- 1Department of Sustainable Design Engineering, Faculty of Industrial Design Engineering, Delft University of Technology, Delft, Netherlands
- 2Department of Biomechanical Engineering, Delft University of Technology, Delft, Netherlands
Healthcare facilities in low-resource settings in Sub-Saharan Africa are plagued with issues of non-functional and obsolete medical devices, which ultimately end up prematurely disposed of as waste. With increasing healthcare demands, stopping medical device disposal is imperative. One way to achieve this is to leverage circular economy principles in designing medical devices. Circular economy principles aim to retain products and their constituent materials to be reused over time in the economic system. However, to what extent this has been applied in designing medical devices specifically for low-resource settings in Sub-Saharan Africa is missing in literature. Based on a systematic review of 29 out of 1,799 screened scientific papers, we identified the use of circular economy principles of durability, maintenance, repair, and upgrade in designing medical devices for this setting. Whether these principles were intentionally applied from a circular economy approach could not be inferred in this study. The motivational basis for using these principles was to ensure medical device longevity to providing healthcare. No attention was given to the circular economy principles of refurbishment, remanufacturing, and recycling, ensuring that device components and constituent materials are recovered. These study findings serve as a launchpad for exploring how circular principles can be used to support the design of medical devices for low-resource settings in Sub-Saharan Africa. Academicians and designers of medical devices can leverage this research to contribute towards developing medical devices that support access to healthcare for people in low-resource settings and preserve earth's finite resources.
1. Introduction
To provide healthcare for all, medical devices are highly needed. This need is significantly felt in low-resource settings (LRS) in Sub-Saharan Africa (SSA), which are areas with limited infrastructure, materials, supplies and human resources (Anyangwe and Mtonga, 2007; Masum et al., 2010; Aranda Jan et al., 2016). According to the World Health Organization (WHO), this region suffers from most of the world's diseases and medical devices are highly needed to overcome this burden (World Health Organization, 2017). So far, the need for medical devices in this region has primarily been met through international donations or by importing medical devices from high-income countries (Piaggio et al., 2019; Ssekitoleko et al., 2021). These devices donated or imported with the best intentions towards providing healthcare are usually not optimised to work in the LRS healthcare system (Howitt et al., 2012). Once installed, they face context-specific challenges in LRS, such as a lack of spare parts, repair or maintenance services, accessories, and consumables, which render them obsolete (Richards-Kortum and Oden, 2013; Di Pietro et al., 2020). Estimates suggest that 30–40% of medical devices in LRS are non-functional due to these context-specific challenges (Perry and Malkin, 2011; Ssekitoleko et al., 2021), resulting in their premature disposal as waste (Miesen, 2013). Apart from the fact that non-functioning devices do not contribute to improving access to healthcare, their disposal contributes to the 282,447 tonnes of waste generated by the healthcare sector in Africa each year (Udofia et al., 2015; Chisholm et al., 2021). These medical devices, which are prematurely disposed of as waste, still contain valuable parts and materials that could be reused or recycled. Given the increasing healthcare demands and population growth in SSA (United Nations Department of Economic and Social Affairs, 2017; Bigna and Noubiap, 2019), it is imperative to slow down or stop the disposal of medical devices.
One of the ways to slow down or stop the disposal of medical devices is to leverage the concept of circular economy (CE). The goal of CE is to preserve the economic and environmental value of products and their constituent materials as long as possible (Bakker and Poppelaars, 2018). CE is vital for moving away from the current global linear economy. In a linear economy, materials are taken from the Earth, made into products, and eventually, at some point, thrown away as waste (Sariatli, 2017). In contrast, CE aims to eliminate “waste”. This means that products and their constituent materials that enter CE must always remain accountable. To achieve this, rethinking the design of products is critical (Hapuwatte and Jawahir, 2021). Rethinking the design of products from a CE perspective will mean first designing products to remain in their original state or as close as possible to their original state by leveraging on CE principles of physical durability and product maintenance, repair and upgrade (den Hollander, 2018). In the event that products and their constituent material reach their lifespan, they can be recovered by leveraging CE principles of recontextualisation, refurbishment, and remanufacturing. When these products cannot be recovered, they can be disintegrated and returned to their material form through the CE principle of recycling. Note that though product design is vital, it is but one factor in the transition to CE. A successful transition will require other factors such as changes in business models, government policies, rules, and regulations. However, this paper focuses only on the aspect of design.
CE in the medical device design domain is not yet a distinct field. Efforts from the scientific community toward integrating CE and medical device design are only starting to emerge (Kane et al., 2018). For example, frameworks advocating for the design of medical devices for LRS to have extended lifespans are now being seen in literature (Piaggio et al., 2021b). Though this framework does not explicitly stem from the CE mindset, it, however, advocates for the extension of product lifespans which is idiosyncratic to CE. Medical devices with extended lifespans can remain functional and available to provide health care for people in need. At the industry level, Koninklijke Philips is demonstrating the use of CE principle in the design of their high-value Diamond Select X-Ray machines. These devices are designed to be reliable, durable, and can be maintained and repaired (Jensen et al., 2019; Oturu et al., 2021). The modular design of these X-ray machines allows for future upgrades, refurbishing, and remanufacturing activities to be performed, so that they can remain in use over time (Jensen et al., 2019; Oturu et al., 2021). Designing the X-ray machines while considering matters of long and extended use, recovery, and recycling, start to drive sustainable practises in the medical device domain. However, it is worth noting that such a device is an exception and is backed by financial support for several research and development iterations, not available to other medical devices.
Even though the integration of CE and medical device design is still in the nascent stage, other domains and industries have gone ahead to make use of CE. For example, in Europe, a mobile phone company is leveraging on CE principles of durability, and reuse and recycling to ensure a mobile phones have multiple life cycles and consequently contribute to a positive social and environmental impact (Mestre and Cooper, 2017). Similarly, China 4.0 plan, also known as “Made-in-China 2025”, emphasises the use of CE to minimise resource input, maximise economic output, lessen the influence on the natural environment and improve resource recycling efficiency. Kenya, Uganda and Rwanda's implementation of a ban on plastic bags has attracted widespread praise from global circular economy and environmental leadership (Behuria, 2021). This ban advocated for a transition from single-use plastic disposable packaging to reusable alternatives (Ncube et al., 2021; Oberoi and Garg, 2021). Single-use plastics negatively impact the environment aesthetically and pose serious health challenges (Andrady, 2011; Stoler et al., 2012; Adam et al., 2020) and transitioning into reusable alternatives as in CE was essential.
Particularly to LRS in SSA, several studies have identified CE practises. For example, the Ellen MacArthur Foundation found that in Nigeria and Ghana, repair and refurbishing of electronics for reuse, the harvesting of components for reuse in the manufacturing of new products are common place (Ellen MacArthur Foundation, 2022a). In another example, Suame Magazine Automotive Centre in Ghana leverages CE principles of repair and refurbishment in the automotive industry to keep vehicles in use over time (Ellen MacArthur Foundation, 2022a). These CE principles are used to restore and retain the value of goods and materials for as long as possible to be reused. In other cases, it is a frugal way of approaching daily life to ensure product longevity due to resource scarcity. We expect to find similar principles when looking at medical device design, such as durable and repairable designs that can ensure products are used over time. This is the starting point of this study and aims to answer the following research question: to what extent have CE principles been applied in the design of medical devices specifically for use in LRS in SSA? The answer to this question will contribute to literature on CE in Africa by showing the extent to which CE principles have been incorporated in the domain of medical device design for LRS in SSA. Similarly, this research contributes to the CE discourse in Africa by showing the gaps that need to be addressed when designing future medical devices for LRS in SSA while considering sustainability.
2. Theoretical background
2.1. Circular economy
Several schools of thought have defined circular economy (CE) since its inception in the 1970s (Ellen MacArthur Foundation, 2013). The definition adopted in this paper stems from the field of industrial ecology and defines CE from a material flow perspective (Ayres, 1994; Stahel, 1994, 2010; Lifset and Graedel, 2002). That is, CE aims to preserve the economic and environmental value of products and their constituent materials as long as possible by lengthening their lifespan or looping them back into the system to be reused (Bakker and Poppelaars, 2018; Kane et al., 2018). Fundamentally, CE seeks to eliminate the notion of “waste” and ensure products and their materials re-enter the economic system at the end of their use (Kane et al., 2018; Sikdar, 2019). Although there will always be a certain amount of unavoidable “waste” (Ciacci et al., 2015), a CE intends to work towards a closed loop (Prendeville et al., 2014; Bourguignon, 2016; Hapuwatte and Jawahir, 2021). In this closed loop, resources that have entered the CE must always remain accounted for (den Hollander et al., 2017; Haupt et al., 2017).
The notion of CE aims to support the shift from the “take-make-waste” linear economy (Webster, 2015; Bocken et al., 2016). In a CE, “waste” should not exist as products and materials are reused as long as possible over time. The mechanism by which products become “waste” in a linear economy is “obsolescence”—defined as a loss of perceived value of the product, which leads to it being discarded as waste (den Hollander, 2018). According to the core principles of a CE, obsolescence should not lead to waste. Instead, CE activities of “recovery”—defined as any operation with the primary aim of reversing obsolescence, should be performed (den Hollander et al., 2017). With CE activities of recovery, products and materials can be removed from their obsolete state and their perceived value restored and returned to the economic system for reuse. Recovery activities in CE can be ranked according to the “inertia” principle proposed by Walter Stahel: “Do not repair what is not broken, do not remanufacture something that can be repaired, do not recycle a product that can be remanufactured. Replace or treat only the smallest possible part in order to maintain the existing economic value of the technical system” (Stahel, 2010, p. 195). As such, keeping a product in its original state or as close as possible to the original state is at the core of CE. In product design terms, this is considered the maximisation of “product integrity”—defined as the extent to which a product remains identical to its original state over time (den Hollander, 2018).
