (2022) 10:977590. doi: 10.3389/fbioe.2022.977590
94. De Rötth A. Plastic repair of conjunctival defects with fetal membranes. Arch Ophthalmol. (1940) 119:522–5. doi: 10.1001/archopht.1940.00860130586006
95. Malhotra C, Jain AK. Human amniotic membrane transplantation: different modalities of its use in ophthalmology. World J Transplant. (2014) 4:111–21. doi: 10.5500/wjt.v4.i2.111
96. Costa E, Murta JN. Amniotic membrane in ophthalmology. In: Mamede AC, Botelho MF, editors. Amniotic membrane. Netherlands: Springer (2015). p. 105–22.
97. Pachigolla G, Prasher P, Di Pascuale MA, McCulley JP, McHenry JG, Mootha VV. Evaluation of the role of ProKera in the management of ocular surface and orbital disorders. Eye Contact Lens. (2009) 35:172–5. doi: 10.1097/ICL.0b013e3181a66a12
98. Kotomin I, Valtink M, Hofmann K, Frenzel A, Morawietz H, Werner C, et al. Sutureless fixation of amniotic membrane for therapy of ocular surface disorders. PLoS One. (2015) 10:e0125035. doi: 10.1371/journal.pone.0125035
99. Hofmann N, Salz A-K, Kleinhoff K, Möhle N, Börgel M, Diedenhofen N, et al. AmnioClip-Plus as sutureless alternative to amniotic membrane transplantation to improve healing of ocular surface disorders. Transplantology. (2021) 2:425–32. doi: 10.3390/transplantology2040040
100. Shay E, Khadem JJ, Tseng SCG. Efficacy and limitation of sutureless amniotic membrane transplantation for acute toxic epidermal necrolysis. Cornea. (2010) 29:359–61. doi: 10.1097/ICO.0b013e3181acf816
101. Cheng AMS, Zhao D, Chen R, Yin HY, Tighe S, Sheha H, et al. Accelerated restoration of ocular surface health in dry eye disease by self-retained cryopreserved amniotic membrane. Ocul Surf. (2016) 14:56–63. doi: 10.1016/j.jtos.2015.07.003
102. McDonald MB, Sheha H, Tighe S, Janik SB, Bowden FW, Chokshi AR, et al. Treatment outcomes in the DRy eye amniotic membrane (DREAM) study. Clin Ophthalmol. (2018) 12:677–81. doi: 10.2147/OPTH.S162203
103. Burman S, Tejwani S, Vemuganti GK, Gopinathan U, Sangwan VS. Ophthalmic applications of preserved human amniotic membrane: a review of current indications. Cell Tissue Bank. (2004) 5:161–75. doi: 10.1023/B:CATB.0000046067.25057.0a
104. Kethiri AR, Basu S, Shukla S, Sangwan VS, Singh V. Optimizing the role of limbal explant size and source in determining the outcomes of limbal transplantation: an in vitro study. PLoS One. (2017) 12:1–17. doi: 10.1371/journal.pone.0185623
105. Kate A, Basu S. A review of the diagnosis and treatment of limbal stem cell deficiency. Front Med. (2022) 9:836009. doi: 10.3389/fmed.2022.836009
106. Koizumi N, Rigby H, Fullwood NJ, Kawasaki S, Tanioka H, Koizumi K, et al. Comparison of intact and denuded amniotic membrane as a substrate for cell-suspension culture of human limbal epithelial cells. Graefes Arch Clin Exp Ophthalmol. (2007) 245:123–34. doi: 10.1007/s00417-005-0095-3
107. Grueterich M, Tseng SCG. Human limbal progenitor cells expanded on intact amniotic membrane ex vivo. Arch Ophthalmol. (2002) 120:783–90. doi: 10.1001/archopht.120.6.783
108. Grueterich M, Espana EM, Tseng SCG. Ex vivo expansion of limbal epithelial stem cells: amniotic membrane serving as a stem cell niche. Surv Ophthalmol. (2003) 48:631–46. doi: 10.1016/j.survophthal.2003.08.003
109. Davis JS. II. Skin grafting at the johns hopkins hospital. Ann Surg. (1909) 50:542–9. doi: 10.1097/00000658-190909000-00002
111. Stern M. The grafting of preserved amniotic membrane to burned and ulcerated surfaces, substituting skin grafts. J Am Med Assoc. (1913) 60:973–4. doi: 10.1001/jama.1913.04340130021008
112. Schmiedova I, Dembickaja A, Kiselakova L, Nowakova B, Slama P. Using of amniotic membrane derivatives for the treatment of chronic wounds. Membranes. (2021) 11:941. doi: 10.3390/membranes11120941
113. Kogan S, Sood A, Granick MS. Amniotic membrane adjuncts and clinical applications in wound healing: a review of the literature. Wounds. (2018) 30:168–73.30059334
114. Troensegaard-Hansen E. Amniotic grafts in chronic skin ulceration. Lancet. (1950) 255:859–60. doi: 10.1016/S0140-6736(50)90693-3
115. Zelen CM. An evaluation of dehydrated human amniotic membrane allografts in patients with DFUs. J Wound Care. (2013) 22:347–8; 350–351. doi: 10.12968/jowc.2013.22.7.347
116. Zelen CM, Serena TE, Denoziere G, Fetterolf DE. A prospective randomised comparative parallel study of amniotic membrane wound graft in the management of diabetic foot ulcers. Int Wound J. (2013) 10:502–7. doi: 10.1111/iwj.12097
117. Zelen CM, Gould L, Serena TE, Carter MJ, Keller J, Li WW. A prospective, randomised, controlled, multi-centre comparative effectiveness study of healing using dehydrated human amnion/chorion membrane allograft, bioengineered skin substitute or standard of care for treatment of chronic lower extremity diabetic ulcers. Int Wound J. (2015) 12:724–32. doi: 10.1111/iwj.12395
