- 1Department of Civil Engineering, School of Engineering, University of Birmingham, Edgbaston, Birmingham, United Kingdom
- 2Kenule Beeson Saro-Wiwa Polytechnic, Bori, Nigeria
Municipal solid waste constitutes environmental challenges globally, especially in developing countries, due to increasing waste generation, population growth, inadequate infrastructure, lack of data and poor planning. This study aims to conduct a comprehensive waste audit on the municipal solid waste generated in Aba, a metropolis in southeastern Nigeria. Aba is a commercial city considered the messiest because of the massive municipal solid waste generation and poor management. The study investigated the energy potential and waste regeneration. Municipal solid waste data was sought to provide insight into the quantity and composition of municipal solid waste. The methodology was site-based, in line with the standard test method for determining unprocessed municipal solid waste (ASTM-D5231-92) and SowaCLINK software, a computer-based environmental application, was used for characterization. Linear extrapolation was adopted to quantify the rate of municipal solid waste generated. The geometric mean was applied to forecast the area’s population for a 10-year design period. The chemical elements of the characterized municipal solid waste were utilized based on the ASTM-D5291 standard for municipal solid waste thermochemical conversion, and the high and low heating values were analyzed. The outcomes provided energy recovery potential, the electrical power potential, and the power to the grid of electrical power of the municipal solid waste. The results obtained were 0.7813 kg/p/d and 490,268 t/y for a population of 1,719,185 persons. The percentage of the municipal solid waste components with energy potential was 71%, comprising 48% combustible and 23% organic components on average. The high heating value computed was 176.5 MJ/kg, and the low heating value was 14 MJ/kg. The energy recovery potential was 3,709,463 MWh, the electrical power potential was 38,680 MW, and the power to the grid was 26.1 MW daily. The research reveals a promising direction in transitioning from the linear economy of municipal solid waste management toward implementing an integrated sustainable municipal solid waste management based on the circular economy model. The study recommends adopting detailed steps to proffer solutions to the environmental challenges associated with municipal solid waste in most low-middle-income countries to achieve sustainable municipal solid waste management while generating electricity and bio-fertilizers through incineration and anaerobic digestion.
1 Introduction
Identifying a suitable technological approach to achieving a well-planned infrastructure for sustainable municipal solid waste management (MSWM) requires quantifying and stratifying the waste stream (Orhorhoro et al., 2017; Seshie, 2020). Factors such as population growth, culture, socioeconomic status, increase in industrialization, commercial activities, natural resource extraction, waste mismanagement and lack of MSWM facilities have led to the global rise in the rate of MSW generation (Guerrero et al., 2013; Abubakar et al., 2022). This is evident in the increase in the rate of MSW generation from 1.3 billion tons as of 2012 to 2.1 billion tons at 0.74 kg/person/day (k/p/d) in 2016. Predictions have estimated a further increase of 70%, which will result in 3.40 billion tons of MSW generation in 2050 at 1.42 kg/p/d. This increase is calculated to affect the developed countries at only 19% (Kaza et al., 2018). Based on the above, given the high volume of municipal solid waste (MSW) generated in developing countries, poor MSWM practice, and the lack of MSWM facilities, it will be promising to identify appropriate techniques for MSWM based on the circular economy (CE) model for the utilization of the abundant waste as diagrammatically presented in Figure 1.
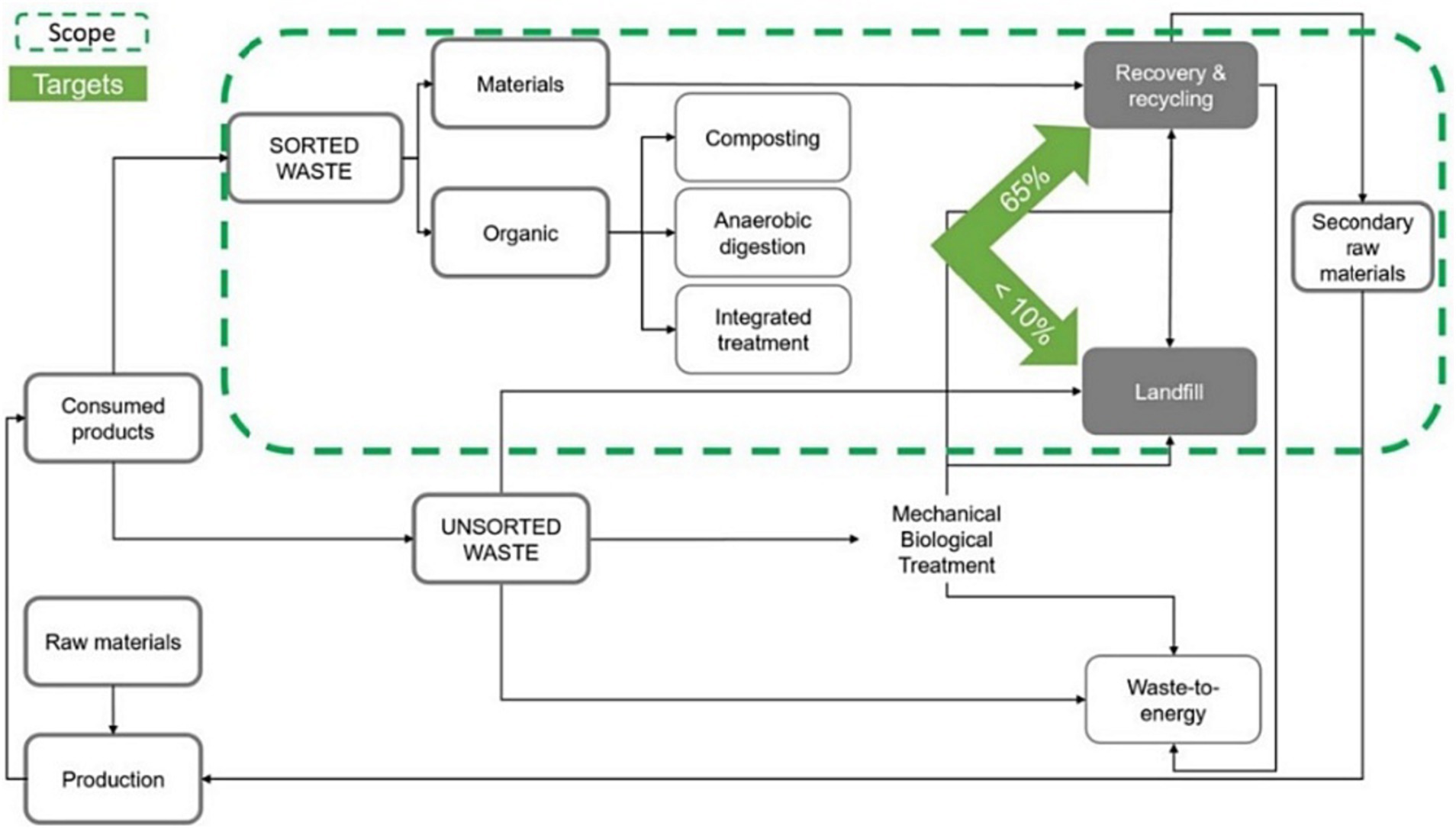
Figure 1. Circular economy roadmap within the MSWM chain (Di Foggia and Beccarello, 2023).
MSW comprises domestic, small commercial, institutional and construction discarded materials that fall within the jurisdiction of the municipalities or the local government to collect, process, and dispose of Letcher and Vallero (2019) and Hemidat et al. (2022). The large volume of MSW in the various components indicates the availability of raw material in transitioning to circularity in MSWM (Salguero-Puerta et al., 2019; Lara-Topete et al., 2022). This justifies the selection of the study area beyond the assertion that it is the messiest commercial city in Nigeria (Odoemene and Ofodu, 2016), with a lack of proper planning and the absence of novel infrastructure for effective MSWM (Nwankpa and Scandrett, 2020; Iyamu et al., 2022). However, this research intends to consider the voluminous MSW within the study area as a resource upon which waste regeneration solutions can be proffered. This will be achieved after a comprehensive MSW characterization, which is a primary step in identifying the components of MSW predominant within a municipality upon which the planning, implementation and sustainable MSWM can be achieved (Smyth et al., 2010; Miezah et al., 2015).
Because a comprehensive knowledge of the MSW composition enables effective, economical, and environmentally friendly MSWM systems for efficient material recovery (Faisal et al., 2019; Triassi et al., 2023), this study aims to develop a structured approach for MSW quantification. It also seeks to determine the composition of the MSW, identify current MSWM practices, ascertain the energy contents of the MSW generated in the Aba metropolis, and propose sustainable MSWM techniques based on circularity.
This research intends to view the MSW available within the study as a raw material for CE transition toward integrated sustainable MSWM implementation. This research sought to provide implementable solutions for managing MSW components in megacities, the case of Aba metropolis. The research objectives (ROs) to which these aims will be actualized are:
RO1—To quantify the rate of MSW generated in megacities.
RO2—To conduct MSW audits using SowaClink software to determine the waste stream and percentage composition of the MSW components and analyze the energy contents of characterized MSW.
RO3—To recommend MSWM techniques in line with the CE model based on the RO1 and RO2.
This research methodology can be adapted to other cities with demography, like the study area. The combination of computer-based applications and manual analytical processes validates the data and procedures adopted while justifying the quality of the research.
This study is limited to MSW quantification, stratification, and characterization to determine the composition of MSW components. The characterized MSW’s biological and chemical energy content was analyzed, and recommendations were proposed for regenerating the waste stream. Notably, the MSW contamination audit to assess the effect of leachate on underground water, soil, and air pollution within the environment was not the primary focus of this current research.
1.1 Municipal solid waste generation
Municipal solid waste generation is unavoidable as human endeavors from domestic, industrial, commercial, agricultural, and educational activities continue (Owamah et al., 2017; Debrah et al., 2021). Waste generation has been a significant concern since the advent of industrialization and technological revolution with an impact on the environment ecologically and socioeconomically as the various components of waste keep increasing in most of the municipalities within low-income countries, especially (Ferronato and Torretta, 2019; Otumawu-Apreku, 2020).
Despite the rate at which waste is generated, MSWM infrastructure, policy framework, and MSW data are lacking in Nigeria (Amasuomo and Baird, 2017; Onungwe et al., 2023) and other developing countries (Fernando and Zutshi, 2023). However, Nigeria has an estimated average rate of 0.51 kg/c/d of MSW generation (Kaza et al., 2018). Abuja is calculated to generate 0.63 kg/p/d (Dickson et al., 2023), Lagos 0.63 kg/p/d (Nnaji, 2015), Ilorin 0.78 kg/p/d (Ibikunle et al., 2019), Port Harcourt 0.60 kg/p/d, Onitsha 0.53 kg/p/d, and Kano 0.56 kg/p/d (Orhorhoro et al., 2017). MSW data is challenging to obtain or access due to limited or lack of open data sources (Ezechi et al., 2017; Ezeudu et al., 2021), like in the case of Aba metropolis, which necessitated this study.
MSW generated in some selected countries in 2018 includes 2.58, 2.33, 2.23, 2.11, 2.0, 1.92, 1.79, 1.71, 1.3, 1.24, 2.4, 1.03, 1.02, 0.93, and 0.34 kg/p/d for the United States of America, Canada, Australia, Germany, South Africa, France, United Kingdom, Japan, Saudi Arabia, Mexico, South Korea, Brazil, China, Russia, and India respectively (Beka and Meng, 2021). This research intends to close the gap due to the absence of MSW data in Nigeria, particularly in the Aba metropolis.
