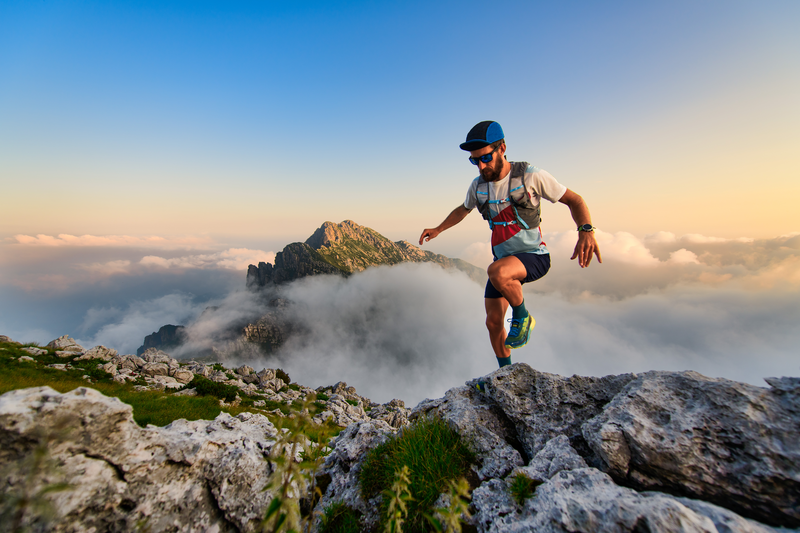
95% of researchers rate our articles as excellent or good
Learn more about the work of our research integrity team to safeguard the quality of each article we publish.
Find out more
ORIGINAL RESEARCH article
Front. Sustain. , 09 January 2023
Sec. Quantitative Sustainability Assessment
Volume 3 - 2022 | https://doi.org/10.3389/frsus.2022.943635
This article is part of the Research Topic Sustainametrics - Envisioning a Sustainable Future with Data Science View all 16 articles
The use of eco-friendly materials, waste prevention protocols, the support and participation of building construction stakeholders, polluter pays concepts, producer responsibility, life-cycle system thinking, and the application of cost-efficiency and cost minimization strategies are some of the guiding philosophies that are of extreme value when designing a waste management system via circular economy initiatives. However, it is crucial to measure the waste management strategy used in each building project. In order to measure the life-cycle performance of waste management systems and to assess how sustainable they are, this study offers a statistical methodology using a sustainametric technique to indicate how sustainable waste management system performance in emerging construction industries, particularly in South Africa. This study employs a sustainametric approach to evaluate the life-cycle performance of the waste management system of South Africa, with evidence of its sustainability performance measurement that can help advance the its waste minimization policy and implementation. The result indicates the viability of the measuring model and the findings of each metric utilized. The conclusion confirms that South Africa has not fully adopted and/or implemented a more sustainable waste management system for efficient waste minimization during its construction activities. Moreover, it is the reality that most emerging economies urgently need to expand and improve the waste reduction method employed in its construction building projects.
The acceleration in construction activity has brought about urbanization and, consequently, a quick increase in populations in several countries. However, rapid urbanization and industrialization have increased production and consumption processes, resulting in waste generation. Furthermore, because no concrete waste disposal standards exist, the environment has been clogged with garbage in numerous developing countries (Aboginije et al., 2020). Every year, thousands of demolitions occur, all of which have significant environmental and economic consequences since building materials have become unrecoverable and must be disposed of in landfills (Akinade et al., 2018; Huang et al., 2018). Furthermore, construction and building operations consume 3 billion metric tons of raw materials per year, accounting for 40% of global consumption. Similarly, annual construction production requires 170 million metric tons of basic materials and goods, 125 million metric tons of mining products, and 70 metric tons of secondary recycled and recovered products. An estimated 6 million metric tons of energy are used, and 23 metric million tons of CO2 are emitted from the process. According to global research, at least 9.0% of materials purchased for construction operations end up as waste due to on-site waste generation (Abioye and Rao, 2015).
Furthermore, as shown in Figure 1, waste created by building, demolition, and remodeling activities account for up to 40% of total waste generated in most nations. As a result, building or demolition waste might be found on job sites. Most countries dispose of approximately 15–30% of their waste in landfills (Thomas and Lizzi, 2011; Aboginije et al., 2021). Waste may be efficiently controlled at its source. Also, the amount of waste generated fluctuates depending on population density and urban growth, with roughly 80% of on-site waste being recyclable and usable. As a result, every effort is made around the world to manage building waste in a more sustainable manner (Liu et al., 2019). A sustainable waste management system (WMS) is anticipated to prevent harmful effects of waste on the environment or aesthetics, according to the UN 2017, but its efficiency is visible when it is efficiently managed (United Nations Environment Programme, 2017; Islam et al., 2020). Several waste management strategies are used to minimize on-site waste, although some of the strategies are unsuitable. Thus, a sustainable waste management system following circular economy principles is essential to reduce or eliminate waste in the construction sector, but the circular economy principles are painstakingly implemented (Ginindza and Muzenda, 2013; Aboginije et al., 2021).