Based on the inertia principle and the concept of product integrity, product design in CE should first prevent a product from becoming obsolete. That is, designed for long and extended use. Designing products for prolonged use can be achieved by creating products with high physical and emotional durability (den Hollander, 2018). Designing products for extended use can be achieved by providing opportunities for product maintenance (also known as preventive maintenance), repair (also known as corrective maintenance) and upgrade. Secondly, design in a CE should ensure that products that have reached obsolescence can be recovered with the highest level of integrity and put back in the system for reuse. This means creating products that can be recontextualised (also known as repurposing), refurbished and remanufactured. These first two guiding principles are aimed at preserving product integrity. But, at some point, products will reach their lifetime. Product lifetime is the period that starts when a product is released for use after manufacture and ends at the moment a product becomes obsolete beyond recovery at the product level (Cooper, 1994, 2010; Babbitt et al., 2009; Brouillat, 2015).
When products reach their lifetime in a CE, the last resort of recycling is needed. Recycling involves the dismantling and disintegration of a product and its constituent components and the subsequent reprocessing of the product's materials. This process is the least preferred option in CE since it destroys a product's integrity. However, it ensures that product materials are captured and looped back into the economic system for reuse. Figure 1 shows an overview of these CE principles that underpin product design with a focus on tangible durable consumer products proposed by den Hollander (2018).
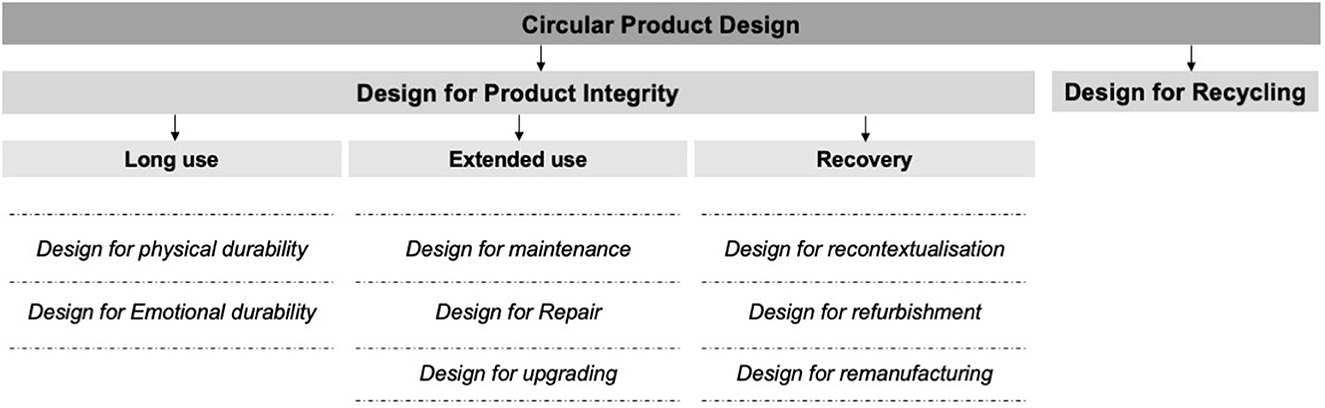
Figure 1. Guiding principles that underpin product design for a CE (den Hollander, 2018).
2.2. Circular economy design principles in the field of medical device design
Medical devices must comply with stringent safety standards before use. Safety standards, for instance, include the safe functioning of medical devices before they are used on patients. In other cases, safety standards require medical device cleaning or reprocessing before use. The cleaning or reprocessing of medical devices involves disinfection and sterilisation processes to render a contaminated medical device hygienically safe before reuse (Großkopf and Jäkel, 2008). The disinfection or sterilisation required to render a product clean is determined by the Spaulding scale (Rutala and Weber, 2008; McDonnell and Burke, 2011). This scale categorises products according to hygiene criticality and describes the requirement for reprocessing medical devices for reuse. That is, “Critical items” (e.g., surgical devices) are those which enter tissue or the vascular system. These devices must be sterilised (all organic material removed) by high pressure or steam. “Semi-critical items” (e.g., respiratory therapy and anaesthetic equipment) come in contact with the mucus membrane and require high-level chemical disinfection before reuse. Lastly, “Non-critical items” (e.g., stethoscopes and tables) are those that do not enter the body and may be lightly disinfected before reuse. These hygienic safety standards are pertinent and must be considered during medical device design. This means that applying circular product design principles in medical device design must also comply with these safety standards and thus ensure patients' health is not endangered.
So far, the aforementioned CE principles that underpin product design (as in Figure 1) have focused on consumer products, not specific on medical devices. For example, the CE principle of physical durability only considers how product performance over time degrades slower than comparable consumer products (den Hollander et al., 2017). But in the healthcare domain, medical devices designed for physical durability must withstand degradation after reprocessing over their intended lifespan (Medicare.gov, 2022). In another example, the CE principle of emotional durability concerns consumer-product relationships of attachment and how this attachment influences product replacement decisions (Page, 2014; den Hollander et al., 2017). However, a medical device's function, use or reuse is not determined by consumer-product relationships of attachment. Instead, medical devices are designed to be functional under stringent safety and regulatory standards for improving health outcomes. This CE principle of emotional durability is applicable for consumer products; however, out of scope in the medical device design domain. Table 1 shows definitions of CE design principles from a medical device perspective as derived from the literature.
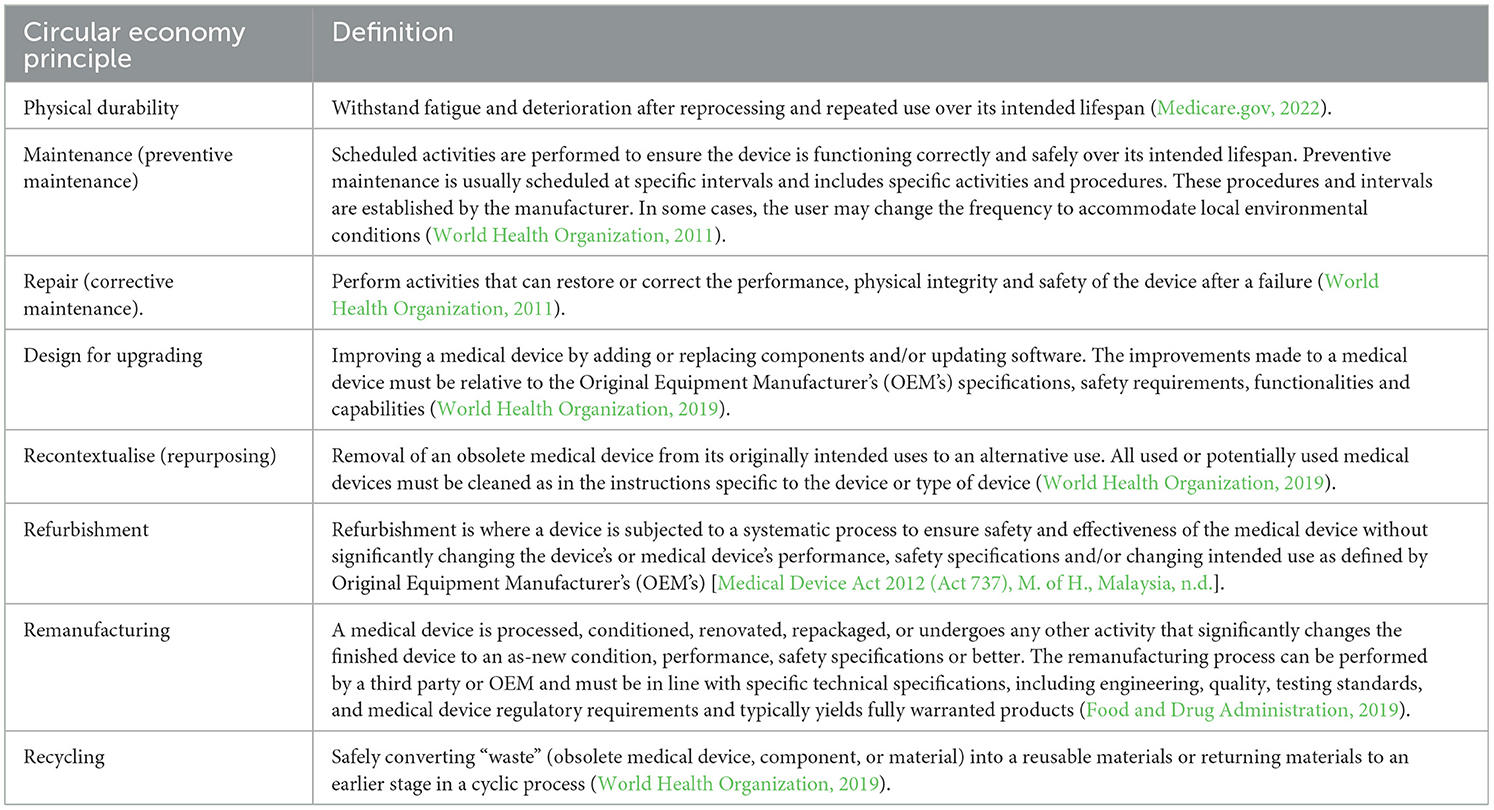
Table 1. Circular economy design principles from a medical device perspective as inferred in literature.
