- 1Centre for International Development and Environmental Research, Justus Liebig University Giessen, Giessen, Germany
- 2Water Resources Management Group, Technical University of Cotopaxi, Latacunga, Ecuador
- 3Research Centre for BioSystems, Land Use and Nutrition (iFZ), Justus Liebig University Giessen, Institute for Landscape Ecology and Resources Management (ILR), Giessen, Germany
The political, economic, social, technological, environmental, and legal (PESTEL) dimensions in a local community shape the adoption of specific nature-based solutions (NbS). This study provides crucial insights on NbS tailored to smallholder indigenous and peasant communities heavily reliant on water ecosystem services from headwater streams, lakes, ponds, and reservoirs in the páramo ecosystems of the central Ecuadorian Andes. Combining a multi-stakeholder workshop with bibliometric analysis, we developed a framework that integrates NbS with local communities’ PESTEL dimensions to sustain water ecosystem services. As a result, the lack of political will to sustain healthy aquatic ecosystems, urban-centered environmental investment, and agricultural expansion mainly influence the sustainability of water ecosystem services in the political, economic and environmental dimensions. Social, legal, and technological dimensions encompass community dissatisfaction, resistance to conservation, neglect of clean water and land use regulations, and limited innovation investment. Artificial floating islands and passive river restoration were the NbS adaptable to these PESTEL dimensions in our local communities. Artificial floating islands, a macrophyte-based technology that integrates community plant knowledge, are feasible even with limited financial resources. Passive river restoration complements them to promote headwater vegetation recovery and governance of water ecosystem services. Their integration supports drinking water supply, irrigation, fisheries, water purification, habitat conservation, soil formation carbon sequestration, and the achievement of several Sustainable Development Goals (SDGs). We provide decision-makers with a rigorous assessment of NbS for local communities, with the potential to scale to countries with similar contexts and highlight the need for future research to explore NbS in regional or national frameworks.
1 Introduction
Relying on nature to improve human well-being and the environment is a shared goal of ecosystem services and nature-based solutions (Grizzetti et al., 2016; Jarosiewicz et al., 2022). Thus, ecosystem services are crucial for delivering social, ecological, and economic benefits to humanity. Moreover, nature-based solutions (NbS) maintain these benefits by conserving or restoring terrestrial and aquatic ecosystems (Blahna et al., 2017; Keesstra et al., 2018; Oral et al., 2020). In aquatic ecosystems, including rivers, lakes, ponds, and reservoirs and their diverse habitats, like riparian zones (Grizzetti et al., 2016; Culhane et al., 2019), NbS are crucial for sustaining several water ecosystem services. These include maintaining water quality and quantity, supporting nutrient cycling, maintaining populations and habitats, and promoting recreational and cultural services (Jarosiewicz et al., 2022; Possantti and Marques, 2022; Souliotis and Voulvoulis, 2022).
In developing countries, where ecological degradation of aquatic ecosystems is widespread, and local communities rely heavily on water ecosystem services for survival and livelihoods, NbS are crucial for achieving sustainability (Kenter et al., 2011; Telwala, 2023; UNCTAD, 2022). Cost-effective alternatives (Colares et al., 2020), promoting efficient and sustainable use of water resources (Souliotis and Voulvoulis, 2022), stakeholder engagement (Pagano et al., 2019), incorporation of community knowledge (Baustian et al., 2020), developing a healthier relationship between humans and the nonhuman world (Hoffman, 2023), improving the delivery of a range of ecosystem services (Liquete et al., 2016), and contribution to achieving multiple Sustainable Development Goals (SDGs) (Sowińska-Świerkosz and García, 2022) are some of the benefits highlighted by NbS.
Artificial floating islands (AFIs), one of the latest phytotechnology innovations in the remediation of degraded water bodies, stand out among NbS due to their low operational costs and maintenance, lack of land requirements, and ease of implementation (Afzal et al., 2019; Fonseca et al., 2021). The low operating cost of AFIs is due to the high removal efficiency, mainly dependent on macrophytes, supported by inexpensive flotation materials (Souliotis and Voulvoulis, 2022; Cui et al., 2022; Samal, 2019; Yeh et al., 2015). The main component of AFIs is macrophytes with their associated microbial communities of biofilms and zooplankton, which play a dual role in directly assimilating pollutants into their tissues and acting as catalysts for purification reactions (Cui et al., 2022; Benvenuti et al., 2018). Moreover, passive ecological restoration, also known as passive river restoration, is especially suitable for headwater streams where water quality conditions are restored by eliminating disturbance factors and allowing for natural vegetation recovery (Muller et al., 2016; Taniwaki et al., 2019; Trujillo-Miranda et al., 2018).
To maximize their potential in local communities’ aquatic ecosystems, AFIs and passive river restoration should be tailored to the broader macro-environment in which they operate. This macro-environment includes political, economic, social, technological, environmental, and legal factors, commonly known as PESTEL (Den Heijer and Coppens, 2023; Kansongue et al., 2023). However, the impact of PESTEL factors on water ecosystem services initiatives remains largely unknown.
Due to these complex adaptive dynamics of those NbS, PESTEL analysis has become a crucial tool for decision-makers to identify factors influencing their performance in improving environmental conditions and to guide adaptive responses (Den Heijer and Coppens, 2023). Similarly, the involvement of local communities is a vital aspect of NbS inclusion, as the degradation of aquatic ecosystems is not only critical on a global scale but also profoundly impacts locally (Baustian et al., 2020; Carroll et al., 2019; Giordano et al., 2020). Local communities, deeply rooted in agriculture and ancestral traditions (Conable, 2015), stand out as primary stakeholders in NbS design due to their experience gained through their long interaction with the environment and heavy reliance on ecosystem services (Balzan et al., 2022; Gbedemah, 2023).
Involving local communities and other key stakeholders, from problem identification to decision-making, contributes to more scientifically legitimate and publicly accountable decisions (Corburn, 2007; Khatibi et al., 2021). Therefore, community knowledge is growing in significance as it extends beyond individual ideas, is accessible to multi-stakeholders and the public, and offers opportunities for networked participation (Corburn, 2007; Hong and Scardamalia, 2014). Also, positive changes in resource management are more likely to be initiated when the attitudes, beliefs, or preferences of multi-stakeholders are considered in problem identification and the development of solutions (Lynam et al., 2007). Furthermore, for a practical application, Corburn (2007) recommends critical and sustained analysis of community knowledge. Thus, incorporating policy documents, grey literature, academic articles, and fieldwork strengthens community knowledge integration in research (Bisaga et al., 2021; Skrydstrup et al., 2020).
In developing countries, ensuring the health of aquatic ecosystems to sustain their services is a critical challenge; however, it also offers an opportunity for community collaboration, fostering community learning and innovation (Carroll et al., 2019; Vollmer et al., 2022). In this context, we developed a framework that integrates artificial floating islands and passive river restoration aligned with the PESTEL dimensions of local communities to sustain water ecosystem services in the central Ecuadorian Andes. Combining a multi-stakeholder workshop with a bibliometric analysis, we answered our research questions: (i) How do PESTEL dimensions of local communities influence the water ecosystem services’ sustainability? (ii) How does integrating artificial floating islands with passive river restoration within the PESTEL dimensions of local communities contribute to the sustainability of water ecosystem services? In this study, we selected several Andean communities in Ecuador to illustrate the importance of healthy aquatic ecosystems in sustaining the water ecosystem services providing livelihoods for millions of people (Mosquera et al., 2023; Thompson et al., 2021).
2 Materials and methods
2.1 Study area
The study focused on local communities situated in the provinces of Pichincha, Cotopaxi, Bolivar and Chimborazo, encompassing 26,424 km2 in the central Andean region of Ecuador (Figure 1). In these provinces, three major river basins (Guayas, Esmeraldas, and Pastaza) emerge, housing 68% of the Ecuadorian Andean population (INEC, 2022).
Agriculture, tourism, and mining are essential pillars of the economy of these provinces, collectively contributing 32% to Ecuador’s gross domestic product (BCE, 2021). Despite this, the population grapples with significant levels of extreme poverty, with Chimborazo at 38.9%, Bolívar at 34.1%, and Cotopaxi at 26.3% (InfoMIES, 2022), rendering them particularly vulnerable. In addition, Chimborazo, Bolívar and Cotopaxi rank as Ecuador’s top provinces for chronic malnutrition in children under five, with rates of 35.2, 35.1, and 31.8%, respectively (INEC, 2019).
2.1.1 Water ecosystem services delivered to our local communities
High-elevation tropical ecosystems, known as páramo ecosystems, are home to local communities in our study area (Figure 1). Local communities, including smallholder indigenous and peasant communities, in Ecuador’s páramo ecosystems are authorised by articles 32, 45 and 73 of the Ecuadorian water law (Asamblea Nacional, 2014) to establish drinking water and irrigation boards and participate in the use and the community management of the water resources that flow through their territory.
The páramos encompass crucial aquatic ecosystems, i.e., the headwaters of many Andean river basins, along with lakes, ponds and reservoirs, which serve as primary drinking and irrigation water sources for the local communities (Mosquera et al., 2023; SENAGUA, 2021). Despite the benefits that urban and rural areas in Pichincha, Cotopaxi, Bolívar., and Chimborazo gain from the páramo ecosystems (SENAGUA, 2021; Hofstede et al., 2014), several studies indicate that their degradation threatens their sustainability (Mosquera et al., 2023; Thompson et al., 2021; García et al., 2019; Vinueza et al., 2021; Zapata et al., 2021). Hence, the provision of water ecosystem services, like drinking water, irrigation water, fisheries, and aquaculture, is directly compromised. Indirectly, the regulation and maintenance of further ecosystem services, including water purification, habitat and population maintenance, soil formation and composition, and carbon sequestration, are also affected. Moreover, there is a significant cultural impact concerning the indigenous cosmovision, where aquatic ecosystems hold a vital place in Mother Earth “Pachamama” alongside people, animals, ancestors, and land to achieve a holistic and well-balanced way of living, known in the indigenous language as “Sumak kawsay” (Kleemann et al., 2022; Salmoral et al., 2018).
2.2 Multi-stakeholder participation
Representatives of local communities, alongside decentralized autonomous governments at provincial, municipal, and parish levels in each province, were the primary stakeholders in building community knowledge. Additionally, participants from a non-governmental organization (NGO) CARE (Cooperative for Assistance and Relief Everywhere), the Environmental Fund for Water Protection of Quito (FONAG), professors and researchers from the Technical University of Cotopaxi and the Justus Liebig University Giessen, were involved.
The selection of participation sectors was guided by Ecuadorian water law (Asamblea Nacional, 2014), which emphasizes collective responsibility for sustainable water resource management shared among the national government, various governmental levels, and local communities, as outlined in articles 12 and 19. Furthermore, universities were invited to participate according to this law (Asamblea Nacional, 2014).
2.3 Developing a framework for NbS adaptation
We applied Albert et al. (2021) six-step NbS adaptation, divided into two stages. In the first stage, a participatory workshop was conducted to (1) co-defining the setting, which involved the workshop kick-off and clarifying the context, overarching societal challenges, aims, and processes, and (2) assessing challenges through multidimensional assessment, choosing the PESTEL dimension for our study (Den Heijer and Coppens, 2023; Kansongue et al., 2023). Subsequently, through bibliometric analysis of scientific data, we (3) identified that AFIs and passive river restoration have the potential to sustain water ecosystem services in our local communities. Hence, those NbS were (4) aligned with most of their PESTEL dimensions and related to several SDGs. Additionally, we (5) identified opportunities and obstacles and suggested future research directions for their implementation. The first NbS actions, outlined by Albert et al. (2021) as a six-step, do not align with the scope of this study.
