- 1AZTI, Marine Research, Basque Research and Technology Alliance (BRTA), Sukarrieta, Spain
- 2GAIKER Technology Centre, Basque Research and Technology Alliance (BRTA), Zamudio, Spain
- 3Antex, Carrer Josep Hereu i Aulet, Girona, Spain
- 4Ternua Group, Gipuzkoa, Spain
Tropical tuna purse seiners are one of the most important contributors to end-of-life (EoL) fishing gears in the world, and these fishing nets can become a promising secondary raw material. Thus, tuna companies are looking for possibilities to valorize them by applying circular economy (CE). This contribution aims at assessing the viability of creating a circular business model out of recycled tropical tuna purse seine EoL nets. The yearly contribution of the Spanish tuna freezer purse seine companies to EoL fishing nets was estimated at 900 tons. Three pilot projects were implemented (involving 80 tons of EoL tuna nets) to learn about the monetary and material flows, supply chain, stakeholders' perception, and the environmental impacts of upcycling polyamide nets into four marketable products (i.e., conditioned fishing nets, backpacks, fishers' dungarees, and sunglasses). The results indicate that recycled regrinds/flakes and pellets were 37 and 50%, respectively, more expensive than virgin counterparts, but the yarn may achieve competitive production costs in the textile industry, with an additional environmental benefit close to 69% per kg of virgin–origin yarn. The challenges faced when recycling EoL polyamide fishing nets were discussed. Innovation and logistics appear to play a fundamental role in making the business sustainable. Besides, the circular business model methodology to assess the value proposition was also discussed in its empirical application.
Introduction
Circular economy (CE) has become a cornerstone of the quest of finding solutions to the marine plastic pollution. The three theoretical strategies under the CE paradigm fit with the desired solution for marine plastic: (i) minimize inputs of raw material and outputs of waste, (ii) keep the resource as long as possible within the system, and (iii) reintegrate products into the system when they reach the end of life (Suárez-Eiroa et al., 2019). Public administrations have echoed this challenge by developing a regulatory framework (Vollmer et al., 2020; Williams and Rangel-Buitrago, 2022). The European Strategy for Plastics in a CE and related action plans (European Commission, 2021) foster the adoption of sustainability criteria along the entire plastic supply chain from primary producers to converters, brand owners and retailers, waste collectors, and recyclers (Foschi and Bonoli, 2019). Particularly, the entry into force of policies designed to limit the use of single-use plastic products as a way to reduce the contribution of marine plastic [Directive (EU) 2019/904] together with the will of entrepreneurs for new opportunities has led to the creation of new initiatives from emerging market niches and business models on recovery of marine plastic (Dijkstra et al., 2021). In parallel, the textile and fashion industries have started to shift their focus toward sustainable fashion-making recycled marine plastic textiles (Khandual and Pradhan, 2019). Some examples are Adidas-Parley Ocean Plastic(R), Prada, Converse (Luo and Deng, 2021), Ecoalf's Upcycling the Ocean, Ternua Group (Peña-Rodriguez et al., 2021), Inditex, H&M, Hérmes (Ramos et al., 2020), and Patagonia (Leal Filho et al., 2019). This increasing number of initiatives indicates not only a new production model but also a consumption change in society.
Among marine plastics, end-of-life (EoL) fishing gears are particularly gaining attention within the CE paradigm (Bishop et al., 2020). Despite that the available regulations promote the sustainable management of recovered marine plastic [Directive (EU) 2019/883, Directive EC 2018/251], knowledge of the amount of EoL fishing gear generation is limited (Basurko et al., 2022). Likewise, the management of EoL fishing gear, today, is yet to be consistent with the waste hierarchy (Argüello, 2020), due to fishing gear waste is often dispensed in the “cheapest container,” i.e., the sea (Sherrington et al., 2016; Richardson et al., 2021), or it is sent to landfill or abroad because of the non-existence of recovery and valorization industry nearby the ports they are discarded. This latter point was highlighted by two studies analyzing the management of fishing gear in Spain and Norway. Basurko et al. (2022) studied the EoL fishing gear management practices by Spanish fishing fleets and ports and concluded that EoL fishing gear management is heterogeneous across the country, and the type of management depends on the location, nature of the ports, and whose responsibility it falls (i.e., regionally or nationally managed). Deshpande et al. (2020), in contrast, estimated that of the 4,000 tons of annually discarded fishing gears, 55% were sent abroad for recycling; they also underpinned the need to improve the recycling capabilities of the country to deal with discarded fishing gears.