The definitions in Table 1 are derived from literature published by medical device regulatory and authorisation bodies. For example, Food and Drug Administration (FDA), World Health Organization (WHO) Medical Devices. These authorities are in charge with the task of defining what constitutes a medical device, its safety requirement, and its effective use or reuse (Kramer et al., 2012). As such, these are key sources to use in generating definitions on CE principles pertaining to the domain of medical devices as seen in Table 1. The CE definitions in Table 1 explicitly consider stringent safety standards that are required in the medical domain. These safety standards ensure patients' health is not put at risk in the process of improving health outcomes. The emphasis on the safety concerns in the definitions in Table 1 makes them different from CE definitions in other fields since issues of safety in improving patients' health outcomes are not required. These safety issues are critical in the medical device domain and thus important in this study that seeks to understand the extent to which CE principles have been applied in the design of medical devices for LRS in SSA.
3. Method
To understand to what extent circular economy (CE) principles have been applied in the design of medical devices specifically for low-resource settings (LRS) in Sub-Saharan Africa (SSA), a systematic review was performed. Below, the review steps are outlined and graphically summarised in Figure 2.
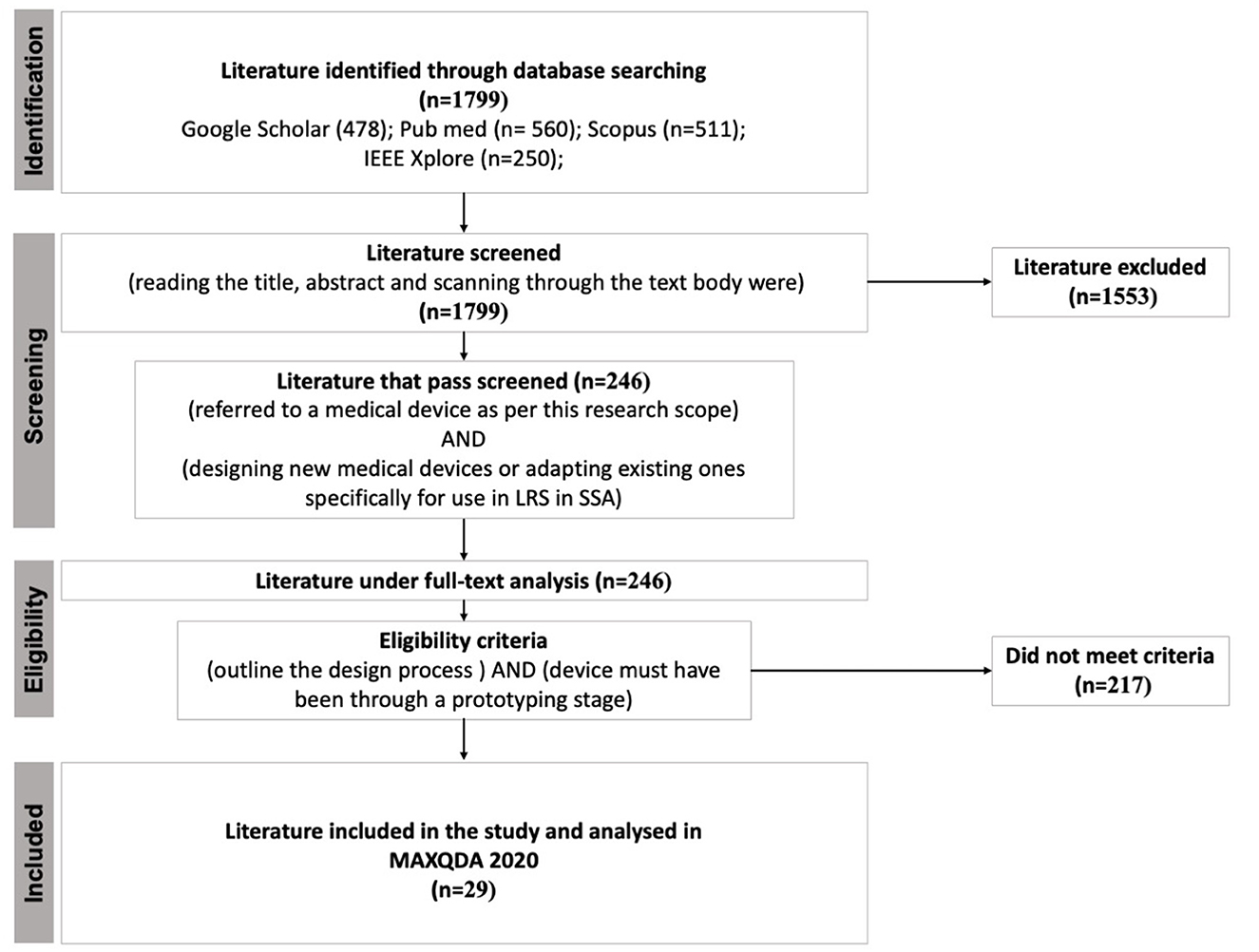
Figure 2. Summary of the systematic review process. Acronyms LRS and SSA are low-resource settings and Sub-Saharan Africa, respectively.
Studies were searched in databases such as Google Scholar, Pubmed, Scopus, and IEEE Xplore. Using logical operators AND or OR, the key terms medical, device, equipment, use, design, frugal, low cost, development, implementation, low-resource settings, Sub-Saharan Africa, Africa, developing countries, low and middle-income countries, remanufacturing, repair, refurbishing, remanufacturing, recycling, circular economy, sustainability were combined and searched. The search resulted in a total of 1,799 scientific papers that mention the design of medical devices. Only studies in English were included.
Next, studies were screened by title, abstract and scan reading of the text body by two reviewers according to the following criteria.
1. The study must refer to a medical device as per this research scope. That is, a medical device is “any instrument, apparatus, or appliance, manufactured specifically to be used for diagnosis, prevention, monitoring, and alleviation of disease or treatment of the human body, that are not solely pharmaceutical goods” (Moultrie et al., 2015; Aranda Jan et al., 2016; Medical Device Regulation (EU) 2017/745, 2017). Due to our interest in hardware design for hospitals in LRS, medicines, devices for home care, vaccines, in-vitro, mHealth or eHealth, and other telecommunication systems in healthcare were out of scope in this research.
2. The study must focus on designing new medical devices or adapting existing ones specifically for use in LRS in SSA. Studies that described misuse or unintended use of medical devices in LRS in SSA were excluded.
As a result, 246 studies fulfilled the above criteria. Subsequently, studies included in the full-text analysis were selected based on the third and fourth eligibility criteria.
3. The study must refer to and outline the design process of the described medical device. This criterion ensured that the medical device being described could be examined for content relating to the application of circular economy principles.
4. Lastly, the described device must have been through a prototyping stage.
Consequently, 29 studies met the third and fourth inclusion criteria and were entered in MAXQDA 2020 for analysis.
In MAXQDA, the 29 studies were analysed to identify CE principles (see Table 1) applied in the design of medical devices for LRS in SSA. The analysis was done by descriptive coding. During descriptive coding, a text fragment was highlighted and assigned a code (Rädiker and Kuckartz, 2020; Saldana, 2021) whenever a CE principle was mentioned (see Table 2). These text fragments that explained CE principles were identified in the selected studies by code recording units (see Table 2). A recording unit is a portion of text, sentence, word or word meanings to which an evaluator applies a code (Weber, 1990). The recording units were established in two stages. In stage one, recording units were compiled from preliminary reading through the 29 selected studies and recording keywords, text, and sentences that explained the respective CE principles as in Table 1. For example, design for “robustness” is a word that described the CE principle of “design for durability”. This process continued until the repetition of compiled recording units began to emerge. In stage two, the compiled recording units were examined by two experts with expertise in CE and medical device design. These experts examined the compiled recordings units to ensure other possible recording units were not omitted. None of the experts proposed the removal of any recording unit. Similarly, no new recording unit was proposed by the experts.
