- Institute of Environmental and Biological Sciences, School of Biological Science, University of Aberdeen, Aberdeen, United Kingdom
The volume of municipal solid waste produced in many cities in low to middle income countries exceeds the capacity of handling facilities causing environmental and health risks. This study provides the most comprehensive analysis to date of municipal solid waste to energy options for Abuja, Nigeria. We use most recently available data on waste generation from Abuja municipal authorities, integrated with secondary data from the literature. This is combined with standard relationships between waste composition and energy production to compare the potential for thermochemical conversion and anaerobic digestion to meet the energy requirement of Abuja. In addition, we use assumptions about digestate production and nitrogen release to estimate the potential production of organic fertilizer from digestate. The organic fraction of municipal solid waste from Abuja [7.1 × 107 (±4 × 106) kg y−1] has potential to produce 3.6 × 106 (±2 × 105) m3 y−1 biogas. This could provide 7.8 × 103 (±4 × 102) MWh y−1 electricity, equivalent to 8% of annual electricity requirement in Abuja, 4.0 × 107 (±2 × 106) MJ y−1 heat, and 5.3 × 105 (±3 × 104) kg y−1 digestate use as bio-fertilizer from organic fraction only. Potential power generation by thermochemical conversion for combined heat and electricity is much greater, 7.73 × 104 (±4 × 103) MWh y−1, equivalent to 83% of power requirement in Abuja, but does not produce bio-fertilizer. Using the organic fraction for anaerobic digestion and the remaining waste for thermochemical conversion provides combined heat and energy that is 91.5% of Abuja power requirements, while also producing digestate. Barriers to implementation include informal waste recyclers with poor collection and handling procedures, absence of public education, weak environmental policies and lack of funding. There is a need for periodic review of policies and waste legislation to create a circular economy in Abuja.
1. Introduction
Globally, there is an exponential rise in energy consumption; fossil fuels currently contribute ~88% of this energy demand with a projected 50% rise in global energy consumption by 2050 (Obidike et al., 2020). Fossil fuel consumption in Abuja, Nigeria, is currently 5.21 × 106 kW d−1 (Scarlat et al., 2015; Obidike et al., 2020; Biodun et al., 2021) so alternative energy sources are needed, both to meet demand (Ezeah and Roberts, 2010; Biodun et al., 2021) and to reduce greenhouse gas (GHG) emissions from fossil fuels (Afon and Okewole, 2007; Arukwe et al., 2012; Biodun et al., 2021). An annual increase in GHG emissions of ~3.79 × 1010 t CO2e globally was reported in 2022, primarily due to fossil fuel combustion, with carbon dioxide (CO2) being the largest contributor (Biodun et al., 2021; EDGAR, 2022). One potential alternative to fossil fuels is to use municipal solid waste (MSW) to provide energy, through thermochemical conversion or production of biogas by anaerobic digestion (AD) (Barati et al., 2017; Rajaeifar et al., 2017; Aghbashlo et al., 2019; Kazemi et al., 2019).
The accumulation of MSW is a major environmental and socio-economic problem in many cities in developing countries. This increase in MSW generation is mostly associated with the growth in urban population due to massive human migration from rural to urban centers (Bongaarts, 2009; Obidike et al., 2020). Management of MSW in Abuja follows a routine process of collection, transportation and open dumping at designated dumpsites (Ezeah and Roberts, 2010; Scarlat et al., 2015). However, there is an absence of planned waste recycling and energy recovery through any centralized MSW management system (Arukwe et al., 2012). Data from 2000 to 2020 indicates that Abuja city has an average solid waste generation of about 1.51 × 105 t y−1, out of which the organic content constitutes ~47% (AEPB, 2020). Therefore, there is large potential for energy generation from these wastes.
In Abuja, private waste collection services provide house-to-house waste collection (Ogwueleka, 2009). However, the introduction of a tipping fee means they often dump the waste at the side of the road instead of using the designated waste collection points, which are organized by the Abuja Environmental Protection Board (Ajani, 2008). The Abuja MSW system is characterized by inefficient collection methods, inadequate coverage of collection points and inappropriate disposal systems (Ogwueleka, 2009). Arukwe et al. (2012) suggested that this is a direct result of not having waste separation implemented at the point of collection, and weak individual and political will to drive waste management policies and enforcement. Others also include absence of modern waste management technologies, poor monitoring and control operation, low levels of household income and lack of effective environmental education and awareness (Arukwe et al., 2012). Afon and Okewole (2007) concluded that education, income level and social status control per capita generation of MSW in Abuja (Afon and Okewole, 2007). Ajani (2008) suggested that age, location, occupation and the waste collection tipping fee affects collection services of both private and public sectors (Ajani, 2008). Increased generation of MSW in Abuja, therefore, poses a serious potential risk to the environment, socio-economic conditions and health of the inhabitants if management strategies are not urgently improved.
In addition, waste dumpsites negatively impact environmental and health conditions for local residents through release of contaminants into the wider environment (Arukwe et al., 2012). Release of hazardous substances such as bisphenol-A, phthalates and non-ionic surfactants, which include perfluorinated compounds, alkylphenol polyethoxylates, pharmaceuticals and personal care products, has been linked to contaminants detected in biota and environmental matrices in aquatic environments (Yeung et al., 2009; Murray et al., 2010).
Thermochemical technologies, such as combined heat and power (CHP) and incineration, can be suitable for energy generation especially from the non-degradable MSW. Combined heat and power is an integrated system of waste treatment, designed to generate electricity and heat simultaneously by waste combustion. Hot flue gases generate steam, which is used in the plant to turn turbines for electricity generation; this is typical of the existing CHP topping cycle (Portugal-Pereira and Lee, 2015). Industries or local communities also benefit from the recovered heat through extra thermal energy provision (Aldrich et al., 2011). However, waste combustion is associated with many negative effects, such as pungent odors, surface and ground water contamination, GHG emissions, topographical instability and risks of fire outbreaks, although these could all be mitigated by good site design, such as leachate collection and treatment, adequate liner provision and coverage of incinerators (Song and Li, 2014; Yadav et al., 2018). An advantage of incineration is the reduction in MSW volume to between 80 and 90% of the original volume (Ezeah and Roberts, 2012).
Anaerobic digestion is an effective waste-to-energy technology that is currently in-use in many developed and developing countries (Eddine and Salah, 2012; Vogeli et al., 2014), and could provide a less polluting option for waste-to-energy generation. It is a process of biochemical conversion that generates biogas through an oxygen free digester. It is widely used for management of both wet and dry waste resources and for energy recovery purposes. Biogas is a colorless, non-toxic, combustible gas composed mainly of methane (CH4) and carbon dioxide (CO2) with about 1–5% other gases (Deressa et al., 2015; Amasuomo and Baird, 2016).
A number of recent studies have considered the potential for use of the organic fraction of MSW (OFMSW) for energy generation in cities in developing countries (e.g., Aguilar-Virgen et al., 2014; Gebreegziabher et al., 2014; Kumar and Samadder, 2017; Hettiarachchi et al., 2018; Amo-Asamoah et al., 2020; Dehkordi et al., 2020; Paul and Soren, 2020; Rolewicz-Kalinska et al., 2020; Ribeiro et al., 2021; Chaianong and Pharino, 2022). Analysis of the potential energy generation of OFMSW in developing countries suggests significant energy generation, economic and environmental benefits through AD; for example 8.5 × 103 kWh y−1 with a value of 1.13 × 106 US$ y−1 in Guadalajara, Mexico (Ibarra-Esparza et al., 2022) and 2.2 × 109 kWh y−1 with a value of 2.2 × 108 US$ y−1 across Malaysia (Khairuddin et al., 2015). Moya et al. (2017) estimated that the thermochemical potential for energy generation in Quito, Ecuador, was 5.97 × 103 kWh t−1 while the biochemical potential was 6.2 × 101 kWh t−1. Others have assessed the challenges associated with biogas production in developing countries, highlighting key barriers as lack of appropriate segregation facilities and inconsistent characteristics and supply of wastes (Yadav et al., 2022). Pandyaswargo et al. (2019) developed an implementation framework to help planning of sustainable municipal organic waste facilities.