1.2 Composition
Practical knowledge of the composition of MSW generated within a city enhances appropriate and sustainable MSWM (Miezah et al., 2015; Salami et al., 2018). Several variables, including the residents, operational activities, income level, and environmental management expertise, are needed to ascertain the composition of MSW (Miezah et al., 2015). MSW is classified as either biodegradable or non-biodegradable (Babatunde et al., 2013; Yakah et al., 2023). More than half of the volume of MSW generated in Nigeria is composed of biodegradable components; the remaining ingredients are distributed among polythene, plastic, paper, tin/can and other elements constituting the non-biodegradable waste (Oyelola et al., 2017; Ugwu et al., 2020), as shown in Figure 2.
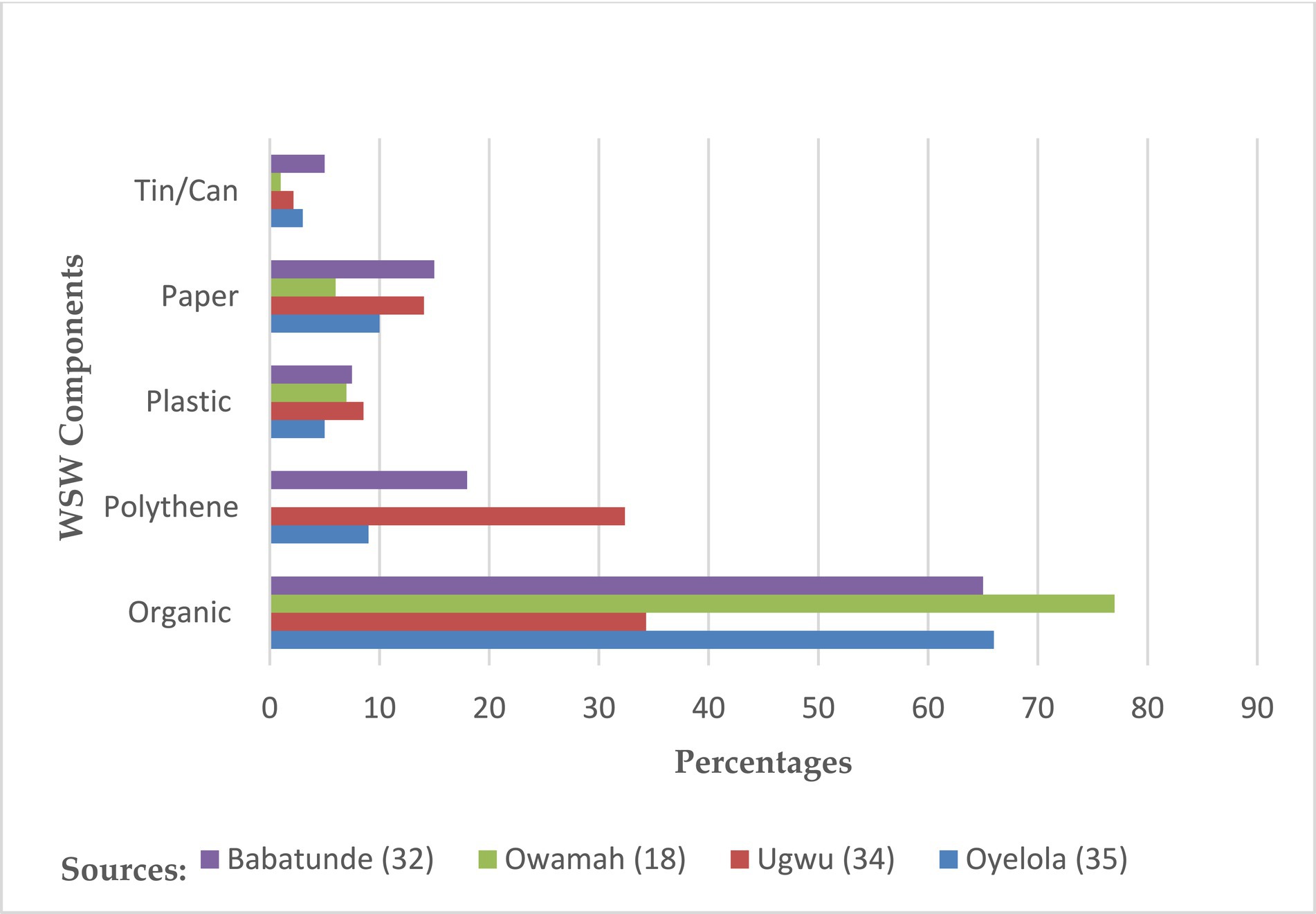
Figure 2. Percentage composition of prominent MSW components in Nigeria (Babatunde et al., 2013; Owamah et al., 2017; Oyelola et al., 2017; Ugwu et al., 2020).
Global research on the composition of MSW reveals that the biodegradable waste components range between 28, 58, and 41% for high, middle, and low-income countries (Karak et al., 2012). However, predictions have estimated approximately 33, 50, and 60% by 2025 in high, middle, and low-income nations, respectively (Hoornweg and Thomas, 1999; Ozcan et al., 2016). Figure 3 shows the MSW composition in Sub-Saharan Africa.
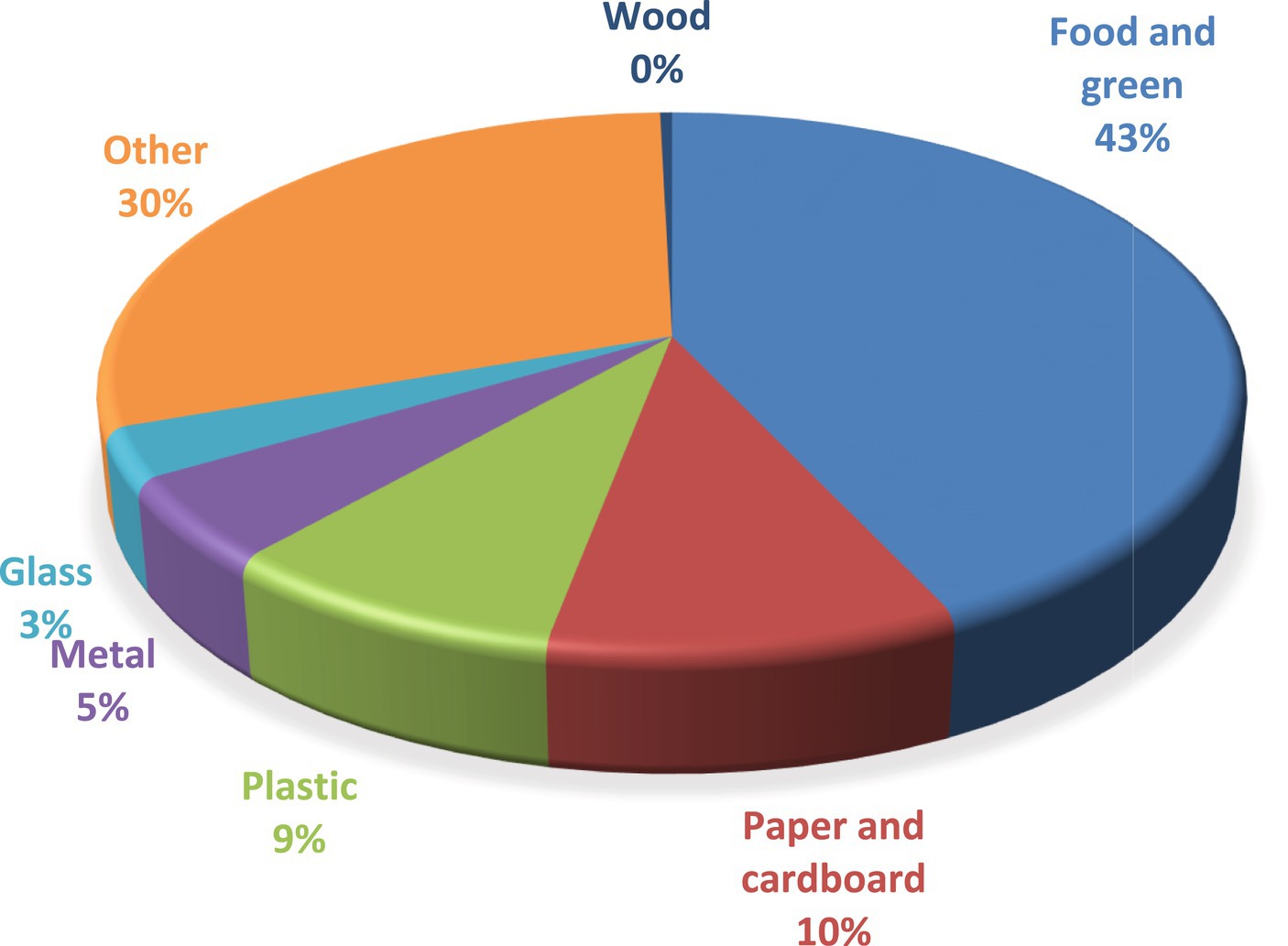
Figure 3. Waste composition (%) in Sub-Saharan Africa (Kaza et al., 2018).
1.3 Characterization
MSW characterization is a process of determining the components that constitute a waste stream (Aderoju Olaide, 2020). Waste characterization enhances effective planning for sustainable MSWM aimed at waste reduction, efficient recycling programs, resource conservation, money and resources and waste regeneration (Ugwu et al., 2020; Mehdi Hassan et al., 2023). The MSW stream must be audited through characterization to monitor an existing MSWM system effectively aimed at policy review for decision-making. This can be achieved by sorting, stratification, quantifying, and identifying the MSW composition (Edjabou et al., 2015; Pathak et al., 2020). Some approaches to achieve integrated MSWM are characterization and quantification of MSW. Therefore, to ensure effective and sustainable MSWM, these processes must provide reliable sources for data acquisition during the planning, review, and implementation stages (Al-Jarallah and Aleisa, 2014; Cheela et al., 2021).
The two main approaches used for MSW characterization are the material flow and site-specific approaches (Kumar and Garg, 2021). The latter requires sorting the various MSW components at the source, carried out at the generation point or disposal facility. For bulky samples, this can be done visually at the source or from the waste truck (Giang, 2017; Ugwu and Ozor, 2021). MSW characterization can be achieved using SowaCLINK software, a computer-based environmental MSW analytical tool developed based on Dulong’s model (Sincero and Sincero, 1996).
The software characterizes the MSW into fourteen (14) waste components, including food, yard, paper, cardboard, plastics, textiles, rubber, leather, wood, glass, tin/can, aluminum, other metals, dirt, and ash.
The output of the characterization process is utilized for further analyses of waste regeneration and sustainable MSWM. This is because the software provides the potential energy content of MSW, which can be used to determine the high and low heating values of the characterized MSW. Results of the characterized MSW can be extracted in statistical charts for straightforward interpretation (Onungwe et al., 2021). The software performs MSW characterization with an accurate analytical algorithm to determine waste heating or energy value content, which is the combustible energy contained in the material in the presence of oxygen (Bhuiya et al., 2020).
The Dulong model is a reliable computational for analyzing the high heating value (HHV) and low heating value (LHV) of MSW when compared with the Vandralek model and other experimental processes (Nzihou et al., 2014). However, the Steuer and Scheurer-Kestner model is helpful for energy value analysis, and the Dulong model is widely reliable and widely adopted (Liu et al., 1996; Khuriati et al., 2017).
In a typical scenario of the Waste-to-Energy (W-to-E) process, MSW was found to have the energy potential of producing 1 MW of electricity from 45 tons of waste; in other words, about 550-kilowatt hours (kWh) of energy is generated per ton of MSW (Cheremisinoff, 2013; O'Neill, 2022). A calorimeter can be used to ascertain the energy value of MSW directly (Amber et al., 2012). Similarly, for a sample MSW with an average calorific value of 17.23 MJ/kg and MC ranging between 20 and 49%, it was analyzed that 700 kW of power per day can be generated when 1,500 tons of characterized MSW is incinerated per day (Amber et al., 2012). Alternatively, an ultimate analysis can be used to analyze the LHV of MSW components with known energy value by adopting the chemical element of the characterized waste (Sincero and Sincero, 1996; Ibikunle et al., 2019).