118. Zelen CM, Snyder RJ, Serena TE, Li WW. The use of human amnion/chorion membrane in the clinical setting for lower extremity repair: a review. Clin Podiatr Med Surg. (2015) 32:135–46. doi: 10.1016/j.cpm.2014.09.002
119. Serena TE, Carter MJ, Le LT, Sabo MJ, DiMarco DT, EpiFix VLU Study Group. A multicenter, randomized, controlled clinical trial evaluating the use of dehydrated human amnion/chorion membrane allografts and multilayer compression therapy vs. multilayer compression therapy alone in the treatment of venous leg ulcers. Wound Repair Regen. (2014) 22:688–93. doi: 10.1111/wrr.12227
120. DiDomenico LA, Orgill DP, Galiano RD, Serena TE, Carter MJ, Kaufman JP, et al. Use of an aseptically processed, dehydrated human amnion and chorion membrane improves likelihood and rate of healing in chronic diabetic foot ulcers: a prospective, randomised, multi-centre clinical trial in 80 patients. Int Wound J. (2018) 15:950–7. doi: 10.1111/iwj.12954
121. Perepelkin NMJ, Hayward K, Mokoena T, Bentley MJ, Ross-Rodriguez LU, Marquez-Curtis L, et al. Cryopreserved amniotic membrane as transplant allograft: viability and post-transplant outcome. Cell Tissue Bank. (2015) 17(1):39–50. doi: 10.1007/s10561-015-9530-9
122. Laurent I, Astère M, Wang KR, Cheng Q, Li QF. Efficacy and time sensitivity of amniotic membrane treatment in patients with diabetic foot ulcers: a systematic review and meta-analysis. Diabetes Ther. (2017) 8:967–79. doi: 10.1007/s13300-017-0298-8
123. Ruiz-Cañada C, Bernabé-García Á, Liarte S, Insausti CL, Angosto D, Moraleda JM, et al. Amniotic membrane stimulates cell migration by modulating transforming growth factor-β signalling. J Tissue Eng Regen Med. (2018) 12:808–20. doi: 10.1002/term.2501
124. Ruiz-Cañada C, Bernabé-García Á, Liarte S, Rodríguez-Valiente M, Nicolás FJ. Chronic wound healing by amniotic membrane: TGF-β and EGF signaling modulation in re-epithelialization. Front Bioeng Biotechnol. (2021) 9:689328. doi: 10.3389/fbioe.2021.689328
125. Barski D, Gerullis H, Ecke T, Varga G, Boros M, Pintelon I, et al. Repair of a vesico-vaginal fistula with amniotic membrane - step 1 of the IDEAL recommendations of surgical innovation. Cent European J Urol. (2015) 68:459–61. doi: 10.5173/ceju.2015.683
126. Barski D, Gerullis H, Ecke T, Varga G, Boros M, Pintelon I, et al. Human amniotic membrane dressing for the treatment of an infected wound due to an entero-cutaneous fistula: case report. Int J Surg Case Rep. (2018) 51:11–3. doi: 10.1016/j.ijscr.2018.08.015
127. Leila G, Saeideh P, Hajar K, Ali B, Vahid HS, Ali N, et al. A randomized trial study on the effect of amniotic membrane graft on wound healing process after anal fistulotomy. J Coloproctology. (2017) 37:187–92. doi: 10.1016/j.jcol.2017.03.006
128. Grossi U, Romano M, Rossi S, Gallo G, Picciariello A, Felice C, et al. Anal fistula human amniotic membrane endosealing (F-HAME): a proof of concept study. Front Surg. (2022) 9:869923. doi: 10.3389/fsurg.2022.869923
129. Ratto C, Litta F, Lucchetti D, Parello A, Boninsegna A, Arena V, et al. Immunopathological characterization of cryptoglandular anal fistula: a pilot study investigating its pathogenesis. Colorectal Dis. (2016) 18:O436–44. doi: 10.1111/codi.13527
130. Silini AR, Cancelli S, Signoroni PB, Cargnoni A, Magatti M, Parolini O. The dichotomy of placenta-derived cells in cancer growth. Placenta. (2017) 59:154–62. doi: 10.1016/j.placenta.2017.05.011
131. Ratto C, Parolini O, Marra AA, Orticelli V, Parello A, Campennì P, et al. Human amniotic membrane for the treatment of cryptoglandular anal fistulas. J Clin Med. (2022) 11:1350. doi: 10.3390/jcm11051350
132. DCunha AR, Jehangir S, Rebekah G, Thomas RJ. Human amniotic membrane vs collagen in the treatment of superficial second-degree burns in children. Wounds. (2022) 34:135–40. doi: 10.25270/wnds/2022.135140
133. Ahuja N, Jin R, Powers C, Billi A, Bass K. Dehydrated human amnion chorion membrane as treatment for pediatric burns. Adv Wound Care. (2020) 9:602–11. doi: 10.1089/wound.2019.0983
134. Ramakrishnan KM, Jayaraman V. Management of partial-thickness burn wounds by amniotic membrane: a cost-effective treatment in developing countries. Burns. (1997) 23(Suppl 1):S33–36. doi: 10.1016/S0305-4179(97)90099-1
135. Ravishanker R, Bath AS, Roy R. “Amnion bank”–the use of long term glycerol preserved amniotic membranes in the management of superficial and superficial partial thickness burns. Burns. (2003) 29:369–74. doi: 10.1016/S0305-4179(02)00304-2
136. Mohammadi AA, Sabet B, Riazi H, Tavakko-lian AR, Mohammadi MK, Iranpak S. Human amniotic membrane dressing: an excellent method for outpatient management of burn wounds. Iran J Med Sci. (2015) 34:61–4.