The aim of this study is to evaluate the potential for energy production from MSW in Abuja using both thermochemical and AD technologies. The novelty of this study is that it provides a comprehensive analysis of the waste-to-energy value chain in Abuja city, integrating the socio-environmental and economic impacts of MSW in sustainability of energy provision that could be used for waste legislation reviews by policy makers more widely in developing countries. We consider factors impacting the rate of MSW production, determine factors affecting composition of the MSW, and estimate the environmental and socio-economic benefits of energy production from MSW in Abuja. Finally, we provide recommendations for enhancement of energy generation from MSW in Abuja and the establishment of a circular economy. This represents the most comprehensive analysis to date of the potential in Abuja for energy production from MSW. While the study provides recommendations specific to the case study of Abuja, a similar approach could be applied across Nigeria and more widely in low to middle income countries.
2. Method and materials
2.1. Study focus: Abuja
Abuja is the federal capital city of Nigeria. It is sub-divided into six area councils for administrative purposes; these include Abuja municipal area council, Bwari, Kuje, Gwagwalada, Abaji, and Kwali. It was established in 1976 and is situated north of the confluence of rivers Benue and Niger, between latitudes 7° 25′N and 9° 20′N and longitudes 5° 45′E and 7° 39′E. Abuja occupies a land area of 7,753.85 km2 with an estimated population of 3,324,000 in 2020 (National Population Commission Nigeria, 2014; United Nations Fund for Population Activities-UNFPA, 2015; Aderoju and Guerner, 2020). It has two main seasons in a year, in common with the climate of Nigeria as a whole; the dry and wet (rainy) seasons. The dry season is normally between November and March which constitute months of lowest rainfall and humidity, while wet or rainy season is from April to October, with highest mean rainfall of ~119 mm in August and mean humidity of ~58% (Aderoju et al., 2018). The population of Abuja is projected to exceed 5.8 million by 2026, based on a 2001 population of 1,724,205 (National Population Commission Nigeria, 2014). This, therefore, poses serious environmental and socio-economic challenges to the city, including MSW management. Note that this study is limited to Abuja capital city under the Municipal Area Council, so data considered here, such as the human population and waste generation, are also limited to this area.
2.2. Socio-environmental and economic analysis
In order to provide an analysis of the potential socio-environmental and economic impact of energy production from MSW in Abuja, data were collated from a wide range of sources. Data were collected from Nigerian government-agencies on MSW and from government agency waste reports (AEPB, 2020). Secondary data were obtained from the peer reviewed literature, and from workshop and seminar presentations. The standard measure from the World Bank 2020 was used to determine MSW generation for Abuja capital city (Table 1). These data indicate that the rate of MSW generation ranges from an average over the year of 0.406 kg per capita per day in Garki I & II to 0.633 kg per capita per day in Maitama. Data on MSW generation, composition and management strategies were obtained from the Abuja Environmental Protection Board (AEPB, 2020). Data on the 2020 population of Abuja districts, with corresponding income levels were obtained from Nigerian National Bureau of Statistics by direct request (NBS, 2020). Photographic evidence was collected from designated solid waste collection centers within the city and the major dumpsite in the Idu Industrial Districts of Abuja with permission from the authorities. Secondary data on the occupations of residents of Abuja and other supporting data required to analyze the effects of economic activities on waste generation were identified from a review of information available in the scientific literature and during our visit to the disposal site. This involved a critical review of more than 20 peer reviewed papers on MSW production and disposal in Abuja and other developing cities around the world.
The data gathered were used to determine factors influencing the rate of waste generation and composition in different districts of Abuja. A rank correlation was calculated to establish the relationship between income level and waste generation. The null hypothesis used states that there is no significant relationship between income level and waste generation. The alternative hypothesis states that the relationship is significant.
2.3. Energy output potential from thermochemical conversion
In the below text, all symbols used are summarized in Table A1. The higher heating value of the energy provided by complete degradation of the MSW, EHHV (MJ kg−1), was calculated from the elemental content of the waste in Abuja using the below formula (Shi et al., 2016);
where PC is the carbon (C) content (%), PO is the oxygen (O) content (%), PH is the hydrogen (H) content (%), all expressed as a weight percentage of dry matter.
The percentage content of the different elements in the MSW, Pe (%) (where Pe is PC, PO and PH), was obtained from the percentage dry matter content of each component of the waste, Ptype (g per 100 g fresh waste), and the standard elemental compositions of each waste type, Pe,type (%) using Equation (2).
The standard elemental compositions of combustible waste types, Pe,type, are given in Table 2 (Shi et al., 2016). The dry matter content of each waste component type in Abuja, Ptype, was obtained from the AEPB (2020) data (Table 3) combined with typical bulk densities of the waste types from literature (Foday et al., 2017).
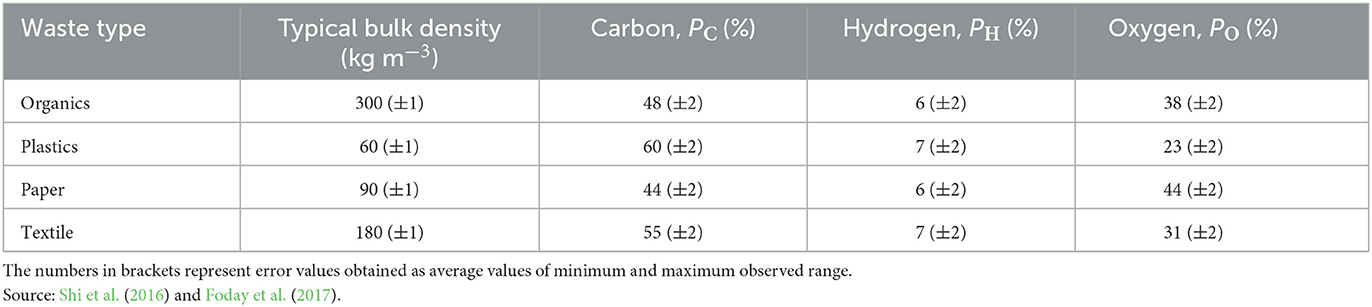
Table 2. Typical bulk density and elemental composition of municipal solid waste as a percentage of the dry matter content.
The maximum potential gross energy production from the MSW (EHHV) is never achieved due to energy losses from the system. The energy that is actually available for energy generation (the lower heating value, ELHV, MJ kg−1) was estimated by accounting for the amount of fuel product used to evaporate the moisture in the fuel during the different conversion methods (Shi et al., 2016) as,
where PH is the percentage hydrogen in MSW. The value of PH was obtained using Equation (2) for each component type (Shi et al., 2016; Aderoju et al., 2019).
