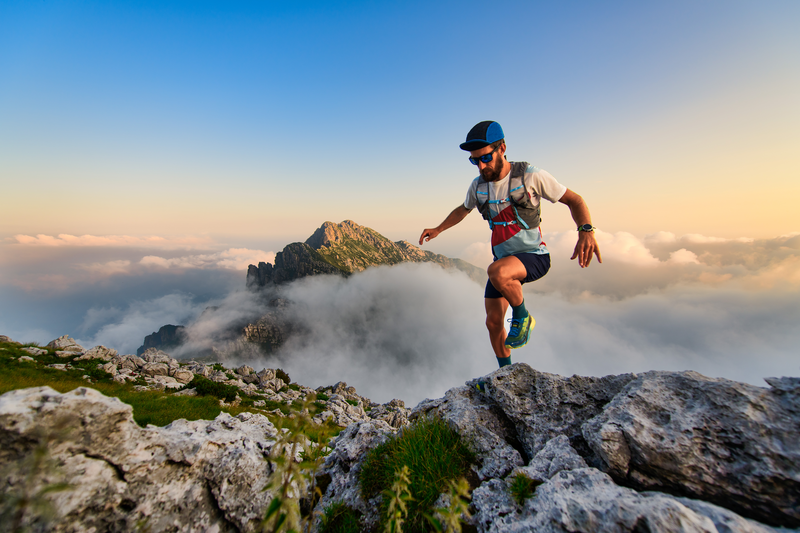
94% of researchers rate our articles as excellent or good
Learn more about the work of our research integrity team to safeguard the quality of each article we publish.
Find out more
ORIGINAL RESEARCH article
Front. Sustain. , 16 September 2022
Sec. Circular Economy
Volume 3 - 2022 | https://doi.org/10.3389/frsus.2022.931417
This article is part of the Research Topic Early-stage Quantitative Sustainability Assessment: Approaches for Policy, Processes and Materials View all 6 articles
We have studied the impacts of polypropylene (PP) and poly lactic acid (PLA) to quantify the differences between fossil-based and first generation biosourced plastics. Preliminary results on impact assessment from manufacturing stages suggested that the smaller the lot size and part weight of each injection molded plastic material, the higher the economic and environmental impacts. When lot size and part weight were equal, PLA performed better than PP. In three regional development scenarios, we have studied the impacts of end-of-life (EOL) options for smaller-sized and potentially landfilled single-use food packaging materials in town (population <10 k), city (population 30–250 k), and province (population >1 M) regional scales. The impacts of the change from PP to PLA as well as landfill (L) and open incineration (OI) to other EOL options, such as recycling (R), composting (CP), and incineration with energy recovery (IwE), were studied. Impacts of toxic damages are calculated as their impact on the healthcare sector. Thus, microplastics (MP) as a vector of bioaccumulation of toxins, such as dioxins, resulted in 16,5 $/kg MP on a province scale. In the Province scenario, where L PP (90%), a mix of R and OI PP was changed to a mix of R and CP PLA resulting in 63% economic gain and 39% lower global warming potential (GWP). In the City scenario, where L PP was changed to a mix of R PP (50%), IwE PP (25%), and IwE PLA (25%) resulting in 22% economic gain and 26% lower GWP. However, the higher the waste management activities such as sorting and waste processing, the higher the high-carcinogens (+137%), high non-carcinogens (+456%), and toxic release for total air (+9%) emissions. Future work should be done to study the impacts of other toxic compounds such as food contact chemicals to compare different food packaging materials to obtain more comprehensive results.
- A new model to simulate and optimize societal impacts of plastic utilization was developed for regional strategic decision-making.
- Composting systems and toxic effects of microplastic pollution were included in the model.
- Lot size and part weight optimization reduced the impacts of injection molded plastics.
- Controlled minimization of waste management activities may significantly reduce toxic releases and damage to the human health of plastic waste management.
The climate crisis challenges economic systems to act in support of sustainability; however, unrevealing the complete picture to accomplish a change requires sound global resolutions. Thus, contradictory perspectives of global and regional actors in our current economic system have brought up heated debates. Some of the famous debates include the environmental impacts of the utilization of plastics. Initially, fossil fuel depletion and greenhouse gas (GHG) emissions caused by plastic manufacturing processes and as a result of mismanaged plastic waste, the drastic increase in plastic pollution all around the world have been the majority part of the utilization of plastics debates. Indeed, the importance of plastics in our society cannot be overlooked, since they may create value for consumer goods such as providing protection during transportation and a desired shelf life, allowing the diversification of certified products throughout the supply chain (Van Herpen and Immink, 2016), improving the general experience of goods by providing affordable, durable, transparent, flexible and stable products, as well as support other sectors such as lowering fuel consumption by providing lighter parts for vehicles (Al-Fatlawi et al., 2021). However, the pollution caused by various fragments of plastics, which is mostly called microplastic pollution has direct and indirect effects on the ecosystem and human health (Green et al., 2016; Rodrigues et al., 2019; Pinto Da Costa et al., 2020; Anderson and Shenkar, 2021; Chagas et al., 2021; Huerta-Lwanga et al., 2021; Petrlik et al., 2021; Torres et al., 2021; Wang et al., 2021). Mismanaged plastics accumulate all around the world because of resistance to degradation, especially in marine debris and landfills (Coyle et al., 2020; Folino et al., 2020; Weinstein et al., 2020; Alimi et al., 2021; Silva et al., 2021; Zurier and Goddard, 2021) and GHGs such as methane are found to be emitted from plastic wastes littered in various natural environments (Royer et al., 2018).
Degradation of polymers occurs by biotic and abiotic forces in three processes (Emadian et al., 2017; Zhang et al., 2021): (1) Deterioration which is the change in the chemical, physical and mechanical properties of the polymers, (2) fragmentation which is the change in the structure of polymer by becoming smaller pieces and/or conversion of the polymer into oligomers and monomers, and (3) assimilation which is the transformation of plastics to CO2, water, biomass, and minerals. Regardless of their origins, the environmental factors affecting the degradation of plastics are pH, temperature, moisture, oxygen content, microbial level, and UV light. The intrinsic factors of the plastic material are the physical structure, chemical composition, polymer chain and its complexity, crystallinity, and the complexity of the polymer formula. Plastics are not the only compound that is resistant to degradation, which are found in the environment. Persistent organic pollutants (POPs) are known to be resistant against environmental degradation. These include dioxins and dioxin-like-compounds (DLCs; EPA, 2021a; Stockholm Convention, 2021). These compounds can be highly toxic, released from industrial processes, absorbed by microplastics (MPs) and nanoplastics in nature, and they will then be bioaccumulated in animal and human tissues (Rodrigues et al., 2019; Petrlik et al., 2021; Wang et al., 2021). Accumulation and concentration of dioxins and DLCs in the food chain are one of the dangerous pathways of these pollutants toward reaching the human body. Other toxic compounds such as heavy metals, pesticides, and polycyclic aromatic compounds (PAHs) emitted to the environment by human activities might follow the same path (Gong et al., 2019; Tubić et al., 2019; Chai et al., 2020; Shen et al., 2021). Thus, the emerging risk to human health by the interaction between these pollutants and plastics in nature is a disregarded subtopic of the plastic pollution problem.
MPs' trojan-horse effect of transferring organic compounds and more specifically for POPs into biological systems are documented in the literature (Tubić et al., 2019; Wang et al., 2021). Hydrophobic interactions between plastics and these compounds cause the sorption of pollutants on the surface and inside MP particles. This phenomenon is not expected to be resolved by the utilization of bioplastics, such as PLA, because neither industrial nor home composting facility conditions (e.g., depacking, environmental factors, and retention time) are currently not optimized for the complete degradation of some PLA (Zhu and Wang, 2020; Briassoulis et al., 2021; Kakadellis et al., 2021). When the compost obtained from PLA is used as fertilizer or dumped into the environment, the digest may contribute to the microplastic pollution by biomicroplastics (BMPs). There are studies related to the damage of PLA BMPs on soil and water organisms' health (Green et al., 2016; Weinstein et al., 2020; Anderson and Shenkar, 2021; Chagas et al., 2021; Huerta-Lwanga et al., 2021; Liwarska-Bizukojc, 2021). Thus, mistreated biopolymers may indeed cause problems in the ecosystem. In addition, sorption of nanoplastics by other anthropogenic materials such as cigarette butts is proven to contribute to another pollution mechanism of MPs' transportation and fate in the marine environment (El Hadri et al., 2021) thus, making the plastic problem a more complex issue. Because of these mechanisms, plastic pollution should be accounted for bioaccumulation of POPs and toxic contaminants in our food chain (Wang et al., 2021). However, there is a lack of scientific consensus on the methodologies and results of the studies related to bioaccumulation mechanisms and ecotoxicity results (Liwarska-Bizukojc, 2021). We approached the current plastic problem by taking into account the mechanisms that would result in worst-case scenarios; thus, we may be able to understand and quantify the trade-offs of the possible decisions and developments. On the other hand, we did not include sector-specific additives and their potential contaminants to be able to create a more general model and a tool for assessing plastic utilization. However, toxic damages occur during the use stages from compounds such as food contact chemicals (FCCs) and their contaminants, as well as migration mechanisms of these compounds into food products (Hahladakis et al., 2018; De Tandt et al., 2021; Kirchkeszner et al., 2022) could alter the results of impact assessment on human health. FCCs may have effects on human health by using virgin plastics and recycled materials, as well as polluting the environment through mismanaged plastics.
