- 1Faculty of Civil Engineering and Geosciences, Delft University of Technology, Delft, Netherlands
- 2Loughborough Business School, Loughborough University, Loughborough, United Kingdom
- 3Faculty of Architecture and the Built Environment, Delft University of Technology, Delft, Netherlands
- 4School of Humanities and Social Sciences, Harbin Institute of Technology (Shenzhen), Shenzhen, China
- 5Rotterdam School of Management, Erasmus University Rotterdam, Rotterdam, Netherlands
- 6School of International Relations and Public Affairs, Fudan University, Shanghai, China
Urban infrastructure development is one principal way people are transforming the natural world and their living conditions. It is important for humanity, but it can also cause major impacts to the environment, such as huge amounts of solid waste and CO2 emissions. Considering this, the circular economy (CE) is a promising alternative to the traditional “make, use, and dispose” linear economy model. However, as a strategy for sustainable development (SD), the CE is still in its infancy in the urban transport infrastructure sector. Therefore, this article aims to guide the implementation of CE during transport infrastructure projects. To achieve this goal, a literature review and case study were adopted as the research methods. After reviewing existing well-established CE frameworks, the iReSOLVE (implement, Regenerate, Share, Optimize, Loop, Virtualize, Exchange) framework is recognized as the most comprehensive one. Upon it, an analytical framework containing specific-related aspects of CE in urban transport infrastructure projects (which belongs to meso-scale) is proposed (coined as the 4Wh-iReSOLVE framework). The 4Wh means Who, When, Where, and What. The proposed framework offers insight into potential CE activities for transport infrastructure projects and assists in assessing the performance and impacts of CE of these projects to cover the gap of the neglected meso-scale. Ten circular viaduct project initiatives in the Netherlands are used as case analyses with the 4Wh-iReSOLVE framework. The results present the highlights of the circular viaduct initiatives in the Netherlands, with CE activities categorized into five groups (design-related strategies, general CE strategies, implementation, management, and related digital technologies and materials, as well as environmental sustainability). As verified by several experts of the projects studied, it can be concluded that the 4Wh-iReSOLVE framework is suitable for transport infrastructure project CE analyses and implementations. It can potentially be a suggested guideline in future policy documents.
1 Introduction
Surfacing environmental concerns have made both public and private organizations look toward and embrace the circular economy (CE) within their material-related practices (Han et al., 2017; Jedelhauser and Binder, 2018; Veleva and Bodkin, 2018). Projections highlight that a growing population with rising average wealth could push material extraction up to 183 billion metric tons per year by 2050 (Lecerf et al., 2017). Europe sends over 50% of its waste straight to landfill and incinerators, which cause climate change, destroying valuable resources and resulting in a huge missed opportunity for job creation (Seas at Risk, 2018). This warrants a shift away from the traditional “linear” material-related practices, which are based on a “take-make-dispose” mode of dealing with materials. This shift has already been initialized in the European Commission by mandate of the “Roadmap to a Resource Efficient Europe” (2011) and “The Action Plan towards the Circular Economy” (2015) (Domenech and Bahn-Walkowiak, 2017).
With so much widespread attention, it could be reasonably assumed that different sectors are keen to follow up on the changes that the CE aspires to. Surprisingly though, one critical sector, the urban transport infrastructure sector, has been scarcely observed in the CE arena (Mantalovas et al., 2020). The urgency for this sector is certainly not trivial, since urban infrastructure projects have high environmental impacts due to the long lifespans and lengthy periods of influence on the surrounding environment (Malekpour et al., 2015). Ambition and action on this front would entail significant opportunities such as reducing energy use, greenhouse gas (GHG) emissions, and waste production.
Two explorative studies into the meaning of the CE have opened up a possible way to understand and address the observed inactivity of the CE in the urban infrastructure sector. Both Pomponi and Moncaster (2017) and Kirchherr et al. (2017) have elaborated that the CE can be studied at three systemic scales (levels), namely: micro, meso, and macro. Yet, these scales are hierarchical in nature and undefined in scope. For example, Pomponi and Moncaster (2017) seem to disagree with Kirchherr et al. (2017) on whether eco-industrial parks are part of the meso-scale. Irrespective of this ambiguity, it seems that the outer extreme examples in “micro” or “macro” are receiving more attention than the middle stream, i.e., “meso” of this division (Pomponi and Moncaster, 2017). Kirchherr et al.’s (2017) views are similar to Heshmati’s (2015) and Su et al.’s (2013), and they provided examples for the three scales, respectively: a single object (micro-scale), a symbiosis association (meso-scale), and a city (macro-scale). In this research, it is considered the meso-scale falls into the range between object level and city/region level. It is assumed that most urban transport infrastructure projects belong to the meso-scale.
In this article, we take the view that the underdevelopment of the CE at the meso-scale helps to contextualize why the urban transport infrastructure sector has not picked up on the changes instigated by the CE. At the heart of this argument lies the observation that the urban transport infrastructure sector is a very hierarchically operating business environment, in which projects are made up of contracts between clients, contractors, and subcontractors. These dependencies and the complexity of the construction value chain complicate any motion of changes in projects, including the implementation of circular principles (Munaro and Tavares, 2023). In this setting, it is also difficult for the private sector to invest in innovations if there is little certainty about potential benefits within this system. Therefore, this article stresses the unattended meso-scale for urban transport infrastructure projects to be more circular.
The research question of this article is: How can CE be made actionable for urban transport infrastructure projects?
To answer this research question, the focus of this article is to build an analytical framework, through which we would be empirically empowered to comprehend how the CE changes must occur in the context of urban transport infrastructure projects. The framework also includes an analysis of some impacts caused by CE changes. As a prerequisite of economic development, transport infrastructure is the backbone of global trade and globalization, representing a key facilitator of economic growth and welfare and providing crucial services, both shaping and supporting urban development (Tsamboulas et al., 2007; Achour and Belloumi, 2016; Schuckmann et al., 2012; Efthymiou and Antoniou, 2013).
We build the analytical framework through two steps: first, we uncover the currently available well-established CE “frameworks”1 that have been described to comprehend CE and assess their potential for studying the CE in the urban transport infrastructure context with a set of criteria; second, after selecting one “framework,” we propose contextually driven modifications (including CE activity impact analysis) to make it better suited and more useful to studying real-life CE practices in the context of urban transport infrastructure projects. The proposed framework is specifically designed to figure out the potential areas where the CE principles could be embedded during the main lifecycle phases of urban transport infrastructure projects. The framework aims for the meso-scale, and with such novelty, it can not only be used to inspire CE activities for specific project stakeholders, guiding locations of the activities; but also to learn finished projects, which is the case of this article, showing the applicability of the framework.
2 Literature review
At the project level, CE has been implemented in, for example, energy infrastructure projects (Invernizzi et al., 2020; Mignacca and Locatelli, 2021) and water infrastructure (Vera-Puerto et al., 2020). For construction projects, some studies use frameworks such as a CE evaluation framework (Dams et al., 2021), a CE index for the built environment (O’Grady et al., 2021), circular environmental impact indicators (Foster et al., 2020), and CE in buildings (adaptive reuse of building components) (Sanchez et al., 2020).
