- 1Institute of Environmental Planning, Leibniz University of Hannover, Hanover, Lower Saxony, Germany
- 2Department of Civil and Environmental Engineering, Darmstadt University of Applied Sciences, Darmstadt, Hessen, Germany
- 3Center for Research on Sustainable Development Studies (CIEDES), University of Costa Rica, San José, Costa Rica
Unplanned urban expansion in Latin America has profoundly disturbed the natural hydrological processes of watersheds, particularly in tropical regions with extreme weather conditions. This has resulted in substantial runoff volumes, necessitating extensive engineering interventions to avoid damage. Urbanization has led not only to flooding problems, but also to a lack of recreational spaces for residents and an ecological degradation, negatively affecting the watersheds health. This study shows a methodology for creating scenarios making use of available sites for Nature-based-Solutions (NbS). Taking account of existing land cover, the methodology harnesses NbS multifunctionality to achieve hydrological, ecological and social benefits counteracting the threats to the health of a 23 km2 urban tropical watershed located in Costa Rica. In these scenarios, suitable public roads in residential areas were used as sites for implementing permeable pavement, bioretention cells, infiltration trenches and street planters, while appropriate flat roofs and car parks were converted into green roofs and permeable pavements in industrial areas. In addition, several green public spaces were transformed into multifunctional storage areas. Hydrological and hydraulic modeling was used to evaluate the performance of each scenario, while the increase in green area of each scenario was used as an indicator of the ecological and social benefits. The concurrent implementation of the residential, industrial and green space scenarios provides an area available for NbS of 165.3 ha (7.2% of the watershed area), potentially leading to a decrease of up to 63.7% in peak flow and up to 46.2% in runoff volume for a specific rainfall event. As haphazard urban growth is a common feature of most urban watersheds in Latin America, this methodology, based on the use of different land cover types to create scenarios for NbS implementation, is transferable to and adaptable for other tropical urban watersheds.
1 Introduction
Latin America is a highly urbanized region, with the majority of its population concentrated in urban areas. The continuing haphazard urban growth in Latin America is leading to increased water body channelization, sewage discharge, solid waste disposal, and reduced green spaces, which threatens connectivity, biodiversity, and riparian habitats (Walteros and Ramírez, 2020) without proper development plans for a healthy balance between impermeable built-up areas and permeable water-sensitive surfaces (Barros, 2004; Dos-Santos et al., 2021; Hack et al., 2024), resulting inter alia in accelerated runoff during precipitation, in turn increasing the risk of flooding and compromising the safety of residents.
Especially in the tropics, this hydrological response is exacerbated by intense, short-duration rainfall events. These generate higher peak flows in shorter times, causing more frequent flooding (Oreamuno and Villalobos, 2015). The traditional stormwater systems prevalent in Latin America prioritize rapid runoff conveyance, neglecting natural retention processes of the water cycle (IANAS and UNESCO, 2015; Jha et al., 2012).
Increased urbanization not only disrupts hydrological responses but also causes ecological degradation (e.g., habitat destruction, fragmentation and pollution) and social issues (e.g., heat islands, aesthetic degradation) (Hack et al., 2020; Hughes et al., 2014; Ohwo et al., 2015; Wiegels et al., 2021), and traditional drainage systems fail to address these problems, aggravating the health of watersheds and becoming unsustainable over time (Beißler and Hack, 2019; Hack and Schröter, 2021; Silveira, 2002; Verworn, 2002; Yazdanfar and Sharma, 2015).
A healthy watershed, according to the United States Environmental Protection Agency (U.S. EPA), is defined by the natural soil cover sustaining dynamic hydrological and geomorphic processes. This includes a habitat that supports native aquatic and riparian species, with water quality fostering robust biological communities (U.S. EPA, 2012). A healthy watershed is crucial for human wellbeing, acting as a natural filter for water, providing essential ecosystem services like pollination and pest control, and offering recreational opportunities such as fishing and hiking. Preserving watershed health is essential for sustaining ecosystems and enhancing human quality of life.
Urban watershed health is impacted by land cover changes disturbing hydrology, habitat, and water quality and leading to a complex set of stressors known collectively as the “urban stream syndrome”. With multiple factors contributing to degradation, a new integral focus on the recovery of hydraulic, ecological and social aspects is required (Hughes et al., 2014). Watershed recovery refers to systematic efforts aimed at improving or restoring the health of a hydrographic watershed, constituting an iterative adaptation process that requires updated methodologies to develop more effective results and protection strategies over time (U.S. EPA, 2012). The effectiveness of recovery strategies depends on matching interventions to the scale of the issue, whereby results may not be immediate due to prolonged recovery times (Hughes et al., 2014).
Optimizing the multifunctionality and connectivity of open spaces can enhance the carrying, adaptive, and regenerative capacities of urban landscape systems (Schlee et al., 2012). Watershed recovery projects emphasizing ecological, urban, and social aspects through participative management (de Alencar da Silva and do Amaral Porto, 2021) require a holistic approach integrating watershed hydrological processes (Hack and Schröter, 2021; Ward et al., 2001). Furthermore, recent watershed recovery projects are increasingly considering social and environmental challenges amid urbanization conflicts (Travassos and Schult, 2013). Nevertheless, despite theoretical progress, more work is needed to integrate all relevant aspects to guarantee effective water management (Bernhardt and Palmer, 2007).
Nature-based solutions offer an alternative to traditional drainage systems, promoting the conservation, improvement and creation of green spaces. NbS offer multifunctional benefits, such as pollutant reduction, runoff control, heat island mitigation, and ecosystem connectivity, significantly enhancing the quality of life. (Kabisch et al., 2017; Raymond et al., 2017).
However, NbS implementation in urban watersheds is limited by the scarcity of and competition for available space, the specific land use characteristics of settlements and the competing aims of the stakeholders concerned (Fluhrer et al., 2021). Each urban area has specific social and environmental conditions requiring tailored NbS designs, such as using streets and sidewalks in densely built residential areas with limited green spaces. By contrast, industrial areas are often characterized by large buildings with flat roofs and extensive parking lots that can constitute a significant portion of a watershed's impermeable area (Aparicio Uribe et al., 2022). However, they hold great potential for conversion to NbS for runoff control (Jayasooriya et al., 2020). The conservation and expansion of public green spaces are crucial in the rehabilitation of an urban watershed, however, within uncoordinated urbanized watersheds they are usually scarce and lack connectivity (Arthur and Hack, 2022), a situation exacerbated by informal settlements in river protection zones hindering habitat conservation and biodiversity enhancement.
With the help of hydrological models, considerable effort has been invested in analyzing the effects of urban growth on increasing flood risks (Bonilla Brenes et al., 2023; Osuide, 2021). Simulating site conditions, these models allow the magnitude and spatial distribution of the problem to be assessed (Rossman and Huber, 2016). Moreover, they can incorporate NbS in various scenarios, assessing their effectiveness in mitigating flood risks. However, such scenarios can only be evaluated by these models from a technical, i.e., hydrological perspective. Applying a scenario approach and including further functional perspectives—alongside hydrological recovery—this study assesses how NbS can contribute to the ecological and social recovery of an urban watershed. Based on a detailed, multi-level spatial analysis of the existing urban green infrastructure (UGI) and urban land use, the siting potential for different NbS types was determined for residential and industrial areas as well as for open green spaces. In combination with a deficit analysis of the ecological and social service provisions of the existing UGI, NbS implementation scenarios aimed at improving hydrological functions, ecological connectivity and public green space accessibility were developed as watershed recovery strategies. These scenarios were subsequently modeled to assess their hydrological performance with regard to flood reduction.
Within a six-year research project, the SEE-URBAN-WATER (SUW) research group (www.see-urban-water.uni-hannover.de) developed multifunctional NbS scenarios based on different area characteristics aiming the improvement of existing green infrastructure and reducing flood risks in tropical urban watersheds, using the Quebrada Seca watershed in the Greater Metropolitan Area (GAM) of Costa Rica as a case study area (Hack et al., 2023). The watershed faces significant environmental and safety challenges due to extensive residential and industrial development, including reduced pervious land cover, inadequate graywater treatment, and solid waste disposal issues. Legal actions, such as Resolution 4050 of the Constitutional Court of Costa Rica, highlight the need for alternative water management solutions, where NbS can play a crucial role in mitigating runoff, enhancing water quality, and restoring biodiversity.
Looking for a response to the following research question: What are potential ecological and social benefits of NbS scenarios for different land uses and how do they relate to flood reduction for the entire watershed and in different parts of it? This study builds on and integrates the findings from prior research conducted in the Quebrada Seca watershed on how NbS can mitigate flood risks and improve environmental and social conditions in the Quebrada Seca watershed, considering different land cover types and the available space for NbS in different land use contexts. Addressing the hydraulic issue of recurrent floods exacerbated by urban growth, NbS implementation offers a holistic (multifunctional) approach integrating social and ecological considerations, while enhancing watershed resilience and promoting comprehensive recovery. Fostering a system of complementary solutions, this approach contrasts with conventional hydraulic structures which fail to address broader environmental and social challenges associated with urbanization, such as pollution, deforestation, and fragmentation.
2 Materials and methods
2.1 Study area
The Quebrada Seca watershed, located in the northwestern sector of the Greater Metropolitan Area (GAM) on the Pacific side of Costa Rica, encompasses approximately 23 km2, with elevations ranging from 869 m.a.s.l. to 1,626 m.a.s.l. (Chen et al., 2021) With an average slope of 20%, it consists of three watercourses: Quebrada Seca, Burío River, and Quebrada Aries (Masís-Campos and Vargas-Picado, 2014). Politically, the watershed covers six municipalities: Alajuela, Barva, Belén, Flores, Heredia, and San Rafael (Figure 1).
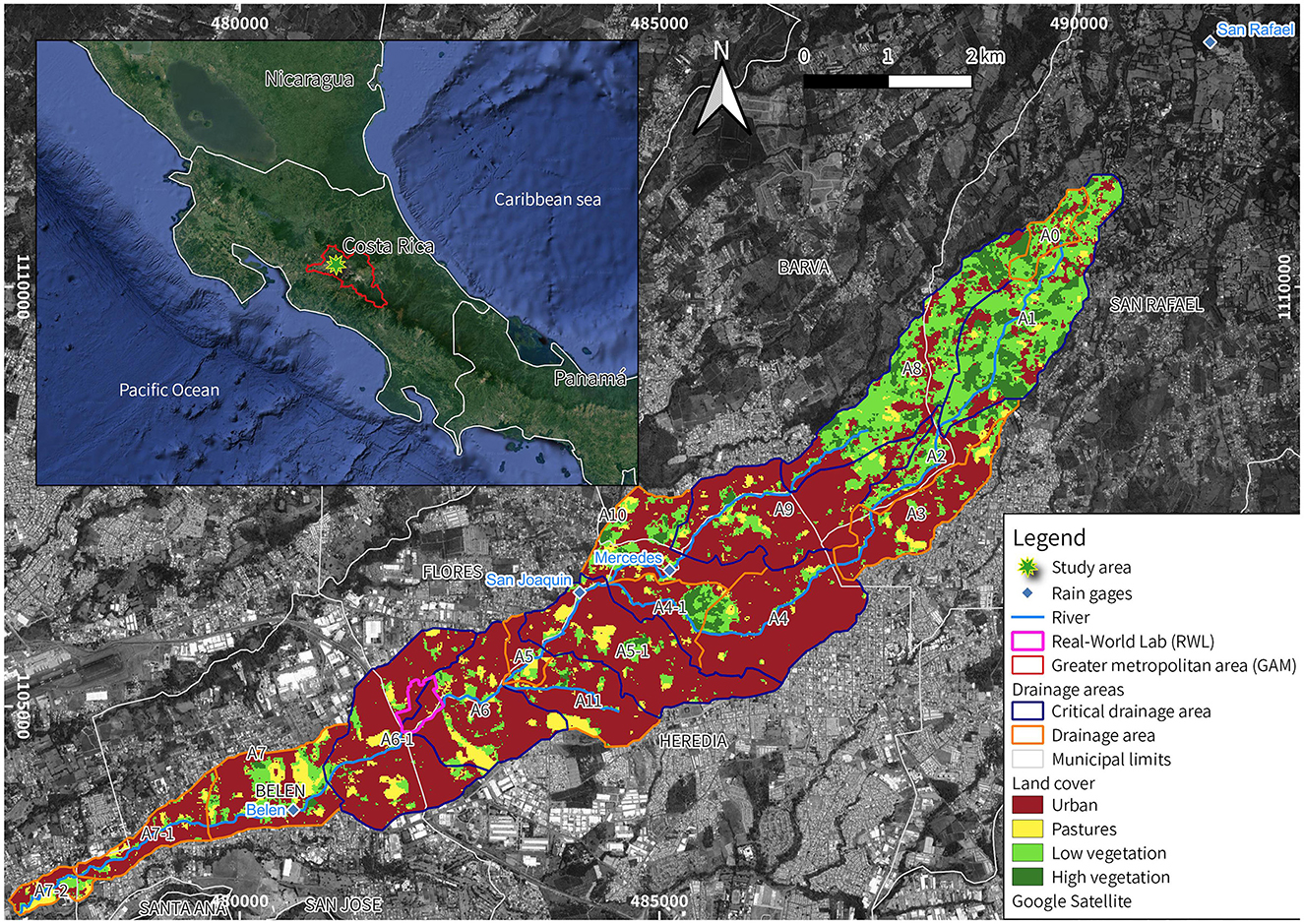
Figure 1. Location of the study area, Real-World Lab, drainage areas, municipal limits and land cover classification.
The watershed experiences distinct dry and rainy seasons, with an average annual temperature of 24.8°C and around 2,000 mm of precipitation (Masís-Campos and Vargas-Picado, 2014; Solano and Villalobos, 2012). The watershed is home to ~116,000 people, with an average density of 4,160 per square kilometer, nearly half of whom reside in the canton of Heredia (Masís-Campos et al., 2020).
Currently, 66% of the watershed is urbanized, a sharp increase from just 1.6% in 1,945 when the land was primarily coffee plantations, pastures, and wooded vegetation (Bonilla Brenes et al., 2023). Unfortunately, the increase in impermeable coverage occurred without a comprehensive land-use plan guiding residential and industrial expansion or road infrastructure development. In the same vein, there was little or no planning of drainage systems to control the extra runoff volume generated by urban growth. The 64% increase in impermeable coverage between 1,945 and 2,019 resulted in an almost 80% surge in the basin's total runoff volume and a reduction of 25 min (20%) in the time-to-peak of the hydrograph, essentially tripling its peak flow and maximum specific discharge (Bonilla Brenes et al., 2023).
Offering < 7.4 m2 of green space per inhabitant (Arthur and Hack, 2022), the remaining green areas feature limited connectivity and biodiversity, underscoring the urgent need for coordinated efforts toward sustainable urban development.
2.2 Watershed recovery
The methodology for analyzing the recovery potential of the Quebrada Seca watershed is based on the U.S. EPA approach (U.S. EPA, 2012) in which six main aspects of a healthy watershed are defined: (1) Landscape condition, (2) Habitat, (3) Hydrology, (4) Geomorphology, (5) Water Quality, and (6) Biological State. These aspects were analyzed within an urban context, to identify key issues characterizing specific vulnerabilities of the study area. Figure 2 shows the aspects considered to detect the watershed's vulnerabilities and recovery potential, forming the foundation for NbS implementation scenarios. The focus of this study lies on landscape condition, habitat, hydrology and geomorphology, while water quality and biological condition were not evaluated due to a lack of relevant information.
