- College of Science and Engineering, Hamad Bin Khalifa University, Qatar Foundation, Doha, Qatar
The Energy-Water-Food (EWF) nexus is a complex and multidimensional system, in which the energy, water and food resources are strongly interconnected. EWF nexus systems are seriously threatened by natural hazards (e.g., climate change and extreme weather events) along with other human-driven threats, including rapid population growth, urbanization, terrorism, and geopolitical uncertainty. As such, integrated analysis, as encouraged by the EWF nexus can facilitate the identification of essential connections and potential conflicts that may arise in the planning and operation of resource systems. Moreover, in order to consider immediate shocks and long-term pressures, it is imperative to prioritize the strengthening of EWF system resilience by incorporating robust and efficient resource management strategies, which consider various dimensions of sustainability and security such as technical, environmental, economic, and societal aspects. Decentralization is one concept that has the potential of improving the resilience of nexus systems faced to the multiple risks governing them through reducing single points of failure and enabling swifter responses to sudden shocks and continuous volatilities. However, in order to achieve a holistic system resilience through decentralization, the assessment of the different risks impacting each nexus sector is fundamental, yet it can be particularly challenging. Therefore, the objective of this study is to review the currently available resilience assessment methods for the EWF nexus system. The systematic literature review will connect various assessment methods used within decentralization studies that are applied to improve the resilience performance of the EWF nexus system. A total of 84 journal papers were evaluated. The review demonstrates that the deployment of multi-criteria decision-making framework based on composite indicators can be effective in addressing risks and uncertainties within EWF systems. Furthermore, findings of this review illustrate complementary connections between decentralization and resilience concepts, which when integrated with the EWF nexus approach, can be effectively utilized for integrated sustainable resource management.
1 Introduction
During the 1960s, rapid urban and economic growth brought to light the environmental challenges of development. Climate change, pollution and other types of chemical contamination are the main catalysts triggering the need for sustainable development (Hoyos et al., 2010). There are various definitions of sustainable development. The most commonly cited is from the ‘Our Common Future report,’ which was issued in 1987 by the World Commission on Environment and Development, known as the Brundtland report. It stipulates that “Sustainable development is the development that meets the needs of the present without compromising the ability of future generations to meet their own needs” (Visser and Brundtland, 2013). In addition to the global environmental deterioration, rapid population growth has also resulted in the subsequent increase in energy, water, and food consumption amongst other resources, primarily to meet the demand for products and services, leading to increased resource competition and depletion. In contemporary society, there are apprehensions regarding the availability and accessibility of energy, water, and food resources, as well as the disparities in their distribution. These concerns arise from the consequences of inadequate management and unsuitable governance structures (Bonn2011Conference, 2011). Therefore, there has been a transition towards holistic approaches in establishing potential long-term solutions (Mohtar, 2017). Today, the energy, water, food (EWF) nexus concept is widely recognized as a comprehensive approach to addressing issues around environmental impacts and resource security. Several US and international initiatives address the EWF nexus directly or indirectly (SIWI, 2018; WWF8, 2018), while the 2030 Sustainable Development Goals also include EWF nexus components (FAO, 2018).
In the context of climate change, population increase, and global health concerns; providing sustainable, sufficient and clean energy, water and food is the ultimate aim for many nations (UN, 2019). In this regard, EWF nexus methods are widely acknowledged as crucial tools that can be utilized for achieving sustainable development goals (Biggs et al., 2015). Moreover, EWF nexus methods can identify system interlinkages, and thus provide additional advantages in comparison to other analytical or policy analysis approaches, which may have only focused on a single specific development goal (e.g., development of hydropower source to enhance energy access) (Kishore et al., 2021). Thus, failure in considering the trade-offs across multiple systems, or even the inadequate defining the interconnections between energy, water and food resources, along with their effects on human health systems, may lead to overestimation or underestimation of socioeconomic consequences from policy change, management change or infrastructural development perspective (Baker and Van Houtven, 2021). Consequently, the complex interaction between the natural environment and human activities can be examined and analyzed efficiently through implementing an integrated approach for energy, water, and food resources, and thus contribute to a better understanding of complex and dynamic inter-relationships between them. In this context, it is crucial to develop a comprehensive decision-making framework through the implementation of an effective EWF nexus system that tackles the multifaced issues that EWF systems might face in order to achieve resilient resource management. From an EWF nexus perspective, there are various decision-making techniques and associated tools that have been deployed to tackle the challenges of resources management, such as optimization techniques (Ferdowsi et al., 2021), life cycle assessment (Corona-López et al., 2021), composite indicators (Corona-López et al., 2021), agent-based modelling (Zhu et al., 2023) and game theory models (Namany et al., 2023).
The EWF nexus has become more significant and considerable in many countries, especially those situated within hyper-arid and desert climates, which import most of their food, and/or are dependent on energy-intensive desalination for their freshwater requirements (Netherlands Enterprise Agency, 2019). Hence, it is necessary for those countries to re-configure their resource sub-systems to enhance resilience with regard to integrated resource management, in light of growing risks and uncertainties. Resilience refers to the ability of a system to adapt and bounce back from disruptions or shocks while maintaining its basic structure and function. In the context of EWF systems, a resilient EWF system is one that can withstand and recover from disruptions, such as natural disasters, climate change, or social unrest (Walker et al., 2002). The objective is to enhance resource security while obeying sustainability principles and striving toward resilience. There are multiple strategies system designers can adopt to improve the resilience of the EWF nexus and its constituting resource systems. One of which is the decentralization of resources systems, to avoid the single point of failure risks associated with centralized systems (Perez and dos Santos, 2017). The main difference between the two systems is that decision-making and resource allocation in a centralized system are concentrated in a central authority, while in a decentralized system, they are distributed among many entities or individuals. In the context of EWF, a centralized EWF system is one in which power generation, water supply, and food production are organized and managed by large-scale centralized entities (Bartelmus, 1998), whereas in a decentralized EWF system, these resources are localized and distributed across multiple small-scale units (Koenig et al., 2018). Yet, there are concerns related to the production capacity of decentralized systems. Therefore, adequate, and sufficient capacity over multiple facilities needs to be strategically planned for. Regardless of the mode (i.e., centralized, or decentralized), it is essential to integrate renewable energy to improve the sustainability and resilience of EWF nexus systems. In the context of resilience theory and EWF nexus thinking, decentralization exhibits higher resilience levels thanks to the diversity of the contributing systems which reduces the impact of shocks and disruptions. Having multiple sources for every resource is fundamental to maintaining a continuous supply of products in instances of operational disfunctions or sudden events where some systems are affected while others are intact. From a social perspective, decentralization is also beneficial for community involvement as it fosters local investments and encourages the contribution of the private sector which can fuel collaboration that might reduce shortages.
There are numerous studies that review the EWF nexus, methods, models, and applications, as listed in Table 1. Besides this, some of the previous studies have also included a review of resilience within the EWF nexus. As such, Núñez-López et al. (2022) proposed a mathematical formulation based on a non-linear programming model that integrates the optimization of the resilience of the water-energy-food nexus. The model objectives aim to maximize the system’s resilience, based on the total profit, and minimize CO2 emissions. Another study by Zhu et al. (2022) established an evaluation index system based on the water–energy–food nexus to measure the resilience level of megacities. Further studies assessing the resilience of the EWF nexus system are summarized in Table 2. However, there is a need for a comprehensive review of existing resilience assessment methods considering the decentralization concept that can be employed for EWF nexus studies. The aim of which is to enhance the sustainability and resilience within EWF nexus systems, especially in risky areas (e.g., areas that will probably experience the highest food or water stresses), considering a set of factors, which include, geospatial, technical, environmental, social, economic, and other risk factors that can expose vulnerabilities within EWF nexus systems.
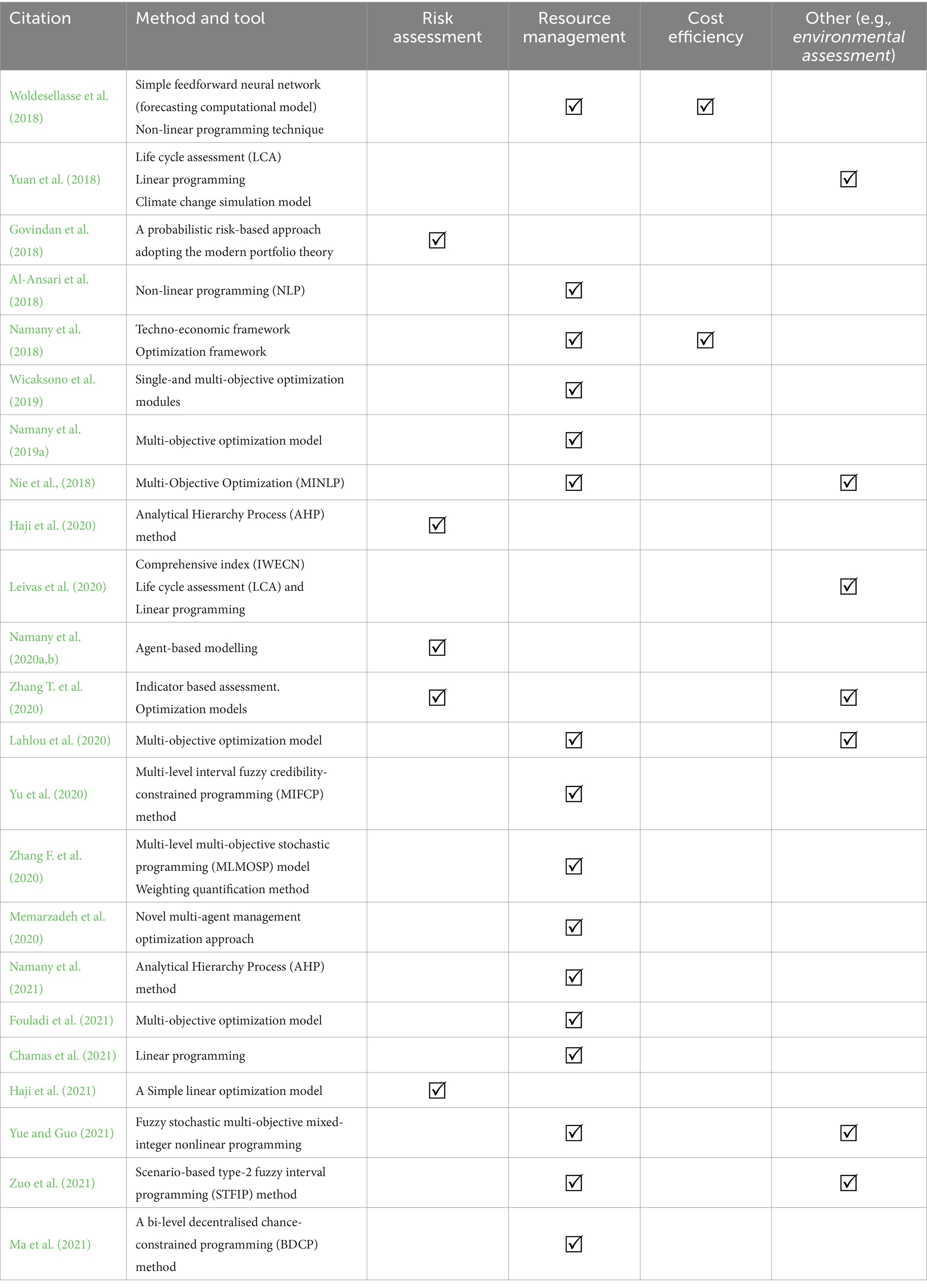
Table 1. Summary table of the current decision-making models and tools employed to EWF nexus systems.
1.1 Review objectives and outline
Deploying an EWF nexus approach could be extremely challenging, given the system’s multi-scale nature, which poses various difficulties and modelling complications. Besides this, uncertainties can arise in the EWF resource systems due to various factors such as unpredictable trade policies, the emergence of new markets and technologies, as well as fluctuations in commodities markets. These uncertainties introduce additional complexities when modeling and analyzing EWF resource systems which are further exacerbated by the multi-sectoral, and multi-uncertainty characteristics of these nexus sectors. Failure to account for such difficulties may affect the resilience of EWF nexus systems and increase the possibility of creating cascading failures. Hence, the objective of this review is to emphasize the various available risk measurement and quantification tools that have been used to address risks governing the EWF nexus systems. Moreover, it highlights the need to build the nexus based on a decentralization perspective in terms of space and time. For that, the review also covers the integrated decision-making methods that have modeled decentralized systems as a strategy to increase resilience and sustainability of the overall nexus and to alleviate resource management challenges in risky environments. To highlight the importance of well-informed decision-making in achieving resilience of the EWF nexus systems, this study will also suggest an Multi-criteria decision-making (MCDM) based strategy to improve the resilience of nexus systems by employing a complete set of criteria such as resource availability, environmental impact, economic feasibility, and social acceptance.
Based on various literature reviewed, this study introduces a perspective through integrating resilience into the decision-making process, marking a significant contribution to the field, and filling up the current literature gaps. Resilience plays a pivotal role in food security, ensuring that a system can withstand shocks and disturbances while sustaining food production and access. By incorporating resilience criteria, this review offers in conclusion a practical method to evaluate the robustness and adaptability of EWF systems, thereby enhancing their overall performance. What sets this approach apart is its hybrid nature, combining qualitative and quantitative methods. By integrating expert knowledge and empirical data, this approach enables a more precise and holistic assessment of the intricate nexus interactions within EWF systems. Policymakers, researchers, and practitioners involved in food security planning and implementation can benefit significantly from the findings and recommendations presented in this study. The strategy outlined here can assist in identifying sustainable interventions that optimize resource allocation, minimize environmental impact, and enhance resilience across EWF systems. In summary, this review emphasizes the importance of adopting an integrated and comprehensive approach to tackle food security challenges by emphasizing the critical role of decentralization in enhancing the resilience of the EWF nexus systems. The novelty lies in underlining that the initial step to quantify the effectiveness of decentralization in improving resilience is to measure it accurately. The review demonstrates that MCDM techniques serve as tools for this purpose, offering a systematic approach to assess the impact of decentralization strategies. Notably, the fuzzy AHP method emerges as particularly significant due to its ability to account for uncertainties inherent in complex, real-world scenarios involving EWF systems. The review aims to underscore the significance of adopting a decentralized approach and employing advanced MCDM techniques, especially the fuzzy method, to accurately measure and enhance the resilience of EWF nexus systems.