As for both buildings and infrastructures in the construction sector, Wuni and Shen (2022) revealed 21 significant success factors for integrating CE principles into modular construction projects in Hong Kong. Kooter et al.’s (2021) aim is to understand the dynamics of circular construction projects and how these inter-organizational projects contribute to the transition toward a CE. Both studies are from a project management perspective.
Urban transport infrastructure projects typically involve diverse stakeholders (e.g., agencies/clients, consultants, contractors, and suppliers), with contracts signing typically for the design, construction, and maintenance, with often separate contracts for each stage. Therefore, all the stakeholders (during the whole life cycle phases of projects) need to realize the importance of the CE and collaborate. Additionally, because of the long lifespan of most transport infrastructures, the CE should be considered not only during the construction phase but also during the operation/maintenance phase and decommissioning/demolition phase.
Winans et al. (2017) reviewed the history of the CE concept to provide a context for a critical examination of how it is applied currently. They studied specifically three thematic categories. First, they considered policy instruments and approaches that mainly cover eco-industrial parks, eco-industrial networks, and industrial symbiosis. Second, they considered value chains, material flows, and products, which include wood, paper, plastics, metals, phosphorus and other chemicals, agricultural products and waste, water, as well as land. Third, they looked into technological, organizational, and social innovations, like biological and technical product and material innovations, economic and business models, and enabling conditions and systems for the energy sector. It is noticeable that there is no CE implementation in urban transport infrastructure projects in Winans et al.’s (2017) review.
In a more general sense, since 2017 there have been various notable attempts to develop a framing of the CE concept as a whole. For purposes of this study, we depart from an overview of viable candidates for this framing to set the contours for an urban transport infrastructure relevant conception of CE. Therefore, we developed four critical criteria for identifying the right elements befitting the infrastructure context. These include:
i. The framework should embody diverse CE aspects (such as reduce, reuse, recycle, etc.) to be able to comprehensively capture CE principles;
ii. In terms of the CE dimension, it can be applied to the scope of urban transport infrastructure projects, rather than macro- or micro-scales;
iii. It should contain enough information for implementation, rather than being too simple or not suitable for implementation;
iv. Concerning the potential CE application areas, it can be related to infrastructure projects, which means it is not specifically and exclusively designed for application to the food or agriculture sector, for instance.
The four criteria can be supported by Wisse’s (2016) thesis, which included “Relevant,” “Applicable to scope,” and “Useable and easily interpreted.” The four criteria are used in Supplementary Table S1.
In making sure that the CE frameworks are reviewed comprehensively, we performed the review in a systematic stepwise approach. Figure 1 shows the process of this systematic literature review. First, the Scopus database was used to search peer-reviewed journals because it has long-term, worldwide coverage. By searching Scopus with a query,1 2,551 articles could be seen for CE “frameworks.”2 However, these still needed to be filtered. Some articles describe CE itself as the “framework,” e.g., Mesa et al. (2018), Masullo (2017), Chen et al. (2017), Hu et al. (2011). After a process of proactive reading, 1,596 articles were obtained. These were then further reduced in case articles did not have clearly identifiable names for the framework that they proposed. This led to a final selection of 18 articles (see Supplementary Table S1).
The “frameworks” of the 18 articles can be sometimes referred to as models, instruments, or tools, but still contain a framing of the CE concept before a study uses them as an instrument, model, or tool. Therefore, the review labels all of these as frameworks for consistency hereafter. The frameworks are presented in detail for overview in Supplementary Table S1.
After comparing the 13 “frameworks,” three frameworks were found to meet all four criteria. This meant that in terms of the CE and urban transport infrastructure projects, the BECE (Backcasting and Eco-design for CE) framework, the CEIMA (Circular Economy Interface Matrix Analysis) framework, and the ReSOLVE (implement, Regenerate, Share, Optimize, Loop, Virtualize, Exchange) framework are regarded as the most suitable cornerstones to be further developed into the targeted analytical framework. From their pros and cons, it can be seen that the three frameworks can complement each other. Therefore, the three frameworks are studied more and compared in the next section.
3 Analytical framework for CE in urban transport infrastructure projects
In this section, the analytical framework is developed step by step. The right ingredients and building blocks have been found in the three previous frameworks. These are reviewed in more detail in the following section, and the useful building blocks are extracted. Next, the framework’s contours and building blocks are synthesized as the analytical framework. Finally, the case contours are sketched for a relevant application, including justification.
3.1 Building blocks for a CE analytical framework for transport infrastructure projects
3.1.1 BECE/iReSOLVE/ReSOLVE
Mendoza et al. (2017) presented the BECE framework to ensure that businesses can implement the CE requirements more readily. BECE empowers organizations to tackle the CE holistically by embedding the concept into corporate decision-making and by bringing operational and systems thinking together, thus increasing the likelihood of successful implementation. In their article, four categories correspond to key strategies that can contribute to building the CE business models, including sustainable business model innovation (SBMI); sustainable product design (SPD); closed-loop systems (CLS); and product-service systems (PSS). The BECE framework embodies diverse CE, can be applied to the scope of urban transport infrastructure projects, contains enough information for implementation, and can be related to infrastructure projects. As a leading CE tool used by businesses for building CE business models, the ReSOLVE checklist proposed by the Ellen MacArthur Foundation (EMF) was used in Mendoza et al.’s (2017) paper.
The BECE framework was informed and described after Mendoza et al. (2017) conducted a literature review of existing CE frameworks to examine their congruence with the CE principles, actions, and requirements. Mendoza et al.’s (2017) analysis of existing CE frameworks raises questions on how the CE can be brought about effectively, given that implementation aspects are often missing and that few of the frameworks consider innovation at a systems level. Thus, they coupled Backcasting (a way to reach a common understanding of successful futures and the steps required to achieve them) (Circular Academy, 2023) and Eco-Design to propose the BECE framework.
There are 10 steps in the BECE framework, from which steps 1 to 3 belong to the application of Backcasting; steps 4–7 belong to the application of an eco-design analysis; and steps 8–10 are the implementation of the vision by defining and validating scenarios and action plans. As a case study, the BECE framework was tested in a pilot workshop in preparation for a real-life application at a later stage in collaboration with a major retailer (Mendoza et al., 2017). However, the BECE framework is still company-oriented, not project-oriented. This has a downside that the overarching deployment of the model is not focused on multiple actors but just one business.
On this basis, the BECE framework originated from the ReSOLVE checklist [or the ReSOLVE framework, as cited by Lewandowski (2016), proposed by EMF]. As ReSOLVE lacks guidance on the implementation of the ideas in business practice, Mendoza et al. (2017) added the action IMPLEMENT (it has several underpinning requirements taken from project management) to ReSOLVE, resulting in the “iReSOLVE” checklist (Supplementary Table S2).
