- 1UCL Plastic Waste Innovation Hub, University College London, London, United Kingdom
- 2UCL Centre for Behavior Change, University College London, London, United Kingdom
Compostable and biodegradable plastics are growing in popularity but their environmental credentials need to be more fully assessed to determine how they can be a part of the solution to the plastic waste crisis. We present results and analysis on home compostable packaging. This type of packaging requires the citizen to be able to correctly identify the packaging as “home compostable,” to have composting facilities at home, and to successfully compost the plastic. Using a citizen science approach, we engaged with 9,701 UK citizens geographically spread across the UK to examine their capability, opportunity, and motivation to do this. Of this cohort 1,648 citizens performed home compost experiments to test the environmental performance of compostable plastics. We report on the type of plastics they tested and their disintegration under real home composting conditions. The results show that the public are confused about the meaning of the labels of compostable and biodegradable plastics. 14% of sampled plastic packaging items tested were certified “industrial compostable” only and 46% had no compostable certification. Of the biodegradable and compostable plastics tested under different home composting conditions, the majority did not fully disintegrate, including 60% of those that were certified “home compostable.” We conclude that for both of these reasons, home composting is not an effective or environmentally beneficial waste processing method for biodegradable or compostable packaging in the UK.
Introduction
In response to the plastic waste crisis countries all round the world have set targets to make plastic packaging 100% recyclable, reusable, or compostable, and to eliminate all unnecessary single-use packaging by 2025 (WRAP, 2018). Including compostable plastics in the targets was important for two reasons: firstly, there are some items such as food packaging, wipes, tea bags, sachets, that being small and highly food contaminated are not suited to recycling or reuse; secondly, food waste is of major environmental importance and compostable liners can play an important role in the route out of the home. But there are some fundamental problems. Although the processing of compostable and biodegradable plastics is regulated under industrial organic waste management processes, the existence of systems of collection and industrial composting for this packaging are rare in the UK. Compostable and biodegradable plastics are currently incompatible with most anaerobic digestion (AD) systems and recycling systems (WRAP, 2020). Hence their fate is either landfill or incineration. Disposal of compostable packaging in landfill is not environmentally beneficial, however under certain instances where incineration processes use energy from waste recovery incinerating compostable packaging may offer some benefit in overall emissions reductions (WRAP, 2020). The typical fate of landfill or incineration is not usually communicated to customers so the environmental claims made for compostable and biodegradable packaging can be misleading (WRAP, 2020).
The current bioplastics market share is relatively small at 1% of the total 335 million tons of plastics produced globally, the global production capacity for biodegradable plastics is set to increase from around 0.91 million tons in 2018 to around 1.3 million tons in 2023 (European Bioplastics, 2018) but it could be much greater if small item formats such as snack packets and chocolate wrappers (Ricardo Energy Environment, 2019) are switched to compostable plastics. Manufacturers of compostable plastics and the companies using them are aware of the lack of infrastructure for sorting and collecting these plastics but counter them through a number of arguments. Firstly, that the public are in favor of biodegradable packaging; secondly the organic waste collection initiatives in the EU's Waste Framework Directive and UK Government's Environment Bill will increase capacity of the organic waste processing systems and closed loop systems that can handle these plastics, and thirdly by designing biodegradable packaging designed to compost at home. In this research we used a citizen science approach to test these arguments by: (1) assessing the public's attitudes and understanding of compostable and biodegradable materials; and (2) inviting citizens to carry out experiments to test the effectiveness of home composting as a means to biodegrade compostable plastics.
Composting processes
Understanding product performance and user behavior is vital to the success of circular economy models of material and product use (Wastling et al., 2018). Several studies have been carried out in the field of user behavior in relation to biobased and biodegradable packaging (WRAP, 2007; Taufik et al., 2020), home composting practices (Brook Lyndhurst Ltd, 2009; Garden Organic, 2017) and the performance of compostable plastics under home composting conditions (Scion, 2020; WasteMINZ, 2020). To our knowledge this is the first citizen science study combining large-scale collection of data on home compostable plastic use and behavior, and disintegration performance of home compostable plastics in the UK.
Biodegradability refers to the capability of being degraded by biological activity (Kjeldsen et al., 2019). Many materials are biodegradable such as paper, cardboard, wood, and certain types of plastic. The word biodegradable does not describe under what conditions and how long a plastic will take to biodegrade. The term “compostable plastic” is more specific, it describes a material that is capable of undergoing biological degradation in a compost site at a rate consistent with other known compostable materials, leaving no visibly distinguishable or toxic residues (Vert et al., 2012).
There are two types of composting environment for which compostable plastics are designed, industrial composting and home composting. Industrial composting is a controlled biotechnological process for transforming biodegradable organic waste into compost, a resource used in agriculture to improve soil (Ruggero et al., 2019). Depending on the process, industrial composting facilities are designed to undertake aerobic composting or anaerobic digestion (biogasification). In aerobic composting, microorganisms consume oxygen while breaking down organic waste to produce CO2, water, compost, and heat. In anaerobic digestion, bacteria degrade the organic waste in the absence of oxygen, producing biogas (methane and CO2) and digestate (Bátori et al., 2018). The two different processes are performed in different facilities. Industrial composting (IC) facilities digest garden and green waste under aerobic methods, whilst anaerobic digestors (AD) normally deal with food waste (WRAP, 2016a). Typically AD facilities are not optimized to take compostable plastics which are generally removed even at low volumes (Rujnić-Sokele and Pilipović, 2017).
Home composting is a general term for the process by which biodegradable garden waste or domestic food waste is collected and placed in either a container or heap to allow natural processes to turn it into compost. It is a manual process whereby the composition and process temperatures remain largely unregulated. Both aerobic and anaerobic conditions can occur in home composting, although aerobic conditions are more normal. The time frame for home composting depends on personal preference and the use to which the compost is put, but 3–12 months is typical. The process of microbial biodegradation in soil can vary according to location and season due to variation in uncontrolled parameters influenced by geographical location such as temperature, water content, chemical composition and pH (Siracusa, 2019). Little is known about the variation of microbial biodegradation processes in home compost environments.
Industrial composting has a different legal status to home composting. Under Directive 2008/98/EC (2008) and UK Government's Compost Quality Protocol organic waste segregated at source can obtain legal “end-of-waste” status if the resulting compost complies with specific conditions such as those carried out under authorized industrial composting systems and is considered to be a recycling process. Home composting activities do not comply with these conditions therefore organic waste and compostable packaging processed in this way cannot achieve “end-of-waste” status and is not considered to be a recycling process. However, home composting is encouraged by Article 22 (2b) of Directive 2008/98/EC as it can help minimize the volume of organic waste entering the waste management system. Compostable packaging which complies with relevant European standards (EN 13432 or BS EN 13432 in the UK) or equivalent national standards for packaging recoverable through composting and biodegradation, can be collected separately and processed together with bio-waste under Article 22 of Directive 2008/98/EC.
Compostable plastics standards
The European Directive 94/62/EC (Packaging and Packaging Waste) 1994 sets out the EU's rules on managing packaging and packaging waste, including the recovery of biodegradable packaging waste with bio-waste (i.e., food or garden waste) by means of organic recycling (e.g., composting). The Packaging (Essential Requirements) Regulations 1998 implements these legal principles in the UK. Biodegradation testing standards (ISO and ASTM) have been designed to determine the biodegradability of plastics in soil, compost, landfill, marine, or other aquatic environments (Funabashi et al., 2009; Siracusa, 2019). EN 13432:2000 (or BS EN 13432:2000 in the UK) is a harmonized EU standard for compostable and biodegradable packaging that defines the criteria that must be met for a material to be suitable for commercial industrial composting (European Bioplastics, 2016). Similar requirements for non-packaging plastic items are specified by the European standard EN 14995. Under EN 13432:2000 (1) Test material (packaging and organic waste) has to show disintegration and loss of visibility in the final compost; (2) after 3 months, no more than 10% of the initial weight of the test material should be retained after sieving it through 2 mm mesh size; (3) Within a maximum of 6 months, 90% of the carbon in the test material must be converted to CO2, having the same rate of biodegradation as natural materials; (4) The test material must have no negative effects on the composting process and no adverse effect on the quality of the compost produced, including the heavy metals content.