In the case of biogenic carbon storage and release, it is noteworthy to consider emissions from agricultural practices, energy mixes of regions for biorefinery processes and end-of-life of bioplastics (Bishop et al., 2021b). Undoubtedly, even though mechanical recycling of conventional plastics is a promising option to save the day in developed parts of the world, emissions from conventional plastic manufacturing processes, economic and technical challenges of recycling processes, and exploitation of fossil fuels are under the confining layer of atmosphere-earth surface challenge to obtain realistic sustainability of conventional plastics compared to bio-sourced plastics. Although bioplastics and composting systems were emphasized in this study, a careful consideration should be done on implementing organizational changes. Manufacturing more sustainable materials may require additional equipment and technical service. What is more, constructing a strong network of supplier ecosystem might be challenging to gain experience organizationally, maintain a competitive option in the market, and innovate toward a bio-circular economy.
It is noteworthy to have an understanding about the motivational differences of decision makers and their approaches toward implementing interventions to overcome the problems that occur from the utilization of plastics. Two different discourses proposed by Dryzek (1997), the survivalist and the innovator (Promethean) are great examples to elaborate on the motivational differences (Milne and Gray, 2013). The survivalist stands on a more radical point which requires a change in human behavior for environmental governance by global policy makers and institutions to avoid depletion of the natural resources and disruption in the balance of ecosystems. On the contrary, the innovator perceives radical changes as unnecessary because either trends related to the crisis are exaggerated, or the technological advancements will prevail the depletion of natural resources and the price changes will shape up human behavior. When circular economy policy approaches all around the world were compared (Fitch-Roy et al., 2021), we might conclude that the world displays an innovator approach since the radical changes required to obtain transformative policies were found to be not sufficient. Moreover, the sustainability interventions should concern and be based on the economic, social, and environmental resource availability at a regional scale rather than an ideal global circular economy approach (Smetana et al., 2015). However, there is a further debate about whether geo region or subnational region should be concerned, considering differences such as meteorological, geological, socio-economic, and political. What is more, the size of the regions and the lack of specific data from some of the areas are among other challenges, which are being discussed. Overall, decision-making in an innovation process to fight against the plastic problem requires consideration and quantification of the mentioned points in a tailored approach rather than one-size fit all.
Bans on single-use plastics (Figure 1; EU Regulation 2020/2151, 2020), restrictions on importation of plastic waste by China and Türkiye (Qu et al., 2019; Gündogdu and Walker, 2021), and other movements (Circular Plastics Alliance, 2018; EPP, 2020; ANZPAC, 2021; Break Free from Plastic, 2021; Plastic Pollution Coalition, 2021) are established to stop plastic utilization and pollution. Basel and Stockholm conventions are amongst other actions taken to avoid toxic and hazardous chemical pollution which are related to our debate (Basel Convention, 2021; Stockholm Convention, 2021). Considering these interventions, research and development to obtain more sustainable materials, additives, and end-of-life solutions are in urgent need now.
Figure 1. Example from the EU Regulation 2020/2151 regarding the rules on harmonized marking specifications on single-use plastic products.
There are several options in the plastic material market, such as fossil-based polymers which have claims related to their compostability in certain conditions (e.g., PVA and PBAT), and other biosourced but non-compostable polymers which are produced from biobased feedstock called drop-in bioplastics (e.g., Bio-PP and Bio-PE). Additionally, there are bioplastics, which have both characteristics at the same time (e.g., PLA and PHAs). Another classification method for bioplastics depends on the origin of the materials used for feedstock. The first-generation bioplastics are produced from agricultural feedstocks (maize, potatoes, etc.; Wellenreuther et al., 2022), the second-generation bioplastics are valorization of waste and side streams (wastewater treatment plants, food processing, etc.; Jõgi and Bhat, 2020), and the third-generation bioplastics are from polymer production in plant tissues (switchgrass, algae, etc.; Roy Chong et al., 2022). Indeed, a downstream approach to tackle the plastic problem could be realized by focusing on waste management technologies and systems (BASF, 2018; Ayvaz-Cavdaroglu et al., 2019; Banias et al., 2020; Macquarie, 2020; Paes et al., 2020; Pluskal et al., 2021).
Meanwhile, the research and developments continue, economies that lack the liquidity to invest and safely operate these waste systems are encouraged to adopt Extended Producer Responsibility (EPR) schemes for national and even global Circular Economy, when waste exportation is included in these schemes. Thus, in essence, the EPR perspective follows the OECD's “Polluter Pays Principle” (OECD, 2022) which holds polluter stakeholders accountable for the investments and developments required to eliminate or lower the hazardous impacts on the environment and human health. Nowadays, these schemes are systemized by including extra taxes levied for circularity of plastics through systems, such as recycling of conventional plastics (Ellen MacArthur Foundation, 2021).
Exportation of waste and pollution from OECD to non-OECD countries and remote parts of the world is one of the hot topics of today's plastic sustainability debates (Wang et al., 2020; Liang et al., 2021). The informal waste sector and low management capacities in developing regions of the world have been neglected, thus social and environmental disasters followed (Lavigne et al., 2014). Furthermore, other waste management issues such as handling the incineration fly ash and bottom ash, landfill leachate, and toxic contaminants from recycling facilities are topics hopefully sooner than later need more research (Chai et al., 2020; Petrlik et al., 2021; Shen et al., 2021; Silva et al., 2021). The research as well as corrective and preventive actions are required primarily for those who settle and work around these waste facilities. Since a batch of waste that will be incinerated may include all kinds of plastics, e-waste, and other industrial wastes, the formation of dioxins through waste management activities and complications in the containment of POPs are major concerns for the safe continuation of plastic waste management systems around the world (Petrlik et al., 2021).
There are additional sociological considerations on bioplastics and developing bioeconomy. Responsible sourcing of biomaterials may be achieved by a bioplastic feedstock alliance (BFA, 2022). However, discussions and decisions related to land use need regional and local considerations, thus participation and inclusion of local stakeholders play an important role (Umuhoza et al., 2019; Gerassimidou et al., 2022). Furthermore, considering large-scale monoculture agricultural practices to produce biomaterials, studies should be done to prevent drainage of soil health and to understand the eutrophication impacts of these practices. High-yielding genetically modified organisms and consumer behaviors toward the use of these crops are other inseparable discussions related to bioeconomy. Moreover, the effects of mismanaged biopolymer waste on the environment, and the upcoming issue of the insufficient waste management of these biopolymers in parts of the world due to utilization or waste exportation are yet other issues that are required to be shed light on.
The aim of our study was to develop a Life Cycle Assessment (LCA) and Techno-Economic Analysis (TEA) model to address the plastic problem and quantify the impacts of some of the most utilized fossil- and bioplastics polypropylene (PP) and first-generation polylactic acid (PLA), while considering the current debates and discussion points related to sustainability of plastics. The model can be considered for plastics utilized by various sectors. However, we have deep-dived into the issues of food packaging applications during our scenario analysis. The issues included economic (manufacturing, waste management, and health sector), environmental (emissions and microplastic pollution), and social (waste exportation and social inequalities) as an interconnected system. The study is a combination of cradle-to-grave LCA and TEA of PLA and PP polymers with a “Polluter Pays Principle” perspective. This perspective enables TEA to include required investments for the deployment, operating and maintenance of municipal solid waste management (MSWM) systems as well as to quantify the impacts of the worst-case scenarios for mismanaged plastics. Finally, the results are interpreted to generate strategies for plastic sustainability and obtain systematic implications for the circularity of plastics.
The research method for this study was the updated version of cradle-to-grave LCA and TEA modeling developed by Haylock and Rosentrater (2017). Indeed, the identification of different arguments and perspectives related to the sustainability of plastics was the first step in this work. Thus, a literature review was conducted to investigate different approaches, practices, and studies related to plastics manufacturing, EOL management, EOL impact assessment, toxic pollution mechanisms, and microplastic pollution from academia, websites, technical reports, and policies. The second step was the combination of the Economic Input-Output Life Cycle Assessment (EIO-LCA) method and Polluter Pays LCA (PP-LCA) model to evaluate costs and environmental impacts associated with material acquisition, processing, transport during plastic manufacturing, end-of-life (EOL) treatment during solid waste management (SWM), toxic damage on human health as well as positive externalities from energy recovery, material recovery, and fertilizer compost.