The iReSOLVE framework has a pro. First, it is compatible with using stakeholders and big data to accomplish CE (Virmani et al., 2022; Jabbour et al., 2019; Alonso-Almeida et al., 2021). It allows, for example, to help track and manage materials such that the collection and return of products and waste can be improved (Nascimento et al., 2019; Nobre and Tavares, 2017; Muller et al., 2022). The ReSOLVE framework is a well-known theoretical framework for CE (Lejardi et al., 2021) and has been used often (Dev et al., 2020; Heyes et al., 2018; Jabbour et al., 2019; Mastos et al., 2021; Mhatre et al., 2021; Pizzi et al., 2021; Tu et al., 2020).
The iReSOLVE framework also has several cons. The problem lies in the scope definition and the interrelation of the CE strategies, and the implementation path could strongly vary depending on the sector or product in which the strategy is applied (Lejardi et al., 2021).
3.1.2 CEIMA/9Rs
Coenen et al. (2020) developed the CEIMA framework following a Design Science Research-based approach, linking a bottom-up asset stakeholder perspective to the existing top-down conceptualizations of CE. With the CEIMA framework, identified interfaces between stakeholders and circular actions reveal key opportunities for stakeholders within the infrastructure sector to start with the implementation of circular actions (Coenen et al., 2020). It covers the whole lifecycle of assets, which is a useful building block for the framework to be proposed in this article. The CEIMA framework is designed for urban transport infrastructure projects; it also embodies diverse CE aspects and contains enough information for implementation.
It consists of 23 CE actions based on the operationalization of the 9Rs. The 9R concepts underlie a circular economic framework that examines how materials can be used and reused at their highest value while minimizing waste and environmental destruction (Potting et al., 2017). This signifies that the 23 actions identified by Coenen et al. (2020) are particularly focused on strategies related to handling materials or products.
Coenen et al. (2020) focus on the identification of actions, when people are following the referencing structure as the researchers have prepared it. In pursuance of identifying contextually driven actions, such a fixed CE action list is user-friendly for non-CE experts, but it restricts potential CE innovation actions or new ideas, while with a heuristic format, the focus on 9Rs may therefore narrow the innovation focus to only the particular referencing frame of 9Rs, and disallow for more indirect circular innovations to be recognized.
There are some pros to the CEIMA framework, as it refers to its usefulness. First, CEIMA makes an interface possible between stakeholders and circular actions, and it uses a bottom-up approach to associate linkage among CE practices and actions (Virmani et al., 2022). Second, Coenen et al. (2020) discussed that a broad, unified, and clear perspective is needed to generate a better understanding of how organizations can implement CE in practice (Muller et al., 2022).
There are also some cons to the CEIMA framework. Coenen et al. (2020), in their study, took the view that professionals without expertise can use frameworks to arrive at the same outcome without having to understand the multitude of circular principles and approaches (Lejardi et al., 2021). It thereby assumes that it is beneficial to arrive at the same actionable outcome when it comes to CE. This, however, restricts potential CE innovation actions or new ideas.
3.1.3 Building blocks: CEIMA and iReSOLVE inspirations
As the building blocks are selected for CEIMA and iReSOLVE, a few reasons can be named. First, both frameworks consider multiple lifecycle phases and different stakeholders of the project or asset; they both do not constrain a certain lifecycle phase or one stakeholder. Second, 9Rs, which underlies CEIMA, missed a broader category range to identify circular strategies; iReSOLVE takes a broader set of categories into view, as it transcends the mere focus on material or product choices (Okorie et al., 2018) by also encompassing supporting and systemic actions. The 9R strategies were developed for assessing CE levels of materials in a product. It thereby tries to understand the CE system and determine the extent to which a product or structure contributes to the CE (Disli and Ankaralıgil, 2023). The ReSOLVE framework has six business actions to aid organizations in the principles of CE (Okorie et al., 2018).
Taking these reasons into account, the analytical framework needs a combination of building blocks from both sides. In the original “iReSOLVE” checklist, there are “iReSOLVE actions” and “iReSOLVE requirements” (each “iReSOLVE requirement” can have one or more corresponding CE activities, which can be viewed as “What”). Next to that, CEIMA illustrated that certain additional characteristics need to be considered for urban transport infrastructure projects. These include most notably multiple stakeholders (“Who”), lifecycle stages (“When”), and spatial dispersion (“Where”) involved.
In addition, as it can be easily figured out that all the “frameworks” reviewed do not consider the impacts of CE practices in a project, therefore, for the analysis of impacts these activities can induce, an added part of “iReSOLVE requirement (CE) activities Impact analysis” after the four “Wh”s part is forged.
Taken together, this helps to enhance the framework as 4Wh-iReSOLVE in Figure 2. The framework hosts three main categories. First, it offers space to specify the generic situation of the urban infrastructure project that is analyzed with categories signifying the “Who,” “What,” and “Where.” Second, the action categories are listed based on the iReSOLVE structure. Finally, in order to analyze the proper impact that these actions help accomplish, three impact categories are formulated along the lines of the sustainability dimensions (environmental, economic, and social).
3.2 Analytical function of the framework
The situation categories show that for a certain urban transport infrastructure project, “When,” “Where,” and “Who” can do “iReSOLVE actions” to implement CE. As the current “iReSOLVE requirements” are comprehensive, due to reasons such as technology, for typical urban transport infrastructure projects, not all the “iReSOLVE requirements” are likely to be analyzed (e.g., digest anaerobically). In addition, in practice, each “iReSOLVE requirement” can have diverse forms of activities. Additionally, the actions can bring about certain impacts.
To use the analytical framework, all the “iReSOLVE requirements” are analyzed for the project. The purpose of this is to find out whether certain CE actions apply to the involved stakeholders as well as to the different lifecycle phases of a project, and if so, the specific locations for executing these CE activities are further identified.
To facilitate this goal, the framework has been operationalized in Supplementary Table S2. On the top left, the generic situation can be described about the project in terms of the “Who,” “When,” and “Where.” In the bottom left, the seven action categories are outlined, with a total of 23 activities formulated as examples. This is the part where the results of finding the relevant CE actions in a project can be specified. In the bottom right, for each action, the expected impact on the environmental, economic, or social aspects can be described. In Supplementary Table S2, some examples are written about the long- or short-term impact of an action. However, this acts simply as an example and may include actual metrics or LCA (life cycle assessment) output. Finally, at the top right corner, the total noted impact of the activities can be summarized or accumulated to the project level if needed.
In essence, the outcome of applying the analytical framework to an urban transport infrastructure project is a detailed table that shows what “iReSOLVE requirement activities” different stakeholders of the project can conduct during which project phase(s) and where to conduct. Particularly, the impact analysis can show the impacts (benefits) after the executions of the CE activities.
3.3 Case analysis with the framework
The framework is used to analyze 10 innovation cases in the Netherlands as part of open-ended innovation efforts for designing circular viaducts. In 2020, Rijkswaterstaat (RWS, the executive agency of the Ministry of Infrastructure and Water Management) in the Netherlands launched an SBIR (Strategic Business Innovation Research) call, challenging entrepreneurs to present innovative solutions for circular viaducts. Based on impact, feasibility, and economic perspective, Rijkswaterstaat selected 10 (out of more than 30 offers) for further feasibility studies (prototypes are being developed) (RWS, 2022).