Although there is currently no harmonized international or European standard for compostable or biodegradable plastics suitable for home composting, the following national regulations, standards, and certifications exist: UNI 11183 (Italy), AS 5810 (Australia), NT T 51–800 (France), and OK Compost (Belgium) (Association for Organics Recycling, 2011). In the UK, the Publicly Available Specifications PAS100 and PAS110 provide a baseline quality and safety specification for compost and digestate respectively (British Standards Institution, 2018).
Organic waste management and composting in the UK is regulated and enforced by the Environment Agency (England and Wales) and the Scottish Environment Protection Agency and Northern Ireland Environment Agency under the Environmental Permitting Regulations and Pollution Prevention Control Regulations. These Regulations allow certain activities to be carried out under restrictions imposed through permits and registered exemptions to protect the environment and human health. The T23 exemption allows composting of small volumes (up to 80 tons) of vegetation, cardboard and food waste under aerobic composting conditions for use a soil improver or fertilizer undertaken in small scale scenarios such as schools, allotment associations and community composting groups.
Compostable plastic types and manufacture
Main applications of compostable plastics today include food packaging films, protective transportation films, bags, cups, plates and cutlery, biowaste bags, and agricultural films and mulches (Siracusa et al., 2008; Narancic et al., 2018). Compostable plastics are manufactured using either fossil-based or bio-based feedstocks. Commonly marketed bio-based compostable plastics include polylactic acid (PLA), polyhydroxyalkanoate (PHA), with the most prevalent being polyhydroxybutyrate (PHB), and polybutylenesuccinate (PBS), starch blends, and cellulose blends. Common fossil-based compostable plastics include polybutylenadipatterephthalat (PBAT) and polycaprolactone (PCL) (WRAP, 2020).
Key performance criteria for compostable plastic packaging relate to desired characteristics of the material (such as flexibility, strength, transparency, barrier properties) and the ability to biodegrade under different conditions of industrial composting or anaerobic digestion, and/or home composting. Biodegradability is not only a functional requirement but also an important environmental attribute (Siracusa et al., 2008). Antioxidants and stabilizers are added to protect polymers against mechano-oxidation during the processing operation and to provide the required shelf-life, and to improve performance.
Biodegradation timescales
Timescale of biodegradation is a key consideration for compostable plastic use. For example, the majority of biodegradable plastics can technically degrade under AD conditions, however their degradation time is three to six times longer than the retention time in industrial AD plants (Narancic et al., 2018), exceeding commercial processing timeframes. Compostable plastic degradation time must be compatible with composting maturation timeframes and cycles of compost use in order to prevent the spread of plastic pollution in the environment (Siracusa, 2019). Home composting certification scheme disintegration test timescales allow for longer periods of composting (up to 12 months) whereas industrial composting certificates are typically shorter.
Citizen science
We used a citizen science method to collect data on attitudes to products packaged with compostable or biodegradable plastics, citizens' knowledge of how to correctly dispose of the packaging, and the effectiveness of home compostable plastics to biodegrade in a home compost. Our study, The Big Compost Experiment, was designed with citizen science principles at its core (European Citizen Science Association, 2015). The format consists of a publicly accessible website (www.bigcompostexperiment.org.uk) containing a 5-min online survey, and an optional home composting experiment facilitated through an online personal login facility. The website also contains additional educational information and links on biodegradable plastics and composting, and a social media blog, to engage the public in the science of biodegradable and compostable plastics.
Materials and methods
Before participating, citizens are directed to website information relevant to consent: the project aims, data protection, image guidelines, health, and safety advice, where to direct questions or complaints. Ethics Approval for the study was granted by the UCL Research Ethics Committee (Project ID/Title: 16747/001: Big Compost Experiment) until 07 November 2022.
Method part 1—Survey
The survey begins with illustrated questions enquiring about opinions and behaviors surrounding biodegradable plastics and food waste (see Figure 1). At this point a participant has the option to end participation in the survey and to submit their responses anonymously. If a participant chooses to continue, they are provided with further illustrated questions enquiring about the type of composter they use and their composting habits. At the end of the survey, a participant is given the option to end participation and to submit their responses anonymously. At this point a participant is also given the option to take part in a home composting experiment.
Method part 2—Home composting experiment
Participants are offered the option of taking part in a home composting experiment by setting up a user login account with which to share their experiment setup and to record their results (see Figure 2). The account setup asks for their contact email, information about their composter type and postcode location (first three letters of their post-code, giving only general information about which area they compost in) with the option to display this on a map of the UK (see Figure 3E), the method of composting and the usual time taken to make compost in their composter and what organisms live in their composter. They are asked to select and log a range of biodegradable plastic items they wish to test, and the rate at which the item degraded in their composter. Participants can select the type and quantity of biodegradable plastic items from an illustrated list that they would like to test, such as “cutlery,” “cups,” “shopping bags, and “newspaper wraps” (see Figure 2). Participants are advised to only test items that display the following manufacturer information:
“compostable” (only)
“home biodegradable”
“home compostable”
“suitable for home composting”
TUV OK Compost “Home” certification mark
Din Certco “Home Compostable” certification mark
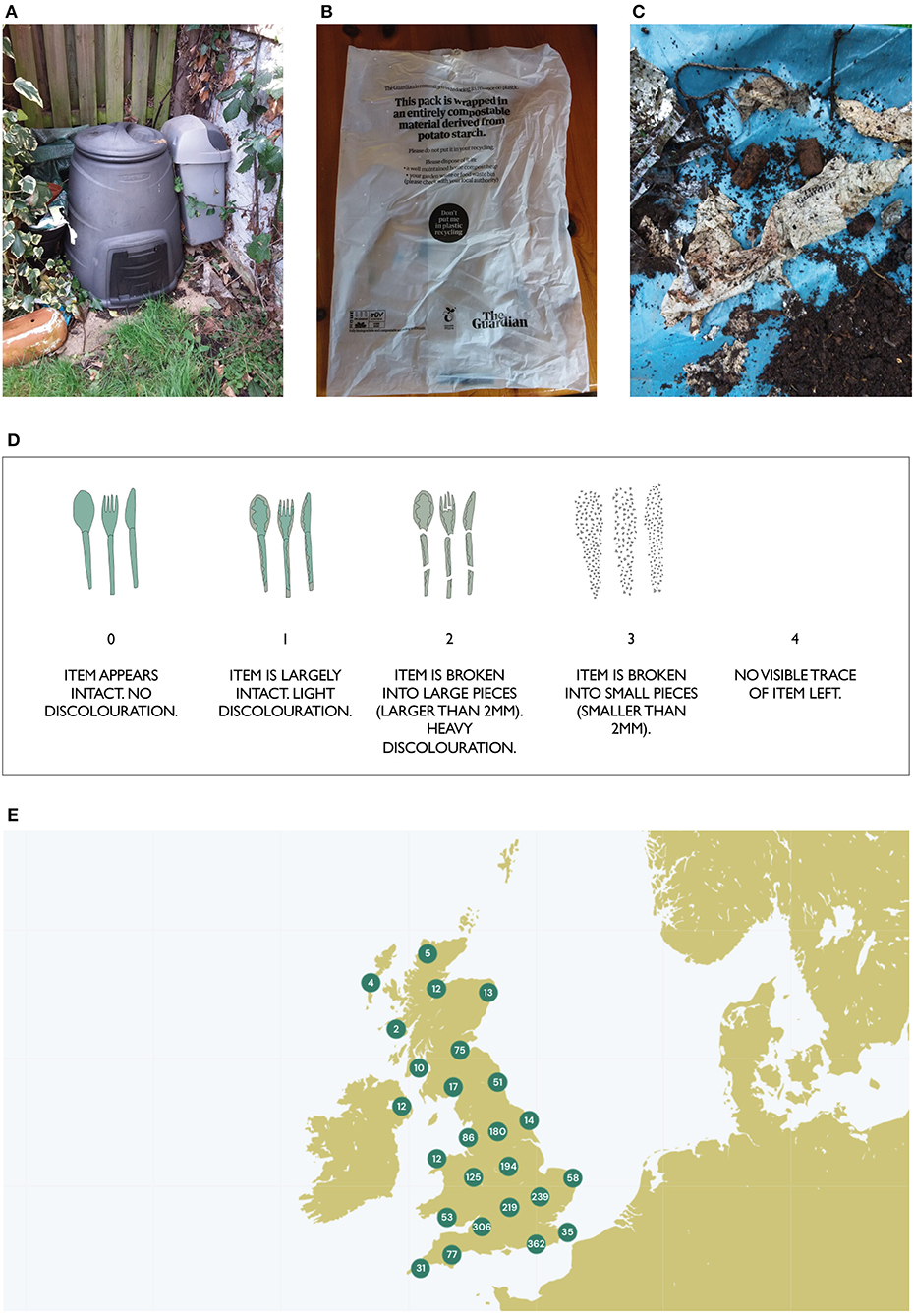
Figure 3. Panel showing home compost experiment participant photo uploads showing composter type used (A), item tested (B) and result (C), scale of degradation used for results comparison (D) and UK home compost experiment participation map (E).