After identifying vulnerabilities, recovery potential was assessed for each aspect. Using insights from previous SUW studies, scenarios were created for residential, industrial, and green spaces to explore the achievable recovery levels for each land type.
The SUW project employed a Real-World Laboratory (RWL) approach (Fluhrer et al., 2021) as a tool for conducting transdisciplinary research to explore public perceptions, social acceptability, and willingness to adopt NbS, as well as investment capacity and local implementation costs, through activities such as meetings, interviews, workshops, and environmental initiatives in addition to the co-design and experimental prototyping of NbS (Chapa et al., 2020, 2023; Neumann and Hack, 2020, 2022; Perez Rubi et al., 2024; Pérez Rubi and Hack, 2021). A four-step methodology for assessing the implementation of retrofitted, multifunctional NbS in public spaces was developed, including: (1) site analysis, (2) setting design criteria and strategies, (3) exploring multifunctionality to create spatial typologies, and (4) evaluating spatial suitability for NbS placement (Fluhrer et al., 2021). The prioritized criteria for NbS implementation were: runoff control in the hydrological dimension to reduce stormwater via infiltration and retention; biodiversity promotion in the ecological dimension by enhancing connectivity and ecological value; and accessibility in the social dimension to improve walkability, traffic calming, and connection to green spaces for recreation. Subsequently, the knowledge acquired in the RWL was applied to formulate NbS implementation scenarios in specific drainage areas designated for residential and industrial use, as well as for the entire watershed (Aparicio Uribe et al., 2022; Chen et al., 2021). These were developed with the aim of optimizing the existing urban green infrastructure, emphasizing crucial aspects of its multifunctionality (Arthur and Hack, 2022).
To mitigate the vulnerabilities identified (Figure 2), the study assessed the multifunctional benefits of NbS scenarios, focusing on hydrological, ecological, and social aspects. Going beyond a purely technical perspective focused on reducing flood risk in the watershed, the study uses a comprehensive approach encompassing progress in the social domain and enhancements in ecological conditions and aiming to provide a tool for urban planning policy-making fostering economic sustainability.
The hydrological benefits were quantified using the results from the model to determine the level of improvement of each scenario in reducing flood risk based on estimations of runoff volume, peak flow, and maximum specific flow in each drainage area within the watershed. This indicator is directly related to the hydrological and geomorphological aspects of the methodology shown in Figure 2, as it describes the hydrological efficiency of each scenario in terms of its capacity to re-establish the natural processes of the hydrological cycle and educe flood risk.
To quantify ecological benefits, it was hypothesized that increasing vegetative cover at strategic sites would enhance connectivity of biological corridors and contribute to habitat recovery (Figure 2) (U.S. EPA, 2012). This would be achieved by increasing structural habitat heterogeneity and providing habitats for biodiversity in urban green areas (Monberg et al., 2018). Therefore, the expansion of green areas achievable through each proposed scenario was considered as an indicator of ecological benefit.
Turning to the social benefits, an increase in green public space per inhabitant and improved spatial distribution (accessibility to citizens) were regarded as variables for quantifying potential social benefits offered by NbS, following the consideration that providing urban biodiversity will facilitate environmental recreation and education (Rupprecht and Byrne, 2014) as well as enhancing human health (Grahn and Stigsdotter, 2003) and improving landscape conditions.
2.3 Scenario development
Complementing the available space aspect with the watershed health aspects for hydrological, ecological and social recovery potential, three types of NbS implementation scenarios were defined. Reflecting the main land uses in the watershed, these focused on the potential of the urban area (residential and industrial) and the pasture and vegetation areas (types of green spaces) (Figure 1). These scenarios were based on previous research results of the SUW research group. Figure 3 outlines the methodology used to construct the scenarios.
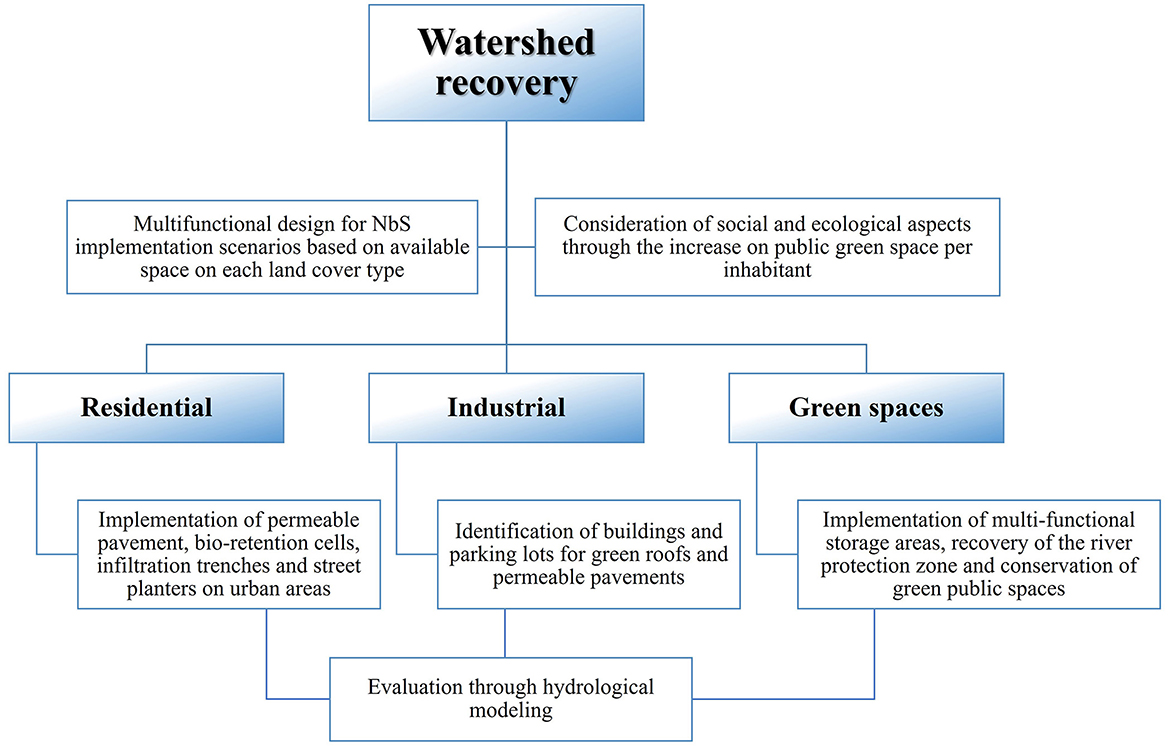
Figure 3. Methodology based on previous studies for creating NbS implementation scenarios and reflecting the three defined main land uses in the watershed: residential, industrial and green spaces.
2.3.1 Residential area scenario (RWL co-design insights, space availability)
The residential area analysis was initiated at neighborhood level with the objective of defining the potential for NbS implementation at specific key sites. This analysis laid the groundwork for upscaling to watershed-level scenarios.
2.3.1.1 Neighborhood level
The focus of the residential level scenarios was on land cover classification, with the aim of identifying potential sites for implementing NbS in public spaces. Leveraging the findings of Fluhrer et al. (2021) in a Real-World Lab (RWL) established by SUW in the watershed's midsection (Figure 1), this methodology starts out from residents' preferences for NbS types and strategic sites identified during a comprehensive co-design process in the RWL, followed by a thorough physical analysis of each site. Key landscape elements, topography, the spatial distribution of properties, green spaces, land uses, drainage systems, and the road network were meticulously evaluated to define the area's physical characteristics.
Following a site's characterization, design criteria and NbS types were formulated, creating hypotheses to harness the multifunctional nature of NbS. Priority goals for each NbS type, including flood risk reduction, ecological recovery, social cohesion, and urban planning, were established. Subsequently, an evaluation of potential NbS elements was conducted in accordance with their functions, with the most suitable ones selected on the basis of multifunctionality, geometric characteristics, and implementation requirements (Fluhrer et al., 2021). The final step involved drafting design proposals with suggestions for location, dimensions, and technical configurations, taking account of space availability to maximize benefits and ensure social acceptance and appropriation by residents (Chapa et al., 2023).
This methodology yielded a preliminary neighborhood-level scenario for the RWL featuring infiltration trenches (IT), bioretention cells (BC), permeable pavement (PP), and street planters (SP). In a subsequent study (Aparicio Uribe et al., 2022), two further study areas, characterized by different urban configurations, were analyzed to assess their potential for NbS implementation, considering site-specific space constraints. This analysis resulted in the development of two distinct neighborhood-level scenarios, each with varying potential for implementing IT, BC, PP, and SP. These efforts culminated in three neighborhood-level scenarios with varied potential for NbS implementation, defined as Neighborhood 1-Real-World Lab (N1-RWL), Neighborhood 2 (N2) and Neighborhood 3 (N3), as illustrated in Figure 4.
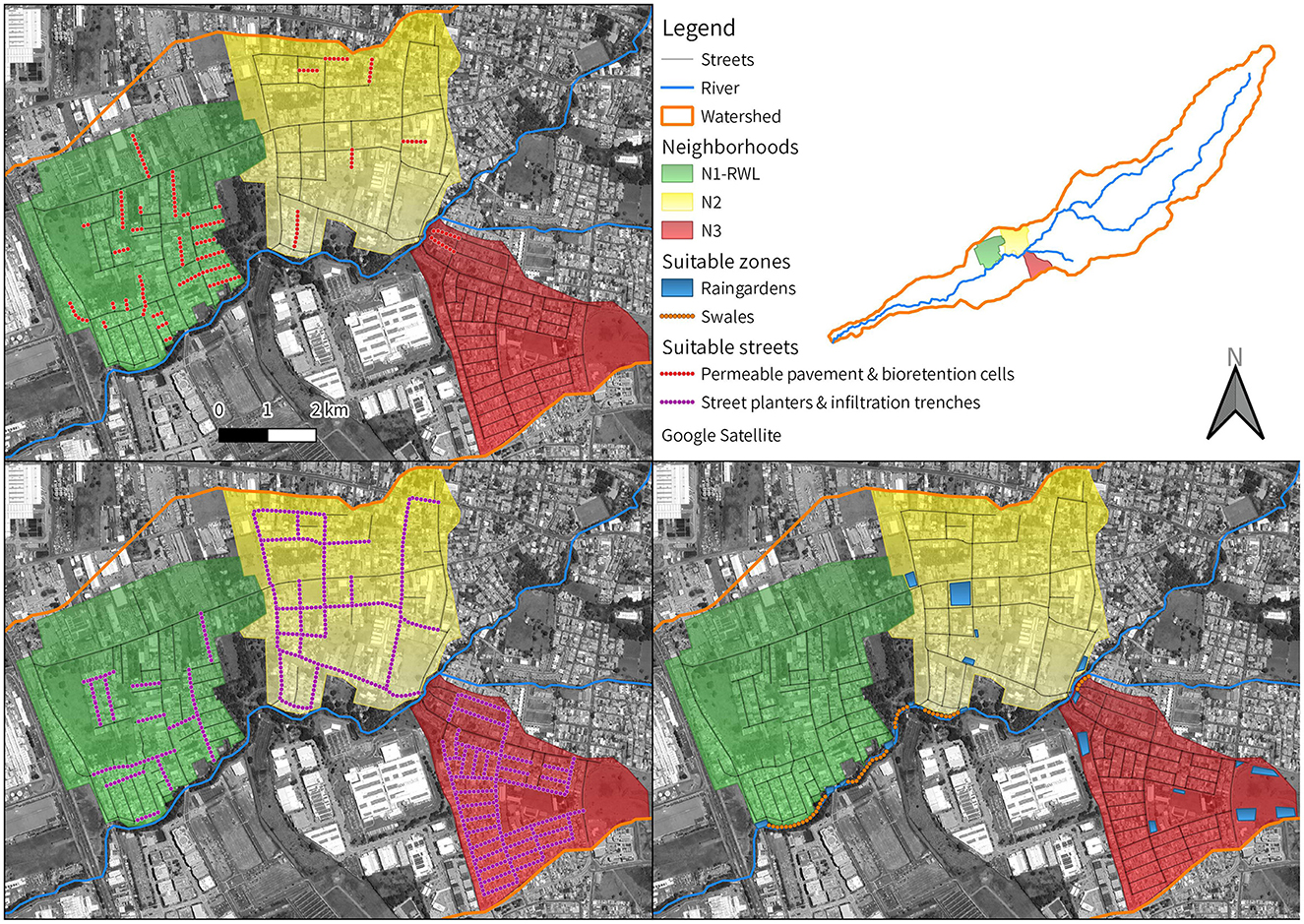
Figure 4. Initially identified NbS implementation potential for residential areas at neighborhood level (Aparicio Uribe et al., 2022).
2.3.1.2 Watershed level
The neighborhood-level scenarios served as a conceptual basis for extending the potential for NbS implementation to the watershed level. The primary objective was to formulate comprehensive scenarios at watershed level for residential areas, allowing an examination of the hydrological response of the entire watershed following deployment of IT, BC, PP and SP. A classification of the entire urban area was conducted, examining urban configuration, property distribution, and road networks, leading to the categorization of the residential area into three groups (N1, N2 and N3) based on neighborhood-level potentials (N1-RWL, N2, N3). The hypothesis underlying this classification was that residential areas sharing similar urban characteristics and road network configurations would exhibit similar potentials for NbS implementation. Figure 5 illustrates the classification of the total urban residential area based on the potential initially identified for N1, N2, N3.