The feasibility studies of all 10 consortia were retrieved from public sources (Circulaire viaducten, 2022). The documents have been read and studied by the first author. Afterward, the 4Wh-iReSOLVE framework was used to analyze the 10 consortia. The contact persons of the 10 project initiatives have been contacted, and they are asked: (1) whether they think our analysis covers all the CE activities of their projects; (2) whether the CE activities are attributed to the correct lifecycle phases; and (3) their overall views about the framework (including the impact analysis, if applicable).
After the identification of CE activities, we requested feedback from the responsible circular viaduct design. Four of these project initiatives responded and offered feedback. Specifically, these participants were asked whether the CE activities are attributed to the right positions in the framework, and the third author of the article also did the judgment of the analysis from the first author for validation. Finally, it turns out that the analysis of the four project initiatives by the first author was found to be robust.
4 Results of CE actions in the 10 circular viaduct designs
The results of all 10 projects are presented in the following subsections. To be concise, these projects are briefly introduced, following impact analysis (if applicable), and the overall main results of all 10 projects are summarized in Figure 3. All the CE activities of the 10 projects can be seen in Supplementary Table S3, with lifecycle phases presented for each CE activity. For the 10 projects, the companies can be seen as designers and builders.
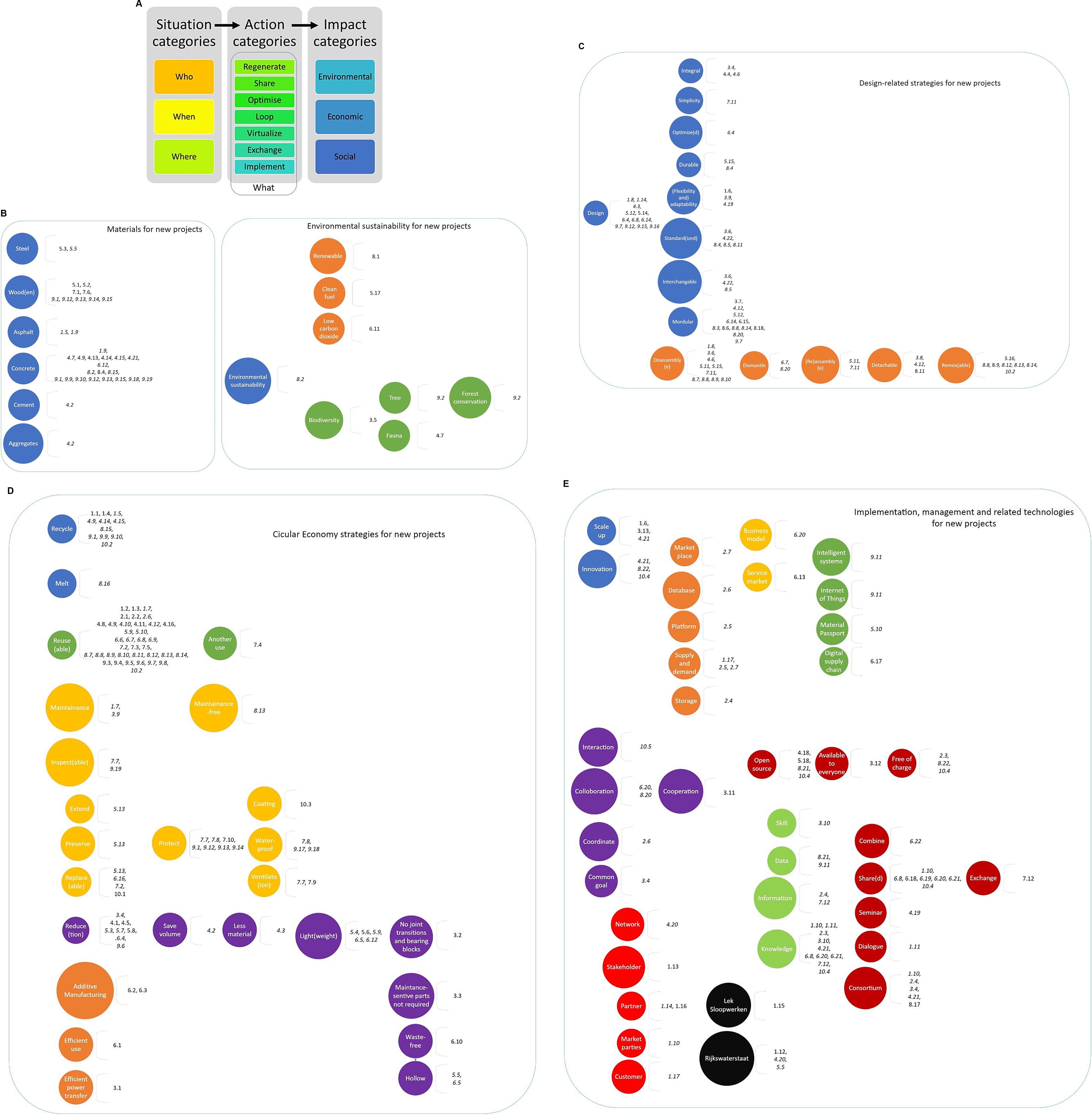
Figure 3. Group analysis of all the CE activities of Project 1–10 [the numbers are in correspondence with those in Supplementary Table S3; the numbers in italics mean the corresponding CE statements contain more than one core word, and in such cases, if the core words belong to different groups, we call the groups are “connected,” thus forging (E)] (own figure). (A) Group 1. Design-related strategies for new projects; (B) Group 2. Circular economy strategies; (C) Group 3. Implementation, management, and related digital technologies; (D) Group 4 and Group 5. Materials and environmental sustainability, respectively; (E) Connectivity among the five groups.
4.1 Project 1–3
Project 1 is about closing the circle for the high-quality reuse of existing viaducts, which is technically feasible, constructively safe, and economically attractive. Project 2 is mainly about the reuse of physically unaltered inverted T-beam prefab girders. The design innovation of Project 3 offers a total solution for a circular construction system for viaducts. The system consists of a fully recoverable, modular arch construction and substructure. The solution is demountable and reusable at the element level.
4.2 Project 4
Project 4 is Circular Arch Viaduct, which means:
Substantial material reduction by applying ultra-high strength fiber-reinforced concrete (UHSVB) in the ultimate bend-free shape, the pressure arc.
A modular, demountable, and reusable viaduct of UHSVB elements with a service life of up to 200 years and reusable foundation piles;
Abutments of circular and cementless geopolymer concrete were granulated from old viaducts as 100% recycled building materials were reused.
Table 1 shows the iReSOLVE requirement activities impact analysis of Project 4.
4.3 Project 5–7
Project 5 is about the development of Variaduct into a preliminary design with the following innovative and distinctive elements: slim steel deck sections; foundation without piles; columns with jacking facility; substructure of prefab concrete elements; application of wood; reduction of the construction height.
Table 2 shows the iReSOLVE requirement activities impact analysis of Project 5.
The proposal of Project 6 for the circular viaduct, like the honeycomb, consists of an efficient light spatial structure of 3D-printed concrete.
Table 3 shows the iReSOLVE requirement activities impact analysis of Project 6.