The energy recovery potential of MSW, Erp (kWh), was calculated using the standard energy recovery formula (Equation 4) for the thermochemical conversion of biodegradable and non-biodegradable MSW (Tsunatu et al., 2015; Ibikunle et al., 2019),
where Mt is the total mass of MSW produced (t) per day (multiplied by 1,000 to convert to kg and divided by 3.6 × 24 to convert from MJ to kWh) (Ibikunle et al., 2019), while Pconv is the conversion efficiency ranging between 22 and 28% (Aderoju et al., 2019); here set to 22% to provide a conservative estimate of the energy recovery potential. The mass of MSW produced in Abuja (Mt) was assumed to be 1.95 × 102 t per day (Vogeli et al., 2014; AEPB, 2020).
The potential power generation each day, EGP (kW d−1), was then obtained by multiplying by 24,
2.4. Energy output potential from anaerobic digestion
In the below text, all symbols used are summarized in Table A1. The AD plant will be required to process and treat all of the organic fraction of MSW produced by Abuja city, including residential, industrial and commercial solid wastes. The digester should operate through a continuous feed process because this achieves a 40% higher biogas generation potential than a batch system (Monson et al., 2007).
The size of the AD plant was calculated using equation 6 assuming a 5% safety factor (Sf) (Monson et al., 2007).
where VD is the digester volume in m3, Q is the ultimate feedstock influent volume (m3 d−1) and θc is the mean cell residence time or hydraulic retention time (θc = 30 days). The feedstock volume was obtained from the organic fraction of MSW assuming 1-part biowaste to 2 parts water (so the volume of the organic fraction of MSW is multiplied by 3),
where MMSW is the mass of MSW produced each day (1.95 × 105 kg per day; AEPB, 2020), POFMSW is the dry mass of organic matter in the MSW (47.2 g per 100 g; AEPB, 2020), and BDOFMSW is the bulk density of the organic fraction of the MSW (170 kg m−3; Ogwueleka, 2013). The choice of 170 kg m−3 from the given range as BDOFMSW in Abuja is associated to 47% organic fraction by volume of MSW generated.
The biogas production rate per day, Rb (m3 d−1), was then calculated using Equation (8), assuming a typical biogas yield, Y, for organic waste of 0.67 m3 kg−1 VS (Vogeli et al., 2014),
where Qr is the organic loading rate (kg d−1 m−3) and VD is the digester volume in m3.
The organic loading rate (Qr) was calculated from the feedstock influent volume (Q) and digester volume (VD) as shown in Equation (9):
where Cf is the concentration of volatile solids (VS) in the feedstock (kg m−3). The organic matter is assumed to constitute 20% total solids (TS) of which 80% is VS (Vogeli et al., 2014), so Cf can be calculated as
where PTS is the percentage TS in the organic fraction of the MSW (PTS = 20%), and PVS is the percentage VS in the TS (PVS = 80%).
Electric and heat energy generation are dependent on the capacity of the thermal energy produced by biogas combustion. The thermal energy output potential of biogas powered CHP, ETh (MJ d−1), was estimated by assuming 1 m3 of biogas generates 22.3 MJ of ETh (Olugasa et al., 2014).
The potential electrical energy, Ee (MWh d−1), and heat energy, Eh (MJ d−1), were estimated by assuming 35% of ETh is Ee equivalent generation from the biogas powered CHP, 50% of ETh is Eh generation while 15% are energy losses (Banks, 2011).
2.5. Nitrogen fertilizer produced by anaerobic digestion
In the below text, all symbols used are summarized in Table A1. The digestate output, Md (kg d−1) was estimated as a fixed percentage, Pd (%), of the feedstock,
where the values of the percentage of the MSW that is organic (POFMSW) and the mass of MSW (MMSW) are as specified in Equation (7), and Pd is assumed to be 90% of the organic feedstock produced in Abuja (Chowdhury, 2020).
The available nitrogen content in the MSW digestate, Nd (kg d−1) was estimated assuming the conversion of 10% of the feedstock into methane (100 – Pd) releases ammonium which is available to the crop when the digestate is applied (Smith et al., 2014),
where PN (%) is the percentage nitrogen content in the OFMSW. The value of PN was assumed to be 10% (Boyd, 2009).
3. Results and discussion
3.1. Factors affecting the amount of municipal solid waste generation
Data obtained from Abuja Environmental Protection Board for the average annual quantity of MSW collected in Abuja is shown in Figure 1A. Excluding the outlier years of 2000, 2011, and 2014, the data shows a consistent increasing trend in solid waste generation from 2000 to 2020, with an annual increase of ~1.5 × 104 t y−1 (R2 = 0.9, p ≤ 0.05). This increase in MSW generation is reflected in the overstretched treatment facilities and designated disposal sites. This was observed during our visit to the disposal sites, but the lack of data limits further quantitative analysis of the shortfall in facilities. Aderoju et al. (2019) reported an increase in persistent complaints about odor and air pollution by the inhabitants of surrounding areas due to continuous combustion of waste at the dumpsites, which seems to be the only method used for waste volume reduction.
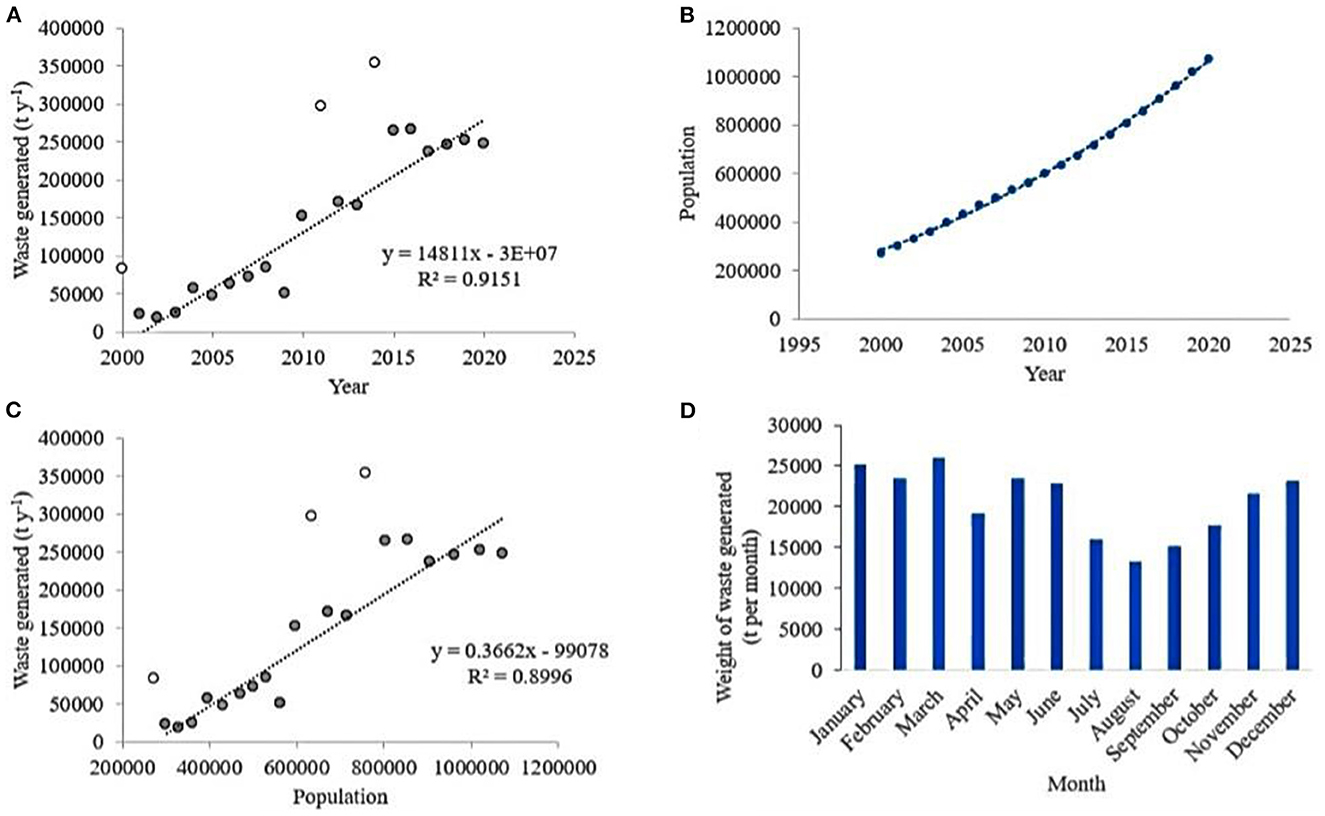
Figure 1. Quantified relationship of factors affecting municipal solid waste in Abuja: (A) average annual generation of municipal solid waste (2000–2020) (data from AEPB, 2020); (B) city population against year 2000–2020 [data from Nigerian National Bureau of Statistics (NBS, 2021)]; (C) waste generated and population (data for population 2000–2020 obtained from United Nations Population Projection, 2021; data for waste generation 2000–2020 obtained from AEPB, 2020); (D) monthly generation for year 2020 (data obtained from AEPB, 2020).