Participants can then submit details about their selected items and the length of time they wish to run their experiment for, based on how long it usually takes them to make compost. Participants are asked to put test items into a loosely woven non-biodegradable net bag (i.e., a polypropylene net bag for supermarket fruit) before adding them into their composter to help locate items at the end of the experiment. Participants are also given the option to submit photographs of their home composter and/or items with the option to display them on the public website gallery (see Figures 3A,B). The Big Compost Experiment website automatically logs this information to a database and is used to setup an automated email reminder for participants to report their results at the end of their experiment.
Method part 3—Reporting
Participants are sent an email once their experiment is complete with a request to search for traces of their items in their composter. Experiment guidelines recommend using a household spade/trowel and sieve to look for the items in their compost and are advised that under 18s must be accompanied by a responsible adult. Participants are advised to collect any traces of their items they can find (if there are any), compare them with a “Degradation Scale” (see Figure 3D), and record any other useful observations about their items via their user login account. They also have the option to upload images of their item results (see Figure 3C). Once a participant has completed their experiment and reported their results, disposal of items in general waste collection is advised.
Results
The data presented and analyzed were collected over 24 months from 7th November 2019 to 7th November 2021, during that time 9,701 participants from across the UK completed the attitudes survey, 1,648 of which engaged actively in a home composting experiment, and 902 completed the experiment.
The geographical distribution of these participants is shown in Figure 3E showing good coverage across the UK, with the highest proportion in the Midlands and the South of England. This roughly correlates with the distribution of population density in the UK. The 91% of these participants indicated that they separate their food waste, this is a higher than the UK average (WRAP, 2016b) and is an indicator that this sample is not representative of the general population. In answer to the question “Are you more likely to buy products with packaging marked “compostable” or “biodegradable”?” 85% answered “yes,” 7% answered “no” and 8% answered “don't know.” The high proportion of “yes” is another indication that we have biased sample. It is likely that the people attracted to take part were those who are already interested in composting, evidence for this is that 84% of our participants use home composting which compares with a UK national average of 34% (DEFRA, 2009). When participants were asked which food waste strategy they use to dispose of biodegradable plastics, if any, 16% answered “using council organic waste collection,” 42% answered “using home composting,” and 4% answered “using another organic waste strategy”.
The participants use a wide variety of composters ranging from indoor wormery to outdoor trenches, with the most popular (64%) being an outdoor closed-bin composter (see Figure 4 for the full distribution). When asked what they do with their compost 83% replied that they used it to enrich their soil to grow edible plants, fruit and vegetables (see Figure 5). This is important as it indicates that the food chain, albeit a home-grown one, is affected by whatever substances are put in home compost.
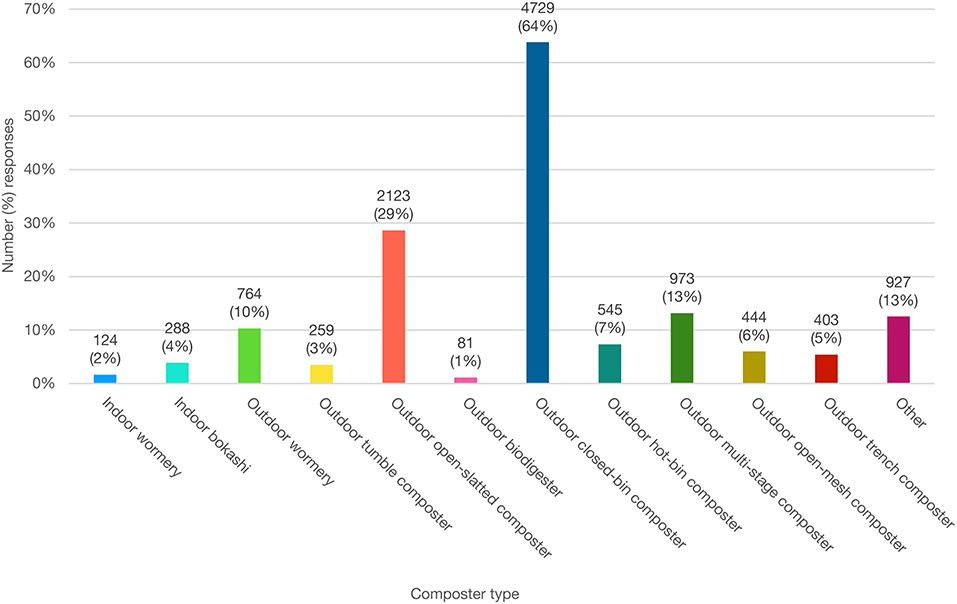
Figure 4. Chart showing the frequency and types of composter used by survey participants. Values calculated from 7,413 participants who home compost. Multiple responses included.
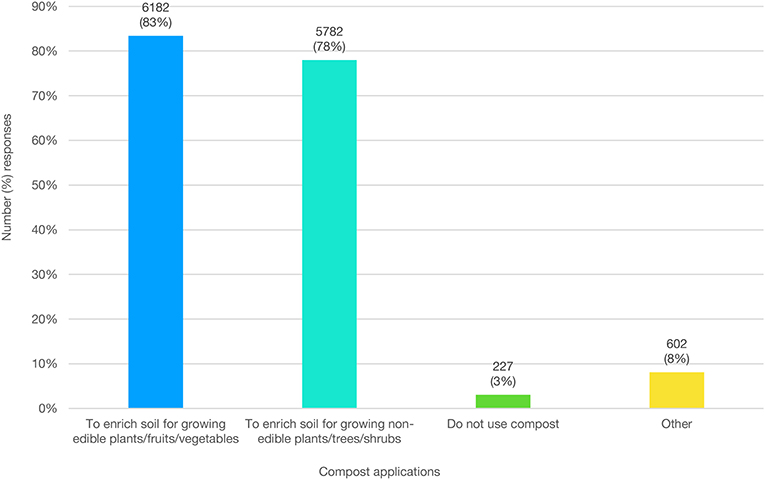
Figure 5. Chart showing the range of compost applications used by survey participants. Values calculated from 7,413 participants who home compost. Multiple responses included.
The importance of home composting not just as a means to enrich soil but also as an important site of biodiversity is confirmed in Figure 6 which shows that 14 categories of organism are visible to the naked eye in the home composters, from worms, to mites, to fungi. It is this ecosystem of organisms that is responsible for biodegrading items put in the composter, including the range of compostable plastics tested in this experiment.
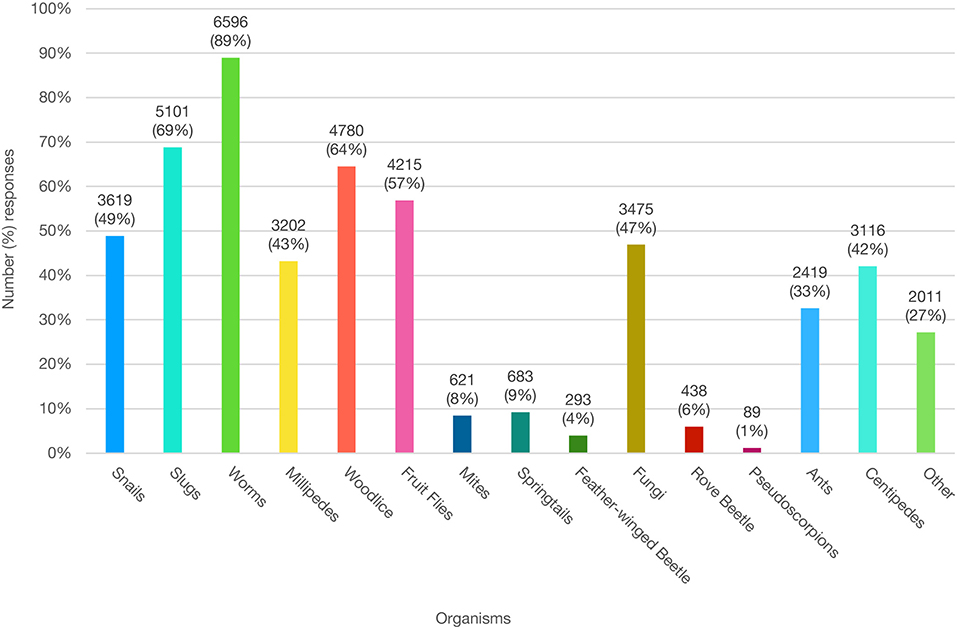
Figure 6. Chart showing the range of organisms observed in participant composters. Values calculated from 7,413 participants who home compost. Multiple responses included.