137. Mohammadi AA, Eskandari S, Johari HG, Rajabnejad A. Using amniotic membrane as a novel method to reduce post-burn hypertrophic scar formation: a prospective follow-up study. J Cutan Aesthet Surg. (2017) 10:13–7. doi: 10.4103/JCAS.JCAS_109_16
138. Faulk WP, Matthews R, Stevens PJ, Bennett JP, Burgos H, Hsi BL. Human amnion as an adjunct in wound healing. Lancet. (1980) 1:1156–8. doi: 10.1016/S0140-6736(80)91617-7
139. Loeffelbein DJ, Rohleder NH, Eddicks M, Baumann CM, Stoeckelhuber M, Wolff K-D, et al. Evaluation of human amniotic membrane as a wound dressing for split-thickness skin-graft donor sites. Biomed Res Int. (2014) 2014:572183. doi: 10.1155/2014/572183
140. Hermans MHE. Preservation methods of allografts and their (lack of) influence on clinical results in partial thickness burns. Burns. (2011) 37:873–81. doi: 10.1016/j.burns.2011.01.007
141. Singh R, Chouhan US, Purohit S, Gupta P, Kumar P, Kumar A, et al. Radiation processed amniotic membranes in the treatment of non-healing ulcers of different etiologies. Cell Tissue Bank. (2004) 5:129–34. doi: 10.1023/B:CATB.0000034077.05000.29
142. Singh R, Purohit S, Chacharkar MP, Bhandari PS, Bath AS. Microbiological safety and clinical efficacy of radiation sterilized amniotic membranes for treatment of second-degree burns. Burns. (2007) 33:505–10. doi: 10.1016/j.burns.2006.08.004
143. Tehrani FA, Ahmadiani A, Niknejad H. The effects of preservation procedures on antibacterial property of amniotic membrane. Cryobiology. (2013) 67:293–8. doi: 10.1016/j.cryobiol.2013.08.010
144. Murphy KG, Gunsolley JC. Guided tissue regeneration for the treatment of periodontal intrabony and furcation defects. A systematic review. Ann Periodontol. (2003) 8:266–302. doi: 10.1902/annals.2003.8.1.266
145. Needleman I, Tucker R, Giedrys-Leeper E, Worthington H. Guided tissue regeneration for periodontal intrabony defects–a cochrane systematic review. Periodontol. (2005) 2000(37):106–23. doi: 10.1111/j.1600-0757.2004.37101.x
146. Talmi YP, Sigler L, Inge E, Finkelstein Y, Zohar Y. Antibacterial properties of human amniotic membranes. Placenta. (1991) 12:285–8. doi: 10.1016/0143-4004(91)90010-D
147. Kumar A, Chandra RV, Reddy AA, Reddy BH, Reddy C, Naveen A. Evaluation of clinical, antiinflammatory and antiinfective properties of amniotic membrane used for guided tissue regeneration: a randomized controlled trial. Dent Res J. (2015) 12:127–35.
148. Kesting MR, Wolff K-D, Mücke T, Demtroeder C, Kreutzer K, Schulte M, et al. A bioartificial surgical patch from multilayered human amniotic membrane-in vivo investigations in a rat model. J Biomed Mater Res Part B Appl Biomater. (2009) 90:930–8. doi: 10.1002/jbm.b.31365
149. Rommel N, Rohleder NH, Gabriel C, Hennerbichler S, Bauer F, Mücke T, et al. Secondary correction of posttraumatic orbital wall adhesions by membranes laminated with amniotic membrane. Br J Oral Maxillofac Surg. (2013) 51:e224–229. doi: 10.1016/j.bjoms.2013.01.016
150. Sharma Y, Maria A, Kaur P. Effectiveness of human amnion as a graft material in lower anterior ridge vestibuloplasty: a clinical study. J Maxillofac Oral Surg. (2011) 10:283–7. doi: 10.1007/s12663-011-0230-0
151. Sikkerimath BC, Dandagi S, Gudi SS, Jayapalan D. Comparison of vestibular sulcus depth in vestibuloplasty using standard clark’s technique with and without amnion as graft material. Ann Maxillofac Surg. (2012) 2:30–5. doi: 10.4103/2231-0746.95313
152. Kothari CR, Goudar G, Hallur N, Sikkerimath B, Gudi S, Kothari MC. Use of amnion as a graft material in vestibuloplasty: a clinical study. Br J Oral Maxillofac Surg. (2012) 50:545–9. doi: 10.1016/j.bjoms.2011.09.022
153. Kar IB, Singh AK, Mohapatra PC, Mohanty PK, Misra S. Repair of oral mucosal defects with cryopreserved human amniotic membrane grafts: prospective clinical study. Int J Oral Maxillofac Surg. (2014) 43:1339–44. doi: 10.1016/j.ijom.2014.07.018
154. Li W, Ma G, Brazile B, Li N, Dai W, Butler JR, et al. Investigating the potential of amnion-based scaffolds as a barrier membrane for guided bone regeneration. Langmuir. (2015) 31:8642–53. doi: 10.1021/acs.langmuir.5b02362
155. Wu P-H, Chung H-Y, Wang J-H, Shih J-C, Kuo MY-P, Chang P-C, et al. Amniotic membrane and adipose-derived stem cell co-culture system enhances bone regeneration in a rat periodontal defect model. J Formos Med Assoc. (2016) 115:186–94. doi: 10.1016/j.jfma.2015.02.002
156. Kiany F, Moloudi F. Amnion membrane as a novel barrier in the treatment of intrabony defects: a controlled clinical trial. Int J Oral Maxillofac Implants. (2015) 30:639–47. doi: 10.11607/jomi.3590
157. Ghahroudi AAR, Khorsand A, Rokn AR, Sabounchi SS, Shayesteh YS, Soolari A. Comparison of amnion allograft with connective tissue graft for root coverage procedures: a double-blind, randomized, controlled clinical trial. J Int Acad Periodontol. (2013) 15:101–12.24364174
158. Keerthi R, Vaibhav N, Raut R. Amniotic membrane as a biological scaffold after vestibuloplasty. J Maxillofac Oral Surg. (2015) 14:383–7. doi: 10.1007/s12663-014-0631-y
159. Amemiya T, Nakamura T, Yamamoto T, Kinoshita S, Kanamura N. Autologous transplantation of oral mucosal epithelial cell sheets cultured on an amniotic membrane substrate for intraoral mucosal defects. PLoS One. (2015) 10:e0125391. doi: 10.1371/journal.pone.0125391