From the annual waste generation data for Abuja (2000–2020) presented in Figure 1A, it was estimated that the average volume of solid wastes generated in the Abuja districts between 2000 and 2020 was 0.658 kg per capita per day and the waste generation recorded for 2020 was 0.630 kg per capita per day (0.229 t y−1 per capita). Per capita waste generation was highest in 2014, at 0.466 t y−1, equivalent to 1.276 kg d−1. This is above the national average of 1.02 kg per capita per day (Bogoro et al., 2014). This may be due to higher average income of the population of Abuja compared to most Nigerian cities.
3.1.1. Population
The population increase in Abuja since 2000 is shown in Figure 1B, while the relationship between population and waste generation in Abuja for 2000–2020 is plotted in Figure 1C. Excluding the outlier years, per capita waste production increased by 0.36 t y−1 between 2000 and 2020 (R2 = 0.9, p < 0.05).
3.1.2. Time of year
Figure 1D shows the MSW generated in Abuja on monthly basis for the year 2020. The lowest rate of waste generation was in August; this is a month of intense rainfall, so the lower rate may reflect failures in collection due to the weather conditions.
3.1.3. Income level
Adeleke et al. (2020) identified income level as the main factor controlling per capita MSW generation. This study used rank correlation for data obtained from National Bureau of Statistics, Nigeria (2019) on income level of Abuja residents based on the percentage of districts survey respondents. The data on income level and the organic content of waste produced in Abuja is found in Table 4.
The rank correlation between income level and the quantity of MSW generated in Abuja districts was 0.82, indicating a positive correlation between the two variables and supporting the alternative hypothesis that there was a significant relationship between income level and quantities of waste generated in Abuja.
3.2. Factors affecting the composition of municipal solid waste
3.2.1. Income
The data in Table 3 indicates that 47% by volume of the MSW in Abuja in 2020 was organic. The increased consumption of packaged goods was responsible for paper and plastic film becoming the second and third largest components of the waste from all districts. Jabi/Utako/Mabushi with lowest percentage of low-income earners at 18.2% also generated the lowest proportion of organic waste of 40% (Table 3). Garki I & II generated the highest proportion of organic waste at 57% and was composed of the second highest proportion of low-income earners; 37.4% with 24.3% with no income.
The composition of MSW from different waste producing group predicts the amount of energy that is recoverable. The composition of Abuja MSW given in Table 3 shows the viable economic potential of waste for recycling and reuse. The rank correlation coefficient of 0.90 indicates that there is a significant increase in the proportion of non-organic wastes in the MSW with increased income level.
3.2.2. Location of waste composition
Table 3 provides data on the composition of waste generated by different districts of Abuja. This indicates that solid waste composition differs with location with highest organic matter content (57%) generated by Garki I & II districts, while the highest paper content (22%) was generated by Gudu/Durumi/Wuye districts. A higher proportion of plastic film waste was generated in Kado/Lifecamp (15%) and Gwarinpa (14%). This may be determined by the type of activities occurring in the location of waste generation.
3.3. Power generation potential of municipal solid waste
3.3.1. Combustion
The waste types provided by the AEPB (2020) (Table 3) were collated into the four waste categories by combining plastic film (nylon) and dense plastic into the category “Plastics”, and paper, cardboard and miscellaneous combustibles into the category “Paper” as shown in Table 5. Combustible material accounted for 91% of the MSW, with the remainder being non-combustible; glass, ferrous metals, non-ferrous metals, waste electrical equipment and miscellaneous non-combustibles.
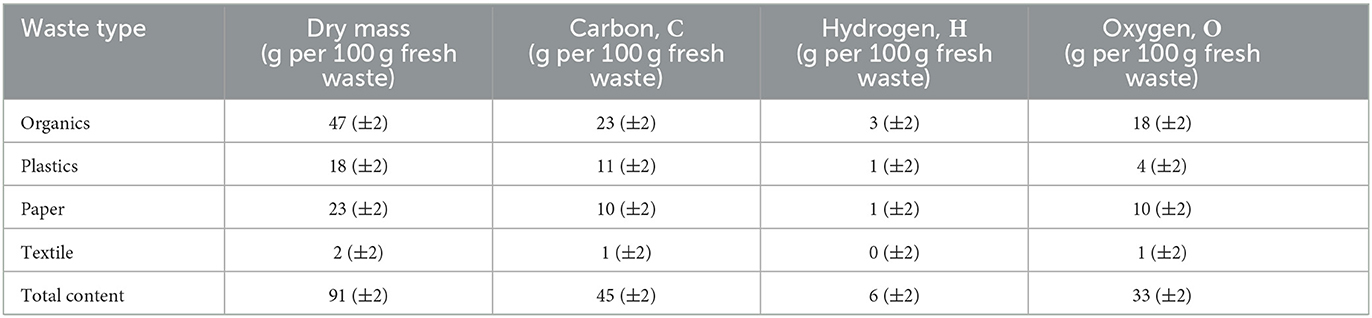
Table 5. Estimated fresh waste elemental content of Abuja municipal solid waste type as mass fraction including moisture.
The higher heating value of the MSW (EHHV) was 19 (±2) MJ kg−1 and the amount of energy available for energy generation on thermochemical conversion (ELHV) was 18 (±2) MJ kg−1. The power generation potential (EGP) was 2.1 × 105 (±1 × 104) kWh d−1, equivalent to 7.7 × 104 (±4 × 103) MWh y−1. The total power requirement of Abuja city in 2020 was 9.3 × 104 MWh y−1 (combined heat and electricity) (AEDC, 2020), so this was 83% of the power requirement. The total electricity generation Ee from thermochemical conversion in CHP was 2.7 × 104 (±1 × 103) MWh y−1 which is 29% of the electricity requirement of Abuja city in 2020, while the total heat generation Eh, 1.39 × 108 (±7 × 106) MJ y−1, greatly exceeded the Abuja heat requirements (2.1 × 105 MJ y−1; AEDC, 2020). Output potentials of thermochemical conversion of waste in Abuja is shown in Table 6.