2.3.1 Local communities’ PESTEL dimensions captured by the workshop
In the first stage, multi-stakeholders were engaged in a workshop to analyse the multidimensional challenges in their local communities influencing the sustainability of water ecosystem services. Four participant groups were established based on their respective provinces to answer the research question: (i) How do PESTEL dimensions of local communities influence the water ecosystem services’ sustainability? Subsequently, the information from these groups was consolidated and combined with studies from other developing countries to extend its applicability to countries experiencing similar contexts. A total of 39 participants attended the workshop held on 29 and 30 November 2022 in Latacunga, Ecuador.
2.3.2 Exploring and analysing scientific data for NbS adaptation
We began by selecting the Web of Science platform for scientific literature and bibliometric analysis because of its access to high-quality research published in scientific journals and alignment with bibliometric analysis tools. In the scientific literature search, we combined artificial floating islands and passive river restoration with aquatic ecosystems and water ecosystem services specific to our local communities using the following keywords: “artificial floating islands” “constructed wetlands,” “passive river restoration,” “passive ecological restoration” with “páramo,” “headwater stream,” “lake,” “pond,” and “reservoir” as well as “drinking water,” “irrigation water,” “fisheries,” “aquaculture,” “water purification,” “habitat and population maintenance,” “soil formation and composition,” “carbon sequestration,” “climate regulation,” “cultural impact” and “SDGs,” respectively. After identifying relevant studies, we conducted an abstract screening to select studies aligned with PESTEL dimensions of local communities. The literature screening concluded once no new information was obtained. In total, data was extracted from 62 peer-reviewed articles, academic books, and book chapters published between 1991 and 2023.
Subsequently, we utilized the VOSviewer software to create network view maps of the collected scientific knowledge based on Colares et al. (2020) and Donthu et al. (2021). Following data cleaning, the extracted terms were visualized as network view maps, showcasing clusters, core terms (large circles), and items (small circles). These clusters encompassed main core terms and items, with connections denoting links between them, and distances reflect the strength of relationships. The network view maps of artificial floating islands and passive river restoration were created using terms extracted from text data, including titles and abstracts. These maps allowed analysis from cluster and term perspectives (Colares et al., 2020) to explore the existing or future relationships among topics in a research field (Emich et al., 2020).
3 Results and discussion
This section presents the PESTEL dimensions influencing the sustainability of water ecosystem services in the local communities, identified by a multi-stakeholder workshop (Figure 2), along with NbS adapted to these dimensions through a bibliometric analysis. Combining these results with discussion extends the applicability to countries facing similar contexts.
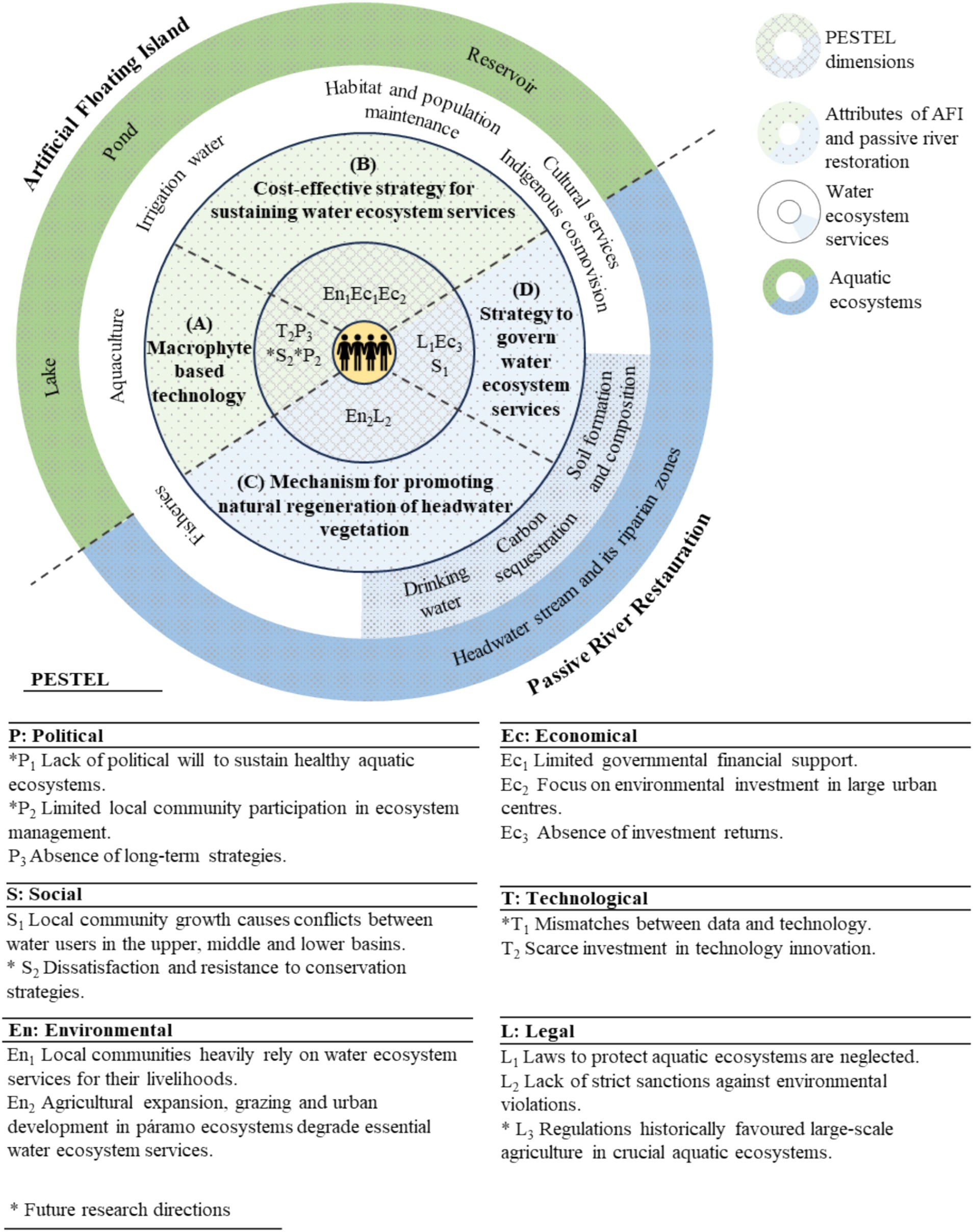
Figure 2. NbS to sustain water ecosystem services embedded in the local communities’ PESTEL dimensions.
3.1 The PESTEL dimensions in the water ecosystem services’ sustainability
3.1.1 Political (P) dimension
Workshop participants reported that the main challenge faced by local communities is the lack of political will to support strategies that sustain healthy aquatic ecosystems (P1), a frequent obstacle in water resources management in several developing countries. Warner et al. (2014) partly attributed these political challenges in developing countries to the complexity of managing aquatic ecosystems and conflicting interests. For example, in recent decades, the river basin scale has been deemed the “natural” scale for water planning and management (Molle, 2008), but it is not a priority in countries like Ecuador. In contrast, politicians often use more relevant issues, such as land tenure conflicts, as a political platform to gain political support, as reported by Coral et al. (2021) in their study on lessons from Ecuador’s history.
The undervaluation of water ecosystem services due to political priorities in developing countries hinders the implementation of sustainable practices in key sectors, like agriculture, that impact the sustainability of water ecosystem services (Salmoral et al., 2018; Nahuelhual et al., 2018). Similarly, the limited local community participation in ecosystem management (P3) exacerbates these challenges from a multi-stakeholder perspective. Inadequacies of poor communities’ political socioeconomic infrastructure and knowledge base and distrust are the main issues for the governance of sustained participation. Deficiencies in the socio-political infrastructure of local communities and mistrust in these systems are the main problems, as per Khatibi et al. (2021), for sustained participation in governance. Carroll et al. (2019) observed that lack of community engagement can increase the risks of water catastrophes. These findings align with our local communities, where unsustainable management in agriculture, livestock grazing, and urban growth contribute to the degradation of aquatic ecosystems (Castelo-Cabay et al., 2022; Hofstede et al., 2023).
Concerning policies aimed at sustaining water ecosystem services in our local communities, a lack of long-term strategies is common (P2). Research by Wiegant (2022) and Wiegant et al. (2020) on strategies related to water ecosystem services in Ecuador shows that short-term election cycles and the desire to meet political interests at the governance scale conflict with long-term restoration timelines.
3.1.2 Economic (Ec) dimension
The limited governmental financial support (Ec1), as recognized by participants in the workshop and noted by Saud et al. (2019), is usual in most countries’ economies because environmental protection strategies are not a priority and are often neglected in government expenditure. This situation is particularly acute in developing countries, where economic and financial crises frequently result in significant cuts to public spending on the environment (Coral et al., 2021; Sarkar et al., 2007). In addition, international and private funding is insufficient to protect and restore water-related ecosystems (Wiegant et al., 2020; UNEP, 2023). While developing countries urgently need increased efforts to address the escalating crisis of freshwater resource scarcity, current initiatives and research predominantly concentrate on developed nations (Chen et al., 2019; UN-Water, 2016).
Furthermore, workshop participants considered that the focus on environmental investment in large urban centers (Ec2) impacts local communities. Policies at the national government level in environmental protection, as described by Chen et al. (2019), frequently overlook the surrounding areas of large cities. For instance, in Quito, the capital of Ecuador, around 98% of the population has access to safe drinking water, in contrast to rural areas where access is only 54% (Vinueza et al., 2021; EPMAPS, 2021). Similarly, strategies for agricultural water management are absent in many developing nations across Eastern Europe, South Asia, and South America (Chen et al., 2019).
Meanwhile, investment in environmental protection and green innovation encounters the absence of investment returns (Ec3) in our local communities. The unavailability of immediate revenue streams to offset considerable up-front costs, the lack of public accounting practices to assess the economic value of water ecosystem services (Den Heijer and Coppens, 2023) and the extended waiting period (five to 10 years or more) before reaping the benefits (Hudson et al., 2023), hinder environmental investment.
3.1.3 Social (S) dimension
Participants acknowledged that local community growth causes conflicts between water users in the upper, middle and lower basins (S1). Local community growth, inadequate land use planning and migration to urban centers exacerbate competition for water resources in the basins (Mulligan et al., 2010). It is worth noting that population migration to large cities intensifies the demand for various ecosystem services such as water, food, and others provided by rural areas to meet the needs of the urban population (Mulligan et al., 2010). As urbanization accelerates, competition for freshwater between cities and agriculture is expected to escalate, with urban water demand projected to rise by 80% by 2050 (UN-Water, 2023).