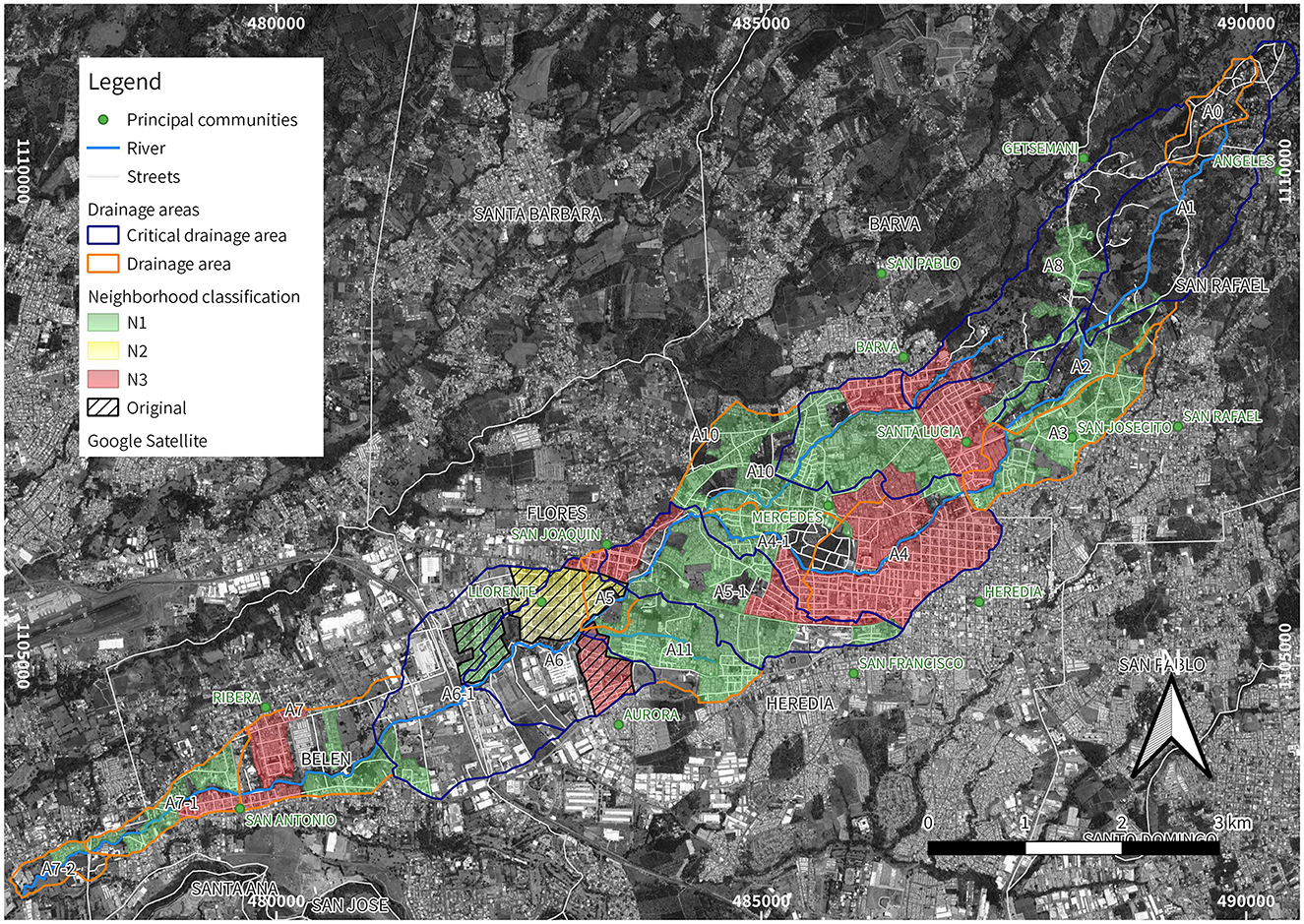
Figure 5. Classification of the total urban residential area of each drainage area, based on the potential initially identified for N1-RWL, N2, N3.
2.3.2 Industrial area scenario (adaptation of existing built structures)
The industrial area scenario is based on the results obtained from Arthur and Hack (2022) where extensive parking lots and large buildings with flat roofs (comprising shopping centers, supermarkets, free-trade zones, and other industrial buildings) throughout the watershed were identified. To determine the optimal areas for NbS implementation, a land cover classification was carried out, based on Google Earth imagery and Open Street Map data, with the objective of identifying all appropriate buildings and parking areas located mainly within the industrial estates situated in the middle part of the watershed. The scenario focuses on transforming parking lots into permeable pavement and using buildings with extensive flat or low-pitched roofs to implement green roofs (see Arthur and Hack, 2022 for details). Available sites for green roofs (buildings) and permeable pavement (parking lots) are shown in Figure 6.
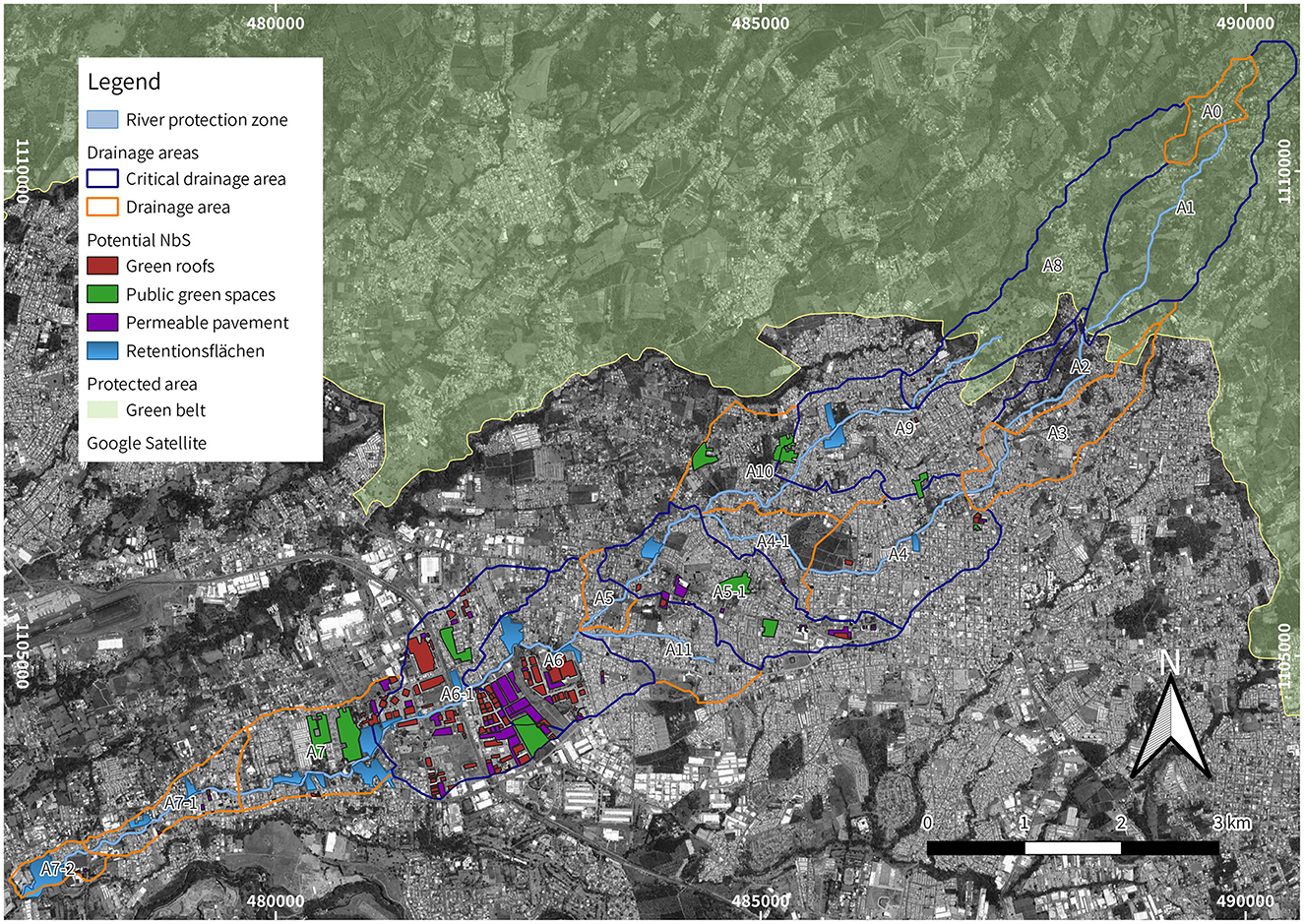
Figure 6. Potential available areas for NbS in each drainage area. River protection zone and green belt.
2.3.3 Green space scenario (undeveloped public areas and ecosystem restoration)
The scenario considering green spaces addresses the optimization and harnessing of existing green spaces and unbuilt areas, with a view to enhancing habitat connectivity and increasing accessibility to as well as availability of public green spaces. This includes restoring river protection zones and establishing multifunctional storage areas to retain river runoff.
To identify potential NbS sites at watershed level, insights from Arthur and Hack (2022) were used. In this study, the authors conducted a land cover classification, determining space availability and identifying spaces lacking vegetation areas within the watershed. The degree of fragmentation and connectivity of urban ecosystems, crucial for ecological processes, were assessed through an analysis of the spatial distribution of vegetation patterns. Larger and less fragmented areas were prioritized for greater ecological benefits (Beninde et al., 2015). The evaluation of accessibility to public green spaces involved establishing a criterion where each resident should have access to a public green area of at least 0.5 hectares within 300 meters of their home, or a minimum area of 10 hectares within 700 meters for settlements. This criterion was based on various recommendations for maximum distance and minimum area of public green spaces (Grunewald et al., 2016; Handley, 2003; World Health Organization Regional Office for Europe, 2016).
Areas within 300 meters with low vegetation cover and bare soil (< 10%) were selected, with a view to identifying multifunctional zones for runoff retention along the river. Criteria prioritized ecological, hydraulic, and social benefits, addressing flooding reduction, while consideration of such factors as accessibility, recreation, biodiversity, habitat recovery, and cultural values (Arthur and Hack, 2022). Lopes Monteiro et al. (2023) created a scoring methodology for the potential storage sites (defined in Arthur and Hack, 2022), based on structure location in critical and flood-prone areas. Priority was given to areas with bare soil exceeding the average (0.9 hectares) for increased storage capacity and social-ecological benefits. Sites enhancing public green space per inhabitant were prioritized for improved accessibility and recreation. Sites with native vegetation, unmodifiable buildings, or no river connection due to street crossings were excluded. This methodology allowed 12 multifunctional storage areas to be defined.
As part of the scenario development, restoration of the river protection zone was considered, in accordance with Article 33 of the National Policy on Protection Areas for Rivers, Creeks, Streams, and Springs by the Ministry of Environment and Energy of Costa Rica (MINAE). This policy mandates a protective strip of 10 m in urban areas, measured horizontally on both sides of a river, ensuring the preservation of this zone to avoid changes to the river's hydraulic capacity and mitigate flood risks during precipitation events. The proposed recovery involves revegetation with species conducive to slope stability and the improvement of natural habitat conditions, guided by Subcomisión de Heredia–CGICRG Tárcoles (2016). Figure 6 shows the potential sites for the implementation of green roofs, permeable pavement, multifunctional storage areas, river protection zones and conservation of green areas.
An overall scenario unifying the residential, industrial, and green space scenarios was ultimately developed, encompassing the entirety of available areas for NbS implementation. Its primary objective was to assess the potential extent to which the watershed's health and ecological balance could be restored through implementing NbS in all available areas.
2.4 Hydrological and hydraulic modeling
This study employed the EPA Storm Water Management Model Version 5.2 (SWMM) to simulate the watershed's response to precipitation events. SWMM's hydraulic transport component facilitates runoff routing through pipes, channels, junctions, and outfalls at the sub-watershed level, including the implementation of NbS using the “Low Impact Development Control” tool to assess their effectiveness in mitigating flooding risks across the Quebrada Seca drainage area. The model calculates runoff and conduit flow rates and depths for each sub-watershed across multiple time steps, utilizing various hydrological and hydraulic processes such as time-varying rainfall, evaporation, interception, flow routing, surcharging, and ponding. The routing method employed was the “dynamic wave”, providing the most theoretically accurate results (Rossman and Huber, 2015).
Rainfall abstractions were determined using the Natural Resources Conservation Service (NRCS) method (Chow et al., 1994), with infiltration calculated via the Curve Number (CN) method based on land use and hydrological soil groups. CN values of 55, 73, 68, and 92 were assigned to high vegetation, low vegetation, pastures, and urban cover, respectively, based on a hydrological soil group categorized as B (Oreamuno and Villalobos, 2015). The model is based on a Digital Elevation Model (DEM) with a 1-meter pixel size, sourced from the National Land Information System of Costa Rica (SNIT), and soil type information obtained from the Ministry of Agriculture and Livestock (MAG) database (Oreamuno and Villalobos, 2015). Under natural conditions, volcanic rock has developed into highly permeable soils (Masís-Campos and Vargas-Picado, 2014), conducive to aquifer recharge and surface runoff control. Refer to Supplementary Tables A1–A3, Figure A1 in the Appendix for details on modeling parameters, soil types, and calibration and validation.
To facilitate a distributed analysis, the watershed was subdivided into 17 drainage areas (Figure 1). This division was guided by topographic considerations and the characteristics of drainage systems, allowing for a more specific analysis of both hydrological and hydraulic conditions across the watershed.
Calibration and validation of the model involved the use of rainfall records and river water levels obtained at 5-min intervals from four key stations: Belén, San Joaquín, Heredia, and San Rafael (Figure 1). These stations are integral components of the Early Warning System (EWS) managed by the Municipality of Belén, located at the downstream end of the watershed. Thiesen polygons methodology was applied to spatially distribute the precipitation over the drainage areas. The dataset used for the analysis spans a period from January 2020 to September 2022. Within this time range, 20 carefully selected precipitation events were examined in order to calibrate and validate the model. They were chosen based on their representation of the hydrological conditions within the watershed and the elevated risk of flooding, as indicated by EWS-recorded levels. The results of this study refer specifically to the precipitation event observed on 29 September 2020, with a total rain volume of 138 mm. It shows concurrent precipitation occurrence in Belén and the central basin, with a concentration in San Joaquín and/or Heredia. Events of this type exhibited a duration of 1.6 h, with the peak water level in Belén occurring within the initial 30 min. The precipitation volume capable of inducing overflow or flooding at the lower part of the watershed has a return period lower than 2 years according to the study by Oreamuno and Villalobos (2015).
3 Results
3.1 Evaluation of ecological and social benefits of the NbS scenarios
The increase in green areas provides significant social and ecological benefits, improving both environmental sustainability and quality of life. Ecologically, green spaces may support biodiversity by providing habitats when properly designed and managed, help regulate urban temperatures by reducing the heat island effect, improve air and water quality by filtering pollutants, and contribute to climate change mitigation through carbon sequestration (Babí Almenar et al., 2021). Socially, they can enhance mental and physical health by offering spaces for relaxation and exercise, foster social cohesion through communal gathering spots, increase property values, and provide opportunities for environmental education (Giannico et al., 2021). These benefits are interconnected, as ecological improvements, such as cleaner air and better water management, directly enhance public health and overall liveability, creating a positive cycle of mutual reinforcement between human wellbeing and environmental health (Nguyen et al., 2021).
3.1.1 Residential area scenario
The residential area scenario is the most complex in terms of available space, due to the high percentage of built-up land and the scarce existence of public green areas. Therefore, the scenario focuses on harnessing the area available along public roads. 1.6 ha of vegetative area along these roads was found to be available, equivalent to 0.1% of the total area of the Quebrada Seca watershed. Through its recovery, new public green space of 0.1 m2/inhabitant could be achieved. The NbS implementation potential of this scenario depends on the landscape distribution of each neighborhood type (N1-3) analyzed.
With NbS implementation in this scenario primarily within the remit of public administration, installation of such solutions could take place during road restoration work or new road developments, facilitated by updated technical standards such as the country's new Hydrological Code.
3.1.1.1 Neighborhood type 1 (N1)
The total area identified as N1 covers 724 ha or 31.7% of the entire watershed. Predominantly situated in the middle-upper part of the watershed, 16.8% of N1 drains into drainage area A9 and 14.6% into drainage area A5-1 (Figure 5). Both drainage areas are identified as critical due to increased runoff volume generation (Bonilla Brenes et al., 2023). The remaining N1 area drains into drainage areas A10, A11, and A3, accounting for 12.1%, 12.3%, and 11.6%, respectively.