3.3.2. Anaerobic digestion
The output potentials of the proposed AD plant in Abuja are shown in Table 7. From the organic component of the MSW which is the average volatile solids (VS) amount at 7.1 × 107 (±4 × 106) kg y−1 (AEPB, 2020), biogas production (Rb) was estimated to be 3.06 × 106 (±2 × 105) m3 y−1. This could be converted into electrical energy, Ee = 7.8 × 103 (±4 × 102) MWh y−1 and heat energy, Eh = 4.0 × 107 (±2 × 106) MJ y−1. This is equivalent to 8% of the annual electricity requirement of Abuja city in 2020. The heat generated again greatly exceeded the annual heat requirement. System energy losses accounted for 3.62% of the biogas produced.
The electricity output potential from the combustion of biogas produced from MSW, Ee, was 28% of that available by thermochemical conversion, while heat output Eh from biogas CHP was 29% of that produced thermochemically. However, if the remaining plastics, paper and textiles were combusted, they could produce an additional 1.35 × 104 (±7 × 102) MWh y−1 of electricity; 15% of the electricity requirement (Figure 2). Therefore, the total electricity produced by AD of the organic wastes with burning of the remaining combustibles would be 23% of the electricity requirement of Abuja city compared to 29% by burning alone. The heat produced by burning only plastics, paper and textiles would be 6.95 × 107 (±4 × 106) MJ y−1, again greatly exceeding the heat requirement of Abuja.
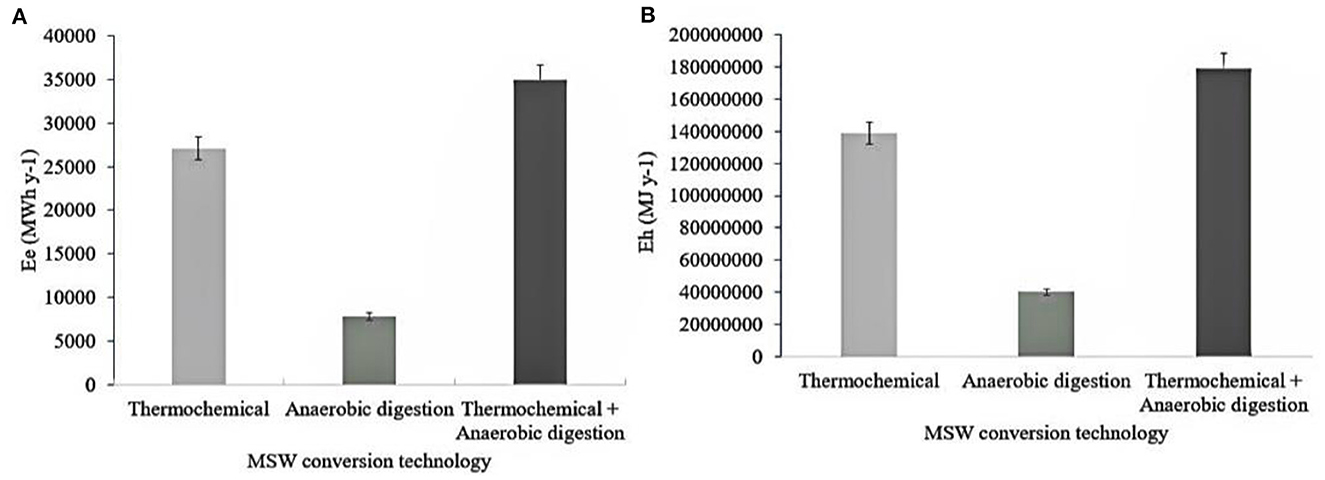
Figure 2. Potential energy provision from municipal solid waste in Abuja (A) electrical energy, Ee and (B) heat energy, Eh, for thermochemical, AD and thermochemical + AD combined.
The use of the organic fraction of MSW for biogas production has the added benefit of digestate production, estimated at 5.34 × 105 (±3 × 104) kg y−1. This digestate is important for agricultural purposes, which could be used as biofertilizer and soil amendment. The plant available nitrogen in the digestate (Nd) was estimated to be 1.28 × 105 (±6 × 103) kg y−1 which is 1.22% of the current conventional fertilizer demand in Abuja at 1.05 × 107 kg y−1 (Balana et al., 2022). This could increase agricultural yields in the city and encourage environmental sustainability.
The power generation potentials from the organic fraction for AD and the remaining waste for thermochemical conversion could provide combined heat and electricity that is ~92% of Abuja power requirements in addition to providing the benefits of the digestate that can be used as a fertilizer.
3.4. Implications for municipal solid waste management and society
3.4.1. How should municipal solid waste be managed for energy provision in Abuja?
The results from Section 3.3 suggest that municipal solid waste should be managed by combined AD of the organic fraction, and thermochemical conversion of the remaining combustible materials. This will provide the best energy returns of the options considered, as well as producing an organic fertilizer that is of value to farmers. Implementation of this combined management process would require the development of a recycling culture, correct wastes handling procedures and thorough sorting and separation of waste types. It would be facilitated by a change in the attitudes of waste producers, increased government funding for equipment, banning of waste landfilling, economic support, and social and private partnerships. Consistent legislation review and policy implementation is also needed to achieve change in the behavior of policy makers, government regulatory authorities and all stakeholders for more effective MSW management.
3.4.2. What are the potential economic and commercial impacts?
Power generation from the combined use of AD and thermochemical conversion could provide 92% of the 2020 combined heat and electricity requirement of Abuja (Tables 6, 7). In 2020, the cost of combined heat and power used by Abuja was 106,288$. Therefore, the economic value of the energy produced is equivalent to 97,785$. In addition, assuming the 2020 price for nitrogen fertilizer in Nigeria of 15.3$ kg−1 N (IFDC, 2020), the estimated plant available nitrogen in the digestate [1.28 × 105 (±6 × 103) kg y−1] has a value of 39,168$ y−1. The use of MSW for energy generation in Abuja would complement the current electricity supply, which is inconsistent due to unstable fossil fuel gas production and an unreliable distribution network, causing persistent power outages that impact both domestic and commercial activities (NBS, 2021).
3.4.3. How should public policy be changed to facilitate use of municipal solid waste for energy provision?
Given the economic value of the energy and fertilizers provided by the OFMSW, use for energy provision could be incentivized by payment for delivered waste. This would change attitudes of waste handlers to the organic wastes and reduce dumping after collection. Periodic review of waste management policies in Abuja, such as establishment of economic and environmental public awareness and strengthening of the enforcement power of existing agencies, would further facilitate the use of waste-to-energy technologies.
3.4.4. What is the potential impact on society?
Energy generation from MSW has many benefits to society in promoting healthy living conditions and environmental sustainability, in addition to the provision of energy and organic fertilizer. Potential negative impacts include land acquisition for siting of the treatment plant, and the impacts of operating the plant, such as the potential discharge of hazardous materials remaining after sorting of the wastes (Afon and Okewole, 2007; Arukwe et al., 2012; Paolini et al., 2018; Biodun et al., 2021). These disbenefits are likely to be reduced compared to the negative impacts of landfill.
4. Conclusions
Adoption of AD and CHP into the Abuja system of MSW management could be a viable and promising strategic innovation for all the districts of the city. Combining AD of the organic fraction with combustion could produce 23% of the city's electricity requirements. This is less than could be produced by combustion alone (29%) but has the advantage of producing a biofertilizer and reducing air pollution from burning. Anaerobic digestion of waste was estimated to provide 7.81 × 103 (±4 × 102) MWh y−1 electricity and 4.02 × 107 (±2 × 106) MJ y−1 heat in Abuja in addition to production of 5.34 × 105 (±3 × 104) kg y−1 digestate that could be used as bio-fertilizer in agriculture. Thermochemical conversion of the remaining non-digestible waste would provide 7.73 × 104 (±4 × 103) MWh y−1. This is 83% of Abuja heat and electricity requirement.