Additionally, dissatisfaction and resistance to conservation strategies (S2) arise from factors like perception outcomes and familiarity with specific practices within our local communities. Perception of ecosystem recovery from restoration projects often hinges more on aesthetic criteria than recognition of ecological quality (Junker and Buchecker, 2008). As indicated by Jourdain et al. (2023), recognizing the environmental effects of protecting aquatic ecosystems is challenging, given that the outcomes heavily rely on the diverse perspectives of multi-stakeholders with varying interests in water ecosystem services and different opinions on problem definitions and potential solutions. Similarly, different backgrounds, educational levels, and traditional beliefs can lead to varying perceptions, often resulting in misunderstandings about environmental impacts (Arsénio et al., 2020; Porras et al., 2018). Additionally, people frequently resist transitioning from conventional to environmentally friendly strategies due to economic uncertainty, financial investment, and the need to develop new skills (Rode, 2021).
3.1.4 Technological (T) dimension
Improving the health of aquatic ecosystems is a significant challenge because of the mismatches between data and technology in our local communities (T1). Scientific data regarding the effects of implemented technologies on natural resources and their benefits for humans and ecosystems are not easily accessible (Jourdain et al., 2023; Apostolaki et al., 2019; Grigg, 2016). Strategies to address this data problem, such as governments embracing mandatory environmental information disclosure, have emerged worldwide (Chen and Cho, 2019). However, the mechanisms by which this disclosure can effectively garner public support for environmental initiatives remain unclear, while decision-making processes continue to be influenced by the information available (Grigg, 2016; Glaser et al., 2021). Hence, decision-makers are either economically rational, making consistent decisions when they gather sufficient information, or economically irrational, varying their choices based on available options (Grigg, 2016; Glaser et al., 2021).
In addition, according to UN-Water (2023), the innovation with partners from the North and South could go a long way towards developing technically feasible, economically viable, socially acceptable and locally adaptable solutions to core water-related challenges. Considering patenting activity for water-related innovation has more than doubled since 1990 and is monopolised in developing countries (OECD, 2018), and the scarce investment in technology innovation (T2) within our local communities, achieving collaborative innovation remains a far-off objective.
3.1.5 Environmental (En) dimension
Our local communities heavily rely on water ecosystem services for their livelihoods (En1); nevertheless, agricultural expansion, grazing and urban development in páramo ecosystems degrade essential water ecosystem services (En2). Páramo ecosystems, along with several high-elevation ecosystems across continents, act as water towers providing numerous ecosystem services while remaining vulnerable to anthropogenic pressures and highly sensitive to climate change (Buytaert et al., 2006; Shahgedanova et al., 2021). For instance, agricultural development in páramo ecosystems is attributed to páramo soils, which have a high carbon content, a high soil moisture content, a high water retention capacity, and a high hydraulic conductivity (Buytaert et al., 2005; Calispa et al., 2021; García et al., 2020). Besides, the páramo ecosystems experience high frequencies of precipitation, which are key for agriculture, primarily attributable to orographic effects, resulting in annual sums of precipitation exceeding 1,500 mm (Crespo et al., 2019; Ilbay-Yupa et al., 2021).
In local communities, agriculture is crucial in providing ecosystem services, such as food (Mosquera et al., 2023). However, the long-term impact of agriculture significantly undermines other ecosystem services (Gordon et al., 2010). For example, water quality is affected by unsustainable agriculture, even in regions with abundant water availability (Mulligan et al., 2010). Agricultural land management, plant protection and fertilizer application contribute significantly to water pollution, as heavy rainfall leads to surface runoff, erosion and subsequent sediment transport into rivers, or nutrients, pollutants (agrochemicals) and faecal coliform bacteria are washed out and enter surface waters (Clausen and Meals, 1989; Rey-Romero et al., 2022). New data from Lu and Tian (2017) show that the use of N and P fertilizers per agricultural area has increased by a factor of eight for nitrogen and three for phosphorus since the 1960s, contributing to widespread eutrophication and the development of hypoxic zones in coastal zones (Maúre E De et al., 2021), but also to an immense spread of eutrophic conditions in inland waters (Wang et al., 2018).
At the same time, livestock grazing intensifies nutrient inputs (from manure), alters plant diversity through trampling and consumption, compacts the soil, and induces stream bank collapse, thus affecting stream morphology and aquatic and riparian zones (Muller et al., 2016). Additionally, the introduction of non-native species in the páramo, despite habitat alterations, is usual to boost economic returns in less viable agricultural areas (Buytaert et al., 2007). In the same way, urbanization significantly modifies the hydrology of basins and the transport of sediment, nutrients, and pollutants, thereby impacting water quality (Gyawali et al., 2013; Sheldon et al., 2019).
As a result, in Ecuador, aquatic ecosystems used for agriculture, livestock, fish farming, and drinking contain high levels of total coliforms, and agrochemical and metal concentrations that exceed acceptable water quality standards (Vinueza et al., 2021; Capparelli et al., 2020). It exposes the population to waterborne diseases related to faecal contamination and heavy metals, leading to significant health risks, including high morbidity and mortality (Mitra et al., 2022; Some et al., 2021). Specifically, the Ecuadorian rural sector is vulnerable, as only 48.5% have access to drinking water that meets the national regulations (INEC, 2022). Apart from the impacts on providing and regulating water ecosystem services, impacts on cultural ecosystem services affect indigenous populations due to their cosmovision regarding the meaning of water (Kleemann et al., 2022).
3.1.6 Legal (L) dimension
The right to use water must be addressed by water law so that people and organizations can have security of their access to water to meet their needs (Grigg, 2016). Nonetheless, participants reported that laws to protect aquatic ecosystems are neglected in the local communities (L1), resulting in deteriorating water quality (Vinueza et al., 2021; Zapata et al., 2021). This global concern impacts the sustainability of water ecosystem services despite efforts with modern paradigms such as stringent regulations, integrated water resources management, and sustainable sanitation (UN-Water, 2016; Jourdain et al., 2023). A significant challenge in regulation is that it aims to enforce laws to control behavior in the public interest, yet defining the public interest remains a complex and elusive objective (Grigg, 2016).
From the point of view of participants, in the local communities, there is a lack of strict sanctions against environmental violations (L2). According to Grigg (2016), water users should not expect to self-regulate, and oversight is essential to ensure that the bodies responsible for creating and enforcing rules are held accountable. In addition, specialized institutions must be vigilant to ensure that organisations that have violated the social contract, like environmental damage, are liable to bear the cost of the damage caused to society as per legitimacy theory (Buccina et al., 2013). An aspect highlighted in Wiegant (2022) is that limitations on water ecosystem services for local actors, without fair compensation, are ineffective. Instead of complying, local actors may circumvent regulations, like cutting fences around protected areas and enabling illegal grazing by livestock again.
A final point derived from participants was that regulations historically favored large-scale agriculture in crucial aquatic ecosystems (L3), thereby disadvantaging peasant farmers (Solo De Zaldívar, 2015). These regulations limit restoration strategies to cover private areas or pose problems in addressing widespread ecosystem degradation (Wiegant, 2022; Coronel, 2019; Partridge, 2016).
3.2 NbS embedded in the local communities’ PESTEL dimensions
We examined the network view maps of two specific NbS for our local communities: artificial floating islands (AFIs) and passive river restoration. The terms macrophytes in Figure 3, water quality in Figure 4, restoration in Figure 5, and governance in Figure 6, as well as their associated items, caught our attention in the network view maps, respectively. By associating these terms and items, and supported by the literature review, AFIs were characterized as (A) macrophyte-based technology and (B) a cost-effective strategy for sustaining water ecosystem services, aligning with multiple PESTEL dimensions of our local communities (Figure 2). In turn, passive river restoration complemented the PESTEL dimension that AFIs did not accommodate. Hence, it serves as a (C) mechanism to promote the natural regeneration of headwater vegetation and as a (D) strategy to govern water ecosystem services (Figure 2).
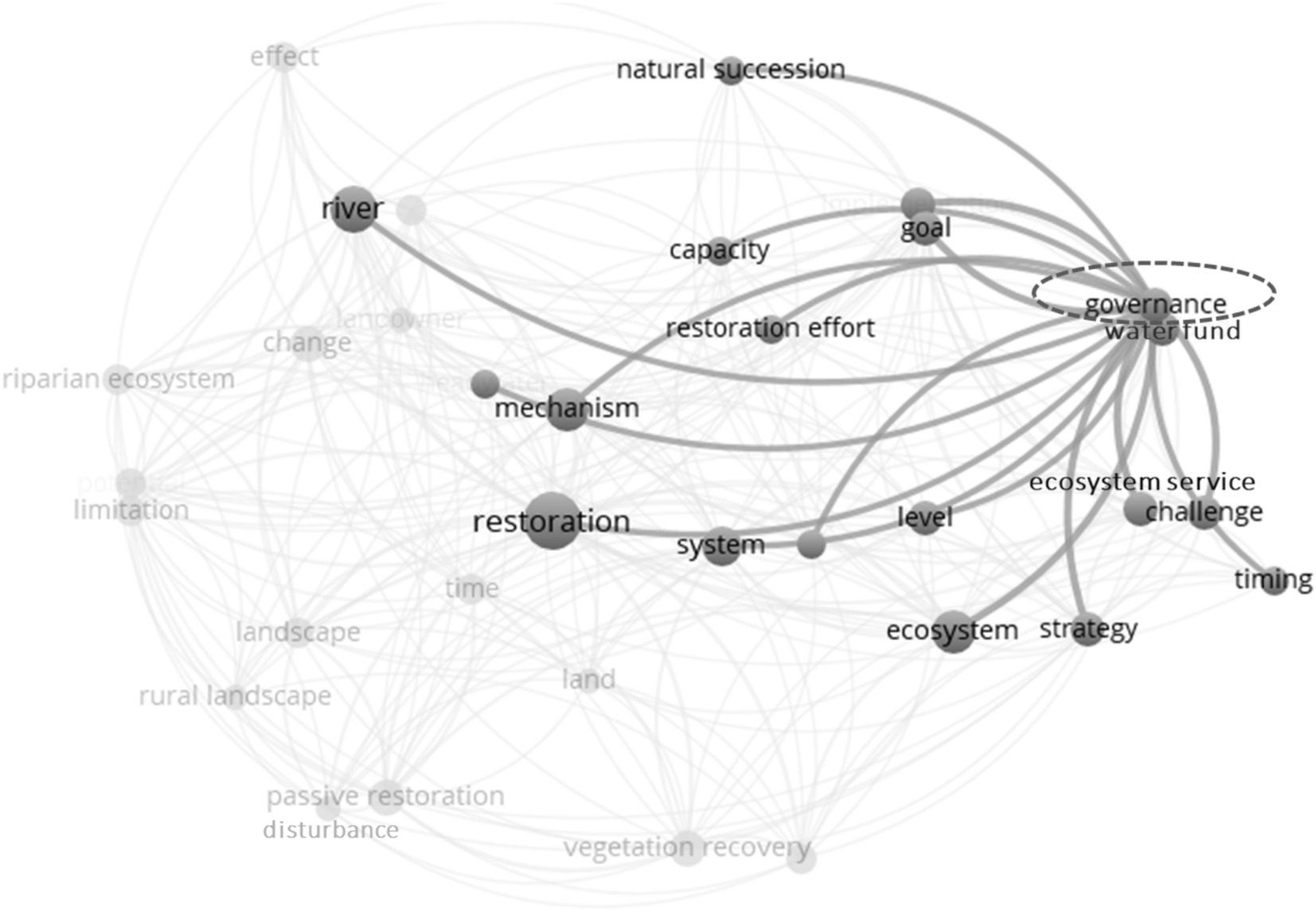
Figure 6. Choosing “governance” in the passive river restoration network view map highlights connected items.