Project 7 demonstrates that the BoLT design (viaduct with a span of 25 m consisting of wooden decking on wooden beams) is technically feasible, reduces CO2 emissions, and provides a sound business case within the existing (or modified) design standards.
4.4 Project 8–10
The goal of Project 8 is to show that: (1) all the viaduct components are modular and circular with reduced CO2 emissions and minimal material consumption; (2) the viaduct can be built following current standards and guidelines; (3) the viaduct satisfies all set safety factors; (4) the business case becomes feasible in collaboration with RWS.
Table 4 shows the iReSOLVE requirement activities impact analysis of Project 8.
The viaduct of Project 9 consists of a prefab wood-concrete girder system with a maximum span of 15–35 meters and a deck width of approximately 26 meters. The prefab beam has a width of 1.6 meters and consists of ¼ part of recycled concrete and ¾ part of European spruce. The wood is protected against weather influence by the concrete. As a result, the system has a lifespan of at least 100 years.
Table 5 shows the iReSOLVE requirement activities impact analysis of Project 9.
The aim of Project 10 is to demonstrate the financial and technical feasibility of a traffic viaduct in the material biocomposite.
Table 6 shows the iReSOLVE requirement activities impact analysis of Project 10.
4.5 iReSOLVE requirement (CE) activities of project 1–10 based on different iReSOLVE action categories
The results of CE activities of Project 1–10 based on different iReSOLVE action categories are shown in Supplementary Table S4. In terms of action categories, different projects vary a lot. It can be noted that about half of the CE activities of Project 1 belong to the “Implement” category. The contact person of Project 1 expressed that certain quotes to life cycle phases depend on how to approach it. For example, the following quote can be perceived as the Design/Preparation phase for the “new” circular viaduct as well as the demolition phase of the old viaduct: “Harvest all materials from the viaducts to be demolished in the A76.” For Project 2, five out of the total seven CE activities were attributed to the “Implement” category. Unlike Project 1 and Project 2, the CE activities of Project 8 cover more categories, and 12 activities were ascribed to “Share.” The numbers 10.3 and 10.4 of Supplementary Table S4 are in brackets because the contact person of Project 10 does not think activities 10.3 and 10.4 are CE activities but are ways to cooperate in the market.
Generally for Supplementary Table S4, R3, S1, L1, L3, L4, and V2 are the sub-categories that are not covered, half of which (R3, L3, and L4) are about biology, while S1, L1, and V2 are potential areas for viaducts to be more circular. On the contrary, S2, S3, and I3 are the most “popular” sub-categories, as “Reuse” as well as “Prolong product” are both common CE strategies, and most of the 10 projects are conscious of stakeholder engagement.
4.6 Group analysis of all the CE activities of project 1–10
To have a different perspective besides the iRESOLVE framework, a group analysis of all the CE activities of Project 1–10 was also conducted. To do so, the core words of all the CE activities in Supplementary Table S3 were extracted to stand for those CE activity statements; then all the core words were written in notes and grouped in a workshop; finally, the groups were summarized based on their main characteristics. The results of the group analysis can be seen in Figure 3.
In Figures 3A–D, closely related core words were positioned close to each other. It can be seen from Figure 3E that the five groups form a “kite” shape, in which the first four groups form a diamond-like shape, as each of them is connected with the other three. Group 5 (Environmental sustainability), as a small group, is only connected to Group 4; therefore, Group 4 (Materials) can be viewed as the central group. The biggest group is Group 3, which aligns with the “Implement” category in Supplementary Table S4. In Group 4, concrete and wood are the materials that attracted the most attention from the 10 viaduct projects. Figure 3 offers us a clear map of the different strategy groups of circular viaducts from the 10 projects studied. The group analysis can support the analysis of projects based on the iRESOLVE framework, and it is also possible to do the group analysis with certain software.
5 Discussion
With the 4Wh-iReSOLVE framework, there are more CE activities obtained from, for example, Projects 1 and 8 than from, for instance, Projects 2 and 10, since Projects 2 and 10 are about a certain kind of component and material, respectively, not covering CE principles broadly. According to the framework, there is still quite a large potential for projects to be more circular. This is shown by Supplementary Table S4, as even if all the 10 projects were summarized in this table, not alliReSOLVE action categories were covered (e.g., S1 Share assets, L1 Remanufacture products or components, V2 Dematerialize indirectly, etc.). The original “iReSOLVE requirements” are comprehensive; currently, a certain project probably cannot meet all the “requirements,” since some action categories of Supplementary Table S4 belong to the biological loop (i.e., R3 Return recovered biological resources to biosphere, L3 Digest anaerobically, and L4 Extract biochemicals from organic waste). With more bio-based materials used in the future, it is potential that the action categories R3, L3, and L4 will be achieved by transport infrastructure projects. As circular viaduct projects, the CE activities of the 10 projects analyzed also present five different CE streams (groups): design-related strategies for new objects, high-level CE strategies, implementation, management, and related digital technologies, as well as materials and environmental sustainability. This part of analysis is similar to Munaro and Tavares’s (2023) categorization of barriers and drivers to CE in the construction sector. The five CE streams direct applicable CE implementation directions for (viaduct) infrastructure projects. However, as the five CE streams were extracted from only the 10 projects in the Netherlands, there can be more implementation directions.
As for the impacts of CE activities, it can happen that sometimes “Environmental impact” resonates with “Economic impact.” For instance, if a certain kind of energy consumption is lower, not only the corresponding energy cost decreases, but also related CO2 emissions would become less. In addition, the three kinds of impacts correspond to the “Triple bottom line” of “Sustainability.” From the analysis of the 10 projects, no social impacts were found from the project documents. The impact analysis (which is an innovation point) can also make practitioners pay more attention to the long-term impacts and the corresponding activities. One limitation of this article is that the data for the cases were from the contractors only. Further application of the enlightening 4Wh-iReSOLVE analytical framework should contain all the main stakeholders (each stakeholder with a resultant table), and there are possible overlaps as well as collaborations of certain “iReSOLVE requirement activity” among multiple stakeholders.
6 Conclusion
The research question of this article has been answered by forging and using the 4Wh-iReSOLVE framework via literature review and case study. With the 4Wh-iReSOLVE analytical framework, a comprehensive view can be drawn of where to implement CE for different stakeholders during which lifecycle phases of a certain urban transport infrastructure project. After a systematic analysis, the framework can offer project stakeholders directions for embedding CE principles into their projects. In addition, it is flexible enough to be applied to different stages of a project. The outcomes can also be compared to draw lessons if the framework is applied to different projects in various contexts (such as with geographical variability). With the CE activities impact analysis part, stakeholders of a certain project can grasp the benefits that CE practices can bring. The analysis of the 10 circular viaduct projects offers practitioners circular initiatives of viaduct design and construction in the Netherlands, and such initiatives can be valuable experiences for different stakeholders (e.g., policymakers) of the sector from other countries.