Figure 1. Projection of waste generation worldwide in 2016, 2030, and 2050 by region in million metric tons (Statista, 2022).
Similarly, sustainability, utilization of eco-friendly materials, waste preventive protocol, support and involvement of building construction stakeholders, polluter pays concepts, producer responsibility, life-cycle system thinking, and the implementation of cost-efficiency and cost minimization strategies are some of the important guiding principles to follow when designing a waste system using circular economy initiatives (Nagapan et al., 2012; Velenturf et al., 2019). Furthermore, there should be legislative laws and guidance to enable the application of such sustainable WMSs. For instance, European waste legislation and prevention strategies aim to reduce waste before construction begins; this is accomplished by detailed design and material-use plans, which are critical in lowering purchase prices and the volume of recyclable materials. Although waste prevention and reduction begin with the manufacture of building materials, it is necessary to improve waste generation throughout the production process to avoid waste later in the construction process (Nagapan et al., 2012; Jingkuang and Yue, 2022). The major characteristic of a sustainable waste management system (WMS) is that it uses waste as an input material to create new value products. The goal is to reduce waste generation through reuse and recycling, minimizing the need for landfill space, extracting the maximum value from waste, and limiting the environmental effects of unavoidable wastes.
This means that by recovering materials, the volume of waste dumped in landfills may be minimized, and a sustainable waste management system can recover 90.0% of building waste (Kumar et al., 2017). Contractors also employ a variety of reuse techniques when building. For example, broken bricks and stones can be used as a subgrade to enable access to the construction site, and timber or plywood can be used to build temporary structures on site. According to Shen et al. (2004), reusing and recycling of construction materials greatly reduce landfill areas. Furthermore, storage equipment must be developed to meet the requirements for proper waste storage. Following waste storage guidelines, it should be ensured that necessary actions are taken after waste has been stored (Begum et al., 2010; Udawatta et al., 2015; Wu et al., 2016; Jingkuang et al., 2020). Unfortunately, most construction companies, especially those in developing countries, do not prioritize proper waste storage in any of their projects. Waste components must be minimized in product and building materials, or the quantity of material used, and the potential toxicity of waste generated during manufacturing and after utilization must be decreased (Jingkuang et al., 2022; Yuan, 2017).
Therefore, a sustainable waste management system is required, and while developing a waste management system, the volume or size of the trash and the composition of the waste should be considered. As many major towns are intending to close their landfills, these considerations will aid project managers in allocating the appropriate volume of landfills for each waste concern. Furthermore, this will take long time to eradicate garbage generated in construction sites each year (Bojan et al., 2017). The purpose of the WMS design is to develop a sustainable environment by meeting the waste management mandates of countries. Other suitable standards such as waste avoidance, total waste generation reduction, and creation of a product reuse system should also be an integral part of the waste management system (Aboginije et al., 2021). The requirement for sustainability measurement, which can be applied in determining any waste management system performance, including improvement in operations, performance benchmarking, progress tracking device, and process evaluation, has gained the attention of researchers.
Therefore, this research aims to provide new knowledge and understanding by providing a mechanism that can be used to assess the sustainability of any country's WMS. The scoring mechanism is used to specify whether the system is sustainable and/or whether there is a consequential exigency to optimize the system. In addition, the solution provided will be beneficial to the construction sector of several economies, especially developing countries. The research objective is to design a mechanism to measure the performance of the waste management system of any country using sustainametric techniques. The sustainametric techniques are a set of measurement variables that obey sustainability principles.
The aim of any sustainable construction in the construction sector is to achieve sustainable development, which entails integrating sustainable principles into effective strategic frameworks. The goal of this research is to provide an indicator-based framework for measuring and evaluating the sustainability of any construction and demolition waste management system. As a result, developing a good sustainability indicator is essential, and an indicator-based framework is created to accomplish this goal. The performance of social, environmental, and economic aspects is used to evaluate sustainability, according to the U.S. Department of Transportation (Moldan et al., 2012; Singh et al., 2012). Some of the most well-known and widely used sustainability measures, according to Singh et al. (2012), are corporate sustainability reporting, triple bottom line accounting, and estimates of the quality of sustainability governance for individual countries using the Global Green Economy Index (GGEI), Environmental Sustainability Index (ESI), and Environmental Performance Index (EPI).