3.2.1 Macrophyte-based technology
The term macrophyte in Figure 3 served as a crucial term for bibliometric analysis, as highlighted by Colares et al. (2020), and its items such as phytotechnology, root, shoot, mechanism, removal, system, performance, water body, time, total phosphorus, total nitrogen, chemical oxygen demand, biochemical oxygen demand, turbidity, heavy metals, and macrophytes (Canna and Juncus), lead to characterize AFIs. AFIs are one of the latest phytotechnology innovations in the remediation of degraded water bodies. Its natural mechanism for removing pollutants, low maintenance, lack of land requirements, and ease of implementation (Colares et al., 2020; Afzal et al., 2019; Prashant, 2020) make it ideal for local communities like ours, considering the scarce investment in technology innovation (T2) and the absence of long-term strategies (P3) (Figure 2).
The low operating cost of AFIs is due to the high removal efficiency, mainly dependent on macrophytes, supported by inexpensive flotation materials (Souliotis and Voulvoulis, 2022; Cui et al., 2022; Samal, 2019; Yeh et al., 2015). The main component of AFIs is macrophytes with their associated microbial communities of biofilms and zooplankton, which play a dual role in directly assimilating pollutants into their tissues and acting as catalysts for purification reactions (Cui et al., 2022; Benvenuti et al., 2018).
Floating, floating-leaf, emergent and submersed macrophytes are used to improve the polluted water bodies (De Stefani et al., 2011; Zhao et al., 2012). A broad range of plants are suitable for these purposes, with the most common genera Canna, Carex, Cyperus, Juncus, and Typha (Colares et al., 2020). Also, Phragmites australis (common reed), Oenanthe javanica (water celery), Iris pseudacorus (yellow flag), Glyceria maxima (sweet manna grass), Chrysopogon zizanioides (vetiver grass), and Ipomea aquatica (water spinach) are commonly used (Samal, 2019; De Stefani et al., 2011; Chance et al., 2022). Using different types of plants leads to diversity within the island, which results in more biodiversity, better functioning, and more stable islands (Idris et al., 2010).
Hence, AFIs restore aquatic ecosystems through mechanisms, including water self-purification, bioaccumulation, plant uptake, microbial assimilation, adsorption-sedimentation, and other pathways (Yeh et al., 2015; Hwang et al., 2020; Tanner and Headley, 2011). Consequently, a reduction in pollutants, suspended solids, oxygen demand and excessive nutrients is achieved in the water (Afzal et al., 2019; Benvenuti et al., 2018; Prashant, 2020). Similarly, concentrations of metals like arsenic, iron, lead, copper, chromium, cadmium, and nickel (Afzal et al., 2019; Fonseca et al., 2020; Gaballah et al., 2021). In addition, AFIs effectively remove bacteria such as faecal coliforms from water, reduce turbidity, and increase dissolved oxygen (DO) (Prashant, 2020; Olguín et al., 2017).
This water improvement is analysed over short-term periods such as seven days (Gaballah et al., 2021), 20 days (Guo et al., 2014), and 120 days (Fonseca et al., 2020), as well as over long-term periods including 12 months (Benvenuti et al., 2018), 18 months (Afzal et al., 2019), and 24 months (Olguín et al., 2017).
3.2.2 A cost-effective strategy for the water ecosystem services’ sustainability
The term water quality includes items such as low cost, efficiency, system, performance, water purification, wastewater, lake, reservoir, biomass, water body, application, and development, as depicted in Figure 4. This guided the alignment of AFIs for local communities heavily reliant on water ecosystem services for their livelihoods (En1), despite facing challenges of limited governmental financial support (Ec1), and focus on environmental investment in large urban centers (Ec2), as shown in Figure 2.
AFIs contribute directly to vital water ecosystem services such as irrigation water supply, fisheries, and aquaculture and indirectly to water purification and maintaining populations and habitats by reducing pollution, aligning with Target 6.3 of Sustainable Development Goal 6 in various water bodies (De Moraes et al., 2023; Fletcher et al., 2024; Masi et al., 2018).
In particular, in developing countries, AFIs have shown success in regulating severe eutrophic river conditions (Guo et al., 2014), treating oil-polluted water in pits (Afzal et al., 2019), reducing mainly pollutants in wastewater treatment plants (Benvenuti et al., 2018; Prashant, 2020), providing water for agriculture (Fonseca et al., 2020) and fisheries from reservoirs and lakes (De Moraes et al., 2023), and enhancing the aesthetic appreciation of ponds (Olguín et al., 2017).
To enhance pollutant removal, key attributes of the materials used for AFIs construction, such as buoyancy, durability, anchoring, flexibility, easy installation, affordability, and hydrophobic materials, must be considered (Samal, 2019; Sharma et al., 2021). Bamboo, PVC pipes, polypropylene pipes, polystyrene sheets, and inflatable vinyl are used for the main features of the system’s buoyancy (Samal, 2019). To fix plants and facilitate biofilm attachment, plastic nets and mediums such as rice straw, bristle coir fiber, and volcanic gravel, among others, are used (Samal, 2019; Wang et al., 2020). The selection of materials depends to a large extent on their mechanical resistance associated with biological, chemical, and weather resistance to prevent the potential degradation of materials such as plastic (De Stefani et al., 2011; Ware and Callaway, 2019).
In addition, macrophyte type, environmental conditions, water depth, buoyancy, coverage rate, macrophyte configuration mode, and periodic removal are crucial factors for ensuring optimal performance of AFIs. The selection of macrophytes is based mainly on the ability of the roots to distribute throughout the water column, on the activity of the leaves to transpire water, nutrients and heavy metal storage in plant material, and root filtration (Hwang et al., 2020; Barco and Borin, 2020). A water depth consideration is crucial depending on the type of macrophyte species planted in the water to ensure that the roots float in the aquatic ecosystem and prevent them from adhering to the sediment (Samal, 2019). Furthermore, flotation enables plants to remain unaffected by water level fluctuations (De Stefani et al., 2011). Moreover, vegetation coverage ratio and different configurations of macrophytes can improve pollutant removal. A high vegetation cover (more than 50%) may create anoxic conditions in the water as it prevents diffusion of oxygen from the air to water due to wind activity, whereas low coverage (9–18%) may add an insignificant amount of treatment effect (Samal, 2019). The diversity of macrophytes composing the AFIs is also relevant, with single-species AFIs showing lower total biomass production (Liu et al., 2014). Finally, periodic removal of plant biomass (harvest) from water bodies is essential to maintain purification efficiency and prevent pollutants from returning to the water during decomposition (Zhao et al., 2012). Harvesting above-mat vegetation is a common practice to enhance nutrient removal efficiency, considering seasonal variations and the type of macrophyte involved (Samal, 2019; Barco and Borin, 2020).
Plants on floating islands can be harvested for various purposes, including producing saleable plants that generate profits over the lifetime of AFIs, serving as animal feeds, or processing into biogas, bio-fertilizer, and bio-materials (Yeh et al., 2015; Zhao et al., 2012). Yet, questions remain about AFIs use for human food consumption due to health concerns, lack of definitive safety tests, and economic potential for reselling the whole plant post-harvest from a treatment system (Chance et al., 2022).
3.2.3 A mechanism for promoting natural regeneration of headwater vegetation
In Figure 5, the term restoration and items like mechanism, land, time, headwater, river, riparian ecosystem, vegetation recovery, landowner, stakeholder, perception, restoration effort, potential, effect, limitation, disturbance, natural succession, and rural landscape, are highlighted to align with two crucial PESTEL dimension. Agricultural expansion, grazing and urban development in páramo ecosystems degrade essential water ecosystem services (En2) due to the lack of strict sanctions against environmental violations (L2), which could be mitigated through passive river restoration (Figure 2).
Passive river restoration is particularly well-suited for headwater streams (first and second-order streams), where water quality conditions are restored by eliminating disturbance factors and allowing for natural vegetation recovery (Taniwaki et al., 2019; Trujillo-Miranda et al., 2018; Buytaert et al., 2007). Headwater streams are characterized by controlling both the structure and operation of superior-order rivers (Forget et al., 2013), often located outside the economic centre of a country, isolated and commonly neglected (Haigh and Křeček, 1991).
Passive river restoration, such as livestock enclosure, is promoted because it saves time, effort, and money and can be implemented along an entire stream, whereas active restoration often occurs only on a short river section (Jähnig et al., 2010; McIver and Starr, 2001; Prach and Hobbs, 2008). By limiting or removing sources of disturbance from streams with some existing level of bank stability and riparian vegetation, autogenic primary processes may allow some level of recovery to in-stream habitats (Hough-Snee et al., 2013). Mechanisms like fencing out livestock, fallowing cropland, removing over-abundant lianas or thinning and controlled burns in fire-suppressed forests promoting natural succession through minimal management intervention in an ecosystem offer legal, economic and environmental advantages (Arsénio et al., 2020; Brauman et al., 2019).
Land ownership, water users, and management rights are factors that can either limit or drive restoration efforts. Some actors may perceive restoration efforts as a means to assist ecosystem recovery and its biotic community, while others may prioritize more highly managed or commercially focused interventions (Chazdon et al., 2021). Also, gaining the support of mainly landowners is a challenge, as it often entails the loss of grazing land. For instance, in the Oir River basin in France, according to Muller et al. (2016), the maximum distance a river manager can handle is 1 m away from the river bank. Therefore, it is recommended that restoration efforts be integrated within a restoration governance framework to enhance the likelihood of success and sustainability of restoration programs (Sapkota et al., 2018).
3.2.4 A strategy for governing water ecosystem services
In Figure 6, the term governance and associated items such as goal, water fund, ecosystem service, restoration effort, challenge, capacity, mechanism, restoration, and system are highlighted to align several PESTEL dimensions. Thus, neglect of laws protecting aquatic ecosystems in local communities (L1), the absence of investment returns (Ec3), and conflicts between water users in the upper, middle and lower basins caused by local community growth (S1) are depicted in Figure 2.
Along a headwater stream, interactions between the stream and riparian area are tightly coupled (Richardson and Danehy, 2007), playing a crucial role in restoring aquatic ecosystems (target 6.6; SDG 6). Riparian vegetation contributes to water purification by filtering sediments, pesticides, and particulate organic matter and reduces nutrients such as nitrate and phosphorous in groundwater (Muller et al., 2016; Yang et al., 2021). It creates deep, dense root networks to protect the soil (Forget et al., 2013). In particular, in the headwaters of the important Andean river basins, natural páramo vegetation contributes to soil carbon accumulation (Mosquera et al., 2023; Brauman et al., 2019). Thus, water, soil and vegetation, the three elements of the riparian area (Yang et al., 2021), are the focus of passive river restoration to directly support the sustainability of drinking water sources and ensure access to adequate, safe, and affordable basic services, aligning with Target 11.1 of SDG 11. Indirectly, this restoration contributes to soil formation and carbon sequestration (Grizzetti et al., 2016; Mosquera et al., 2023; Khorchani et al., 2022) by reducing land degradation (target 15.3; SDG 15) (Muller et al., 2016), and promoting the conservation of mountain ecosystems (target 15.4; SDG15) (Brauman et al., 2019).