1.4 Waste to energy conversion
Several techniques of waste-to-energy conversion exist (Foster et al., 2021), such as gasification, pyrolysis, anaerobic digestion, incineration, and hydrothermal liquefaction. However, waste audits provide insight into sustainable processes through characterization (Pan et al., 2015; Rafey et al., 2020). Characterized MSW is composed of high energy content with the potential to generate steam in a boiler capable of powering an electric generator turbine (Wilson et al., 2013). MSW are categorized into various components such as yard, paper, food, plastic, cardboard, wood, rubber, textile, leather, etc. (Yusoff et al., 2018). Mining these components without recycling used products from the waste stream will deplete the ecosystem and increase the effect of climate change and global warming on the environment (Bello et al., 2022). Therefore, the need to utilize waste as feedstock in a conversion facility benefits the environment and livability (Alao et al., 2022; Salem et al., 2023) while promoting a sustainable MSWM system (Farooq et al., 2021). However, waste conversion is rewarding socially, economically and environmentally (Kalkanis et al., 2022); risk analysis to adopt a safe technology remains vital (Luo et al., 2021; Cao et al., 2022). Different energy products are derived from various feedstock based on the waste composition and waste-to-energy conversion technique, as presented in Figure 4.
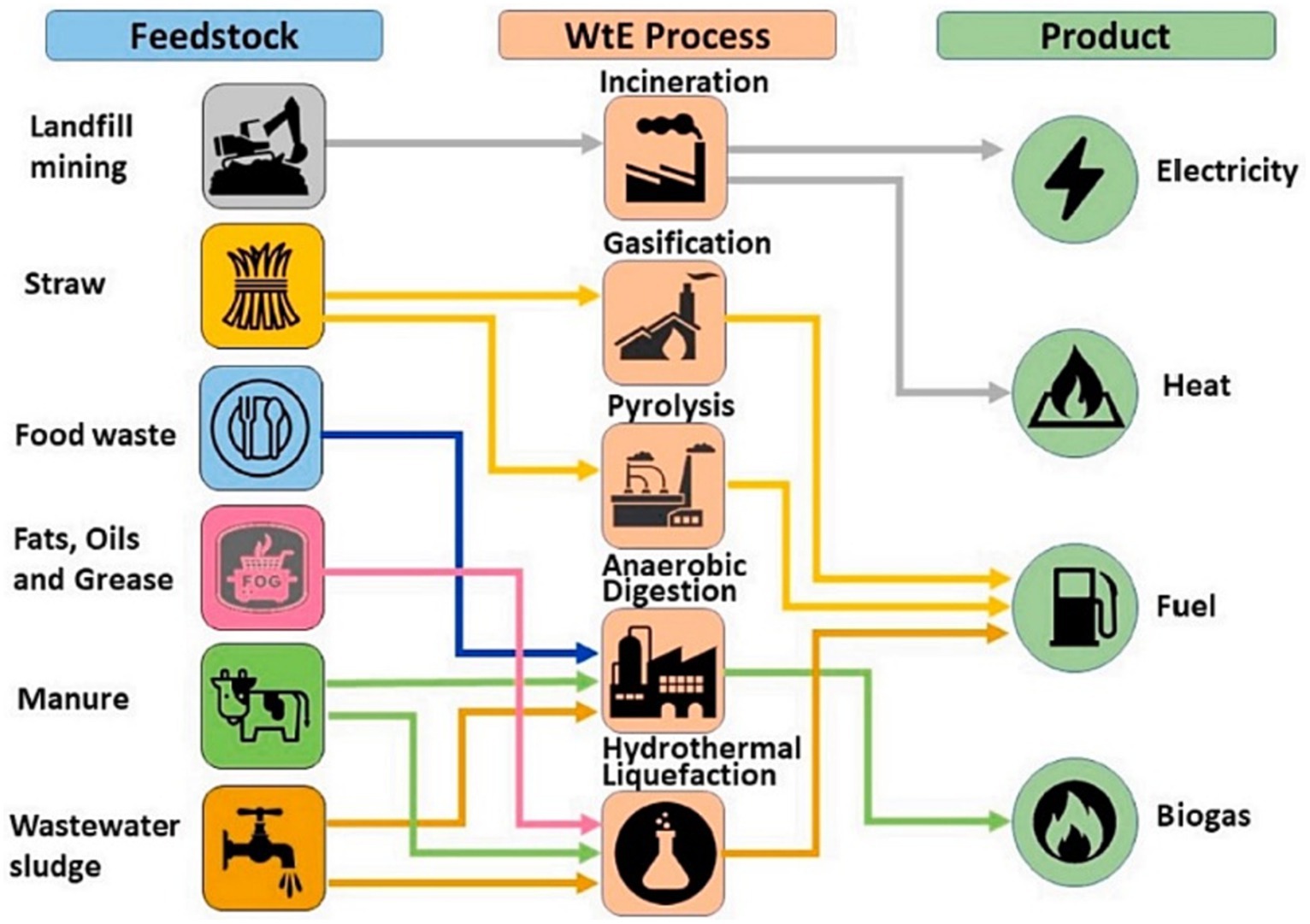
Figure 4. Feedstock, process of waste-to-energy, and energy product derived (Foster et al., 2021).
Incineration is a process of MSWM that leads to an alternative energy source with substantial environmental benefits of waste volume reduction (Bello et al., 2022), though there has been some public criticism on the adoption of the technique (Wong, 2016); incineration as a power generating source has been significant toward waste-to-energy globally. Notably, the by-products from incineration can be used to remove methylene blue (MB) from water during purification. Some of these by-products are MSW bottom ash (MSW-BA) and MSW fly ash (MSW-FA), which are sources of aluminosilicate to produce geopolymer (GEO) adsorbents (GEO-MSWBA and GEO-MSWFA) (Al-Ghouti et al., 2021). Figure 5 shows a diagrammatic presentation of MSW combustion with energy recovery in a mass-burn incinerator.
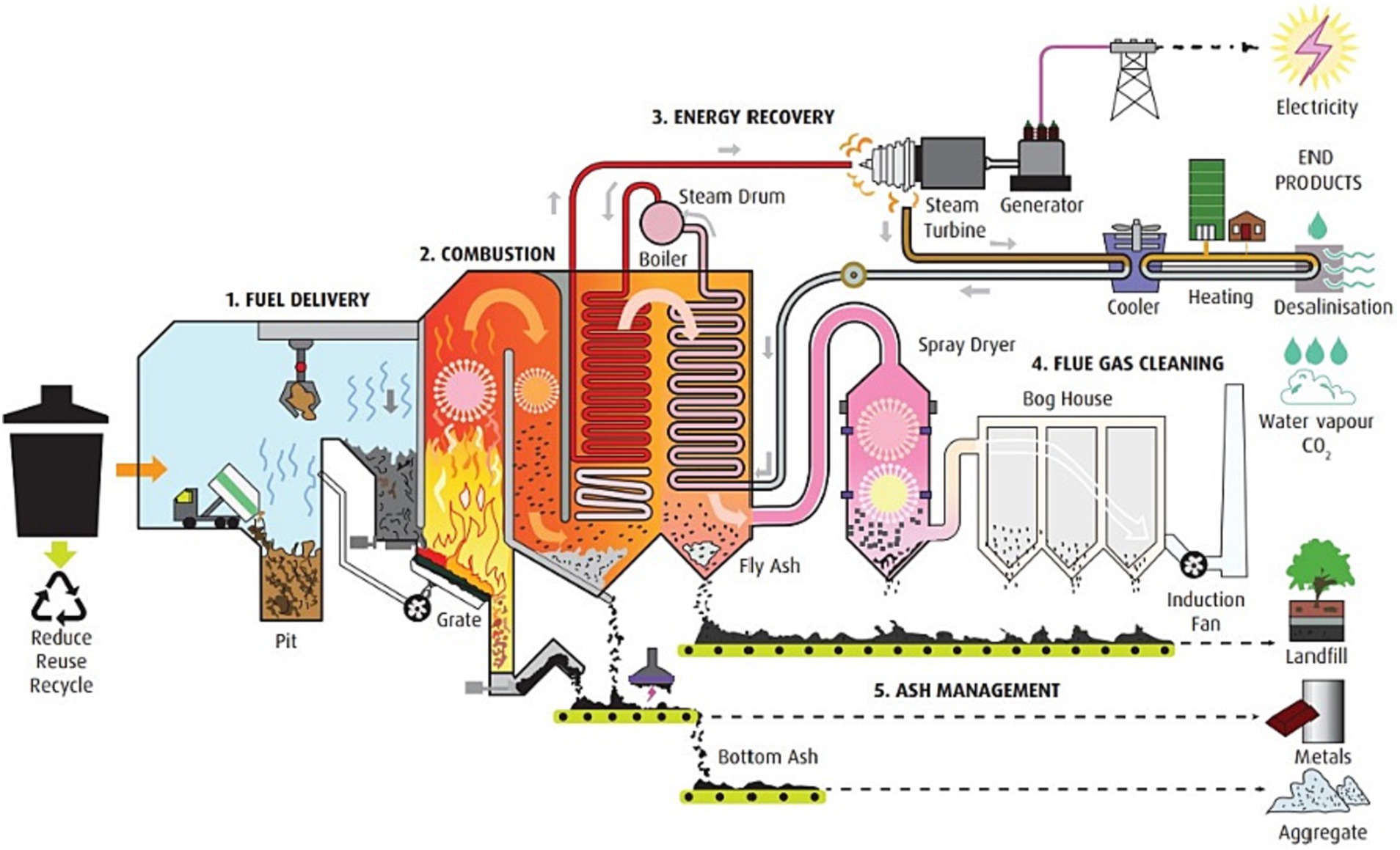
Figure 5. MSW combustion with energy recovery in a mass-burn incinerator (Van Ewijk and Stegemann, 2023).
Considering the high rate of organic fraction of MSW generated in developing countries, anaerobic digestion has been a viable technique for producing biogas energy while utilizing the MSW as feedstock (Hoornweg and Bhada-Tata, 2012; Mousania et al., 2023). Composting is a sustainable process of managing organic waste components (Sánchez et al., 2016); this can be achieved either anaerobically or aerobically in line with the CE model (Ayilara et al., 2020; Zamri et al., 2021). Bio-fertilizers for agricultural soil improvement can be produced by composting bio-degradable MSW components, a circulatory process of waste regeneration. As a composting technique, Anaerobic digestion has low operational cost, high environmental benefits, and social acceptability (Ayilara et al., 2020; Roy et al., 2023). Promisingly, composting rapidly reduces the volume of MSW, the ruination of pathogenic bacteria, the elimination of weeds, and the destruction of seeds (Bello et al., 2022).
For composting to be sustainably integrated into an anaerobic process of MSWM, the MSW collection and transportation are essential, leading to the pre-treatment of the waste in the digester, which results in biogas or digestate—the post-treatment results in either energy or nutrients (Van Fan et al., 2018). Hence, energy and bio-fertilizer are products of anaerobic digestion of organic MSW components in a biogas plant (Cheremisinoff, 2013; Onungwe et al., 2021), as presented in Figure 6.