EIO-LCA allows users to estimate total economic activity and emissions in a supply chain of a specific industry. EIO-LCA Purchaser models provided the data for the cradle-to-factory gate and all the transportation steps until the point of purchase. Therefore, using the Purchaser 2002 model of EIO-LCA (Carnegie Mellon University Green Design Institute, 2022), we calculated the normalized EIO impact category per dollar basis Ecat (unit of emission/$) values for all the sectors studied in our study. Following EIO-LCA, TEA values per kg of material in each sector or kg of toxic compound which causes an economic activity in the healthcare sector were described as Csector ($/kg) in this article. Csector values were obtained from a combination of the MB database (M-Base, 2020), Brazilian Bank for Economic and Social Development Annual Report (BNDES, 2014), and literature review. Then, the costs related to each activity in that specific sector were multiplied by Ecat (unit of emission/$) to obtain environmental impact per kg material (Xunit, unit of emission/kg) as seen by the Eq. 1. This equation was used to calculate plastic manufacturing emissions (cradle-to-factory gate). Emissions during use and transportation were neglected. Finally, MSWM, positive externalities and toxic damage emissions were calculated from Eq. 2 for each end-of-life scenarios in each region. Therefore, each impacted sectoral activity was expressed by i, and Ecat, i defined the EIO-LCA normalized values of a sector (see Figure 2).
Emission categories analyzed included global warming potential (GWP), toxic releases for total air (TR TA), surface water (TR SW), underground water (TR UW) and land (TR L), air acidification (AA), air eutrophication (AE), water eutrophication (WE), air smog (AS), high carcinogens (HC), and high non-carcinogens (HNC). Sectoral Ecat values used in this study can be found in Supplementary Table 1. A detailed diagram to present PP-LCA calculations is shown in Figure 2. Therefore, the cost of plastics was multiplied by normalized plastic sector impact factors for each emission category analyzed; the cost of screening and waste management activities was multiplied by normalized waste management sector impact factors for each emission category analyzed; the monetary value of MSWM end products was multiplied by normalized sector impact factors according to the specific EOL scenario for each emission category analyzed; the cost of toxic damage on human health was multiplied by normalized healthcare and social assistance sector impact factors for each emission category analyzed. Thus, emission results for each material and scenario were calculated.
The impact flow diagram and system boundaries are presented in Figure 3. Therefore, the monetary value of MSWM end products was described as positive externalities. Positive externalities considered as end products of MSWM systems are demonstrated in the system boundaries of the PP-LCA model. Figure 3 visualizes emissions from plastic manufacturing, MSWM activities as well as toxic damage mechanism of POPs in MPs through water streams. The parameters compared between PP and PLA were the part size of the manufactured material (0.01 kg; 0.1 kg; 1 kg), the lot size of the production (1,000 kg; 100,000 kg; 1, 000,000 kg), the scale of the MSWM practice (<10 k inhabitants, Town; 30–250 k inhabitants, City; >1 M inhabitants, Province) and the EOL option (OI, open incineration; IwE, Incineration with energy recover; L, landfill; R, recycling; CP, composting). In addition, the details regarding different EOL options for MSWM practices in the model were created with the research funded by the Brazilian National Bank for Economic and Social Development (BNDES, 2014) and recent literature. Data from Brazil was used as an example of a developing country to achieve a realistic simulation of a development scenario. Brazilian Reals (R$) per ton of solid waste managed was converted to $/kg according to the exchange rates of the year studied. Furthermore, inflation converted values were used when needed according to the U.S. Bureau of Labor Statistics (BLS, 2021).
TEA of plastic manufacturing was done by using Eq. 3.
where N is the unit cost for plastic manufacturing, mt is the material type, pw is the part weight of the material, and ls is the lot size of a production batch. Economic impacts of MSWM and toxic damage caused by microplastic pollution were quantified by using Eq. 4, where W is the waste management system for region Rg, S is the screening or sorting, TP is the toxic pollution, and EP is the end product.
KT is the total cost, KS is the screening cost, KW is the selected MSWM system cost, KTP is the toxic pollution damage cost, VEP,W is the monetary value of the end product produced by W, and ηW is the output efficiency of the WRg. Sectoral costs were obtained by direct sectoral activity such as plastic manufacturing, MSWM, and healthcare costs. Furthermore, positive externality related to the same sector was subtracted to calculate the total economic activity in the sector. Therefore, the unit cost of open incineration (OI) for a region (Rg) was calculated by using Eq. 5.
Only toxic pollution damage costs from emitted PAHs by combustion of the specific material type were considered. The unit cost of IwE for a region Rg was calculated by using Eq. 6.
Costs of MSWM activity, toxic pollution (TP) damage from emitted PAHs by combustion of the specific material type mt, and the monetary value VEP from energy produced by the IwE with an efficiency of ηIwE were considered. The unit cost of landfilling for a region Rg was calculated by using Eq. 7.
Costs of MSWM activity and toxic pollution (TP) from bioaccumulation of dioxin-like compounds (DLCs) by microplastics (MPs) of the specific material type (mt) were considered. The unit cost of recycling (R) for the region (Rg) was calculated by using Eq. 8.
Costs of screening (S), MSWM activity from recycling (R) and IwE of unsorted parts, and monetary value (VEP) from material recovered by recycling (R) with an efficiency of ηR and energy produced by the IwE with an efficiency of ηIwE were considered. The unit cost of CP for a region (Rg) was calculated by using Eq. 9.
Costs of screening (S), MSWM activity, toxic pollution (TP) damage from bioaccumulation of polycyclic aromatic compounds (PAHs) by microplastics (MPs) of the specific material type (mt), and monetary value (VEP) from organic fertilizer produced by the composting (CP) with an efficiency of ηC were considered. Overall, Ci values to calculate Xunit in Eq. 2 were obtained by costs related to a particular sector, see Figure 2. Furthermore, a detailed assumption list of the values used for the end-of-life (EOL) scenario modeling is summarized in Table 1.
The model was used to simulate three development scenarios as described below (Table 2). Material part weights, lot sizes, and regions were kept constant for each of the scenarios.
Table 2. Development scenarios (OI, Open Incineration; IwE, Incineration with Energy recovery; L, Landfill; R, Recycling; CP, Composting).
This scenario is described as the development of the plastic waste management system of a town where 100% of utilized PP is sent to the landfill of the town. After necessary collaborative and participatory efforts are taken, the town has now replaced its PP utilization to 100% PLA and decided to 100% of the waste to be composted. The organic compost will be used to fertilize the town's farms and gardens.
This scenario is described as the development of the plastic waste management system of a coastal city where 100% of utilized PP is sent to the landfill of the city. After necessary collaborative and participatory efforts are taken, the city has now replaced 25% of its PP utilization with PLA and decided that 50% of the PP will be recycled; the rest of the waste to be incinerated with energy recovery. Recycled PP will be sold to the industry and the energy will be supplied for municipal use.
This scenario is described as the development of the plastic waste management system of a coastal province where 90% of utilized PP is sent to the landfill of the province and the remaining 10% is equally recycled and open incinerated. After necessary collaborative and participatory efforts are taken, the province has now replaced all the PP utilization with PLA and decided to recycle and compost PLA equally. Recycled PLA will be sold to the industry and the organic compost will be used to fertilize the province's farms and gardens.
Tables 3, 4 present TEA results of PP and PLA with different part weights and lot sizes.
The manufacturing costs of different part weights and lot sizes included equipment, electricity, maintenance, and labor costs. Assumptions related to product manufacturing cost calculations were done according to the model used by the past study (Haylock and Rosentrater, 2017), and Tables 3, 4 only include the results for cradle-to-point of purchase. Therefore, PLA performed better in terms of cost compared to PP when both materials have the same part weight and lot size. Furthermore, the higher the part weight is lower the material cost for each kg of material since the production of smaller part weight demands finer size and costlier pellet material. At a low lot size such as 1,000 kg, materials had the worst performance in terms of price. As expected, the fixed capital cost to manufacture plastic materials results in higher prices regardless of the material type. In literature (Van den Oever et al., 2017), price of PLA (2.2 $/kg) are found to be higher than of fossil-based plastics (0.95–1.7 $/kg) and around two times more expensive than PP (1.2 $/kg). However, the literature did not indicate specific data related to part weight or lot sizes. Moreover, more specific conditions such as unstable fossil-based plastic prices due to the fluctuations in oil prices (Van den Oever et al., 2017), thinner and lighter material utilization thanks to the higher stiffness of PLA compared to polystyrene (PS; Schut, 2016), and economy of scale could result in favor of PLA.
Table 5 presents TEA results of different municipal practices in terms of regional scales and EOL options. The MSWM costs for different scenarios included capital investment, equipment, and labor costs. Cost data and classifications of regional levels and SWM systems were obtained from BNDES (2014)' report about treatment technologies and final disposal of solid waste. In addition, CAPEX and OPEX values for recycling systems were gathered from Larrain et al. (2021)'s work, which reflected the costs related to a fully developed mechanical recycling system. The monetary value of end products from these MSWM activities included data from various literature sources, see Table 1. Therefore, the TEA comparison of each EOL option for both materials was found as L > OI > CP > IwE > R. R provided the best results on any scale when Brouwer et al. (2020)'s optimum recycling and polymer purity rates are assumed to be effective for both PP and PLA.