Restoration mechanisms are gradually emerging as a useful concept within the law, which can develop and enforce mechanisms to manage degraded ecosystems to restore them to desirable states (Sapkota et al., 2018). In this context, external financing mechanisms like water funds, whose goal is to invest in priority strategies such as private land acquisition, communal and private agreements, and coordinated or co-managed public lands, have facilitated long-term passive restoration efforts and managed conflicts between these actors in Ecuador and other developing countries (Coronel, 2019; Brauman et al., 2019; Castanier, 2015). Also, the focus on monetary valuation and payment mechanisms contributes to attracting political support for supporting conservation and commodifying a growing number of ecosystem services (Coral et al., 2021).
In terms of investing in passive river restoration, Brauman et al. (2019) anticipate a positive financial return over the next 20 years for Water Utility Company in Quito. This projection is based on savings in water treatment costs resulting from reduced levels of nutrients, bacteria, turbidity, and sediments in the source water. Combining this strategy with enforcement mechanisms, adapting large-scale restoration programs, re-framing conventional top-down management, and prioritizing areas for restoration contribute to the sustainability of water ecosystem services (Khorchani et al., 2022; Csákvári et al., 2022; Sippi and Parmar, 2024).
3.3 Future research directions for AFIs and passive river restoration
The network view maps of AFIs and passive river restoration facilitated alignment with multiple PESTEL dimensions of local communities (Figure 2). Nonetheless, crucial elements such as local community involvement, stakeholders, and experts were absent.
Integrating community knowledge into phytotechnology advancements could considerably influence AFIs (Fletcher et al., 2024; Gutierrez-Gines et al., 2021). Research should focus on understanding local communities’ views on systems designed by manipulating macrophyte composition to provide various ecosystem services, a significantly underexplored field (Fletcher et al., 2024; Yongabi et al., 2018). Combining local place-based perspectives with expert and scientific insight is essential in ecosystem management (Balzan et al., 2022; Gbedemah, 2023). For example, AFIs are perceived as simple in their construction, operation and maintenance; however, numerous complex processes directly influence system performance and pollutant removal efficiency. Factors such as inlet contaminant concentrations, water retention time, pH, salinity condition, and microorganisms in the rhizosphere of macrophytes impact the system (Colares et al., 2020; Yeh et al., 2015) and require expert analysis. Public participation, including local communities and experts, also helps to identify concerns on AFIs installation, such as degradation of the plastic matrix, long-term maintenance and disturbance of native macrophytes (Ware and Callaway, 2019).
Hence, AFIs as a macrophyte-based technology could incentivise multi-stakeholder involvement to address local community dissatisfaction and resistance to conservation strategies (S2) and limited local community involvement in ecosystem management (P2) in our study area (Figure 2). Involving multiple stakeholders not only helps in understanding accurate perceptions of ecosystem recovery but also provides operational and guidance information for future ecological restoration with an adaptive approach (Arsénio et al., 2020; Mercado et al., 2024). Furthermore, by enhancing local community livelihoods following Target 1.4 of SDG 1 and by mobilizing and sharing knowledge, expertise, technology, and financial resources, AFIs support the achievement of these SDGs.
Moreover, a significant knowledge gap exists regarding AFIs on headwater streams for drinking water sources, a critical necessity upon which local communities heavily rely. Only a few studies highlight AFIs implementation on aquatic vegetation near riversides and mosaic floating islands, mainly addressing the mitigation of nutrient enrichment (Yeh et al., 2015; Zhao et al., 2012). It led us to combine AFIs with passive river restoration to tackle the PESTEL dimensions that the AFIs alone do not effectively address, as shown in Figure 2. Nonetheless, the lack of political will to support strategies for maintaining healthy aquatic ecosystems (P1), the mismatches between data and technology (T1) and regulations historically favoring large-scale agriculture in crucial aquatic ecosystems (L3) of the PESTEL dimensions of local communities extends beyond the AFIs and passive river restoration. Since these challenges cannot be addressed by local community solutions alone, there is a need for future research to explore NbS in regional or national frameworks.
4 Conclusion
We developed a novel framework that demonstrates how integrating artificial floating islands (AFIs) with passive restoration addresses the complexity of local community PESTEL dimensions that influence the sustainability of water ecosystem services. AFIs, a cost-effective macrophyte-based technology, are adapted to political, economic, social, technological and environmental dimensions. They offer short- and long-term results with low investment in technology and a perspective of incorporating community plant knowledge. By improving the health of lakes, ponds and reservoirs in rural and urban landscapes in the local communities, AFIs contribute directly to vital water ecosystem services such as irrigation water supply, fisheries, and aquaculture and indirectly to water purification and maintaining populations and habitats.
Moreover, passive river restoration primarily addresses the legal dimension and complements the environmental and social dimensions AFIs cannot align. It promotes the natural regeneration of headwater vegetation to sustain drinking water sources while indirectly contributing to soil formation and carbon sequestration. At the same time, it demonstrates how restoration efforts have prompted laws to safeguard aquatic ecosystems in other local realities. Improving aquatic ecosystems also could help achieve the cultural values embedded in indigenous cosmovision.
Integrating AFIs with passive river restoration can also achieve several SDGs, including promoting sustainable and efficient natural resource use (Target 12.2, SDG 12), reducing water pollution (Target 6.3, SDG 6), restoring aquatic ecosystems (Target 6.6, SDG 6), mitigating land degradation (Target 15.3, SDG 15), and conserving mountain ecosystems (Target 15.4, SDG 15). This integration also supports sustaining drinking water sources and ensuring access to adequate, safe, and affordable basic services (Target 11.1, SDG 11), improving local community livelihoods (Target 1.4, SDG 1), and mobilizing and sharing knowledge, expertise, technology, and financial resources to further the SDGs (Target 17.16, SDG 17).
The framework will facilitate informed decision-making for water ecosystem protection by a critical NbS assessment in a developing country while remaining adaptable to countries with similar contexts. Furthermore, as a policy tool, it highlights the benefits of AFIs and passive river restoration for water ecosystem services and advocates their integration into conservation and restoration projects.
Data availability statement
The raw data supporting the conclusions of this article will be made available by the authors, without undue reservation.
Ethics statement
Written informed consent was obtained from the individual(s) for the publication of any potentially identifiable images or data included in this article.
Author contributions
KF: Conceptualization, Investigation, Methodology, Supervision, Writing – original draft, Writing – review & editing. EE-S: Methodology, Supervision, Visualization, Writing – original draft. MI-Y: Data curation, Formal analysis, Methodology, Writing – review & editing. LB: Funding acquisition, Project administration, Resources, Supervision, Visualization, Writing – original draft.
Funding
The author(s) declare that financial support was received for the research, authorship, and/or publication of this article. This project was funded by the German Academic Exchange Service (DAAD) from funds of the Federal Ministry for Economic Cooperation (BMZ), SDGnexus Network (grant number 57526248), program ‘‘exceed - Hochschulexzellenz in der Entwicklungszusammenarbeit”.
Acknowledgments
The first author would like to acknowledge Justus Liebig University Giessen and Technical University of Cotopaxi for providing the facilities for developing this research.
Conflict of interest
The authors declare that the research was conducted in the absence of any commercial or financial relationships that could be construed as a potential conflict of interest.
Publisher’s note
All claims expressed in this article are solely those of the authors and do not necessarily represent those of their affiliated organizations, or those of the publisher, the editors and the reviewers. Any product that may be evaluated in this article, or claim that may be made by its manufacturer, is not guaranteed or endorsed by the publisher.
References
Afzal, M., Rehman, K., Shabir, G., Tahseen, R., Ijaz, A., Hashmat, A. J., et al. (2019). Large-scale remediation of oil-contaminated water using floating treatment wetlands. Npj Clean Water 2:25. doi: 10.1038/s41545-018-0025-7
Albert, C., Brillinger, M., Guerrero, P., Gottwald, S., Henze, J., Schmidt, S., et al. (2021). Planning nature-based solutions: principles, steps, and insights. Ambio 50, 1446–1461. doi: 10.1007/s13280-020-01365-1
Apostolaki, S., Koundouri, P., and Pittis, N. (2019). Using a systemic approach to address the requirement for integrated water resource management within the water framework directive. Sci. Total Environ. 679, 70–79. doi: 10.1016/j.scitotenv.2019.05.077
Arsénio, P., Rodríguez-González, P., Bernez, I., Dias, F., Bugalho, M., and Dufour, S. (2020). Riparian vegetation restoration: does social perception reflect ecological value? River Res. Appl. 36, 907–920. doi: 10.1002/rra.3514
Asamblea Nacional . (2014) Ley Orgánica de Recursos Hídricos Usos y Aprovechamiento del Agua. Registro Oficial-Ecuador.
Balzan, M. V., Geneletti, D., Grace, M., de Santis, L., Tomaskinova, J., Reddington, H., et al. (2022). Assessing nature-based solutions uptake in a Mediterranean climate: insights from the case-study of Malta. Nat. Based Solut. 2:100029. doi: 10.1016/j.nbsj.2022.100029
Barco, A., and Borin, M. (2020). Treatment performances of floating wetlands: a decade of studies in North Italy. Ecol. Eng. 158:106016. doi: 10.1016/j.ecoleng.2020.106016
Baustian, M. M., Jung, H., Bienn, H. C., Barra, M., Hemmerling, S. A., Wang, Y., et al. (2020). Engaging coastal community members about natural and nature-based solutions to assess their ecosystem function. Ecol. Eng. X 143:100015. doi: 10.1016/j.ecoena.2019.100015
BCE (2021) Cuentas nacionales regionales serie anuales de cuentas provinciales. Banco Central del Ecuador.
Benvenuti, T., Hamerski, F., Giacobbo, A., Bernardes, A. M., Zoppas-Ferreira, J., and Rodrigues, M. A. S. (2018). Constructed floating wetland for the treatment of domestic sewage: a real-scale study. J. Environ. Chem. Eng. 6, 5706–5711. doi: 10.1016/j.jece.2018.08.067
Bisaga, I., Parikh, P., and Tomei, J.To LS (2021). Mapping synergies and trade-offs between energy and the sustainable development goals: a case study of off-grid solar energy in Rwanda. Energy Policy 149:112028. doi: 10.1016/j.enpol.2020.112028
Blahna, D. J., Asah, S. T., and Deal, R. L. (2017) An ecosystem services framework. In: D. H. Olson and B. HorneVan (eds) People, forests, and change: Lessons from the Pacific northwest. Island Press, Washington, DC, pp. 62–75.
Brauman, K. A., Benner, R., Benitez, S., Bremer, L., and Vigerstøl, K. (2019). “Water Funds” in Green growth that works. 1st ed (Washington, DC: Island Press), 319.