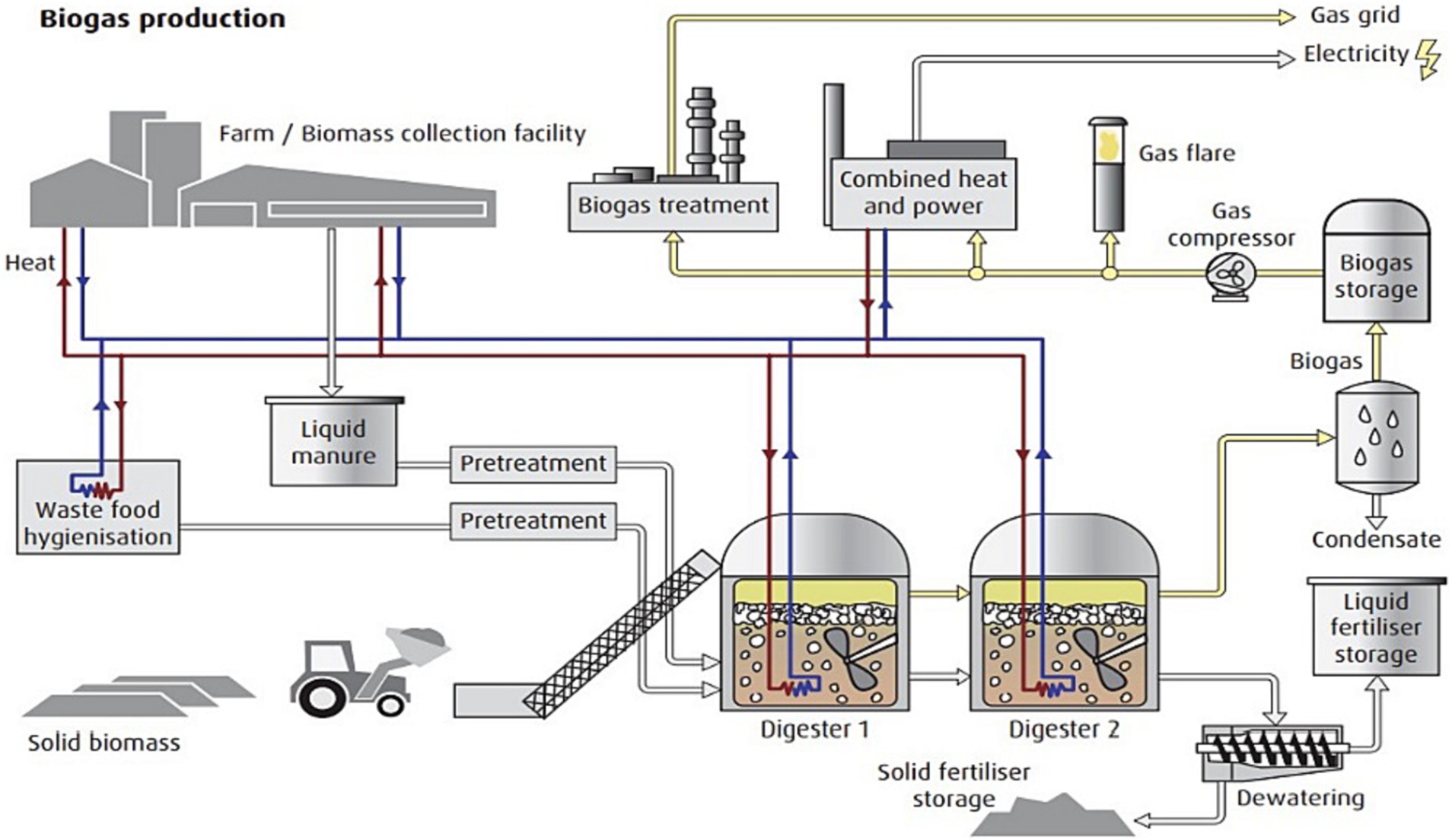
Figure 6. Anaerobic digestion of organic component of MSW in a biogas plant (Van Ewijk and Stegemann, 2023).
1.5 Circularity in municipal solid waste management
The concept of circular economy (CE) hinges on sustainability, aimed at protecting and improving the environment while offering economic and social benefits to the citizens (Mandpe et al., 2023; Van Ewijk and Stegemann, 2023). The circular economy enables the restoration of product value when it is damaged or has reached the end of life. CE helps extend the product life cycle. Waste reduction is also achieved while conserving scarce natural resources. Techniques upon which CE can be performed include reuse, remanufacturing, recycling, etc. (Vanson et al., 2022). For CE to be effectively implemented, a conscious effort must be made in regulatory legislation, governmental policy, administrative model, product design model, supply chain management, and waste management (Smol et al., 2020; Kazancoglu et al., 2021). CE can be integrated into the MSWM system through waste sorting, plastic waste recycling, incineration techniques, and life cycle assessment (Tsai et al., 2020; Aldhafeeri and Alhazmi, 2022). Apart from the 3Rs; reduce, reuse and recycle which is widely discussed in MSWM, the principle of the 10Rs; refuse, rethink, reduce, reuse, repair, refurbish, remanufacture, repurpose, recycle, and recover can enhance circularity in MSWM (Listiningrum et al., 2023). Also, transferring product ownership, routine maintenance, product performance monitoring, energy recovery through thermal treatment for heat or fuel extraction, combustion of MSW for electricity and heat generation are circulatory processes that can integrated into the MSWM system (Reike et al., 2018; Gherheş et al., 2022).
2 Materials and methods
2.1 Study area
MSW components constitute the primary material used for this research. The MSW was collected within Aba metropolis, Eastern Nigeria, West Africa. Aba, which represents a megacity as adopted by this research, is located between latitude 5o7 “N and longitude 7o22 “E, at a height of 205 meters above sea level. The city has four major designated open dumpsites, with one closed permanently at the time of this study. Under this section, each research methodology (RM) is carried out to achieve each research objective (RO).
2.2 RM1—analysis to determine the volume of MSW generated
To achieve RO1, the population of the city leading to the rate of MSW generation was computed by applying the geometric mean method given in Equation 1. The operational population was projected for a 10-year design period, with the Nigerian growth factor kept at 2.53% (O'Neill, 2022). The geometric mean is a statistically proven reliable approach for obtaining a realistic population projection to achieve a safe infrastructural design over a specific period (Agunwamba, 2008).
Pn is the projected design population. P is the current population of the study area; IG is the percentage geometric mean or growth factor of the study nationality; n is the design period in years. With the absence of MSW data for the study area, linear extrapolation becomes applicable in obtaining the rate of MSW generation. Equation 2 was adopted to achieve this objective.
X1 and X2 are the years with known waste rates from government agencies and academic research, respectively. X is the year that the population census was conducted with an unknown waste rate, Y1 and Y2 are the rates of known waste for the year X1 and X2, respectively, and Y is the unknown MSW rate for the year that the population census was conducted, which the projected design population was based. The value of Y becomes the operational rate of the MSW generation per person per day.
2.3 RM2—characterize MSW components generated using SowaCLINK software
The MSW components generated and collected within the study area were handled with consideration to health and safety precautions for characterization based on the Standard Test Method for Determination of the Composition of Unprocessed MSW method D5231-92 (ASTM International, 1992), which describes the steps for manual sorting to determine the mean composition of MSW generated within an environment in line with the material flow method of MSW characterization (Tchobanoglous and Kreith, 2002). The procedures followed are outlined in the steps below based on the site-specific approach:
Step 1: Firstly, on the arrival of the delivery trucks, samples were collected at various dumpsites using a site-specific approach by scooping a mixed portion of the MSW delivered to the dumpsites from 3 different locations within the metropolis (i.e., Osisioma, South Aba, and North Aba). The exercise was conducted for 48 days within 16 consecutive weeks between July and November 2021. The process cuts across both the wet and dry seasons, as the country of the study has seasonal climatic conditions.
Step 2: The second procedure followed the quartering process by dividing the MSW sample into four equal parts on a clean surface and eliminating two diagonal portions (Zurbrugg and Imanol, 2018). The remaining portions were processed, sorted into components, and weighed in line with the standard test method for determining the details of unprocessed MSW (ASTM International, 1992; Nadeem et al., 2016).
Step 3: The third procedure involved imputing the weighted values (kg) of the sorted MSW components into the SowaCLINK software for analysis and characterization (Onungwe et al., 2021). This waste audit step enables appropriate MSW utilization based on the outcome. The characterized representative MSW sample for each study location is attached in Supplementary Appendices A–C, which led to further analysis as detailed in the result sections. The comparative composition of MSW components generated within the study area is presented in Figure 7. This gives an idea toward selecting the most sustainable MSWM techniques based on the circularity principle.
2.4 RM3—analysis to determine the energy potential of the characterized MSW
Following the procedure of ASTM D5291, which focuses on standard simultaneous determination of carbon, hydrogen, and nitrogen in petroleum products (Titiladunayo et al., 2018). In addition to utilizing from Supplementary Appendix, which shows selected chemical elements comprising of carbon, hydrogen, oxygen, nitrogen, sulfur and ash with percentage value by mass on a dry basis obtained based on ultimate analysis carried out on some MSW components with high energy potential (Sincero and Sincero, 1996), the high heating value (HHV) of the identified MSW from the waste stream sample was determined by adopting Equation 3.
The total volume of the MSW sample was 947 tons, representing 71% of the MSW generated within the study area. From the weight (Wt) of each MSW component in percentage, as presented in Table 13, the computation of the low heating value (LHV) of the characterized MSW with energy-generating potential was analyzed by applying Equation 4 (Ibikunle et al., 2019).
LHV is the low heating value, HHV is the high heating value, and Wt. is the weight of each MSW component in percentage. From the determined HHV and LHV, Equations 5–7 (Ibikunle et al., 2019; Ajaero et al., 2023; Dickson et al., 2023) can be applied to select the energy recovery potential ERP (MWh), the electrical power potential EPP (MW), and the power to the grid of electrical power PG (MW) generated from the MSW.
ERPMSW is the energy recovery potential, LHVMSW is the low heating value (MJ/kg), 1,000/3.6 is the conversion ratio from MJ to kWh since 1 kWh equals 3.6 MJ and WMSW is the mass of MSW in tons.
EPPMSW is the electrical power potential, LHVMSW is the low heating value, and 1,000/3.6 is the conversion ratio from MJ to kWh since 1 kWh equals 3.6 MJ, WMSW is the mass of MSW in tons and cε is the conversion efficiency usually between 20 and 28%.
PGMSW is the power to the grid, EPPMSW is electrical power potential, gε is generator efficiency, adopted at 90%, tε is transmission efficiency at 75% for incineration turbine output, and 1/1,000 is a conversion factor.
2.5 RM4—circular economy transition roadmap for MSW utilization
MSW quantity, characteristics, physical properties, composition, availability of land, social factors, capital investment, treatment processes, environmental factors, etc., are some of the determinants for suitable MSW treatment techniques (Aich and Ghosh, 2016; Zabaleta et al., 2020). This is because identifying sustainable methods for MSW utilization enhances circularity in the MSWM system. This section focuses on selecting sustainable MSW treatment technologies that will be productive for MSW regeneration. Relevant literature on sustainability factors such as environment, economy, technology and social indicators (Alam et al., 2022); the outcome of RM1, RM2, and RM3, which produced the prominent MSW components available within the study area, and the findings from background research preceding this current study, which identified potential enablers for MSWM transition to CE (Onungwe et al., 2023), gave insight on the suitable options to include incineration and anaerobic digestion under the thermochemical and biochemical techniques respectively (Agbejule et al., 2021; Farooq et al., 2021).
3 Results and discussion
This section chronologically presents the results obtained from the analysis using RM1, RM2, RM3, and RM4 based on RO1, RO2, and RO3. The projected population computed for the current research based on a design period of 10 years by adopting Equation 1 was 1,719,185 persons. The variables substituted into the equation were the existing population of the city (P) 1,339,100, the design period in years (n) 10, and the percentage geometric mean or growth factor of the study nationality (IG) 2.253%.
The rate of MSW in kilogram per person per day (kg/p/d) generated within the study area was analyzed using Equation 2. The linear extrapolation with “Y,” the unknown variable representing the rate of MSW (kg/p/d), resulted in 0.7813 kg/p/d. Other variables substituted into the equation were X1 = 1994, X2 = 2006, X = 2016 and Y1 = 0.58, Y2 = 0.69.