Table 5. TEA of different municipal practices for PP and PLA (OI, 100% Open Incineration; IwE, 100% Incineration with energy recovery; L, 100% Landfill; R, 100% Recycling; CP, 100% Composting).
Assuming the best possible scenario of a mechanical R option, this system would provide maximum economical gain for the economy. However, there are technical limits for achieving a 100% circular economy with R. The higher thermal degradation during R processes of bioplastics and the lower final recyclate quality of PLA compared to PP increase the criteria to consider whether to go for R of bioplastics (Briassoulis et al., 2021; Larrain et al., 2021). Although in our study the final recyclate quality difference was neglected, higher recoverable energy from unrecycled and incinerated parts of PP compared to those of PLA created a small difference between TEA results for R. In addition, a higher unrecycled ratio of plastics leads to higher incineration rates and encourages the use of incineration options. Thus, design for recyclability in all stages of the circular plastic chain is needed. Furthermore, there are environmental and health impacts caused by toxins and contaminants released during R processes (Petrlik et al., 2021). In addition, uncollected, unsorted, and unrecyclable parts of manufactured plastic materials have avoidable and mitigable impacts, together with unmeasurable ones because of the unclear interactions between plastics, other waste materials, and the environment.
The second most preferable EOL option found was IwE in cities and provinces however mismanaged toxic externalities and GHGs might alter total societal cost. Thus, the potencies of toxic emissions and the formation of compounds, such as POPs during combustion-based MSWM systems, should be carefully understood and studied. In addition, current literature points out the risks of DLCs formation primarily in electronic waste management facilities (Petrlik et al., 2021). Moreover, the different VEP, IwE results for PP and PLA arise from the specific potential energy characteristics of the materials as they are shown in Table 1. Thus, the available recovered energy from each material was found to be different.
CP option for PLA might be the third best option in every region when PLA was considered as a part in CP of food waste. Even when the toxic damages from biomicroplastic pollution simulated in the model according to Näkki et al. (2021)'s results of PAH absorption by PLLA, there were no significant toxic damage cost differences found for any of the regional scales (Table 6). However, there is an urgent need for more research related to the sorption of toxic compounds by biodegradable plastics (Torres et al., 2021). Furthermore, for towns, OI is found to be more preferable than CP when data from BNDES were considered. In the scale of a town, the costs of managing composting systems made CP less preferable than OI. What is more, the formation of other toxic pollutants through OI of contaminated waste streams and potential fire hazards were neglected. When traditional backyard CP, or home CP was considered, MWMS costs might be discarded for the CP option. Because do-it-yourself methods for this CP equipment and operation cost little to no money for households. Thus, CP systems could have benefits for the local communities. However, circumstances of potential external economic and environmental impacts due to the requirements for home composting equipment and increased water usage for cleaning/washing must be included in calculations, especially when city and province regions are considered.
Table 6. TEA of externalities (OI, 100% Open Incineration; IwE, 100% Incineration with energy recovery; L, 100% Landfill; R, 100% Recycling; CP, 100% Composting).
Finally, L showed the worst results for all the regions apart from town L since toxic damage of DLCs from town L was neglected in the model. However, if microplastic pollution is carried by any water stream such as by floods or rivers to coastal regions (Gündogdu et al., 2018; Alimi et al., 2021), eventually the toxic damage would be comparable to city and province landfills. Furthermore, the results from coastal regions, such as Taitung City and New Taipei City, where they were found to be already contaminated with DLCs demonstrated the devastating effects of microplastic pollution due to toxin concentration and bioaccumulation mechanisms (Wang et al., 2021). In addition, composting of PLA in non-optimal conditions may contribute to a similar toxic mechanism of microplastics as presented in landfill results. Indeed, sorption and bioaccumulation mechanisms of toxins should be studied further for clear TEA results. The values in the model may be developed further by data from future studies.
Table 6 and Figure 4 further describe the externalities of the results from Table 5. TEA results of waste management depending on the scale of the region and the complexity of the waste management process, as well as toxic damage and monetary value of end products, are shown. TEA results of S and MSWM systems included CAPEX and OPEX costs for each region.
Figure 4. TEA of solid waste management systems (OI, 100% Open Incineration; IwE, 100% Incineration with energy recovery; L, 100% Landfill; R, 100% Recycling; CP, 100% Composting).
Generally, R was the most expensive method while generating the most economical value as demonstrated in Table 6. In an optimum R scenario, our results demonstrate that R systems would be profitable in any region unless required investments are made. Indeed, the influence of oil prices on virgin plastic prices is a global concern, thus price fluctuations might impact recycled material markets. What is more, cost savings in screening or sorting had a significant impact on the final performance of the SWM system. However, for developing regions, the reality of collecting, sorting, and waste processing systems as well as the development level of the recycled material market are far different than in developed regions. Thus, case studies should be examined to understand the economic benefits of R systems according to the development level of a specific region. Furthermore, toxic externalities from OI increase according to the population density of the region, further details are described in the Holland and Watkiss (2002) work. Even though OI was the cheapest option in terms of management costs, damages on the society and the low efficiency of energy recovery make it unfavorable. In terms of economic gain from incineration activities, the energy mix used in the country and the price of MWh influence the feasibility of these systems as it is further discussed in the BNDES (2014)'s annual report.
CP could be an option to lower the costs and environmental impact of microplastic pollution, MSWM, and food waste. After all, food-packaging materials are usually contaminated with organic residues, and CP is recognized as an effective option to lower the environmental impacts of food waste issues (EPA, 2021b). However, in our model, the indirect effects of PLA CP on lowering food waste emissions are not completely addressed. Therefore, industrial investments and research about compostable plastic waste management can promote innovation to fight against both plastic pollution and food waste issues. However, the quality of final compost from 100% PLA plastic waste is found to be poor and Nitrogen-Phosphorus-Potassium (N-P-K) ratios are to be low (Gerassimidou et al., 2021), even though a “rich quality” compost depends on the purpose of the application. Furthermore, current municipal compost projects support CP and the local use of compost fertilizer (ISTAÇ, 2021; Lyon, 2021). Urban gardening was found to stimulate a change toward more sustainable consumer behavior as well as grant food security for households especially during COVID-19 (Nova et al., 2020; Niles et al., 2021). Thus, in our study PLA was considered as a part of food waste CP and planned to be used for gardening purposes in local communities. Taking into account packaging and food waste together in LCA studies was considered to provide more accurate results in terms of environmental impact assessment of such food packaging solutions (Bishop et al., 2021a). Furthermore, for the Town scenario, we assumed that PLA was home compostable, which will be technologically possible in the future (Carbiolice, 2021).
Overall, TEA results indicate that the manufacturing of plastics with a part weight of 0.01 kg costs the highest compared to 0.1 kg and 1 kg. Considering the current single-use plastics debate, a plastic part with a weight of about 10 g would be produced for food packaging (bottle, yogurt cup, etc.), thus continuing the analysis with a 0.01 kg part weight would generate more meaningful results for addressing the mentioned debate in this article. Furthermore, selecting 100,000 kg as the lot size for PP-LCA would represent purchasing orders from medium to large-scale companies and a more realistic scenario considering the low production rate of PLA around the world.
Table 7 represents the economic and environmental impact results of the scenarios compared. Results indicate economic gains for each scenario but higher environmental effects in high carcinogens (HC) and high non-carcinogens (HNC) categories for every scenario. Additionally, toxic release for total air (TR TA) values are found to be higher for town and city scenarios. According to the model, the increase in these emission categories was related to higher MSWM costs, increased waste management sectoral activity, and advanced complexity of the systems in the new projects. In addition, TR TA levels were found to be increased in the Town and City scenarios, this may be caused by the air trapping effect of L. Thus, changing L with CP or IwE maybe the cause of this result. Overall, interventions in the screening/sorting systems to lower costs of MSWM and corrective actions to contain toxic pollutants would significantly lower the impacts of R, IwE, and CP, as discussed in this study. Finally, projected changes in these scenarios prove that correct investments and interventions can lower societal costs and environmental impacts. However, achieving positive change may require consideration in certain trade-offs, such as net-zero GHG emissions over economic costs.
First, Ecat values used in the model to calculate emission results were from the EIO-LCA tool, which considers the US economy from 2002. Land-use and eutrophication impacts from agricultural activities were not considered, even though the importance of these impacts is questioned in the literature (Morão and de Bie, 2019). Nonetheless, the severity of these impacts depends on the geographical location and the type of agricultural practices. Furthermore, working conditions, allergies, and intoxications in working environments were not considered in the model. A further toxicological investigation is needed to be included in the model to include other toxic compound impacts and address toxic compound formation during SWM of different municipal waste mixtures. Assuming the toxic potency of all DLCs and PAHs, and their impacts on the health sector in different parts of the world as equal have resulted in another error margin for our scenario results. Additionally, operational issues for manufacturing and MSWM were examined only briefly. Overall, propagation of uncertainty was not studied in our model.