Implementing NbS in Neighborhood Type 1 (N1) is seen as a relatively achievable process due to the available space and favorable traffic conditions. The potential NbS area was determined by assessing the available space along residential, secondary, and service roads, building on findings from the study by Aparicio Uribe et al. (2022). The aggregate area of these roads within the N1 zone amounts to 95.8 ha. Within this area, there is potential for implementing various NbS elements: 1.8 ha for permeable pavement, 0.2 ha for bioretention cells, 0.2 ha for street planters, and 0.2 ha for infiltration trenches. Their strategic implementation would enhance sustainable practices and mitigate runoff-related challenges in critical areas.
3.1.1.2 Neighborhood type 2 (N2)
Implementing NbS in Neighborhood Type 2 (N2) areas within the watershed is more complex than in N1 due to higher road connectivity (absence of dead-end roads) and higher traffic volumes. Covering 63.5 ha, this scenario accounts for 2.8% of the total watershed area. A large share of N2 belongs to drainage area A6 (63.4%) and drainage area A6-1 (18.3%), both of which are assigned to the critical category due to intensified runoff volume generation during precipitation events (Bonilla Brenes et al., 2023), with the remainder belonging to drainage area A5 (16.7%). All of the N2 area is concentrated in the middle part of the watershed. The available road area for NbS is 7.2 ha, offering opportunities for specific interventions. A surface of 0.04 ha can be allocated to permeable pavement, providing a sustainable alternative for a portion of the street space. However, the available area for bioretention cells is limited to 0.004 ha, while the suitable space for street planters and infiltration trenches is 0.02 ha for each structure. Despite its limited area, strategic NbS implementation in N2 would address runoff challenges in critical drainage areas, contributing to the watershed's overall sustainability.
3.1.1.3 Neighborhood type 3 (N3)
The N3 scenario is more challenging due to its higher traffic density, limited available space, and complex road configurations. This urban layout predominates in the primary urban centers within the watershed and its outskirts. In addition to integrating NbS within the designated N3 area, a transformative approach modifying streets connecting the four main quadrants of these urban centers is proposed. These quadrants typically house public institutions such as municipalities, schools, churches, social recreation sites, parks, sports squares, and various commercial establishments. Inspired by practices in many European cities (Yassin, 2019), the objective is to reclaim public space currently dedicated to vehicle traffic and repurpose it for pedestrians through NbS and modifications to the road network. Urban centers classified under N3 within the study area include San Antonio, La Ribera, and Santa Lucia. Furthermore, the centers of Heredia, Barva, San Rafael, Llorente, Mercedes Norte, Mercedes Sur and San Joaquin, located outside the N3 area, were also considered.
Considering the cross profile of secondary roads and quadrant lengths, modifying all urban centers would yield a total area of 4.4 ha. Allocating 85% of this available area would allow for 2.8 ha of permeable pavement, primarily used by pedestrians. The remaining area would be used for bioretention cells (0.2 ha), and for street planters and infiltration trenches (0.8 ha). The total N3 area covers 427.7 ha, with drainage areas A4, A9, A5-1, and A6 constituting 37.4%, 19.3%, 9.8%, and 7.3% respectively. Situated in the middle-upper part of the watershed, these drainage areas are classified as critical areas due to their high runoff volume generation (Bonilla Brenes et al., 2023). The available road area for NbS incorporation totals 66.1 ha, enabling the realization of 0.18 ha of permeable pavement, 0.02 ha of bioretention cells, 0.3 ha of street planters, and 0.3 ha of infiltration trenches. This comprehensive strategy aims to address the challenges posed by vehicular density and paves the way for sustainable urban development.
3.1.2 Industrial area scenario
This scenario entails collaborating with private companies, crucial individual contributors to a significant portion of the watershed's impervious cover, and thus major runoff generators. The scenario is based on identifying extensive flat roof areas suitable for greening. Additionally, extensive parking lots suitable for permeable pavement were identified, referencing insights from Aparicio Uribe et al. (2022) and Arthur and Hack (2022).
Although the analysis covers the entire watershed, a major portion of the available surface area is concentrated in drainage areas A6, A6-1, and A5-1, situated in the central segment of the watershed and categorized as critical runoff generation areas. The identified roof area suitable for greening totals 45.7 ha, with 61% located in area A6-1 and 26% in area A6. The remaining area is distributed among supermarkets and other industrial buildings across the watershed, notably in areas A7, A4, and A5-1, constituting 5.2%, 3.1%, and 2.4% respectively.
Parking lots totaling 31.3 ha are outlined, primarily concentrated in drainage areas A6, A6-1, and A5-1, and making up 39.0%, 34.8%, and 13.7% respectively of the total parking area. The remaining parking areas are located in areas A7 (5.1%) and A11 (2.4%). The strategic implementation of permeable pavement is emphasized, given its substantial impact on reducing runoff volumes, as highlighted by Chen et al. (2021). Such an NbS intervention is particularly significant due to its strategic placement within three critical areas in the central part of the watershed. Collaboration with private entities, coupled with targeted NbS implementation, emerges as a promising strategy to strengthen the watershed's resilience and address runoff challenges effectively.
Through fully implementing the industrial scenario, up to 45.7 ha of green areas could be recovered, equivalent to 2% of the total area of the basin and generating 4.0 m2 of new green area per inhabitant.
3.1.3 Green space scenario
This scenario targets the restoration of the protection zone along the river, mandated by law to be a 10-meter buffer on each side of a river in urban areas. This designated zone serves as essential floodable space to accommodate changes in river levels during precipitation events, necessitating low vegetation cover that does not impede the natural flow of the water body. For the Quebrada Seca stream, the protection zone has a surface area of ~48.1 ha. It is noteworthy that about 14% of this zone was identified as built up, based on land cover classification, obstructing the river's natural flow and leading to higher flooding risks.
Complementing this protective measure, potential areas for runoff volume retention during rainfall events were identified and modeled (Arthur and Hack, 2022; Lopes Monteiro et al., 2023). These areas could serve both as storage zones for managing runoff in response to intensive rainfall and as recreational spaces during dry seasons. Drawing on insights from the studies of Arthur and Hack (2022) and Lopes Monteiro et al. (2023), an estimated 30.2 ha exhibit retention potential, distributed across drainage areas A7-2 (33.6%), A6-1 (27.4%), A9 (15.0%), A5-1 (9.9%), A7 (6.6%), A7-1 (4.2%), and A4 (3.3%). A4, A5-1, A6-1 and A9, were classified as critical areas due to their significant runoff volume generation.
In addition to addressing hydrological concerns, this scenario identifies potential green spaces for conservation totaling approximately 46.1 ha. Strategically located primarily in drainage areas A7 (35.3%), A6-1 (23.9%), A5-1 (14.7%), and A10 (13.3%), these green spaces could not only serve as recreational areas but also as crucial inter-urban biological corridors, enhancing the overall ecological conditions and connectivity of the watershed. Furthermore, consideration was given to the protected area outlined by GAM's Green Belt, revealing that drainage areas A8, A1, A0, and part of A2 fall within this demarcated protective zone.
Focusing on multifunctional storage areas and the conservation of public green areas, this scenario provides the greatest recovery potential in ecological terms due to the nature of its development in taking advantage of existing green areas. It would recover up to 83.2 ha of green public spaces, equivalent to 3.6% of the total area of the watershed, thus achieving an increase in green areas of 7.2 m2/inhabitant.
This comprehensive protection and retention scenario emerges as a holistic approach to strengthening the watershed's resilience, encompassing legal compliance, hydrological management, and ecological enhancement for sustainable and integrated watershed development.
3.1.4 Overall scenario for NbS implementation
Featuring the maximum use of available space within the watershed through implementation of all three scenarios, the overall scenario presents a maximum NbS potential of 165.3 hectares, corresponding to 7.2% of the total watershed area. Figure 7 shows the combination of residential, industrial and green space scenarios within the watershed.
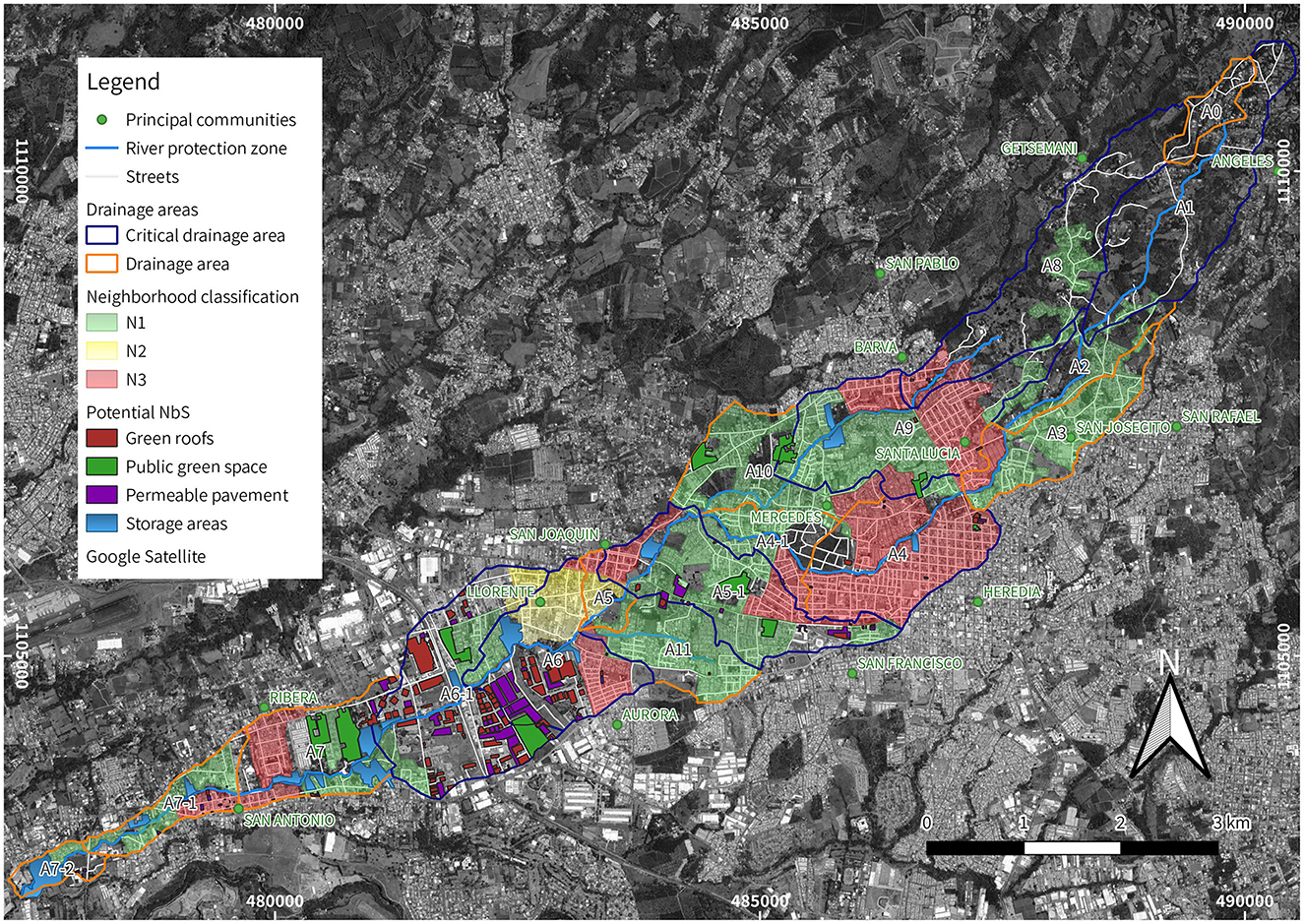
Figure 7. Potential sites for NbS implementation of the residential area (N1-N3), industrial area (green roofs, permeable pavement) and green space (retrofitted public green space, storage areas) scenarios.
Drainage area A6-1 exhibits the greatest potential, with 58.8 hectares (equivalent to 25.2% of its area) available for NbS interventions. This is of great significance for the overall recovery of the watershed as A6-1 is categorized as critical and is located in the middle part of the watershed, this means that NbS effects will help reduce the risk of flooding in the drainage areas located downstream, in addition to providing greater connectivity of green areas to counteract the adverse effects of the extensive impervious surfaces of the industrial estates. Drainage area A6 ranks second in terms of available space, covering 26.9 hectares (15.5% of its area). Situated in the mid-lower part of the watershed, both A6-1 and A6 are considered critical areas. A7 is the third drainage area with significant availability, with a potential to transform 16.3% of its total area (22.7 hectares). Further significant NbS potential lies in the critical drainage area A5-1, mainly due to its location in the middle part of the watershed and its proximity to the main urban centers of San Joaquín, Mercedes and Heredia where a large share of the population is concentrated. In A5-1, 16.2 hectares (8.2% of its total area) are available for NbS, improving the connectivity of green spaces in the middle part of the watershed and helping reduce the risk of flooding in the lower part of the watershed. Table 1 illustrates the total available area for NbS for each scenario and drainage area.
3.2 Hydraulic modeling of NbS scenarios
The hydraulic analysis examined the watershed's hydrological response to the September 29, 2020, precipitation event, notable for its extreme hydrological conditions and documented impact as an event contributing to flooding in the lower watershed. The event was characterized by concentrated convective rainfall in the middle-upper part of the watershed, specifically in the San Joaquín and Heredia sectors. Recorded precipitation during this event amounted to 59.4 mm in Heredia, 54.1 mm in San Joaquín, 23.7 mm in Belén, and 0.8 mm in San Rafael.
NbS effectiveness is manifested in various ways within the model, depending upon the specific NbS structures considered in each scenario. The visualization of modeling results varies, encompassing a spatially distributed examination of independent drainage areas (distributed analysis) and an evaluation of junctions (watershed analysis). The distributed analysis allows the NbS effects in specific drainage areas to be identified for each scenario, while the watershed approach permits an in-depth analysis of the cumulative runoff volumes and their consequent impacts on downstream sectors.
3.2.1 Distributed analysis for each scenario
Depicted in Figure 8, the results highlight the percentage reduction achieved in peak flow, runoff volume, and maximum specific flow in comparison to the baseline scenario, for each individual drainage area and for each NbS scenario in a distributed manner, without considering the effects from the upstream drainage areas.