160. Odet S, Louvrier A, Meyer C, Nicolas FJ, Hofman N, Chatelain B, et al. Surgical application of human amniotic membrane and amnion-chorion membrane in the oral cavity and efficacy evaluation: corollary with ophthalmological and wound healing experiences. Front Bioeng Biotechnol. (2021) 9:685128. doi: 10.3389/fbioe.2021.685128
161. Kesting MR, Wolff K-D, Nobis CP, Rohleder NH. Amniotic membrane in oral and maxillofacial surgery. Oral Maxillofac Surg. (2014) 18:153–64. doi: 10.1007/s10006-012-0382-1
162. Ragazzo M, Trojan D, Spagnol L, Paolin A, Guarda Nardini L. Use of amniotic membrane in the treatment of patients with BRONJ: two case reports. J Surg Case Rep. (2018) 2018:rjy073. doi: 10.1093/jscr/rjy073
163. Ragazzo M, Val M, Montagner G, Trojan D, Fusetti S, Guarda Nardini L. Human amniotic membrane: an improvement in the treatment of medication-related osteonecrosis of the jaw (MRONJ)? A case-control study. Cell Tissue Bank. (2022) 23:129–41. doi: 10.1007/s10561-021-09922-y
164. Odet S, Meyer C, Gaudet C, Weber E, Quenot J, Derruau S, et al. Tips and tricks and clinical outcome of cryopreserved human amniotic membrane application for the management of medication-related osteonecrosis of the jaw (MRONJ): a pilot study. Front Bioeng Biotechnol. (2022) 10:936074. doi: 10.3389/fbioe.2022.936074
165. Parsons GS, Mathog RH. Orbital wall and volume relationships. Arch Otolaryngol Head Neck Surg. (1988) 114:743–7. doi: 10.1001/archotol.1988.01860190047020
166. Hwang K, You SH, Sohn IA. Analysis of orbital bone fractures: a 12-year study of 391 patients. J Craniofac Surg. (2009) 20:1218–23. doi: 10.1097/SCS.0b013e3181acde01
167. Burnstine MA. Clinical recommendations for repair of isolated orbital floor fractures: an evidence-based analysis. Ophthalmology. (2002) 109:1207–10; discussion 1210–11; quiz 1212–13. doi: 10.1016/S0161-6420(02)01057-6
168. Kushner BJ. Paresis and restriction of the inferior rectus muscle after orbital floor fracture. Am J Ophthalmol. (1982) 94:81–6. doi: 10.1016/0002-9394(82)90195-7
169. Hessling KH, Eckardt A, Schmelzeisen R, Mayer H. Indication for, technique and results of reconstruction of traumatic defects of the bony orbital floor. Fortschr Kiefer Gesichtschir. (1991) 36:207–9.1907943
170. Rinna C, Ungari C, Saltarel A, Cassoni A, Reale G. Orbital floor restoration. J Craniofac Surg. (2005) 16:968–72. doi: 10.1097/01.scs.0000186308.16795.8b
171. Ti SE, Tow SL, Chee SP. Amniotic membrane transplantation in entropion surgery. Ophthalmology. (2001) 108:1209–17. doi: 10.1016/S0161-6420(01)00599-1
172. Strube YNJ, Conte F, Faria C, Yiu S, Wright KW. Amniotic membrane transplantation for restrictive strabismus. Ophthalmology. (2011) 118:1175–9. doi: 10.1016/j.ophtha.2010.10.034
173. Mehendale RA, Dagi LR. Amniotic membrane implantation to reduce extraocular muscle adhesions to a titanium implant. J AAPOS. (2011) 15:404–6. doi: 10.1016/j.jaapos.2011.05.001
174. Cheon HW, Kim JW, Bae JH. The effect of amniotic membrane on the fibrosis between orbital connective tissue and porous polyethylene sheet implant. J Korean Ophthalmol Soc. (2003) 44:396–401.
175. Chen Y, Cui H. Amniotic membrane transplantation for porous sphere orbital implant exposure. J Zhejiang Univ Sci B. (2007) 8:616–9. doi: 10.1631/jzus.2007.B0616
176. Amer MI, Abd-El-Maeboud KH. Amnion graft following hysteroscopic lysis of intrauterine adhesions. J Obstet Gynaecol Res. (2006) 32:559–66. doi: 10.1111/j.1447-0756.2006.00454.x
177. Amer MI, Abd-El-Maeboud KHI, Abdelfatah I, Salama FA, Abdallah AS. Human amnion as a temporary biologic barrier after hysteroscopic lysis of severe intrauterine adhesions: pilot study. J Minim Invasive Gynecol. (2010) 17:605–11. doi: 10.1016/j.jmig.2010.03.019
178. Peng X, Li T, Zhao Y, Guo Y, Xia E. Safety and efficacy of amnion graft in preventing reformation of intrauterine adhesions. J Minim Invasive Gynecol. (2017) 24:1204–10. doi: 10.1016/j.jmig.2017.08.005
179. Gan L, Duan H, Sun F-Q, Xu Q, Tang Y-Q, Wang S. Efficacy of freeze-dried amnion graft following hysteroscopic adhesiolysis of severe intrauterine adhesions. Int J Gynaecol Obstet. (2017) 137:116–22. doi: 10.1002/ijgo.12112
180. Zheng F, Zhu B, Liu Y, Wang R, Cui Y. Meta-analysis of the use of amniotic membrane to prevent recurrence of intrauterine adhesion after hysteroscopic adhesiolysis. Int J Gynaecol Obstet. (2018) 143:145–9. doi: 10.1002/ijgo.12635
181. Vitale SG, Riemma G, Carugno J, Perez-Medina T, Alonso Pacheco L, Haimovich S, et al. Postsurgical barrier strategies to avoid the recurrence of intrauterine adhesion formation after hysteroscopic adhesiolysis: a network meta-analysis of randomized controlled trials. Am J Obstet Gynecol. (2022) 226:487–498.e8. doi: 10.1016/j.ajog.2021.09.015
182. Yu J, Zhang W, Huang J, Gou Y, Sun C, Zhang Y, et al. Management of intrauterine adhesions using human amniotic mesenchymal stromal cells to promote endometrial regeneration and repair through notch signalling. J Cell Mol Med. (2021) 25:11002–15. doi: 10.1111/jcmm.17023
183. Carvalho MJ. Amniotic membrane in gynaecology. In: Mamede AC, Botelho MF, editors. Amniotic membrane. Netherlands: Springer (2015). p. 165–75.