The significance of the regional decision-making and covering the total effects of societal impact mechanisms in LCA studies were demonstrated in PP-LCA. Regional perspectives for LCA modeling and EOL options were found to be also in line with other literature related to MSWM (Rosecký et al., 2021). The necessity of public body approaches on specific territorial levels according to socio-economic and demographic variables on waste generation as well as the relationship between MSWM and EP market were highlighted in the literature. What is more, a similar approach to quantify the societal lifetime impacts of fossil-based plastics was presented in a report (DeWit et al., 2021). The report presented the costs of plastics by market, unaccounted production processes, waste management, and mismanaged waste. It was found that the total quantifiable societal lifetime cost of plastics was between 6 and 12 times the cost of all plastics in the global market in 2019. In our study, we have found up to 63% societal economic cost reductions in the scenario analysis, and even up to 88% in the province region by replacing landfilled PP with composted PLA (Supplementary Table 2).
Since it would be impossible to take the right precautions without quantifying the EOL impacts of plastics, current findings support the importance of the PP-LCA approach. Therefore, the outcomes of our study are presented as possible intervention strategies corresponding to the waste management market and plastic material products, see Table 8.
Existing materials imply both fossil-based plastics and first-generation bioplastics found in the plastics market. New materials imply bioplastics sourced by second- or third-generation biopolymers (Wellenreuther et al., 2022) and materials that are manufactured or designed with an attention to their recyclability and biodegradability properties. New and existing waste management markets imply the required systemic changes, as well as technical points. Therefore, for both existing materials and waste management markets, preliminary results suggested that regardless of the material type; part weight and lot size optimizations could result in benefits in all categories studied. However, high consumption of energy-dense food products and growing food waste issues should be considered. Furthermore, literature points out the necessary regional approaches on handling the current waste materials (Zhao et al., 2016; Espinoza Pérez et al., 2021) and opportunities of promising recycling systems, such as anaerobic co-digestion of bioplastics (Abraham et al., 2021) and chemical recycling of fossil plastics (Jiang et al., 2022). What is more, a ban on waste trade would limit current criminal activities in the sector, although transparent waste exportation is believed to improve recycling markets in developing regions. Moreover, our study highlighted the impacts of landfilled or littered plastic waste by toxic compound sorption on MPs, and bioaccumulation mechanisms of toxins through these MPs. Therefore, in the event of no recycling system placed in a region, we suggest that those materials which are biodegradable by consumers or nature (soil, marine, compost) itself in a safe manner should be utilized to limit the societal costs of plastics. One of the interesting waste management systems is so-called backyard composting or home composting does not demand a facility for waste management. Thus, it offers a great potential to address solid waste management challenges for local authorities and higher the accessibility to fertilizers (FAO, 2022). However, plastics should have certain biodegradability properties to be organically recycled, especially in home composting conditions. Consequently, existing fossil and biobased and newer materials from second- and third-generation bioplastics as well as EP of these materials produced by MSWM activities should be studied carefully to fully understand the hazards and risks of plastics to the environment and our society.
The complexity of sustainability debate on plastics was discussed in the present work. Some of the disregarded topics in other LCA studies, such as the impact mechanisms of microplastic pollution on human health, emissions from the healthcare sector, biogenic emissions from waste management of biosourced materials, challenges of plastic sustainability in developing parts of the world, and advantages of MSWM end products, were represented in the work. Furthermore, a highlight was done on another overlooked point in the debate, compostable materials, composting waste management systems and the advantages of compost fertilizer. We have transformed a new model called “Polluter Pays Life Cycle Assessment” to quantify and simulate societal impacts of different scenarios considering material type, different size products, scales of production batches, development level of the waste management sector of a given region, and EOL planning. Part weight and lot size were kept constant in scenario analysis, which provided an even setting for decision-making of a more sustainable material and EOL option. This setting allowed to keep the consumer behavior and packaging purchasing orders of a food product manufacturer to be consistent. Consequently, the change from L PP to R and C PLA resulted in 63% economic gains and a reduction in all emission categories between 14 and 60% in the province region. However, higher activity in MSWM systems leads to higher emission levels of carcinogenic and non-carcinogenic compounds, and toxic releases to air. Future studies on the health impact assessment of FCCs and pollution risks of other hazardous additives and contaminants used in virgin and recycled plastic manufacturing, as well as the leaching of these chemicals into the environment, should be conducted.
Current interventions do not embrace the whole picture of the plastic problem, in terms of technical realities of waste management systems and social inequalities related to plastic waste importation which have a direct impact on the sustainability of plastics. Therefore, the PP-LCA model can be used as a comprehensive tool to assess and compare the impacts of current and projected plastic utilization and waste management scenarios.
The original contributions presented in the study are included in the article/Supplementary material, further inquiries can be directed to the corresponding authors.
DA and KR conceived the work. DA built the models and drafted the paper. KR and SG supervised the work, helped to write the manuscript, and edited the manuscript. All authors contributed to the article and approved the submitted version.
The authors declare that the research was conducted in the absence of any commercial or financial relationships that could be construed as a potential conflict of interest.
All claims expressed in this article are solely those of the authors and do not necessarily represent those of their affiliated organizations, or those of the publisher, the editors and the reviewers. Any product that may be evaluated in this article, or claim that may be made by its manufacturer, is not guaranteed or endorsed by the publisher.
The Supplementary Material for this article can be found online at: https://www.frontiersin.org/articles/10.3389/frsus.2022.931417/full#supplementary-material
Abraham, A., Park, H., Choi, O., and Sang, B.-I. (2021). Anaerobic co-digestion of bioplastics as a sustainable mode of waste management with improved energy production – a review. Bioresour. Technol. 322, 124537. doi: 10.1016/j.biortech.2020.124537
Al-Fatlawi, A., Károly, J., and György, K. (2021). Optimal design of a fiber-reinforced plastic composite sandwich structure for the base plate of aircraft pallets in order to reduce weight. Polymers 13, 834. doi: 10.3390/polym13050834
Alimi, O. S., Fadare, O. O., and Okoffo, E. D. (2021). Microplastics in African ecosystems: current knowledge, abundance, associated contaminants, techniques, and research needs. Sci. Tot. Environ. 755, 142422. doi: 10.1016/j.scitotenv.2020.142422
Alves, B. (2021). Electricity Supply Prices in Brazil 2019. Statista. Available online at: https://www.statista.com/statistics/1029715/brazil-electricity-supply-rate/ (accessed October 19, 2021).
Americanas (2021). Adubo e Composto Orgânico 15kg Esterco Bovino Leiteiro em promoção: Ofertas na Americanas[AmericanasFertilizer and Organic Compost 15kg Dairy Bovine Manure on sale: Offers at Americanas]. Available online at: https://www.americanas.com.br/produto/73469434?pfm_carac=fertilizante-organico-composto&pfm_index=22&pfm_page=search&pfm_pos=grid&pfm_type=search_page&offerId=6078aa650c07044266c7f0de (accessed October 19, 2021).
Anderson, G., and Shenkar, N. (2021). Potential effects of biodegradable single-use items in the sea: polylactic acid (PLA) and solitary ascidians. Environ. Poll. 268, 115364. doi: 10.1016/j.envpol.2020.115364
ANZPAC (2021). About. Available online at: https://anzpacplasticspact.org.au/ (accessed October 19, 2021).
Ayvaz-Cavdaroglu, N., Coban, A., and Firtina-Ertis, I. (2019). Municipal solid waste management via mathematical modeling: a case study in Istanbul, Turkey. J. Environ. Manag. 244, 362–369. doi: 10.1016/j.jenvman.2019.05.065
Banias, G., Batsioula, M., Achillas, C., Patsios, S. I., Kontogiannopoulos, K. N., Bochtis, D. D., et al. (2020). A life cycle analysis approach for the evaluation of municipal solid waste management practices: the case study of the region of central Macedonia, Greece. Sustainability 12, 8221. doi: 10.3390/su12198221
Basel Convention (2021). Basel ConventionHome Page. Basel.int. Available online at: http://www.basel.int/ [accessed October 20, 2021).
BASF (2018). Chemical Recycling of Plastic Waste. Available online at: https://www.basf.com/global/en/who-we-are/sustainability/we-drive-sustainable-solutions/circular-economy/mass-balance-approach/chemcycling.html (accessed October 20, 2021).
BFA (2022). Encouraging the Responsible Development of Plant-Based Plastics. Available online at: https://bioplasticfeedstockalliance.org (accessed April 28, 2022).
Bishop, G., Styles, D., and Lens, P. N. (2021a). Environmental performance of bioplastic packaging on fresh food produce: a consequential life cycle assessment. J. Clean. Prod. 317, 128377. doi: 10.1016/j.jclepro.2021.128377
Bishop, G., Styles, D., and Lens, P. N. (2021b). Environmental performance comparison of bioplastics and petrochemical plastics: a review of life cycle assessment (LCA) methodological decisions. Resour. Conserv. Recycl. 168, 105451. doi: 10.1016/j.resconrec.2021.105451
BLS (2021). CPI Inflation Calculator. U. S. Bureau of Labor Statistics. Available online at: https://www.bls.gov/data/inflation_calculator.htm (accessed October 19, 2021).