The participants 1,648 engaged, many of whom recorded what they put into their home composter by uploading a photo of that item to the Big Compost Experiment website (see Figures 3A–C) for a sample of these images). Despite our best efforts to guide the participants only to compost items marked clearly as “home compostable,” many items that are marked as industrially compostable or just as biodegradable have been entered into the experiment. Out of a randomized sample of 50 item images analyzed 46% show no identifiable certification or standards labeling. The 14% show industrial composting certification only (see Figure 7), for full results of labeling identified. This suggests participant confusion surrounding labeling and identification of home compostable plastics.
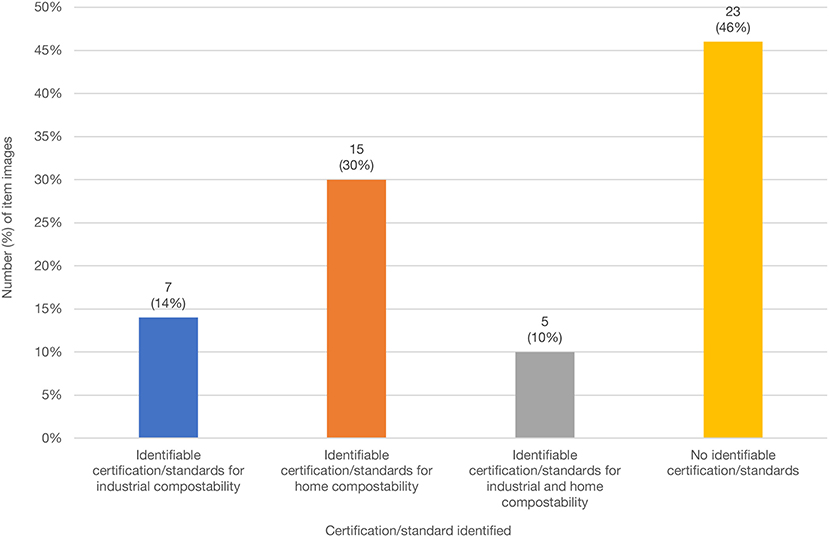
Figure 7. Chart showing the frequency of tested items displaying identifiable certification or standards for compostability. Values calculated from a randomized sample of 50 item images submitted.
Of the 1,648 who undertook a home composting experiment, 902 had finished home compost experiments when we compiled these results, with a total of 1,307 item results submitted. Of these 55% of home compost experiment results report the item remains still clearly visible (Level 0–2), 11% of results contain small pieces (<2 mm but still visible to participant) and 34% no longer visible / not found (Level 4) within participant home composting timeframes. Figure 8 shows the distribution.
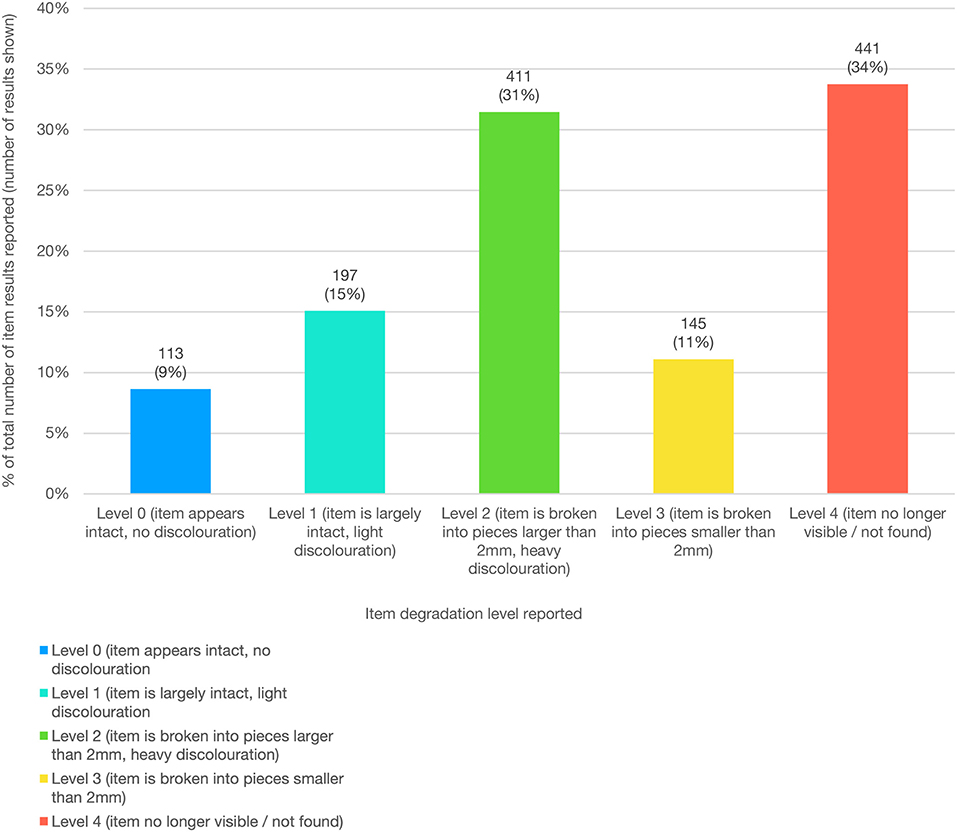
Figure 8. Chart showing home compost experiment item degradation level results. Values calculated from 1,307 item results submitted.
The highest number of results was reported for “outdoor closed-bin composter” category. The 59% of these results report item remains still clearly visible (Level 0–2), 11% of results contain small pieces (<2 mm but still visible to participant), 30% of results report item no longer visible / not found within participant home composting timeframes. Similar distribution of results is seen in three other most frequently used composters “outdoor open-slatted,” “multi-stage,” and “hot-bin” (see Figure 9).
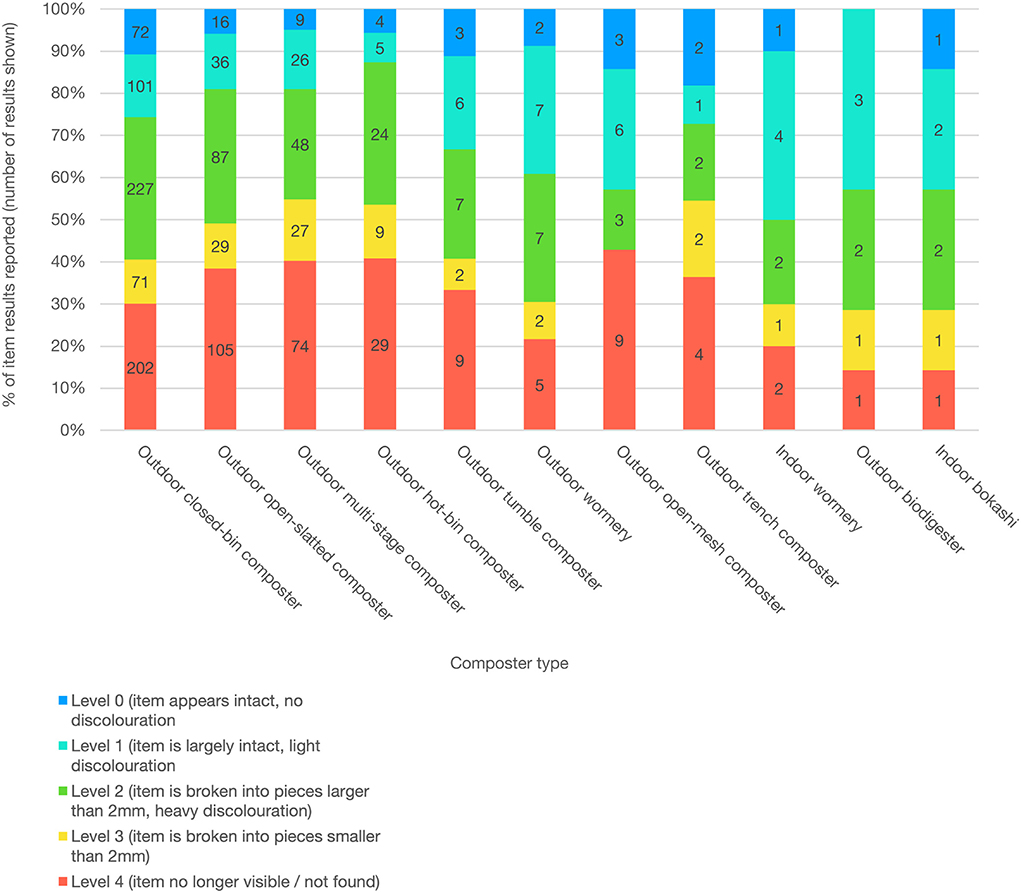
Figure 9. Chart showing home compost experiment item degradation level results according to composter type. Values calculated from 1,307 item results submitted.