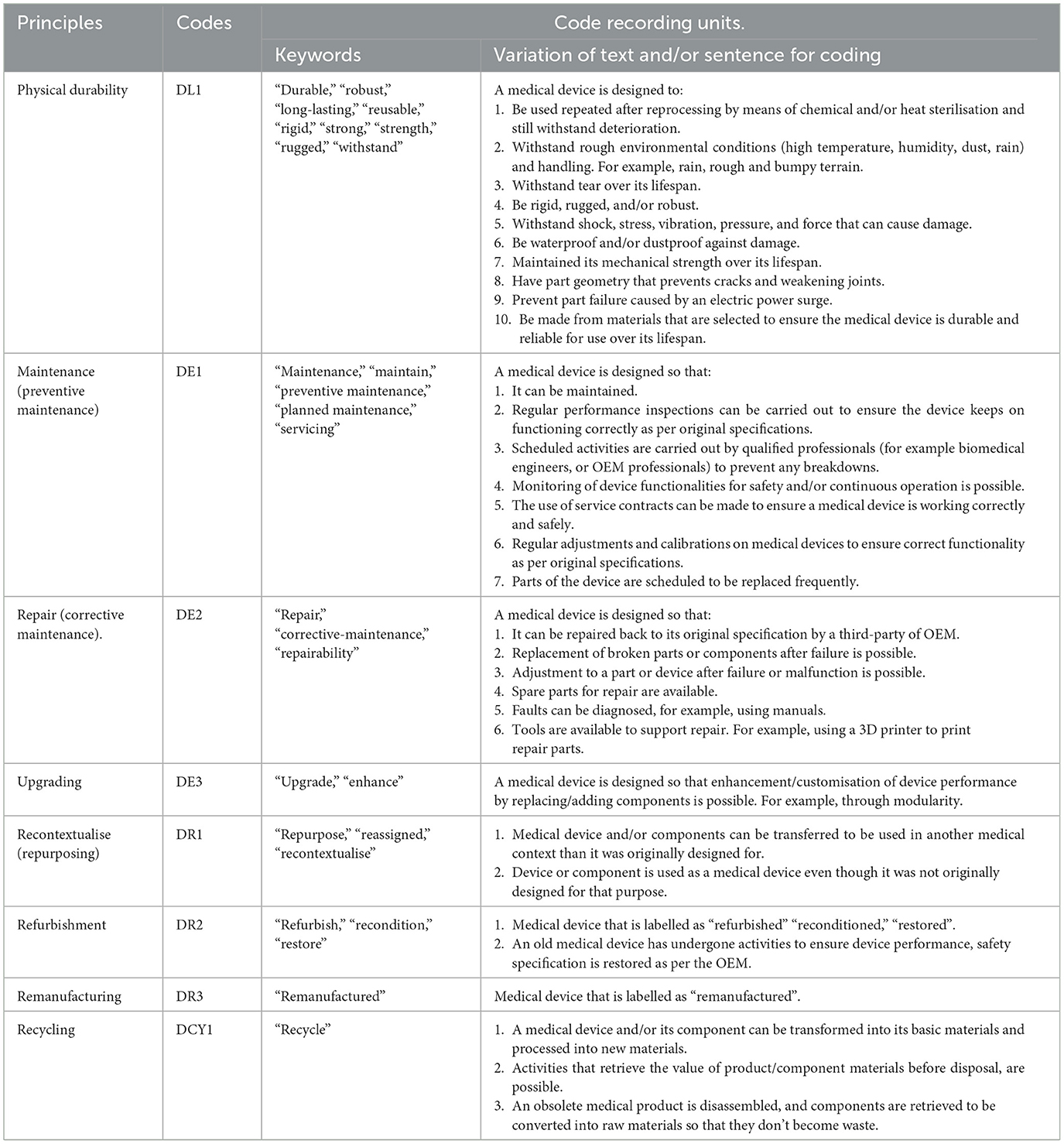
Table 2. Circular economy principles, codes and their respective recording units used in the coding process.
Using the established codes and recording unit, the analysis of the 29 studies by descriptive coding was performed by two persons. This was done to avoid confusion or misalignment of terms and definitions during the coding process. After the first coding round, a second and third iteration was conducted and finalised (see Supplementary material 1 for the coded segments). The outcome of the systematic review produced an overview of the different CE principles applied in the design of medical devices for LRS, as detailed in the results section.
4. Results
Seeking to understand the extent to which circular economy (CE) principles have been applied in the design of medical devices specifically for use in low-resource settings (LRS) in Sub-Saharan Africa (SSA), a systematic review was performed. This review analysed 29 scientific papers. These studies were published between 2006 and 2021 and described the design of 45 medical devices with different hygienic criticality and value (see Table 3). Examples of these 45 devices included a surgical suction pump, wrist flexion contracture, phototherapy unit, blood salvage device, custom-designed implants, a vest for treating jaundice, bubble continuous positive airway pressure—(bCPAP), low field MRI, electrosurgical unit, mechanically powered wound-pump, oxygen delivery system and many others. See Table 3 for the complete list of medical devices. Overall, 55.6% (25) of the devices were electromechanical (i.e., comprised electrical and mechanical parts), and 44.4% (20) were purely mechanical (i.e., had no electronic components). All 45 devices were designed for reuse. None of the devices was designed for single use (i.e., disposable).
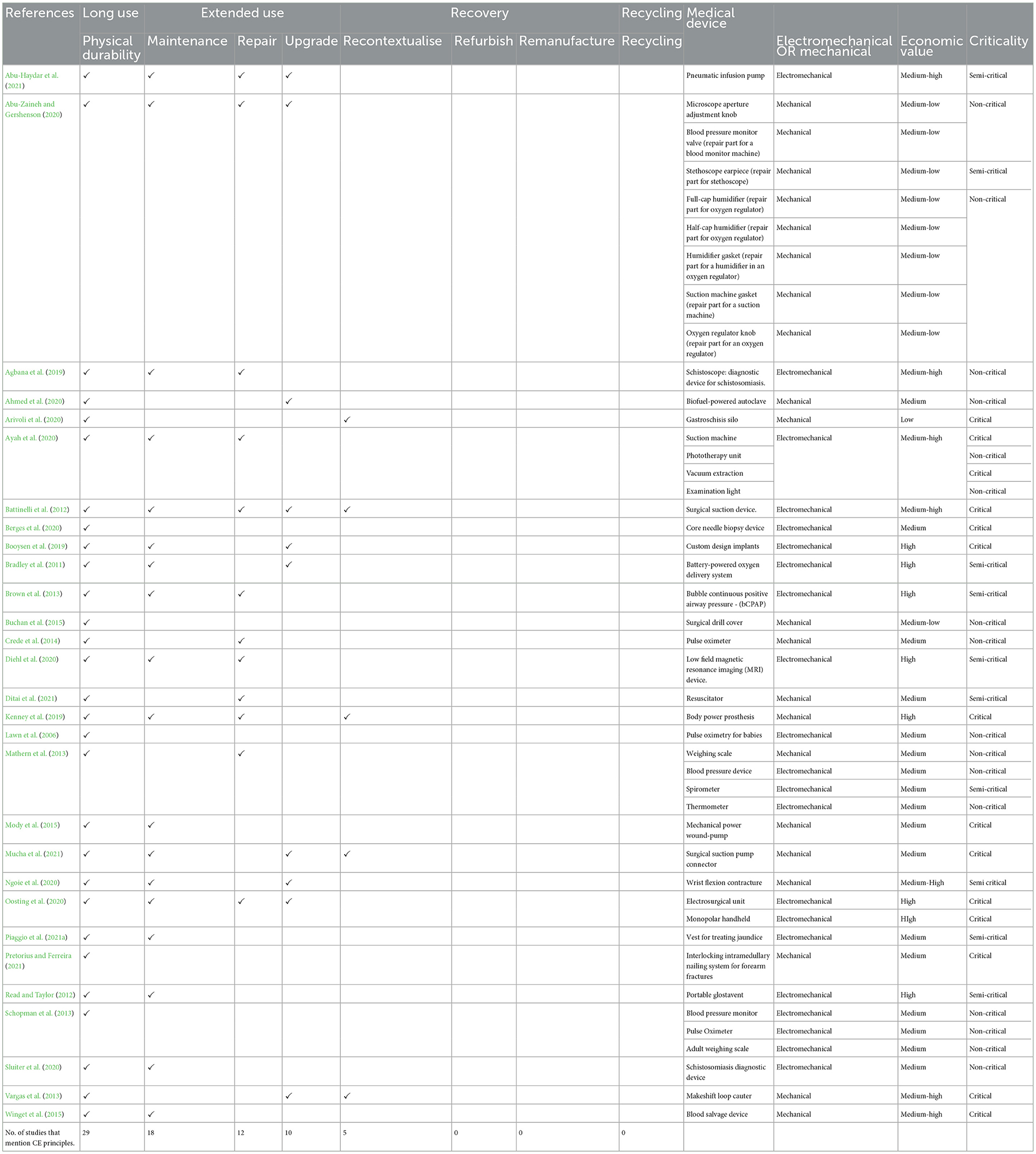
Table 3. The CE principles applied in the design of medical devices for LRS in SSA as pinpointed in the literature.
The review revealed two key findings. Firstly, medical device design for LRS in SSA significantly took into account CE principles aimed at ensuring medical device longevity. As noticed in Table 3, all 29 studies reviewed in this study made use of design for long use by ensuring product durability. Design for extending device use through maintenance was identified in 18 studies, repair in 12, and upgrade in 10. Whether these principles were intentionally applied from a CE thinking approach could not be inferred in this study. We observe that these principles aimed at product longevity were intended to ensure that medical devices are available to provide healthcare. This is, for example, demonstrated in one of the studies reviewed in this study whereby an electrosurgical unit which is often lacking in LRS, is designed to be durable, repairable, upgradable, and reusable over time even in conditions of electrical failures (Oosting et al., 2020). In the event of electrical failures, this electrosurgical unit can be repaired and upgraded by using local foot-paddle power technology to keep it functioning and thus provide surgical healthcare service for people in LRS in SSA. In another example, we observe the adaptation of existing medical devices for use in LRS. For instance, the 3D printing of components or spare parts in rural Kenya to ensure obsolete medical devices can remain functional towards providing healthcare in LRS.
Secondly and most remarkably, the CE principle of recovery through recontextualisation (repurposing) could be inferred in five of the 29 studies used (see Table 3). CE principles of recovery through refurbishment, remanufacturing and recycling could not be identified in any of the studies. The reason why these recovery and recycling principles were not considered could not be inferred in this study.