The review is organized as follows: the subsequent Section 1.2 explains the methods and steps used to collect the relevant literature related to the review objectives; Assessment methods within the EWF nexus are demonstrated in Section 2; Factors affecting the resilience of the EWF system and resilience assessment approaches employed in the EWF nexus are briefly described in Section 3. In Section 4, decentralization is described further. Then, section 5 discusses the most common tools utilized in integrating various indicators into a single composite indicator. Finally, Section 6 presents the directions for future research, and Section 7 is the conclusion of this review.
1.2 Research methodology
This study discusses peer-reviewed and journal articles published in reputable databases including ScienceDirect, Springer, and ResearchGate between 2010 and 2022. The study emphasizes on the importance of integrating resilience and risk during EWF nexus assessments and explores the use of indicator-based assessment along with spatial tools such as Geographical Information Systems (GIS) for integrated resource management within the EWF nexus context. Furthermore, the review considers decentralization as a means to enhance system resilience and hedge against certain risks. The search for relevant journal papers utilized specific keywords related to the review topic, primarily appearing in the title or abstract. Some of the keywords and phrases used include: EWF nexus, EWF decision-making method, decentralization, decentralized EWF nexus, resilience and EWF nexus resilience. The search generated 103 papers, of which only 84 are selected for the review. These steps are illustrated in Figure 1.
2 The energy water and food nexus
EWF resources, which are inherently interdependent, are essential for human health and survival, economic progress, and resilience. Incidentally, food systems require both water and energy for cultivating, while both food and water are utilized in energy processes, yet energy is necessary in the production and supply of nutritious foods. The interconnected nature of energy, water, and food sectors contributes to a rise in uncertainties concerning resources within these sectors. Hence, the United Nations (UN) University initiated a project that introduced the concept of a nexus approach to resource management entitled: Food-Energy Nexus program in the 1980s (Sachs and Silk, 1990), as part of efforts to improve security across the three resources, through the integrated management and governance across various scales and sectors. Indeed, the study of EWF nexus has attracted considerable attention in recent years as a promising framework for resource management as it acknowledges the inherent interconnectedness between energy, water, and food systems (Biggs et al., 2015). By employing integrated analysis within the EWF nexus it becomes possible to identify important synergies and trade-offs in the design and operation of these resource systems (Al-Ansari et al., 2015; Leck et al., 2015). Moreover, the EWF nexus approach supports the shift towards a green economy by reducing negative environmental, social, and economic impacts, thereby enhancing the efficient use of resources and fostering better policy coherence. Besides this, the nexus framework is essential to ensure sustainable water, food, and energy security at different scales, ranging from local to national to global scales (D'Odorico et al., 2018).
2.1 The EWF nexus studies
In order to implement integrated resource management, based on the EWF nexus, a comprehensive analysis of the opportunities and potential risks related to each of the nexus sectors is required, while analyzing the EWF interlinkages between the resource sectors (Al-Zubari, 2019). Analytical approaches can be deployed to assess the impact of various policies on different sectors and to support policymakers in assessing the EWF nexus while considering unpredicted risks (De Strasser et al., 2016). Consequently, the framework implementation of the EWF nexus modeling tools must incorporate both the interconnections between the sectors and defined system boundaries that depict spatial–temporal scales for decision-making (Bazilian et al., 2011). There are various tools and methods that have been used to examine the EWF nexus, which provide a quantitative assessment of the complexities within the system. However, in light of the growing global challenges and uncertainties, it is necessary to incorporate risk (environmental and technological) assessments, forecasting, mitigation, and adaption plans in the future nexus studies as means to improve the system’s resilience. Table 1 summarizes a few studies that represent various methods and techniques that have been applied to the EWF nexus.
2.2 Risks within the EWF system
In today’s rapidly changing world, nations, societies, and systems are subjected to uncertainties that lead to environmental, economic, and social risks, thus hindering sustainable development. In fact, uncertainties exist in nearly every system, process and activity that significantly impact a tremendous range of situations, including issues from everyday life to the most complicated sciences. In these situations, when the comprehensive knowledge and information related to a certain issue is insufficient or unavailable, then the decision-making becomes challenging, and the decision-maker will always be at position to make wrong judgments and conclusions. Although the definition of risk varies depending on the field, such as engineering, finance, sustainability, etc., there is a common understanding that any type of risk will complicate the decision-making process (Soroudi and Amraee, 2013; Prpich et al., 2014). Normally, any risk assessment of the EWF system should consider a variety of factors ranging from geological, hydrological, and ecological factors to environmental, economic, social, availability and accessibility factors. Thus, by considering the complexities of such problems, policymaking necessitates a more detailed analysis of the different factors that contribute to sustainable development objectives. Figure 2 illustrates that to achieve a sustainable and resilient EWF system, there is a need to adopt a holistic approach in terms of understanding the interdependencies amongst the various sub-systems and drivers. Currently, there are numerous mathematical models and risk assessment approaches that could be deployed to this effect. However, these analyses are usually executed for individual components, such as: environmental or social impact assessment, and may not analyze the aggregated system impact or elaborate on how the impact on a single component can cascade to other sub-systems (Baleˇzentis and Streimikiene, 2017).
Besides this, it is essential to identify, assess and resolve the main geospatial risk factors that can expose vulnerabilities within EWF nexus systems. For instance, in Brazil, Pinheiro Neto et al. (2017) proposed a technique for risk assessment and portfolio optimization of power generation resources using hydro, wind, and solar energy based on the Energy of Mechanism for Reallocation method. The results demonstrated that the proposed method decreases the economic risk of hydroelectric while improving financial return, and thus the optimal portfolio was achieved by lowering portfolio risk and increasing portfolio return. Another study by Zhang et al. (2021) developed an integrated approach to assess the composite risk of WEF nexus systems and generate risk-based plans. The study proposed a composite risk assessment model that captured the interconnection between water, energy, and food scarcity risks using a generalized Copula-based chance-constrained programming model. The approach was applied to an agricultural WEF nexus system in northern China, where water, energy, and land shortages affected agricultural outputs. The results indicated that the composite risk of the entire system was higher than the maximum value among subsystem risks but lower than their sum. Higher composite risks could bring greater benefits, and under a certain composite risk, the overall system benefit varied with different combinations of subsystem risks. Coordinating resources from different subsystems could promote system benefits. Thus, these studies highlight the need for methods and tools that can effectively reduce and control the aggregated risk of an EWF system. By employing risk assessment and optimization techniques, researchers aim to improve the resilience and performance of EWF nexus systems in the face of uncertainties and potential disruptions.
3 Achieving resilience within the EWF nexus systems
The security of EWF nexus is often jeopardized by various disruptions, such as the inadequate and inefficient management of natural resources, as well as natural pandemics that can disrupt the production of EWF resources (Mahlknecht et al., 2020). Furthermore, natural disasters, including floods, droughts, and extreme temperature variations, are among the critical issues affecting the security of the EWF nexus, demanding significant attention (Núñez-López et al., 2021). These phenomena significantly impact the availability and accessibility of natural resources within the EWF nexus context, especially in regions where food production serves as the cornerstone of economic development (Chai et al., 2020).
Applying the concept of resilience is one approach to enhancing the security, availability, and sustainability of the EWF nexus (Sánchez-Zarco et al., 2021), which allows for the examination and analysis of the most vulnerable scenarios in the face of existing disruptions (Hogeboom et al., 2021). Walker (2004) defines resilience as a system’s adaptation, recovery, resistance, or reformation after a shock or disturbance occurs. While, Barker et al. (2013) described resilience as a product of interactions between reliability, vulnerability, survivability, and recoverability, in which the two critical drivers for resilience are vulnerability and recoverability. Initially, the term resilience was mainly utilized by materials science (Chen et al., 2021) and process engineering (Mguni and Van Vliet, 2020). As such, a quantitative performance-based resilience assessment method including a resilience matrix that captured different operational temporal periods was established by Moslehi and Reddy (2018) that incorporates critical resilience elements relevant to engineered systems, which were demonstrated for a typical integrated energy system (IES). Lately, several scholars established frameworks, methodologies, and tools to quantify resilience in energy, water, and food systems. Guaranteeing a safe and resilient supply of energy, water and food resources for all people is a difficult task from local to global scale (McGrane, 2019). The establishment of a secure and resilient EWF system can be achieved by ensuring everyone has consistent and sufficient access to clean energy, water and food (Ingram, 2011). However, achieving these objectives is challenging due to the inherent interlinkages that exist between the EWF resources which introduce trade-offs (Scott, 2019), and due to system vulnerability to various risks such as climate change, population growth and urbanization and poverty.
Evidentially, there is a need to introduce approaches to evaluate the resilience of the EWF nexus system. For instance, Shu et al. (2021) proposed a composite indicator as a part of the WEFWEBs project in order to study the resilience and security of the Water-Energy-Food (WEF) nexus in relatively secured industrialized countries. The composite WEF resilience indicator was obtained by combining two sub-indicators: the first sub-indicator indicates the availability level of EWF resources in terms of the three energy, water and food sectors, and the other sub-indicator indicates the accessibility of population to resources at the household scale. Then, an AHP method was utilized to provide a weight for each sub-indicators within each sector, which was based on an expert assessment of the respective criticality of each sub-indicator. Recently, Singh et al. (2022) established an assessment framework to rank individual countries, whilst allowing decision-makers the possibility of allocating resources sufficiently to achieve food security. The indicators of which were categorized into four groups: food availability, food accessibility, maximum utilization, and food production consistency, wherein the set of food security indicators was assessed based on the future climate change scenario. Table 2 summarizes some of the recent studies related to measuring resilience within the EWF nexus at different scales.
Enhancing resilience within the EWF nexus is imperative to address the multifaceted challenges posed by disruptions and ensure the sustained availability of resources. Various approaches can be adopted to bolster resilience in the EWF nexus, including efficient resource management, technological innovations, and adaptive strategies. However, one approach stands out as particularly effective: decentralization. Decentralization involves the distribution of decision-making authority and resource management across multiple local entities rather than relying on a centralized system. While centralized systems allow for a smoother coordination as decision-making is concentrated within a single entity and authority is consolidated at a uniform level, decentralized systems allow for more flexible and adaptive responses to local conditions and disruptions. In addition, in centralized systems where resources distributions are managed by a single unit, there exists the risk of a single point of failure. In contrast, decentralized systems mitigate the risk through having several spatially dispersed central entities, each possibly possessing distinct resources attributes (Perez and dos Santos, 2017). Considering resources sectors, decentralization comes with multiple advantages counting but not limited to reduction of greenhouse gas emissions (Chmutina et al., 2014), reducing transportation costs and minimizing transmission losses, notably in the energy sector (Lauri et al., 2014). Moreover, empirical evidence supports the efficiency of decentralized approaches. For instance, in the renewable energy sector, decentralized solar and wind installations have proven to be cost-effective solutions for remote and rural areas, bypassing the need for extensive and expensive grid infrastructure. Similarly, decentralized water management practices, such as rainwater harvesting and community-managed irrigation, have demonstrated not only a reduction in water stress but also an improvement in water use efficiency at the local level (Kaundinya et al., 2009). In addition, the decentralized approach fosters community-based resilience, allowing for quicker response and adaptation to localized challenges. In the context of the EWF nexus, decentralization implies empowering local communities to manage their energy, water, and food resources autonomously. Decentralized systems exhibit a higher degree of flexibility and adaptability, enabling them to navigate disruptions more effectively. Localized decision-making facilitates a better understanding of community-specific vulnerabilities and tailors resilience strategies accordingly. For instance, in the face of a natural disaster or a disruption in the supply chain, decentralized energy, water, and food systems can pivot swiftly to alternative sources or adjust consumption patterns based on local needs. Moreover, decentralization aligns with the principles of sustainability and community engagement, as it encourages the development of localized solutions that are tailored to the unique socio-environmental context of each community. This grassroots approach not only enhances the overall resilience of EWF nexus but also promotes community ownership and collaboration. The effectiveness of decentralization in achieving resilience within the EWF nexus is underscored by its ability to mitigate the interconnected challenges of climate change, population growth, urbanization, and poverty. By empowering local communities to take charge of their resources, decentralization establishes a foundation for sustainable practices that address the specific needs of each locality. In conclusion, while multiple approaches can contribute to resilience within the EWF nexus, decentralization emerges as a paramount strategy. Its ability to promote adaptability and foster sustainability makes it a key driver in establishing a secure and resilient EWF system capable of withstanding diverse challenges and disruptions. The following section 4 highlights the application of decentralization within the EWF nexus systems.
4 Technological decentralization of the EWF system
Essentially, decentralizing systems within EWF resource sectors may offer enhanced resilience to the overall EWF nexus system (Biswas and Tortajada, 2019). Figures 3A,B illustrates a food system that is driven by centralized energy and water systems. In this case, there would be a single point of failure, and when realized may cause widespread disruption to the energy and water systems. Shifting from centralized to decentralized systems provides ample backup and shared load which can support the food systems in the case of single or even multiple points of failure.