BNDES (2014). Analysis of Various Treatment Technologies and Final Disposal of Solid Waste in Brazil, Europe, USA and Japan. REPORT UFPE FADE BNDESFEP N°02/2010. Available online at: https://www.researchgate.net/publication/268811782_Analysis_of_Various_Treatment_Technologies_and_Final_DiDispos_of_Solid_Waste_in_Brazil_Europe_USA_and_Japan (accessed October 20, 2021).
Break Free from Plastic (2021). Envisioning a Future Free From Plastic Pollution. Break Free From Plastic. Available online at: https://www.breakfreefromplastic.org/ (accessed October 19, 2021).
Briassoulis, D., Pikasi, A., and Hiskakis, M. (2021). Recirculation potential of post-consumer /industrial bio-based plastics through mechanical recycling—techno-economic sustainability criteria and indicators. Polym. Degr. Stability 183, 109217. doi: 10.1016/j.polymdegradstab.2020.109217
Brouwer, M. T., Thoden van Velzen, E. U., Ragaert, K., and Ten Klooster, R. (2020). Technical limits in circularity for plastic packages. Sustainability 12:10021. doi: 10.3390/su122310021
Carbiolice (2021). Carbiolice= Compostable Plastic Solutions. Available online at: https://www.carbiolice.com/en/ (accessed October 19, 2021).
Carnegie Mellon University Green Design Institute (2022). Economic Input-Output Life Cycle Assessment (EIO-LCA) US 2002 (428 sectors) Purchaser model. Available online at: http://www.eiolca.net/ (accessed July 5, 2022).
Chagas, T. Q., Araújo, A. P., and Malafaia, G. (2021). Biomicroplastics vs. conventional microplastics: an insight on the toxicity of these polymers in dragonfly larvae. Sci. Tot. Environ. 761, 143231. doi: 10.1016/j.scitotenv.2020.143231
Chai, B., Wei, Q., She, Y., Lu, G., Dang, Z., and Yin, H. (2020). Soil microplastic pollution in an e-waste dismantling zone of China. Waste Manag. 118, 291–301. doi: 10.1016/j.wasman.2020.08.048
Chen, Y.-T. (2016). A cost analysis of food waste composting in Taiwan. Sustainability 8, 1210. doi: 10.3390/su8111210
Chien, Y.-C., Liang, C., Liu, S.-H., and Yang, S.-H. (2010). Combustion kinetics and emission characteristics of polycyclic aromatic hydrocarbons from polylactic acid combustion. Air Waste Manage. Assoc. 60, 849–855. doi: 10.3155/1047-3289.60.7.849
CIEL (2019). Plastic & Climate The Hidden Costs of a Plastic Planet. Available online at: www.ciel.org/plasticandclimate (accessed October 20, 2021).
Circular Plastics Alliance (2018). Circular Plastics Alliance- Internal Market, Industry, Entrepreneurship and SMEs - European Commission. Internal Market, Industry, Entrepreneurship and SMEs - European Commission. Available online at: https://ec.europa.eu/growth/industry/policy/circular-plastics-alliance_en (accessed October 20, 2021).
Coyle, R., Hardiman, G., and Driscoll, K. O. (2020). Microplastics in the marine environment: a review of their sources, distribution processes, uptake and exchange in ecosystems. Case Stud. Chemical Environ. Eng. 2, 100010. doi: 10.1016/j.cscee.2020.100010
De Tandt, E., Demuytere, C., Van Asbroeck, E., Moerman, H., Mys, N., Vyncke, G., et al. (2021). A recycler's perspective on the implications of REACH and food contact material (FCM) regulations for the mechanical recycling of FCM plastics. Waste Manag. 119, 315–329. doi: 10.1016/j.wasman.2020.10.012
DeWit, W., Burns, E. T., Guinchard, J., and Ahmed, N. (2021). Plastics: The Costs to Society, The Environment and The Economy. WWF. Available online at: https://wwf.panda.org/wwf_news/?3507866/These-costs-for-plastic-produced-in-2040-will-rise-to-US71-trillion-unless-urgent-action-is-taken (accessed April 15, 2022).
Dryzek, J. (1997). The Politics of the Earth: Environmental Discourses, 3rd Edn. Oxford: Oxford University Press.
El Hadri, H., Lisa, J. M., Gigault, J., Reynaud, S., and Grassl, B. (2021). Fate of nanoplastics in the environment: implication of the cigarette butts. Environ. Poll. 268, 115170. doi: 10.1016/j.envpol.2020.115170
Ellen MacArthur Foundation (2021). Extended Producer Responsibility Is a Necessary Part of the Solution to Packaging Waste and Pollution. plastics.ellenmacarthurfoundation.org. Available online at: https://plastics.ellenmacarthurfoundation.org/epr?mc_cid=e5c89f04b7&mc_eid=12b422e876#Position-paper (accessed October 19, 2021).
Emadian, S. M., Onay, T. T., and Demirel, B. (2017). Biodegradation of bioplastics in natural environments. Waste Manag. 59, 526–536. doi: 10.1016/j.wasman.2016.10.006
EPA (2021a). Dioxin and Dioxin-Like Compounds Toxic Equivalency Information. US EPA. Available online at: https://www.epa.gov/toxics-release-inventory-tri-program/dioxin-and-dioxin-compounds-toxic-equivalency-information (accessed October 20, 2021).
EPA (2021b). Reducing the Impact of Wasted Food by Feeding the Soil and Composting. US EPA. Available online at: https://www.epa.gov/sustainable-management-food/reducing-impact-wasted-food-feeding-soil-and-composting (accessed October 20, 2021).
EPP (2020). Home - European Plastics Pact. European Plastics Pact. Available online at: https://europeanplasticspact.org/ (accessed October 19, 2021).
Espinoza Pérez, L., Ziegler-Rodríguez, K., Espinoza Pérez, A. T., Vásquez, Ó. C., and Vázquez-Rowe, I. (2021). Closing the gap in the municipal solid waste management between metropolitan and regional cities from developing countries: a life cycle assessment approach. Waste Manag. 124, 314–324. doi: 10.1016/j.wasman,.2021.02.020
EU Regulation 2020/2151 (2020). Harmonised Marking Specifications for Beverage Cups. European Parliament, Council of the European Union. Available online at: https://eur-lex.europa.eu/legal-content/EN/TXT/?uri=CELEX:32020R2151 (accessed October 19, 2021).
FAO (2022). The Benefits of Composting, Reusing and Recycling Nutrients for Agricultural Productivity. Available online at: https://www.fao.org/land-water/overview/covid19/composting/en/ (accessed April 28, 2022).
Fitch-Roy, O., Benson, D., and Monciardini, D. (2021). All around the world: assessing optimality in comparative circular economy policy packages. J. Clean. Prod. 286, 25493. doi: 10.1016/j.jclepro.2020.125493
Folino, A., Karageorgiou, A., Calabrò, P. S., and Komilis, D. (2020). Biodegradation of wasted bioplastics in natural and industrial environments: a review. Sustainability 12, 6030. doi: 10.3390/su12156030
Gerassimidou, S., Lovat, E., Ebner, N., You, W., Giakoumis, T., Martin, O. V., et al. (2022). Unpacking the complexity of the UK plastic packaging value chain: a stakeholder perspective. Sustain. Prod. Consumpt. 30, 657–673. doi: 10.1016/j.spc.2021.11.005
Gerassimidou, S., Martin, O. V., Chapman, S. P., Hahladakis, J. N., and Iacovidou, E. (2021). Development of an integrated sustainability matrix to depict challenges and trade-offs of introducing bio-based plastics in the food packaging value chain. J. Clean. Prod. 286, 125378. doi: 10.1016/j.jclepro.2020.125378
Gong, W., Jiang, M., Han, P., Liang, G., Zhang, T., and Liu, G. (2019). Comparative analysis on the sorption kinetics and isotherms of fipronil on nondegradable and biodegradable microplastics. Environ. Poll. 254, 112927. doi: 10.1016/j.envpol.2019.07.095
Green, D. S., Boots, B., Sigwart, J., Jiang, S., and Rocha, C. (2016). Effects of conventional and biodegradable microplastics on a marine ecosystem engineer (Arenicola marina) and sediment nutrient cycling. Environ. Poll. 208, 426–434. doi: 10.1016/j.envpol.2015.10.010
Greenwood, S. C., Walker, S., Baird, H. M., Parsons, R., Mehl, S., Webb, T. L., et al. (2021). Many Happy Returns: combining insights from the environmental and behavioural sciences to understand what is required to make reusable packaging mainstream. Sustain. Prod. Consumpt. 27, 1688–1702. doi: 10.1016/j.spc.2021.03.022
Gündogdu, S., Çevik, C., Ayat, B., Aydogan, B., and Karaca, S. (2018). How microplastics quantities increase with flood events? An example from Mersin Bay NE Levantine coast of Turkey. Environ. Poll. 239, 342–350. doi: 10.1016/j.envpol.2018.04.042
Gündogdu, S., and Walker, T. R. (2021). Why Turkey should not import plastic waste pollution from developed countries? Mar. Poll. Bullet. 171, 112772. doi: 10.1016/j.marpolbul.2021.112772
Hahladakis, J. N., Velis, C. A., Weber, R., Iacovidou, E., and Purnell, P. (2018). An overview of chemical additives present in plastics: migration, release, fate and environmental impact during their use, disposal and recycling. J. Hazard. Mater. 344, 179–199. doi: 10.1016/j.jhazmat.2017.10.014
Haylock, R., and Rosentrater, K. A. (2017). Cradle-to-grave life cycle assessment and techno-economic analysis of polylactic acid composites with traditional and bio-based fillers. J. Polym. Environ. 26, 1484–1503. doi: 10.1007/s10924-017-1041-2
Holland, M., and Watkiss, P. (2002). Benefits Table Database: Estimates of the Marginal External Costs of Air Pollution in Europe (BETA). London: AEA Technology, NETCEN.