The energy production strategy of AD of MSW could play an important role in improving the city's economic stability and reducing social discrimination faced by informal MSW operators. While poor management of MSW, particularly by informal operators, can constitute environmental risks such as health hazards and soil contamination, energy generation from MSW has many potential benefits, including reduction of GHG emissions, provision of employment opportunities and reduction of the level of harassment and discrimination faced by informal waste collectors. This could turn waste disposal into a valued public service.
Enhancement of MSW energy production in Abuja should be encouraged by both government authorities and private sectors through suitable policy initiatives and enforcement. Financial support for industry operators may not be needed because of the financial benefits of producing energy from MSW. Public awareness on the negative environmental impact of poor MSW management and the potential of improved recycling should be increased. This could provide the citizens of Abuja with modern waste disposal techniques with at-source sorting facilitating collection and enhancing energy generation processing.
Data availability statement
The original contributions presented in the study are included in the article/supplementary material, further inquiries can be directed to the corresponding author.
Author contributions
ED, JS, and AH conceived and design the paper structure and methodology. The research grants were awarded to ED and AH. ED did the investigation, data collection, and wrote the first draft. JS and AH supervised the research, contributed to formal analysis, reviewed, and edited the writing. JS did the final review and improved the manuscript standard. The final manuscript version was reviewed and approved by all authors.
Funding
The research was funded by the Petroleum Technology Development Fund (PTDF), Nigeria as oversea Ph.D. fully funded program with award number (PTDF/ED/OSS/PHD/EMD/1656/19), and by the ADVENT project funded by the UK Natural Environment Research Council (NE/M019691/1) and ADVANCES project funded by the UK Natural Environment Research Council (NE/M019691/1), EPSRC funded by UKERC-4 and AH was funded by UK Research and Innovation Energy Programme grant number EP/S029575/1.
Acknowledgments
Sincere appreciation to PTDF Nigeria for granting the research award and to the management of Abuja Environmental Protection Board (AEPB), Nigeria, for its support in providing data on Abuja municipal solid waste and for granting us access to the major disposal site. The UK Natural Environment Research Council (UKERC) and UK Research and Innovation Energy Programme are also much appreciated for funding support.
Conflict of interest
The authors declare that the research was conducted in the absence of any commercial or financial relationships that could be construed as a potential conflict of interest.
Publisher's note
All claims expressed in this article are solely those of the authors and do not necessarily represent those of their affiliated organizations, or those of the publisher, the editors and the reviewers. Any product that may be evaluated in this article, or claim that may be made by its manufacturer, is not guaranteed or endorsed by the publisher.
References
Adeleke, O. A., Akinlabi, S. A., Jen, T. C., and Dunmade, I. (2020). An overview of factors affecting the rate of generation and physical composition of municipal solid waste. IOP Conf. Series: Mater. Sci. Engg. 1107, 3–9. doi: 10.1088/1757-899X/1107/1/012096
Aderoju, O. M., Gemusse, O. U., and Guerner, A. D. (2019). An optimization of the municipal solid waste in Abuja, Nigeria for electrical power generation. WIT Press 11, 63–74. doi: 10.2495/EQ-V4-N1-63-74
Aderoju, O. M., and Guerner, A. D. (2020). Municipal solid waste characterization as a measure towards sustainable waste management in Abuja, Nigeria. J. Environ. Sci. Publ. Health 4, 43–60. doi: 10.26502/jesph.96120084
Aderoju, O. M., Guerner, A. D., and Alberto, J. G. (2018). A GIS-based analysis for sanitary landfill sites in Abuja, Nigeria. J. Environ. Dev. Sustain. 22, 551–574. doi: 10.1007/s10668-018-0206-z
Afon, A. O., and Okewole, A. (2007). Estimating the quantity of solid waste generation in Oyo, Nigeria. Waste Manag. Res. 25, 371–379. doi: 10.1177/0734242X07078286
Aghbashlo, M., Tabatabaei, M., Soltanian, S., Ghanavati, H., and Dadak, A. (2019). Comprehensive exergoeconomic analysis of a municipal solid waste digestion plant equipped with a biogas genset. Waste Manag. 87, 485–498. doi: 10.1016/j.wasman.2019.02.029
Aguilar-Virgen, Q., Taboada-Gonzalez, P., Ojeda-Benitez, S., and Cruz-Sotelo, S. (2014). Power generation with biogas from municipal solid waste: Prediction of gas generation with in-situ Parameters. Renew. Sustain. Energy Rev. 30, 412–419. doi: 10.1016/j.rser.2013.10.014
Ajani, O. I. Y. (2008). Determinants of an effective solid waste management in Ibadan Metropolis, Oyo State, Nigeria. J. FoodAgric. Environ. 6, 152–157. Available online at: https://www.semanticscholar.org/paper/Determinants-of-an-effective-solid-waste-management-Ajani/ba84f3da919e55af313de291c3138de296b4c4c8#citing-papers (accessed June 30, 2021).
Aldrich, R., Llauro, F. X., Puig, J., Pere, M., and Pelach, M. A. (2011). Allocation of GHG emissions in combined heat and power systems: a new proposal for considering inefficiencies of the system. J. Clean. Prod. 19, 1072–1079. doi: 10.1016/j.jclepro.2011.02.014
Amasuomo, E., and Baird, J. (2016). Solid waste management trends in Nigeria. J. Manag. Sustain. 6, 35. doi: 10.5539/jms.v6n4p35
Amo-Asamoah, E., Owusu-Manu, D. G., Asumadu, G., Ghansah, F. A., and Edwards, D. J. (2020). Potential for waste to energy generation of municipal solid waste (MSW) in the Kumasi metropolis of Ghana. Int. J. Energy Sector Manag. 14, 1315–1331. doi: 10.1108/IJESM-12-2019-0005
Arukwe, A., Eggen, T., and Moder, M. (2012). Solid waste deposits as a significant source of contaminants of emerging concern to the aquatic and terrestrial environments — A developing country case study from Owerri, Nigeria. Sci. Total Environ. 438, 94–102. doi: 10.1016/j.scitotenv.2012.08.039
Balana, B., Andam, K., Amare, M., Adeyanju, D., and Laborde, D. (2022). The Russia-Ukraine Crisis Presents Threats to Nigeria's Food Security, But Potential Opportunities for the Fertilizer, Energy Sectors. IFPRI Blog. Available online at: https://www.ifpri.org/blog/russia-ukraine-crisis-presents-threats-nigerias-food-security-potential-opportunities (accessed August 1, 2022).
Banks, C. (2011). Anaerobic Digestion and Energy. VALORGAS Sevent Framework Progr. Available online at: https://www.valorgas.soton.ac.uk/Pub_docs/JyU%20SS%202011/CB%204.pdf (accessed July 26, 2022).