184. Tancer ML, Katz M, Veridiano NP. Vaginal epithelialization with human amnion. Obstet Gynecol. (1979) 54:345–9.471375
185. Dhall K. Amnion graft for treatment of congenital absence of the vagina. Br J Obstet Gynaecol. (1984) 91:279–82. doi: 10.1111/j.1471-0528.1984.tb04768.x
186. Ashworth MF, Morton KE, Dewhurst J, Lilford RJ, Bates RG. Vaginoplasty using amnion. Obstet Gynecol. (1986) 67:443–6.3945456
187. Morton KE, Dewhurst CJ. Human amnion in the treatment of vaginal malformations. Br J Obstet Gynaecol. (1986) 93:50–4. doi: 10.1111/j.1471-0528.1986.tb07813.x
188. Nisolle M, Donnez J. Vaginoplasty using amniotic membranes in cases of vaginal agenesis or after vaginectomy. J Gynecol Surg. (1992) 8:25–30. doi: 10.1089/gyn.1992.8.25
189. Fotopoulou C, Sehouli J, Gehrmann N, Schoenborn I, Lichtenegger W. Functional and anatomic results of amnion vaginoplasty in young women with mayer-rokitansky-küster-hauser syndrome. Fertil Steril. (2010) 94:317–23. doi: 10.1016/j.fertnstert.2009.01.154
190. Chaudhary R, Dhama V, Singh S, Azad R. Vaginoplasty in mayer-rokitansky-kuster-hauser syndrome using amnion: a case series. Int J Reprod Contracept Obstet Gynecol. (2016) 5:3832–9. doi: 10.18203/2320-1770.ijrcog20163849
191. Vatsa R, Bharti J, Roy KK, Kumar S, Sharma JB, Singh N, et al. Evaluation of amnion in creation of neovagina in women with mayer-rokitansky-kuster-hauser syndrome. Fertil Steril. (2017) 108:341–5. doi: 10.1016/j.fertnstert.2017.05.026
192. Goyal LD, Dhaliwal B, Singh P, Ganjoo S, Goyal V. Management of mullerian development anomalies: 9 years’ experience of a tertiary care center. Gynecol Minim Invasive Ther. (2020) 9:81–7. doi: 10.4103/GMIT.GMIT_13_19
193. Barski D, Gerullis H, Ecke T, Yang J, Varga G, Boros M, et al. Bladder reconstruction with human amniotic membrane in a Xenograft rat model: a preclinical study. Int J Med Sci. (2017) 14:310–8. doi: 10.7150/ijms.18127
194. Hasan O, Johnson GS, Siegert JJ, Garcini F, Nguyen TT. Novel role of dehydrated human amnion/chorion membrane in healing of denuded ureter in robot-assisted excision of endometriosis. Case Rep Obstet Gynecol. (2019) 2019:1920430. doi: 10.1155/2019/1920430
195. Adamowicz J, Pokrywczyńska M, Tworkiewicz J, Kowalczyk T, van Breda SV, Tyloch D, et al. New amniotic membrane based biocomposite for future application in reconstructive urology. PLoS One. (2016) 11:e0146012. doi: 10.1371/journal.pone.0146012
196. Cheng N, Velazquez N, Desroches BR, Munver R. Bioregenerative umbilical cord amniotic membrane allograft ureteral wrap during robot-assisted ureterolysis. J Endourol Case Rep. (2020) 6:431–4. doi: 10.1089/cren.2020.0185
197. Nejad AR, Hamidieh AA, Amirkhani MA, Sisakht MM. Update review on five top clinical applications of human amniotic membrane in regenerative medicine. Placenta. (2021) 103:104–19. doi: 10.1016/j.placenta.2020.10.026
198. Razdan S, Bajpai RR, Razdan S, Sanchez MA. A matched and controlled longitudinal cohort study of dehydrated human amniotic membrane allograft sheet used as a wrap around nerve bundles in robotic-assisted laparoscopic radical prostatectomy: a puissant adjunct for enhanced potency outcomes. J Robot Surg. (2019) 13:475–81. doi: 10.1007/s11701-018-0873-7
199. Barski D, Gerullis H, Ecke T, Boros M, Brune J, Beutner U, et al. Application of dried human amnion graft to improve post-prostatectomy incontinence and potency: a randomized exploration study protocol. Adv Ther. (2020) 37:592–602. doi: 10.1007/s12325-019-01158-3
200. Bourgeois M, Loisel F, Obert L, Pluvy I, Gindraux F. Can the amniotic membrane be used to treat peripheral nerve defects? A review of literature. Hand Surg Rehabil. (2019) 38:223–32. doi: 10.1016/j.hansur.2019.05.006
201. Bai J, Liu C, Kong L, Tian S, Yu K, Tian D. Electrospun polycaprolactone (PCL)-amnion nanofibrous membrane promotes nerve regeneration and prevents fibrosis in a rat sciatic nerve transection model. Front Surg. (2022) 9:842540. doi: 10.3389/fsurg.2022.842540
202. Shah Z, Bakhshi SK, Bajwa MH, Khalil M, Dewan MC, Shamim SM. Human amniotic membrane as a dural substitute in neurosurgery: a systematic review. Surg Neurol Int. (2022) 13:505. doi: 10.25259/SNI_794_2022
203. He Q, Li Q, Chen B, Wang Z. Repair of flexor tendon defects of rabbit with tissue engineering method. Chin J Traumatol. (2002) 5:200–8.12162896
204. Farr J, Gomoll AH, Yanke AB, Strauss EJ, Mowry KC, Study Group ASA. A randomized controlled single-blind study demonstrating superiority of amniotic suspension allograft injection over hyaluronic acid and saline control for modification of knee osteoarthritis symptoms. J Knee Surg. (2019) 32:1143–54. doi: 10.1055/s-0039-1696672
205. Gupta A. Allogenic amniotic tissue for treatment of knee and hip osteoarthritis. Pharmaceuticals. (2022) 15:404. doi: 10.3390/ph15040404