Huerta-Lwanga, E., Mendoza-Vega, J., Ribeiro, O., Gertsen, H., Peters, P., and Geissen, V. (2021). Is the polylactic acid fiber in green compost a risk for Lumbricus terrestris and Triticum aestivum? Polymers 13, 703. doi: 10.3390/polym13050703
ISTAÇ (2021). Geri Kazanim ve Kompost. ISTAÇ. Available online at: https://istac.istanbul/tr/temiz-istanbul/atik-yonetimi/geri-kazanim-ve-kompost (accessed 19 October 19, 2021).
Jiang, J., Shi, K., Zhang, X., Yu, K., Zhang, H., He, J., et al. (2022). From plastic waste to wealth using chemical recycling: a review. J. Environ. Chemical Eng. 10, 106867. doi: 10.1016/j.jece.2021.106867
Jõgi, K., and Bhat, R. (2020). Valorization of food processing wastes and by-products for bioplastic production. Sustain. Chem. Pharm. 18, 100326. doi: 10.1016/j.scp.2020.100326
Kakadellis, S., Woods, J., and Harris, Z. M. (2021). Friend or foe: Stakeholder attitudes towards biodegradable plastic packaging in food waste anaerobic digestion. Resour. Conserv. Recycl. 169, 105529. doi: 10.1016/j.resconrec.2021.105529
Kirchkeszner, C., Petrovics, N., Tábi, T., Magyar, N., Kovács, J., Szabó, B. S., et al. (2022). Swelling as a promoter of migration of plastic additives in the interaction of fatty food simulants with polylactic acid- and polypropylene-based plastics. Food Control 132, 108354. doi: 10.1016/j.foodcont.2021.108354
Larrain, M., Van Passel, S., Thomassen, G., Van Gorp, B., Nhu, T. T., Huysveld, S., et al. (2021). Techno-economic assessment of mechanical recycling of challenging post-consumer plastic packaging waste. Resour. Conserv. Recycl. 170, 105607. doi: 10.1016/j.resconrec.2021.105607
Lavigne, F., Wassmer, P., Gomez, C., Davies, T. A., and Hadmoko, D. S. (2014). The 21 February 2005, catastrophic waste avalanche at Leuwigajah dumpsite, Bandung. Indonesia 1, 12. doi: 10.1186/s40677-014-0010-5
Li, C. T., Zhuang, H., Hsieh, L., Lee, W. J., and Tsao, M. C. (2001). PAH emission from the incineration of three plastic wastes. Environ. Int. 27, 61–67. doi: 10.1016/S0160-4120(01)00056-3
Liang, Y., Tan, Q., Song, Q., and Li, J. (2021). An analysis of the plastic waste trade and management in Asia. Waste Manag. 119, 242–253. doi: 10.1016/j.wasman.2020.09.049
Liwarska-Bizukojc, E. (2021). Effect of (bio)plastics on soil environment: a review. Sci. Tot. Environ. 795, 148889. doi: 10.1016/j.scitotenv.2021.148889
Lyon (2021). Je composte mes déchets - La Métropole de Lyon [I compost my waste - LyonMetropolis]. Available online at: https://www.grandlyon.com/services/je-composte-mes-dechets.html (accessed October 19, 2021).
Macquarie (2020). Financing Waste Infrastructure in Indonesia. Available online at: greeninvestmentgroup.com (accessed October 19, 2021).
Martinez-Sanchez, V., Levis, J. W., Damgaard, A., DeCarolis, J. F., Barlaz, M. A., and Astrup, T. F. (2017). Evaluation of externality costs in life-cycle optimization of municipal solid waste management systems. Environ. Sci. Technol. 51, 3119–3127. doi: 10.1021/acs.est.6b06125
M-Base (2020). Base - M-base. M-Base. Available online at: https://www.m-base.de/en/ (accessed October 19, 2021).
Milne, M., and Gray, R. H. (2013). W(h)ither ecology? The triple bottom line, the global reporting initiative, and corporate sustainability reporting. J. Bus. Ethics 118, 13–29. doi: 10.1007/s10551-012-1543-8
Morão, A., and de Bie, F. (2019). Life cycle impact assessment of polylactic acid (PLA) produced from sugarcane in Thailand. J. Polym. Environ. 27, 2523–2539. doi: 10.1007/s10924-019-01525-9
Näkki, P., Eronen-Rasimus, E., Kaartokallio, H., Kankaanpää, H., Setälä, O., Nyman, E., et al. (2021). Polycyclic aromatic hydrocarbon sorption and bacterial community composition of biodegradable and conventional plastics incubated in coastal sediments. Sci. Tot. Environ. 755, 143088. doi: 10.1016/j.scitotenv.2020.143088
Niles, M. T., Wirkkala, K. B., Belarmino, E. H., and Bertmann, F. (2021). Home food procurement impacts food security and diet quality during COVID-19. BMC Public Health 21, 945. doi: 10.1186/s12889-021-10960-0
Nova, P., Pinto, E., Chaves, B., and Silva, M. (2020). Urban organic community gardening to promote environmental sustainability practices and increase fruit, vegetables and organic food consumption. Gaceta sanitaria. 34, 4–9. doi: 10.1016/j.gaceta.2018.09.001
OECD (2022). Recommendation of the Council on the Implementation of the Polluter-Pays Principle. OECDLegal Instruments. Available online at: https://legalinstruments.oecd.org/en/instruments/11 (accessed July 16, 2022).
Paes, M. X., de Medeiros, G. A., Mancini, S. D., Bortoleto, A. P., Puppim de Oliveira, J. A., and Kulay, L. A. (2020). Municipal solid waste management: Integrated analysis of environmental and economic indicators based on life cycle assessment. J. Clean. Prod. 254, 119848. doi: 10.1016/j.jclepro.2019.119848
Petrlik, J., Bell, L., Beeler, B., Møller, M., Jopkova, M., Arisandi, P., et al. (2021). Plastic Waste Disposal Leads to Contamination of the Food Chain. Gothenburg: International Pollutants Elimination Network (IPEN).
Pinto Da Costa, J., Rocha-Santos, T. C, and Duarte, A. (2020). The Environmental Impacts of Plastics and Micro-Plastics Use, Waste and Pollution: EU and National Measures. Available online at: europa.eu (accessed October 19, 2021).
Plastic Pollution Coalition (2021). Plastic Pollution Coalition. Plastic Pollution Coalition. Available online at: https://www.plasticpollutioncoalition.org/ (accessed October 20, 2021).