5. Discussion
The starting point for our study was the observation that principles of circular economy (CE) can be instrumental in ensuring medical devices designed for low-resource settings (LRS) in Sub-Saharan Africa (SSA) do not end up as waste. However, to what extent these principles have been applied in designing medical devices for LRS was missing in literature. We performed a systematic review to fill this gap. As expected, we observed that the design of medical devices for LRS in SSA significantly took into account CE principles aimed at ensuring medical device longevity. The motivational basis for using these principles aimed at medical device longevity is different from the environmental or resource conservation motivations implicitly assumed in the current academic literature on CE. The reason is to ensure that medical devices are available, functional, and used over time to provide access to healthcare, a fundamental human need. Moreover, in so doing so, we see the use of CE design principles such as durability, maintenance, repair, upgrade and recontextualisation that also aim at resource conservation and retaining products to be used over time. Whether these principles were intentionally applied from a CE thinking approach could not be inferred in this study.
Other studies have noticed similar patterns in LRS in SSA where the unavailability of resources has led to CE practises aimed at retaining or restoring the value of goods for as long as possible without necessarily linking them to the term “CE”. For example, Korsunova and colleagues noticed resource scarcity in LRS that resulted in “necessity-driven CE” practises such as “repair” and “reuse” to retain the goods in circulation for as long as possible (Korsunova et al., 2022). This necessity-driven CE practise adopts a mindset that looks at the long-term functionality of goods and materials that are often unavailable. As expected, this was also seen in this study whereby in providing medical devices that are often unavailable, CE principles that retain the product to be used over time are taken into account.
Designing medical devices for LRS to remain in use over a long time is certainly valuable. This can ensure that healthcare facilities in this region have access to medical devices to provide healthcare. On the other hand, what happens when these medical devices reach their end of life or become obsolete? Besides, a product can be declared obsolete prematurely though still functional. For example, a product can be declared obsolete if outperformed by a newer product (i.e., technological obsolescence) (Clay Whybark, 2007; Kane et al., 2018) or no longer legal to be used (i.e., regulatory obsolescence). In another case, a product might become obsolete when its use is no longer profitable (i.e., economic obsolescence) (Kane et al., 2018). These different forms of obsolescence might push medical devices designed for longevity to be obsolete and end up disposed of as waste. As such, it is important to consider processes that ensure medical devices that become obsolete or reach end of life can be recovered and put back in the economic system for reuse. For example, recovery through refurbishment, remanufacturing, or recycling of medical devices.
Products designed to be recovered and recycled can continue circulating in the economic system at the highest value and not end up disposed of as waste (Mangers et al., 2021; Ellen MacArthur Foundation, 2022b). Most remarkably, this study revealed that CE principles ensuring medical devices can be recovered through refurbishment, remanufacturing, and recycling could not be inferred in any of the studies reviewed. Refurbishment and remanufacturing may have value for medical devices. For example, refurbishment and remanufacturing activities can ensure that medical devices can be recovered and rejuvenated and used again (Steinhilper and Weiland, 2015; Oturu et al., 2022). Similarly, refurbishment and remanufacturing processes can ensure essential product components are brought back to the original condition (Eze et al., 2019, 2020; Boorsma et al., 2021; Oturu et al., 2022) to be reused again.
Refurbishment and remanufacturing activities are already happening in LRS in SSA for other electronic devices. For example, the Otigba computer village in Nigeria, a hub for new and used imported computers, and refurbished devices, has over 2,500 daily sales, including assembling, repairing, and refurbishing units for computers (Zeng, 2008). In Accra and Lagos alone, the repair, refurbishment and remanufacture sector generates income for more than 30,000 people (Schluep et al., 2012). Refurbishment and remanufacturing present a real economic opportunity for LRS in SSA though barriers remain.
Specific to LRS in SSA, Oturu et al. (2022) described some barriers regarding refurbishment and remanufacturing of medical devices. Among these barriers, are the lack of legislation and infrastructure to support the remanufacturing of medical devices (Oturu et al., 2022). Similarly, the lack of established medical device Original Equipment Manufacturers (OEM) in SSA that can provide refurbishment or remanufacturing services (Oturu et al., 2022). However, this review could not identify whether these barriers were the reasons for not considering the CE principles of refurbishment or remanufacturing. Also, the type of medical devices which are most commonly refurbished or remanufactured are devices that are of high value (e.g., Magnetic resonance imaging machine) (Dremed, 2015; Kane et al., 2018; Guzzo et al., 2020). In other cases, small and medium-valued equipment is refurbished by replacing specific components (Kruger, 2008). Based on this rationale, we can speculate that some of the devices designed for longevity, as per this study (see Table 3), can also benefit from refurbishment and remanufacturing processes. For example, the high-value devices mentioned in Table 3. However, another factor influencing whether a device can be refurbished or remanufactured includes financial considerations (Guzzo et al., 2020). That is, whether it is financially viable to recover the product or to discard and replace it. Similarly, hygienic criticality is another factor frequently considered when recovering medical devices. High-criticality devices must be hygienically recovered to be refurbished or remanufactured using more aggressive chemical decontamination or sterilisation processes than low- or medium-criticality devices (Kane et al., 2018). This will mean designing devices with materials which can withstand such chemical decontamination or sterilisation processes (Kane et al., 2018).
The CE principle of recycling in design is also critical to ensure that medical devices and their constituent material are conserved in the economic system. Though none of the studies reviewed mentioned the use of this principle in designing medical devices for LRS in SSA, it is essential to consider them in the future. This is because recycling which is the last resort for resource conservation can ensure a product's constituent materials stay in the economic system and do not end up as waste (Allwood, 2014; Mangers et al., 2021; Ellen MacArthur Foundation, 2022b). For example, recycling electronics allows for precious and unique metals to be recovered and thus reducing the environmental impact associated with electronic manufacturing from raw materials (Namias, 2013). In essence, all the devices identified in this study (see Table 3) could benefit from recycling processes.
Recycling processes and infrastructure are present in LRS in SSA (Korsunova et al., 2022) and can support recycling of medical and other electronic devices. For example, the WEEE centre (Ongondo, 2013; Vanegas et al., 2014; WEEE Centre, n.d.) in Kenya provides electronic waste recycling services in East and Central Africa and the Hinckley recycling centre in Lagos, Nigeria, provides recycling of electronic services in West Africa (Nnorom and Odeyingbo, 2020; Hinckley Associates, 2022). These recycling services include collection, sorting and separating electronic waste to be reused or broken down into raw material form. Similarly, the company Mr. Green Africa provides plastic recycling services in East and Central Africa (Mr. Green Africa, 2022). Its services include collecting, sorting, pelletising, trading and reprocessing recycled plastics into high-quality products (Mr. Green Africa, 2022). With such recycling infrastructures, capturing medical devices' material value is possible in LRS in SSA.
The limitation of our study is that it only investigates the extent to which CE principles have been applied in the design of medical devices for LRS from a scientific literature review perspective. CE design principles could also be implemented at the industry level and has yet to make its way into scientific literature. We speculate that investigating the same topic using industry case inquiry methods can reveal new findings on the extent to which CE has been applied in designing medical devices for LRS in SSA.
6. Conclusion
This study is an endeavour to understand to what extent Circular Economy (CE) principles have been applied in the design of medical devices, specifically for low-resource settings (LRS) in Sub-Saharan Africa (SSA). Based on a systematic review and as expected, this study shows that CE principles of durability, repair, maintenance, and upgrade and medical device longevity can be inferred in the domain of medical device design for LRS in SSA. Whether these principles were intentionally applied from a CE approach could not be inferred in this study. The motivation for using these principles is to ensure medical devices are available to provide access to healthcare in LRS as opposed to the environmental or material flow motivation often found in CE literature. The motivational basis towards providing healthcare for people should remain a core aspect when designing medical devices for LRS in SSA. This research further revealed that other CE principles that ensure medical devices and constituent materials are recovered (i.e., through refurbishment and remanufacturing) and recycled were hardly taken into account. Underlying reasons for this could not be established in this review. This, therefore, presents an opportunity for further research. It is vital to understand why CE principles of refurbishment, remanufacturing, and recycling are not considered when designing medical devices for LRS. And what needs to be in place if these CE principles were considered in medical device design?
The findings in this study are intended to be used as a starting point to explore how CE principles can be used to support the design of medical devices for LRS in SSA. This study now provides insights into the extent to which CE has inadvertently been applied in designing medical devices for LRS in SSA. It further shows the gaps in the lack of attention to recovery and recycling considerations when designing medical devices for LRS in SSA. Designers of medical devices can leverage this research to contribute towards developing medical devices that support access to healthcare for people in LRS and preserve earth's finite resources.
6.1. Implications of this research to academicians and practicians
This study shows CE design principles are incorporated in medical device design for LRS, and a firm grasp of the concept can be instrumental and ensure that medical devices continue to be designed for longevity and do not end up disposed of as waste into the environment. Academicians and medical device designers can explore how CE as a concept can be integrated in the design of medical devices that can remain used over time to provide healthcare for people in LRS. For example, developing methods or approaches that can guide medical device design while explicitly considering CE aspects that benefit people in LRS and the environment. Established medical device design frameworks for LRS could also be updated to explicitly take into account these CE aspects. For example, A Framework for Designing Medical Devices Resilient to Low-Resource Settings (Piaggio et al., 2021b) and Towards A Framework for Holistic Contextual Design for Low-Resource Settings (Aranda Jan et al., 2016).
Design is only one factor that can ensure CE is implemented in the domain of medical device design. Other factors such as policies and regulations are equally needed to for example incentivise a CE thinking approach in the design and use of medical devices in LRS in SSA. Academicians and policymakers can put forward policies and regulations that support the design of medical devices that can remain used over time in LRS to save lives while preserving the environment. For example, regulations that incentivise the remanufacturing, refurbishment, and recycling of medical devices. So far, these CE principles are yet to be implemented in the design of medical devices for LRS as per this study.