206. Willett NJ, Thote T, Lin AS, Moran S, Raji Y, Sridaran S, et al. Intra-articular injection of micronized dehydrated human amnion/chorion membrane attenuates osteoarthritis development. Arthritis Res Ther. (2014) 16:R47. doi: 10.1186/ar4476
207. Vines JB, Aliprantis AO, Gomoll AH, Farr J. Cryopreserved amniotic suspension for the treatment of knee osteoarthritis. J Knee Surg. (2016) 29:443–50. doi: 10.1055/s-0035-1569481
208. Gellhorn AC, Han A. The use of dehydrated human amnion/chorion membrane allograft injection for the treatment of tendinopathy or arthritis: a case series involving 40 patients. PM R. (2017) 9(12):1236–43. doi: 10.1016/j.pmrj.2017.04.011
209. Castellanos R, Tighe S. Injectable amniotic membrane/umbilical cord particulate for knee osteoarthritis: a prospective, single-center pilot study. Pain Med. (2019) 20:2283–91. doi: 10.1093/pm/pnz143
210. Mead OG, Mead LP. Intra-Articular injection of amniotic membrane and umbilical cord particulate for the management of moderate to severe knee osteoarthritis. Orthop Res Rev. (2020) 12:161–70. doi: 10.2147/ORR.S272980
211. Natali S, Farinelli L, Screpis D, Trojan D, Montagner G, Favaretto F, et al. Human amniotic suspension allograft improves pain and function in knee osteoarthritis: a prospective not randomized clinical pilot study. J Clin Med. (2022) 11:3295. doi: 10.3390/jcm11123295
212. Reece DS, Burnsed OA, Parchinski K, Marr EE, White RM, Salazar-Noratto GE, et al. Reduced size profile of amniotic membrane particles decreases osteoarthritis therapeutic efficacy. Tissue Eng Part A. (2020) 26:28–37. doi: 10.1089/ten.TEA.2019.0074
213. Tabet SK, Kimmerling KA, Hale GJ, Munson NR, Mowry KC. Hypothermically stored amniotic membrane for the treatment of cartilage lesions: a single-arm prospective study with 2-year follow-up. Cartilage. (2022) 13:19476035211072212. doi: 10.1177/19476035211072213
214. Zavatti M, Beretti F, Casciaro F, Bertucci E, Maraldi T. Comparison of the therapeutic effect of amniotic fluid stem cells and their exosomes on monoiodoacetate-induced animal model of osteoarthritis. Biofactors. (2020) 46:106–17. doi: 10.1002/biof.1576
215. Etchebarne M, Fricain J-C, Kerdjoudj H, Di Pietro R, Wolbank S, Gindraux F, et al. Use of amniotic membrane and its derived products for bone regeneration: a systematic review. Front Bioeng Biotechnol. (2021) 9:661332. doi: 10.3389/fbioe.2021.661332
216. Rameshbabu AP, Ghosh P, Subramani E, Bankoti K, Kapat K, Datta S, et al. Investigating the potential of human placenta-derived extracellular matrix sponges coupled with amniotic membrane-derived stem cells for osteochondral tissue engineering. J Mater Chem B. (2016) 4:613–25. doi: 10.1039/c5tb02321a
217. Seo JH, Kim YH, Kim JS. Properties of the amniotic membrane may be applicable in cancer therapy. Med Hypotheses. (2008) 70:812–4. doi: 10.1016/j.mehy.2007.08.008
218. Mamede AC, Pires AS, Brito AF. Amniotic membrane in cancer. In: Mamede AC, Botelho MF, editors. Amniotic membrane. Netherlands: Springer (2015). p. 139–52.
219. Niknejad H, Yazdanpanah G, Mirmasoumi M, Abolghasemi H, Peirovi H, Ahmadiani A. Inhibition of HSP90 could be possible mechanism for anti-cancer property of amniotic membrane. Med Hypotheses. (2013) 81:862–5. doi: 10.1016/j.mehy.2013.08.018
220. Niknejad H, Khayat-Khoei M, Peirovi H, Abolghasemi H. Human amniotic epithelial cells induce apoptosis of cancer cells: a new anti-tumor therapeutic strategy. Cytotherapy. (2014) 16:33–40. doi: 10.1016/j.jcyt.2013.07.005
221. Khademi B, Bahranifard H, Azarpira N, Behboodi E. Clinical application of amniotic membrane as a biologic dressing in oral cavity and pharyngeal defects after tumor resection. Arch Iran Med. (2013) 16:503–6. 013169/AIM.00423981151
222. Goktas SE, Katircioglu Y, Celik T, Ornek F. Surgical amniotic membrane transplantation after conjunctival and limbal tumor excision. Arq Bras Oftalmol. (2017) 80:242–6. doi: 10.5935/0004-2749.20170059
223. Ramuta TŽ, Jerman UD, Tratnjek L, Janev A, Magatti M, Vertua E, et al. The cells and extracellular matrix of human amniotic membrane hinder the growth and invasive potential of bladder urothelial cancer cells. Front Bioeng Biotechnol. (2020) 8:554530. doi: 10.3389/fbioe.2020.554530
224. Ramuta TŽ, Starčič Erjavec M, Kreft ME. Amniotic membrane preparation crucially affects its broad-spectrum activity against uropathogenic bacteria. Front Microbiol. (2020) 11:469. doi: 10.3389/fmicb.2020.00469
225. Mamede AC, Laranjo M, Carvalho MJ, Abrantes AM, Pires AS, Brito AF, et al. Effect of amniotic membrane proteins in human cancer cell lines: an exploratory study. J Membr Biol. (2014) 247:357–60. doi: 10.1007/s00232-014-9642-3
226. Teixo R, Pires AS, Pereira E, Serambeque B, Marques IA, Laranjo M, et al. Application of perinatal derivatives on oncological preclinical models: a review of animal studies. Int J Mol Sci. (2022) 23:8570. doi: 10.3390/ijms23158570