The highest number of results was reported for “newspaper and magazine wraps” category. The 58% of these results report items that remain still clearly visible (Level 0–2), 12% of results contain small pieces (<2 mm but still visible to participant), 30% of results report item no longer visible / not found within participant home composting timeframes. Similar distribution of results is seen for frequently reported item categories “caddy and bin bags,” “shopping bags,” “fruit and vegetable films”. See Figure 10 for full distribution.
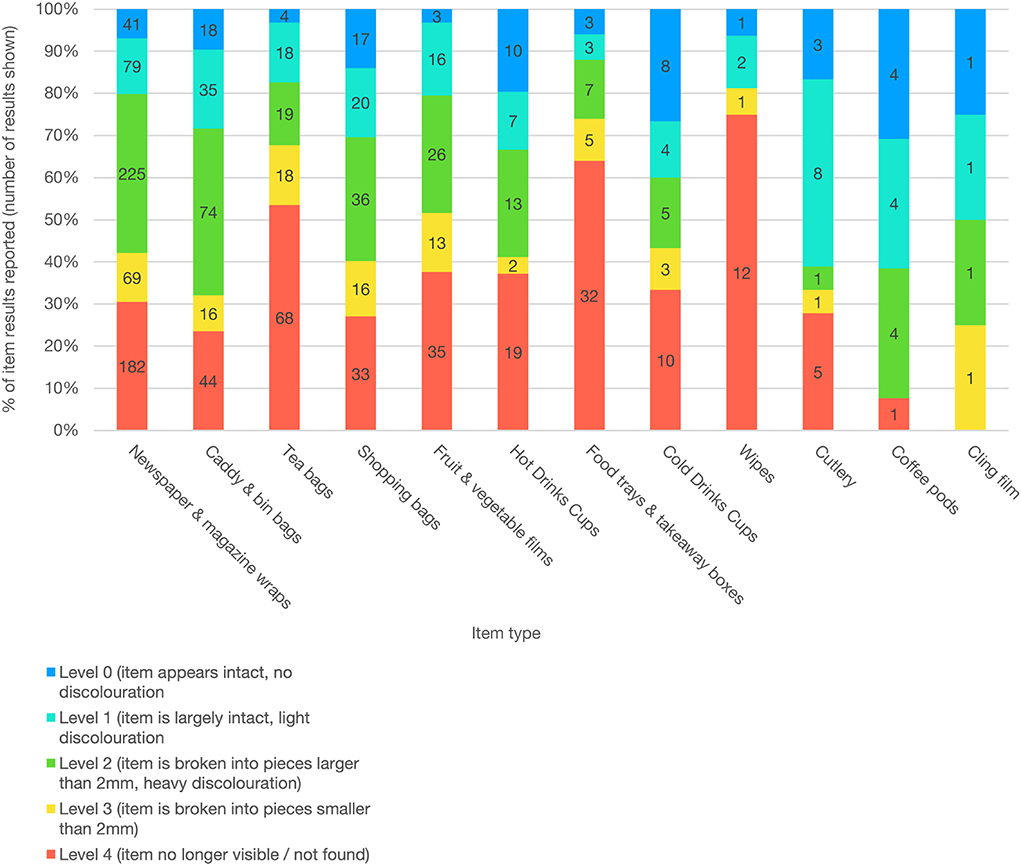
Figure 10. Chart showing home compost experiment item degradation level results according to item type. Values calculated from 1,307 item results submitted.
Nationally, England and Scotland show similar results despite their different climates (further breakdown per region does not show much difference either); Wales shows improved composting effectiveness overall; and there is very little data for Northern Ireland (see Figure 11).
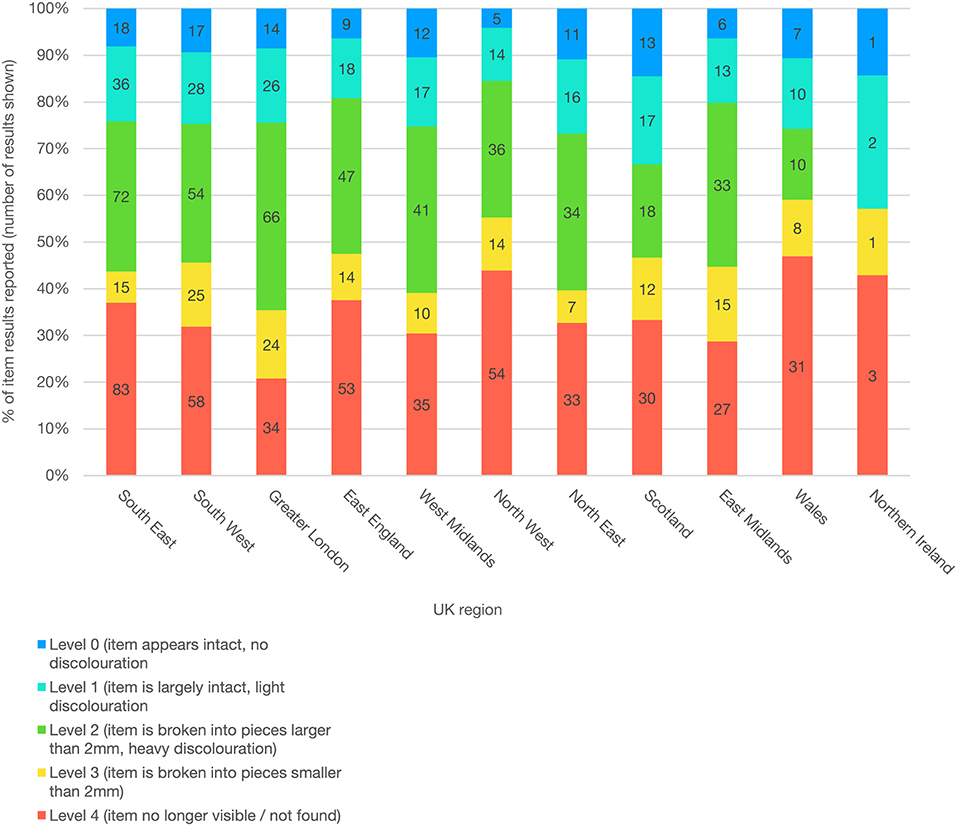
Figure 11. Chart showing home compost experiment item degradation level according to UK region. Values calculated from 1,307 item results submitted.
The composting time periods (3/6/9/12/15/18/21 months) do not seem to greatly affect the results, indicating perhaps that it's the health and dynamics of an individual's composter that is more important. In general, longer composting times does help. For instance, 71% of experiments using 3 month composting duration report item remains still visible (Level 0–3); 65% of experiment using 6 month composting duration report item remains still visible (Level 0–3); 63% of 9 month composting duration report item remains still visible (Level 0–3); 68% of 12 month composting duration report item remains still visible (Level 0–3); 63% of 15 months composting duration report item remains still visible (Level 0–3) (see Figure 12).