Buccina, S., Chene, D., and Gramlich, J. (2013). Accounting for the environmental impacts of Texaco’s operations in Ecuador: Chevron’s contingent environmental liability disclosures. Account. Forum 37, 110–123. doi: 10.1016/j.accfor.2013.04.003
Buytaert, W., Célleri, R., DeBièvre, B., Cisneros, F., Wyseure, G., and Deckers, J. (2006). Human impact on the hydrology of the Andean páramos. Earth Sci. Rev. 79, 53–72. doi: 10.1016/j.earscirev.2006.06.002
Buytaert, W., Iñiguez, V., and Bièvre, B. D. (2007). The effects of afforestation and cultivation on water yield in the Andean páramo. For. Ecol. Manag. 251, 22–30. doi: 10.1016/j.foreco.2007.06.035
Buytaert, W., Sevink, J., De Leeuw, B., and Deckers, J. (2005). Clay mineralogy of the soils in the south Ecuadorian páramo region. Geoderma 127, 114–129. doi: 10.1016/j.geoderma.2004.11.021
Calispa, M., van Ypersele, R., Pereira, B., et al. (2021) Soil organic carbon stocks under different páramo vegetation covers in Ecuador’s northern Andes. Repositorio Digital Institucional de la Escuela Politécnica Nacional.
Capparelli, M. V., Moulatlet, G. M., Abessa, D. M. S., Lucas-Solis, O., Rosero, B., Galarza, E., et al. (2020). An integrative approach to identify the impacts of multiple metal contamination sources on the eastern Andean foothills of the Ecuadorian Amazonia. Sci. Total Environ. 709:136088. doi: 10.1016/j.scitotenv.2019.136088
Carroll, J., Beck, J., Boyer, E., Dhanorkar, S., and Gupta, S. (2019). Empowering community water data stakeholders. Interact. Comput. 31, 492–506. doi: 10.1093/iwcomp/iwz032
Castanier, H. (2015). “Economic valuation for decision making on the protection of water sources” in Conflict resolution in water resources and environmental management. eds. K. W. Hipel, L. Fang, J. Cullmann, and M. Bristow (Cham: Springer International Publishing), 81–108.
Castelo-Cabay, M., Piedra-Fernandez, J. A., and Ayala, R. (2022). Deep learning for land use and land cover classification from the Ecuadorian Paramo. Int. J. Digit. Earth 15, 1001–1017. doi: 10.1080/17538947.2022.2088872
Chance, L., Hall, C., and White, S. (2022). Viability assessment for the use of floating treatment wetlands as alternative production and remediation systems for nursery and greenhouse operations. J. Environ. Manag. 305:114398. doi: 10.1016/j.jenvman.2021.114398
Chazdon, R. L., Falk, D. A., Banin, L. F., Wagner, M., Wilson, S., Grabowski, R. C., et al. (2021). The intervention continuum in restoration ecology: rethinking the active–passive dichotomy. Restor Ecol. 32:e13535. doi: 10.1111/rec.13535
Chen, W., and Cho, F. (2019). Environmental information disclosure and societal preferences for urban river restoration: latent class modelling of a discrete-choice experiment. J. Clean. Prod. 231, 1294–1306. doi: 10.1016/j.jclepro.2019.05.307
Chen, W., He, B., Nover, D., Lu, H., Liu, J., Sun, W., et al. (2019). Farm ponds in southern China: challenges and solutions for conserving a neglected wetland ecosystem. Sci. Total Environ. 659, 1322–1334. doi: 10.1016/j.scitotenv.2018.12.394
Clausen, J. C., and Meals, D. W. (1989). Water quality achievable with agricultural best management practices. J. Soil Water Conserv. 44, 593–596.
Colares, G. S., Dell’Osbel, N., Wiesel, P. G., Oliveira, G. A., Lemos, P. H. Z., da Silva, F. P., et al. (2020). Floating treatment wetlands: a review and bibliometric analysis. Sci. Total Environ. 714:136776. doi: 10.1016/j.scitotenv.2020.136776
Conable, J. (2015). “Foreign land acquisitions, corruption, and sustainable livelihood in sub-Saharan Africa: the cases of Mozambique and Tanzania” in Handbook of research on in-country determinants and implications of foreign land acquisitions. IGI Global Scientific Publishing: IGI global. ed. E. Osabuohien .
Coral, C., Bokelmann, W., Bonatti, M., Carcamo, R., and Sieber, S. (2021). Understanding institutional change mechanisms for land use: lessons from Ecuador’s history. Land Use Policy 108:105530. doi: 10.1016/j.landusepol.2021.105530
Corburn, J. (2007). Community knowledge in environmental health science: co-producing policy expertise. Environ. Sci. Pol. 10, 150–161. doi: 10.1016/j.envsci.2006.09.004
Crespo, M., Rodríguez Díaz, J. A., García Morillo, J., and McNabola, A. (2019). Pump-as-turbine selection methodology for energy recovery in irrigation networks: Minimising the payback period. Water 11:149. doi: 10.3390/w11010149
Csákvári, E., Molnár, Z., and Halassy, M. (2022). Estimates of regeneration potential in the Pannonian sand region help prioritize ecological restoration interventions. Commun. Biol. 5:1136. doi: 10.1038/s42003-022-04047-8
Cui, H., Yang, Y., Zhang, X., Dong, L., Yang, Y., Huang, M., et al. (2022). Nitrogen removal and microbial mechanisms in a novel tubular bioreactor-enhanced floating treatment wetland for the treatment of high nitrate river water. Water Environ. Res. 94:e10767. doi: 10.1002/wer.10767
Culhane, F., Teixeira, H., Nogueira, A. J. A., Borgwardt, F., Trauner, D., Lillebø, A., et al. (2019). Risk to the supply of ecosystem services across aquatic ecosystems. Sci. Total Environ. 660, 611–621. doi: 10.1016/j.scitotenv.2018.12.346
De Moraes, K. R., Souza, A. T., Muška, M., Hladík, M., Čtvrtlíková, M., Draštík, V., et al. (2023). Artificial floating islands: a promising tool to support juvenile fish in lacustrine systems. Hydrobiologia 850, 1969–1984. doi: 10.1007/s10750-023-05204-8
De Stefani, G., Tocchetto, D., Salvato, M., and Borin, M. (2011). Performance of a floating treatment wetland for in-stream water amelioration in NE Italy. Hydrobiologia 674, 157–167. doi: 10.1007/s10750-011-0730-4
Den Heijer, C., and Coppens, T. (2023). Paying for green: a scoping review of alternative financing models for nature-based solutions. J. Environ. Manag. 337:117754. doi: 10.1016/j.jenvman.2023.117754
Donthu, N., Kumar, S., Mukherjee, D., Pandey, N., and Lim, W. M. (2021). How to conduct a bibliometric analysis: an overview and guidelines. J. Bus. Res. 133, 285–296. doi: 10.1016/j.jbusres.2021.04.070
Emich, K. J., Kumar, S., Lu, L., Norder, K., and Pandey, N. (2020). Mapping 50 years of small group research through small group research. Small Group Res. 51, 659–699. doi: 10.1177/1046496420934541
Fletcher, J., Willby, N., Oliver, D., and Quilliam, R. S. (2024). Engineering aquatic plant community composition on floating treatment wetlands can increase ecosystem multifunctionality. Environ. Res. 243:117818. doi: 10.1016/j.envres.2023.117818
Fonseca, K. M., Ruiz Depablos, J. L., Espitia-Sarmiento, E. F., and Llugsha Moreta, N. M. (2020). Artificial floating island with vetiver for treatment of arsenic-contaminated water: a real scale study in high-Andean reservoir. Water 12:3086. doi: 10.3390/w12113086
Fonseca, K., Ruiz, J., Espitia, E., Campaña, E., and Moreno, E. (2021). Phytoremediation of arsenic-contaminated waters by artificial floating island: literature review. Rev. Fac. Agron. Univ. Zulia 38, 199–215. doi: 10.47280/RevFacAgron(LUZ).v38.n1.010
Forget, G., Carreau, C., Le Coeur, D., and Bernez, I. (2013). Ecological restoration of headwaters in a rural landscape (Normandy, France): a passive approach taking hedge networks into account for riparian tree recruitment. Restor. Ecol. 21, 96–104. doi: 10.1111/j.1526-100X.2012.00868.x
Gaballah, M. S., Ismail, K., Aboagye, D., Ismail, M. M., Sobhi, M., and Stefanakis, A. I. (2021). Effect of design and operational parameters on nutrients and heavy metal removal in pilot floating treatment wetlands with Eichhornia Crassipes treating polluted lake water. Environ. Sci. Pollut. Res. 28, 25664–25678. doi: 10.1007/s11356-021-12442-7
García, V. J., Márquez, C. O., Isenhart, T. M., Rodríguez, M., Crespo, S. D., and Cifuentes, A. G. (2019). Evaluating the conservation state of the páramo ecosystem: an object-based image analysis and CART algorithm approach for Central Ecuador. Heliyon 5:e02701. doi: 10.1016/j.heliyon.2019.e02701
García, V. J., Márquez, C. O., Rodríguez, M. V., Orozco, J. J., Aguilar, C. D., and Ríos, A. C. (2020). Páramo ecosystems in Ecuador’s southern region: conservation state and restoration. Agronomy 10:1922. doi: 10.3390/agronomy10121922
Gbedemah, S. F. (2023). Eruditing from indigenous adaptation strategies for resilient and sustainable coastal erosion management in southeastern Ghana. Discov. Sustain. 4:12. doi: 10.1007/s43621-023-00123-z
Giordano, R., Pluchinotta, I., Pagano, A., Scrieciu, A., and Nanu, F. (2020). Enhancing nature-based solutions acceptance through stakeholders’ engagement in co-benefits identification and trade-offs analysis. Sci. Total Environ. 713:136552. doi: 10.1016/j.scitotenv.2020.136552
Glaser, G. L., Miller, M. C., Healy, S. D., and Shuker, D. M. (2021). The rationality of decisions depends on behavioural context. Behav. Process. 182:104293. doi: 10.1016/j.beproc.2020.104293
Gordon, L. J., Finlayson, C. M., and Falkenmark, M. (2010). Managing water in agriculture for food production and other ecosystem services. Agric. Water Manag. 97, 512–519. doi: 10.1016/j.agwat.2009.03.017
Grigg, N. S. (2016). “Social aspects of water management” in Integrated water resource management: An interdisciplinary approach. ed. N. S. Grigg (London: Palgrave Macmillan UK), 319–338.
Grizzetti, B., Lanzanova, D., Liquete, C., Reynaud, A., and Cardoso, A. C. (2016). Assessing water ecosystem services for water resource management. Environ. Sci. Pol. 61, 194–203. doi: 10.1016/j.envsci.2016.04.008
Guo, Y., Liu, Y., Zeng, G., Hu, X., Li, X., Huang, D., et al. (2014). A restoration-promoting integrated floating bed and its experimental performance in eutrophication remediation. J. Environ. Sci. 26, 1090–1098. doi: 10.1016/S1001-0742(13)60500-8
Gutierrez-Gines, M. J., Alizadeh, H., Alderton, E., Ambrose, V., Meister, A., Robinson, B. H., et al. (2021). Phytoremediation of microbial contamination in soil by New Zealand native plants. Appl. Soil Ecol. 167:104040. doi: 10.1016/j.apsoil.2021.104040
Gyawali, S., Techato, K., Monprapussorn, S., and Yuangyai, C. (2013). Integrating land use and water quality for environmental based land use planning for U-tapao River Basin, Thailand. Proc. Soc. Behav. Sci. 91, 556–563. doi: 10.1016/j.sbspro.2013.08.454
Haigh, M. J., and Křeček, J. (1991). Headwater management: problems and policies. Land Use Policy 8, 171–176. doi: 10.1016/0264-8377(91)90028-H
Hoffman, K. N. (2023). Justifying nature-based solutions. Biol. Philos. 38, 1–15. doi: 10.1007/s10539-023-09926-w
Hofstede, R., Calles, J., López, V., Polanco, R., Torres, F., and Ulloa, J. (2014). Estado de conocimiento sobre el impacto del cambio climático en el ecosistema páramo. UICN
Hofstede, R., Mena-Vásconez, P., and Suárez, E. (2023). Los páramos del Ecuador Pasado, presente y futuro. Ecuador: USFQ Press.