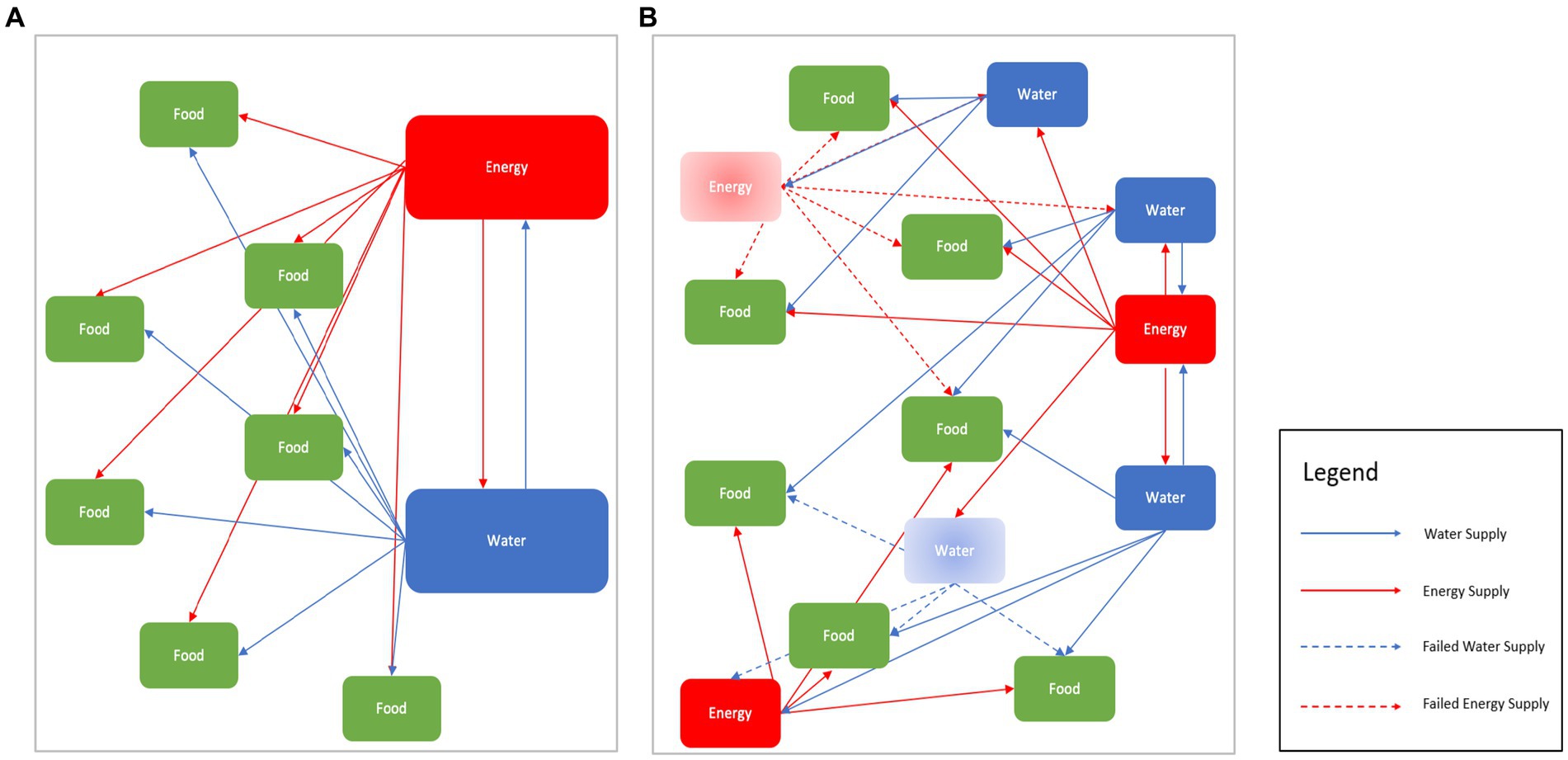
Figure 3. Shifting from centralised into decentralised EWF nexus. (A) Centralised EWF nexus. (B) Decentralised EWF nexus.
Energy networks can be classified as either centralized energy systems, decentralized energy systems, or distributed energy networks. They refer to large-scale power production units that generate energy and distribute it to end users located at various distances from the power generation source. However, decentralized energy systems are based on a variety of small-scale power production units that are generated as per requirement and located close to the end users (Hidayatullah et al., 2011). Decentralized energy systems can be classified further as off-grid (stand-alone or solo-operated), grid-connected, or hybridized models. Likewise, distributed energy networks are another type of small decentralized system, in which individual users establish a complex energy exchange network and at the same time they are energy producers (Vezzoli et al., 2018). For electrification purposes, distributed energy resources (DERs) can be utilized in different configurations, including stand-alone systems, grid-connected systems, or hybrid systems. These systems can be employed to generate electricity at a smaller scale, either independently or in coordination with other systems (Unlocking the Potential of Distributed Energy Resources, 2022). Several studies proved that decentralized energy systems are one of the most effective and long-term sustainable solutions that satisfy the energy demand of future expansion (Ecker et al., 2017). Furthermore, this type of energy system minimizes the overall transmission losses while leaving minimal environmental impacts. For instance, decentralized solar-wind hybrid energy systems can be utilized as an option to meet users’ expanding energy demands while also minimizing overall operational emission levels (Brown, 2009).
The biomass usage in the energy sector is gradually increasing due to several causes, which include its low emission potential and carbon neutrality, growing demand for substitute energy sources, greater governmental initiatives and policies aimed at promoting renewable and environmentally friendly technologies and undiscovered biomass potential (Konuk et al., 2021). Moreover, using biomass for decentralized power production is a rapidly growing concept. However, the economics of biomass-based power plants are highly dependent on a long-term and reliable supply of sustainable feedstock. In terms of agricultural residues, their availability in a certain location along with the transportation cost will determine the feasibility of installing a biomass-based power plant. Thus, region-wise planning is essential to encourage biomass usage for energy purposes (Al-Ansari et al., 2020; Namany et al., 2020a,b).
In India, Singh (2017) utilized the region-wise approach to assess the power potential of agricultural residues. Findings of his research assert that the access to modern energy sources in rural regions is extremely challenging. However, providing power to rural regions through expanding the grid is economically inefficient in almost all situations, notably in urban areas. Simultaneously, the direct connection of rural areas to a centralized energy infrastructure can pose challenges, particularly in developing countries. Centralized energy systems, which are typically large-scale power production units located far away from rural areas, require extensive transmission and distribution networks to reach remote communities. Establishing and maintaining such infrastructure can be financially and logistically be burdensome for developing countries, which are already facing limited resources and underdeveloped power systems (Singh, 2017). On the other hand, the conventional approach to supply electrical energy is by extending the existing transmission and distribution networks to provide access to other disconnected areas (Turkson and Wohlgemuth, 2001). Similarly, the extensive agricultural system is commonly associated with a labor-intensive traditional technique. In many rural countries, agricultural production is affected by farmers with low incomes. Hence, decentralized biomass-powered electrification can provide more consistent and stable supply of energy while also generating revenue obtained from the usage of local resources by farmers (Bisht and Thakur, 2019). Decentralized biomass energy production plays a crucial role in efficiently managing the demand side of an energy system. By distributing energy production across various locations, it becomes possible to align the energy supply with the availability of biomass resources in each area (Herran and Nakata, 2012).
The rapid increase in energy consumption has imposed pressure on both distribution and production systems. Non-renewable energy sources which are limited and harmful to the environment, have traditionally been the primary energy source (Zoungrana and Çakmakci, 2021). Despite electrification efforts in rural areas, the increasing demand resulting from industrial expansion and improved living standards has created a widening gap between energy supply and demand. To alleviate the burden on the centralized grid, there is a global shift towards implementing decentralized energy systems as supplementary support (Burger et al., 2020). Decentralized energy systems offer several advantages, including enhanced energy security, reduced transmission losses, and inclusive growth. These systems hold long-term sustainability potential for future cities (Nadeem et al., 2023). Currently, various renewable energy resources, such as wind, solar, biomass, and hydro, with established and commercially viable technologies, are available to meet daily energy requirements based on their availability. However, to overcome the intermittent nature of renewable energy sources, efficient deployment along with suitable energy storage units is crucial for achieving a consistent energy supply. The proper strategic deployment of these renewable energy resources, taking into consideration their size and compatibility, within decentralized energy systems offers numerous benefits (Sánchez et al., 2022). It does not only reduce the reliance on fossil fuel generation but also minimizes transmission losses. This approach presents a practical and realistic solution for meeting energy needs while promoting sustainability (Javid et al., 2021).
Presently, centralized water systems play a crucial role in supplying freshwater and treating wastewater in many countries (Larsen et al., 2013). They are essential for ensuring adequate water supply, sanitation, and wastewater services in urban areas. However, these centralized systems often require a significant amount of energy for tasks such as collecting, treating, and transporting freshwater and wastewater. As a result, the cost of transportation can be substantial. Additionally, the heavy reliance on energy for water services contributes to the challenges associated with the ‘water-energy nexus,’ which include increasing system vulnerabilities and resource consumption, ultimately impacting the sustainability and resilience of the system (Nair et al., 2014; Valek et al., 2017). Furthermore, centralized water systems usually treat every drop of water to the highest possible quality, resulting in approximately 83% of the treated water being used for non-potable purposes.
In some cases, centralized water systems are challenged by their reliance on conventional ground and surface water resources, which are becoming severely scarce with the increasing demands, and old infrastructure (Hasik et al., 2017), which is costly to renovate and modernize (Grigg, 2015). To address these limitations, decentralized water technologies have emerged as promising solutions for enhancing urban water supply. Decentralized water systems, such as household or community-scale water recycling and rainwater harvesting, are gaining attention and adoption. These systems, located close to or at the point of consumption, can reduce the demand for potable water and eliminate the need for additional treatment and pumping associated with centralized water systems (Stang et al., 2021). However, since it is difficult to predict the energy consequences of integrating decentralized water systems into the current infrastructure of centralized water and wastewater systems (Retamal and Turner, 2010; Sharma et al., 2010), especially in light of unpredictable future climate change, in which, climate change could change the quality and availability of water supply (Mo et al., 2016; Khalkhali et al., 2018). Moreover, it has been proven that climate change has a significant impact on both freshwater needs and wastewater generation (Mo et al., 2016; Khalkhali and Mo, 2020). For example, it has been demonstrated that the consumption of potable water increases when the temperature rises in states such as Nevada (Lott et al., 2013) and Washington (Polebitski et al., 2011). Moreover, climate change also has a significant impact on decentralized water systems, as it could change precipitation patterns and the amount that is essential to the rainwater harvesting systems (Aladenola et al., 2016; Zhang et al., 2019). Thus, the adaptation of decentralized water system while considering the future climate change can have a potential impact on the energy usage of centralized water services. Khalkhali et al. (2021) modeled a framework that combines various empirical models, which was based on multilinear regression analysis, hydrologic modeling, water balance models and life cycle assessment with the purpose of capturing the complex interrelations between centralized water services, decentralized water system adoptions and climate parameters for assessment of cumulative energy demand. The assessment proved that when decentralization was considered as a whole system, it will be an economically feasible system, yet may not always result in energy savings. Adopting rainwater harvesting systems might result in higher energy usage. However, when decentralization and climate change work together simultaneously, the decentralized systems will produce more water and reduce overall costs, while lowering the energy consumption in the centralized systems. Furthermore, when the centralized systems are further included, it was predicted that the total energy demand of the urban water cycle will rise by 0.9% or 2.3% under climate change depending on whether the greywater recycling and rainwater harvesting systems are implemented. Yet, decentralized water systems still can support in eliminating the challenges of water scarcity (Crosson et al., 2021), ecological and environmental concerns (Ren et al., 2017), and the cost associated with the renovation and modernization of centralized water infrastructure through extracting and using different local water sources (Zhang F. et al., 2020). Besides this, it has been discovered that communities are willing to spend more money in order to have higher water quality and less disruptions in their water supply (Wang et al., 2018).
Besides, the current stressors on the water availability, several countries are expressing concerns about the limitations of conventional linear models for urban water management, which do not incorporate onsite water reuse. Thus, decentralized water reuse could be an alternative to the traditional top-down approach based on centralized and linear resource systems (Lu et al., 2019). In this regard, nature-based solutions (referred to as “green” technologies) integrated with advanced technologies (referred to as “grey” technologies) encourage onsite water treatment and reuse in many (Rizzo et al., 2020; Li X. et al., 2021). In many countries, rainwater harvesting (RWH) has been recommended as one of the integrated water management strategies that could deal with stormwater runoff challenges as well as provide alternative residential water sources. Likewise, using greywater recycling (GWR) is another way to improve water supplies, while lowering the load at wastewater treatment plants (Rodrigues et al., 2023). Thus, by integrating RWH and/or GWR systems, there is a possibility to develop a hybrid water system that can be more robust and resilient, cost-effective and energy-efficient; rather than renovating existing centralized water infrastructure (Lu et al., 2013; Cole et al., 2018). Therefore, it’s crucial to facilitate the transition from centralized to hybrid water systems (Hoffmann et al., 2020). In this regard, Li X. et al. (2021) and Li Y. et al. (2021) applied a system dynamic modeling for a case study in Boston, United States to assess the impacts of decentralized technologies on the urban water systems. This was achieved by developing a spatial agent-based model that simulates the adoption of home-based rainwater harvesting (RWH) and graywater recycling for each single-family household. Given the socio-technical consequences and aging water infrastructure systems, the hybridization of water systems might be a promising solution for future urban water system management (Rabaey et al., 2020). As of today, the majority of the studies were conducted to analyze the environmental performance of rainwater harvesting systems (RWHS) and greywater reuse systems (GWRS) independently, and they have shown promising results in comparison to conventional centralized water systems (Teston et al., 2022).
In terms of technological advancement, the coupled “green-grey” technologies (CGGT) have significant capability of enhancing the decentralized treatment schemes in cities through facilitating the water reclamation and/or reuse, improving the treatment of emergent contaminants (by having elevated removal of bio-recalcitrant compounds) and removing the toxic products. Besides that, CGGT considers an appealing alternate solution for minimizing the land footprint (the major obstacle in deploying green technologies in cities), in addition to the energy and costs that are frequently related to standalone grey technologies; as CGGT can enhance the pre-treatment and lower the need for maintenance (Castellar et al., 2022). Yet, limited studies have been conducted to assess the environmental performance of hybrid systems that integrate RWHS and GWRS. Thus, in order to enhance decentralized water management, Gómez-Monsalve et al. (2022) investigated further benefits of these systems in integrated water management. Life cycle assessment was used to quantify and compare the environmental performance of the two hybrid water system options in a high-water consumption household. The result indicated that the deployment of the hybrid system will preserve around 131 m3/year of potable water, 42.5% of annual potable water consumption and decrease flows to the wastewater treatment facility by 20%. When considering the economic aspect, Ghafourian et al. (2022) proposed a framework called Shadow Pricing-Life Cycle Cost–Benefit (SLCCB) in order to assess the economic feasibility of a decentralized hybrid rainwater-wastewater-greywater (HRWG) system based on circular water systems (CWS), in which internal pricing (including: capital and operational expenditure) and External pricing (environmental and social costs-benefits) are the main parameters (costs and benefits) of the implemented SLCCB framework. The proposed SLCCB framework provides a comprehensive approach to evaluate the economic feasibility of decentralized HRWG systems within the context of circular water systems. It considers not only the direct financial costs and benefits but also the broader environmental and social impacts, leading to a more comprehensive understanding of the economic viability of such systems.
Transitioning from the discussion on how decentralization significantly enhances resilience within the EWF nexus, attention now shifts towards the crucial aspect of quantifying the resilience of decentralized systems. Understanding the effectiveness of decentralization necessitates the application of robust assessment tools. The measurement of resilience in decentralized contexts involves a comprehensive analysis of numerous factors, including resource availability, community engagement, and adaptive capacity. This shift in focus directs attention to the diverse methodologies and tools used to assess the resilience of decentralized EWF systems. Unraveling the intricacies of these evaluation techniques offers insights into the tangible impact of decentralization on bolstering resilience within the complex interplay of energy, water, and food resources. The following section 5 summarizes some of the studies that have focused on assessing the level of resilience and stability within the EWF nexus systems.