The volume of MSW generated annually within the municipality is 490,268 tons. The results from the MSW samples collected from the various open dumpsites and the corresponding analytical results using SowaCLINK software are presented in subsequent tables and charts to include mass of moisture (kg), dry weight of MSW sample (kg), heating value (KJ), total energy (KJ), density (kg/cum) and the composition of MSW components in percentage. Equations 3–7 were to determine the MSW energy potential of the characterized waste stream for suitable circularity model adoption for MSWM within the megacity.
3.1 Results of Osisioma
Supplementary Appendix A shows the composition of MSW prominent within Osisioma as input into SowaCLINK. The design shows putrescible waste in large quantities when compared with non-putrescible waste. Supplementary Appendix illustrates the SowaCLINK analytical output based on the simulation of the MSW collected within Osisioma. This set of results provided insight into the characteristics of the MSW sample for decision-making. This is because ascertaining the moisture content (MC) of MSW significantly contributes to its utilization in terms of treatment methods, recycling, and energy consumption rate (Finet, 1987; Lorenzo Llanes and Kalogirou, 2019).
Notably, SowaCLINK software was programmed based on a range of moisture content values for the various MSW components in line with the analysis carried out by Tchobanoglous (Peavy et al., 2010). This resulted in the fourth column showing the percentage moisture content (Tchobacao M.C. Value %) extracted from the software (Table 1).
Table 2 shows some outputs from the software indicating the energy content of the characterized MSW. A comprehensive knowledge of the MSW stream’s heating value and energy potential enhances its optimal circulatory utilization. Also, the engineering design of a thermochemical plant output depends on the heating potential of the MSW feedstock (Zhou et al., 2014). The results of the characterized MSW components presented in Tables 2–6 are consistent with the outcome of a similar analysis on energy recovering from MSW components for electricity generation (Sincero and Sincero, 1996; Pasek et al., 2013).
The results following the wet and dry densities of the characterized MSW were 1.31 and 1.33 (kg/cum), respectively. MSW density is a function of weight, mass, and volume; possessing. The knowledge of such indexes is required to achieve sustainable engineered MSWM, appropriate MSW operational utilization and functional infrastructural design, including collection truck capacity allocation for efficient waste collection (Jaunich et al., 2016). Accurate knowledge of the composition of MSW components within any city is an enabler for integrated and sustainable MSWM (Bisinella et al., 2017).
Supplementary Appendix E shows the percentage of MSW components within Osisioma LGA. The statistical chart indicating the MSW composition in various quantities is a primary requirement for MSWM transition toward circular economy implementation. This is because MSW sorting, collection, selection of transportation equipment, recycling, energy recovery, and extraction of reusable MSW components are tools for sustainable MSWM systems (Siami et al., 2019; Ugwu et al., 2020).
The results presented in this section close the gap in the absence of practical knowledge of MSW composition, quantification, energy potential, leading, and the selection of treatment techniques that utilize waste as a resource. However, the study area is known for the massive rate of MSW generation; MSWM has been rudimental, posing concerns about public health and environmental safety. Also, the lack of MSW data has hindered sustainable MSWM in Aba (Ezechi et al., 2017; Nwankpa and Scandrett, 2020). The significance of the current research hinges on its site-based approach because most research on the study is literature-based.
3.2 Results of Aba South
Table 7 shows the percentage composition, mass (kg), moisture content (%), mass of moisture (kg), and dry weight (kg) of the MSW representative sample of the MSW available in Aba South based on the output of the analysis from SowaCLINK software. Considering the components of the characterized MSW, plastic, textile, rubber, leather, wood, and paper constitute the prominent combustible waste stream. The biodegradable waste components are food and yard waste. This result is consistent with the MSW composition in Sub-Saharan Africa (Kaza et al., 2018).
However, there is a slight difference in the waste stream among the zones comprising the study area; the results presented align with some previous research on the premise that the composition of MSW can vary within the same settlement based on lifestyle, income, education, awareness, and infrastructural availability (Babatunde et al., 2013; Letshwenyo and Kgetseyamore, 2020). An effective plan and design of a functional and implementable integrated sustainable MSWM system is realistic, considering the results obtained thus far.
Some useful variables that can enhance the utilization of MSW for energy conversion include thermal efficiency and heating values. When further analyzed, these factors can result in energy recovery potential, electric power potential and power to the grid (Ajaero et al., 2023; Dickson et al., 2023). Table 4 shows these variables based on the characterized MSW components generated in Aba South LGA. The wet and dry densities of the MSW are 1.23 and 1.30 (kg/cum), respectively. The percentage weight of the MSW on a damp and dry basis is 18.63 and 22.9 (%), respectively. The average percentage of the prolific component of the MSW characterized is 24%. The explosive element is 46.4%, giving a clear insight into the available waste stream. This is shown in Supplementary Appendix on the pie chart extracted from SowaCLINK software in Supplementary Appendix F.
3.3 Results of Aba North
Supplementary Appendix C tabulates the MSW output collected in Aba North. The waste audit results indicate that sustainable energy generation is viable because of the waste stream’s massive volume and identified inert potential (Onungwe et al., 2023).
Table 6 significantly enhances the sustainable and best utilization of the available MSW with specific results produced from the SowaCLINK software analysis. The energy potential of the waste is better known, leading to the most appropriate circular economy application. The wet density resulted in 1.26 (kg/cum), and the dry density was 1.28 (kg/cum).
The wet and dry weights of the MSW sample are 18.39 and 22.54%, respectively. The relevance of these indices is to enable the functional design of a sanitary landfill, transfer station, smooth collection and transportation of MSW within the metropolis. Since compact waste is a requirement in sustainable MSWM in line with the efficient operation of a landfill, ascertaining the optimum density of the waste is essential.
The composition of the waste stream that was collected and characterized in Aba North LGA is presented by a pie chart, which was an analytical output of the SowaCLINK software attached in Supplementary Appendix G. The putrescible and non-putrescible waste has an average value of 23.8%; and 47%, respectively.
The MSW waste stream within the study area predominantly comprises food waste. This result conforms with some MSW characterization outcomes (Babatunde et al., 2013; Owamah et al., 2017; Kaza et al., 2018). However, the organic composition of MSW generated in some developing countries ranges between 33 and 60% (Karak et al., 2012; Ozcan et al., 2016; Aderoju Olaide, 2020). This research revealed that the organic waste available within the study area is about 20.9 to 23.8%. By interpretation, a more practical knowledge of the MSW composition of the study area has been established based on the site-specific approach. This will inform good decision-making by the stakeholders in developing a suitable MSWM which will integrate environmental, economic, social, and commercial factors.
The significance of MSW audit in quantification and characterization cannot be overemphasized based on MSW utilization. Also, the research reveals that MSWM practice within Aba is based on the linear model, which has numerous environmental, public health, social, and ecosystem concerns, as dumpsite disposal is the only operational technique (Ezechi et al., 2017; Salami et al., 2018).
With references to Table 4, the chemical element of the MSW components based on ultimate analysis on percentage by mass on a dry basis was adopted in analyzing the high heating value (HHV) by applying Equation 7 on the identified MSW with energy-generating potential, which resulted in an HHV of 176.5212 MJ/kg as presented in Table 8.
Table 7 shows the Low Heating Value (LHV) of the characterized MSW component in Megajoules per kilogram (MJ/kg). The mean value of each MSW component and the weight of the waste sample with energy potential are also tabulated in percentages. The output from SowaCLINK software on the heating value and total combustion energy in KJ/kg of the characterized MSW is within an acceptable range (Sincero and Sincero, 1996; Rominiyi et al., 2017; Oladejo et al., 2020).
71% of the characterized MSW has energy potential for waste-to-energy conversion. Applying Equations 5–7, the energy recovery potential ERPMSW, electrical power potential EPPMSW, and grid power PGMSW were obtained as 3,709,463 MWh, 38,680 MW and 26.1 MW daily. In secondary research carried out on the organic fraction of municipal solid waste (OFMSW) collected within a region that has similar demography as Aba, the energy recovery potential, electrical power potential, and power to the grid were 1,299,083 MWh, 16,239 MW and 10,961 MW, respectively, (Ajaero et al., 2023).
The results indicate a possible transition to a circular economy model based on the energy potential and the vast availability of MSW within the study area. This will reduce developing countries’ environmental and health challenges, including Nigeria’s, while converting waste into resources (Sharma and Jain, 2020; Hoang et al., 2022). Also, the analytical output of LHV resulting in the above EPR, EPP, and PG of the characterized MSW with quantifiable energy content falls within a range of 7.5–17 MJ/kg, which points to its fuel viability based on calorific analysis (Nnaji, 2015).
Considering the results of the MSW characterized in terms of composition and energy content, biochemical and thermochemical technologies with anaerobic digestion and incineration are suggestive techniques for achieving circularity in the MSWM system in Aba metropolis. With the volume of MSW available within the study area and the poor electricity supply, the incineration technique will reduce the volume of MSW by utilizing it for electricity generation (Lu et al., 2017; Nordi et al., 2017). Incineration has been proven to be a technique that can minimize waste stream by up to 70% and its volume by up to 90% (Akinshilo, 2019; Ajaero et al., 2023). By adopting these biochemical and thermochemical technologies, the narrative about the study areas as the messiest city in Nigeria (Odoemene and Ofodu, 2016) will change through the MSWM circularity model (Gutiérrez et al., 2021; Varjani et al., 2022).
The daily analytical output of 10,961 MW of power to grid potential from the MSW components of Aba metropolis is consistent with the findings of (Cheremisinoff, 2013; Advancing Sustainable Materials Management, 2017), which, when harnessed, will boost the electricity consumption in Nigeria from 156 kWh, which is the least when compared to countries like Venezuela, Ghana, and Ivory Coast with 3,413 kWh, 309 kWh and 174 kWh, respectively (Somorin et al., 2017). The current linear MSWM system of take make and dispose of at dumpsite will transition to waste to energy conversion through MSW utilization, a circular economy model (Kothari et al., 2010; Rezania et al., 2023).
The findings from this practical and analytical-based research will enhance an integrated sustainable MSWM that will boost the participation of stakeholders (Tchobanoglous and Kreith, 2002; Zurbrugg and Imanol, 2018), enhancing functional planning and improving policymaking strategy and review. Also, efficient monitoring of the MSWM system, better knowledge of the waste stream and effective implementation of MSWM techniques based on the CE model will be achieved (Ontario Environment, 2017; Wang et al., 2022). Considering the projected MSW generation with its effect on the developing countries (Kaza et al., 2018) and the findings made by this current study, the roadmap for MSWM based on the circular economy model can be achieved and sustained to the benefit of Aba metropolis environmentally through appropriate MSWM techniques, leading to recycling, reduction in greenhouse gas emissions (GHG), conservation of natural resources, and improved city dweller’s livability (Orlov et al., 2021). Social benefits include public health and sanitation to achieve the SDGs (Van Kruchten and Van Eijk, 2020). Waste to wealth can be categorized as economic benefits as citizens have regular access to energy consumption while contributing revenue generation through service payment (Oba et al., 2015; Dickson et al., 2023).
4 Conclusion
A site-based and analytical municipal solid waste management (MSWM) study aimed at conducting comprehensive waste audits with research objectives on MSW quantification and characterization using a computer-based environmental application, SowaClink software, to ascertain the energy contents of the MSW generated within the Aba metropolis is presented. However, the study area is considered the messiest commercial city in Nigeria; this current research focused on utilizing the available MSW within the study area as raw materials for waste regeneration based on circularity.