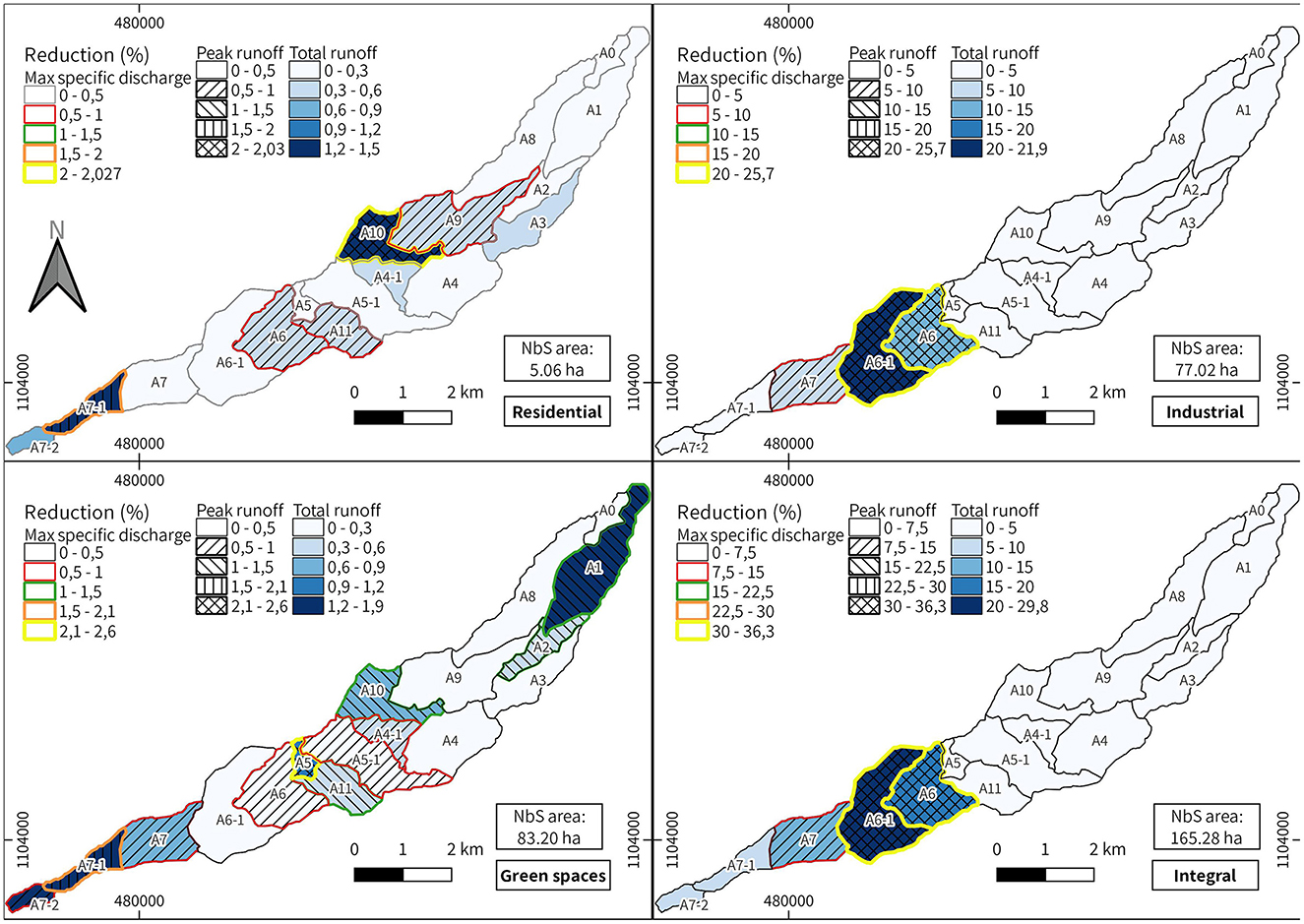
Figure 8. Percentage reductions in peak flow, runoff volume and maximum specific flow, for each independent drainage area, for each scenario analyzed.
3.2.1.1 Baseline scenario
In the baseline scenario representing the existing (non-NbS) condition of the watershed, the five drainage areas with the highest runoff volume generation were A9, A4, A5-1, A6, and A8, all identified as critical areas (Bonilla Brenes et al., 2023). Collectively, these areas account for 60.3% of the watershed's total runoff volume and comprise 45.1% of the total watershed area. Notably, all these areas feature over 50% impervious cover, with the exception of A8 which, despite having 18.7% impervious cover, generated a significant runoff volume due to its size (229 hectares or 10% of the total watershed area).
Among these drainage areas, the primary contributor to runoff volume and peak flow was A9, covering approximately 235.6 hectares (10.3% of the total watershed area) and with half of its area impervious. A9 generated 92.8 million liters (ML), representing 15% of the total runoff volume, with a peak flow of 30.5 m3/s and a maximum specific flow of 12.9 m3/s/km2.
Drainage area A4, spanning 195.7 hectares (8.6% of the watershed) and with 58.8% impervious cover, generated 84.8 ML of runoff volume, equivalent to 13.9% of the total runoff volume for the entire basin. A4 exhibited a peak runoff flow of 29.2 m3/s and a maximum specific flow of 14.9 m3/s/km2.
The third-highest contributor to runoff volume was A5-1, covering 197.8 hectares (8.6% of the watershed) and with 53.2% impervious cover. A5-1 generated 81.2 ML, representing 13.3% of the total runoff volume, with a maximum specific flow rate of 14.4 m3/s/km2 and a peak flow rate of 28.4 m3/s.
Area A6, comprising 174.1 hectares (7.6% of the total basin area) and with 56% impervious cover, contributed 60.4 ML or 9.9% of the total runoff volume. A6 exhibited a peak flow of 27.5 m3/s and a maximum specific flow of 15.8 m3/s/km2.
Covering 100.8 hectares (4.4% of the total area) and with 55.6% impervious cover, area A11 registered the highest maximum specific flow at 18.9 m3/s/km2. The second-highest maximum specific flow (17.9 m3/s/km2) was observed in A5, an area accounting for just 1.2% of the total watershed area (28.3 hectares) and with 46% impervious cover.
3.2.1.2 Residential scenario
The residential scenario demonstrates the best performance in drainage area A10, situated in the upper-middle part of the watershed. Despite having a mere 1.0 hectares available for NbS implementation, equivalent to 0.8% of its drainage area, this intervention produced a reduction of 1.5% in total runoff volume at the drainage area outlet, along with decreases of 2.0% in peak flow and maximum specific flow. It should be stressed that the assessment of available space in the residential scenario exclusively considered public road space. Therefore, concerted efforts between the community and local administrations are required to promote NbS application on private properties with a view to increasing the area available for NbS and thus obtaining greater flood risk mitigation benefits.
The second-highest performance of the residential scenario is observed in area A7-1, located in the lower part of the watershed and identified as highly susceptible to flooding (Lopes Monteiro et al., 2023). With a calculated NbS implementation area of 0.5 hectares, equivalent to 0.7% of its total area, a notable reduction of 1.5% in total runoff volume at the drainage area outlet was achieved, accompanied by decreases of 1.6% in peak flow and maximum specific flow.
The residential scenario extends its positive effects to all areas designated as critical. However, the most favorable outcomes within these critical zones are observed in A9 and A6, exhibiting runoff volume decreases of 0.6% and 0.4% respectively. Similarly, noteworthy reductions in peak flow and maximum specific flow are observed, at 0.76% for A9 and 0.5% for A6.
3.2.1.3 Industrial scenario
The industrial scenario exhibits its most promising outcomes in the central segment of the watershed, home to the industrial estates along with extensive buildings and parking lots. Areas A6-1, A6, A7, and A5-1 stand out as particularly impactful, though A7 is not categorized as a critical area.
The most substantial decrease in runoff volume (−21.9%) is achieved in area A6-1, attributable to the transformation of 16.6% of the drainage area, whereby 12% of roofs would be greened and 4.6% of parking lots converted to permeable pavement. Moreover, this would reduce peak flow and maximum specific flow by 25.7%.
Following closely, area A6, positioned just upstream of A6-1, features the second-best outcome. Approximately 13.9% of its drainage area would be converted to green roofs (6.9%) and permeable pavement (7%), resulting in a reduction of 23.2% in peak flow and maximum specific flow, accompanied by a 13.9% decrease in runoff volume.
Area A7 exhibits the third most noteworthy reduction, with a 9.17% decrease in runoff volume, along with an 8.8% decrease in peak flow and maximum specific flow. 1.7% of its surface area would be converted to green roofs and 1.1% to permeable pavement.
The reductions achieved in area A5-1 are particularly significant, considering its substantial runoff volume contribution in the baseline scenario. Through converting 0.6% of its roofs to green roofs and 2.2% of its parking lots to permeable pavement, a decrease of 3.2% in runoff volume, coupled with a 4.1% reduction in both peak flow and maximum specific flow, could be achieved.
3.2.1.4 Green space scenario
This scenario enables a discrete examination of the effects deriving from the recovery of the river protection zone and the effectiveness of multifunctional storage zones.
Looking first at the recovery of the river protection zone, the most significant outcome is observed in area A7-2 where we see a 1.9% decrease in runoff volume, coupled with a 1.0% reduction in peak flow and maximum specific flow. A total of 0.1 hectares of its protection zone were successfully reclaimed, equivalent to 0.4% of its overall drainage area.
At 2.6%, the most substantial reductions in peak flow and maximum specific flow are noted in area A5. Together with a 1.2% decrease in runoff volume, this positive outcome is attributed to the recovery of 0.41 hectares (1.5% of its drainage area).
Area A11 features the largest recovered surface area, 0.81 hectares, equivalent to 0.8% of its total area. This effort led to a 1.3% reduction in peak flow and maximum specific flow, alongside a 0.6% decrease in runoff volume.
In the critical area A1, a 1.4% decrease is observed in both peak flow and maximum specific flow, stemming from the successful recovery of 0.4 hectares (0.2% of its total drainage area). Moreover, the recovery initiatives contributed to a 1.3% decrease in runoff volume.
The storage areas were modeled as intermediary nodes situated between the drainage area and the junction in the channel. This design involves the runoff volume within the drainage area being received by the storage node, and subsequently conveyed to the junction in the channel through a weir and a discharge orifice. Such multifunctional storage areas emerge as structures significantly influencing the hydraulic response of the watershed, facilitating both the infiltration and retention of runoff volume during precipitation events. The runoff flow from all drainage areas equipped with multifunctional storage areas is effectively held back by each structure, introducing a time lag in the volume entering the channel. This deliberate delay results in a decrease of up to 58.5% in maximum peak flow across the entire watershed, a significant impact given that four of the seven storage areas housing multifunctional storage zones are classified as critical areas, namely A4, A5-1, A6-1, and A9.
Area A7-2 features the multifunctional storage area with the largest surface area, covering 10.2 hectares, equivalent to 33.9% of its total drainage area. With a storage capacity of up to 299 million liters (ML), this area achieves a maximum filling percentage of merely 0.5% during ordinary precipitation events.
Area A6-1 has the second-largest storage area, 8.3 hectares (3.6% of its drainage area), with a total capacity of 244.1 ML. Throughout the precipitation event, this structure attains a maximum fill percentage of 16.2% or 39.6 ML.
The third-largest in extent, the multifunctional storage area of critical drainage area A9 spans 4.5 hectares (1.9%). It ranks third in maximum filling percentage (65.3%), effectively storing a volume of 86.4 ML during precipitation events.
Drainage area A5-1 has 3.0 hectares (1.5% of its total area) available for a multifunctional storage area with a maximum capacity of up to 86.8 ML. During the analyzed precipitation event, a maximum filling level of 86.4% was achieved, storing a volume of 75.1 ML.
The smallest multifunctional zone is situated in critical drainage area A4. Its 1.0 hectares (0.5% of the drainage area) offers a maximum storage capacity of 28.8 ML. During the precipitation event, this specific multifunctional storage area proved to be insufficient, exceeding its maximum filling capacity in view of the generated runoff volume of approximately 84.5 ML. To effectively manage the runoff volume generated by A4, an area at least three times the current size is needed, adhering to the proposed storage structure's geometry.
3.2.2 Watershed results
Figure 9 shows the combined behavior of the watershed regarding the effect of NbS at each junction and their influence on downstream drainage areas, seen as a system of interconnected drainage areas. The junctions receive the information from the upstream areas, evaluate it and pass it on to the downstream areas, showing the cumulative effect of NbS implementation in the upper-middle drainage areas on lower areas. The figure shows the potential sites for the various NbS, as well as the percentage reductions in total runoff volume and peak flow measured at each junction. The total runoff volume reduction is considered as the volume retained during the storm event, which is subsequently discharged into the river in a controlled manner.
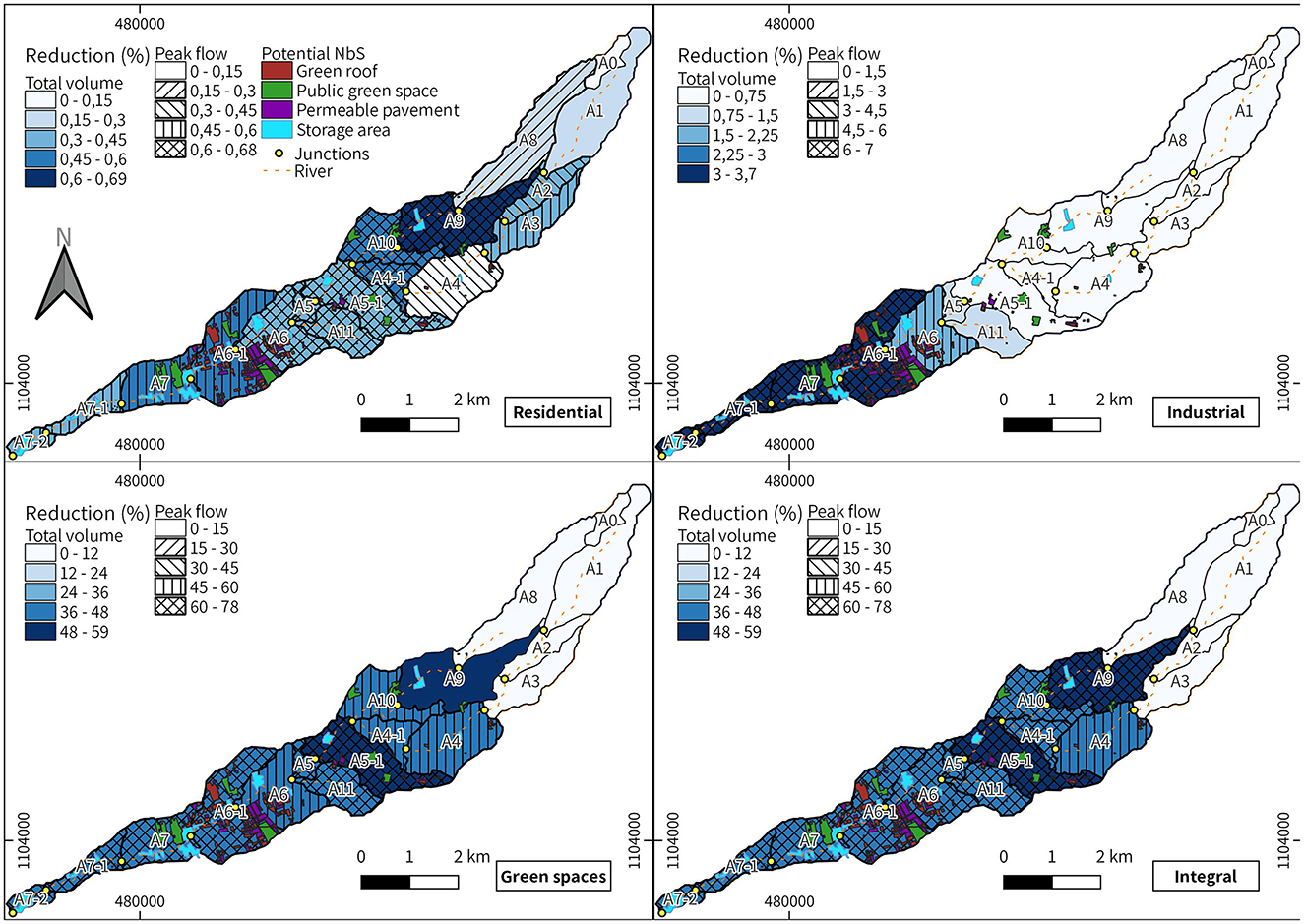
Figure 9. Location of the nature-based solutions in the industrial and green space scenarios and modeled cumulative percentage reductions in peak flow and total runoff volume at each junction, for each scenario analyzed, considering the effect from upstream drainage areas.