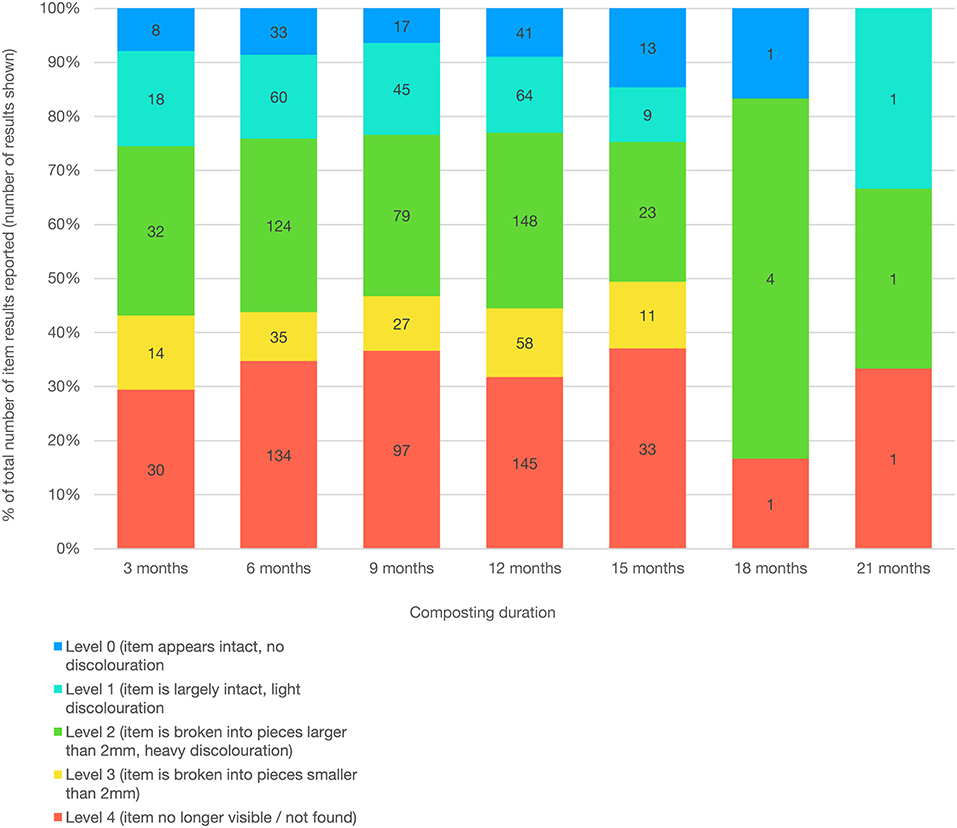
Figure 12. Chart showing home compost experiment item degradation level according to composting duration. Values calculated from 1,307 item results submitted.
A range of certification marks relating to home compostability and industrial compostability were identified on home compost experiment items tested. The most common certification mark displayed was TUV Ok Compost Home (47%), followed by European Bioplastics Seedling Logo (26%), and TUV OK Compost Industrial (2%). The 46% of items tested could not be verified to have any compostable certification. Figure 13 shows this data. This is evidence that many of the participants were confused about the labeling and what it means. Column 1 in Figure 13 shows the results for items displaying TUV OK Compost Home, 60% of which were reported to have item remains still clearly visible (Level 0–2), 12% of results contain small pieces (<2 mm but still visible to participant), 28% of results report item no longer visible / not found within participant home composting timeframes. The results are similar for European Bioplastics Seedling Logo (column 3 in Figure 13). This shows that the majority of certified home compostable plastic packaging tested in this study did not fully compost.
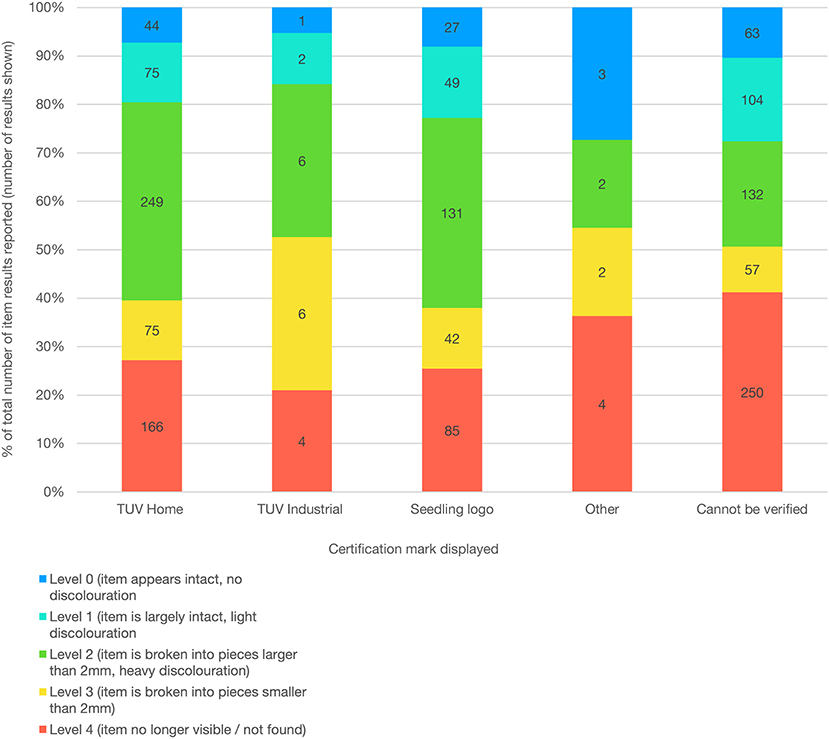
Figure 13. Chart showing home compost experiment item degradation level according to displayed compost certification. Values calculated from 1,307 item results submitted. Items displaying multiple certification marks included.
Discussion
The idea that a material can be sustainable is a widespread misconception. Only a system of production, collection, and reprocessing of a material can be sustainable. The type and amount of energy used to fuel the process, the water usage, and the by-products also contribute to its environmental footprint. This applies to compostable plastics as much as to normal plastics. Although the bio-sources of compostable plastics make this class of material more renewable, the fact that there is no UK-wide system of collection is problematic. Most compostable plastics end up in landfill or are burnt. Neither is a good environmental outcome. Some people put compostable plastics in their food waste collection, but this is generally treated as a contaminant and increases the costs of current anaerobic digester systems. Anaerobic digesters are not optimized to degrade biodegradable plastics, which are instead removed and sent to landfill or incinerated (Rujnić-Sokele and Pilipović, 2017). Several studies suggest the persistence of compostable and biodegradable plastics under simulated compost, sea, or soil environment conditions (Bagheri et al., 2017; Lambert and Wagner, 2017). Further field study evidence is needed to conclude the real-life performance of these plastics in the environment. Our evidence reported in this paper shows that citizens don't understand these complexities of compostable or biodegradable packaging and yet are enthusiastic about buying them (85% reported doing so). Our data also shows that citizens are confused about what the labels on compostable and biodegradable packaging mean. For example 60% of sampled items tested in home composting experiments were not certified home compostable (see Figure 7).
To understand the behavior of the public buying compostable plastics but not understanding where to dispose of them or how to interpret their environmental credentials, we thematically analyzed our survey data using social science analysis methods (Allison et al., 2021). The themes of behavior were categorized as barriers and enablers according to the components of the Capability, Opportunity, Motivation, and Behavior (COM-B) model of behavior.
We found that the key enablers that lead people to buying products with biodegradable or compostable plastic packaging are reflective motivation (positive beliefs about biodegradable or compostable packaging environmental impact and the resolve to behave pro-environmentally); physical opportunity (access to composting or compostable materials collection). The key barriers to buying products with biodegradable or compostable packaging are the psychological capability (not understanding terminology used to label packaging, not taking notice of packaging, and preferring other types of packaging and product qualities); reflective motivation (negative beliefs about biodegradable or compostable plastic packaging's environmental impacts and skepticism over decomposition claims), and physical opportunity (no access to composting or compostable materials collection). The analysis shows that labeling interventions are needed to improve information about the nature of the compostable material, their environmental impact and how to correctly dispose of them (Allison et al., 2021).
Our citizen science data also shows that even when citizens identify the correct disposal route, in this case putting a certified home compostable plastic into their home composter, the majority of these plastics still fail to effectively biodegrade (see Figure 13). The results for TUV OK Compost Home certified material showed successful composting of only 27% of the packaging tested, with a further 12% having small pieces (judged by the participants to be <2 mm in size) but still visible. These small pieces are technically “microplastics” and are likely to biodegrade fully should they be further exposed to the composting conditions. However this still leaves 61% of the packaging not meeting the expectations of a certified home compostable plastic. This is not a labeling problem but one of materials science. The range of item degradation results shows that the biodegradation process for home compostable plastics is complex and presents challenges for the regulation and certification of home compostable materials. A significant challenge is the diverse composition and form of biodegradable plastic packaging being marketed as home compostable and thus the complex mix and volume of polymers ending up in home compost. The time, temperature, humidity, pH, biodiversity and the microbacterial diversity within the home composter all play an important role in successful composting. Our data shows that there is weak impact of composting duration (Figure 12), geographical location (Figure 11) or composter type (Figure 9) which leads to our conclusion that the current mix of compostable polymers on the market will not reliably compost in the wide range of conditions found in home composting.