The TBL concept results from a paradigm of sustainable development that is usually used to measure any performance, but there is a need to find a balance between the three dimensions, as illustrated in Figure 2. The TBL is described as a framework that produces nonpolluting goods and services while preserving energy and natural resources. It is also economically viable, safe, and healthy. Furthermore, it enables an organization to review its actions by considering not only the economic values generated but also the environmental and social values that might be multiplied or diminished. While achieving sustainability by balancing the triple bottom line principles is an ideal objective that can assist and guide decision-making, it will not be possible in every project. It is certainly possible to measure and report the environmental bottom line, albeit it can be a time-consuming and challenging procedure depending on the size of the company (Scerri and James, 2010; Sridhar, 2012).
Figure 2. Triple bottom line concept (Tasdemir et al., 2020).
Executing a sustainable WMS in achieving a green economy will support mitigating the climate crisis in terms of pollution prevention, among other things (Xiao et al., 2018). Since waste management is an integral part of the TBL of sustainability, companies should aim to address these issues, which require strong commitment and leadership as well as drastic changes. Several countries do not stop at merely making it viable, equitable, or bearable but, instead, aim for its sustainability. In this study, the TBL is used to understand the indications of the various impacts of sustainable waste management across the three sustainability patterns (Bell and Morse, 2008; Dalal-Clayton and Sadler, 2009; Dahl, 2012; Singh et al., 2012).
Sustainability can be measured in following ways: accounts of quantitative data, the use of narrative assessments, and the use of indicator systems. Accounting of quantitative data involves changing quantitative data into common units, like money or energy, the use of narrative assessments includes the use of graphics maps and tables, and the use of indicator systems involves organizing information from narrative assessments around indicators. Indicator-based systems can be measured easily, can be compared easily, and are more objective; hence, it is reported to be able to perform better than other methods (Dalal-Clayton and Sadler, 2009). As a result, decision-makers and stakeholders must be involved in the development of indicators in order for their values and concerns to be considered. However, the system must be both technically and scientifically sound. The system must first be specified, with an appropriate system boundary drawn, before it can be studied further. The constituents of the system include the complete input, output, emissions, energy, and other secondary aspects that should be thoroughly investigated (Dong and Hauschild, 2017).
The first step involves indicator selection. This step establishes operating circumstances, process parameters, and characteristics. The indications for which measurement is required are chosen. This serves as the system metric, which will be examined in the following steps. An assessment or measurement is carried out using proper assessing tools that have been confirmed and tested, or experiments for pre-defined indicators. This is carried out to offer a value for the indicator measurement (George and Mallery, 2003; Høgevold and Svensson, 2012). After the results have been gathered, the data are properly analyzed and interpreted, and tools are utilized to improve and change the system procedures. Because of the interdisciplinary character and complexity of the challenges that this topic embodies, measuring sustainability is difficult (Troschinetz et al., 2007; Ferro et al., 2017). Methods have emerged from various fields that are focused on ecological, economic, and social considerations. First, one must know what should be done with the results of a sustainability measure, what are the major concerns, and what are the system limitations. It is often more informative to track the growth of the entity—is it more sustainable now than it was previously? It is challenging to compare similar things due to the data complexity and diversity (countries, companies, institutions, and even products), rather than trying to explain the status of sustainability in one number or a table of numbers. The usage of imagining to portray the data is a useful way to do it (Gasparatos et al., 2008; Garcia-de-Vinuesa DL, 2018).
The ideal technique for measuring sustainability would display a tripod paradigm of pollution prevention, social equity, and economic benefit, which determines actual sustainability, and what the indicators measure must be linked through the metrics. A good indicator will track how a system becomes more or less sustainable over some time (Mayer et al., 2004; Sahely et al., 2005). The work of measuring sustainability is value-laden and socially charged, which makes studying sustainability as an objective science very difficult. According to Hammond et al. (1995) and Lele and Norgaard (1996), if the aim of the analysis is known, a multidisciplinary approach to problem conceptualization and study methodologies can be employed. Sustainability metrics are employed to measure and quantify sustainability beyond general ideas. Different international groups have their various disciplines or policies and political views, and they disagree on how sustainability should be measured.
Although sustainability metrics like reporting systems are popular among public and private sectors, they are unable to influence actual policies and practices in a society (Hermann et al., 2007; Milne and Gray, 2013). Strategies from sustainable waste management in environmental, social, and economic areas help draw the metrics used for sustainability measurement in this work. These metrics include indicators, benchmarks, audits, indexes, and accounting, as well as assessment, appraisal, and other reporting systems that are applied over a wide range of spatial and temporal dimensions, albeit they are continuously evolving. Recently, testing of intents and behaviors that are normally distributed and that pursue goals of sustainability was proposed as a methodology of sustainability monitoring. The selection of sustainability benchmark indicators was founded on sustainable principles and the life-cycle impacts of its implementation in construction projects across the construction phases. Each of the indicated metrics can be used to analyze the degree of application of sustainable strategies in the WMS of any country (Ekanayake and Ofori, 2004; Rahim and Kasim, 2017).