Theoretically, this research study tries to extend CE at the meso-scale, specifically for urban infrastructure projects; from the perspective of practice, the “4Wh-iReSOLVE” framework stimulates CE principles awareness and guides CE implementation in urban infrastructure projects. The CE activities of the 10 circular viaducts in the Netherlands were shown systematically, offering reference to the usage of the framework and future circular viaduct projects.
Data availability statement
The original contributions presented in the study are included in the article/Supplementary material, further inquiries can be directed to the corresponding authors.
Author contributions
XL: Conceptualization, Formal analysis, Funding acquisition, Investigation, Methodology, Visualization, Writing – original draft, Writing – review & editing. DS: Resources, Supervision, Visualization, Writing – review & editing. WM: Validation, Writing – review & editing. MJ: Supervision, Writing – review & editing. MH: Supervision, Writing – review & editing.
Funding
The author(s) declare that financial support was received for the research, authorship, and/or publication of this article. The authors gratefully acknowledge funding offered by the China Scholarship Council (No. 201606040174).
Acknowledgments
The authors sincerely thank Wietse de Jong and Yuri Wolf (RWS); Richard Busse (Antea Group); Arno Van Wittmarschen and Huibert De Brabander (Max Bögl Nederland B.V.); Gert-Jan van Eck and Rob Vergoossen (HaskoningDHV Nederland B.V.); as well as Stephanie Lamerichs (Witteveen+Bos) for their kind help. The reviewers, the guest editor, and the editor-in-chief are greatly appreciated for their constructive comments and pertinent suggestions.
Conflict of interest
The authors declare that the research was conducted in the absence of any commercial or financial relationships that could be construed as a potential conflict of interest.
Publisher’s note
All claims expressed in this article are solely those of the authors and do not necessarily represent those of their affiliated organizations, or those of the publisher, the editors and the reviewers. Any product that may be evaluated in this article, or claim that may be made by its manufacturer, is not guaranteed or endorsed by the publisher.
Supplementary material
The Supplementary material for this article can be found online at: https://www.frontiersin.org/articles/10.3389/frsus.2024.1475155/full#supplementary-material
Footnotes
1. ^(TITLE (“circular economy”) OR AUTHKEY (“circular economy”)) AND (TITLE (“approach” OR “frame” OR “framework” OR “instrument” OR “method” OR “methodology” OR “model” OR “tool”) OR AUTHKEY (“approach” OR “frame” OR “framework” OR “instrument” OR “method” OR “methodology” OR “model” OR “tool”) AND (LIMIT-TO (DOCTYPE, “ar”) OR LIMIT-TO (DOCTYPE, “re”) AND (LIMIT-TO (LANGUAGE, “English”))).
2. ^This was done on 16 June (Disli and Ankaralıgil, 2023).
References
Achour, H., and Belloumi, M. (2016). Investigating the causal relationship between transport infrastructure, transport energy consumption and economic growth in Tunisia. Renew. Sust. Energ. Rev. 56, 988–998. doi: 10.1016/j.rser.2015.12.023
Alonso-Almeida, M., Rodriguez-Anton, J. M., Bagur-Femenías, L., and Perramon, J. (2021). Institutional entrepreneurship enablers to promote circular economy in the European Union: impacts on transition towards a more circular economy. J. Clean. Prod. 281:124841. doi: 10.1016/j.jclepro.2020.124841
Álvarez, R., and Ruiz-Puente, C. (2017). Development of the tool SymbioSyS to support the transition towards a circular economy based on industrial Symbiosis strategies. Waste Biomass Valoriz. 8, 1521–1530. doi: 10.1007/s12649-016-9748-1
Ang, K. L., Saw, E. T., He, W., Dong, X., and Ramakrishna, S. (2021). Sustainability framework for pharmaceutical manufacturing (PM): a review of research landscape and implementation barriers for circular economy transition. J. Clean. Prod. 280:124264. doi: 10.1016/j.jclepro.2020.124264
Çetin, S., De Wolf, C., and Bocken, N. (2021). Circular digital built environment: an emerging framework. Sustain. For. 13:6348. doi: 10.3390/su13116348
Chen, L., Cong, R. G., Shu, B., and Mi, Z. F. (2017). A sustainable biogas model in China: the case study of Beijing Deqingyuan biogas project. Renew. Sust. Energ. Rev. 78, 773–779. doi: 10.1016/j.rser.2017.05.027
Circulaire viaducten. (2022) The RWS website. Available at: https://www.circulaireviaducten.nl/documenten-binnen-sbir/7-haalbaarheidsonderzoeken
Circular Academy. (2023). Backcasting. The circular academy website. Available at: https://www.circular.academy/portfolio/backcasting/
Coenen, T. B. J., Haanstra, W., Jan Braaksma, A. J. J., and Santos, J. (2020). CEIMA: a framework for identifying critical interfaces between the circular economy and stakeholders in the lifecycle of infrastructure assets. Resour. Conserv. Recycl. 155:104552. doi: 10.1016/j.resconrec.2019.104552
D’Urzo, M., and Campagnaro, C. (2023). Design-led repair & reuse: an approach for an equitable, bottom-up, innovation-driven circular economy. J. Clean. Prod. 387:135724. doi: 10.1016/j.jclepro.2022.135724
Dams, B., Maskell, D., Shea, A., Allen, S., Driesser, M., Kretschmann, T., et al. (2021). A circular construction evaluation framework to promote designing for disassembly and adaptability. J. Clean. Prod. 316:128122. doi: 10.1016/j.jclepro.2021.128122
Dev, N. K., Shankar, R., and Qaiser, F. H. (2020). Industry 4.0 and circular economy: operational excellence for sustainable reverse supply chain performance. Resour. Conserv. Recycl. 153:104583. doi: 10.1016/j.resconrec.2019.104583
Diacono, S., and Baldacchino, L. (2023). Identifying entrepreneurial opportunities in the circular economy. J. Manag. Organ. 30, 165–187. doi: 10.1017/jmo.2023.47
Disli, G., and Ankaralıgil, B. (2023). Circular economy in the heritage conservation sector: an analysis of circularity degree in existing buildings. Sustain. Energy Technol. Assessments. 56:103126. doi: 10.1016/j.seta.2023.103126
Domenech, T., and Bahn-Walkowiak, B. (2017). Transition towards a resource efficient circular economy in Europe: policy lessons from the EU and the member states. Ecol. Econ. 155, 7–19. doi: 10.1016/j.ecolecon.2017.11.001
Efthymiou, D., and Antoniou, C. (2013). How do transport infrastructure and policies affect house prices and rents? Evidence from Athens, Greece. Transp. Res. Part A Policy Pract. 52, 1–22. doi: 10.1016/j.tra.2013.04.002
Ferronato, N. (2021). Integrated analysis for supporting solid waste management development projects in low to middle income countries: the NAVA-CE approach. Environ. Dev. 39:100643. doi: 10.1016/j.envdev.2021.100643
Foster, G., Kreinin, H., and Stagl, S. (2020). The future of circular environmental impact indicators for cultural heritage buildings in Europe. Environ. Sci. Eur. 32:141. doi: 10.1186/s12302-020-00411-9
Han, F., Liu, Y., Liu, W., and Cui, Z. (2017). Circular economy measures that boost the upgrade of an aluminum industrial park. J. Clean. Prod. 168, 1289–1296. doi: 10.1016/j.jclepro.2017.09.115