Barati, M. R., Aghbashlo, M., Ghanavati, H., Tabatabaei, M., Sharifi, M., Javadirad, G., et al. (2017). Comprehensive exergy analysis of a gas engine-equipped anaerobic digestion plant producing electricity and bio-fertilizer from organic fraction of municipal solid waste. Energy Convers. Manag. 151, 753–763. doi: 10.1016/j.enconman.2017.09.017
Biodun, M. B., Fayomi, O. S. I., and Okeniyi, J. O. (2021). The possibility of biogas production in nigeria from organic waste material: a review. IOP Conf. Ser. Mat. Sci. Engg. 1107, 012166. doi: 10.1088/1757-899X/1107/1/012166
Bogoro, A. G., Bkar, A., Samson, M. N., and Rasheed, O. (2014). Economic factors that determine the quantity and Characteristics of Solid Waste in Bauchi Metropolis, Nigeria. IOSR J. Envir. Sci. Toxicol. Food Technol. 8, 1–7. doi: 10.9790/2402-08620107
Bongaarts, J. (2009). Human population growth and the demographic transition. Philos. Transact. R. Soc. London Blog Biol. Sci. 364, 2985–2990. doi: 10.1098/rstb.2009.0137
Boyd, C. E. (2009). Carbon-Nitrogen Ratio Management. Global Seafood Alliance. Available online at: https://www.google.com/search?rlz=1C1GCEU_enGB993GB993&q=What+is+the+nitrogen+percentage+in+organic+matter%3F&sa=X&ved=2ahUKEwjnh6Lqu_n8AhWwSUEAHalvAlwQzmd6BAgaEAU (accessed January 24, 2023).
Chaianong, A., and Pharino, C. (2022). How to design an area-based prioritization of biogas production from organic municipal solid waste? Evidence from Thailand. Waste Manag. 138, 243–252. doi: 10.1016/j.wasman.2021.11.042
Chowdhury, T. H. (2020). Technical-economical analysis of anaerobic digestion process to produce clean energy. Energy Rep. 7, 247–253. doi: 10.1016/j.egyr.2020.12.024
Dehkordi, S. M. M. N., Jahromi, A. R. T., Ferdowsi, A., Shumal, M., and Dehnavi, A. (2020). Investigation of biogas production potential from mechanical separated municipal solid waste as an approach for developing countries (case study: Isfahan-Iran). Renew. Sustain. Energy Rev. 119, 109586. doi: 10.1016/j.rser.2019.109586
Deressa, L., Libsu, S., Chavan, R. B., Manaye, D., and Dabassa, A. (2015). Production of biogas from fruit and vegetable wastes mixed with different wastes. Envir. Ecolog. Res. 3, 65–71. doi: 10.13189/eer.2015.030303
Eddine, B. T., and Salah, M. M. (2012). Solid waste as renewable source of energy: current and future possibility in Algeria. Int. J. Energy Environ. Engg. 3, 17. doi: 10.1186/2251-6832-3-17
EDGAR (2022). Fossil CO2 and GHG Emissions of all World Countries. Emissions Database for Global Atmospheric Research. Available online at: https://publications.jrc.ec.europa.eu/repository/bitstream/JRC117610/fossil_co2_emissions_of_all_world_countries_booklet_online_final.pdf (accessed April 11, 2023).
Ezeah, C., and Roberts, C. L. (2010). Analysis of Barriers and Success Factors Affecting the Adoption of Sustainable Management of Municipal Solid Waste in Nigeria (PhD thesis). University of Wolverhampton. Available online at: https://wlv.openrepository.com/handle/2436/110155 (accessed June 28, 2021).
Ezeah, C., and Roberts, C. L. (2012). Analysis of barriers and success factors affecting the adoption of sustainable management of municipal solid waste in Nigeria. J. Environ. Manag. 103, 9–14. doi: 10.1016/j.jenvman.2012.02.027
Foday, E. H., Ramli, N. A., Ismail, H. N., Malik, N. A., Basri, H. F., Aziz, F. S. A., et al. (2017). Municipal solid waste characteristics in Taman Universiti, Skudai, Johore, Malaysia. J. Adv. Res. Des. 38, 13–20. Available online at: https://www.akademiabaru.com/doc/ARDV38_N1_P13_20.pdf (accessed January 25, 2022).
Gebreegziabher, Z., Naik, L., Melamu, R., and Balana, B. B. (2014). Prospects and challenges for urban application of biogas installations in Sub-Saharan Africa. Biomass Bioenergy 70, 130–140. doi: 10.1016/j.biombioe.2014.02.036
Hettiarachchi, H., Meegoda, J. N., and Ryu, S. (2018). Organic waste buyback as a viable method to enhance sustainable municipal solid waste management in developing countries. Int. J. Environ. Res. Public Health 15, 2483. doi: 10.3390/ijerph15112483
Ibarra-Esparza, F. E., Garibay, M. V., Lara-Topete, G. O., Gonzalez-Lopez, M. E., Orozco-Nunnelly, D. A., Aguilar-Juarez, O., et al. (2022). A micro- and macro-scale look at the biochemical methanogenic potential of the organic fraction of municipal solid waste generated in a large city of a developing country. Front. Environ. Sci. 10, 1020208. doi: 10.3389/fenvs.2022.1020208
Ibikunle, R. A., Titiladunayo, I. F., Akinnuli, B. O., Dahunsi, S. O., and Olayanju, T. M. A. (2019). Estimation of power generation from municipal solid wastes: a case study of Ilorin metropolis, Nigeria. Energy Rep. 5, 126–135. doi: 10.1016/j.egyr.2019.01.005
IFDC (2020). Assessment of Fertilizer Distribution Systems and Opportunities for Developing Fertilizer Blends in Nigeria. Available online at: https://agra.org/wp-content/uploads/2020/08/Nigeria-report_Assessment-of-Fertilizer-Distribution-Systems-and-Opportunities-for-Developing-Fertilizer-Blends.pdf (accessed April 22, 2022).
Kazemi, S. P. H., Tabatabaci, M., Aghbashlo, M., Dehhaghi, M., Rehan, M., and Nizami, A. S. (2019). Recent updates on the production and upgrading of bio-crude oil from micro-algae. Biores. Technol. Rep. 7, 100216. doi: 10.1016/j.biteb.2019.100216
Khairuddin, N., Abd-Manaf, L., Hassan, M. A., Halimoon, N., and Ab-Karim, W. A. W. (2015). Biogas harvesting from organic fraction of municipal solid waste as a renewable energy resource in Malaysia: a review. Polish J. Environ. Stud. 24, 1477–1490. doi: 10.15244/pjoes/34670
Kumar, A., and Samadder, S. R. (2017). A review on technological options of waste to energy for effective management of municipal solid waste. Waste Manag. 69, 407–422. doi: 10.1016/j.wasman.2017.08.046
Monson, K. D., Esteves, S. R., Guwy, A. J., and Dinsdale, R. M. (2007). Anaerobic Digestion of Biodegradable Municipal Wastes. A Review. The Sustainable Environment Research Centre, University of Glamorgan. Available online at: https://www.walesadcentre.org.uk/media/1050/anaerobic-digestion-of-bmw-_compressed_-part1.pdf (accessed October 13, 2021).
Moya, D., Aldas, C., Jaramillo, D., Jativa, E., Kaparaju, P., Edited by: Littlewood, J., et al. (2017). Waste-to-energy technologies: an opportunity of energy recovery from municipal solid waste, using Quito - Ecuador as case study. Sustain. Energy Build. 134, 327–336. doi: 10.1016/j.egypro.2017.09.537
Murray, K. E., Thomas, S. M., and Bodour, A. A. (2010). Prioritizing research for trace pollutants and emerging contaminants in the freshwater environment. Envir. Pollut. 158, 3462–3471. doi: 10.1016/j.envpol.2010.08.009
National Population Commission Nigeria (2014). Demographic and Health Survey 2013. Available online at: dhsprogram.com/pubs/pdf/fr293/fr293.pdf (accessed August 12, 2014).
NBS (2020). National Bureau of Statistics. Available online at: https://www.nigerianstat.gov.ng/ (accessed March 30, 2023).