The hydraulic analysis involved a comprehensive evaluation of hydraulic parameters at the junctions linking the drainage areas to the river channel. The primary aim was to assess scenario performance at watershed level, considering the cumulative runoff volume generated in each drainage area and its impact on downstream sectors. Figure 10 illustrates the maximum flow and total volume recorded at each junction along the river channel, providing a comparative analysis between the base condition and the outcomes of each modeled scenario.
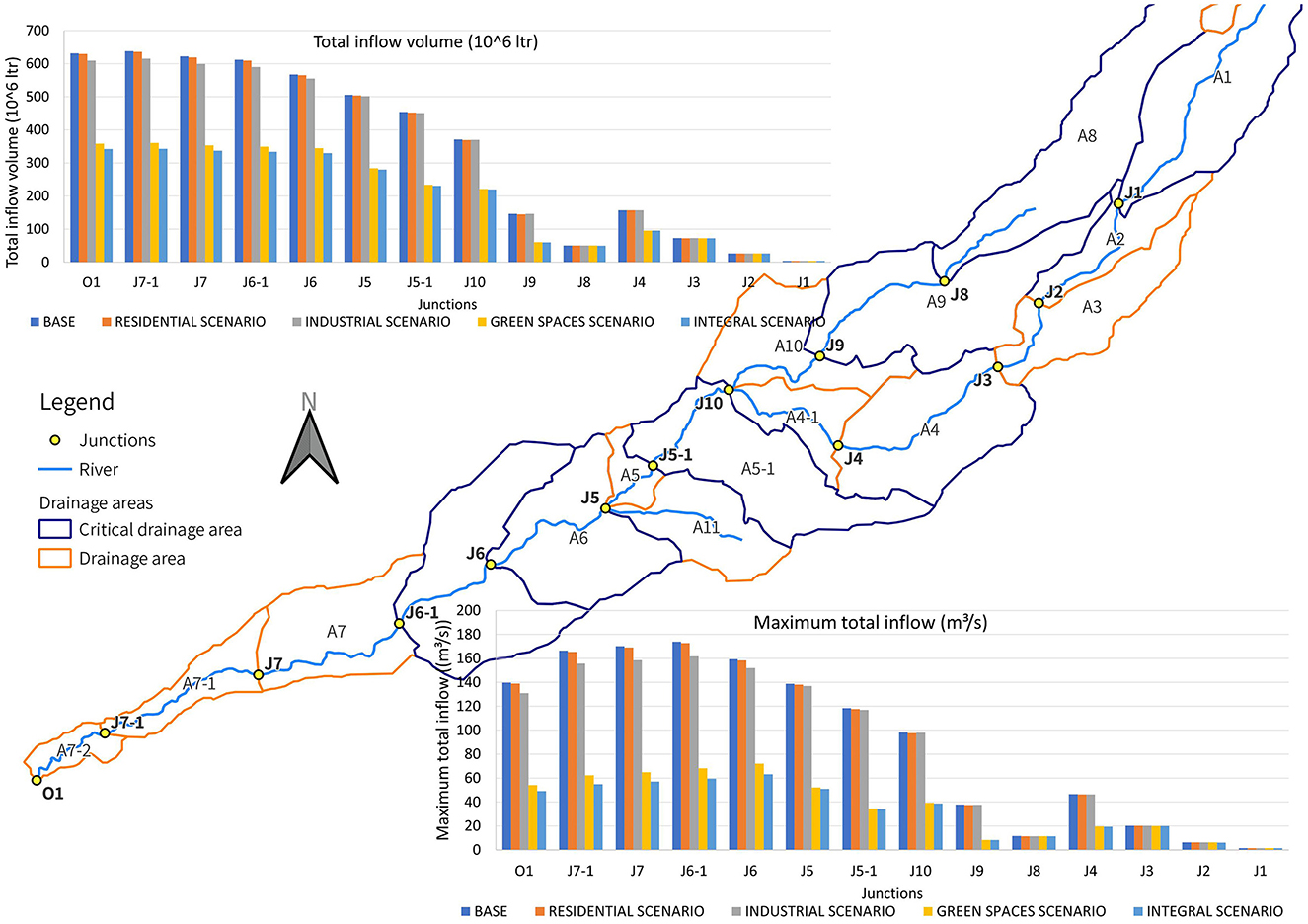
Figure 10. Maximum flow and total volume registered at each junction for the baseline scenario and the modeled scenarios.
In the baseline scenario, the cumulative runoff volume for the entire watershed amounted to 638 million liters (ML), resulting in a maximum peak flow of 173.8 m3/s at junction J6-1 (Figure 11). The residential scenario features a reduction of 2 ML (0.3%) in the total accumulated runoff volume and a 0.6% decrease in the maximum peak flow (172.8 m3/s). The industrial scenario exhibits a more substantial impact, reducing peak flow to 161.7 m3/s (-6.9%) and achieving a 3.6% reduction in the total accumulated runoff volume, which now stands at 615 ML. The green space scenario demonstrates the most favorable outcomes in hydraulic performance, achieving a 58.5% decrease in the maximum peak flow to 72.1 m3/s. Moreover, there is a substantial reduction in the total accumulated runoff volume to 360 ML, marking a significant 43.6% decrease.
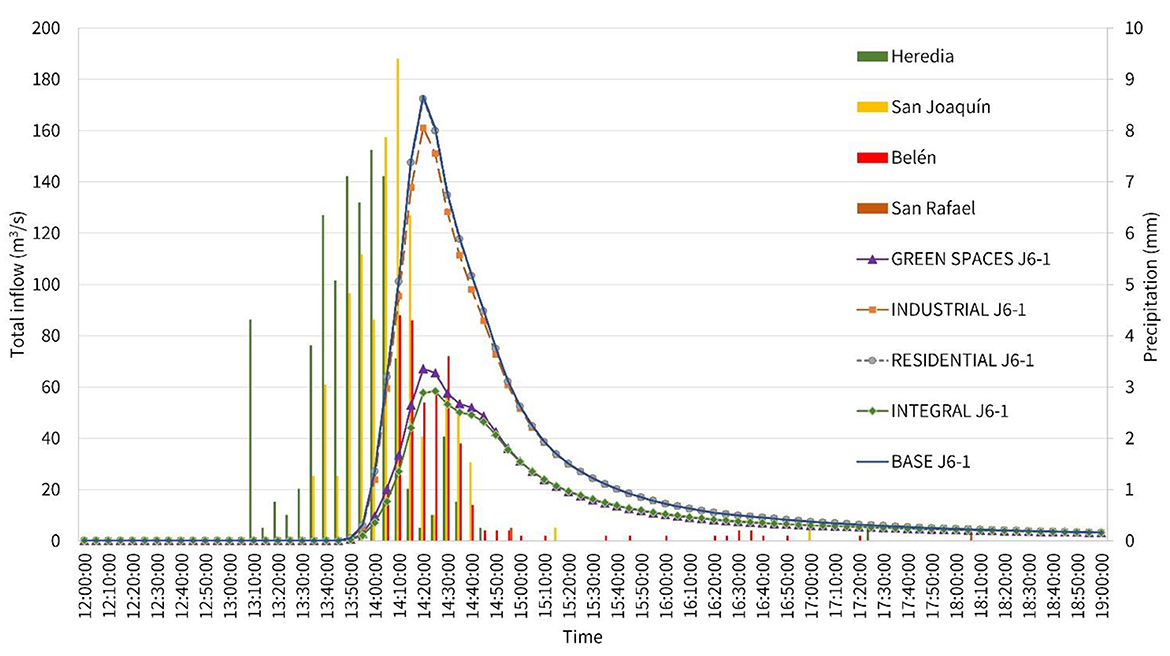
Figure 11. Precipitation distribution for each rain gauge and maximal total inflow for each scenario.
All scenarios show positive results in terms of mitigating the risk of flooding within the watershed. However, it is noticeable that scenarios incorporating larger structures with higher infiltration and retention capacities perform better. Multifunctional storage areas offer a decrease in runoff volumes and peak flows, translating into reductions in the floodable areas registered by the Municipality of Belén's early warning system. Through implementing just three of the twelve multifunctional storage areas identified, a decrease of up to 5.0% in the flooding area in the lower part of the basin could be achieved (Lopes Monteiro et al., 2023).
The combination of the residential, industrial, and green space scenarios culminates in a comprehensive strategy, producing a 63.7% reduction in maximum peak flow, now measured at 63.1 m3/s. Furthermore, this combined approach leads to a decrease in total runoff volume amounting to 343 million liters (ML), i.e., a 46.2% reduction.
4 Discussion
4.1 Impact and implications of NbS scenarios for an urban watershed recovery
Nature-based solutions (NbS) have the advantage of being multifunctional structures that, as seen in the GAM case study, contribute not only to reducing flood risks but also provide ecological and social benefits improving a watershed's health. It was assumed that an increase in vegetation cover would bring ecological benefits and that the new green spaces would have a social impact (Giuffré et al., 2019), promoting recovery of natural processes and recreational functions displaced by land sealing. Nature-based solutions, when properly planned and designed with the inclusion and participation of vulnerable or marginalized groups, can positively influence social dynamics by promoting environmental justice and equitable access to resources (Bremer et al., 2021). They may enhance access to green spaces and reduce environmental hazards, benefiting underserved communities that are often disproportionately affected by environmental risks (Maantay and Maroko, 2018). NbS can improve water and air quality, support local food security, and foster social cohesion through community involvement in co-design and implementation. By creating healthier environments, they reduce health disparities and contribute to public wellbeing (Clark and Miles, 2021). Moreover, NbS can be integrated into urban planning to ensure fair distribution of benefits across different neighborhoods, making them a suitable tool for inclusive and sustainable development (Silva et al., 2018). However, NbS may also negatively impact environmental justice and equitable access by excluding marginalized communities from NbS benefits, leading to displacement, and exacerbating social inequalities through processes like green gentrification (Maantay and Maroko, 2018). If marginalized groups are left out in the decision-making process, these solutions may prioritize ecosystem services over human needs, potentially creating conflicts over land and resources, and often fail to account for local knowledge (Portugal Del Pino and Marquez, 2023). Additionally, NbS projects can lead to the privatization of natural resources, unequal distribution of benefits, and unintended ecological consequences, disproportionately affecting vulnerable populations (Bremer et al., 2021). To avoid these problems, NbS should be designed with inclusive, context-specific, and equity-focused approaches, for instance, as it was developed in the transdisciplinary research within the SEE-URBAN-WATER Real-World Lab.
While ecological and social benefits could not be precisely quantified in this study as no baseline information was available for a proper evaluation and in-depth analysis, they were considered in previous studies which led to the NbS scenarios developed in this study. Access to green spaces is an indicator related to the mental and physical health of a city's population, improving wellbeing, personal satisfaction, social cohesion and a sense of community (Jennings and Bamkole, 2019).
4.1.1 Residential scenario
The hydraulic performance of the residential scenario is the lowest, achieving a mere 0.6% reduction in total peak flow in the watershed. This is due to the limited public space offered by the urban configurations of the neighborhoods for NbS implementation. The residential scenario prioritizes optimizing available areas along public roads within urban areas, guided by the infrastructure characteristics of the study area. This particular scenario exhibits the lowest percentage of land usable for NbS implementation due to the high density of built structures and the limited ratio of public green space per inhabitant in the municipalities of Heredia, Flores, and Belén (Arthur and Hack, 2022), especially in critical drainage areas.
One opportunity to improve the watershed condition is to unseal sealed surfaces using structures such as bioretention cells, infiltration trenches, street planters and permeable pavement, all of which support the absorption and retention of excess runoff volumes during intense precipitation events. However, the space available for NbS in residential areas is limited. It could be increased by using private areas within residential plots and by adopting NbS as building technologies to replace single-functional structures. This requires a collaborative effort between local administrations and residents, involving a conscientious awareness-raising campaign and the integration of NbS into construction regulations for new housing or remodeling projects.
To incentivize such change, the adoption of European practices could be explored. These provide economic incentives for projects promoting NbS (Fetting, 2020). Collaborations with international institutions dedicated to environmental conservation and sustainable development could facilitate the introduction of such incentives. The tangible benefits of the residential scenario would have a direct positive effect on local inhabitants, enhancing road safety, increasing green space, and generating positive hydrological and hydraulic effects (Fluhrer et al., 2021). The primary stakeholders responsible for supporting this scenario are local administration and the residents themselves.
An integral element of the residential scenario entails transforming urban centers into pedestrian zones, drawing inspiration from numerous European cities (Yassin, 2019). These urban centers commonly house key institutions such as churches, schools, municipalities, parks, and stores, attracting significant foot traffic in a current environment of high vehicular flows posing risks to pedestrian safety. Such interventions aim to contribute to watershed recovery and enhance the overall quality of life for residents. The realization of this urban transformation necessitates alterations to traffic routes, an improvement in public transport and public safety, as well as a concerted effort to raise awareness among individuals accustomed to a vehicle-centric urban model where design considerations have historically prioritized vehicular needs over those of residents (e.g., recreational space, walkability, good air quality, noise reduction).
4.1.2 Industrial scenario
The industrial scenario takes advantage of the large flat-roofed buildings and parking lots of industrial areas situated in the central part of the watershed, particularly focusing on critical drainage areas A5-1, A6, and A6-1 (88.8% of the available space for NbS). Strategic NbS interventions in industrial areas are imperative to mitigate the vulnerability caused by changes to hydrologic cycle processes and the risk of downstream flooding, given that these areas predominantly feature impervious surfaces generating substantial runoff volumes which are rapidly transported to water bodies during precipitation events.
The hydraulic effect of the industrial scenario is essential to counteract the impact of the sealing commonly found in the industrial zones and its influence on the lower part of the catchment. The effect is mainly visible in the lower middle part of the watershed, as most of the NbS interventions are in drainage areas A5-1, A6, A6-1, and A7. They are aimed at increasing retention capacity and achieving a 6.9% decrease in the total peak flow at the outlet of the watershed.
Within these industrial zones, the regulatory plans of each municipality mandate the installation of storage structures capable of managing the runoff volumes determined by the size of the developed terrain. However, these structures occasionally prove insufficient in controlling the total runoff due to the convective nature of precipitation events in the region. Installing green roofs and permeable pavement would enable the retention and infiltration of a portion of the runoff volume, thereby enhancing the effectiveness of existing storage structures (Ferreira and da Rocha, 2023; Twohig et al., 2022).
NbS implementation in industrial areas can benefit the wellbeing of workers by providing green spaces for recreation, exercise and relaxation, enhancing working conditions and boosting productivity (Kiss et al., 2019). Given the private nature of these infrastructures, the positive outcomes of the industrial scenario will be mostly experienced by employees within the industrial zones, though neighboring residents will benefit indirectly from the hydraulic, ecological, and social revitalization of the watershed. Implementing this scenario requires local administrations to collaborate with private companies to promote the use of NbS, possibly through incentives and through tracing a route toward the recovery of the watershed (Thomas, 2023).