The demographic factors employed are grouped. Each was given a code number (e.g., 1 and 2). The objective was to show the degree to which sustainable waste reduction strategies are executed in the building construction project. This can be obtained through the contribution of respondents in a semi-structured survey put together on a scale of 1–100 with the highest score indicating very high (i.e., more than 70% execution rate). The correspondence is required to be construction professionals with vast experience and expertise with track records. From the data collected, a TBL dimension was developed to show the sustainability-based reason that includes all the dimensions of sustainability that should be considered in any project scope (Lozano, 2006; Milne and Gray, 2013; Montabon et al., 2016). This shows the three vital aspects of sustainability (social, economic, and environmental) and the variables under each as utilized by the construction industry. In terms of the distribution of each variable under an indicator across the construction life-cycle phases is tabulated. Furthermore, the set of indicators for each stage of the construction life cycle, from planning to feasibility testing to refurbishment, is described. However, certain variables cover one or more stages, indicating that they can be used in many phases.
Data collected in this study were captured, extracted, and analyzed. The last step developed a material flowchart using life-cycle mapping. This makes the sustainable performance measurement using the triple bottom line dimension possible to indicate each variable execution rate across the construction life-cycle phases. A decisive step to provide the SPM as the requirement to measure the sustainability performance of the WMS was taken. As a result, the sustainability measurement is intended to support a decision-making mechanism by providing significant information for planning future actions prioritized in any waste sector, but it is only classified as “dimensionless,” which means it is expressed in relative (percentage) measures (Nardo et al., 2005). There are also important evidence and asymmetry between the number of SPI for each triple bottom line dimension and their combination in tri-dimensional indicators (Pontius and Mclntosh, 2020). Table 1 presents the phases of the construction life cycle as construction projects advance (from the initial to the finish).
Table 1. Criteria for assessing the performance of WMS (Aboginije et al., 2020).
South Africa is the second largest economy in Africa, with a growth of 1.25% predicted in 2022 from 0.98% in 2019. The country is located in the southernmost part of the African continent and covers a total size of 1.2 million square kilometers. It is noted for its cultural variety. It is bordered by Namibia, Botswana, Zimbabwe, and Mozambique. South Africa is the largest country in Southern Africa, with three capital cities, namely, Pretoria (executive), Cape Town (legislative), and Bloemfontein (administrative; judicial). It is a multicultural society with many cultures, languages, and religions. Afrikaans, English, Ndebele, Northern Sotho, Swati, Tswana, Tsonga, Venda, Xhosa, and Zulu are the 11 official languages of South Africa, which are spoken by a diverse ethnic population. The country is divided into nine provinces, as illustrated in Figure 3 (Bundy et al., 2022). South Africa now possesses a comprehensive legislative framework because it is still a relatively emerging economy.
Figure 3. Map of South Africa showing the nine provinces (Bundy et al., 2022).
However, the significant waste management problem of the country requires rapid care. Population expansion, urbanization, a lack of compliance, and general waste management behavior are some of the predominant waste sources. The population of South Africa was predicted to reach 60.14 million in mid-2021, up around 604 281 (1.01%), from mid-2020. The country is quickly urbanizing, with one of the fastest urbanization rates in the world (DEAT, 2001; Aboginije et al., 2021). As a result, the 'trash creation rate of the country is increasing daily, and attempts are being made to reduce it to a negligible level. Figure 4 illustrates the flowchart of LCA material flow.
In this study, men formed a large proportion of respondents. From a total of 150 data samples that were retrieved, 73.8% were men and 25% were women, while 1.2% preferred not to identify their gender. In addition, construction stakeholders were evenly distributed to avoid any form of bias and to prevent any among the professionals from constituting a larger proportion of the population unnecessarily. On average, the respondents' years of experience were more than 15 years, and they had a bachelor's degree as their minimum qualification. The preponderance of respondents works in public consulting and contracting firms, followed by private firms, with government employees accounting for the least percentage of respondents. Also, it can be seen that 29.2% of respondents have worked on house estate projects, 17% have constructed roads, 12.5% have built government offices, 4.2% have experiences in civil works, 3.0% have worked on renovations, and only 1.1% have worked on other projects in construction. This is shown in Figure 5. A total of 83.9% of the respondents have had strong experience in CWM for the past 2 years.
George and Mallery (2003) indicated that internal consistency is a statistic and research metric that is based on the correlations between distinct test items, and it determines whether many items used to measure the same fundamental construct produce similar findings. Cronbach's alpha is used to determine the internal consistency of any collected sample. The complete variation was accounted for. An exploratory factor analysis (EFA) was also performed using the SPSS statistical tool (Peters, 2014). As shown in Table 2, principal axis factoring separates the variables into seven factorial components. Each cumulative deviation was calculated in percentage, and the total deviation was derived for the life-cycle phase of each building.