NBS (2021). National Bureau of Statistics. Available online at: punchng.com/generators-provide-48-6-of-electricity-in-nigeria-nbs/ (accessed March 30, 2023).
Obidike, E. E., Jatau, S., and Ayu, M. (2020). A critical analysis of the role of energy generation from municipal solid waste (MSW). AIMS Environ. Sci. 7, 387–405. doi: 10.3934/environsci.2020026
Ogwueleka, T. (2009). Municipal solid waste characteristics and management in Nigeria. Iran J. Environ. Health Sci. Engg. 6, 173–180.
Ogwueleka, T. C. (2013). Survey of household waste composition and quantities in Abuja, Nigeria. Res. Conserv. Recycl. 77, 52–60. doi: 10.1016/j.resconrec.2013.05.011
Olugasa, T. T., Odesola, I. F., and Oyewola, M. O. (2014). Energy production from biogas: a conceptual review for use in Nigeria. Renew. Sustain. Energy Rev. 32, 770–776. doi: 10.1016/j.rser.2013.12.013
Pandyaswargo, A. H., Gamaralalage, P. J. D., Liu, C., Knaus, M., Onoda, H., Mahichi, F., et al. (2019). Challenges and an implementation framework for sustainable municipal organic waste management using biogas technology in emerging Asian Countries. Sustainability 11, 6331. doi: 10.3390/su11226331
Paolini, V., Petracchini, F., Segreto, M., Tomassetti, L., Naja, N., and Cecinato, A. (2018). Environmental impact of biogas: a short review of current knowledge. J. Environ. Sci. Health Part A 53, 899–906. doi: 10.1080/10934529.2018.1459076
Paul, T., and Soren, N. (2020). An overview of municipal solid waste-to-energy application in Indian scenario. Environ. Dev. Sustain. 22, 575–592. doi: 10.1007/s10668-018-0235-7
Portugal-Pereira, J., and Lee, L. (2015). Economic and environmental benefits of waste-to-energy technologies for debris recovery in disaster-hit Northeast Japan. J. Clean Produc. 112, 4419–4429. doi: 10.1016/j.jclepro.2015.05.083
Rajaeifar, M. A., Ghanavati, H., Dashti, B. B., Heijungs, R., Aghbashlo, M., and Tabatabaei, M. (2017). Electricity generation and GHG emission reduction potentials through different municipal solid waste management technologies: a comparative review. Renew. Sustain. Energy Revol. 79, 414–439. doi: 10.1016/j.rser.2017.04.109
Ribeiro, N. D., Barros, R. M., Dos-Santos, I. F. S., Cio, G. L., and Da-Silva, S. P. G. (2021). Electric energy generation from biogas derived from municipal solid waste using two systems: landfills and anaerobic digesters in the states of Sa tilde o Paulo and Minas Gerais, Brazil. Sustain. Energy Technol. Assess. 48, 101552; doi: 10.1016/j.seta.2021.101552
Rolewicz-Kalinska, A., Lelicinska-Serafin, K., and Manczarski, P. (2020). The circular economy and organic fraction of municipal solid waste recycling strategies. Energies 13, 4366. doi: 10.3390/en13174366
Scarlat, N., Motola, V., Dallemand, J. F., Monforti-Ferrario, F., and Linus, M. (2015). Evaluation of energy potential of municipal solid waste from African urban areas. Renew. Sustain. Energy Revol. 50, 1269–1286. doi: 10.1016/j.rser.2015.05.067
Shi, H., Mahinpey, N., Aqsha, A., and Silbermann, R. (2016). Characterization, thermochemical conversion studies, and heating value modeling of municipal solid waste. Waste Manag. 48, 34–47. doi: 10.1016/j.wasman.2015.09.036
Smith, J., Abegaz, A., Matthews, R. B., Subedi, M., Orskov, E. R., Tumwesige, V., et al. (2014). What is the potential for biogas digesters to improve soil fertility and crop production in Sub-Saharan Africa? Biomass Bioenergy 70, 58–72. doi: 10.1016/j.biombioe.2014.02.030
Song, Q., and Li, J. (2014). Environmental effects of heavy metals derived from the e-waste recycling activities in China: a systematic review. Waste Manag. 34, 2587–2594. doi: 10.1016/j.wasman.2014.08.012
Tsunatu, D. Y., Tickson, T. S., San, K. D., and Namo, J. M. (2015). Municipal solid waste as alternative source of energy generation: A case study of Jalingo metropolis, Taraba State, Nigeria. Int. J. Engg. Technol. 5, 185–193. Available online at: https://www.researchgate.net/publication/284133769 (accessed February 3, 2023).
United Nations Fund for Population Activities-UNFPA (2015). UNFPA in FCT, Abuja. Available online at: https://www.unfpa.org/sites/default/files/pub-pdf/UNFPA_2015_Annual_Report.pdf (accessed March 12, 2021).
United Nations Population Projection (2021). Nigeria Metro Macrotrends Publication. Available online at: https://www.macrotrends.net/cities/21976/abuja/population (accessed August 25, 2021).
Vogeli, Y., Lohri, C. R., Gallardo, A., Diener, S., and Zurbrugg, C. (2014). Anaerobic Digestion of Biowaste in Developing Countries: Practical Information and Case Studies. Dubendorf: Swiss Federal Institute of Aquatic Science and Technology, 43.
Yadav, H., Kumar, P., and Singh, V. P. (2018). Hazards from the municipal solid waste dumpsites: a review. Intern. Conf. Sustain. Waste Manag. Through Design. 2018, 336–342. doi: 10.1007/978-3-030-02707-0_39
Yadav, P., Yadav, S., Singh, D., Giri, B. S., and Mishra, P. K. (2022). Barriers in biogas production from the organic fraction of municipal solid waste: a circular bioeconomy perspective. Bioresour. Technol. 362, 127671. doi: 10.1016/j.biortech.2022.127671
Yeung, L. W. Y., Yamashita, N., Taniyasu, S., Paul, K. S. L., Ravindra, K. S., Dnyandev, V. B., et al. (2009). A survey of perfluorinated compounds in surface water and biota including dolphins from the Ganges River and in other waterbodies in India. Chemosphere 76, 55–62. doi: 10.1016/j.chemosphere.2009.02.055
APPENDIX A
Keywords: organic fraction of municipal solid waste, anaerobic digestion, greenhouse gas emissions, combined heat and power, municipal solid waste management, low-income countries
Citation: Dickson EM, Hastings A and Smith J (2023) Energy production from municipal solid waste in low to middle income countries: a case study of how to build a circular economy in Abuja, Nigeria. Front. Sustain. 4:1173474. doi: 10.3389/frsus.2023.1173474
Received: 24 February 2023; Accepted: 10 May 2023;
Published: 30 May 2023.
Edited by:
Sampriti Kataki, Cactus Communications Pvt. Ltd., IndiaReviewed by:
Georgia Makridou, ESCP Business School, United KingdomDimitris Ipsakis, Technical University of Crete, Greece
Jolita Kruopienė, Kaunas University of Technology, Lithuania
Copyright © 2023 Dickson, Hastings and Smith. This is an open-access article distributed under the terms of the Creative Commons Attribution License (CC BY). The use, distribution or reproduction in other forums is permitted, provided the original author(s) and the copyright owner(s) are credited and that the original publication in this journal is cited, in accordance with accepted academic practice. No use, distribution or reproduction is permitted which does not comply with these terms.
*Correspondence: Emmanuel Mela Dickson, ZS5kaWNrc29uLjIwQGFiZG4uYWMudWs=