4.1.3 Green space scenario
Two aims of rehabilitating the river protection zone and establishing multifunctional storage areas are to increase public green area per inhabitant and to create new public recreational spaces, thereby counteracting most of the vulnerabilities defined in Figure 2. Green spaces in urban watersheds play a crucial role in enhancing the urban environment and residents' quality of life. These areas serve to absorb, filter and store rainwater, thereby reducing the risk of flooding and improving water quality. Moreover, when interconnected, they form ecological corridors promoting biodiversity (Monberg et al., 2018). The establishment of natural habitats such as wetlands and wooded areas enhances biodiversity and improves ecosystem health. Green spaces also serve as centers for environmental education and help mitigate the urban heat island effect by providing shade and cooling the air, thereby benefiting residents' physical and mental wellbeing, encouraging outdoor activities, reducing stress, and promoting social cohesion.
Such retention areas can improve control over runoff from residential areas lacking dedicated storage structures, thereby extending the time-to-peak and enhancing infiltration. The envisioned outcome is a reduction in maximum runoff flows within each drainage area.
Featuring the largest recovered green area (83.2 ha), the green space scenario offers the greatest decrease in runoff volumes (43.6%) and peak flow (58.5%) due to its ability to retain substantial runoff volumes and attenuate the hydrograph in the river. The location of the multifunctional retention areas is of great significance because they affect four critical drainage areas generating the greatest runoff volumes (A4, A5-1, A6-1, A9). This scenario requires a larger surface area for its implementation, with extensive (private) land to be set aside for public use by municipal administrations, as well as a ban on urban developments on the potential areas (Lopes Monteiro et al., 2023). However, through protecting these green spaces, a significant increase of 7.2 m2/inhabitant of green space would be achieved, providing opportunities for recreational activities and improving inhabitants' physical and mental health.
This scenario promises to benefit a larger population, as it involves the development of public infrastructure directly affecting the entire community. With responsibility for executing this scenario lying with local administrations, incentives and an implementation process fostering community engagement and ensuring the safety of those using these structures are required (Dushkova and Haase, 2020). The success of this initiative hinges on fostering a sense of ownership among local residents and guaranteeing the effective functioning of the constructed facilities (Seddon et al., 2021).
4.2 Transferability and limitations
Although this study is based on different scenarios, these should be seen as a complementing each other to form a comprehensive strategy seeking the best possible solution for each drainage area, with a view to counteracting the vulnerabilities affecting each aspect of watershed health. Looking at the landscape aspect, the lack of green spaces in urban areas is compensated by NbS implementation along public roads in residential areas, while green roofs and permeable pavement can be installed in industrial areas. However, these two scenarios (residential and industrial) need to be complemented by a further scenario to achieve the desired broader ecological and social impact. This is accomplished by the green space scenario targeting recovery of the river protection zone. Recovery makes green spaces accessible for use both as recreational areas and as biological corridors increasing connectivity and thus counteracting the vulnerabilities of the habitat aspect.
Any comparison of the hydraulic performance of the individual scenarios is biased due to the size of the proposed structures in each scenario, whereby sizes are dictated by space availability. Though their complexity and effectiveness differ, all scenarios improve the hydrology and geomorphology aspects of watershed health, and are complementary in terms of being a long-term solution to the flooding problem and offering a route toward the recovery of urban watersheds.
Nature-based solutions have a multifunctional character that should be exploited within the configurations of urban developments. Their use can be recommended as comprehensive solutions to such problems as urban flooding, while also offering ecological and social benefits. A change in construction technologies is of great importance to promote the use of NbS, accompanied by a co-design process and technical guidance, such as the creation of the new Costa Rican Hydrological Code. The co-design process (Chapa et al., 2023; Neumann and Hack, 2022; Pérez Rubi and Hack, 2021) offers a holistic approach to problem-solving, fostering awareness, acceptance and ownership of proposed solutions, as well as ensuring application security and desired functionality. This methodology involves active collaboration between stakeholders, including local communities, environmental experts, and government authorities, from the initial stages of design onwards. This approach not only adapts solutions to specific needs and contexts, but also fosters a shared understanding of the problem and collective responsibility for its resolution. Such a collaborative and participatory process ensures effective NbS implementation and future maintenance, strengthening environmental and social resilience.
NbS implementation in industrial zones requires adapting developments to the characteristics of the environment and ensuring the involvement of all stakeholders, including companies, local administrations and communities. It is imperative to design solutions that do not compromise workers' safety or aggravate industrial risks. In addition to environmental and social benefits, NbS implementation can cut costs and improve corporate images. Continuous NbS performance monitoring is essential to ensure their long-term effectiveness.
We emphasize that these results are general, relating to the entire watershed and to a given precipitation event. They serve as preliminary, pre-feasibility analyses and should not be interpreted as conclusive results representing final design conditions. A more detailed exploration is imperative, incorporating geometric design intricacies, topographic conditions, and a precise determination of the ROI period for which the structures are to be designed. More investigation is needed into the watershed health aspects of habitat, water quality and biological conditions in order to develop an exhaustive analysis with more representative results of the study area.
Uncertainty and limitations of a hydrologic and hydraulic model arises from several factors that affect the accuracy of its predictions. These include the quality of input data, such as precipitation and soil characteristics, and the difficulty of accurately estimating parameters representing processes such as infiltration and runoff (Herrera et al., 2022). In addition, the structure of the model, which simplifies natural processes, introduces uncertainty if it does not adequately reflect the complexity of the real system. Also, future climate predictions, such as changes in precipitation, add uncertainty in the long term (Feng and Beighley, 2020). Model calibration and validation with robust and quality data is crucial to improve the reliability of hydrological models as well as their adaptation and update to new study cases with different base information (Bessar et al., 2020).
In Europe, there are examples of NbS implementation of similar extent as presented in this study that show decreases in extreme temperatures, increases in biodiversity, carbon sequestration and decreases in flood risk (Epelde et al., 2022). However, in Latin America the topic of NbS is rather recent and most of its research is focused on models to determine its effectiveness. Several projects (Ecoparque, Tijuana; Plan Yaque, Dominican Republic; Novas Árvores por aí, São Paulo; STGO+ Infrasteructura Verde; Santiago) show progress in increasing social/community resilience and ecological/social connectivity through green infrastructure, however, there is a deficiency in monitoring and evaluation of the infrastructure (Hack et al., 2024). Deficiencies in NbS monitoring and evaluation may be related to the time scales in which the projects are developed and to the fact that most examples show a lack of funding provision, which has been previously reported as a barrier for implementation (Pauleit et al., 2021).
The Quebrada Seca watershed shares its problems with other urban watersheds such as the Tiribí, María Aguilar, Bermúdez and Torres rivers which later form the Virilla river and finally the Grande de Tárcoles river. This geographical similarity is complemented by land cover and space availability similarities, as they are all part of the GAM and have suffered from haphazard urban growth (IANAS and UNESCO, 2015). They also share their problems with other watersheds in Latin America that have been developed without urban planning and now present flooding problems, as well as environmental and social affectations (Aragón-Durand, 2014; Silveira, 2002; Walteros and Ramírez, 2020). These similarities make it possible to replicate the methodology applied in this study, however, each case study must consider unique aspects and specific conditions to ensure the representativeness of the results for each new urban watershed analyzed.
5 Conclusions
Dependent on the availability of space for each land cover type, NbS multi-functionality can be harnessed to counteract vulnerabilities affecting the health of an urban watershed. Available spaces, such as public roads located within residential areas, offer potential for permeable pavement, infiltration trenches, bioretention cells and street planters, while flat roofs and parking lots in industrial areas can be converted to green roofs and permeable pavement. Similarly, public green spaces offer potential for multifunctional storage areas. The residential scenario recovers public pedestrian spaces and improves infiltration, while the industrial scenario reduces the runoff generated within the industrial zones and the green space scenario offers multifunctional runoff storage areas for public recreational and ecological connectivity. By considering ecological and social benefits in the development of NbS scenarios, hydraulic benefits dependent on the location, size, and design of different NbS elements in each scenario emerge. While each scenario provides ecological, social, and hydraulic benefits to different degrees, they complement each other in improving overall watershed health.
A comprehensive solution addressing the interaction of social, ecological, and hydraulic factors must be accompanied by a co-design process involving all scenario stakeholders from the initial stage of the project onwards. The objective is to spotlight each of the vulnerabilities to be improved through NbS implementation from a multi-sectoral point of view, generating a shared vision and collective responsibility in the pursuit of effective implementation and adequate maintenance.
This methodology is based on the use of available space in different land use scenarios present in an urban watershed. As the considered land uses and their unplanned development are a common factor at the origin of the problems shared by many urban watersheds in Latin America, the methodology used for analyzing the problems and initiating recovery of urban watersheds is transferable.
To achieve significant social and ecological benefits when implementing NbS for flood control, the development of national guidelines that promote local leadership and the integration of NbS into regulations and planning, fostering centralized cooperation and multisectoral coordination at the municipal and regional levels should be a priority. We suggested to articulate existing institutions and legal frameworks, strengthening capacities through sustainable training, and developing specific NbS planning instruments. Additionally, and based on our insights, we recommend to implement pilot projects for transdisciplinary learning, with the support of academia and civil organizations, and to accompany these initiatives with communication strategies. Furthermore, government actors should plan the monitoring and evaluation of NbS, actively involving key stakeholders to ensure long-term sustainability.
Data availability statement
The raw data supporting the conclusions of this article will be made available by the authors, without undue reservation.
Author contributions
RB: Conceptualization, Data curation, Formal analysis, Funding acquisition, Investigation, Methodology, Resources, Software, Supervision, Validation, Visualization, Writing – original draft, Writing – review & editing. JH: Conceptualization, Funding acquisition, Investigation, Methodology, Project administration, Resources, Supervision, Writing – review & editing. MM: Writing – review & editing, Conceptualization, Investigation, Methodology, Supervision. RO: Writing – review & editing, Conceptualization, Investigation, Methodology, Supervision.
Funding
The author(s) declare financial support was received for the research, authorship, and/or publication of this article. We acknowledge support by the Deutsche Forschungsgemeinschaft (DFG – German Research Foundation) and the Open Access Publishing Fund of Hochschule Darmstadt – University of Applied Sciences. DAAD (German Academic Exchange Service) and CONARE-UCR (National Council of Rectors—University of Costa Rica) are funding the PhD program for the student Ricardo Bonilla Brenes through an ALECOSTA scholarship. This research was funded by the German Federal Ministry of Education and Research (BMBF), grant number 01UU1704.
Conflict of interest
The authors declare that the research was conducted in the absence of any commercial or financial relationships that could be construed as a potential conflict of interest.
Publisher's note
All claims expressed in this article are solely those of the authors and do not necessarily represent those of their affiliated organizations, or those of the publisher, the editors and the reviewers. Any product that may be evaluated in this article, or claim that may be made by its manufacturer, is not guaranteed or endorsed by the publisher.
Supplementary material
The Supplementary Material for this article can be found online at: https://www.frontiersin.org/articles/10.3389/frsus.2024.1425732/full#supplementary-material
References
Aparicio Uribe, C. H., Bonilla Brenes, R., and Hack, J. (2022). Potential of retrofitted urban green infrastructure to reduce runoff—A model implementation with site-specific constraints at neighborhood scale. Urban Forest. Urban Green. 69, 1618–8667. doi: 10.1016/j.ufug.2022.127499
Aragón-Durand, F. (2014). Inundaciones en zonas urbanas de cuencas en América Latina. Lima: Soluciones Prácticas. Available at: https://floodresilience.net/resources/item/inundaciones-en-zonas-urbanas-de-cuencas-en-america-latina/
Arthur, N., and Hack, J. (2022). A multiple scale, function, and type approach to determine and improve Green Infrastructure of urban watersheds. Urban Forest. Urban Green. 68:127459. doi: 10.1016/j.ufug.2022.127459
Babí Almenar, J., Elliot, T., Rugani, B., Philippe, B., Navarrete Gutierrez, T., Sonnemann, G., et al. (2021). Nexus between nature-based solutions, ecosystem services and urban challenges. Land Use Policy 100:104898. doi: 10.1016/j.landusepol.2020.104898
Barros, J. X. (2004). Urban Growth in Latin American Cities: Exploring Urban Dynamics Through Agent-Based Simulation (Doctoral Thesis). Bartlett School of Architecture and Planning, University of London, London, England.
Beißler, M. R., and Hack, J. (2019). A combined field and remote-sensing based methodology to assess the ecosystem service potential of urban rivers in developing countries. Remote Sens. 11:1697. doi: 10.3390/rs11141697
Beninde, J., Veith, M., and Hochkirch, A. (2015). Biodiversity in cities needs space: a meta-analysis of factors determining intra-urban biodiversity variation. Ecol. Lett. 18, 581–592. doi: 10.1111/ele.12427
Bernhardt, E. S., and Palmer, M. A. (2007). Restoring streams in an urbanizing world. Freshw. Biol. 52, 738–751. doi: 10.1111/j.1365-2427.2006.01718.x
Bessar, M. A., Matte, P., and Anctil, F. (2020). Uncertainty analysis of a 1d river hydraulic model with adaptive calibration. Water 12:561. doi: 10.3390/w12020561
Bonilla Brenes, R., Morales, M., Oreamuno, R., and Hack, J. (2023). Variation in the hydrological response within the Quebrada Seca watershed in Costa Rica resulting from an increase of urban land cover. Urban Water J. 20, 575–591. doi: 10.1080/1573062X.2023.2204877
Bremer, L. L., Keeler, B., Pascua, P., Walker, R., and Sterling, E. (2021). “Nature-based solutions, sustainable development, and equity,” in Nature-Based Solutions and Water Security: An Action Agenda for the 21st Century, 81–105. doi: 10.1016/B978-0-12-819871-1.00016-6
Chapa, F., Perez Rubi, M., and Hack, J. (2023). A systematic assessment for the co-design of green infrastructure prototypes—A case study in urban Costa Rica. Sustainability 15:2478. doi: 10.3390/su15032478
Chapa, F., Pérez, M., and Hack, J. (2020). Experimenting transition to sustainable urban drainage systems—identifying constraints and unintended processes in a tropical highly urbanized watershed. Water 12:3554. doi: 10.3390/w12123554
Chen, V., Bonilla, R., Chapa, F., and Hack, J. (2021). Development and modelling of realistic retrofitted Nature-based Solution scenarios to reduce flood occurrence at the catchment scale. Ambio 50, 1462–1476. doi: 10.1007/s13280-020-01493-8
Chow, V., Maidment, D., and Mays, L. (1994). Hidrología Aplicada. Bogotá: McGraw-Hill Interamericana S.A.