Author contributions
KS determined the overall structure of the article, wrote the manuscript, and managed co-author inputs from RO, CB, and JCD. All authors reviewed the research design and interpretation and provided recommendations for critical content. All authors read and approved the final manuscript.
Acknowledgments
We thank the Faculty of Industrial Design Engineering at the Delft University of Technology for their support in conducting this research.
Conflict of interest
The authors declare that the research was conducted in the absence of any commercial or financial relationships that could be construed as a potential conflict of interest.
Publisher's note
All claims expressed in this article are solely those of the authors and do not necessarily represent those of their affiliated organizations, or those of the publisher, the editors and the reviewers. Any product that may be evaluated in this article, or claim that may be made by its manufacturer, is not guaranteed or endorsed by the publisher.
Supplementary material
The Supplementary Material for this article can be found online at: https://www.frontiersin.org/articles/10.3389/frsus.2023.1079685/full#supplementary-material
References
Abu-Haydar, E., Katuntu, D., Bauer, J., Wollen, A., Eisenstein, M., Sherman-Konkle, J., et al. (2021). User-centered design: developing the RELI delivery system – a low-cost, non-electric, pneumatic infusion pump. Med. Devices 14, 185–192. doi: 10.2147/MDER.S295893
Abu-Zaineh, R., and Gershenson, J. (2020). “Medical device spare part pricing strategy analysis for developing nations,” in 2020 IEEE Global Humanitarian Technology Conference (GHTC) (Seattle, WA), 1–5. doi: 10.1109/GHTC46280.2020.9342869
Adam, I., Walker, T. R., Bezerra, J. C., and Clayton, A. (2020). Policies to reduce single-use plastic marine pollution in West Africa. Marine Policy 116, 103928. doi: 10.1016/j.marpol.2020.103928
Agbana, T., Oladepo, O., Vdovin, G., Oyibo, W., Van, G.-Y., and Diehl, J. C. (2019). “Schistoscope: towards a locally producible smart diagnostic device for Schistosomiasis in Nigeria,” in 2019 IEEE Global Humanitarian Technology Conference (Seattle, WA).
Ahmed, Y. K., Ibitoye, M. O., Zubair, A. R., Oladejo, J. M., Yahaya, S. A., Abdulsalam, S. O., et al. (2020). Low-cost biofuel-powered autoclaving machine for use in rural health care centres. J. Med. Eng. Technol. 44, 489–497. doi: 10.1080/03091902.2020.1825847
Allwood, J. M. (2014). “Chapter 30 - squaring the circular economy: the role of recycling within a hierarchy of material management strategies,” in Handbook of Recycling, eds E. Worrell, and M. A. Reuter (Boston, MA: Elsevier), 445–477.
Andrady, A. L. (2011). Microplastics in the marine environment. Mar. Pollut. Bull. 62, 1596–1605. doi: 10.1016/j.marpolbul.2011.05.030
Anyangwe, S. C. E., and Mtonga, C. (2007). Inequities in the global health workforce: the greatest impediment to health in sub-Saharan Africa. Int. J. Environ. Res. Public Health 4, 93–100. doi: 10.3390/ijerph2007040002
Aranda Jan, C. B., Jagtap, S., and Moultrie, J. (2016). Towards a framework for holistic contextual design for low-resource settings. Int. J. Design 10, 43–63. doi: 10.17863/CAM.7254
Arivoli, M., Biswas, A., Burroughs, N., Wilson, P., Salzman, C., Kakembo, N., et al. (2020). Multidisciplinary development of a low-cost gastroschisis silo for use in sub-Saharan Africa. J. Surg. Res. 255, 565–574. doi: 10.1016/j.jss.2020.05.037
Ayah, R., Ong'ech, J., Mbugua, E. M., Kosgei, R. C., Waller, K., and Gathara, D. (2020). Responding to maternal, neonatal and child health equipment needs in Kenya: a model for an innovation ecosystem leveraging on collaborations and partnerships. BMJ Innovat. 6, 391. doi: 10.1136/bmjinnov-2019-000391
Ayres, R. U. (1994). Industrial Metabolism: Theory and Policy. The Greening of Industrial Ecosystems. Washington, DC: National Academy Press, 23–37.
Babbitt, C. W., Kahhat, R., Williams, E., and Babbitt, G. A. (2009). Evolution of product lifespan and implications for environmental assessment and management: a case study of personal computers in higher education. Environ. Sci. Technol. 43, 5106–5112. doi: 10.1021/es803568p
Bakker, C., and Poppelaars, R. (2018). Design for product integrity in a circular economy. Design. Circ. Econ. 148–156. doi: 10.4324/9781315113067-14
Battinelli, E., Holmquest, K., Musso, J., Singh, P., and Dougherty, E. (2012). “Low cost, low power 12VDC surgical suction device for use in developing countries,” in 2012 IEEE Global Humanitarian Technology Conference (Seattle, WA), 342–344. doi: 10.1109/GHTC.2012.51
Behuria, P. (2021). Ban the (plastic) bag? Explaining variation in the implementation of plastic bag bans in Rwanda, Kenya and Uganda. Environ. Plann. C Polit. Space 39, 1791–1808. doi: 10.1177/2399654421994836
Berges, A. J., Callanan, M., Zawicki, V., Shi, R., Athey, T., Ayyappan, V., et al. (2020). A novel intermediate attachment to reduce contamination in reusable core needle biopsy devices. J. Med. Devices Transact. ASME 14, 1. doi: 10.1115/1.4045967
Bigna, J. J., and Noubiap, J. J. (2019). The rising burden of non-communicable diseases in sub-Saharan Africa. Lancet Global Health 7, e1295–e1296. doi: 10.1016/S2214-109X(19)30370-5
Bocken, N. M. P., de Pauw, I., Bakker, C., and van der Grinten, B. (2016). Product design and business model strategies for a circular economy. J. Ind. Prod. Eng. 33, 308–320. doi: 10.1080/21681015.2016.1172124
Boorsma, N., Balkenende, R., Bakker, C., Tsui, T., and Peck, D. (2021). Incorporating design for remanufacturing in the early design stage: a design management perspective. J. Remanufact. 11, 25–48. doi: 10.1007/s13243-020-00090-y
Booysen, G. J., van der Merwe, A. F., and de Beer, D. J. (2019). Additive manufacturing for sustainable custom-designed implants. South Afr. J. Ind. Eng. 30, 21–31. doi: 10.7166/30-3-2266
Bradley, B., Cheng, Y.-L., Peel, D., Mullally, S., and Howie, S. (2011). “Assessment of power availability and development of a low-cost battery-powered medical oxygen delivery system: for use in low-resource health facilities in developing countries,” in 2011 IEEE Global Humanitarian Technology Conference (Seattle, WA), 148–153. doi: 10.1109/GHTC.2011.25
Brouillat, E. (2015). Live fast, die young? Investigating product life spans and obsolescence in an agent-based model. J. Evol. Econ. 25, 447–473. doi: 10.1007/s00191-014-0385-1
Brown, J., Machen, H., Kawaza, K., Mwanza, Z., Iniguez, S., Lang, H., et al. (2013). A high-value, low-cost bubble continuous positive airway pressure system for low-resource settings: technical assessment and initial case reports. PLoS ONE 8, e53622. doi: 10.1371/journal.pone.0053622
Buchan, L. L., Black, M. S., Cancilla, M. A., Huisman, E. S., Kooyman, J. J. R., Nelson, S. C., et al. (2015). Making safe surgery affordable: Design of a surgical drill cover system for scale. J. Orthop. Trauma 29, S29–S32. doi: 10.1097/BOT.0000000000000403
Chisholm, J. M., Zamani, R., Negm, A. M., Said, N., Abdel daiem, M. M., Dibaj, M., et al. (2021). Sustainable waste management of medical waste in African developing countries: a narrative review. Waste Manag. Res. 39, 1149–1163. doi: 10.1177/0734242X211029175
Ciacci, L., Reck, B. K., Nassar, N., and Graedel, T. (2015). Lost by design. Environ. Sci. Technol. 49, 9443–9451. doi: 10.1021/es505515z
Clay Whybark, D. (2007). Issues in managing disaster relief inventories. Int. J. Prod. Econ. 108, 228–235. doi: 10.1016/j.ijpe.2006.12.012
Cooper, T. (2010). “The significance of product longevity,” in Longer Lasting Products: Alternatives to the Throwaway Society, ed T. Cooper (Surrey: Gower Publishing), 3–36.