Among all fisheries, tropical tuna purse seiners stand out as one of the most important contributors to EoL fishing gears in the world. The nets employed by this fishery are made mainly of high-quality polyamide (PA6) and can reach dimensions of up to 2,000 m long in perimeter and about 300 m in depth (Zudaire et al., 2020). The net size can vary depending on vessel characteristics, e.g., power or target species (ICCAT, 2006–2016). This leaves tropical purse seine shipowners with a high quality but large quantity of material (currently unquantified) in their base ports where they normally are deposited. This circumstance has been triggered, in part, by the prohibition of net reuse in the construction of drifting fish aggregating devices (dFADs) in the Indian Ocean (IOTC-2021-WGFAD02-INF02, 2021). Thus, the amount of tropical tuna purse seiners' EoL fishing gears (hereafter “EoL tuna nets”) stored in their fishing ports (an example is shown in Figure 1) calls for actions to foster their valorization.
Fishing gear valorization is feasible by establishing proper management, collection, conditioning, and recycling scheme; in turn, this can prevent fishing gears from becoming marine litter by being dumped at sea (Brodbeck, 2016). However, recovered fishing gears are often dirty and very degraded. Thus, the conditioning, recycling, and transport of such raw materials tend to be costly both in time and resources (Madricardo et al., 2020). In general terms, EoL fishing gears can be recycled mechanically (Mondragon et al., 2020) or chemically, or they can be incinerated (Arandes et al., 2004). The knowledge regarding the recyclability of polyamide-based fishing gears has particularly increased in recent years (Klun and Kržan, 2000; Brodbeck, 2016; Kamimura et al., 2019; Bertelsen et al., 2020; Feary et al., 2020; Garrido et al., 2020; Mondragon et al., 2020; Peña-Rodriguez et al., 2021), identifying the limitations of mechanical recycling (Madricardo et al., 2020). Despite the logistic chains derived from the recycling being studied (van Giezen and Wiegmans, 2020), there are still few examples of real implementation quantifying the cost-benefit along the value chain (from the fishing gear collection to the final product development) (Boldrini and Antheaume, 2021) together with the environmental impacts. Those who have succeeded omit to discuss the challenges encountered along the entire value chain producing valuable information that could help others achieve a competitive product made of marine plastic when developing a circular business model.
In line with the European strategies, business models in this field should be framed within the perspective of the CE. Circular business models (CBMs) stand as enablers for the implementation of the CE (Kirchherr et al., 2017). CBMs are rising as a more comprehensive version to define new business models within a CE perspective than traditional or linear business models (Nußholz, 2018), which only describe the rationale of how an organization creates, delivers, and captures value (Osterwalder and Pigneur, 2010). CBM aims to reconcile the creation of commercial value with the adoption of circular strategies that can prolong the useful life of products and parts and close material loops (Nußholz, 2017). CBMs are considered a class of sustainable business models (Geissdoerfer et al., 2018); they assess the environmental, economic, and social viability of the real implementation of a business (de Kwant et al., 2021) and envisage the environmental status while creating a business model. The scientific literature on CBM has increased in recent years providing several CBM definitions (Bocken et al., 2013; Nußholz, 2017; Manninen et al., 2018; Geissdoerfer et al., 2020) and theoretical reviews on its concept (Pieroni et al., 2018, 2019; Bocken et al., 2019; Lüdeke-Freund et al., 2019; Reim et al., 2019; Rosa et al., 2019; Centobelli et al., 2020; Geissdoerfer et al., 2020). The most general definition is the one proposed by Geissdoerfer et al. (2020) where CBM is defined as a “business model that are cycling, extending, intensifying, and/or dematerialising material and energy loops to reduce the resource inputs into and the waste and emission leakage our of an organizational system. This comprises recycling measures (cycling), use phase extension (extending), a more intense use phase (intensifying), and the substitution of products by service and software solution (dematerialising).” Some authors have also proposed different conceptual frameworks and tools to build CBMs (Joyce and Paquin, 2016; Nußholz, 2017; Manninen et al., 2018; Bocken et al., 2019; Lüdeke-Freund et al., 2019; Donati et al., 2020; Geissdoerfer et al., 2020; Boldrini and Antheaume, 2021); others have already discussed the implementation of CBM, barriers, and limitations (Núñez-Cacho et al., 2018; Dijkstra et al., 2020; Guldmann and Huulgaard, 2020; Liu et al., 2021). No contribution was found, however, on studies quantifying monetary and material flows and also environmental benefits of an empirical application of CBM of recycling EoL fishing nets, including also the stakeholders' knowledge of the whole value chain.