5 Multi-criteria decision making for resilience assessment
The decision-making process within EWF nexus systems and natural resources management is complex due to their multi-sectoral, multi-stakeholder, and multi-scale components (Madani et al., 2015). Therefore, it is necessary to acquire an adequate and extensive understanding of EWF nexus systems, and to capture the complexity when performing multi-dimensional and multi-sectoral assessment. Comprehensive assessment of the interlinkages between EWF systems will enable improvement of the sectors alone and collectively at various decision-making levels, starting from the process up to governance (Okonkwo et al., 2023), such that cascading losses and significant vulnerabilities could be eliminated. Consequentially, given the complexity of EWF nexus interdependences, effective and reliable decision-making methods can capture the dynamics, while mitigating the issues associated with the complexities of the nexus system. In this regard, a review by Namany et al. (2019a,b) and highlighted three decision-making methods that are significantly efficient in solving resource management challenges, which include mathematical optimization, agent-based modelling, and game theory. Mathematical optimization encompasses a range of techniques and solution algorithms that can manage the multiple challenges of EWF Nexus systems, such as multi-spatial, multi-temporal, multi-scale, and multi-sectoral problems. These optimization methods help find optimal solutions to resource allocation and utilization, considering various constraints and objectives. However, agent-based modelling and simulation can capture the activities and unpredictability associated with specific concerns within the EWF Nexus. This approach emphasizes the interactions and behaviors of individual agents, allowing for a more nuanced understanding of complex dynamics and the identification of emergent patterns. In terms of the multi-stakeholder and multi-objective nature of the EWF Nexus, game theory proves to be valuable. Game theory provides a framework to address competition and conflicts among stakeholders, especially when optimization solutions may be sub-optimal. It allows for the exploration of strategic interactions, decision-making processes, and potential cooperative solutions.
Several computational approaches and methods have been established to enhance decision-making across EWF Nexus systems. For instance, Schull et al. (2020) demonstrated that by integrating the WEF Nexus Tool 2.0 with a comprehensive watershed model (e.g., SWAT), a more comprehensive understanding of the WEF Nexus can be achieved. Integrating forecast meteorological information into the study methods can support the identification of future challenges regarding water resource management approaches and establish robust solutions. Recently, Chamas et al. (2021) developed a regional-scale optimization model for managing and allocating energy, water and food nexus resources under different resource, policy and technical constraints. The study findings demonstrated that optimization models can enhance the understanding of the interconnections between the EWF nexus sectors, in which the optimal resource allocation strategies will be achieved despite the imposed constraints on the natural resources, environment and technology. As such, enforcing the constraint on food will increase water consumption by a magnitude while more than doubling energy requirements. Furthermore, deploying renewable/sustainable energy may increase land requirements, although it will tremendously reduce CO2 emissions.
Prior to delving into optimization strategies and tools aimed at improving the resilience of decentralized EWF systems, it is imperative to conduct a thorough assessment of their performance and identify potential weaknesses. This initial step lays the foundation for quantifying resilience, providing insights into the system’s vulnerabilities and strengths. Assessing the current state of decentralized EWF systems allows for a comprehensive understanding of their dynamics. This assessment not only informs subsequent optimization efforts but also serves as a baseline for measuring the effectiveness of resilience-enhancing interventions. By scrutinizing the existing challenges and limitations within decentralized EWF systems, stakeholders can develop targeted strategies to fortify their capacity to withstand disruptions. This proactive approach ensures that optimization efforts are tailored to address specific weaknesses, fostering a more resilient system overall. Therefore, the assessment phase serves as a critical precursor to the implementation of optimization tools, offering valuable insights that guide decision-making and resource management. The following sections 5.1 and 5.2 focus on some multi-criteria decision-making tools that can be used for the resilience assessment of decentralized nexus systems.
5.1 Indicator-based assessment within EWF nexus
In the context of the EWF Nexus systems, the complexity calls for the development of indicators that can effectively evaluate their performance. These indicators play a crucial role in informing decision-making processes and improving communication among stakeholders. According to the Organisation for Economic Cooperation and Development (2000), any observable and measurable variable can be transformed into an indicator, where it can serve as representatives of specific aspects or characteristics of the system under consideration, and can provide valuable information and insights into the performance, trends, or conditions of the system (Giupponi and Gain, 2017). Moreover, indicators are particularly important when assessing progress towards sustainable development. They provide valuable perspectives on the environmental, economic, and social performance of the EWF Nexus system (OECD, 2005). They provide better insight into decisions and result in more effective actions through clarifying, simplifying and aggregating information to be available for decisions and policymakers; since indicators can help in incorporating social and physical science knowledge and translating them into manageable units of information. Furthermore, as progress can be quantified, indicators can provide early warning signals to avoid environmental, economic and social setbacks (United Nations, 2007). In terms of measuring progress toward sustainable development, there are various developed models that measure the status at any given point. One such model is the Index of Sustainable Economic Welfare (ISEW) analyses the impact of used resources (Cobb et al., 1994). Other models focus on assessing the sustainability of specific sectors or sub-sectors, such as studying the organic food consumption (Venghaus and Dieken, 2019). In fact, environmental problems often involve intricate interconnections and factors, making direct measurement challenging. Indicators play a crucial role in providing a simplified representation of the environmental state, which can be easily communicated to policymakers and other stakeholders. These indicators encapsulate complex information and metrics into concise measures that can be tracked over time, enabling policymakers to monitor progress and identify areas of concern. By using indicators, policymakers gain a clearer understanding of the environmental status, trends, and potential challenges. They provide valuable insights into the environmental performance of specific sectors or the overall sustainability of development efforts. Indicators can capture key aspects such as resource usage, pollution levels, biodiversity, and other relevant environmental factors. This information helps policymakers make informed decisions, set targets, and develop effective strategies to address environmental issues.
Environmental indicators are necessary to evaluate environmental and economic states as they relate to one another, identify causes to support management for proper actions, detect harmful changes in early stages in order to prevent permanent damage and identify environmental trends over time (Rubio and Bochet, 1998). In the past few decades, various indicator-based methods have been developed to assess the sustainability and environmental impacts of agricultural systems and activities. These methods provide valuable insights into different aspects of agricultural sustainability and resource management within the EWF Nexus (Bockstaller et al., 2009). Some of the indicators commonly used in assessing agricultural sustainability include the Water Scarcity Index, which measures the availability of water resources relative to water demand (Ren et al., 2021), Energy Intensity, which quantifies the energy input required for agricultural production (Elsoragaby et al., 2019), Food Loss and Waste, which evaluates the amount of food lost or wasted throughout the supply chain (FAO, 2021), Carbon Footprint, which measures the greenhouse gas emissions associated with agricultural activities (Bhatia et al., 2023) and Water Footprint, which assesses the water consumption and pollution associated with agricultural production (Mekonnen and Hoekstra, 2021). Furthermore, these indicators can be used as synthetic information in geographic information systems (GIS) in order to assess the spatial distribution of environmental impacts and link anthropogenic activities to specific areas. This allows for a comprehensive understanding of the geographic extent and variability of environmental challenges associated with agricultural practices (Rubio and Bochet, 1998). In cases where existing indicators may not be suitable or available for a specific problem or scale, new and specific indicators can be developed to address the unique challenges within the EWF Nexus (Flammini et al., 2014). This highlights the importance of tailoring indicators to the specific context and objectives of the study, as distinct aspects of the EWF Nexus may require different indicators to capture the relevant resource sub-systems.
Currently, there are several indicators for individual nexus elements, such as; the Energy Supply or Demand Index (Kruyt, 2009), Share of Renewable Energy in the Energy Mix (REN21, 2021), Carbon Dioxide Emissions per Capita (World Bank, 2021), Water Stress Index (WRI, 2021), Food Waste per Capita (FAO, 2021) and Global Food Security Index (Rosegrant, 2003). Generally, these indicators consider the stresses imposed by the availability of the other two EWF resources. However, it does not specifically reflect the feedback effects on the essential resources. Moreover, many indicators that capture the EWF nexus as a whole have been established for a particular scale or application, such as irrigated agriculture (Martin-Gorriz, 2014).
Composite indicators represent an effective tool that is commonly used across a wide range of academic fields to describe different types of information (Becker, 2017). Within the EWF nexus, the establishment of composite indicators is a common method for a quantitative assessment (EC, 2020). There are several established EWF nexus composite indicators that account for the inter-sectoral interactions; at the national level to facilitate the inter-country evaluation and assessment, or at various geographical locations to evaluate resources (Giupponi, 2017). These indicators focus on resource availability while giving less attention to the population’s accessibility to utilize those resources. For instance, recently an indicator was established that focuses on the Mediterranean climatic zone (Saladini, 2018). This type of composite indicator enables the focus on challenges that are extremely crucial in specific regions, such as cereal crop production and demographic characteristics. This type of zoning by climatic area is a common method of combining regions with relatively identical natural resource characteristics, mainly those characteristics related to water and agriculture, or the need of highlighting the socioeconomic characteristics of the EWF nexus.
5.2 Development of composite indicators
Multi-criteria decision-making (MCDM) techniques and the Analytical Hierarchy Process (AHP) method have been deployed to develop composite indicators within various resource management studies. The AHP is a compensatory MCDM technique created in 1970 by Saaty. It is employed to create a hierarchy among a group of decision options by considering both subjective and objective evaluations (Saaty, 1988). Numerous studies focusing on energy, water, or food dimensions have used the AHP tool to determine the relative importance of factors, prioritize various alternatives and determine optimal solutions. Table 3 highlights recent studies utilizing an integrated AHP-GIS approach applied in different sectors. Consequently, previous studies proved that the aggregated MCDM-AHP-GIS method is a beneficial approach that can be utilized to develop a risk map that can offer critical information for determining the relative risk of a region, which may then be utilized for future planning and management. Although, MCDM approaches have proven to be effective and beneficial in decision-making in a wide range of fields, including hybrid energy systems (Perera et al., 2013), solar power plant site selection (AlGarni and Awasthi, 2017) and renewable energy project risk assessment (Liang et al., 2021). However, sometimes the conventional MCDM methods are unable to control and eliminate subjective biases (Tadić et al., 2014). In an inadequate MCDM environment, the conventional MCDM methods are replaced with Fuzzy MCDM methods that allow for objective judgments, where this method will objectively capture the inconsistencies in human judgment, and efficiently addresses the uncertainties in the existing information (Kaya et al., 2019). Thus, fuzzy MCDM methods were introduced to handle instances where the information driving decision-making is imprecise or vague. The fuzzy framework employs qualitative words such as high and low to capture uncertainties and it has proven its effectiveness in dealing with real-world problems where crisp information is often unavailable or unprecise (Abdullah, 2013). In this regard, a very recent study by Shweta et al. (2022) introduced a “Social, Economic, Environmental, and Technical Assessment model.” This model integrated four Fuzzy MCDM methods: Fuzzy Stepwise Weighted Assessment Ratio Analysis, Fuzzy Multi-Objective Optimization by Ratio Analysis, and Fuzzy Weighted Assessment Ratio Analysis. The proposed approach prioritizes the site selection of 14 existing hydropower plants in India based on social, economic, environmental, technical, and logistical criteria.
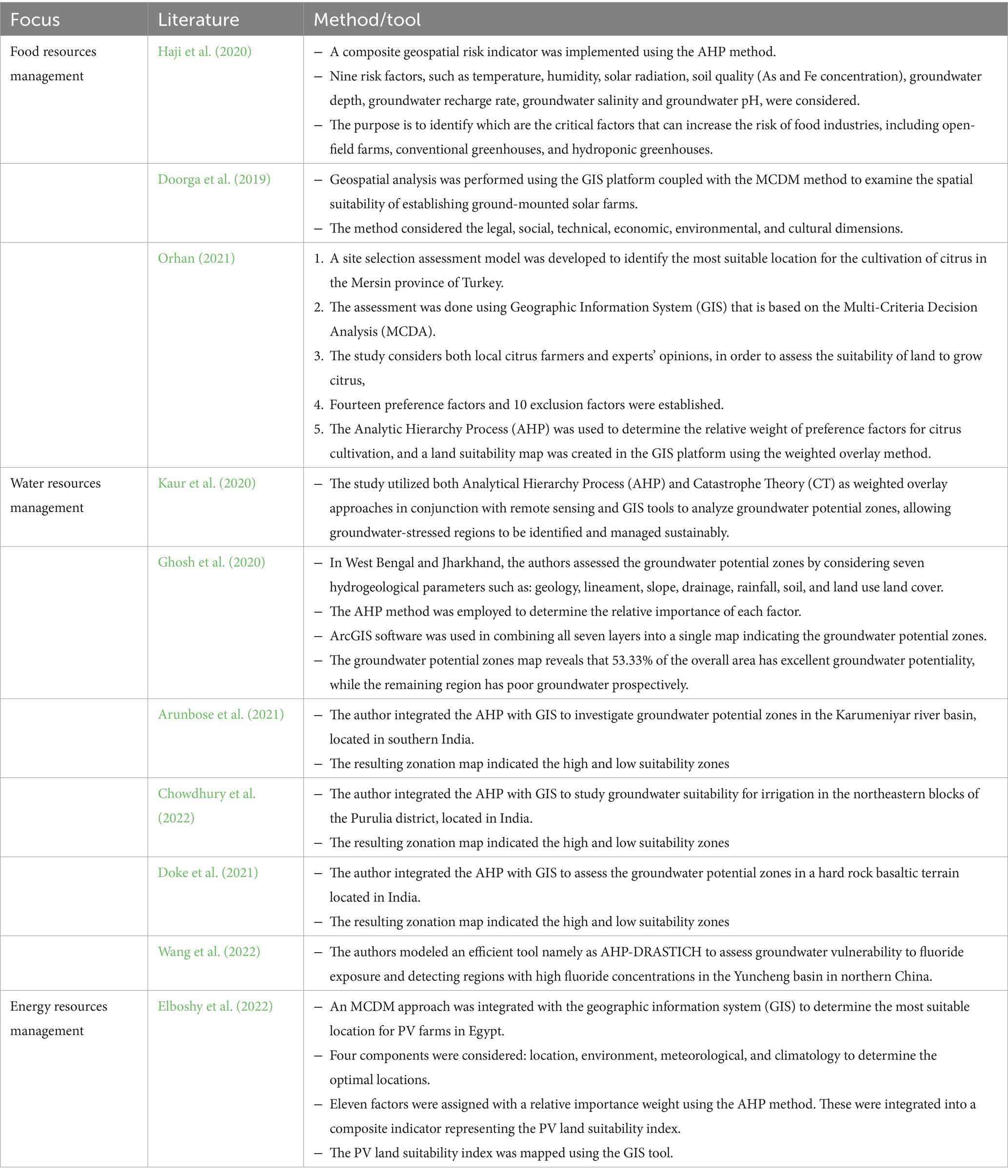
Table 3. Summary table of the existing Analytical Hierarchy Process (AHP) applied to different sectors within EWF nexus systems.