Clark, S. S., and Miles, M. L. (2021). Assessing the integration of environmental justice and sustainability in practice: a review of the literature. Sustainability 13:11238. doi: 10.3390/su132011238
de Alencar da Silva, J. C., and do Amaral Porto, M. F. (2021). Urban Brazilian watersheds: when to opt for restoration, revitalisation or recovery. Water Managem. 174, 70–83. doi: 10.1680/jwama.18.00080
Dos-Santos, M. F. N., Barbassa, A. P., Vasconcelos, A. F., and Ometto, A. R. (2021). Stormwater management for highly urbanized areas in the tropics: life cycle assessment of low impact development practices. J. Hydrol. 598:126409. doi: 10.1016/j.jhydrol.2021.126409
Dushkova, D., and Haase, D. (2020). Not simply green: nature-based solutions as a concept and practical approach for sustainability studies and planning agendas in cities. Land 9:19. doi: 10.3390/land9010019
Epelde, L., Mendizabal, M., Gutiérrez, L., Artetxe, A., Garbisu, C., and Feliu, E. (2022). Quantification of the environmental effectiveness of nature-based solutions for increasing the resilience of cities under climate change. Urban For. Urban Green. 67:127433. doi: 10.1016/J.UFUG.2021.127433
Feng, D., and Beighley, E. (2020). Identifying uncertainties in hydrologic fluxes and seasonality from hydrologic model components for climate change impact assessments. Hydrol. Earth Syst. Sci. 24, 2253–2267. doi: 10.5194/hess-24-2253-2020
Ferreira, M. J., and da Rocha, H. R. (2023). Green roof infrastructure outperforms grey technology in flood mitigation in São Paulo's urbanized region. Front. Built Environm. 9:1254942. doi: 10.3389/fbuil.2023.1254942
Fluhrer, T., Chapa, F., and Hack, J. (2021). A methodology for assessing the implementation potential for retrofitted and multifunctional urban green infrastructure in public areas of the global south. Sustainability 13:384. doi: 10.3390/su13010384
Giannico, V., Spano, G., Elia, M., D'Este, M., Sanesi, G., and Lafortezza, R. (2021). Green spaces, quality of life, and citizen perception in European cities. Environm. Res. 196:110922. doi: 10.1016/j.envres.2021.110922
Giuffré, L., Bonafina, C., Vespasiano, C., and Ciarlo, E. (2019). Social impact of urban green spaces. IOSR J. Human. Soc. Sci. 24, 29–35. doi: 10.9790/0837-2408062935
Grahn, P., and Stigsdotter, U. A. (2003). Landscape planning and stress. Urban Forest. Urban Green. 2, 1–18. doi: 10.1078/1618-8667-00019
Grunewald, K., Richter, B., and Herold, H. (2016). “Vorschlag bundesweiter Indikatoren zur Erreichbarkeit öffentlicher Grünflächen,” in Bewertung der Ökosystemleistung “Erholung in der Stadt.” In Article in Naturschutz und Landschaftsplanung. Available at: https://www.zensus2011.de/SharedDocs/Aktu-
Hack, J., Molewijk, D., and Beißler, M. R. (2020). A conceptual approach to modeling the geospatial impact of typical urban threats on the habitat quality of river corridors. Remote Sens. 12:1345. doi: 10.3390/rs12081345
Hack, J., Ojeda-Revah, L., Rubí, M. P., Pradilla, G., Borbor-Cordova, M., Burgueño, G., et al. (2024). Avances de infraestructura verde urbana para la gestión de agua en América Latina. Cuadernos de Geografía 33:1. doi: 10.15446/rcdg.v33n1.101947
Hack, J., Peréz Rubí, M., Beissler, M., Ortega Sandoval, A., and Bonilla Brenes, R. (2023). “Implementing nature-based solutions and green infrastructure for cities,” in Citizens and Rivers - The SEE-URBAN-WATER Project. Hannover: Institutionelles Repositorium der Leibniz Universität Hannover. doi: 10.15488/15438
Hack, J., and Schröter, B. (2021). “Nature-based solutions for river restoration in metropolitan areas,” in The Palgrave Encyclopedia of Urban and Regional Futures, ed. R. Bears (Cham: Palgrave Macmillan), 1–10.
Handley, J. (2003). English Nature Research Reports Number 526 Accessible Natural Green S pace Standards in Towns and Cities: A Review and Toolkit for their Implementation. Peterborough: English Nature Research Reports Number 526.
Herrera, P. A., Marazuela, M. A., and Hofmann, T. (2022). Parameter estimation and uncertainty analysis in hydrological modeling. Wiley Interdiscipl. Rev.: Water 9:e1569. doi: 10.1002/wat2.1569
Hughes, R. M., Dunham, S., Maas-Hebner, K. G., Yeakley, J. A., Schreck, C., Harte, M., et al. (2014). A review of urban water body challenges and approaches: (1) rehabilitation and remediation. Fisheries 39, 18–29. doi: 10.1080/03632415.2013.836500
IANAS and UNESCO. (2015). Urban Water Challenges in the Americas: A Perspective From the Academies of Sciences. Santo Domingo: IANAS.
Jayasooriya, V. M., Ng, A. W. M., Muthukumaran, S., and Perera, C. B. J. (2020). Optimization of green infrastructure practices in industrial areas for runoff management: a review on issues, challenges and opportunities. Water 12:1024. doi: 10.3390/w12041024
Jennings, V., and Bamkole, O. (2019). The relationship between social cohesion and urban green space: an avenue for health promotion. Int. J. Environm. Res. Public Health 16:452. doi: 10.3390/ijerph16030452
Jha, A. K., Bloch, R., and Lamond, J. (2012). Cities and Flooding: A Guide to Integrated Urban Flood Risk Management for the 21st Century. Cities and Flooding. Washington, DC: The World Bank. doi: 10.1596/978-0-8213-8866-2
Kabisch, N., van den Bosch, M., and Lafortezza, R. (2017). The health benefits of nature-based solutions to urbanization challenges for children and the elderly – A systematic review. Environm. Res. 159, 362–373. doi: 10.1016/j.envres.2017.08.004
Kiss, B., Sekulova, F., and Kotsila, P. (2019). International Comparison of Nature-Based Solutions. Naturvation Project Report. Durham University, European Union's Horizon.
Lopes Monteiro, C., Bonilla Brenes, J. R., Serrano-Pacheco, A., and Hack, J. (2023). Multi-criteria site selection and hydraulic modeling of green flood retention measures in a highly urbanized basin in Costa Rica. Urban Forest. Urban Green. 85:127957. doi: 10.1016/j.ufug.2023.127957
Maantay, J. A., and Maroko, A. R. (2018). Brownfields to greenfields: environmental justice versus environmental gentrification. Int. J. Environm. Res. Public Health 15:2233. doi: 10.3390/ijerph15102233
Masís-Campos, R., Avendaño-Flores, I., and Reyes-Chaves, J. (2020). Cartografía Del Perfil Socioeconómico Y De Vivienda En La Microcuenca Río Burío Quebrada Seca (Costa Rica). InterSedes 21, 175–209. doi: 10.15517/isucr.v21i43.41991
Masís-Campos, R., and Vargas-Picado, H. (2014). Incremento de áreas impermeables por cambios de usos de la tierra en la microcuenca del río Burío. Revista Reflexiones 93, 33–46. doi: 10.15517/rr.v93i1.13742
Monberg, R. J., Howe, A. G., Ravn, H. P., and Jensen, M. B. (2018). Exploring structural habitat heterogeneity in sustainable urban drainage systems (SUDS) for urban biodiversity support. Urban Ecosyst. 21, 1159–1170. doi: 10.1007/s11252-018-0790-6
Neumann, V., and Hack, J. (2022). Revealing and assessing the costs and benefits of nature-based solutions within a real-world laboratory in Costa Rica. Environm. Impact Assessm. Rev. 93:106737. doi: 10.1016/j.eiar.2022.106737
Neumann, V. A., and Hack, J. (2020). A methodology of policy assessment at the municipal level: Costa Rica's readiness for the implementation of nature-based-solutions for urban stormwater management. Sustainability 12:230. doi: 10.3390/su12010230
Nguyen, P. Y., Astell-Burt, T., Rahimi-Ardabili, H., and Feng, X. (2021). Green space quality and health: a systematic review. Int. J. Environm. Res. Public Health 18:11028. doi: 10.3390/ijerph182111028
Ohwo, O., Abotutu, A., and Farooqi, A. (2015). Environmental impact of urbanization in Nigeria. Curr. J. Appl. Sci. Technol. 9, 212–221. doi: 10.9734/BJAST/2015/18148
Oreamuno, R., and Villalobos, R. (2015). Estudios hidrológicos e hidráulicos en la cuenca Quebrada Seca Río-Burío. Research Report. San José, CA: Centro de Investigación y Estudios para el Desarrollo Sostenible (CIEDES); Universidad de Costa Rica.
Osuide, E. E. (2021). Implementation of measures to mitigate the risk of pluvial flooding in urban areas. Model. Earth Syst. Environm. 2021, 1–14. doi: 10.1007/s40808-021-01308-z
Pauleit, S., Vasquéz, A., Maruthaveeran, S., Liu, L., and Cilliers, S. S. (2021). Urban green infrastructure in the global south. Cities and Nature. 337, 107–143. doi: 10.1007/978-3-030-67650-6_5
Pérez Rubi, M., and Hack, J. (2021). Co-design of experimental nature-based solutions for decentralized dry-weather runoff treatment retrofitted in a densely urbanized area in Central America. Ambio 50, 1498–1513. doi: 10.1007/s13280-020-01457-y
Perez Rubi, M., Schiffmann, C., and Hack, J. (2024). Multidimensional assessment of a Nature-based Solution for decentralized greywater treatment in Costa Rica. Nature-Based Solut. 6:100156. doi: 10.1016/j.nbsj.2024.100156
Portugal Del Pino, D., and Marquez, J. F. (2023). Complementary ideas for the implementation of nature-based solutions. Environ. Sci. Policy 141, 146–157. doi: 10.1016/J.ENVSCI.2023.01.008
Raymond, C. M., Frantzeskaki, N., Kabisch, N., Berry, P., Breil, M., Razvan Nita, M., et al. (2017). A framework for assessing and implementing the co-benefits of nature-based solutions in urban areas. Environ. Sci. Policy 77, 15–24. doi: 10.1016/j.envsci.2017.07.008
Rossman, L. A., and Huber, W. C. (2015). Storm Water Management Model - User's Manual Version 5.1. Available at: https://www.epa.gov/sites/default/files/2019-02/documents/epaswmm5_1_manual_master_8-2-15.pdf (accessed September 1, 2022).
Rossman, L. A., and Huber, W. C. (2016). Storm Water Management Model Reference Manual Volume III-Water Quality Office of Research and Development Water Supply and Water Resources Division. Available at: https://nepis.epa.gov/Exe/ZyPDF.cgi/P100P2NY.PDF?Dockey=P100P2NY.PDF%0Ahttps://cfpub.epa.gov/si/si_public_record_report.cfm?Lab=NRMRL&dirEntryId=327450 (accessed October 19, 2022).
Rupprecht, C. D. D., and Byrne, J. A. (2014). Informal urban greenspace: A typology and trilingual systematic review of its role for urban residents and trends in the literature. Urban Forest. Urban Green. 13, 597–611. doi: 10.1016/j.ufug.2014.09.002
Schlee, M. B., Tamminga, K. R., and Tangari, V. R. (2012). A method for gauging landscape change as a prelude to urban watershed regeneration: the case of the Carioca River, Rio de Janeiro. Sustainability 4, 2054–2098. doi: 10.3390/su4092054
Seddon, N., Smith, A., Smith, P., Key, I., Chausson, A., Girardin, C., et al. (2021). Getting the message right on nature-based solutions to climate change. Global Change Biol. 27, 1518–1546. doi: 10.1111/gcb.15513
Silva, C. de S., Viegas, I., Panagopoulos, T., and Bell, S. (2018). Environmental justice in accessibility to green infrastructure in Two European Cities. Land 7:134. doi: 10.3390/land7040134
Silveira, A. L. L. (2002). Problems of modern urban drainage in developing countries. Water Sci. Technol. 45, 31–40. doi: 10.2166/wst.2002.0114
Solano, J., and Villalobos, R. (2012). Regiones y sub-regiones climáticas de Costa Rica. Costa Rica: Instituto Meteorológico Nacional, 32. Available at: http://www.imn.ac.cr/publicaciones/estudios/Reg_climaCR.pdf
Subcomisión de Heredia–CGICRG Tárcoles. (2016). Protocolo De Recuperación De Áreas De Protección Y Árborización Para La Provincia De Heredia. Available at: http://www.academia.edu/8178581/Informe_Alineamiento_topografico (accessed Septenber 23, 2022).
Thomas, M. J. (2023). Investing in Nature-Based Solutions State-of-Play and Way Forward for Public and Private Financial Measures in Europe. Luxembourg: European Investment Bank.
Travassos, L., and Schult, S. I. M. (2013). Recuperação socioambiental de fundos de vale urbanos na cidade de São Paulo, entre transformações e permanências. Cadernos Metrópole 15, 289–312.
Twohig, C., Casali, Y., and Aydin, N. Y. (2022). Can green roofs help with stormwater floods? A geospatial planning approach. Urban Forest. Urban Green. 76:127724. doi: 10.1016/j.ufug.2022.127724
U.S. EPA (2012). Identifying and Protecting Healthy Watersheds Concepts, Assessments, and Management Approaches. Washington, DC: U.S. Environmental Protection Agency; Office of Water; Office of Wetlands, Oceans, and Watersheds. EPA 841-B-11-002.
Verworn, H. R. (2002). Advances in urban-drainage management and flood protection. Philosoph. Trans. Royal Soc. A. 360, 1451–1460. doi: 10.1098/rsta.2002.1009
Walteros, J. M., and Ramírez, A. (2020). Urban streams in Latin America: current conditions and research needs. Rev. Biol. Trop. 68, 13–28. doi: 10.15517/rbt.v68iS2.44330
Ward, J. V., Tockner, K., Uehlinger, U., and Malard, F. (2001). Understanding natural patterns and processes in river corridors as the basis for effective river restoration. Regul. Rivers: Res. Manag. 17, 311–323. doi: 10.1002/rrr.646
Wiegels, R., Chapa, F., and Hack, J. (2021). High resolution modeling of the impact of urbanization and green infrastructure on the water and energy balance. Urban Climate 39:100961. doi: 10.1016/j.uclim.2021.100961
World Health Organization Regional Office for Europe (2016). Urban Green Spaces and Health. A Review of Evidence. Copenhagen: World Health Organization (WHO). WHO/EURO:2016-3352-43111-60341
Yassin, H. H. (2019). Livable city: an approach to pedestrianization through tactical urbanism. Alexandria Eng. J. 58, 251–259. doi: 10.1016/j.aej.2019.02.005
Keywords: urbanization, land cover change, flooding, hydrological-modeling, nature-based solutions, multifunctionality, watershed recovery
Citation: Bonilla Brenes R, Hack J, Morales M and Oreamuno R (2024) Integral recovery of an urban watershed through the implementation of nature-based solutions. Front. Sustain. 5:1425732. doi: 10.3389/frsus.2024.1425732
Received: 30 April 2024; Accepted: 30 October 2024;
Published: 20 November 2024.
Edited by:
Cristina Sousa Coutinho Calheiros, University of Porto, PortugalReviewed by:
Patrizia Piro, University of Calabria, ItalyWilliam Shuster, Wayne State University, United States
Anupam Khajuria, United Nations University, Japan
Copyright © 2024 Bonilla Brenes, Hack, Morales and Oreamuno. This is an open-access article distributed under the terms of the Creative Commons Attribution License (CC BY). The use, distribution or reproduction in other forums is permitted, provided the original author(s) and the copyright owner(s) are credited and that the original publication in this journal is cited, in accordance with accepted academic practice. No use, distribution or reproduction is permitted which does not comply with these terms.
*Correspondence: Jochen Hack, am9jaGVuLmhhY2tAaC1kYS5kZQ==
†ORCID: Jochen Hack orcid.org/0000-0002-8060-7990