Findings reveal that the status of MSWM in the Aba metropolis is poor, as MSW is disposed of at dumpsites based on the linear economy model. The large quantity of various components of MSW generated within the area indicates the availability of raw material as feedstock for circular economy implementation for waste reuse. This can be initiated through waste sorting, recycling, characterization, and adopting the 10Rs to boost the conservation of natural resources for improved livability within the environment. Also, further results reveal MSW generation at a rate of 0.7813 kg/p/d and 490,268 t/y for a population of 1,719,185 persons. 71% of the MSW, comprising 48% combustible components and 23% organic fraction on average, have energy content such that, when converted, can produce electricity and bio-fertilizer. The high heating value of the MSW was 176.5 MJ/kg, and the low heating value was 14 MJ/kg. The energy recovery potential of the MSW (ERPMSW) was 3,709,463 MWh, the electrical power potential (EPPMSW) was 38,680 MW, and the power to the grid (PGMSW) was 26.1 MW per day.
The global environmental challenges associated with MSW due to the increase in population growth, rural–urban migration, attitude of citizens, educational awareness, industrialization, commercial activities, natural resource mining, waste mismanagement, and lack of MSWM facilities keep increasing, especially in developing countries. Therefore, engaging in MSWM practices promoting environmental sustainability through waste recovery should be encouraged at all levels. Because the MSW generation is a sign of economic activities and societal functionality, which cannot be stopped, instead incorporating MSW characterization and composition stratification at source is necessary to achieve waste conversion for an integrated sustainable waste management based on the circular economy model.
Data availability statement
The original contributions presented in the study are included in the article/Supplementary material, further inquiries can be directed to the corresponding author.
Author contributions
IO: Conceptualization, Data curation, Formal analysis, Investigation, Methodology, Software, Writing – original draft, Writing – review & editing. DH: Funding acquisition, Project administration, Supervision, Visualization, Writing – review & editing. IJ: Project administration, Supervision, Visualization, Writing – review & editing.
Funding
The author(s) declare financial support was received for the research, authorship, and/or publication of this article. DH received financial support from the UK EPSRC under grant EP/J017698/1 (Transforming the Engineering of Cities to Deliver Societal and Planetary Wellbeing, known as Livable Cities) and EP/P002021 (From Citizen to Co-innovator, from City Council to Facilitator: Integrating Urban Systems to Provide Better Outcomes for People, known as Urban Living Birmingham).
Acknowledgments
IO gratefully acknowledges the financial support of the Tertiary Education Trust Fund and Kenule Beeson Saro-Wiwa Polytechnic, Bori, Rivers State of Nigeria, given to him during his doctoral studies.
Conflict of interest
The authors declare that the research was conducted in the absence of any commercial or financial relationships that could be construed as a potential conflict of interest.
The author(s) declared that they were an editorial board member of Frontiers, at the time of submission. This had no impact on the peer review process and the final decision.
Publisher’s note
All claims expressed in this article are solely those of the authors and do not necessarily represent those of their affiliated organizations, or those of the publisher, the editors and the reviewers. Any product that may be evaluated in this article, or claim that may be made by its manufacturer, is not guaranteed or endorsed by the publisher.
Supplementary material
The Supplementary material for this article can be found online at: https://www.frontiersin.org/articles/10.3389/frsus.2024.1321329/full#supplementary-material
References
Abubakar, I. R., Maniruzzaman, K. M., Dano, U. L., AlShihri, F. S., AlShammari, M. S., Ahmed, S. M. S., et al. (2022). Environmental sustainability impacts of solid waste management practices in the global south. Int. J. Environ. Res. Public Health 19:12717. doi: 10.3390/ijerph191912717
Aderoju Olaide, M. (2020). Municipal solid waste characterization as a measure towards sustainable waste Management in Abuja, Nigeria. J. Environ. Sci. Public Health 4, 43–60. doi: 10.26502/jesph.96120084
Advancing Sustainable Materials Management. (2017). Fact Sheet. Assessing Trends in Material Generation, Recycling, Composting, Combustion with Energy Recovery and Landfilling in the United States. Available at: https://www.epa.gov/sites/default/files/2019-11/documents/2017_facts_and_figures_fact_sheet_final.pdf (Accessed May 08, 2023).
Agbejule, A., Shamsuzzoha, A., Lotchi, K., and Rutledge, K. (2021). Application of multi-criteria decision-making process to select waste-to-energy technology in developing countries: the case of Ghana. Sustain. For. 13:12863. doi: 10.3390/su132212863
Aich, A., and Ghosh, S. K. (2016). Application of SWOT analysis for selecting technology for processing and disposal of MSW. Procedia Environ. Sci. 35, 209–228. doi: 10.1016/j.proenv.2016.07.083
Ajaero, C. C., Okafor, C. C., Otunomo, F. A., Nduji, N. N., and Adedapo, J. A. (2023). Energy production potential of organic fraction municipal solid waste (OFMSW) and its implications for Nigeria. Clean Technol. Recycl. 3, 44–65. doi: 10.3934/ctr.2023003
Akinshilo, A. (2019). Energy potential from municipal solid waste (MSW) for a developing metropolis. J. Therm. Eng. 5, 196–204. doi: 10.18186/thermal.654322
Alam, S., Rahman, K. S., Rokonuzzaman, M., Salam, P. A., Miah, M. S., das, N., et al. (2022). Selection of waste to energy technologies for municipal solid waste management—towards achieving sustainable development goals. Sustain. For. 14:11913. doi: 10.3390/su141911913
Alao, M. A., Popoola, O. M., and Ayodele, T. R. (2022). Waste-to-energy nexus: an overview of technologies and implementation for sustainable development. Clean. Energy Syst. 3:100034. doi: 10.1016/j.cles.2022.100034
Aldhafeeri, Z. M., and Alhazmi, H. (2022). Sustainability assessment of municipal solid waste in Riyadh, Saudi Arabia, in the framework of circular economy transition. Sustain. For. 14:5093. doi: 10.3390/su14095093
Al-Ghouti, M. A., Khan, M., Nasser, M. S., al-Saad, K., and Heng, O. E. (2021). Recent advances and applications of municipal solid wastes bottom and fly ashes: insights into sustainable management and conservation of resources. Environ. Technol. Innov. 21:101267. doi: 10.1016/j.eti.2020.101267
Al-Jarallah, R., and Aleisa, E. (2014). A baseline study characterising the municipal solid waste in the State of Kuwait. Waste Manag. 34, 952–960. doi: 10.1016/j.wasman.2014.02.015
Amasuomo, E., and Baird, J. (2017). Solid waste management trends in Nigeria. Br. J. Environ. Sci. 5, 25–37. doi: 10.5539/jms.v6n4p35
Amber, I., Kulla, D. M., and Gukop, N. (2012). Generation, characteristics and energy potential of solid municipal waste in Nigeria. J. Energy S. Afr. 23, 47–51. doi: 10.17159/2413-3051/2012/v23i3a3172
ASTM International, ASTM D 5231–92 standard test method for determination of the composition of unprocessed municipal solid waste. (1992).
Ayilara, M. S., Olanrewaju, O., Babalola, O., and Odeyemi, O. (2020). Waste management through composting: challenges and potentials. Sustain. For. 12:4456. doi: 10.3390/su12114456
Babatunde, B., Vincent-Akpu, I. F., Woke, G. N., Atarhinyo, E., Aharanwa, U. C., Green, A. F., et al. (2013). Comparative analysis of municipal solid waste (MSW) composition in three local government areas in Rivers state, Nigeria. Afr. J. Environ. Sci. Technol. 7, 874–881.
Beka, D. D., and Meng, X.-Z. (2021). Redesign solid waste collection and transference system for Addis Ababa (Ethiopia) based on the comparison with Shanghai, China. Open Access Libr. J. 8, 1–23. doi: 10.4236/oalib.1107470
Bello, A. S., Al-Ghouti, M. A., and Abu-Dieyeh, M. H. (2022). Sustainable and long-term municipal solid waste management: a review. Bioresour. Technol. Rep. 18:101067. doi: 10.1016/j.biteb.2022.101067
Bhuiya, M., Rasul, M. G., Khan, M. M. K., and Ashwath, N. (2020). Biodiesel production and characterisation of poppy (Papaver somniferum L.) seed oil methyl ester as a source of 2nd generation biodiesel feedstock. Ind. Crop. Prod. 152:112493. doi: 10.1016/j.indcrop.2020.112493
Bisinella, V., Götze, R., Conradsen, K., Damgaard, A., Christensen, T. H., and Astrup, T. F. (2017). Importance of waste composition for life cycle assessment of waste management solutions. J. Clean. Prod. 164, 1180–1191. doi: 10.1016/j.jclepro.2017.07.013
Cao, G., Guo, C., and Li, H. (2022). Risk analysis of public–private partnership waste-to-energy incineration projects from the perspective of rural revitalisation. Sustain. For. 14:8205. doi: 10.3390/su14138205
Cheela, V. R. S., Goel, S., John, M., and Dubey, B. (2021). Characterization of municipal solid waste based on seasonal variations, source and socio-economic aspects. Waste Dispos. Sustain. Energy 3, 275–288. doi: 10.1007/s42768-021-00084-x
Debrah, J. K., Vidal, D. G., and Dinis, M. A. P. (2021). Raising awareness on solid waste management through formal education for sustainability: a developing countries evidence review. Recycling 6:6. doi: 10.3390/recycling6010006
Di Foggia, G., and Beccarello, M. (2023). Sustainability pathways in European waste management for meeting circular economy goals. Environ. Res. Lett. 18:124001. doi: 10.1088/1748-9326/ad067f
Dickson, E. M., Hastings, A., and Smith, J. (2023). Energy production from municipal solid waste in low to middle-income countries: a case study of how to build a circular economy in Abuja, Nigeria. Front. Sustain. 4:1173474. doi: 10.3389/frsus.2023.1173474
Edjabou, M. E., Jensen, M. B., Götze, R., Pivnenko, K., Petersen, C., Scheutz, C., et al. (2015). Municipal solid waste composition: sampling methodology, statistical analyses, and case study evaluation. Waste Manag. 36, 12–23. doi: 10.1016/j.wasman.2014.11.009
Ezechi, E. H., Nwabuko, C. G., Enyinnaya, O. C., and Babington, C. J. (2017). Municipal solid waste management in aba, Nigeria: challenges and prospects. Environ. Eng. Res. 22, 231–236. doi: 10.4491/eer.2017.100
Ezeudu, O. B., Agunwamba, J. C., Ugochukwu, U. C., and Ezeudu, T. S. (2021). Temporal assessment of municipal solid waste management in Nigeria: prospects for circular economy adoption. Rev. Environ. Health 36, 327–344. doi: 10.1515/reveh-2020-0084
Faisal, M. N., Talib, F., and Khurrum Bhutta, M. (2019). Enablers of sustainable municipal solid waste management system in India. Int. J. Environ. Waste Manag. 23, 213–237. doi: 10.1504/IJEWM.2019.099002
Farooq, A., Haputta, P., Silalertruksa, T., and Gheewala, S. H. (2021). A framework for selecting suitable waste-to-energy technologies for a sustainable municipal solid waste management system. Front. Sustain. 2:27. doi: 10.3389/frsus.2021.681690
Fernando, S. J., and Zutshi, A. (2023). Municipal solid waste management in developing economies: a way forward. Clean. Waste Syst. 5:100103. doi: 10.1016/j.clwas.2023.100103
Ferronato, N., and Torretta, V. (2019). Waste mismanagement in developing countries: a review of global issues. Int. J. Environ. Res. Public Health 16:1060. doi: 10.3390/ijerph16061060
Finet, C. (1987). Heating value of municipal solid waste. Waste Manag. Res. 5, 141–145. doi: 10.1177/0734242X8700500117
Foster, W., Azimov, U., Gauthier-Maradei, P., Molano, L. C., Combrinck, M., Munoz, J., et al. (2021). Waste-to-energy conversion technologies in the UK: processes and barriers–a review. Renew. Sust. Energ. Rev. 135:110226. doi: 10.1016/j.rser.2020.110226
Gherheş, V., Fărcaşiu, M. A., and Para, I. (2022). Environmental problems: an analysis of students’ perceptions towards selective waste collection. Front. Psychol. 12:803211. doi: 10.3389/fpsyg.2021.803211
Giang, H. M. A study on development methodology of sustainable solid waste management system by using multi-objective decision making model-a case study in Hoi An City, Vietnam. Doctor of Philosophy thesis. Graduate School of Environmental and Life Science. Vietnam: Okayama University. (2017).