When transitioning from the exploration of individual and collective indicators for assessing decentralized systems, it is crucial to acknowledge that specific indicators can also evaluate the level of decentralization and its impact on resilience within the context EWF nexus systems. The complexity of these systems necessitates the development of indicators that effectively evaluate their performance, offering valuable information for decision-making and stakeholder communication. In the context of the nexus systems, the quantification of resilience within decentralized frameworks requires a nuanced approach. While specific references directly addressing the quantification of resilience in decentralized EWF Nexus systems might be limited, insights from related fields offer valuable perspectives. It is essential to recognize that beyond technical assessments, a comprehensive evaluation must encompass socio-economic governance aspects. The previously discussed indicators for assessing decentralization become pivotal in this scenario. Quantifying decentralization involves a comprehensive assessment of various indicators that illuminate the distribution of decision-making authority and resource management across diverse levels of governance. These indicators, such as local autonomy, fiscal decentralization, administrative decentralization, and political decentralization, provide insights into the degree of decision-making authority, financial resource allocation, administrative functions, and political power granted to local or regional entities (OECD, 2019). Furthermore, assessing service delivery efficiency, decision-making processes, community participation, legal frameworks, policy implementation, transparency and capacity building contributes to a holistic understanding of the decentralization dynamics, encompassing both technical and socio-economic dimensions. For the EWF nexus, these indicators serve as integral components in multi-criteria decision-making processes specifically designed to evaluate the performance of decentralized EWF nexus systems. Together, they offer a nuanced and comprehensive picture of the decentralized EWF nexus system’s performance, shedding light on the distribution of powers, responsibilities, and resources across various tiers of governance. The selection of these indicators is contingent upon the specific goals and objectives of decentralization initiatives, adapting to the unique contexts and requirements within different regions or sectors. They provide a practical link between the conceptual understanding of decentralization and the applied assessment of decentralized EWF nexus systems, considering both technical and socio-economic governance factors and their implications for system resilience.
6 Discussion and future research endeavors
The key driver for reviewing and evaluating the EWF nexus, resilience and decentralization concepts described in the preceding section is mainly to demonstrate their effectiveness in tackling the complex challenges in resource management from a sustainability and resilience standpoint. The decision for integrating resilience and decentralization concepts along with EWF Nexus in one review was not decided at random. This is due to the fact that when these methods are combined, they can convey exceptional efficacy in solving significant and complex issues. In reality, it is not a minor effort to develop resilient and sustainable resource management systems, while considering the environmental, economic, social, and technological risks. Hence, it is crucial to develop a comprehensive framework that can efficiently handle various scenarios within complex systems, while considering their dynamic interlinkages. Moreover, the possible risk and uncertainties existing within the systems’ environment must be taken into account and integrated with these models. Developing such schemes involves precise decision-making processes that can deliver sustainable solutions at minimal cost and risk, but more crucially, is to achieve solutions that are feasible, relevant, and practical to be applied in a real-world setting.
The studies reviewed in this study have demonstrated the effectiveness of decision-making tools in addressing and tackling the current existing challenges of resource management. Yet, many studies have assumed about the system boundaries, which in turn makes it difficult to assess the system holistically. In fact, many existing studies explored the interconnectedness of EWF systems by focusing only on two sectors of the EWF nexus. As a result, the robustness of these models will decline, especially when addressing real-life issues that fundamentally need a multi-sectoral approach to attain resilient and reliable allocation and utilization of resources. Indeed, among the three aspects of the EWF Nexus, water-related issues, including drinking water and wastewater management, have received significant attention in research. However, to enhance our understanding of the interconnected roles of energy, water, and food in improving natural resource resilience and reducing multidimensional poverty, it is crucial to expand initiatives that simultaneously address all three aspects. By taking a comprehensive approach and considering the interdependencies and synergies between energy, water, and food systems, we can develop more effective strategies to enhance resource resilience and address the complex challenges associated with poverty alleviation. Such initiatives can provide valuable insights and contribute to sustainable development efforts.
However, there is another portion of some existing studies that have highlighted this deficiency through integrating all the sub-systems of EWF resources into a single system boundary. But even so, the findings from the employed decision-making approaches have contributed to optimal but impractical solutions. Moreover, from natural resources decentralization perspective, existing studies have mostly focused on decentralized water technologies considering technical development, economic feasibility, and water quality control. However, the future transition should look to the entire EWF system, which is complicated, dynamic, and unpredictable. Hence, in order to tackle these resource management issues, a comprehensive systems approach integrating all EWF sectors and focusing on the internal and external interactions should be employed. In reality, these systems are often comprised of complex interlinkages. Thus, integrating the decentralization concept along with EWF Nexus can simplify overall modeling and improve system visibility and transparency. Besides this, identifying the appropriate decentralized technologies mix is crucial to the success of the entire system. Moreover, there is a lack of knowledge on the spatial adoption and understanding of decentralization and its impact on the EWF system. To model the complex interaction between EWF systems, Nexus “node” approach can be used to study the dynamic nature of EWF system technologies (Haji et al., 2020).
One of the main challenges with the existing indicator framework is its failure to explicitly capture the essential interactions between EWF resources. These interactions play a crucial role in addressing the primary challenges and risk factors associated with the EWF system. The current framework may overlook the complex interdependencies and trade-offs between these resources, which can hinder a comprehensive understanding of the system and hinder effective decision-making. Hence, the risk component has to be incorporated into the EWF nexus framework to have a more precise representation of the interrelationship within the nexus sectors. As such, in energy sectors, the transmission constraints can potentially prohibit achieving the optimal energy mix due to capacity, so neglecting the transmission constraints in mathematical modeling can lead to unachievable impractical optimal mix. Furtherer, one of the major gaps in all of the existing models is the spatial representation of energy, water and food systems including renewable and non-conventional resources. The growing adoption of renewable sources necessitates geographical differentiation in portfolio analysis, as the availability of resources could vary dramatically between various locations/regions. This emphasizes the importance of geospatial representation in understanding renewable and nonconventional energy and water resources, as they may exhibit potential synergies that can minimize output variability and support a stable system. Thus, to achieve a sustainable system, a robust EWF network is essential to effectively utilize renewable and nonconventional resources distributed across the region. In general, the focus must be given to the critical factors and parameters that influence the overall performance of integrated technologies. Besides this, due to the novelty of the coupled green-gray/gray-green technologies, it is necessary to further investigate their performance compared to standalone technologies. This examination should include aspects such as cost reduction, energy demand, and the land footprint required.
Consequently, the knowledge gap can be tackled by the development of natural resources atlas by applying the concept of decentralization using GIS-based approaches. This involves identifying suitable locations for energy, water, and food resources, systems, and sub-systems, and understanding their spatial relationships; additionally, it is important to identify risk factors that affect EWF systems, taking into account technical, environmental, economic, and social aspects. These risk factors can be used to develop composite risk indicators using the Fuzzy AHP method; and then integrating fuzzy AHP, specifically interval type-2 fuzzy set into EWF risk associated portfolio optimization of different Energy-Water technologies mix to enhance resilience by mitigating and adapting to risks. This includes the identification of the optimal location of various EW technologies mix, in order to reduce risks and costs associated with the overall EWF system. The advantage of using an interval type-2 fuzzy set is that it is capable of providing more accurate modeling of high-order uncertainty in EWF risk-associated portfolio optimization. Moreover, in comparison to type-1 fuzzy sets, interval type-2 fuzzy sets focus on indeterminate information with high uncertainty and provide an adequate representation of experts’ knowledge in terms of fuzzy rules. This is effective for addressing the challenges within EWF risk-associated portfolio optimization through capturing the discrepancies of cost variables such as the cost of water or energy and expressing the expert’s recognition and awareness of some qualitative variables like public acceptance. In addition, assessing the change in EWF resource availability and identifying tradeoffs associated with the large-scale implementation of decentralized energy and water technologies is crucial to achieve sustainable development.
Identification of optimal location based for an energy-water technology mix with the aim of achieving resilient food production requires an integrated assessment of the overall EWF system. In general, the energy subsystem is accountable for fulfilling energy and power requirements using renewable and non-renewable energy sources. The water subsystem comprises a variety of water sources, including groundwater aquifers, desalinated water, and treated wastewater. While the food subsystem encompasses agriculture activities that are ranging from food processing to cultivation for livestock raising. Where in fact all subsystems are interrelated, the output of one sector can be utilized as an input to another sector. According to the system boundary that specifies the EWF Nexus scale and unit (for example: regional, national, or global), the interlinkages between each system can alter. Functionally, the food sectors are driven by the outputs from sectors. At the same time, the outputs from the food systems are also fed back into the energy and water systems establishing an interlinked cycle. Normally, the energy system splits into renewable and non-renewable energy sources; this includes fuel, solar, wind and bioenergy. The water system comprises groundwater, desalinated water, and treated wastewater. Whereas the food system encompasses agriculture (open field and greenhouse farming) and fertilizers production. These kinds of complex systems necessitate several strategic, tactical, and operational decision-making during the life cycle of the comprising sub-systems. Therefore, similar techniques to those discussed previously can be deployed in order to analyze the interlinkages between the energy, water, and food sectors, with the objective of achieving a resilient EWF system.
In summary, upon reviewing the existing literature on the EWF nexus, resilience, and decentralization, a significant knowledge gap was uncovered, highlighting several under-investigated areas crucial for advancing sustainable resource management. Firstly, the decentralization of EWF sectors through GIS-based methodologies emerged as a pivotal yet scarcely explored field. Such approaches promise to significantly enhance resource management within the EWF nexus, yet their potential remains untapped in current studies. Additionally, the development of composite indicators, especially from a risk and resilience perspective employing fuzzy set theory, was identified as a critical gap. These indicators are essential for capturing the nuanced interdependencies and uncertainties inherent within the EWF nexus, yet comprehensive research in this area is lacking. Furthermore, the application of optimization techniques specifically tailored for risk mitigation and adaptation, aimed at efficient resource utilization within the EWF nexus, was another area found to be insufficiently addressed. These strategies are vital for the sustainable management of resources but have not been extensively explored. Lastly, the absence of a comprehensive, user-friendly geospatial assessment tool designed for visualizing and analyzing integrated data within the EWF nexus was noted. Such a tool is imperative for a deeper understanding of the interconnections and dynamics of the nexus, yet its development and implementation are markedly absent from the literature. Addressing these gaps through future research is essential for developing innovative solutions to the complex challenges faced within the interconnected domains of energy, water, and food, thereby advancing the field of sustainable resource management.
It is also worth noting that in the context of the EWF nexus and resources management, achieving resilience sometimes implies the aggregation of centralized and decentralized sub-systems to insure the overall system stability. One representative example consists of the combination centralized electricity grids and decentralized renewable energy sources such as photovoltaics and biomass-based power plants to meet the local demands especially in environments with limited non-renewable energy resources such as oil and gas but with an abundant access to solar radiations. It is also important to highlight that the efficacy of either method in improving the resilience of the system is subject to multiple conditions associated with socio-economic, political, and environmental set-ups of every region or area adopting a certain EWF nexus system. For instance, the success or failure of decentralized systems along with their performances are highly influenced by the socio-cultural habits and collaborative behaviors amongst the involved stakeholders in addition to the regulations and rules governing them. Participation of the communities where the decentralized systems are located is for their prosperity as it diminishes transaction costs and bureaucratic decisions. In addition, strong community engagement, and capacity building are also paramount in order to effectively make use of the available resources by decentralized units. For example, in Nepal, it was suggested that the community-based method, fostering cooperation and community engagement, has enhanced long-lasting viability through local involvement and facilitated the expansion of decentralized energy initiatives, thanks to successful partnerships and creative financing methods (Gollwitzer, 2014). However, the social conditions of decentralization are also bounded by several political and institutional restrictions that restrain the success of decentralized systems. In fact, even in developed countries such as Germany, the Netherlands, and Norway, the adoption of decentralized renewable energy investment did not occur without the intervention of the government (Wohlgemuth and Madlener, 2010). Indeed, the success of decentralization is subject to the legislation of policies and supportive governmental rules to enforce the implementation of associated decisions and plans. As for the economic conditions, thorough economic viability analyses should be conducted prior to the execution of decentralized projects in order to maximize their benefits and ensure their alignment with the country’s financial capabilities.
7 Conclusion
In an increasingly unpredictable global context characterized by environmental, economic, and social fluctuations, the need for resilient systems, particularly within the EWF Nexus, has been underscored. The EWF Nexus, impacted by population growth and climate change, necessitates sophisticated decision-making frameworks capable of addressing its inherent complexities. The potential of decentralized strategies, enhanced by analytical methods such as Fuzzy MCDMs, in building resilience within EWF systems, is emphasized in this study. These methods provide a detailed understanding of the challenges, allowing for the identification of viable, sustainable solutions that consider the unique spatial and temporal dimensions of the EWF Nexus. Key findings highlight the effectiveness of decentralized approaches, particularly when coupled with Fuzzy MCDM techniques, in enhancing the resilience of the EWF Nexus by accommodating the uncertainties typical of decentralized systems. This combination of methodologies offers a refined toolset for quantifying resilience, facilitating informed decision-making in scenarios where data may be incomplete or uncertain. This paper also reviews essential resilience assessment tools for resource management and advocates for the integration of Fuzzy MCDM methods into the EWF nexus framework as a robust approach to decision-making. This integration signals a shift towards more resilient and sustainable EWF systems capable of adapting to global changes. Future directions consist of the focus on the practical implementation and application of these decision-making techniques to further enhance decision processes within the EWF Nexus, especially in ensuring food security amid global uncertainties. The advancement in applying these methodologies is crucial for the development of resilient, sustainable EWF systems prepared to navigate the complexities of dynamic environments.
To this foundation, it is imperative to acknowledge the non-linear interactions within the EWF nexus, where external factors such as policy changes, technological advancements, and market dynamics can have ripple effects across energy, water, and food systems. For example, an innovation in agricultural technology might reduce water and energy usage, significantly altering the nexus dynamics. Conversely, energy policy shifts can impact water usage and food production costs. Such interdependencies underscore the importance of a holistic view in Nexus management, considering how actions in one sector can lead to unexpected outcomes in others. Addressing these non-linearities and embracing a systems thinking approach will enrich our understanding and management of the EWF Nexus, ensuring that resilience strategies are robust, adaptable, and grounded in the reality of interconnected resource systems.
Author contributions
MH: Conceptualization, Investigation, Methodology, Writing – original draft.. SN: Conceptualization, Investigation, Methodology, Writing – review & editing. TA-A: Conceptualization, Methodology, Writing – review & editing, Supervision.