Our data also backs up the assessment by Siracusa (2019) that it is difficult to reproduce “natural experiments” in lab testing environments. The diversity of microbial communities, the catalytic pathways of nutrient transformation, the material chemical differences cannot be fully controlled and reproduced in vitro and hence home composability standards are likely to be unreliable, as Figure 13 shows clearly. The argument that the many citizens do not manage their home composter properly, which is the understandable reaction of many certifying organizations to this data, fails to take into account the real behavior of normal people, who have a range of abilities in regard to managing composting. This range of abilities is clearly not being captured by the lab tests which are used to certify home compostable plastics otherwise the failure rates of composting in the real world conditions would not be as large (see Figure 13).
We know from our data that the compost produced goes into the food chain of UK citizens. Even if some home compostable plastics are shown to fully compost in all UK home composts, it would be wise to assess the environmental impact of these materials, the inks and glues used, before assessing the environmental impact of home compost to properly dispose of biodegradable plastics.
With all these issues in mind, it is worth asking the question: What economic or environmental problem do biodegradable plastics solve? The bio-source of their carbon moves the packaging sector away from fossil-based plastics which typically do not fit in the framework of sustainable development (D'Adamo et al., 2020; Wurster and Ladu, 2020; Gerassimidou et al., 2021). But this is also true of bioplastic versions of PE, PP, and PET, which are fully recyclable, as well as being compatible with the current collection and sorting systems. Biodegradables are potentially useful for some product types that are not suited to recycling due to contamination such as tea bags, fruit labels, take-away food packaging, nappies, wipes and absorbent hygiene products. These products typically end up in landfill and, if the use of biodegradables were to divert them to industrial composting, then this might lead to a better outcome. We have shown home composting, being uncontrolled, is largely ineffective and would not be a good method of disposal. A separate collection for industrial composting might be the best environmental outcome. Work needs to be done to assess the design of such a system, to ensure that labeling drives correct behavior and that it could be economically viable.
There is also much materials science to be done in the design of compostable plastics. The requirements are stringent. They need to meet the mechanical, chemical and aesthetic property requirements of packaging applications and able to be stable and inert for long periods of time in warehouses and other storage conditions such in containers at sea. Once disposed of and collected they then need to switch from protective and inert, to being food for microorganisms. At the moment the switch is designed to be triggered through changes in the humidity and temperature but in the future other switches might be programmed into the material making them more robust as packaging material and more predictable as compostable plastics. Such compostable materials that sense their environment, compute a decision about their desired state, and react by chemically or mechanically transforming are part of the class of animate materials (The Royal Society, 2021). Applications for such animate compostable materials go beyond packaging to applications such as biodegradable tree guards (Chau et al., 2021), biodegradable fishing nets (Kim et al., 2016), and other useful plastic products that by the nature of their application are likely to end up polluting the environment.
We could not have assessed the effectiveness of home composting without using a citizen science approach. By its very nature the data we were trying to obtain was only available from citizens themselves and their back gardens. We believe it is the first data of its kind for compostable plastics. Nevertheless, there are defects with our citizen science experiment methodology that are worth noting. We chose not to be prescriptive about how the experiment was carried out and so relied on citizens being methodical and reliable observers. Participants were asked to identify and select items themselves according to experiment guidelines (i.e., items displaying words “compostable,” “home biodegradable,” “home compostable,” “suitable for home composting,” or TUV OK Compost “Home” or Din Certco “Home Compostable” certification marks). Although it is possible some participants might have made a mistake when inputting this information into our website, certification marks were verified from optional uploaded photos and/or from written descriptions submitted by participants. Some of the data classified as scale 4 may not have been fully biodegraded but just lost. Equally participants classification of scale 1 (intact), scale 2 (slight degradation) or scale 3 (heavy degradation) will have been subjective. There is also the potential that manufacturers of compostable packaging may have enrolled on the experiment and biased the results to make their products appear to compost more effectively. The large number of participants mitigates both of these potential problems to some extent and clearly the data does not vindicate any one product, so we consider that these potential biases are unlikely to have been dominant. We also restricted the number of items that participants could add to the composter, but this may not be representative of regular composting habits. In normal conditions composting greater quantities of home compostable packaging could be problematic due to their composition: effective composting requires a balance of sources of carbon and nutrients for the microorganisms. The result of using more compostable plastics would most likely have reduced the effectiveness of the composting of compostable plastic, so in this respect the experiment is biased toward better results. We asked participants to put items into a self selected net bag to help locate them at the end of the experiment. Although participants were advised to use a net bag with a loose weave to mitigate any secondary impacts such as slowing disintegration times or reducing contact with surrounding compost, the effect of this is not controlled for or verified. Food is widely reported to biodegrade completely when in net bags. However a slowdown of item disintegration times cannot be excluded. The most important bias of the experiment is likely to be due to self-selecting nature of the participants. Of those who signed up, the vast majority already separate their food waste and used a home composter, whereas these are both minority activities at present in the UK. There is no reason to think this bias would make the participant less able to carry out an effective experiment or to report it accurately. Thus, despite the use of hundreds of untrained citizens we have obtained an invaluable and important dataset on the effectiveness of home composting of compostable plastic packaging. Our conclusion is that home composting is not at present a viable, effective or environmentally beneficial waste processing method for compostable or biodegradable plastics in the UK.
Data availability statement
The datasets presented in this study can be found in online repositories. The names of the repository/repositories and accession number(s) can be found below: https://www.bigcompostexperiment.org.uk/gallery, www.bigcompostexperiment.org.uk/reports.
Ethics statement
Ethics approval for the study was granted by the UCL Research Ethics Committee (Project ID/Title: 16747/001: Big Compost Experiment) until 07 November 2022. The patients/participants provided their written informed consent to participate in this study.
Author contributions
DP, SM, and MM: conceptualization. DP and MM: methodology and writing—original draft preparation. DP and AA: formal analysis. DP, AA, FL, SM, and MM: writing—review and editing. DP: project administration. MM: funding acquisition. All authors have read and agreed to the published version of the manuscript.
Funding
This research was funded by EPSRC, NERC, and UKRI, under grants EP/S024883/1 and NE/V010735/1.
Acknowledgments
We would like to thank all members of the UCL Plastic Waste Innovation Hub, past and present, for their help developing the Big Compost Experiment and contributing to this research.
Conflict of interest
The authors declare that the research was conducted in the absence of any commercial or financial relationships that could be construed as a potential conflict of interest.
Publisher's note
All claims expressed in this article are solely those of the authors and do not necessarily represent those of their affiliated organizations, or those of the publisher, the editors and the reviewers. Any product that may be evaluated in this article, or claim that may be made by its manufacturer, is not guaranteed or endorsed by the publisher.
References
Allison, A. L., Lorencatto, F., Michie, S., and Miodownik, M. (2021). Barriers and enablers to buying biodegradable and compostable plastic packaging. Sustainability. 13, 1463. doi: 10.3390/su13031463
Association for Organics Recycling (2011). Concise guide to Compostable Products and Packaging: UK Local Authority Guidance. Available online at: www.organicsrecycling.org.uk/uploads/article1983/Concise%20guide%20to%20Compostable%20Products%20and%20Packaging.pdf. (accessed September 10, 2019).
Bagheri, A. R., Laforsch, C., Greiner, A., and Agarwal, S. (2017). Fate of so-called biodegradable polymers in seawater and freshwater. Global Chall. 1, 1700048. doi: 10.1002/gch2.201700048
Bátori, V., Åkesson, D., Zamani, A., Taherzadeh, M. J., and Horváth, I. S. (2018). Anaerobic degradation of bioplastics: a review. Waste Manage. 80, 406–413. doi: 10.1016/j.wasman.2018.09.040
British Standards Institution (2018). PAS 100:2018 Specification for Composted Materials. Available online at: http://www.standardsdevelopment.bsigroup.com/projects/9017-01020. (accessed October 10, 2019).
Brook Lyndhurst Ltd (2009). Household waste prevention evidence review : L3 m3-5 (T)—Attitudes and behaviours: Home composting; a report for Defra's waste and resources evidence programme. Available online at: http://sciencesearch.defra.gov.uk/ (accessed June 08, 2021).