Heshmati, A. (2015). A review of the circular economy and its implementation. IZA Discuss. Pap. No. 9611:63. doi: 10.1504/IJGE.2017.089856
Heyes, G., Sharmina, M., Mendoza, J. M. F., Gallego-Schmid, A., and Azapagic, A. (2018). Developing and implementing circular economy business models in service-oriented technology companies. J. Clean. Prod. 177, 621–632. doi: 10.1016/j.jclepro.2017.12.168
Hu, J., Xiao, Z., Zhou, R., Deng, W., Wang, M., and Ma, S. (2011). Ecological utilization of leather tannery waste with circular economy model. J. Clean. Prod. 19, 221–228. doi: 10.1016/j.jclepro.2010.09.018
Iacovidou, E., Millward-Hopkins, J., Busch, J., Purnell, P., Velis, C. A., Hahladakis, J. N., et al. (2017). A pathway to circular economy: developing a conceptual framework for complex value assessment of resources recovered from waste. J. Clean. Prod. 168, 1279–1288. doi: 10.1016/j.jclepro.2017.09.002
Invernizzi, D. C., Locatelli, G., Velenturf, A., Love, P. E. D., Purnell, P., and Brookes, N. J. (2020). Developing policies for the end-of-life of energy infrastructure: coming to terms with the challenges of decommissioning. Energy Policy 144:111677. doi: 10.1016/j.enpol.2020.111677
Jabbour, C. J. C., Jabbour, A. B. L. D. S., Sarkis, J., and Filho, M. G. (2019). Unlocking the circular economy through new business models based on large-scale data: an integrative framework and research agenda. Technol. Forecast. Soc. Change 144, 546–552. doi: 10.1016/j.techfore.2017.09.010
Jedelhauser, M., and Binder, C. R. (2018). The spatial impact of socio-technical transitions – the case of phosphorus recycling as a pilot of the circular economy. J. Clean. Prod. 197, 856–869. doi: 10.1016/j.jclepro.2018.06.241
Kirchherr, J., Reike, D., and Hekkert, M. (2017). Conceptualizing the circular economy: an analysis of 114 definitions. Resour. Conserv. Recycl. 127, 221–232. doi: 10.1016/j.resconrec.2017.09.005
Kooter, E., van Uden, M., van Marrewijk, A., Wamelink, H., van Bueren, E., and Heurkens, E. (2021). Sustainability transition through dynamics of circular construction projects. Sustain. For. 13, 1–19. doi: 10.3390/su132112101
Lauten-Weiss, J., and Ramesohl, S. (2021). The circular business framework for building, developing and steering businesses in the circular economy. Sustain. For. 13, 1–14. doi: 10.3390/su13020963
Lecerf, L., Skinner, A., Kunz, S., van Meesche, M., and Paquot, S. (2017). Moving towards a circular economy with EMAS. Brussels: European Commission.
Lejardi, E.S., Franke, M., Deng, Q., and Rial, R.M., (2021). Circularity protocols for extending the useful lifetime of obsolete large industrial equipment and assets. 26th IEEE international conference on emerging technologies and factory automation (ETFA), Vasteras, Sweden. 1–8.
Lewandowski, M. (2016). Designing the business models for circular economy-towards the conceptual framework. Sustain. For. 8, 1–28. doi: 10.3390/su8010043
Malekpour, S., Brown, R. R., and de Haan, F. J. (2015). Strategic planning of urban infrastructure for environmental sustainability: understanding the past to intervene for the future. Cities 46, 67–75. doi: 10.1016/j.cities.2015.05.003
Mantalovas, K., Di Mino, G., Del Barco Carrion, A. J., Keijzer, E., Kalman, B., Parry, T., et al. (2020). European national road authorities and circular economy: an insight into their approaches. Sustain. For. 12, 1–19. doi: 10.3390/su12177160
Mastos, T. D., Nizamis, A., Terzi, S., Gkortzis, D., Papadopoulos, A., Tsagkalidis, N., et al. (2021). Introducing an application of an industry 4.0 solution for circular supply chain management. J. Clean. Prod. 300:126886. doi: 10.1016/j.jclepro.2021.126886
Masullo, A. (2017). Organic wastes management in a circular economy approach: rebuilding the link between urban and rural areas. Ecol. Eng. 101, 84–90. doi: 10.1016/j.ecoleng.2017.01.005
Mendoza, J. M. F., Sharmina, M., Gallego-Schmid, A., Heyes, G., and Azapagic, A. (2017). Integrating Backcasting and eco-Design for the Circular Economy: the BECE framework. J. Ind. Ecol. 21, 526–544. doi: 10.1111/jiec.12590
Mesa, J., Esparragoza, I., and Maury, H. (2018). Developing a set of sustainability indicators for product families based on the circular economy model. J. Clean. Prod. 196, 1429–1442. doi: 10.1016/j.jclepro.2018.06.131
Mhatre, P., Panchal, R., Singh, A., and Bibyan, S. (2021). A systematic literature review on the circular economy initiatives in the European Union. Sustain. Prod. Consum. 26, 187–202. doi: 10.1016/j.spc.2020.09.008
Mignacca, B., and Locatelli, G. (2021). Modular circular economy in energy infrastructure projects: enabling factors and barriers. J. Manag. Eng. 37:04021053. doi: 10.1061/(ASCE)ME.1943-5479.0000949
Muller, L., Delai, I., and Alcantara, R. L. C. (2022). Circular value chain practices for developing resource value retention options. J. Clean. Prod. 359:131925. doi: 10.1016/j.jclepro.2022.131925
Munaro, M. R., and Tavares, S. F. (2023). A review on barriers, drivers, and stakeholders towards the circular economy: the construction sector perspective. Clean. Respons. Consum. 8:100107. doi: 10.1016/j.clrc.2023.100107
Muranko, Z., Andrews, D., Newton, E. J., Chaer, I., and Proudman, P. (2018). The pro-circular change model (P-CCM): proposing a framework facilitating behavioural change towards a circular economy. Resour. Conserv. Recycl. 135, 132–140. doi: 10.1016/j.resconrec.2017.12.017
Nascimento, D. L. M., Alencastro, V., Quelhas, O. L. G., Caiado, R. G. G., Garza-Reyes, J. A., Rocha-Lona, L., et al. (2019). Exploring industry 4.0 technologies to enable circular economy practices in a manufacturing context: a business model proposal. J. Manuf. Technol. Manag. 30, 607–627. doi: 10.1108/JMTM-03-2018-0071
Nobre, G. C., and Tavares, E. (2017). Scientific literature analysis on big data and internet of things applications on circular economy: a bibliometric study. Scientometrics 111, 463–492. doi: 10.1007/s11192-017-2281-6
O’Grady, T., Minunno, R., Chong, H., and Morrison, G. M. (2021). Design for disassembly, deconstruction and resilience: a circular economy index for the built environment. Resour. Conserv. Recycl. 175:105847. doi: 10.1016/j.resconrec.2021.105847
Okorie, O., Salonitis, K., Charnley, F., Moreno, M., Turner, C., and Tiwari, A. (2018). Digitisation and the circular economy: a review of current research and future trends. Energies 11:3009. doi: 10.3390/en11113009
Pizzi, S., Corbo, L., and Caputo, A. (2021). Fintech and SMEs sustainable business models: reflections and considerations for a circular economy. J. Clean. Prod. 281:125217. doi: 10.1016/j.jclepro.2020.125217
Pomponi, F., and Moncaster, A. (2017). Circular economy for the built environment: a research framework. J. Clean. Prod. 143, 710–718. doi: 10.1016/j.jclepro.2016.12.055
Potting, J., Hekkert, M., Worrell, E., and Hanemaaijer, A. (2017). Circular economy: Measuring innovation in the product chain. Den Haag: PBL.