In the descriptive statistics, there were seven extracted items loaded into seven clusters. The variables used for this study were obtained from previous studies and primary literature sources reviewed by the researchers. In cluster 1, “Rethink and Redesign”, three factors were loaded. Avoidance of a complex design and detailing was scored the highest, with a 66.6% rating in the application, while contractual agreement on waste reduction, and design and purchase of reusable, recycled, or sustainably renewable materials were rated 33.4%, respectively, which implies that the latter was a barely used sustainable waste management strategy in the South African construction industry. In cluster 2, “Reuse”, three factors were loaded with the indication that 56.2% used a selection of materials that maximize the usable lifespan and opportunities for continuous use, 23.2% minimizing the quantity and maximizing the quality of materials, and 22.6% implementation of sustainable procurement.
In cluster 3, “Recycling”, three factors were loaded, indicating that 40.1% for use of resilience secondary materials market optimization, 30.6% utilization of recycled materials, and 20.3% provision for incentives in transactions on secondary materials. In cluster 4, “Material Recovery”, two factors were loaded, with 67.0% for material recovery maximization and 33.0% recovery of resources and energy if possible. In cluster 5, “Residual Management”, three factors were loaded, with 44.2% for the encouragement of natural resources, 34.8% minimization of negative impact on the environment, and 21.0% landfill site conservation. In cluster 6, “Policy Implementation”, two factors were loaded, with 41.4% for imposture of landfill tax by the government and 40.0% institution of laws against incineration. In cluster 7, “On-site waste management plan”, two factors were loaded, with awareness among clients and contractors scored 55.1% and waste expertise involvement on sites scored 44.9%. Table 2 shows the grading score of each of the variables.
While the normality test was used to evaluate if variables were regularly distributed or not. The normality test was conducted with 0.05 as the lowest value. For sample sizes < 50, statistical results were based on the “Kolmogorov–Smirnov” test, while results for sample sizes < 50 were based on the “Shapiro–Wilk” test. Because our sample size was greater than 50 in this study, the “Kolmogorov–Smirnov” was used. The p-value was < 0.05, according to the normality test, which makes it a suitable analysis. In each cluster, there are signs that some of the variables indicate a high performance of the waste management system, while others show a very low implementation rate. Table 3 shows the grading score for the normality test. The result indicates that the most common waste minimization strategy achieved is avoidance of complex design and detailing, material recovery maximization, and selection of materials that maximize the usable lifespan, while landfill site conservation was found to be the least of the waste minimization strategy in operation in South Africa. There is obviously a poor procurement mechanism, inadequate landfill site conservation, and lack of provision made for incentives in transactions on secondary materials.
In South Africa, there is a noteworthy advance in the implementation of the sustainable WMS. However, the governments and other building stakeholders must ensure that a sustainable WMS is in operation from the feasibility study through project completion to decrease waste to the lowest possible level. In this study, the model applied for the grading system can be validated, and the result of each metric used for the measurement is viable. In a nutshell, the construction industry of South Africa is yet to fully adopt and implement a sustainable waste management system for effective waste minimization, although the overall performance shows that the construction sector is thriving and improving in its approach to waste management. There is an imperative requirement to upscale and upgrade the current waste management system applied to minimizing waste in the construction industry of South Africa. At the moment, there is an increase in research on sustainametric application in sustainability performance measurement, but further sustainametric/and statistical mechanisms can be used to model a pattern for the optimization of the WMS in any construction sector.
Furthermore, the waste management system implemented in South Africa has the potential to be much more evident in terms of job creation and possibilities, cost savings, and resource conservation, especially when integrated into the process of recycling and reusing waste materials. In addition, despite government taxes and penalties for unlawful dumping, many municipalities in South Africa still dispose of their waste in landfills. Therefore, there is still room for improvement in the waste management sector operations, given the low compliance rate with the sustainable waste management policy and framework. If appropriately applied, the sustainability assessment approaches mentioned in this study can aid in understanding any waste management systems in place and determining their sustainability.
The raw data supporting the conclusions of this article will be made available by the authors, without undue reservation.
Written informed consent was obtained from the individual(s) for the publication of any potentially identifiable images or data included in this article.
AA pioneers the research focus and does the literature background study. CA and WT give advice, correction on the technicality, and significance of the study. All authors contributed to the article and approved the submitted version.
This research was fully funded by the National Research Foundation, South Africa (Grant No. UID214365).
The authors declare that the research was conducted in the absence of any commercial or financial relationships that could be construed as a potential conflict of interest.
All claims expressed in this article are solely those of the authors and do not necessarily represent those of their affiliated organizations, or those of the publisher, the editors and the reviewers. Any product that may be evaluated in this article, or claim that may be made by its manufacturer, is not guaranteed or endorsed by the publisher.