Funding
The author(s) declare financial support was received for the research, authorship, and/or publication of this article. This research was made possible by an Award (GSRA7-1-0407-20014) and supported by proposal number NPRP11S-0107-180216 from Qatar National Research Fund (a member of Qatar Foundation). The contents herein are solely the responsibility of the authors[s].
Conflict of interest
The authors declare that the research was conducted in the absence of any commercial or financial relationships that could be construed as a potential conflict of interest.
The author(s) declared that they were an editorial board member of Frontiers, at the time of submission. This had no impact on the peer review process and the final decision.
Publisher’s note
All claims expressed in this article are solely those of the authors and do not necessarily represent those of their affiliated organizations, or those of the publisher, the editors and the reviewers. Any product that may be evaluated in this article, or claim that may be made by its manufacturer, is not guaranteed or endorsed by the publisher.
References
Abdullah, L. (2013). Fuzzy multi criteria decision making and its applications: a brief review of category. Procedia. Soc. Behav. Sci. 97, 131–136. doi: 10.1016/j.sbspro.2013.10.213
Aladenola, O., Cashman, A., and Brown, D. (2016). Impact of El Niño and climate change on rainwater harvesting in a Caribbean state. Water Resour. Manag. 30, 3459–3473. doi: 10.1007/s11269-016-1362-2
Al-Ansari, T., AlNouss, A., al-Thani, N., Parthasarathy, P., ElKhalifa, S., Mckay, G., et al. (2020). “Optimising multi biomass feedstock utilisation considering a multi technology approach” in 30th European symposium on computer aided process engineering. eds. S. Pierucci, et al., vol. 48 (Elsevier B. V.), 1633–1638.
Al-Ansari, T., Korre, A., Nie, Z., and Shah, N., (2015). “Development of a life cycle assessment tool for the assessment of food production systems within the energy, water and food nexus,” Sustain. Prod. Consum., 2, 52–66.
Al-Ansari, T., Govindan, R., Korre, A., Nie, Z., and Shah, N. (2018). An energy, water and food nexus approach aiming to enhance food production systems through CO2 fertilization. Comput. Aided Chem. Engin. 43:1487–1492. doi: 10.1016/B978-0-444-64235-6.50259-X
AlGarni, H., and Awasthi, A. (2017). Solar PV power plant site selection using a GIS-AHP based approach with application in Saudi Arabia. Appl. Energy 206, 1225–1240. doi: 10.1016/j.apenergy.2017.10.024
Al-Zubari, W. (2019) The water-energy-food nexus in the Arab region: understanding the nexus and associated risks., Bonn: Water, Energy & Food Security Resource Platform.
An, R., Liu, P., Feng, M., Cheng, L., Yao, M., Wang, Y., et al. (2021). Resilience analysis of the nexus across water supply, power generation and environmental systems from a stochastic perspective. J. Environ. Manag. 289:112513. doi: 10.1016/j.jenvman.2021.112513
Arunbose, S., Srinivas, Y., Rajkumar, S., Nair, N. C., and Kaliraj, S. (2021). Remote sensing, GIS and AHP techniques based investigation of groundwater potential zones in the Karumeniyar river basin, Tamil Nadu, southern India. Groundwater Sustain. Develop. 14:100586. doi: 10.1016/j.gsd.2021.100586
Baker, J. S., and Houtven, G.Van (2021) A hydro-economic energy-water Nexus. doi: 10.3768/rtipress.2021.mr.0044.2105
Baleˇzentis, T., and Streimikiene, D. (2017). Multi-criteria ranking of energy generation scenarios with Monte Carlo simulation. Appl. Energy 185, 862–871. doi: 10.1016/j.apenergy.2016.10.085
Barker, K., Ramirez-Marquez, J. E., and Rocco, C. M. (2013). Resilience-based network component importance measures. Reliabil. Engin. Syst. Safety 117, 89–97. doi: 10.1016/j.ress.2013.03.012
Bartelmus, P. (1998). Centralised versus decentralised systems for environmental management: lessons from the history of economic thought. Ecol. Econ. 27, 121–137.
Bazilian, M., Rogner, H., Howells, M., Hermann, S., Arent, D., Gielen, D., et al. (2011). Considering the energy, water and food nexus: towards an integrated modelling approach. Energy Policy 39, 7896–7906. doi: 10.1016/j.enpol.2011.09.039
Becker, W. S. (2017). Weights and importance in composite indicators: closing the gap. Ecol. Indic. 80, 12–22. doi: 10.1016/j.ecolind.2017.03.056
Bhatia, L., Jha, H., Sarkar, T., and Sarangi, P. K. (2023). Food Waste Utilization for Reducing Carbon Footprints towards Sustainable and Cleaner Environment: A Review. International Journal of Environmental Research and Public Health, 20, 2318. doi: 10.3390/ijerph20032318
Biggs, E. M., Bruce, E., Boruff, B., Duncan, J. M. A., et al. (2015). Sustainable development and the water–energy–food nexus: a perspective on livelihoods. Environ. Sci. 54, 389–397. doi: 10.1016/j.envsci.2015.08.002
Bisht, A. S., and Thakur, N. S. (2019). Small scale biomass gasification plants for electricity generation in India: Resources, installation, technical aspects, sustainability criteria & policy. Renewable Energy Focus, 28:112–126. doi: 10.1016/j.ref.2018.12.004
Biswas, W. K., and Tortajada, C. (2019). Water, energy, and food security in the Asia Pacific region. UNIVERSITY OF HAWAII AT MANOA.
Bockstaller, C., Guichard, L., Keichinger, O., Girardin, P., Galan, M. B., and Gaillard, G. (2009). Comparison of methods to assess the sustainability of agricultural systems: a review. Sustain. Agric. 29, 769–784. doi: 10.1007/978-90-481-2666-8_47
Bonn2011Conference (2011) The water, energy and food security Nexus – Solutions for a Green Economy. Bonn, Nordrhein-Westfalen, Germany.
Burger, C., Froggatt, A., Mitchell, C., and Weinmann, J.. (2020) Decentralised energy: a global game changer, decentralised energy: a global game changer. London: Ubiquity Press.
Chai, J., Shi, H., Lu, Q., and Hu, Y. (2020). “Quantifying and predicting the water-energy- food-economy-society-environment nexus based on Bayesian networks-a case study of China,” J. Clean. Prod., 256, 120266.
Castellar, J. A. C., Torrens, A., Buttiglieri, G., Monclús, H., Arias, C. A., Carvalho, P. N., et al. (2022). Nature-based solutions coupled with advanced technologies: An opportunity for decentralised water reuse in cities. J. Cleaner Product. 340:130660. doi: 10.1016/j.jclepro.2022.130660
Chamas, Z., Abou Najm, M., al-Hindi, M., Yassine, A., and Khattar, R. (2021). Sustainable resource optimisation under water-energy-food-carbon nexus. J. Cleaner Product. 278:123894. doi: 10.1016/j.jclepro.2020.123894
Chen, H., Koh, J. J., Long, C., Liu, S., Shi, H., Min, J., et al. (2021). Speed-induced extensibility elastomers with good resilience and high toughness. Macromolecules 54, 3358–3365. doi: 10.1021/acs.macromol.1c00175
Chmutina, K., Wiersma, B., Goodier, C. I., and Devine-Wright, P. (2014). Concern or compliance? Drivers of urban decentralised energy initiatives. Sustain. Cities Soc. 10, 122–129. doi: 10.1016/j.scs.2013.07.001
Chowdhury, P., Mukhopadhyay, B. P., and Bera, A. (2022). Hydrochemical assessment of groundwater suitability for irrigation in the north-eastern blocks of Purulia district, India using GIS and AHP techniques. Physics Chem Earth 126:103108. doi: 10.1016/j.pce.2022.103108
Cobb, C. W., and Cobb, J. B. and Center, H. E. (1994) The green national product: a proposed index of sustainable economic welfare
Cole, J., Sharvelle, S., Fourness, D., Grigg, N., Roesner, L., and Haukaas, J. (2018). Centralised and decentralized strategies for dual water supply: case study. J. Water Resour. Plan. Manag. 144:5017017. doi: 10.1061/(ASCE)WR.1943-5452.0000856
Corona-López, E., Román-Gutiérrez, A. D., Otazo-Sánchez, E. M., Guzmán-Ortiz, F. A., and Acevedo-Sandoval, O. A. (2021). Water–Food Nexus Assessment in Agriculture: A Systematic Review. International Journal of Environmental Research and Public Health, 18, 4983. doi: 10.3390/ijerph18094983
Crosson, C., Tong, D., Zhang, Y., and Zhong, Q. (2021). Rainwater as a renewable resource to achieve net zero urban water in water stressed cities. Resour. Conserv. Recycl. 164:105203. doi: 10.1016/j.resconrec.2020.105203
de Strasser, L., Lipponen, A., Howells, M., Stec, S., and Bréthaut, C. (2016). A methodology to assess the water energy food ecosystems Nexus in Transboundary River basins. Water 8, 1–28. doi: 10.3390/w8020059
D'Odorico, P., Davis, K. F., Rosa, L., Carr, J. A., Chiarelli, D., Dell'Angelo, J., et al. (2018). The global food-energy-water nexus. Rev. Geophys. 56, 456–531. doi: 10.1029/2017RG000591
Doke, A. B., Zolekar, R. B., Patel, H., and das, S. (2021). Geospatial mapping of groundwater potential zones using multi-criteria decision-making AHP approach in a hardrock basaltic terrain in India. Ecol. Indicators 127:107685. doi: 10.1016/j.ecolind.2021.107685
Doorga, J. R. S., Rughooputh, S. D. D. V., and Boojhawon, R. (2019). Multi-criteria GIS-based modelling technique for identifying potential solar farm sites: a case study in Mauritius. Renew. Energy 133, 1201–1219. doi: 10.1016/j.renene.2018.08.105
EC (2020) Indicators for the EU’s resource efficiency framework : DIRECTORATE GENERAL FOR INTERNAL POLICIES, European Parliment.
Ecker, F., Hahnel, U. J. J., and Spada, H. (2017). Promoting decentralised sustainable energy systems in different supply scenarios: the role of autarky aspiration. Front. Energy Res. 5. doi: 10.3389/fenrg.2017.00014
Elsoragaby, S., Yahya, A., Mahadi, M. R., Nawi, N. M., and Mairghany, M. (2019). Energy utilization in major crop cultivation. Energy, 173, 1285–1303. doi: 10.1016/j.energy.2019.01.142
Elboshy, B., Alwetaishi, M., Aly, R., and Zalhaf, A. S. (2022). A suitability mapping for the PV solar farms in Egypt based on GIS-AHP to optimise multi-criteria feasibility. Ain Shams Engin. J. 13:101618. doi: 10.1016/j.asej.2021.10.013
Ferdowsi, A., Singh, V. P., Ehteram, M., and Mirjalili, S. (2021). Multi-objective Optimization Approaches for Design, Planning, and Management of Water Resource Systems. in Essential Tools for Water Resources Analysis, Planning, and Management. Ed. O Bozorg-Haddad. Singapore: Springer Water. Springer. doi: 10.1007/978-981-33-4295-8_11
Flammini, Alessandro, Puri, Manas, Pluschke, L., and Dubois, O. (2014) Environment and natural resources management working paper in the context of the sustainable energy for all initiative walking the Nexus talk: assessing the water-energy-food Nexus. 150.
Fouladi, J., AlNouss, A., and Al-Ansari, T. (2021). Sustainable energy-water-food nexus integration and optimisation in eco-industrial parks. Comput. Chem. Engin. 146:107229. doi: 10.1016/j.compchemeng.2021.107229
Ghafourian, M., Nika, C. E., Mousavi, A., Mino, E., al-Salehi, M., and Katsou, E. (2022). Science of the Total environment economic impact assessment indicators of circular economy in a decentralised circular water system — case of eco-touristic facility. Sci. Total Environ. 822:153602. doi: 10.1016/j.scitotenv.2022.153602
Ghosh, D., Mandal, M., Banerjee, M., and Karmakar, M. (2020). Impact of hydro-geological environment on availability of groundwater using analytical hierarchy process (AHP) and geospatial techniques: a study from the upper Kangsabati river basin. Groundwater Sustain. Develop. 11:100419. doi: 10.1016/j.gsd.2020.100419
Giupponi, C. A. (2017). Integrated spatial assessment of the water, energy and food dimensions of the sustainable development goals. Reg. Environ. Chang. 17, 1881–1893. doi: 10.1007/s10113-016-0998-z
Gollwitzer, L., (2014). Community-based Micro grids: a common property resource problem. STEPS working paper 68.
Gómez-Monsalve, M., Domínguez, I. C., Yan, X., Ward, S., and Oviedo-Ocaña, E. R. (2022). Environmental performance of a hybrid rainwater harvesting and greywater reuse system: a case study on a high water consumption household in Colombia. J. Clean. Prod. 345:131125. doi: 10.1016/j.jclepro.2022.131125
Govindan, R., and Al-Ansari, T. (2019). Computational decision framework for enhancing resilience of the energy, water and food nexus in risky environments. Renew. Sust. Energ. Rev. 112, 653–668. doi: 10.1016/j.rser.2019.06.015
Govindan, R., al-Ansari, T., Korre, A., and Shah, N. (2018). Assessment of technology portfolios with enhanced economic and environmental performance for the energy, water and food nexus. Comput. Aided Chem. Engin., 43:537–542. doi: 10.1016/B978-0-444-64235-6.50095-4
Grigg, N. (2015). Infrastructure report card: purpose and results. Infrastruct. Syst. 21. doi: 10.1061/(ASCE)IS.1943-555X.0000186
Haji, M., Govindan, R., and Al-Ansari, T. (2020). Novel approaches for geospatial risk analytics in the energy-water-food Nexus using an EWF Nexus node. Comput. Chem. Eng. 140:106936. doi: 10.1016/j.compchemeng.2020.106936
Haji, M., Govindan, R., and Al-Ansari, T. (2021). Optimising the energy, water and food nexus node to support decision making for sustainable food security in risky environments Elsevier Masson SAS.