RWS. (2022). Circulaire viaducten. The RWS website. Available at: https://www.circulaireviaducten.nl/
Sadeghi, M., Mahmoudi, A., Deng, X., and Luo, X. (2023). Prioritizing requirements for implementing blockchain technology in construction supply chain based on circular economy: fuzzy ordinal priority approach. Int. J. Environ. Sci. Technol. 20, 4991–5012. doi: 10.1007/s13762-022-04298-2
Sanchez, B., Rausch, C., Haas, C., and Saari, R. (2020). A selective disassembly multi-objective optimization approach for adaptive reuse of building components. Resour. Conserv. Recycl. 154:104605. doi: 10.1016/j.resconrec.2019.104605
Schuckmann, S. W., Gnatzy, T., Darkow, I. L., and von der Gracht, H. A. (2012). Analysis of factors influencing the development of transport infrastructure until the year 2030 - a Delphi based scenario study. Technol. Forecast. Soc. Change 79, 1373–1387. doi: 10.1016/j.techfore.2012.05.008
Seas at Risk. (2018). The seas at risk website. Available at: http://www.seas-at-risk.org/images/pdf/High_res_NGOstatement.pdf
Sinclair, M., Sheldrick, L., Moreno, M., and Dewberry, E. (2018). Consumer intervention mapping-a tool for designing future product strategies within circular product service systems. Sustain. For. 10:2088. doi: 10.3390/su10062088
Su, B., Heshmati, A., Geng, Y., and Yu, X. (2013). A review of the circular economy in China: moving from rhetoric to implementation. J. Clean. Prod. 42, 215–227. doi: 10.1016/j.jclepro.2012.11.020
Superti, V., Houmani, C., Hansmann, R., Baur, I., and Binder, C. R. (2021). Strategies for a circular economy in the construction and demolition sector: identifying the factors affecting the recommendation of recycled concrete. Sustain. For. 13:4113. doi: 10.3390/su13084113
Tsamboulas, D. A., Yiotis, G., and Mikroudis, G. (2007). A method for multi-criteria analysis in transportation infrastructure investments. Int. J. Transp. Econ. 34, 113–131. doi: 10.1016/j.tranpol.2006.06.001
Tu, J. C., Chan, H. C., and Chen, C. H. (2020). Establishing circular model and management benefits of enterprise from the circular economy standpoint: a case study of Chyhjiun Jewelry in Taiwan. Sustain. For. 12:4146. doi: 10.3390/su12104146
van Bueren, B. J. A., Argus, K., Iyer-Raniga, U., and Leenders, M. A. A. M. (2023). The circular economy operating and stakeholder model “eco-5HM” to avoid circular fallacies that prevent sustainability. J. Clean. Prod. 391:136096. doi: 10.1016/j.jclepro.2023.136096
van Stijn, A., Malabi Eberhardt, L. C., Wouterszoon Jansen, B., and Meijer, A. (2021). A circular economy life cycle assessment (CE-LCA) model for building components. Resour. Conserv. Recycl. 174:105683. doi: 10.1016/j.resconrec.2021.105683
Veleva, V., and Bodkin, G. (2018). Corporate-entrepreneur collaborations to advance a circular economy. J. Clean. Prod. 188, 20–37. doi: 10.1016/j.jclepro.2018.03.196
Vera-Puerto, I., Valdes, H., Correa, C., Agredano, R., Vidal, G., Belmonte, M., et al. (2020). Proposal of competencies for engineering education to develop water infrastructure based on “nature-based solutions” in the urban context. J. Clean. Prod. 265:121717. doi: 10.1016/j.jclepro.2020.121717
Virmani, N., Saxena, P., and Raut, R. D. (2022). Examining the roadblocks of circular economy adoption in micro, small, and medium enterprises (MSME) through sustainable development goals. Bus. Strat. Env. 31, 2908–2930. doi: 10.1002/bse.3054
Winans, K., Kendall, A., and Deng, H. (2017). The history and current applications of the circular economy concept. Renew. Sust. Energ. Rev. 68, 825–833. doi: 10.1016/j.rser.2016.09.123
Winning, M., Calzadilla, A., Bleischwitz, R., and Nechifor, V. (2017). Towards a circular economy: insights based on the development of the global ENGAGE-materials model and evidence for the iron and steel industry. Int. Econ. Econ. Policy 14, 383–407. doi: 10.1007/s10368-017-0385-3
Wiprächtiger, M., Haupt, M., Froemelt, A., Klotz, M., Beretta, C., Osterwalder, D., et al. (2023). Combining industrial ecology tools to assess potential greenhouse gas reductions of a circular economy. J. Ind. Ecol. 27, 254–271. doi: 10.1111/jiec.13364
Wisse, E. (2016). Assessment of indicators for circular economy: The case for the metropole region of Amsterdam, Utrecht, the Netherlands: Utrecht University.
Witjes, S., and Lozano, R. (2016). Towards a more circular economy: proposing a framework linking sustainable public procurement and sustainable business models. Resour. Conserv. Recycl. 112, 37–44. doi: 10.1016/j.resconrec.2016.04.015
Keywords: circular economy (CE), framework, transport infrastructure project, meso-scale, 4Wh-iReSOLVE, circular viaduct
Citation: Liu X, Schraven D, Ma W, de Jong M and Hertogh M (2024) Achieving a framework of the circular economy in urban transport infrastructure projects: a meso-scale perspective. Front. Sustain. 5:1475155. doi: 10.3389/frsus.2024.1475155
Edited by:
Yang Yang, Oxford Brookes University, United KingdomReviewed by:
Zhikang Bao, Heriot-Watt University, United KingdomGiacomo Di Foggia, University of Milano-Bicocca, Italy
Copyright © 2024 Liu, Schraven, Ma, de Jong and Hertogh. This is an open-access article distributed under the terms of the Creative Commons Attribution License (CC BY). The use, distribution or reproduction in other forums is permitted, provided the original author(s) and the copyright owner(s) are credited and that the original publication in this journal is cited, in accordance with accepted academic practice. No use, distribution or reproduction is permitted which does not comply with these terms.
*Correspondence: Xinyu Liu, eC5saXVAbGJvcm8uYWMudWs=; Daan Schraven, ZC5mLmouc2NocmF2ZW5AdHVkZWxmdC5ubA==