Hong, H. Y., and Scardamalia, M. (2014). Community knowledge assessment in a knowledge building environment. Comput. Educ. 71, 279–288. doi: 10.1016/j.compedu.2013.09.009
Hough-Snee, N., Roper, B. B., Wheaton, J. M., Budy, P., and Lokteff, R. L. (2013). Riparian vegetation communities change rapidly following passive restoration at a northern Utah stream. Ecol. Eng. 58, 371–377. doi: 10.1016/j.ecoleng.2013.07.042
Hudson, G., Hart, S., and Verbeek, A. (2023) Investing in nature-based solutions. European Investment Bank.
Hwang, J.-I., Li, Z., Andreacchio, N., Ordonez Hinz, F., and Wilson, P. C. (2020). Potential use of floating treatment wetlands established with Canna flaccida for removing organic contaminants from surface water. Int. J. Phytoremediation 22, 1304–1312. doi: 10.1080/15226514.2020.1768511
Idris, A., Liew, A., Hung, Y.-T., and Wang, L. (2010). “Wetlands for wastewater treatment” in Environmental Bioengineering. eds. M. Nemati and C. Webb (Totowa, NJ: Humana Press).
Ilbay-Yupa, M., Ilbay, F., Zubieta, R., García-Mora, M., and Chasi, P. (2021). Impacts of climate change on the precipitation and streamflow regimes in equatorial regions: Guayas River basin. Water 13:3138. doi: 10.3390/w13213138
INEC (2019). Boletín Técnico. Encuesta Nacional de Salud y Nutrición. Indicadores de Salud y Nutrición de la población ecuatorian. Instituto Nacional de Estadística y Censos -Ecuador. INEC.
INEC (2022) Cifras Claves del Censo del Ecuador 2022. Available at: https://inec.censoecuador.gob.ec/public/BoletinNacional_SegundaPublicacion.html#414_Distribuci%C3%B3n_geogr%C3%A1fica (Accessed December 11, 2023).
InfoMIES (2022) Información Estadística. Aseguramiento no contributivo. Consulta de datos interactivos. MIES.
Jähnig, S. C., Brabec, K., Buffagni, A., Erba, S., Lorenz, A. W., Ofenböck, T., et al. (2010). A comparative analysis of restoration measures and their effects on hydromorphology and benthic invertebrates in 26 central and southern European rivers. J. Appl. Ecol. 47, 671–680. doi: 10.1111/j.1365-2664.2010.01807.x
Jarosiewicz, P., Fazi, S., and Zalewski, M. (2022). How to boost Ecohydrological nature-based solutions in water quality management. Ecohydrol. Hydrobiol. 22, 226–233. doi: 10.1016/j.ecohyd.2021.11.005
Jourdain, D., Namakando, N., Mungatana, E., Mirzabaev, A., and Njiraini, G. (2023). Revealing salient aquatic ecosystem services bundles in the Olifants River catchment, SouthAfrica. Wetl. Ecol. Manag. 31, 267–286. doi: 10.1007/s11273-023-09915-6
Junker, B., and Buchecker, M. (2008). Aesthetic preferences versus ecological objectives in river restorations. Landsc. Urban Plan. 85, 141–154. doi: 10.1016/j.landurbplan.2007.11.002
Kansongue, N., Njuguna, J., and Vertigans, S. (2023). A PESTEL and SWOT impact analysis on renewable energy development in Togo. Front. Sustain. 3:990173. doi: 10.3389/frsus.2022.990173
Keesstra, S., Nunes, J., Novara, A., Finger, D., Avelar, D., Kalantari, Z., et al. (2018). The superior effect of nature based solutions in land management for enhancing ecosystem services. Sci. Total Environ. 611, 997–1009. doi: 10.1016/j.scitotenv.2017.08.077
Kenter, J. O., Hyde, T., Christie, M., and Fazey, I. (2011). The importance of deliberation in valuing ecosystem services in developing countries—evidence from the Solomon Islands. Glob. Environ. Change 21, 505–521. doi: 10.1016/j.gloenvcha.2011.01.001
Khatibi, F. S., Dedekorkut-Howes, A., Howes, M., and Torabi, E. (2021). Can public awareness, knowledge and engagement improve climate change adaptation policies? Discov. Sustain. 2:18. doi: 10.1007/s43621-021-00024-z
Khorchani, M., Nadal-Romero, E., Lasanta, T., and Tague, C. (2022). Carbon sequestration and water yield tradeoffs following restoration of abandoned agricultural lands in Mediterranean mountains. Environ. Res. 207:112203. doi: 10.1016/j.envres.2021.112203
Kleemann, J., Koo, H., Hensen, I., Mendieta-Leiva, G., Kahnt, B., Kurze, C., et al. (2022). Priorities of action and research for the protection of biodiversity and ecosystem services in continental Ecuador. Biol. Conserv. 265:109404. doi: 10.1016/j.biocon.2021.109404
Liquete, C., Udias, A., Conte, G., Grizzetti, B., and Masi, F. (2016). Integrated valuation of a nature-based solution for water pollution control, highlighting hidden benefits. Ecosyst. Serv. 22, 392–401. doi: 10.1016/j.ecoser.2016.09.011
Liu, J., Liu, J., Zhang, R., Zou, Y., Wang, H., and Zhang, Z. (2014). Impacts of aquatic macrophytes configuration modes on water quality. Water Sci. Technol. 69, 253–261. doi: 10.2166/wst.2013.573
Lu, C., and Tian, H. (2017). Global nitrogen and phosphorus fertilizer use for agriculture production in the past half century: shifted hot spots and nutrient imbalance. Earth Syst. Sci. Data 9, 181–192. doi: 10.5194/essd-9-181-2017
Lynam, T., DeJong, W., Sheil, D., Kusumanto, T., and Evans, K. (2007). A review of tools for incorporating community knowledge, preferences, and values into decision making in natural resources management. Ecol. Soc. 12:art5. doi: 10.5751/ES-01987-120105
Masi, F., Rizzo, A., and Regelsberger, M. (2018). The role of constructed wetlands in a new circular economy, resource oriented, and ecosystem services paradigm. J. Environ. Manag. 216, 275–284. doi: 10.1016/j.jenvman.2017.11.086
Maúre E De, R., Terauchi, G., Ishizaka, J., Clinton, N., and De Witt, M. (2021). Globally consistent assessment of coastal eutrophication. Nat. Commun. 12:6142. doi: 10.1038/s41467-021-26391-9
McIver, J., and Starr, L. (2001). Restoration of degraded lands in the interior Columbia River basin: passive vs. active approaches. For. Ecol. Manag. 153, 15–28. doi: 10.1016/S0378-1127(01)00451-0
Mercado, G., Wild, T., Hernandez-Garcia, J., Baptista, M. D., van Lierop, M., Bina, O., et al. (2024). Supporting nature-based solutions via nature-based thinking across European and Latin American cities. Ambio 53, 79–94. doi: 10.1007/s13280-023-01920-6
Mitra, S., Chakraborty, A. J., Tareq, A. M., Emran, T. B., Nainu, F., Khusro, A., et al. (2022). Impact of heavy metals on the environment and human health: novel therapeutic insights to counter the toxicity. J. King Saud. Univ. Sci. 34:101865. doi: 10.1016/j.jksus.2022.101865
Molle, F. (2008) Nirvana concepts, Narratives and Policy Models. Water Alternatives: Insights from the Water Sector.
Mosquera, G. M., Hofstede, R., Bremer, L. L., Asbjornsen, H., Carabajo-Hidalgo, A., Célleri, R., et al. (2023). Frontiers in páramo water resources research: a multidisciplinary assessment. Sci. Total Environ. 892:164373. doi: 10.1016/j.scitotenv.2023.164373
Muller, I., Delisle, M., Ollitrault, M., and Bernez, I. (2016). Responses of riparian plant communities and water quality after 8 years of passive ecological restoration using a BACI design. Hydrobiologia 781, 67–79. doi: 10.1007/s10750-015-2349-3
Mulligan, M., Rubiano, J., Hyman, G., White, D., Garcia, J., Saravia, M., et al. (2010). The Andes basins: biophysical and developmental diversity in a climate of change. Water Int. 35, 472–492. doi: 10.1080/02508060.2010.516330
Nahuelhual, L., Saavedra, G., Henríquez, F., Benra, F., Vergara, X., Perugache, C., et al. (2018). Opportunities and limits to ecosystem services governance in developing countries and indigenous territories: the case of water supply in southern Chile. Environ. Sci. Pol. 86, 11–18. doi: 10.1016/j.envsci.2018.04.012
OECD (2018). OECD water governance Indicator framework in implementing the OECD Principles on Water Governance. France: OECD.