EPI, Environmental Performance Index; ESI, Environmental Sustainability Index; GGEI, Global Green Economy Index; SPM, sustainability performance measurement; SPI, sustainability performance indicator; TBL, triple bottom line; WMS, waste management system.
Abioye, A., and Rao, B. (2015). Sustainable approach to managing construction and demolition waste: an opportunity or a new challenge. J. Innov. Res. Sci. Eng. Tech. 4, 10368–10377. doi: 10.15680/IJIRSET.2015.0411007
Aboginije, A. J., Aigbavboa, C. O., and Thwala, W. (2021). A holistic assessment of construction and demolition waste management in Nigerian construction projects. Sust. J. Sust. 13, 6241. doi: 10.3390/su13116241
Aboginije, A. J., Aigbavboa, C. O., Thwala, W., and Samuel, S. (2020). “Determining the impact of construction and demolition waste reduction practices on green building projects in Gauteng province, South Africa,” in 10th Annual International Conference of Industrial Engineering and Operational Management, Dubai, United Arab Emirate, 2428–2437.
Akinade, O. O., Oyedele, L. O., and Ajayi, S. O. (2018). Designing out construction waste using BIM technology: Stakeholders' expectations for industry deployment. J. Clean. Prod. 180, 375–385. doi: 10.1016/j.jclepro.2018.01.022
Begum, R. A., Satari, S. K., and Pereira, J. J. (2010). Waste generation and recycling: comparison of conventional and industrialized building systems. Am. J. Environ. Sci. 6, 383–388. doi: 10.3844/ajessp.2010.383.388
Bell, S., and Morse, S. (2008). Sustainability Indicators: Measuring the Immeasurable? London: Earthscan.
Bojan, R., Neven, V., and Branka, I. (2017). Concept of sustainable waste management in the city of Zagreb: towards the implementation of the circular economy approach. J. Air Waste Manage. Assoc. 67, 241–259. doi: 10.1080/10962247.2016.1229700
Bundy, C. J. Thompson, L. M., Gordon, D. F., Vigne, R., Cobbing, R. D., and Hall, M. N. (2022). South Africa Encyclopedia Britannica Available online at: https://www.britannica.com/place/South-Africa (accessed 11 May 2022).
Dahl, A. L. (2012). Achievements and gaps in indicators for sustainability. Ecol. Indic. 17, 14–19. doi: 10.1016/j.ecolind.2011.04.032
Dalal-Clayton, B., and Sadler, B. (2009). Sustainability Appraisal. A Sourcebook and Reference Guide to International Experience. London: Earthscan publisher.
Daylath, P. M. (2011).Contractual obligations analysis for construction waste management. Master thesis submitted to the University of British Columbia, Vancouver, BC, Canada.
DEAT (2001). White paper on integrated pollution and waste management for South Africa. A policy on pollution prevention, waste minimization, impact management, and remediation. Available online at: https://www.dffe.gov.za/sites/default/files/legislations/integrated_pollutionand_wastemanagement_1.pdf (accessed February 12, 2022).
Dong, Y., and Hauschild, M. Z. (2017). Indicator for environmental sustainability. Procedia CIRP. 61, 697–702. doi: 10.1016/j.procir.2016.11.173
Ekanayake, L. L., and Ofori, G. (2004). Building waste assessment score: design-based tool. Build. Environ. 39, 851–861. doi: 10.1016/j.buildenv.2004.01.007
Ferro, C., Padin, C., Svensson, G., Sosa Varela, J. C., Wagner, B., and Høgevold, N. M. (2017). Validating a framework of stakeholders in connection to business sustainability effort in supply chains. J. Bus. Ind. Market. 32, 124–137. doi: 10.1108/JBIM-12-2015-0253
Garcia-de-Vinuesa, DL, Aguayo-Gonzalez, F, and Cordoba-Roldan, A. (2018). A proposal of a framework for the evaluation of the sustainability of products from the paradigm of the circular economy based on industry 4.0 (2nd part). DYNA 93, 488–492. doi: 10.6036/8718
Gasparatos, A., El-Haram, M., and Horner, M. (2008). A critical review of reductionist approaches for assessing the progress towards sustainability. Environ. Impact Assessment Rev. 28, 286–311. doi: 10.1016/j.eiar.2007.09.002
George, D., and Mallery, P. (2003). SPSS for Windows Step by Step: A Simple Guide and Reference. 11, 0. Update. Boston: Allyn and Bacon.
Ginindza, B., and Muzenda, E. (2013). “Community Perception on Waste Management and Minimization: a Case Study for Mogale City and Westonaria Municipalities” in Paper presented at the International Conference on Integrated Waste Management and Green Energy Engineering, April 15-16, Johannesburg, South Africa 2013.