Crede, S., Van der Merwe, G., Hutchinson, J., Woods, D., Karlen, W., and Lawn, J. (2014). Where do pulse oximeter probes break? J. Clin. Monit. Comput. 28, 309–314. doi: 10.1007/s10877-013-9538-2
den Hollander, M. C. (2018). Design for Managing Obsolescence: A Design Methodology for Preserving Product Integrity in a Circular Economy. Delft: Delft University of Technology. doi: 10.4233/uuid:3f2b2c52-7774-4384-a2fd-7201688237af
den Hollander, M. C., Bakker, C. A., and Hultink, E. J. (2017). Product design in a circular economy: development of a typology of key concepts and terms. J. Ind. Ecol. 21, 517–525. doi: 10.1111/jiec.12610
Di Pietro, L., Piaggio, D., Oronti, I., Maccaro, A., Houessouvo, R. C., Medenou, D., et al. (2020). A framework for assessing healthcare facilities in low-resource settings: field studies in Benin and Uganda. J. Med. Biol. Eng. 40, 526–534. doi: 10.1007/s40846-020-00546-3
Diehl, J. C., van Doesum, F., Bakker, M., van Gijzen, M., O'Reilly, T., Muhumuza, I., et al. (2020). “The embodiment of low-field MRI for the diagnosis of infant hydrocephalus in Uganda,” in 2020 IEEE Global Humanitarian Technology Conference (GHTC) (Seattle, WA), 1–8. doi: 10.1109/GHTC46280.2020.9342879
Ditai, J., Barry, A., Burgoine, K., Mbonye, A. K., Wandabwa, J. N., Watt, P., et al. (2021). The babysaver: design of a new device for neonatal resuscitation at birth with intact placental circulation. Children 8. doi: 10.3390/children8060526
Dremed (2015). Refurbished Medical Equipment Options for Healthcare Facilities. Available online at: https://www.dremed.com/catalog/index.php/cPath/45_674 (accessed September 23, 2022).
Ellen MacArthur Foundation (2022a). Circular Economy in Africa: Examples and Opportunities. Available online at: https://ellenmacarthurfoundation.org/circular-economy-in-africa/overview (accessed September 21, 2022).
Ellen MacArthur Foundation (2022b). Circular Economy Introduction. Available online at: https://ellenmacarthurfoundation.org/topics/circular-economy-introduction/overview (accessed August 5, 2022).
Eze, S., Ijomah, W., and Wong, T. C. (2019). Accessing medical equipment in developing countries through remanufacturing. J. Remanufactur. 9, 207–233. doi: 10.1007/s13243-018-0065-7
Eze, S., Ijomah, W., and Wong, T. C. (2020). Remanufacturing: a potential sustainable solution for increasing medical equipment availability. J. Remanufactur. 10, 141–159. doi: 10.1007/s13243-020-00080-0
Food and Drug Administration (2019). FDA Report on the Quality, Safety, and Effectiveness of Servicing of Medical Devices.
Großkopf, V., and Jäkel, C. (2008). Legal framework conditions for the reprocessing of medical devices. GMS Krankenhhyg Interdiszip 3, Doc24.
Guzzo, D., Carvalho, M. M., Balkenende, R., and Mascarenhas, J. (2020). Circular business models in the medical device industry: paths towards sustainable healthcare. Resour. Conserv. Recycl. 160, 104904. doi: 10.1016/j.resconrec.2020.104904
Hapuwatte, B. M., and Jawahir, I. S. (2021). Closed-loop sustainable product design for circular economy. J. Ind. Ecol. 25, 1430–1446. doi: 10.1111/jiec.13154
Haupt, M., Vadenbo, C., and Hellweg, S. (2017). Do we have the right performance indicators for the circular economy?: Insight into the swiss waste management system. J. Ind. Ecol. 21, 615–627. doi: 10.1111/jiec.12506
Hinckley Associates (2022). Collect & Recycle Service. HP Service Center, Lagos - Nigeria. Available at: https://hinckley.com.ng/collect-and-recycle-service/ (accessed August 17, 2022).
Howitt, P., Darzi, A., Yang, G.-Z., Ashrafian, H., Atun, R., Barlow, J., et al. (2012). Technologies for global health. Lancet 380, 507–535. doi: 10.1016/S0140-6736(12)61127-1
Jensen, J. P., Prendeville, S. M., Bocken, N. M., and Peck, D. (2019). Creating sustainable value through remanufacturing: three industry cases. J. Clean. Prod. 218, 304–314. doi: 10.1016/j.jclepro.2019.01.301
Kane, G. M., Bakker, C. A., and Balkenende, A. R. (2018). Towards design strategies for circular medical products. Resour. Conserv. Recycl. 135, 38–47. doi: 10.1016/j.resconrec.2017.07.030
Kenney, M., Ramirez, K., alibbala, Ackers,, L., and Ssekitoleko, R. (2019). Prosthetics Services in Uganda -A Series of Studies to Inform the Design of a Low Cost, but Fit-for-Purpose, Body-Powered Prosthesis. Geneva: World Health Organization. Available online at: https://usir.salford.ac.uk/id/eprint/52174
Korsunova, A., Halme, M., Kourula, A., Levänen, J., and Lima-Toivanen, M. (2022). Necessity-driven circular economy in low-income contexts: how informal sector practices retain value for circularity. Global Environ. Change 76, 102573. doi: 10.1016/j.gloenvcha.2022.102573
Kramer, D. B., Xu, S., and Kesselheim, A. S. (2012). Regulation of medical devices in the United States and European Union. N. Engl. J. Med. 366, 848–855. doi: 10.1056/NEJMhle1113918
Kruger, C. M. (2008). Processing single-use medical devices for use in surgery-importance, status quo and potential. GMS Krankenhhyg Interdisziplinar 3.
Lawn, J., Wyatt, J., Woods, D., Bezuidenhout, H., Lawn, J., Wyatt, J., et al. (2006). “Poster 3: are you blue yet? Developing low cost, alternative powered pulse oximetry for ill babies and children,” in 2006 The 4th Institution of Engineering and Technology Seminar on Appropriate Healthcare Technologies for Developing Countries (London), 83–88.
Lifset, R., and Graedel, T. E. (2002). “Industrial ecology: goals and definitions,” in A Handbook of Industrial Ecology (Elgar Online from Edward Elgar Publishing), 3–15. doi: 10.4337/9781843765479.00009
Mangers, J., Minoufekr, M., Plapper, P., and Kolla, S. (2021). An innovative strategy allowing a holistic system change towards circular economy within supply-chains. Energies 14, 4375. doi: 10.3390/en14144375
Masum, H., Chakma, J., Simiyu, K., Ronoh, W., Daar, A. S., and Singer, P. A. (2010). Venture funding for science-based African health innovation. BMC Int. Health Hum. Rights 10, S12. doi: 10.1186/1472-698X-10-S1-S12
Mathern, R. M., Schopman, S., Kalchthaler, K., Mehta, K., and Butler, P. (2013). Design of affordable and ruggedized biomedical devices using virtual instrumentation. J. Med. Eng. Technol. 37, 237–251. doi: 10.3109/03091902.2013.785608
McDonnell, G., and Burke, P. (2011). Disinfection: is it time to reconsider Spaulding? J. Hosp. Infect. 78, 163–170. doi: 10.1016/j.jhin.2011.05.002
Medical Device Act 2012 (Act 737) M. of H. Malaysia (n.d.). GRPMD - Medical Device Authority (MDA). Available online at: https://www.mda.gov.my/announcement/275-grpmd.html (accessed October 10 2022).
Medical Device Regulation (EU) 2017/745. (2017). Regulation (EU) 2017/745 of the European Parliament and of the Council of 5 April 2017 on medical devices, amending Directive 2001/83/EC, Regulation (EC) No 178/2002 and Regulation (EC) No 1223/2009 and repealing Council Directives 90/385/EEC and 93/42/EEC (Text with EEA relevance). Available online at: https://eur-lex.europa.eu/eli/reg/2017/745/oj (accessed March 9, 2022).
Medicare.gov (2022). Durable Medical Equipment Coverage. Available online at: https://www.medicare.gov/coverage/durable-medical-equipment-dme-coverage (accessed May 9, 2022).
Mestre, A., and Cooper, T. (2017). Circular product design. A multiple loops life cycle design approach for the circular economy. Design J. 20, S1620–S1635. doi: 10.1080/14606925.2017.1352686
Miesen, M. (2013). The Inadequacy of Donating Medical Devices to Africa. The Atlantic. Available at: https://www.theatlantic.com/international/archive/2013/09/the-inadequacy-of-donating-medical-devices-to-africa/279855/ (accessed December 2 2021).
Mody, G. N., Mutabazi, V., Zurovcik, D. R., Bitega, J. P., Nsanzimana, S., Harward, S. H., et al. (2015). Design, testing, and scale-up of medical devices for global health: negative pressure wound therapy and non-surgical male circumcision in Rwanda. Global. Health 11, 20. doi: 10.1186/s12992-015-0101-4
Moultrie, J., Sutcliffe, L., and Maier, A. (2015). Exploratory study of the state of environmentally conscious design in the medical device industry. J. Clean. Prod. 108, 363–376. doi: 10.1016/j.jclepro.2015.06.014
Mr. Green Africa (2022). Mr. Green Africa| Plastic Recycling. Kenya. mrgreenafrica. Available online at: https://www.mrgreenafrica.com (accessed October 11, 2022).
Mucha, A., Dubbink, J. H., Persaud, S., Athiban, A. S., and Diehl, J. C. (2021). “Improving the use of surgical suction pumps in Sierra Leone,” in 2021 IEEE Global Humanitarian Technology Conference (GHTC) (Seattle, WA), 119–126. doi: 10.1109/GHTC53159.2021.9612501
Namias, J. (2013). The Future of Electronic Waste Recycling in the United States: Obstacles and Domestic Solutions. Columbia University.
Ncube, L. K., Ude, A. U., Ogunmuyiwa, E. N., Zulkifli, R., and Beas, I. N. (2021). An overview of plastic waste generation and management in food packaging industries. Recycling 6, 12. doi: 10.3390/recycling6010012
Ngoie, M., Degez, F., Sané-Diatta, I., Diamé-Seydi, Y., Gueye, M., and Coulibaly-Ndiaye, N. F. (2020). Wrist opener splint: an effective way to treat chronic wrist flexion contracture. Hand Surg. Rehabil. 39, 256–260. doi: 10.1016/j.hansur.2020.02.003
Nnorom, I. C., and Odeyingbo, O. A. (2020). “14 - Electronic waste management practices in Nigeria,” in Handbook of Electronic Waste Management, eds M. N. V. Prasad, M. Vithanage, and A. Borthakur (Oxford: Butterworth-Heinemann), 323–354.