Hasik, V., Anderson, N. E., Collinge, W. O., Thiel, C. L., Khanna, V., Wirick, J., et al. (2017). Evaluating the life cycle environmental benefits and trade-offs of water reuse systems for net-zero buildings. Environ. Sci. Technol. 51, 1110–1119. doi: 10.1021/acs.est.6b03879
Herran, D., and Nakata, T. (2012). Design of decentralised energy systems for rural electrification in developing countries considering regional disparity. Appl. Energy 91, 130–145. doi: 10.1016/j.apenergy.2011.09.022
Hidayatullah, N. A., Stojcevski, B., and Kalam, A. (2011). Analysis of distributed generation systems, smart grid technologies and future motivators influencing change in the electricity sector. Smart Grid Renewable Energy 2, 216–229. doi: 10.4236/sgre.2011.23025
Hoffmann, S., Feldmann, U., Bach, P. M., Binz, C., Farrelly, M., Frantzeskaki, N., et al. (2020). A research agenda for the future of urban water management: exploring the potential of nongrid, small-grid, and hybrid solutions. Environ. Sci. Technol. 54, 5312–5322. doi: 10.1021/acs.est.9b05222
Hogeboom, R. J., Borsje, B., Deribe, M., van der Meer, F., Mehvar, S., Meyer, M., et al. (2021). Resilience meets the water–energy–food nexus: mapping the research landscape. Front. Environ. Sci. 38:1–18. doi: 10.3389/fenvs.2021.630395
Hoyos, D., Bermejo, R., and Arto, I. (2010) Sustainable development in the Brundtland report and its distortion: implications for development economics and international cooperation, 13–34. doi: 10.978-1-935709-02-2
Ingram, J. (2011). A food systems approach to researching food security and its interactions with global environmental change. Food Secur. 3, 417–431. doi: 10.1007/s12571-011-0149-9
Javid, I., Chauhan, A., Thappa, S., Verma, S. K., Anand, Y., Sawhney, A., et al. (2021). Futuristic decentralised clean energy networks in view of inclusive-economic growth and sustainable society. J. Cleaner Product. 309:127304. doi: 10.1016/j.jclepro.2021.127304
Kaundinya, D. P., Balachandra, P., and Ravindranath, N. H. (2009). Grid-connected versus stand-alone energy systems for decentralized power—a review of literature. Renew. Sust. Energ. Rev. 13, 2041–2050. doi: 10.1016/j.rser.2009.02.002
Kaur, L., Rishi, M. S., Singh, G., and Nath Thakur, S. (2020). Groundwater potential assessment of an alluvial aquifer in Yamuna sub-basin (Panipat region) using remote sensing and GIS techniques in conjunction with analytical hierarchy process (AHP) and catastrophe theory (CT). Ecol. Indicat. 110:105850. doi: 10.1016/j.ecolind.2019.105850
Kaya, I., Çolak, M., and Terzi, F. (2019). A comprehensive review of fuzzy multi criteria decision making methodologies for energy policy making. Energy Strateg Rev. 24, 207–228. doi: 10.1016/j.esr.2019.03.003
Khalkhali, M., Dilkina, B., and Mo, W. (2021). The role of climate change and decentralization in urban water services: a dynamic energy-water nexus analysis. Water Res. 207:117830. doi: 10.1016/j.watres.2021.117830
Khalkhali, M., and Mo, W. (2020). The energy implication of climate change on urban wastewater systems. J. Clean. Prod. 267:121905. doi: 10.1016/j.jclepro.2020.121905
Khalkhali, M., Westphal, K., and Mo, W. (2018). The water-energy nexus at water supply and its implications on the integrated water and energy management. Sci. Total Environ. 636, 1257–1267. doi: 10.1016/j.scitotenv.2018.04.408
Kishore, T. S., Patro, E. R., Harish, V. S. K. V., and Haghighi, A. T. (2021). A comprehensive study on the recent progress and trends in development of small hydropower projects. Energies 14, 1–31. doi: 10.3390/en14102882
Koenig, A., Jakobs, S., and Brutschin, K. (2018). Decentralization and energy system transformation: towards a bottom-up approach. Energy Res. Soc. Sci. 38, 96–105.
Konuk, F., Zeren, F., Akpınar, S., and Yıldız, Ş. (2021). Biomass energy consumption and economic growth: further evidence from NEXT-11 countries. Energy Rep. 7, 4825–4832. doi: 10.1016/j.egyr.2021.07.070
Kruyt, B. V. (2009). Indicators for energy security. Energy Policy 37, 2166–2181. doi: 10.1016/j.enpol.2009.02.006
Lahlou, F. zahra Mackey, H. R., McKay, G., Onwusogh, U., and al-Ansari, T. (2020) Water planning framework for alfalfa fields using treated wastewater fertigation in Qatar: an energy-water-food nexus approach, Comput. Chem. Eng. doi: 10.1016/j.compchemeng.2020.106999, 141::106999.
Larsen, T. A., Udert, K. M., and Lienert, J. (2013). Source separation and decentralization for wastewater management. Water Intelligence Online 12. doi: 10.2166/9781780401072
Lauri, P., Havlík, P., Kindermann, G., Forsell, N., Böttcher, H., and Obersteiner, M. (2014). Woody biomass energy potential in 2050. Energy Policy 66, 19–31. doi: 10.1016/j.enpol.2013.11.033
Leck, H., Conway, D., Bradshaw, M., and Rees, J. (2015). Tracing the water-energy-food Nexus: description, theory and practice. Geogr. Compass 9, 445–460. doi: 10.1111/gec3.12222
Leivas, R., Laso, J., Abejón, R., Margallo, M., and Aldaco, R. (2020). Environmental assessment of food and beverage under a NEXUS water-energy-climate approach: application to the spirit drinks. Sci. Total Environ 720:137576. doi: 10.1016/j.scitotenv.2020.137576
Li, Y., Khalkhali, M., Mo, W., and Lu, Z. (2021). Modeling spatial diffusion of decentralised water technologies and impacts on the urban water systems. J. Cleaner Product. 315:128169. doi: 10.1016/j.jclepro.2021.128169
Li, X., Yang, L., Xu, K., Bei, K., Zheng, X., Lu, S., et al. (2021). Application of constructed wetlands in treating rural sewage from source separation with high-influent nitrogen load: a review. World J. Microbiol. Biotechnol. 37:138. doi: 10.1007/s11274-021-03105-3
Liang, Y., Ju, Y., Qin, J., and Pedrycz, W. (2021). Multi-granular linguistic distribution evidential reasoning method for renewable energy project risk assessment. Inf Fusion 65, 147–164. doi: 10.1016/j.inffus.2020.08.010
Liu, D. (2019). Evaluating the dynamic resilience process of a regional water resource system through the nexus approach and resilience routing analysis. J. Hydrol. 578:124028. doi: 10.1016/j.jhydrol.2019.124028
Lott, C., Tchigriaeva, E., and Rollins, K. (2013). The effects of climate change on residential municipal water demand in Nevada. Nevada, USA: University of Nevada.
Lu, Z., Mo, W., Dilkina, B., Gardner, K., Stang, S., Huang, J. C., et al. (2019). Decentralised water collection systems for households and communities: household preferences in Atlanta and Boston. Water Res. 167:115134. doi: 10.1016/j.watres.2019.115134
Lu, Z., Noonan, D., Crittenden, J., Jeong, H., and Wang, D. (2013). Use of impact fees to incentivize low-impact development and promote compact growth. Environ. Sci. Technol. 47, 10744–10752. doi: 10.1021/es304924w
Ma, Y., Li, Y. P., Zhang, Y. F., and Huang, G. H. (2021). Mathematical modeling for planning water-food-ecology-energy nexus system under uncertainty: a case study of the Aral Sea basin. J. Clean. Prod. 308:127368. doi: 10.1016/j.jclepro.2021.127368
Madani, K., Darch, G., Parra, F., and Workman, M.. (2015) Using game theory to address modern resource management problems. :Grantham Institute, Imperial College London.
Mahlknecht, J., González-Bravo, J., and Loge, F. J. (2020). “Water-energy-food security: a nexus perspective of the current situation in Latin America and the Caribbean.,” Energy, 194, 116824.
Martin-Gorriz, B. S. G. A. (2014). Energy and greenhouse-gas emissions in irrigated agriculture of SE (southeast) Spain. Effects of alternative water supply scenarios. Energy 77, 478–488. doi: 10.1016/j.energy.2014.09.031
McGrane, S. A. (2019). Scaling the nexus: towards integrated frameworks for analysing water, energy and food. Geogr. J. 185, 419–431. doi: 10.1111/geoj.12256
Mekonnen, M. M., and Hoekstra, A. Y. (2021). National water footprint accounts: the green, blue and grey water footprint of production and consumption. Netherlands: Routledge.
Memarzadeh, M., Moura, S., and Horvath, A. (2020). Multi-agent management of integrated food-energy-water systems using stochastic games: from Nash equilibrium to the social optimum. Environ. Res. Lett. 15. doi: 10.1088/1748-9326/abadca
Mguni, P., and Van Vliet, B. J. (2020). Rethinking the urban nexus-resilience and vulnerability at the urban nexus of water, energy and food (WEF). An introduction to the special issue. J. Integr. Environ. Sci. 17, i–v. doi: 10.1080/1943815X.2020.1866617
Mo, W., Wang, H., and Jacobs, J. M. (2016). Understanding the influence of climate change on the embodied energy of water supply. Water Res. 95, 220–229. doi: 10.1016/j.watres.2016.03.022
Moglen, R. L., Barth, J., Gupta, S., Kawai, E., Klise, K., and Leibowicz, B. D. (2023). A nexus approach to infrastructure resilience planning under uncertainty. Reliabil. Engin. Syst. Safety 230:108931. doi: 10.1016/j.ress.2022.108931
Mohtar, R. H. (2017). A call for a new business model valuing water use and production: the water, energy and food nexus holistic system approach. Water Int. 42, 773–776. doi: 10.1080/02508060.2017.1353238
Moslehi, S., and Reddy, T. A. (2018). Sustainability of integrated energy systems: a performance-based resilience assessment methodology. Appl Energy 228, 487–498. doi: 10.1016/j.apenergy.2018.06.075
Nadeem, T. B., Siddiqui, M., Khalid, M., and Asif, M. (2023). Distributed energy systems: a review of classification, technologies, applications, and policies. Energ. Strat. Rev. 48:101096. doi: 10.1016/j.esr.2023.101096
Nair, S., George, B., Malano, H. M., Arora, M., and Nawarathna, B. (2014). Water–energy–greenhouse gas nexus of urban water systems: review of concepts, state-of-art and methods. Resour. Conserv. Recycl. 89, 1–10. doi: 10.1016/j.resconrec.2014.05.007
Namany, S., Al-Ansari, T., and Govindan, R. (2018). Integrated techno-economic optimisation for the design and operations of energy, water and food nexus systems constrained as non-cooperative games. Computer Aided Chem. Engin. doi: 10.1016/B978-0-444-64241-7.50162-2
Namany, S., Al-Ansari, T., and Govindan, R. (2019a). Optimisation of the energy, water, and food nexus for food security scenarios. Comput. Chem. Engin. 129:106513. doi: 10.1016/J.COMPCHEMENG.2019.106513
Namany, S., Al-Ansari, T., and Govindan, R. (2019b). Sustainable energy, water and food nexus systems: a focused review of decision-making tools for efficient resource management and governance. J. Clean. Prod. 225, 610–626. doi: 10.1016/j.jclepro.2019.03.304
Namany, S., AlNouss, A., Govindan, R., Mckay, G., and al-Ansari, T. (2020a). An agent-based model for sustainable power generation using optimal biomass utilisation. Comput. Aided Chem. Engin. doi: 10.1016/B978-0-12-823377-1.50309-8
Namany, S., Govindan, R., and Al-Ansari, T. (2023). Operationalising transboundary cooperation through game theory: an energy water food nexus approach for the Middle East and North Africa. Futures, 152, 103198. doi: 10.1016/j.futures.2023.103198
Namany, S., Govindan, R., Alfagih, L., McKay, G., and al-Ansari, T. (2020b). Sustainable food security decision-making: An agent-based modelling approach. J. Cleaner Product. 255:120296. doi: 10.1016/J.JCLEPRO.2020.120296
Namany, S., Govindan, R., Martino, M. D., Pistikopoulos, E. N., Linke, P., Avraamidou, S., et al. (2021). An energy-water-food Nexus-based decision-making framework to guide National Priorities in Qatar. Sustain. Cities Soc. 75:103342. doi: 10.1016/J.SCS.2021.103342
Nie, Y., Avraamidou, S., Xiao, X., Pistikopoulos, E. N., Li, J., Zeng, Y., et al. (2018). A food-energy-water Nexus approach for land use optimization. Sci. Total Environ. 659, 7–19. doi: 10.1016/j.scitotenv.2018.12.242
Núñez-López, J. M., Rubio-Castro, E., and Ponce-Ortega, J. M. (2021). Involving resilience in optimizing the water-energy-food nexus at macroscopic level. Process Saf. Environ. Prot. 147, 259–273. doi: 10.1016/j.psep.2020.09.037
Núñez-López, J. M., Rubio-Castro, E., and Ponce-Ortega, J. M. (2022). Optimizing resilience at water-energy-food nexus. Comput. Chem. Eng. 160:107710. doi: 10.1016/j.compchemeng.2022.107710
Okonkwo, E. C., Namany, S., Fouladi, J., Almanassra, I. W., Mahmood, F., and al-Ansari, T. (2023). A multi-level approach to the energy-water-food nexus: from molecule to governance. Cleaner Environ. Syst. 8:100110. doi: 10.1016/J.CESYS.2023.100110
Organisation for Economic Cooperation and Development (2000) Towards sustainable development. Indicators to measure progress: Paris.