Guerrero, L. A., Maas, G., and Hogland, W. (2013). Solid waste management challenges for cities in developing countries. Waste Manag. 33, 220–232. doi: 10.1016/j.wasman.2012.09.008
Gutiérrez, A. S., Mendoza Fandiño, J. M., and Cabello Eras, J. J. (2021). Alternatives of municipal solid wastes to energy for sustainable development. The case of Barranquilla (Colombia). Int. J. Sustain. Eng. 14, 1809–1825. doi: 10.1080/19397038.2021.1993378
Hemidat, S., Achouri, O., el Fels, L., Elagroudy, S., Hafidi, M., Chaouki, B., et al. (2022). Solid waste management in the context of a circular economy in the MENA region. Sustain. For. 14:480. doi: 10.3390/su14010480
Hoang, A. T., Varbanov, P. S., Nižetić, S., Sirohi, R., Pandey, A., Luque, R., et al. (2022). Perspective review on municipal solid waste-to-energy route: characteristics, management strategy, and role in the circular economy. J. Clean. Prod. 359:131897. doi: 10.1016/j.jclepro.2022.131897
Hoornweg, D., and Bhada-Tata, P. (2012). What a waste: a global review of solid waste management. Urban development series; knowledge papers no. 15. ©World Bank, Washington, DC. Available at: Urban development series; knowledge papers no. 15. ©World Bank, Washington, DC. License: CC BY 3.0 IGO.
Hoornweg, D., and Thomas, L. (1999). What a waste: Solid waste management in Asia. Washington, D.C, USA: The World Bank.
Ibikunle, R., Titiladunayo, I. F., Akinnuli, B. O., Dahunsi, S. O., and Olayanju, T. M. A. (2019). Estimation of power generation from municipal solid wastes: a case study of Ilorin metropolis, Nigeria. Energy Rep. 5, 126–135. doi: 10.1016/j.egyr.2019.01.005
Ibikunle, R., Titiladunayo, I. F., Uguru-Okorie, D. C., Osueke, C. O., and Olayanju, A. (2019). Predicting the quantity of municipal solid waste required for power generation using power plant design parameters. J. Phys. Conf. Ser. 1378:032090. doi: 10.1088/1742-6596/1378/3/032090
Iyamu, H., Anda, M., and Ho, G. (2022). Exploring the multi-level perspective in municipal solid waste management transition. Habitat Int. 129:102664. doi: 10.1016/j.habitatint.2022.102664
Jaunich, M. K., Levis, J. W., DeCarolis, J. F., Gaston, E. V., Barlaz, M. A., Bartelt-Hunt, S. L., et al. (2016). Characterization of municipal solid waste collection operations. Resour. Conserv. Recycl. 114, 92–102. doi: 10.1016/j.resconrec.2016.07.012
Kalkanis, K., Alexakis, D. E., Kyriakis, E., Kiskira, K., Lorenzo-Llanes, J., Themelis, N. J., et al. (2022). Transforming waste to wealth, achieving circular economy. Circ. Econ. Sustain. 2, 1541–1559. doi: 10.1007/s43615-022-00225-2
Karak, T., Bhagat, R., and Bhattacharyya, P. (2012). Municipal solid waste generation, composition, and management: the world scenario. Crit. Rev. Environ. Sci. Technol. 42, 1509–1630. doi: 10.1080/10643389.2011.569871
Kaza, S., Yao, L. C., Bhada-Tata, P., and Van Woerden, F. (2018). What a waste 2.0: a global snapshot of solid waste management to 2050. Washington, DC: World Bank Publications.
Kazancoglu, I., Sagnak, M., Kumar Mangla, S., and Kazancoglu, Y. (2021). Circular economy and the policy: a framework for improving the corporate environmental management in supply chains. Bus. Strateg. Environ. 30, 590–608. doi: 10.1002/bse.2641
Khuriati, A., Budi, W., Nur, M., Istadi, I., and Suwoto, G. (2017). Modeling municipal solid waste's heating value based on ultimate analysis using stepwise multiple linear regression in Semarang. ARPN J. Eng. Appl. Sci. 12, 2870–2876.
Kothari, R., Tyagi, V. V., and Pathak, A. (2010). Waste-to-energy: a way from renewable energy sources to sustainable development. Renew. Sust. Energ. Rev. 14, 3164–3170. doi: 10.1016/j.rser.2010.05.005
Kumar, V., and Garg, N. (2021). National and regional waste stream in the United States: conformance and disparity. Environ. Res. 1:031002. doi: 10.1088/2634-4505/ac36bd
Lara-Topete, G. O., Yebra-Montes, C., Orozco-Nunnelly, D. A., Robles-Rodríguez, C. E., and Gradilla-Hernández, M. S. (2022). An integrated environmental assessment of MSW management in a large developing country city: taking the first steps towards a circular economy model. Front. Environ. Sci. 10:838542. doi: 10.3389/fenvs.2022.838542
Letshwenyo, M. W., and Kgetseyamore, D. (2020). Generation and composition of municipal solid waste: case study, extension 7, Palapye, Botswana. SN Appl. Sci. 2, 1–8. doi: 10.1007/s42452-020-03496-2
Listiningrum, P., Dahlan, M., Anwary, M. A. Z. A., Wahyuni, H. S., and Bachtiar, R. (2023). Waste management without direction in Indonesia: a proposed legal reform towards intelligent cities. Legality 31, 224–244. doi: 10.22219/ljih.v31i2.27375
Liu, J.-I., Paode, R. D., and Holsen, T. M. (1996). Modeling the energy content of municipal solid waste using multiple regression analysis. J. Air Waste Manage. Assoc. 46, 650–656. doi: 10.1080/10473289.1996.10467499
Lorenzo Llanes, J., and Kalogirou, E. (2019). Waste-to-energy conversion in Havana: technical and economic analysis. Soc. Sci. 8:119. doi: 10.3390/socsci8040119
Lu, J.-W., Zhang, S., Hai, J., and Lei, M. (2017). Status and perspectives of municipal solid waste incineration in China: a comparison with developed regions. Waste Manag. 69, 170–186. doi: 10.1016/j.wasman.2017.04.014
Luo, C., Ju, Y., Dong, P., Gonzalez, E. D. R. S., and Wang, A. (2021). Risk assessment for PPP waste-to-energy incineration plant projects in China based on hybrid weight methods and weighted multigranulation fuzzy rough sets. Sustain. Cities Soc. 74:103120. doi: 10.1016/j.scs.2021.103120
Mandpe, A., Paliya, S., Gedam, V. V., Patel, S., Tyagi, L., and Kumar, S. (2023). Circular economy approach for sustainable solid waste management: a developing economy perspective. Waste Manag. Res. 41, 499–511. doi: 10.1177/0734242X221126718
Mehdi Hassan, A. M., Asif, M., al-Mansur, M. A., Ripaj Uddin, M., Alsufyani, S. J., Yasmin, F., et al. (2023). Characterization of municipal solid waste for effective utilisation as an alternative source for clean energy production. J. Radiat. Res. Appl. Sci. 16:100683. doi: 10.1016/j.jrras.2023.100683
Miezah, K., Obiri-Danso, K., Kádár, Z., Fei-Baffoe, B., and Mensah, M. Y. (2015). Municipal solid waste characterisation and quantification as a measure towards effective waste management in Ghana. Waste Manag. 46, 15–27. doi: 10.1016/j.wasman.2015.09.009
Mousania, Z., Rafiee, R., Moeinaddini, M., and Atkinson, J. D. (2023). Anaerobic digestion of the organic fraction of municipal solid waste in a simulated bioreactor to improve predictive modelling of landfill systems. J. Hazard. Mater. Adv. 13:100396. doi: 10.1016/j.hazadv.2023.100396
Nadeem, K., Farhan, K., and Ilyas, H. (2016). Waste amount survey and physio-chemical analysis of municipal solid waste generated in Gujranwala-Pakistan. Int. J. Waste Resour. 6, 196–204. doi: 10.4172/2252-5211.1000196
Nnaji, C. C. (2015). Status of municipal solid waste generation and disposal in Nigeria. Manag. Environ. Q. 26, 53–71. doi: 10.1108/MEQ-08-2013-0092
Nordi, G. H., Palacios-Bereche, R., Gallego, A. G., and Nebra, S. A. (2017). Electricity production from municipal solid waste in Brazil. Waste Manag. Res. 35, 709–720. doi: 10.1177/0734242X17705721
Nwankpa, S., and Scandrett, E. (2020). History and contexts of municipal solid waste management in Aba–recounting the stories of residents. J. Environ. Earth Sci. 10, 61–74. doi: 10.7176/JEES/10-9-06
Nzihou, J. F., Hamidou, S., Bouda, M., Koulidiati, J., and Segda, B. (2014). Using Dulong and Vandralek formulas to estimate the calorific heating value of a household waste model. Int. J. Sci. Eng. Res. 5, 1878–1883.
Oba, A., George, A., Amgbara, T., and Onungwe, I. (2015). Waste to wealth; the utilization of scrap Tyre as aggregate in bituminous mixes for road construction. Int. J. Engg. Res. Apps. 5:6.
Odoemene, U. D., and Ofodu, J. (2016). Solid wastes management in aba Metropolis. Int. J. Adv. Acad. Res 2, 1–7.
Oladejo, O., Abiola, A. O., Olanipekun, A. A., Ajayi, O. E., and Onokwai, A. O. (2020). Energy potential of solid waste generated in landmark university, Omu-Aran, Kwara state, Nigeria. LAUTECH J. Civil. Environ. Stud. 5, 43–52. doi: 10.36108/laujoces/0202/50(0150)
O'Neill, A. (2022). Population growth in Nigeria 2021. Available at: Statista.com: https://www.statista.com/statistics/382235/population-growth-in-nigeria/.
Ontario Environment (2017). Strategy for a waste-free Ontario building the circular economy. Ministry of the Environment, Conservation, and Parks. Available at: https://files.ontario.ca/finalstrategywastefreeont_eng_aoda1_final-s.pdf (Accessed February 08, 2023).
Onungwe, I., Hunt, D. V. L., and Jefferson, I. (2021). Estimating energy content and characterization of municipal solid waste using ®Sowaclink. in 3rd Annual Conference on Transforming Solid Waste To Renewable Energy, October 29–30. Rivers State University, Port Harcourt, Rivers State, Nigeria: The Nigerian Institution of Environmental Engineers (NIEE) Port Harcourt Chapter (A Division of The Nigerian Society of Engineers).
Onungwe, I., Hunt, D. V., and Jefferson, I. (2023). Transition and implementation of circular economy in municipal solid waste management system in Nigeria: a systematic review of the literature. Sustain. For. 15:12602. doi: 10.3390/su151612602
Orhorhoro, E. K., Ebunilo, P. O., and Sadjere, E. (2017). Determination and quantification of household solid waste generation for planning suitable sustainable waste management in Nigeria. Int. J. Emergi. Eng. Res. Technol. 5, 1–9.