Oberoi, G., and Garg, A. (2021). Single-use plastics: a roadmap for sustainability? Supremo Amicus 24, 585.
Ongondo, F. O. (2013). Trends, Challenges and Innovation in Management of WEEE in Kenya. Available online at: https://eprints.soton.ac.uk/358484/ (accessed August 5, 2022).
Oosting, R. M., Ouweltjes, K., Hoeboer, M. D. B., Hesselink, L., Madete, J., Diehl, J., et al. (2020). A context-specific design of an electrosurgical unit and monopolar handheld to enhance global access to surgical care: a design approach based on contextual factors. J. Med. Device. 14, 12. doi: 10.1115/1.4045966
Oturu, K., Ijomah, W., Broeksmit, A., Reig, D. H., Millar, M., Peacock, C., et al. (2021). Investigation of remanufacturing technologies for medical equipment in the UK and context in which technology can be exported in the developing world. J. Remanufactur. 11, 227–242. doi: 10.1007/s13243-021-00102-5
Oturu, K., Ijomah, W., Orr, A., Verpeaux, L., Broadfoot, B., Clark, S., et al. (2022). Remanufacturing of single-use medical devices: a case study on cross-border collaboration between the UK and Nigeria. Health Technol. 12, 273–283. doi: 10.1007/s12553-022-00641-2
Page, T. (2014). Product attachment and replacement: implications for sustainable design. Int. J. Sustain. Design 2, 265–282. doi: 10.1504/IJSDES.2014.065057
Perry, L., and Malkin, R. (2011). Effectiveness of medical equipment donations to improve health systems: how much medical equipment is broken in the developing world? Med. Biol. Eng. Comput. 49, 719–722. doi: 10.1007/s11517-011-0786-3
Piaggio, D., Andellini, M., Taher, M., and Pecchia, L. (2021a). “A vest for treating jaundice in low-resource settings,” in 2021 IEEE International Workshop on Metrology for Industry 4.0 IoT (MetroInd4.0 IoT) (Rome), 122–127. doi: 10.1109/MetroInd4.0IoT51437.2021.9488431
Piaggio, D., Castaldo, R., Cinelli, M., Cinelli, S., Maccaro, A., and Pecchia, L. (2021b). A framework for designing medical devices resilient to low-resource settings. Global. Health 17, 64. doi: 10.1186/s12992-021-00718-z
Piaggio, D., Medenou, D., Houessouvo, R. C., and Pecchia, L. (2019). “Donation of medical devices in low-income countries : preliminary results from field studies,” in IFMBE Proceedings (Banja Luka: International Federation for Medical and Biological Engineering), 423–427.
Prendeville, S., Sanders, C., Sherry, J., and Costa, F. (2014). Circular Economy: Is It Enough. Wales: EcoDesign Centre, 21.
Pretorius, H. S., and Ferreira, N. (2021). Orthopedic implant design from concept to final tested product: a design surgeon's experience. J. Limb Length. Reconstruct. 7, 45. doi: 10.4103/jllr.jllr_2_21
Rädiker, S., and Kuckartz, U. (2020). Focused Analysis of Qualitative Interviews With MAXQDA. Berlin: MAXQDA Press.
Read, E., and Taylor, E. (2012). Portable diamedica glostavent: an anaesthetic machine for the itinerant anaesthetist. Br. J. Anaesth. 109, 648–649. doi: 10.1093/bja/aes334
Richards-Kortum, R., and Oden, M. (2013). Devices for low-resource health care. Science 342, 1055–1057. doi: 10.1126/science.1243473
Rutala, W. A., and Weber, D. J. (2008). Guideline for disinfection and sterilization in healthcare facilities. Centre for Disease Control and Prevention (CDC).
Sariatli, F. (2017). Linear economy versus circular economy: a comparative and analyzer study for optimization of economy for sustainability. Visegrad J. Bioecon. Sustain. Dev. 6, 31–34. doi: 10.1515/vjbsd-2017-0005
Schluep, M., Terekhova, T., Manhart, A., Muller, E., Rochat, D., and Osibanjo, O. (2012). Where are WEEE in Africa? Berlin: IEEE.
Schopman, S., Kalchthaler, K., Malthern, R., Mehta, K., and Butler, P. (2013). Ruggedising biomedical devices for field-testing in resource-constrained environments: context, issues and solutions. J. Human. Eng. 2, 17–26. doi: 10.36479/jhe.v2i1.17
Sikdar, S. (2019). Circular economy: is there anything new in this concept? Clean. Technol. Environ. Policy 21, 1173–1175. doi: 10.1007/s10098-019-01722-z
Sluiter, M., Onasanya, A., Oladepo, O., van Engelen, J., Keshinro, M., Agbana, T., et al. (2020). “Target product profiles for devices to diagnose urinary schistosomiasis in Nigeria,” in 2020 IEEE Global Humanitarian Technology Conference (GHTC) (Seattle, WA), 1–8. doi: 10.1109/GHTC46280.2020.9342953
Ssekitoleko, R. T., Arinda, B. N., Oshabahebwa, S., Namuli, L. K., Mugaga, J., Namayega, C., et al. (2021). The status of medical devices and their utilization in 9 tertiary hospitals and 5 research institutions in Uganda. GlobalCE 4, 15–25. doi: 10.31354/globalce.v4i3.127
Stahel, W. R. (1994). The Utilization-Focused Service Economy: Resource Efficiency and Product-Life Extension. The Greening of Industrial Ecosystems (Washington, DC: National Academy Press), 178–190.
Steinhilper, R., and Weiland, F. (2015). Exploring new horizons for remanufacturing an up-to-date overview of industries, products and technologies. Pro. CIRP 29, 769–773. doi: 10.1016/j.procir.2015.02.041
Stoler, J., Weeks, J. R., and Fink, G. (2012). Sachet drinking water in Ghana's Accra-Tema metropolitan area: past, present, and future. J. Water Sanit. Hyg. Dev. 2, 223–240. doi: 10.2166/washdev.2012.104
Udofia, E. A., Fobil, J. N., and Gulis, G. (2015). Solid medical waste management in Africa. Afr. J. Environ. Sci. Technol. 9, 244–254. doi: 10.5897/AJEST2014.1851
United Nations and Department of Economic and Social Affairs (2017). World Population Prospects: The 2017 Revision, Key Findings and Advance Tables. Working Paper No. ESA/P/WP/248 ed. Department of Economics and Social Affairs PD. New York, NY.
Vanegas, P., Peeters, J. R., Plessers, F., Cattrysse, D., and Duflou, J. R. (2014). Synergizing industrialized and developing countries to improve resource recovery for e–waste: case study Belgium–Kenya. Proc. CIRP 15, 283–288. doi: 10.1016/j.procir.2014.06.089
Vargas, J., Mayegga, E., Nuwas, E., Ellegala, D. B., Kucia, E. J., and Nicholas, J. (2013). Brain surgery in the bush: adapting techniques and technology to fit the developing world. World Neurosurg. 80, E91–E94. doi: 10.1016/j.wneu.2012.01.033
Webster, K. (2015). The Circular Economy. A Wealth of Flows. Cowes: Ellen MacArthur Foundation Publishing, 16.
WEEE Centre (n.d.). WEEE Centre. Waste Electrical Electronic Equipment Centre. Weeecentre. Available online at: https://weeecentre.com/ (accessed August 17 2022).
Winget, C. O., Fisher, T. K., Kumar, R. N., Harrington, A. H., Henker, G. E., Davenport, R. D., et al. (2015). Blood salvage device for use during ruptured ectopic pregnancy in low-resource countries. Int. J. Gynecol. Obstetr. 128, 74–75. doi: 10.1016/j.ijgo.2014.07.036
World Health Organization (2017). Leave No One Behind: Strengthening Health Systems for UHC and the SDGs in Africa. Geneva.
Keywords: circular economy principles, medical device design, low-resource settings, Sub-Saharan Africa, product design
Citation: Samenjo KT, Oosting RM, Bakker C and Diehl JC (2023) The extent to which circular economy principles have been applied in the design of medical devices for low-resource settings in Sub-Saharan Africa. A systematic review. Front. Sustain. 4:1079685. doi: 10.3389/frsus.2023.1079685
Received: 25 October 2022; Accepted: 31 March 2023;
Published: 24 April 2023.
Edited by:
Susan Snyman, African Leadership University, RwandaReviewed by:
Kamalakanta Muduli, Papua New Guinea University of Technology, Papua New GuineaDavide Piaggio, University of Warwick, United Kingdom
Richie Moalosi, University of Botswana, Botswana
Copyright © 2023 Samenjo, Oosting, Bakker and Diehl. This is an open-access article distributed under the terms of the Creative Commons Attribution License (CC BY). The use, distribution or reproduction in other forums is permitted, provided the original author(s) and the copyright owner(s) are credited and that the original publication in this journal is cited, in accordance with accepted academic practice. No use, distribution or reproduction is permitted which does not comply with these terms.
*Correspondence: Karlheinz Tondo Samenjo, ay50LnNhbWVuam8mI3gwMDA0MDt0dWRlbGZ0Lm5s