Hammond, A., Adriaanse, A., Rodenburg, E., Bryant, D., and Woodward, R. (1995). Environmental Indicators: a Systematic Approach to Measuring and Reporting on Environmental Policy Performance in the ‘ Context of Sustainable Development). Washington, DC: World Resources Institute.
Hermann, B. G., Kroeze, C., and Jawjit, W. (2007). Assessing environmental performance by combining life cycle assessment, multi-criteria analysis, and environmental performance indicators. J. Clean. Prod. 15, 1878–1796. doi: 10.1016/j.jclepro.2006.04.004
Høgevold, N. M., and Svensson, G. (2012). A business sustainability model: a European case study. J. Bus. Indu. Market. 27, 142–151. doi: 10.1108/08858621211197001
Huang, B., Wang, X., Kua, H., Geng, Y., Bleischwitze, R., and Renf, J. (2018). Construction and demolition waste management in China through the 3R principle. Res. Conserv. Recycl. 129, 36–44. doi: 10.1016/j.resconrec.2017.09.029
Islam, M. D., Rahman, S. H., and Hassan, M. (2020). Municipal solid waste management using GIS application in Mirpur area of Dhaka city, Bangladesh. Pollution 2, 141–145. doi: 10.7508/pj.2016.02.004
Jingkuang, L., Yedan, L., and Xuetong, W. (2020). An environmental assessment model of construction and demolition waste based on system dynamics: a case study in Guangzhou. Environ. Sci. Pollut. Res. 27, 37237–37259. doi: 10.1007/s11356-019-07107-5
Jingkuang, L., Yixuan, C., and Xuetong, W. (2022). Factors driving waste sorting in construction projects in China. J. Clean. Prod. 336, 130397. doi: 10.1016/j.jclepro.2022.130397
Jingkuang, L., and Yue, T. (2022). Evolution game analysis on behavioral strategies of multiple stakeholders in construction waste resource industry chain. Environ. Sci. Pollut. Res. 12, 1–7. doi: 10.1007/s11356-022-23470-2
Kumar, A., Holuszko, M., and Espinosa, D. C. R. (2017). E-waste: An overview on generation, collection, legislation and recycling practices. Resour. Conserv. Recycl. 122, 32–42. doi: 10.1016/j.resconrec.2017.01.018
Lele, S., and Norgaard, R. B. (1996). Sustainability and the scientist's burden. Conserv. Biol. 10, 354–365. Available online at: http://www.jstor.org/stable/2386852
Liu, J., Li, Q., Gu, W., and Wang, C. (2019). The impact of consumption patterns on the generation of municipal solid waste in China: evidences from provincial data. Int. J. Environ. Res. Publ. Health. 16, 1–19.
Lozano, R. (2006). A tool for graphical assessment of sustainability in universities. J. Clean. Prod. 14, 963–972. doi: 10.1016/j.jclepro.2005.11.041
Mayer, A. L., Thurston, H. W., and Pawlowski, C. W. (2004). The multi-disciplinary influence of common sustainability indices. Ecol. Environ. 2, 419–426. doi: 10.1890/1540-9295(2004)002<0419:TMIOCS>2.0.CO;2
Milne, M., and Gray, R. (2013). W(h)ither ecology? The triple bottom line, the global reporting initiative, and corporate sustainability reporting. J. Bus. Ethics 118, 13–29. doi: 10.1007/s10551-012-1543-8
Moldan, B., Janoušková, S, and Hák, T. (2012). How to understand and measure environmental sustainability: indicators and targets. Ecol. Ind. 17, 4–13. doi: 10.1016/j.ecolind.2011.04.033
Montabon, F., Pagell, M., and Wu, Z. (2016). Making sustainability sustainable. J. Supp. Chain Manage. 52, 11–27. doi: 10.1111/jscm.12103
Muzenda, E., Ntuli, F., and Pilusa, T. J. (2012). “Waste management strategies and the situation in South Africa,” in International Conference on Chemical Engineering and Technology, Oslo, Norway, 13–14 August 2012, 309–312.
Nagapan, I. S., Abul-Rahman, I. A., and Aziz, A. A. (2012). Construction waste management: Malaysian perspective. Int. Conf. Environ. Eng. Sust. 2012, 299–309.
Nardo, M., Saisana, M., Saltelli, A., Tarantola, S., Hoffman, A., and Giovannini, E. (2005). Handbook on constructing composite indicators: Methodology and user guide (STD/DOC (2005)3). Paris: OECD Statistics Directorate. Available online at: http://www.oecd.org/dataoecd/37/42/42495745.pdf (accessed February 26, 2022).
Peters, Y. (2014). The alpha and the omega of scale reliability and validity: Why and how to abandon Cronbach's alpha and the route towards more comprehensive assessment of scale quality. Eur. Health Psychol. 16, 2.
Pontius, J., and Mclntosh, A. (2020). Sustainability Science. Critical Skills for environmental professionals. Springer Textbooks in Earth Sciences, Geography, and Environment. Cham: Springer.