Orlov, A., Klyuchnikova, E., and Korppoo, A. (2021). Economic and environmental benefits from municipal solid waste recycling in the Murmansk region. Sustain. For. 13:10927. doi: 10.3390/su131910927
Otumawu-Apreku, K. (2020). Solid waste management: a socio-economic perspective of urban and Peri-urban communities in Honiara. Int. J. Environ. Sci. Nat. Resour. 25, 180–192. doi: 10.19080/IJESNR.2020.26.556173
Owamah, I. H., Izinyon, O. C., and Igbinewekan, P. (2017). Characterization and quantification of solid waste generation in the Niger Delta region of Nigeria: a case study of Ogbe-Ijoh community in Delta state. J. Mater. Cycl. Waste Manag. 19, 366–373. doi: 10.1007/s10163-015-0426-3
Oyelola, O., Ajiboshin, I., and Okewole, J. (2017). Effective solid waste management in the circular economy: the case study of Lagos state, Nigeria. Proc. Environ. Sci. Eng. Manag. 4, 143–148.
Ozcan, H. K., Guvenc, S., Guvenc, L., and Demir, G. (2016). Municipal solid waste characterisation according to different income levels: a case study. Sustain. For. 8:1044. doi: 10.3390/su8101044
Pan, S.-Y., du, M. A., Huang, I. T., Liu, I. H., Chang, E. E., and Chiang, P. C. (2015). Strategies on implementing waste-to-energy (WTE) supply chain for the circular economy system: a review. J. Clean. Prod. 108, 409–421. doi: 10.1016/j.jclepro.2015.06.124
Pasek, A. D., Gultom, K. W., and Suwono, A. (2013). Feasibility of recovering energy from municipal solid waste to generate electricity. J. Eng. Technol. Sci 45, 241–256. doi: 10.5614/j.eng.technol.sci.2013.45.3.3
Pathak, D. R., Mainali, B., Abuel-Naga, H., Angove, M., and Kong, I. (2020). Quantification and characterisation of the municipal solid waste for sustainable waste management in newly formed municipalities of Nepal. Waste Manag. Res. 38, 1007–1018. doi: 10.1177/0734242X20922588
Peavy, H., Rowe, D. R., and Tchobanoglous, H. (2010). Environmental engineering. McGraw-Hill, New York, NY.
Rafey, A., Prabhat, K., and Samar, M. (2020). Comparison of technologies to serve waste to energy conversion. Int. J. Waste Resour 10:372. doi: 10.35248/2252-5211.20.10.372
Reike, D., Vermeulen, W. J. V., and Witjes, S. (2018). The circular economy: new or refurbished as CE 3.0? - Exploring controversies in the conceptualization of the circular economy through a focus on history and resource value retention options. Resour. Conserv. Recycl. 135, 246–264. doi: 10.1016/j.resconrec.2017.08.027
Rezania, S., Oryani, B., Nasrollahi, V. R., Darajeh, N., Lotfi Ghahroud, M., and Mehranzamir, K. (2023). Review on waste-to-energy approaches toward a circular economy in developed and developing countries. PRO 11:2566. doi: 10.3390/pr11092566
Rominiyi, O., Fapetu, O., Owolabi, J., and Adaramola, B. (2017). Determination of energy content of the municipal solid waste of ado-Ekiti metropolis, southwest, Nigeria. Curr. J. Appl. Sci. Technol. 23, 1–11. doi: 10.9734/CJAST/2017/32340
Roy, P., Mohanty, A. K., Dick, P., and Misra, M. (2023). A review on the challenges and choices for food waste valorisation: environmental and economic impacts. ACS Environ. Au 3, 58–75. doi: 10.1021/acsenvironau.2c00050
Salami, H., Adegite, J. O., Bademosi, T. T., Lawal, S. O., Olutayo, O. O., and Olowosokedile, O. (2018). A review on the current status of municipal solid waste management in Nigeria: problems and solutions. J. Eng. Res. Rep. 3, 1–16. doi: 10.9734/jerr/2018/v3i416884
Salem, K. S., Clayson, K., Salas, M., Haque, N., Rao, R., Agate, S., et al. (2023). A critical review of existing and emerging technologies and systems to optimise solid waste management for feedstocks and energy conversion. Matter 6, 3348–3377. doi: 10.1016/j.matt.2023.08.003
Salguero-Puerta, L., Leyva-Díaz, J. C., Cortés-García, F. J., and Molina-Moreno, V. (2019). Sustainability indicators concerning waste management for implementing the circular economy model on the University of Lome (Togo) campus. Int. J. Environ. Res. Public Health 16:2234. doi: 10.3390/ijerph16122234
Sánchez, A., Gabarrell, X., Artola, A., Barrena, R., Colón, J., Font, X., et al. (2016). “Composting of wastes” in Resource recovery to approach zero municipal waste. Eds. M. J. Taherzadeh and T. Richards, 77–116.
Seshie, V. I. (2020). Municipal solid waste characterisation and quantification as a measure towards effective waste management in the Takoradi Sub-Metro, Ghana. Ghana Min. J. 20, 86–98.
Sharma, K. D., and Jain, S. (2020). Municipal solid waste generation, composition, and management: the global scenario. Soc. Responsib. J. 16, 917–948. doi: 10.1108/SRJ-06-2019-0210
Siami, L., Sotiyorini, T., and Janah, N. M. (2019). Municipal solid waste quantification and characterization in Banyuwangi, Indonesia. Indon. J. Urb. Environ. Technol. 2, 189–200. doi: 10.25105/urbanenvirotech.v0i0.4359
Sincero, A. P., and Sincero, G. A. (1996). Environmental engineering: a design approach. Prentice Hall, Hoboken, New Jersey, U.S.A: Pearson College Division.
Smol, M., Duda, J., Czaplicka-Kotas, A., and Szołdrowska, D. (2020). Transformation towards circular economy (CE) in municipal waste management system: model solutions for Poland. Sustain. For. 12:4561. doi: 10.3390/su12114561
Smyth, D. P., Fredeen, A. L., and Booth, A. L. (2010). Reducing solid waste in higher education: the first step towards ‘greening university campus. Resour. Conserv. Recycl. 54, 1007–1016. doi: 10.1016/j.resconrec.2010.02.008
Somorin, T. O., Adesola, S., and Kolawole, A. (2017). State-level assessment of the waste-to-energy potential (via incineration) of municipal solid wastes in Nigeria. J. Clean. Prod. 164, 804–815. doi: 10.1016/j.jclepro.2017.06.228
Tchobanoglous, G., and Kreith, F. (2002). Handbook of solid waste management. New York: McGraw-Hill Education.
Titiladunayo, I., Akinnuli, B. O., and Ibikunle, R. A. (2018). Analysis of combustible municipal solid waste fractions as fuel for energy production: exploring its physicochemical and thermal characteristics. Int. J. Civil. Eng. Technol. 13, 1557–1575.
Triassi, M., de Simone, B., Montuori, P., Russo, I., de Rosa, E., di Duca, F., et al. (2023). Determination of residual municipal solid waste composition from rural and urban areas: a step toward the optimization of a waste management system for efficient material recovery. Sustain. For. 15:13378. doi: 10.3390/su151813378
Tsai, F. M., Bui, T. D., Tseng, M. L., Lim, M. K., and Hu, J. (2020). Municipal solid waste management in a circular economy: a data-driven bibliometric analysis. J. Clean. Prod. 275:124132. doi: 10.1016/j.jclepro.2020.124132
Ugwu, C. O., Ozoegwu, C. G., and Ozor, P. A. (2020). Solid waste quantification and characterisation in the University of Nigeria, Nsukka campus, and recommendations for sustainable management. Heliyon 6:e04255. doi: 10.1016/j.heliyon.2020.e04255
Ugwu, C. O., and Ozor, P. A. (2021). On suitable solid waste characterization method for Nigerian University Campuses. Proceedings of the 2nd African International Conference on Industrial Engineering and Operations Management Harare, Zimbabwe, December 7-10, 2020.
Van Ewijk, S., and Stegemann, J. (2023). An introduction to waste management and circular economy. London: UCL Press.
Van Fan, Y., Klemeš, J. J., Lee, C. T., and Perry, S. (2018). Anaerobic digestion of municipal solid waste: energy and carbon emission footprint. J. Environ. Manag. 223, 888–897. doi: 10.1016/j.jenvman.2018.07.005
Van Kruchten, S., and Van Eijk, F., (2020). Circular economy & SDGs–how circular economy practices help to achieve the sustainable development goals : The Netherlands. The Netherlands Enterprise Agency.
Vanson, G., Marangé, P., and Levrat, E. (2022). End-of-life decision making in the circular economy using generalised coloured stochastic petri nets. Auton. Intell. Sys. 2:3. doi: 10.1007/s43684-022-00022-6
Varjani, S., Shahbeig, H., Popat, K., Patel, Z., Vyas, S., Shah, A. V., et al. (2022). Sustainable municipal solid waste management through waste-to-energy technologies. Bioresour. Technol. 355:127247. doi: 10.1016/j.biortech.2022.127247
Wang, W., Chu, Z., and Zhang, T. (2022). Synergy degree evaluation of stakeholder engagement in integrated municipal solid waste management: a case study in Harbin, China. Energies 15:5000. doi: 10.3390/en15145000
Wilson, B., Williams, E. P., Liss, B., and Wilson, B. (2013). A comparative assessment of commercial technologies for conversion of solid waste to energy. EnviroPower Renewable: Boca Raton, FL.
Wong, N. W. (2016). Advocacy coalitions and policy change in China: a case study of anti-incinerator protest in Guangzhou. Volunt. Int. J. Volunt. Nonprofit Org. 27, 2037–2054. doi: 10.1007/s11266-015-9603-1
Yakah, N., Samavati, M., Akuoko Kwarteng, A., Martin, A., and Simons, A. (2023). Prospects of waste incineration for improved municipal solid waste (MSW) management in Ghana—a review. Clean. Technol. 5, 997–1011. doi: 10.3390/cleantechnol5030050
Yusoff, M. S., Kamaruddin, M. A., Aziz, H. A., Adlan, M. N., Zaman, N. Q., and Mahmood, N. Z. (2018). Municipal solid waste composition, characterisation and recyclables potential: a case study evaluation in Malaysia. J. Solid Waste Technol. Manag. 44, 330–343. doi: 10.5276/JSWTM.2018.330
Zabaleta, I., Mertenat, A., Scholten, L., and Zurbrügg, C. (2020). Selecting organic waste treatment technologies. SOWATT: Eawag: Swiss Federal Institute of Aquatic Science and Technology, Dübendorf, Switzerland.
Zamri, M., Hasmady, S., Akhiar, A., Ideris, F., Shamsuddin, A. H., Mofijur, M., et al. (2021). A comprehensive review on anaerobic digestion of organic fraction of municipal solid waste. Renew. Sust. Energ. Rev. 137:110637. doi: 10.1016/j.rser.2020.110637
Zhou, H., Meng, A. H., Long, Y. Q., Li, Q. H., and Zhang, Y. G. (2014). An overview of characteristics of municipal solid waste fuel in China: physical, chemical composition and heating value. Renew. Sust. Energ. Rev. 36, 107–122. doi: 10.1016/j.rser.2014.04.024
Keywords: characterization, circular economy, quantification, software, municipal solid waste, transition, sorting
Citation: Onungwe I, Hunt DVL and Jefferson I (2024) Transitioning towards circular economy through municipal solid waste analysis and characterisation using SowaCLINK software. Front. Sustain. 5:1321329. doi: 10.3389/frsus.2024.1321329
Edited by:
Alberto Paucar-Caceres, Manchester Metropolitan University, United KingdomReviewed by:
Rahul Baidya, Institute of Engineering and Management (IEM), IndiaGiacomo Di Foggia, University of Milano-Bicocca, Italy
Copyright © 2024 Onungwe, Hunt and Jefferson. This is an open-access article distributed under the terms of the Creative Commons Attribution License (CC BY). The use, distribution or reproduction in other forums is permitted, provided the original author(s) and the copyright owner(s) are credited and that the original publication in this journal is cited, in accordance with accepted academic practice. No use, distribution or reproduction is permitted which does not comply with these terms.
*Correspondence: Ishmael Onungwe, aXhvOTU4QGJoYW0udWsuYWM=