227. Riedel R, Pérez-Pérez A, Carmona-Fernández A, Jaime M, Casale R, Dueñas JL, et al. Human amniotic membrane conditioned medium inhibits proliferation and modulates related microRNAs expression in hepatocarcinoma cells. Sci Rep. (2019) 9:14193. doi: 10.1038/s41598-019-50648-5
228. Jafari A, Rezaei-Tavirani M, Niknejad H, Zali H. Tumor targeting by conditioned medium derived from human amniotic membrane: new insight in breast cancer therapy. Technol Cancer Res Treat. (2021) 20:15330338211036318. doi: 10.1177/15330338211036318
229. Janev A, Ramuta TŽ, Tratnjek L, Sardoč Ž, Obradović H, Mojsilović S, et al. Detrimental effect of various preparations of the human amniotic membrane homogenate on the 2D and 3D bladder cancer in vitro models. Front Bioeng Biotechnol. (2021) 9:690358. doi: 10.3389/fbioe.2021.690358
230. Modaresifar K, Azizian S, Zolghadr M, Moravvej H, Ahmadiani A, Niknejad H. The effect of cryopreservation on anti-cancer activity of human amniotic membrane. Cryobiology. (2017) 74:61–7. doi: 10.1016/j.cryobiol.2016.12.001
231. Silini AR, Masserdotti A, Papait A, Parolini O. Shaping the future of perinatal cells: lessons from the past and interpretations of the present. Front Bioeng Biotechnol. (2019) 7:75. doi: 10.3389/fbioe.2019.00075
232. Alvim RG, Hughes C, Somma A, Nagar KK, Wong NC, La Rosa S, et al. The potential risk of tumor progression after use of dehydrated human amnion/chorion membrane allograft in a positive margin resection model. Ther Adv Urol. (2019) 11:1756287219837771. doi: 10.1177/1756287219837771
233. Jafari A, Rezaei-Tavirani M, Farhadihosseinabadi B, Zali H, Niknejad H. Human amniotic mesenchymal stem cells to promote/suppress cancer: two sides of the same coin. Stem Cell Res Ther. (2021) 12:126. doi: 10.1186/s13287-021-02196-x
234. Frigerio I, Bannone E, Trojan D, Montagner G, Bergamaschi G, Butturini G. Implantation of amniotic membrane over pancreatic anastomosis after pancreaticoduodenectomy: report of the first case. J Surg Case Rep. (2019) 2019:rjz097. doi: 10.1093/jscr/rjz097
235. Martelloni M, Montagner G, Trojan D, Abate R. Amniotic membrane in palatal epithelial-connective tissue reconstruction. Int Med Case Rep J. (2019) 12:349–54. doi: 10.2147/IMCRJ.S213275
236. Marton E, Giordan E, Gioffrè G, Canova G, Paolin A, Mazzucco MG, et al. Homologous cryopreserved amniotic membrane in the repair of myelomeningocele: preliminary experience. Acta Neurochir. (2018) 160:1625–31. doi: 10.1007/s00701-018-3577-x
237. Faramarzi M, Shishegar M, Kazemi T, Tavakolpour Saleh H, Roosta S. The effect of applying amniotic membrane on post-tonsillectomy pain and bleeding. Eur Arch Otorhinolaryngol. (2021) 278:485–92. doi: 10.1007/s00405-020-06173-7
238. Iravani K, Hashemi SB, Tehrani M, Rashidi M. Amniotic membrane in reconstruction of larynx following chondrosarcoma resection: a case report. Am J Otolaryngol. (2014) 35:520–3. doi: 10.1016/j.amjoto.2014.03.007
239. Elkhenany H, El-Derby A, Abd Elkodous M, Salah RA, Lotfy A, El-Badri N. Applications of the amniotic membrane in tissue engineering and regeneration: the hundred-year challenge. Stem Cell Res Ther. (2022) 13:8. doi: 10.1186/s13287-021-02684-0
240. Rahman MS, Islam R, Rana MM, Spitzhorn L-S, Rahman MS, Adjaye J, et al. Characterization of burn wound healing gel prepared from human amniotic membrane and aloe vera extract. BMC Complement Altern Med. (2019) 19:115. doi: 10.1186/s12906-019-2525-5
241. Li X, Li P, Wang C, Shang T, Han H, Tong Y, et al. A thermo-sensitive and injectable hydrogel derived from a decellularized amniotic membrane to prevent intrauterine adhesion by accelerating endometrium regeneration. Biomater Sci. (2022) 10:2275–86. doi: 10.1039/d1bm01791h
242. Wang B, Wang X, Kenneth A, Drena A, Pacheco A, Kalvin L, et al. Developing small-diameter vascular grafts with human amniotic membrane: long-term evaluation of transplantation outcomes in a small animal model. Biofabrication. (2023) 15(2):025004. doi: 10.1088/1758-5090/acb1da
243. Liu J, Chen D, Zhu X, Liu N, Zhang H, Tang R, et al. Development of a decellularized human amniotic membrane-based electrospun vascular graft capable of rapid remodeling for small-diameter vascular applications. Acta Biomater. (2022) 152:144–56. doi: 10.1016/j.actbio.2022.09.009
244. Grémare A, Thibes L, Gluais M, Torres Y, Potart D, Da Silva N, et al. Development of a vascular substitute produced by weaving yarn made from human amniotic membrane. Biofabrication. (2022) 14. doi: 10.1088/1758-5090/ac84ae
245. Mahbod M, Shahhoseini S, Khabazkhoob M, Beheshtnejad A-H, Bakhshandeh H, Atyabi F, et al. Amniotic membrane extract preparation: what is the best method? J Ophthalmic Vis Res. (2014) 9:314–9. doi: 10.4103/2008-322X.143367