Olguín, E., Sánchez-Galván, G., Melo, F., Hernández, V., and González-Portela, R. (2017). Long-term assessment at field scale of floating treatment wetlands for improvement of water quality and provision of ecosystem services in a eutrophic urban pond. Sci. Total Environ. 585, 561–571. doi: 10.1016/j.scitotenv.2017.01.072
Oral, H. V., Carvalho, P., Gajewska, M., Ursino, N., Masi, F., Kazak, J. K., et al. (2020). A review of nature-based solutions for urban water management in European circular cities: a critical assessment based on case studies and literature. Blue Green Syst. 2, 112–136. doi: 10.2166/bgs.2020.932
Pagano, A., Pluchinotta, I., Pengal, P., Cokan, B., and Giordano, R. (2019). Engaging stakeholders in the assessment of NBS effectiveness in flood risk reduction: a participatory system dynamics model for benefits and co-benefits evaluation. Sci. Total Environ. 690, 543–555. doi: 10.1016/j.scitotenv.2019.07.059
Partridge, T. (2016). Water justice and food sovereignty in Cotopaxi, Ecuador. Environ. Just. 9, 49–52. doi: 10.1089/env.2016.0003
Porras, G., Stringer, L., and Quinn, C. (2018). Unravelling stakeholder perceptions to enable adaptive water governance in dryland systems. Water Resour. Manag. 32, 3285–3301. doi: 10.1007/s11269-018-1991-8
Possantti, I., and Marques, G. (2022). A modelling framework for nature-based solutions expansion planning considering the benefits to downstream urban water users. Environ. Model. Softw. 152:105381. doi: 10.1016/j.envsoft.2022.105381
Prach, K., and Hobbs, R. J. (2008). Spontaneous succession versus technical reclamation in the restoration of disturbed sites. Restor. Ecol. 16, 363–366. doi: 10.1111/j.1526-100X.2008.00412.x
Prashant, B. S. K. (2020). Macroinvertebrates associated with artificial floating islands installed in river Kshipra for water quality improvement. Water Sci. Technol. 81, 1242–1249. doi: 10.2166/wst.2020.219
Rey-Romero, D. C., Domínguez, I., and Oviedo-Ocaña, E. R. (2022). Effect of agricultural activities on surface water quality from páramo ecosystems. Environ. Sci. Pollut. Res. 29, 83169–83190. doi: 10.1007/s11356-022-21709-6
Richardson, J. S., and Danehy, R. J. (2007). A synthesis of the ecology of headwater streams and their riparian zones in temperate forests. For. Sci. 53, 131–147. doi: 10.1093/forestscience/53.2.131
Rode, J. (2021). When payments for ecosystem conservation stop. Nat. Sustain. 5, 15–16. doi: 10.1038/s41893-021-00812-5
Salmoral, G., Khatun, K., Llive, F., and Lopez, C. M. (2018). Agricultural development in Ecuador: a compromise between water and food security? J. Clean. Prod. 202, 779–791. doi: 10.1016/j.jclepro.2018.07.308
Samal, K. (2019). Ecological floating bed (EFB) for decontamination of polluted water bodies_ design, mechanism and performance. J. Environ. Manag. 13, 1–13. doi: 10.1016/j.jenvman.2019.109550
Sapkota, R. P., Stahl, P. D., and Rijal, K. (2018). Restoration governance: an integrated approach towards sustainably restoring degraded ecosystems. Environ. Dev. 27, 83–94. doi: 10.1016/j.envdev.2018.07.001
Sarkar, S. K., Saha, M., Takada, H., Bhattacharya, A., Mishra, P., and Bhattacharya, B. (2007). Water quality management in the lower stretch of the river Ganges, east coast of India: an approach through environmental education. J. Clean. Prod. 15, 1559–1567. doi: 10.1016/j.jclepro.2006.07.030
Saud, M., Guo, P., Haq, I., Pan, G., and Khan, A. (2019). Do government expenditure and financial development impede environmental degradation in Venezuela? PLoS One 14:e0210255. doi: 10.1371/journal.pone.0210255
Shahgedanova, M., Adler, C., Gebrekirstos, A., Grau, H. R., Huggel, C., Marchant, R., et al. (2021). Mountain observatories: status and prospects for enhancing and connecting a global community. Mt. Res. Dev. 41:54. doi: 10.1659/MRD-JOURNAL-D-20-00054.1
Sharma, R., Vymazal, J., and Malaviya, P. (2021). Application of floating treatment wetlands for stormwater runoff: a critical review of the recent developments with emphasis on heavy metals and nutrient removal. Sci. Total Environ. 777:146044. doi: 10.1016/j.scitotenv.2021.146044
Sheldon, F., Leigh, C., Neilan, W., Newham, M., Polson, C., and Hadwen, W. (2019). “Chapter 11 - urbanization: hydrology, water quality, and influences on ecosystem health” in Approaches to water sensitive Urban Design. eds. A. K. Sharma, T. Gardner, and D. Begbie (Sawston, Cambridge: Woodhead Publishing), 229–248.
Sippi, S., and Parmar, D. (2024). Water quality simulation under river restoration measures for the Delhi stretch of river Yamuna, India. Environ. Dev. Sustain. 26, 5891–5914. doi: 10.1007/s10668-023-02943-5
Skrydstrup, J., Madsen, H., Lowe, R., Gregersen, I., Pedersen, A., and Arnbjerg-Nielsen, K. (2020). Incorporating objectives of stakeholders in strategic planning of urban water management. Urban Water J. 17, 87–99. doi: 10.1080/1573062X.2020.1748204
Solo De Zaldívar, V. B. (2015). Tempest in the Andes? Part 1: agrarian reform and peasant differentiation in Cotopaxi (Ecuador): agrarian reform and peasant differentiation in Cotopaxi (Ecuador). J. Agrar Change 15, 89–115. doi: 10.1111/joac.12072
Some, S., Mondal, R., Mitra, D., Jain, D., Verma, D., and das, S. (2021). Microbial pollution of water with special reference to coliform bacteria and their nexus with environment. Energy Nexus 1:100008. doi: 10.1016/j.nexus.2021.100008
Souliotis, I., and Voulvoulis, N. (2022). Operationalising nature-based solutions for the design of water management interventions. Nat. Based Solut. 2:100015. doi: 10.1016/j.nbsj.2022.100015
Sowińska-Świerkosz, B., and García, J. (2022). What are nature-based solutions (NBS)? Setting core ideas for concept clarification. Nat. Based Solut. 2:100009. doi: 10.1016/j.nbsj.2022.100009
Taniwaki, R. H., Cassiano, C. C., Fransozi, A. A., Vásquez, K. V., Posada, R. G., Velásquez, G. V., et al. (2019). Effects of land-use changes on structural characteristics of tropical high-altitude Andean headwater streams. Limnologica 74, 1–7. doi: 10.1016/j.limno.2018.10.002
Tanner, C. C., and Headley, T. R. (2011). Components of floating emergent macrophyte treatment wetlands influencing removal of stormwater pollutants. Ecol. Eng. 37, 474–486. doi: 10.1016/j.ecoleng.2010.12.012
Telwala, Y. (2023). Unlocking the potential of agroforestry as a nature-based solution for localizing sustainable development goals: a case study from a drought-prone region in rural India. Nat. Based Solut. 3:100045. doi: 10.1016/j.nbsj.2022.100045
Thompson, J. B., Zurita-Arthos, L., Müller, F., Chimbolema, S., and Suárez, E. (2021). Land use change in the Ecuadorian páramo: the impact of expanding agriculture on soil carbon storage. Arct. Antarct. Alp. Res. 53, 48–59. doi: 10.1080/15230430.2021.1873055
Trujillo-Miranda, A. L., Toledo-Aceves, T., López-Barrera, F., and Gerez-Fernández, P. (2018). Active versus passive restoration: recovery of cloud forest structure, diversity and soil condition in abandoned pastures. Ecol. Eng. 117, 50–61. doi: 10.1016/j.ecoleng.2018.03.011
UNCTAD (2022) Now 8 billion and counting: where the world’s population has grown most and why that matters. Available at: https://unctad.org/data-visualization/now-8-billion-and-counting-where-worlds-population-has-grown-most-and-why (Accessed December 13, 2023).
UNEP (2023). Adaptation gap report 2023: Underfinanced: underprepared Inadequate investment and planning on climate adaptation leaves world exposed. Nairobi, Kenya: United Nations Environment Programme. Available at: https://www.unep.org/un-2023-water-conference
UN-Water (2016) Towards a worldwide assessment of freshwater quality: A UN-water analytical brief. Available at: https://www.unwater.org/publications/towards-worldwide-assessment-freshwater-quality (Accessed February 22, 2024).
UN-Water (2023) Partnerships and cooperation for water | UN world water development report 2023. Available at: https://www.unesco.org/reports/wwdr/2023/en (Accessed February 22, 2024).
Vinueza, D., Ochoa-Herrera, V., Maurice, L., Tamayo, E., Mejía, L., Tejera, E., et al. (2021). Determining the microbial and chemical contamination in Ecuador’s main rivers. Sci. Rep. 11:17640. doi: 10.1038/s41598-021-96926-z
Vollmer, D., Burkhard, K., Adem Esmail, B., Guerrero, P., and Nagabhatla, N. (2022). Incorporating ecosystem services into water resources management—tools, policies, promising pathways. Environ. Manag. 69, 627–635. doi: 10.1007/s00267-022-01640-9
Wang, S., Li, J., Zhang, B., Spyrakos, E., Tyler, A. N., Shen, Q., et al. (2018). Trophic state assessment of global inland waters using a MODIS-derived Forel-Ule index. Remote Sens. Environ. 217, 444–460. doi: 10.1016/j.rse.2018.08.026
Wang, W.-H., Wang, Y., Sun, L.-Q., Zheng, Y. C., and Zhao, J. C. (2020). Research and application status of ecological floating bed in eutrophic landscape water restoration. Sci. Total Environ. 704:135434. doi: 10.1016/j.scitotenv.2019.135434
Ware, J., and Callaway, R. (2019). Public perception of coastal habitat loss and habitat creation using artificial floating islands in the UK. PLoS One 14:e0224424. doi: 10.1371/journal.pone.0224424
Warner, J. F., Wester, P., and Hoogesteger, J. (2014). Struggling with scales: revisiting the boundaries of river basin management: struggling with scale. Wiley Interdiscip. Rev. Water 1, 469–481. doi: 10.1002/wat2.1035
Wiegant, D. (2022). Ecuadorian water funds’ use of scale-sensitive strategies to stay on course in forest and landscape restoration governance. J. Environ. Manag. 311:114850. doi: 10.1016/j.jenvman.2022.114850
Wiegant, D., Peralvo, M., van Oel, P., and Dewulf, A. (2020). Five scale challenges in Ecuadorian forest and landscape restoration governance. Land Use Policy 96:104686. doi: 10.1016/j.landusepol.2020.104686
Yang, G., Li, Y., Huang, T., Fu, B., Tang, J., Zhang, X., et al. (2021). Multi-scale evaluation of ecological restoration effects in the riparian zone using Landsat series images from 1980 to 2019. Ecol. Indic. 132:108342. doi: 10.1016/j.ecolind.2021.108342
Yeh, N., Yeh, P., and Chang, Y.-H. (2015). Artificial floating islands for environmental improvement. Renew. Sust. Energ. Rev. 47, 616–622. doi: 10.1016/j.rser.2015.03.090
Yongabi, K., Nagabhatla, N., and Rios, P. C. S. (2018). “Phytoremediation eco-models using indigenous Macrophytes and Phytomaterials” in Multifunctional wetlands: Pollution abatement and other ecological services from natural and constructed wetlands. eds. N. Nagabhatla and C. D. Metcalfe (Cham: Springer International Publishing).
Zapata, D., Oleas, N. H., Páez-Vacas, M., and Tobes, I. (2021). Water quality assessment of the Cutuchi River basin (Ecuador): a review of technical documents. IOP Conf. Ser. Earth Environ. Sci. 690:012058. doi: 10.1088/1755-1315/690/1/012058
Keywords: nature-based solutions, local communities, PESTEL, water ecosystem services, SDGs
Citation: Fonseca K, Espitia-Sarmiento EF, Ilbay-Yupa M and Breuer L (2024) Integrating community knowledge into nature-based solutions for the sustainability of water ecosystem services: insight from local communities in Ecuador. Front. Sustain. 5:1491776. doi: 10.3389/frsus.2024.1491776
Edited by:
Cristina Matos Silva, University of Lisbon, PortugalReviewed by:
Luis Alfonso Escudero-Gomez, University of the Balearic Islands, SpainNeil A. Coles, University of Western Australia, Australia
Copyright © 2024 Fonseca, Espitia-Sarmiento, Ilbay-Yupa and Breuer. This is an open-access article distributed under the terms of the Creative Commons Attribution License (CC BY). The use, distribution or reproduction in other forums is permitted, provided the original author(s) and the copyright owner(s) are credited and that the original publication in this journal is cited, in accordance with accepted academic practice. No use, distribution or reproduction is permitted which does not comply with these terms.
*Correspondence: Kalina Fonseca, a2FsaW5hLmZvbnNlY2FAdXRjLmVkdS5lYw==