Rahim, M. A., and Kasim, N. (2017). Conceptual Model for Systematic Construction Waste Management. MATEC Web of Conference. Paris: EDP Sciences.
Sahely, H. R., Kennedy, C. A., and Adams, B. J. (2005). Developing sustainability criteria for urban infrastructure systems. Can. J. Civil Eng. 32, 72–85. doi: 10.1139/l04-072
Scerri, A., and James, P. (2010). Accounting for sustainability: Combining qualitative and quantitative research in developing 'indicators' of sustainability. Int. J. Soc. Res. Methodol. 13, 41–53. doi: 10.1080/13645570902864145
Shen, L. Y., Tam, V, W., Tam, C. M., and Drew, D. (2004). Mapping approach for examining waste management on construction sites. J. Const. Engg. Manage, 130, 472–481.
Singh, R. K., Murty, H. R., Gupta, S. K., and Dikshit, A. K. (2012). An overview of sustainability assessment methodologies. Ecol. Indic. 15, 281–299. doi: 10.1016/j.ecolind.2011.01.007
Sridhar, K. (2012). Corporate conceptions of triple bottom line reporting: an empirical analysis into the signs and symbols driving this fashionable framework. Soc. Res. J. 8, 312–326. doi: 10.1108/17471111211247901
Statista. (2022). Projection of waste generation worldwide in 2016, 2030, and 2050, by region. Available online at: https://www.statista.com/statistics/233613/waste-generation-worldwide-by-region/
Tasdemir, C., Gazo, R., and Quesada, H. J. (2020). Sustainability benchmarking tool (SBT): theoretical and conceptual model proposition of a composite framework. Environ. Dev. Sustain. 22, 6755–6797. doi: 10.1007/s10668-019-00512-3
Troschinetz, A. M., Mihelcic, J. R., and Bradof, K. L. (2007). Developing sustainability indicators for a university campus. Int. J. Eng. Educ. 23, 231–241.
Udawatta, N., Zuo, J., Chiveralls, K., and Zillante, G. (2015). Improving waste management in construction projects: an Australian study. Res. Conserv. Recycl. 101, 73–83. doi: 10.1016/j.resconrec.2015.05.003
United Nations Environment Programme (2017). Guidelines for national waste management strategies: Moving from challenges to opportunities. Available online at: https://www.unep.org/about-un-environment (accessed February 12, 2022).
Velenturf, A., Jopson, P. M., and Juliet, S. (2019). Making the business case for resource recovery. Sci. Total Environ. 648, 1031–1041. doi: 10.1016/j.scitotenv.2018.08.224
Wu, Z., Shen, L., Yu, A. T. W., and Zhang, X. (2016). A comparative analysis of waste management requirements between five green building rating systems for new residential buildings. J. Clean. Prod. 112, 895–902. doi: 10.1016/j.jclepro.2015.05.073
Xiao, S., Dong, H., Geng, Y., and Brander, M. (2018). An overview of China's recyclable waste recycling and recommendations for integrated solutions. Res. Conserv. Recycl. 134, 112–120. doi: 10.1016/j.resconrec.2018.02.032
Yuan, H. (2017). Barriers and countermeasures for managing construction and demolition waste: a case of Shenzhen in China. J. Clean. Prod. 157, 84–93. doi: 10.1016/j.jclepro.2017.04.137
Keywords: building materials, construction and demolition waste, life-cycle assessment, sustainametric technique, sustainable development
Citation: Aboginije A, Aigbavboa C and Thwala W (2023) Modeling and usage of a sustainametric technique for measuring the life-cycle performance of a waste management system: A case study of South Africa. Front. Sustain. 3:943635. doi: 10.3389/frsus.2022.943635
Received: 13 May 2022; Accepted: 16 November 2022;
Published: 09 January 2023.
Edited by:
Alexander Ryota Keeley, Kyushu University, JapanCopyright © 2023 Aboginije, Aigbavboa and Thwala. This is an open-access article distributed under the terms of the Creative Commons Attribution License (CC BY). The use, distribution or reproduction in other forums is permitted, provided the original author(s) and the copyright owner(s) are credited and that the original publication in this journal is cited, in accordance with accepted academic practice. No use, distribution or reproduction is permitted which does not comply with these terms.
*Correspondence: Ademilade Aboginije, YWJvZ2luaWplYWRlbWlsYWRlQGdtYWlsLmNvbQ==
Disclaimer: All claims expressed in this article are solely those of the authors and do not necessarily represent those of their affiliated organizations, or those of the publisher, the editors and the reviewers. Any product that may be evaluated in this article or claim that may be made by its manufacturer is not guaranteed or endorsed by the publisher.
Research integrity at Frontiers
Learn more about the work of our research integrity team to safeguard the quality of each article we publish.