Orhan, O. (2021). Land suitability determination for citrus cultivation using a GIS-based multi-criteria analysis in Mersin, Turkey. Comput. Electron. Agric. 190:106433. doi: 10.1016/j.compag.2021.106433
Perera, A., Attalage, R. A., Perera, K. K. C. K., and Dassanayake, V. P. C. (2013). A hybrid tool to combine multi-objective optimization and multi-criterion decision making in designing standalone hybrid energy systems. Appl. Energy 107, 412–425. doi: 10.1016/j.apenergy.2013.02.049
Perez, I. U., and dos Santos, A. (2017). Distributed economies through open design and digital manufacturing, 3, 261–267. doi: 10.5151/sbds-issd-2017-042
Pinheiro Neto, D., Domingues, E. G., Coimbra, A. P., de Almeida, A. T., Alves, A. J., and Calixto, W. P. (2017). Portfolio optimisation of renewable energy assets: hydro, wind, and photovoltaic energy in the regulated market in Brazil. Energy Econ. 64, 238–250. doi: 10.1016/j.eneco.2017.03.020
Polebitski, A. S., Palmer, R. N., and Waddell, P. (2011). Evaluating water demands under climate change and transitions in the urban environment. J. Water Resour. Plan. Manag. 137, 249–257. doi: 10.1061/(ASCE)WR.1943-5452.0000112
Prpich, G., Darabkhani, H. G., Oakey, J., and Pollard, S. (2014) An investigation into future energy system risks: an industry perspective, 68. https://ukerc.rl.ac.uk/UCAT/PUBLICATIONS/An_Investigation_into_Future_Energy_System_Risks_an_industry_perspective.pdf
Rabaey, K., Vandekerckhove, T., de Walle, A. V., and Sedlak, D. L. (2020). The third route: using extreme decentralization to create resilient urban water systems. Water Res. 185, 1162–1176. doi: 10.1016/j.watres.2020.116276
Ren, L., Liu, X., and Chang, C. (2021). A review of water scarcity index methodologies for the water-energy-food nexus. J. Clean. Prod. 314:128001
Ren, N., Wang, Q., Wang, Q., Huang, H., and Wang, X. (2017). Upgrading to urban water system 3.0 through sponge city construction. Front. Environ. Sci. Eng. 11. doi: 10.1007/s11783-017-0960-4
Retamal, M., and Turner, A. (2010). Unpacking the energy implications of distributed water infrastructure: how are rainwater systems performing? Water Sci. Technol. Water Supply 10, 546–553. doi: 10.2166/ws.2010.136
Rizzo, L., Gernjak, W., Krzeminski, P., Malato, S., McArdell, C. S., Sanchez Perez, J. A., et al. (2020). Best available technologies and treatment trains to address current challenges in urban wastewater reuse for irrigation of crops in EU countries. Sci. Total Environ. 710, 136–312. doi: 10.1016/j.scitotenv.2019.136312
Rodrigues, A., Formiga, K., and Milograna, J. (2023). Integrated systems for rainwater harvesting and greywater reuse: a systematic review of urban water management strategies. Water Supply. 23. doi: 10.2166/ws.2023.240
Rosegrant, M. A. (2003). Global food security: challenges and policies. Science 302, 1917–1919. doi: 10.1126/science.1092958
Rubio, J. L., and Bochet, E. (1998). Desertification indicators as diagnosis criteria for desertification risk assessment in Europe. J. Arid Environ. 39, 113–120. doi: 10.1006/jare.1998.0402
Saaty, T. L. (1988). What is the analytic hierarchy process? Math Models Decision Support. doi: 10.1007/978-3-642-83555-1_5
Sachs, I., and Silk, D. (1990) Food and energy: strategies for sustainable development United Nations University: Tokyo.
Saladini, F. B. (2018). Linking the water-energy-food nexus and sustainable development indicators for the Mediterranean region. Ecol. Indic. 91, 689–697. doi: 10.1016/j.ecolind.2018.04.035
Sánchez, A., Zhang, Q., Martín, M., and Vega, P. (2022). Towards a new renewable power system using energy storage: an economic and social analysis. Energy Convers. Manag. 252:115056. doi: 10.1016/j.enconman.2021.115056
Sánchez-Zarco, X. G., González-Bravo, R., and Ponce-Ortega, J. M. (2021). Multi-objective optimisation approach to meet water, energy, and food needs in an arid region involving security assessment. ACS Sustain. Chem. Eng. 9, 4771–4790. doi: 10.1021/acssuschemeng.0c09322
Schlör, H., and Venghaus, S. (2022). Measuring resilience in the food-energy-water nexus based on ethical values and trade relations. Appl. Energy 323:119447. doi: 10.1016/j.apenergy.2022.119447
Schlör, H., Venghaus, S., and Hake, J. F. (2018). The FEW-Nexus city index – measuring urban resilience. Appl. Energy 210, 382–392. doi: 10.1016/j.apenergy.2017.02.026
Schull, V. Z., Daher, B., Gitau, M. W., Mehan, S., and Flanagan, D. C. (2020). Analyzing FEW nexus modeling tools for water resources decision-making and management applications. Food Bioproducts Processing 119, 108–124. doi: 10.1016/j.fbp.2019.10.011
Scott, M. A. (2019). Geography and the water–energy–food nexus: introduction. Geogr. J. 185, 373–376. doi: 10.1111/geoj.12331
Sharma, A., Burn, S., Gardner, T., and Gregory, A. (2010). Role of decentralised systems in the transition of urban water systems. Water Sci. Technol. Water Supply 10, 577–583. doi: 10.2166/ws.2010.187
Shen, Q., Niu, J., Liu, Q., Liao, D., and du, T. (2022). A resilience-based approach for water resources management over a typical agricultural region in Northwest China under water-energy-food nexus. Ecol. Indicators 144:109562. doi: 10.1016/j.ecolind.2022.109562
Shu, Q., Scott, M., Todman, L., and McGrane, S. D.. (2021). Development of a prototype composite index for resilience and security of water-energy-food (WEF) systems in industrialised nations. Environ. Sustain. Indicators 11:100124. doi: 10.1016/j.indic.2021.100124
Shweta, S., Surya Prakash, U., and Satvasheel, P. (2022). Developing an integrated social, economic, environmental, and technical analysis model for sustainable development using hybrid multi-criteria decision making methods. Appl. Energy. 308:118235. doi: 10.1016/j.apenergy.2021.118235
Singh, J. (2017). Management of the agricultural biomass on decentralised basis for producing sustainable power in India. J. Cleaner Product 142, 3985–4000. doi: 10.1016/j.jclepro.2016.10.056
Singh, R. K., Joshi, P. K., Sinha, V. S. P., and Kumar, M. (2022). Indicator based assessment of food security in SAARC nations under the influence of climate change scenarios. Future Foods 5:100122. doi: 10.1016/j.fufo.2022.100122
SIWI (2018) Stockholm international water institute world water week. https://siwi.org/publications/world-water-week-programme-2018/
Soroudi, A., and Amraee, T. (2013). Decision making under uncertainty in energy systems: state of the art. Renew. Sust. Energ. Rev. 28, 376–384. doi: 10.1016/j.rser.2013.08.039
Stang, S., Khalkhali, M., Petrik, M., Palace, M., Lu, Z., and Mo, W. (2021). Spatially optimized distribution of household rainwater harvesting and greywater recycling systems. J. Clean. Prod. 312:127736. doi: 10.1016/j.jclepro.2021.127736
Tadić, S., Zečević, S., and Krstić, M. (2014). A novel hybrid MCDM model based on fuzzy DEMATEL, fuzzy ANP and fuzzy VIKOR for city logistics concept selection. Expert Syst. Appl. 41, 8112–8128. doi: 10.1016/j.eswa.2014.07.021
Teston, A., Piccinini Scolaro, T., Kuntz Maykot, J., and Ghisi, E. (2022). Comprehensive environmental assessment of rainwater harvesting systems: a literature review. Water, 14, 2716. doi: 10.3390/w14172716
Turkson, J., and Wohlgemuth, N. (2001). Power sector reform and distributed generation in sub-Saharan Africa. Energy Policy 29, 135–145. doi: 10.1016/S0301-4215(00)00112-9
UN (2019) United nations water, food and energy. https://www.unwater.org/water-facts/water-food-and-energy (Accessed Retrieved on December 2, 2023).
United Nations (2007). Indicators of sustainable development: guidelines and methodologies. N. Y. 2, 136–143. doi: 10.1016/j.cirpj.2010.03.002
Unlocking the Potential of Distributed Energy Resources (2022). Unlocking the potential of distributed energy resources. doi: 10.1787/a2ed7a25-en,
Valek, A. M., Suˇsnik, J., and Grafakos, S. (2017). Quantification of the urban water-energy nexus in Mexico City, Mexico, with an assessment of water-system related carbon emissions. Sci. Total Environ. 590-591, 258–268. doi: 10.1016/j.scitotenv.2017.02.234
Valencia, A., Zhang, W., Gu, L., Chang, N. B., and Wanielista, M. P. (2022). Synergies of green building retrofit strategies for improving sustainability and resilience via a building-scale food-energy-water nexus. Resourc Conserv. Recycl. 176:105939. doi: 10.1016/j.resconrec.2021.105939
Venghaus, S., and Dieken, S. (2019). From a few security indices to the FEW security index: consistency in global food, energy and water security assessment. Sustain. Product. Consum. 20, 342–355. doi: 10.1016/j.spc.2019.08.002
Vezzoli, C., Ceschin, F., Osanjo, L., and Kanampiu M’Rithaa, M.. (2018). “Distributed/decentralised renewable energy systems” in Designing sustainable energy for all (Springer), 23–39.
Visser, W., and Brundtland, G. H. (2013). Our common future (‘the Brundtland report’): world commission on environment and development, the top 50 sustainability books. doi: 10.9774/gleaf.978-1-907643-44-6_12,
Walker, B. H. (2004). Resilience, adaptability and transformability in social–ecological systems. Ecol. Soc. 9. doi: 10.5751/ES-00650-090205
Walker, B., Carpenter, S. R., Anderies, J. M., Abel, N., Cumming, G., Janssen, M. A., et al. (2002). Resilience management in social-ecological systems: a working hypothesis for a participatory approach. Conserv. Ecol. 6. doi: 10.5751/ES-00356-060114
Wang, J., Ge, J., and Gao, Z. (2018) Consumers’ preferences and derived willingness-to-pay for water supply safety improvement: The analysis of pricing and incentive strategies, Sustain
Wang, W., Mwiathi, N. F., Li, C., Luo, W., Zhang, X., An, Y., et al. (2022). Assessment of shallow aquifer vulnerability to fluoride contamination using modified AHP-DRASTICH model as a tool for effective groundwater management, a case study in Yuncheng Basin, China. Chemosphere 286:131601. doi: 10.1016/j.chemosphere.2021.131601
Wang, Q., Yi, L., and Yangfan, L. (2021). Realizing a new resilience paradigm on the basis of land-water-biodiversity nexus in a coastal city. Ocean Coast. Manag. 207:104603. doi: 10.1016/j.ocecoaman.2018.09.004
Wicaksono, A., Jeong, G., and Kang, D. (2019). Water-energy-food nexus simulation: An optimisation approach for resource security. Water (Switzerland) 11. doi: 10.3390/w11040667
Wohlgemuth, N., and Madlener, R., (2010). Financial support of renewable energy system: investment vs operating cost subsidies. In Proceedings of the Norwegian Association for Energy Economics (NAEE) conference “towards an integrated European energy market”, Bergen/Norway
Woldesellasse, H., Govindan, R., and Al-Ansari, T. (2018). Role of analytics within the energy, water and food nexus – An alfalfa case study. San Diego, USA Elsevier Masson SAS.
World Bank (2021) World development indicators 2021. https://databank.worldbank.org/source/world-development-indicators (Accessed January 12, 2024).
WRI (2021) Aqueduct global maps 3.0. https://www.wri.org/data/aqueduct-global-maps-30-data (Accessed January 12, 2024).
WWF8 (2018) WWF8 8th world water forum: water-food Nexus high level panel, World Water Council. Brazil.
Yu, L., Xiao, Y., Zeng, X. T., Li, Y. P., and Fan, Y. R. (2020). Planning water-energy-food nexus system management under multi-level and uncertainty. J. Clean. Prod. 251:119658. doi: 10.1016/j.jclepro.2019.119658
Yuan, K.-Y., Lin, Y. C., Chiueh, P. T., and Lo, S. L. (2018). Spatial optimisation of the food, energy, and water nexus: a life cycle assessment-based approach. Energy Policy 119, 502–514. doi: 10.1016/j.enpol.2018.05.009
Yue, Q., and Guo, P. (2021). Managing agricultural water-energy-food-environment nexus considering water footprint and carbon footprint under uncertainty. Agric. Water Manag. 252:106899. doi: 10.1016/j.agwat.2021.106899
Zhang, F., Guo, S., Liu, X., Wang, Y., Engel, B. A., and Guo, P. (2020). Towards sustainable water management in an arid agricultural region: a multi-level multi-objective stochastic approach. Agric. Syst. 182:102848. doi: 10.1016/j.agsy.2020.102848
Zhang, T., Tan, Q., Wang, S., Zhang, T., Hu, K., and Zhang, S. (2021). Assessment and management of composite risk in irrigated agriculture under water-food-energy nexus and uncertainty. Agric. Water Manag. 262:107322. doi: 10.1016/j.agwat.2021.107322
Zhang, T., Tan, Q., Yu, X., and Zhang, S. (2020). Synergy assessment and optimisation for water-energy-food nexus: modeling and application. Renewable Sustain. Energy Reviews 134:110059. doi: 10.1016/j.rser.2020.110059
Zhang, W., Valencia, A., Gu, L., Zheng, Q. P., and Chang, N. B. (2020). Integrating emerging and existing renewable energy technologies into a community-scale microgrid in an energy-water nexus for resilience improvement. Appl. Energy 279:115716. doi: 10.1016/j.apenergy.2020.115716
Zhang, S., Zhang, J., Yue, T., and Jing, X. (2019). Impacts of climate change on urban rainwater harvesting systems. Sci. Total Environ. 665, 262–274. doi: 10.1016/j.scitotenv.2019.02.135
Zhu, Y., Zhang, C., Fang, J., and Miao, Y. (2022). Paths and strategies for a resilient megacity based on the water-energy-food nexus. Sustain. Cities Soc. 82:103892. doi: 10.1016/j.scs.2022.103892
Zhu, M., Yang, G., Jiang, Y., and Wang, X. (2023). "Agent-Based Modeling for Water–Energy–Food Nexus and Its Application in Ningdong Energy and Chemical Base", Sustainability, MDPI, 15, 1–25.
Zoungrana, A., and Çakmakci, M. (2021). From non-renewable energy to renewable by harvesting salinity gradient power by reverse electrodialysis: a review. Int. J. Energy Res., 45:3495–3522. doi: 10.1002/er.6062
Keywords: resilience, decentralization, EWF nexus, decision-making, multi criteria decision making
Citation: Haji M, Namany S and Al-Ansari T (2024) Strengthening resilience: decentralized decision-making and multi-criteria analysis in the energy-water-food nexus systems. Front. Sustain. 5:1367931. doi: 10.3389/frsus.2024.1367931
Edited by:
Pau Chung Leng, University of Technology Malaysia, MalaysiaReviewed by:
Heriberto Cabezas, Széchenyi István University, HungaryShah Md Atiqul Haq, Shahjalal University of Science and Technology, Bangladesh
Copyright © 2024 Haji, Namany and Al-Ansari. This is an open-access article distributed under the terms of the Creative Commons Attribution License (CC BY). The use, distribution or reproduction in other forums is permitted, provided the original author(s) and the copyright owner(s) are credited and that the original publication in this journal is cited, in accordance with accepted academic practice. No use, distribution or reproduction is permitted which does not comply with these terms.
*Correspondence: Tareq Al-Ansari, dGFsYW5zYXJpQGhia3UuZWR1LnFh