246. Chen S-Y, Zhu Y, Zhang Y, Hsu D, Tseng SCG. HC-HA/PTX3 from amniotic membrane reverts senescent limbal niche cells to Pax6+ neural crest progenitors to support limbal epithelial progenitors. Stem Cells. (2021) 39:280–95. doi: 10.1002/stem.3323
247. Baradaran-Rafii A, Asl NS, Ebrahimi M, Jabbehdari S, Bamdad S, Roshandel D, et al. The role of amniotic membrane extract eye drop (AMEED) in in vivo cultivation of limbal stem cells. Ocul Surf. (2018) 16:146–53. doi: 10.1016/j.jtos.2017.11.001
248. Shayan Asl N, Nejat F, Mohammadi P, Nekoukar A, Hesam S, Ebrahimi M, et al. Amniotic membrane extract eye drop promotes limbal stem cell proliferation and corneal epithelium healing. Cell J. (2019) 20:459–68. doi: 10.22074/cellj.2019.5423
249. Bonacci E, Kilian R, Rizzo C, De Gregorio A, Bosello F, Fasolo A, et al. Microscopic corneal epithelial changes and clinical outcomes in simple limbal epithelial transplantation surgery after treatment with amniotic membrane eye drops (AMED): a case report. Am J Ophthalmol Case Rep. (2023) 29:101763. doi: 10.1016/j.ajoc.2022.101763
250. Guo Q, Hao J, Yang Q, Guan L, Ouyang S, Wang J. A comparison of the effectiveness between amniotic membrane homogenate and transplanted amniotic membrane in healing corneal damage in a rabbit model. Acta Ophthalmol. (2011) 89:e315–9. doi: 10.1111/j.1755-3768.2010.02097.x
251. Pérez ML, Barreales S, Sabater-Cruz N, Martinez-Conesa EM, Vilarrodona A, Casaroli-Marano RP. Amniotic membrane extract eye drops: a new approach to severe ocular surface pathologies. Cell Tissue Bank. (2021) 23(3):473–81. doi: 10.1007/s10561-021-09962-4
252. Sabater-Cruz N, Figueras-Roca M, Ferrán-Fuertes M, Agustí E, Martínez-Conesa EM, Pérez-Rodríguez ML, et al. Amniotic membrane extract eye drops for ocular surface diseases: use and clinical outcome in real-world practice. Int Ophthalmol. (2021) 41:2973–9. doi: 10.1007/s10792-021-01856-4
253. Murri MS, Moshirfar M, Birdsong OC, Ronquillo YC, Ding Y, Hoopes PC. Amniotic membrane extract and eye drops: a review of literature and clinical application. Clin Ophthalmol. (2018) 12:1105–12. doi: 10.2147/OPTH.S165553
254. Go YY, Kim SE, Cho GJ, Chae S-W, Song J-J. Differential effects of amnion and chorion membrane extracts on osteoblast-like cells due to the different growth factor composition of the extracts. PLoS One. (2017) 12:e0182716. doi: 10.1371/journal.pone.0182716
255. Russo A, Bonci P, Bonci P. The effects of different preservation processes on the total protein and growth factor content in a new biological product developed from human amniotic membrane. Cell Tissue Bank. (2012) 13:353–61. doi: 10.1007/s10561-011-9261-5
256. Stachon T, Bischoff M, Seitz B, Huber M, Zawada M, Langenbucher A, et al. Growth factors and interleukins in amniotic membrane tissue homogenate. Klin Monbl Augenheilkd. (2015) 232:858–62. doi: 10.1055/s-0034-1383393
257. Stachon T, Wu M-F, Bischoff M, Huber M, Langenbucher A, Seitz B, et al. Amniotic membrane suspension and autologous serum - are they important for wound healing? Klin Monbl Augenheilkd. (2017) 234:1015–8. doi: 10.1055/s-0042-122338
258. Suroto H, Aryawan DM, Prakoeswa CA. The influence of the preservation method and gamma irradiation sterilization on TGF-β and bFGF levels in freeze-dried amnion membrane (FD-AM) and amnion sponge. Int J Biomater. (2021) 2021:6685225. doi: 10.1155/2021/6685225
259. Dudok DV, Nagdee I, Cheung K, Liu H, Vedovelli L, Ghinelli E, et al. Effects of amniotic membrane extract on primary human corneal epithelial and limbal cells. Clin Exp Ophthalmol. (2015) 43:443–8. doi: 10.1111/ceo.12480
260. Huddleston HP, Cohn MR, Haunschild ED, Wong SE, Farr J, Yanke AB. Amniotic product treatments: clinical and basic science evidence. Curr Rev Musculoskelet Med. (2020) 13:148–54. doi: 10.1007/s12178-020-09614-2
Keywords: human amniotic membrane, preparation, preservation, application, characteristics
Citation: Hofmann N, Rennekampff H-O, Salz AK and Börgel M (2023) Preparation of human amniotic membrane for transplantation in different application areas. Front. Transplant. 2:1152068. doi: 10.3389/frtra.2023.1152068
Received: 27 January 2023; Accepted: 20 April 2023;
Published: 5 May 2023.
Edited by:
Nicco Krezdorn, Hannover Medical School, GermanyReviewed by:
Shahrbanoo Jahangir, AO Research Institute, SwitzerlandIbrahim Fathi, Nihon University, Japan
© 2023 Hofmann, Rennekampff, Salz and Börgel. This is an open-access article distributed under the terms of the Creative Commons Attribution License (CC BY). The use, distribution or reproduction in other forums is permitted, provided the original author(s) and the copyright owner(s) are credited and that the original publication in this journal is cited, in accordance with accepted academic practice. No use, distribution or reproduction is permitted which does not comply with these terms.
*Correspondence: Nicola Hofmann bmljb2xhLmhvZm1hbm5AZ2V3ZWJlbmV0endlcmsuZGU=