Pluskal, J., Šomplák, R., Nevrlý, V., Smejkalov, V., and Pavlas, M. (2021). Strategic decisions leading to sustainable waste management: separation, sorting and recycling possibilities. J. Clean. Prod. 278, 123359. doi: 10.1016/j.jclepro.2020.123359
Qu, S., Guo, Y., Ma, Z., Chen, W., Liu, J., Liu, G., et al. (2019). Implications of China's foreign waste ban on the global circular economy. Resour. Conserv. Recycl. 144, 252–255. doi: 10.1016/j.resconrec.2019.01.004
Rodrigues, J. P., Duarte, A. C., Santos-Echeandía, J., and Rocha-Santos, T. (2019). Significance of interactions between microplastics and POPs in the marine environment: a critical overview. Trends Analyt. Chem. 111, 252–260. doi: 10.1016/j.trac.2018.11.038
Rosecký, M., Šomplák, R., Slavík, J., Kalina, J., Bulková, G., and Bednár, J. (2021). Predictive modelling as a tool for effective municipal waste management policy at different territorial levels. J. Environ. Manag. 291, 112584. doi: 10.1016/j.jenvman.2021.112584
Roy Chong, J. W., Tan, X., Khoo, K. S., Ng, H. S., Jonglertjunya, W., Yew, G. Y., et al. (2022). Microalgae-based bioplastics: future solution towards mitigation of plastic wastes. Environ. Res. 206, 112620. doi: 10.1016/j.envres.2021.112620
Royer, S., Ferrón, S., Wilson, S. T., and Karl, D. M. (2018). Production of methane and ethylene from plastic in the environment. PLoS ONE 13, e0200574. doi: 10.1371/journal.pone.0200574
Sarker, M., Rashid, M. M., and Molla, M. A. (2011). Abundant high-density polyethylene (HDPE-2) turns into fuel by using of HZSM-5 catalyst. J. Fundamentals Renew. Energy Appl. 1, 1–12. doi: 10.4303/jfrea/R110201
Schut, J. H. (2016). Pioneering sustainability; biopolymer applications are expanding, in fits and starts. Plastics Eng. 72, 6–13. doi: 10.1002/j.1941-9635.2016.tb01490.x
Shen, M., Hu, T., Huang, W., Song, B., Qin, M., Yi, H., et al. (2021). Can incineration completely eliminate plastic wastes? An investigation of microplastics and heavy metals in the bottom ash and fly ash from an incineration plant. Sci. Tot. Environ. 779, 146528. doi: 10.1016/j.scitotenv.2021.146528
Silva, A. L. P., Prata, J. C., Duarte, A. C., Soares, A. M. V. M., Barceló, D., and Rocha-Santos, T. (2021). Microplastics in landfill leachates: the need for reconnaissance studies and remediation technologies. Case Stud. Chem. Environ. Eng. 3, 100072. doi: 10.1016/j.cscee.2020.100072
Smetana, S., Tamásy, C., Mathys, A., and Heinz, V. (2015). Sustainability and regions: sustainability assessment in regional perspective. Region. Sci. Pol. Practice 7, 163–186. doi: 10.1111/rsp3.12068
Stockholm Convention (2021). Stockholm Convention- Home page. Chm.pops.int. Available online at: http://chm.pops.int/ (accessed October 20, 2021).
Tayeh, R. A., Alsayed, M. F., and Saleh, Y. (2021). The potential of sustainable municipal solid waste-to-energy management in the Palestinian Territories. J. Clean. Prod. 279, 123753. doi: 10.1016/j.jclepro.2020.123753
Torres, F. G., Dioses-Salinas, D. C., Pizarro-Ortega, C. I., and De-la-Torre, G. E. (2021). Sorption of chemical contaminants on degradable and non-degradable microplastics: recent progress and research trends. Sci. Tot. Environ. 757, 143875. doi: 10.1016/j.scitotenv.2020.143875
Tubić, A., Lončarski, M., Maletić, S., Molnar Jazić, J., Watson, M., Tričković, J., et al. (2019). Significance of chlorinated phenols adsorption on plastics and bioplastics during water treatment. Water 11, 2358. doi: 10.3390/w11112358
Umuhoza, M., Kakshapati, S., Guendouz, Z., and Mugisha, B. (2019). A Stakeholder analysis of PET wastes management in Kigali, Rwanda. Int. J. Sci. Res. Publ. 9, p8595. doi: 10.29322/IJSRP.9.01.2019.p8595
Van den Oever, M., Molenveld, K., Van der Zee, M., and Bos, H. (2017). Bio-based and biodegradable plastics: facts and figures : focus on food packaging in the Netherlands. Wageningen Food Biobased Res. 2017, 408350. doi: 10.18174/408350
Van Herpen, E., and Immink, V. (2016). Organics unpacked: the influence of packaging on the choice for organic fruits and vegetables. Food Qual. Pref. 53, 90–96. doi: 10.1016/j.foodqual.2016.05.011
Wang, C., Zhao, L., Lim, M. K., Chen, W.-Q., and Sutherland, J. W. (2020). Structure of the global plastic waste trade network and the impact of China's import Ban. Resour. Conserv. Recycl. 153, 104591. doi: 10.1016/j.resconrec.2019.104591
Wang, L.-C., Lin, J. C.-T., Dong, C.-D., Chen, C.-W., and Liu, T.-K. (2021). The sorption of persistent organic pollutants in microplastics from the coastal environment. J. Hazard. Mater. 420, 126658. doi: 10.1016/j.jhazmat.2021.126658
Weinstein, J. E., Dekle, J. L., Leads, R. R., and Hunter, R. A. (2020). Degradation of bio-based and biodegradable plastics in a salt marsh habitat: another potential source of microplastics in coastal waters. Mar. Poll. Bullet. 160, 111518. doi: 10.1016/j.marpolbul.2020.111518
Wellenreuther, C., Wolf, A., and Zander, N. (2022). Cost competitiveness of sustainable bioplastic feedstocks – a Monte Carlo analysis for polylactic acid. Clean. Eng. Technol. 6, 100411. doi: 10.1016/j.clet.2022.100411
Zhang, K., Hamidian, A. H., Tubić, A., Zhang, Y., Fang, J., Wu, C., et al. (2021). Understanding plastic degradation and microplastic formation in the environment: a review. Environ. Poll. 274, 116554. doi: 10.1016/j.envpol.2021.116554
Zhao, J., Huang, L., Lee, D.-H., and Peng, Q. (2016). Improved approaches to the network design problem in regional hazardous waste management systems. Transport. Res. E Logist. Transport. Rev. 88, 52–75. doi: 10.1016/j.tre.2016.02.002
Zhu, J., and Wang, C. (2020). Biodegradable plastics: green hope or greenwashing? Mar. Poll. Bullet. 161, 111774. doi: 10.1016/j.marpolbul.2020.111774
Zurier, H. S., and Goddard, J. M. (2021). Biodegradation of microplastics in food and agriculture. Curr. Opin. Food Sci. 37, 37–44. doi: 10.1016/j.cofs.2020.09.001
AA, Air Acidification; AE, Air Eutrophication; AS, Air Smog; BMP, Bio Microplastic; BNDES, Brazilian Bank for Economic and Social Development; CP, Composting; CAPEX, Capital Expense; DLC, Dioxin-like-Compound; EIO-LCA, Economical Input-Output Life Cycle Assessment; EOL, End-of-life; EPR, Extended Producer Responsibility; GHG, Greenhouse Gas; GWP, Global Warming Potential; HC, High Carcinogens; HNC, High Non-Carcinogens; IwE, Incineration with Energy Recovery; L, Landfill; LCA, Life Cycle Assessment; MP, Microplastic; MSWM, Municipal Solid Waste Management; N-P-K, Nitrogen-Phosphorus-Potassium; OI, Open Incineration; OPEX, Operational Expense; PAH, Polycyclic Aromatic Compound; FCC, Food Contact Chemical; PLA, Polylactic Acid; POP, Persistent Organic Pollutant; PP, Polypropylene; PP-LCA, Polluter Pays Life Cycle Assessment; R, Recycling; R$, Brazilian Real; SWM, Solid Waste Management; TEA, Techno-Economical Analysis; TR L, Toxic Releases for Land; TR SW, Toxic Releases for Surface Water; TR TA, Toxic Releases for Total Air; TR UW, Toxic Releases for Underground Water; WE, Water Eutrophication.
Keywords: plastic waste, circular economy, biodegradability, microplastics, bioplastics, waste management, toxic pollutants
Citation: Atabay D, Rosentrater KA and Ghnimi S (2022) The sustainability debate on plastics: Cradle to grave Life Cycle Assessment and Techno-Economical Analysis of PP and PLA polymers with a “Polluter Pays Principle” perspective. Front. Sustain. 3:931417. doi: 10.3389/frsus.2022.931417
Received: 29 April 2022; Accepted: 18 August 2022;
Published: 16 September 2022.
Edited by:
Eleni Iacovidou, Brunel University London, United KingdomReviewed by:
Hari Bhakta Sharma, Indian Institute of Technology Kharagpur, IndiaCopyright © 2022 Atabay, Rosentrater and Ghnimi. This is an open-access article distributed under the terms of the Creative Commons Attribution License (CC BY). The use, distribution or reproduction in other forums is permitted, provided the original author(s) and the copyright owner(s) are credited and that the original publication in this journal is cited, in accordance with accepted academic practice. No use, distribution or reproduction is permitted which does not comply with these terms.
*Correspondence: Doğacan Atabay, YXRhYmF5ZG9nYWNhbkBnbWFpbC5jb20=; Kurt A. Rosentrater, a2Fyb3NlbnRAaWFzdGF0ZS5lZHU=; Sami Ghnimi, c2FtaS5naG5pbWlAdW5pdi1seW9uMS5mcg==
Disclaimer: All claims expressed in this article are solely those of the authors and do not necessarily represent those of their affiliated organizations, or those of the publisher, the editors and the reviewers. Any product that may be evaluated in this article or claim that may be made by its manufacturer is not guaranteed or endorsed by the publisher.
Research integrity at Frontiers
Learn more about the work of our research integrity team to safeguard the quality of each article we publish.