Chau, C., Paulillo, A., Lu, N., Miodownik, M., and Lettieri, P. (2021). The Environmental Performance of Protecting Seedlings with Plastic Tree Shelters for Afforestation in Temperate Oceanic Regions: a UK Case Study. Science of The Total Environment. Available online at: https://www.sciencedirect.com/science/article/pii/S0048969721033106 (accessed June 07, 2021).
D'Adamo, P., Falcone, E., Imbert, P., and Morone, A. (2020). Socio-economic Indicator for EOL strategies for Bio-based Products. Ecologic. Econ. 178, 1–14. doi: 10.1016/j.ecolecon.2020.106794
DEFRA (2009). WR1204 Household Waste Evidence Review-A report for Defra L3 m3-5 (T) Attitudes and behaviour-home composting. Available online at: http://randd.defra.gov.uk (accessed April 01, 2009).
European Bioplastics (2018). Bioplastics—Bioplastics Market Data. Available online at: www.european-bioplastics.org/wp-content/uploads/2016/02/Report_Bioplastics-Market-Data_2018.pdf (accessed October 11, 2019).
European Bioplastics. (2016). Bioplastics—Industry Standards and Labels. Available online at: http://www.docs.european-bioplastics.org/2016/publications/fs/EUBP_fs_standards.pdf. (accessed September 10, 2019).
European Citizen Science Association. (2015). Ten Principles Of Citizen Science. Available online at: https://ecsa.citizen-science.net/sites/default/files/ecsa_ten_principles_of_citizen_science.pdf. (accessed July 01, 2020).
Funabashi, M., Ninomiya, F., and Kunioka, M. (2009). Biodegradability evaluation of polymers by ISO14855-2. Int. J. Mol. Sci. 10, 3635–3654. doi: 10.3390/ijms10083635
Garden Organic (2017). Compostable Packaging: How Well Does It Live Up To Its Claims? Available online at: https://www.gardenorganic.org.uk/compostable-packaging-how-well-does-it-live-its-claim (accessed June 08, 2021).
Gerassimidou, S., Martin, O., Chapman, S., Hahladakis, J., and Iacovidou, E. (2021). Development of an integrated sustainability matrix to depict challenges and trade-offs of introducing bio-based plastics in the food packaging value chain. J. Clean. Product. 20, 125378. doi: 10.1016/j.jclepro.2020.125378
Kim, S., Kim, P., Lim, J., An, H., and Suuronen, P. (2016). Use of biodegradable driftnets to prevent ghost fishing: physical properties and fishing performance for yellow croaker. Anim. Conserv. 19, n/a-n/a. doi: 10.1111/acv.12256
Kjeldsen, M., Price, C., Lilley, E., and Guzniczak, A. (2019). Review of Standards for Biodegradable Plastics. Available online at: http://www.assets.publishing.service.gov.uk/government/uploads/system/uploads/attachment_data/file/817684/review-standards-for-biodegradable-plastics-IBioIC.pdf (accessed October 10, 2019).
Lambert, S., and Wagner, M. (2017). Environmental performance of bio-based and biodegradable plastics: the road ahead. Chem. Soc. Rev. 46, 6855–6871. doi: 10.1039/C7CS00149E
Narancic, T., Verstichel, S., Reddy Chaganti, S., Morales-Gamez, L., Kenny, S. T., De Wilde, B., et al. (2018). Biodegradable plastic blends create new possibilities for end-of-life management of plastics but they are not a panacea for plastic pollution. Environ. Sci. Technol. 52, 10441–10452. doi: 10.1021/acs.est.8b02963
Ricardo Energy and Environment (2019). Plastics in the Bioeconomy: Report for the Biomass Biorefinaery Network ED12430. Available online at: https://cdn.ricardo.com/ee/media/downloads/ed12430-bb-net-report-final-issue-2.pdf. (accessed July 28, 2023).
Ruggero, F., Gori, R., and Lubello, C. (2019). Methodologies to assess biodegradation of bioplastics during aerobic composting and anaerobic digestion: a review. Waste Manage. Res. 37, 959–975. doi: 10.1177/0734242X19854127
Rujnić-Sokele, M., and Pilipović, A. (2017). Challenges and opportunities of biodegradable plastics: a mini review. Waste Manage. Res. 35, 132–140. doi: 10.1177/0734242X16683272
Scion (2020). Compostability Testing. Available online at: https://www.scionresearch.com/science/bio-based-products-and-technologies/compostability-testing (accessed July 17, 2020).
Siracusa, V. (2019). Microbial degradation of synthetic biopolymers waste. Polymers (Basel). 11, 1066. doi: 10.3390/polym11061066
Siracusa, V., Rocculi, P., Romani, S., and Rosa, M. D. (2008). Biodegradable polymers for food packaging: a review. Trends Food Sci. Technol. 19, 634–643. doi: 10.1016/j.tifs.2008.07.003
Taufik, D., Reinders, M. J., Molenveld, K., and Onwezen, M. C. (2020). The paradox between the environmental appeal of bio-based plastic packaging for consumers and their disposal behaviour. Sci. Total Environ. 705, 135820. doi: 10.1016/j.scitotenv.2019.135820
The Royal Society. (2021). Animate Materials perspective. Available online at: https://royalsociety.org/-/media/policy/projects/animate-materials/animate-materials-report.pdf (accessed June 02, 2021).
Vert, M., Doi, Y., Hellwich, K.-H., Hess, M., Hodge, P., Kubisa, P., et al. (2012). Terminology for biorelated polymers and applications (IUPAC recommendations 2012). Pure Appl. Chemistr. 84, 377–410. doi: 10.1351/PAC-REC-10-12-04
WasteMINZ (2020). Trial To Investigate Certified Compostable Materials In New Zealand Composting Systems. Available online at: https://www.wasteminz.org.nz/2019/11/trial-to-investigate-certified-compostable-materials-in-new-zealand-composting-systems/ (accessed July 17, 2020).
Wastling, T., Charnley, F., and Moreno, M. (2018). Design for circular behaviour: considering users in a circular economy. Sustainability (Switzerland) 10, 1743. doi: 10.3390/su10061743
WRAP (2007). Consumer Attitudes to Biopolymers. RSI 003 003, 37. Available online at: http://www.wrap.org.uk (accessed September 10, 2019).
WRAP (2016a). Anaerobic Digestion—The Process. Available online at: http://www.wrap.org.uk/collections-and-reprocessing/organics/anaerboic-digestion/guidance/adthe-process (accessed September 10, 2019).
WRAP (2016b). A Food Waste Recycling Action Plan for England. Available online at: https://archive.wrap.org.uk/sites/files/wrap/A_Food_Waste_Recycling_Action_Plan_For_England_0.pdf. (Accessed June 02, 2021).
WRAP (2018). The UK Plastics Pact. Available online at: http://www.wrap.org.uk/content/the-ukplastics-pact (accessed October 11, 2019).
WRAP (2020). Considerations For Compostable Plastic Packaging. Available online at: https://www.wrap.org.uk/sites/files/wrap/Considerations-for-compostable-plastic-packaging.pdf (accessed July 10, 2020).
Keywords: compostable, biodegradable, plastic packaging, citizen science, behavior change, home compostable certification
Citation: Purkiss D, Allison AL, Lorencatto F, Michie S and Miodownik M (2022) The Big Compost Experiment: Using citizen science to assess the impact and effectiveness of biodegradable and compostable plastics in UK home composting. Front. Sustain. 3:942724. doi: 10.3389/frsus.2022.942724
Received: 13 May 2022; Accepted: 03 October 2022;
Published: 03 November 2022.
Edited by:
Takuro Uehara, Ritsumeikan University, JapanReviewed by:
Francesco Degli-Innocenti, Novamont S.p.A.—Chimica Vivente per la Qualità della Vita, ItalySpyridoula Gerassimidou, University of Leeds, United Kingdom
Copyright © 2022 Purkiss, Allison, Lorencatto, Michie and Miodownik. This is an open-access article distributed under the terms of the Creative Commons Attribution License (CC BY). The use, distribution or reproduction in other forums is permitted, provided the original author(s) and the copyright owner(s) are credited and that the original publication in this journal is cited, in accordance with accepted academic practice. No use, distribution or reproduction is permitted which does not comply with these terms.
*Correspondence: Mark Miodownik, bS5taW9kb3duaWsmI3gwMDA0MDt1Y2wuYWMudWs=; Danielle Purkiss, ZGFuaWVsbGUucHVya2lzcyYjeDAwMDQwO3VjbC5hYy51aw==