The recovery and recycling of EoL tuna nets emerge as a promising business opportunity that can profit from CE approaches, fitting in the CBM archetype “creating value from waste” (Bocken et al., 2014). Within this context, this study aims to (1) provide the first estimate on the amount of EoL tuna nets created by the Spanish tropical tuna freezer purse seine fleet, (2) assess the potential of building new CBM based on recycled EoL tropical tuna nets, and (3) identify the constraints and strengths of applying CBM models. For the first, the Spanish tropical tuna freezer purse seine shipowners were interviewed, and the annual amount of EoL fishing net was estimated, detailing the contribution of net component, material, current management, and discarding reason. To have enough stock to guaranteeing a cost effective production and a continuation of the new business is identified as a constraint, so are the low recycling capabilities of certain polymers and gears (Feary et al., 2020). For the second and third objectives, three case studies were carried out involving the real recovery, recycling, and upcycling of 80 tons of EoL tuna nets of Seychelles into four textile products marketed in Europe. Material balances, transport needs, and costs of each valorization stage (i.e., recovery, conditioning, recycling, and product creation) were measured empirically. The technical, economic, and environmental data obtained from each valorization stage of the value chain served to design a CBM for tuna purse seine net recycling. The CBM was built and contrasted with the stakeholders involved along the entire value chain. The results helped to identify the phase of the value chain that needs to be improved to achieve a competitive commercial product derived from the recycled fishing nets. Challenges faced by private companies when recycling EoL tuna nets were also assessed in the three levels of CE: micro, meso, and macro levels (Suárez-Eiroa et al., 2019).
Theoretical framework
According to the European Green Deal, the CE will create sustainable growth in Europe. This statement is supported by other authors that recommend CE as an approach for reconciling economic growth with sustainable environment and economic development (George et al., 2015; Korhonen et al., 2018a; Busu and Trica, 2019; Androniceanu et al., 2021). The acquiring importance of the CE concept is reflected in the growing number of studies on the subject. There are several literature reviews on the definition of the CE (Geissdoerfer et al., 2017; Kirchherr et al., 2017), one of the more complete definitions being the one by Geissdoerfer et al. (2017): “a regenerative system in which resource input and waste, emission, and energy leakage are minimized by slowing, closing, and narrowing material and energy loops. This can be achieved through long-lasting design, maintenance, repair, reuse, remanufacturing, refurbishing, and recycling”. However, a lack of theoretical analysis of the CE was identified (Corvellec et al., 2022), arguing that it has been mainly developed by practitioners and because a single generally accepted definition is still lacking. Thus, it has been claimed that CE is not a theory but an emerging approach to industrial production and consumption (Korhonen et al., 2018b).
Following the premise that CE is an approach and not a theory, several authors (George et al., 2015; Geissdoerfer et al., 2017; Gao et al., 2020) attribute the embryonic idea of CE to Boulding (1966) and the introduction of CE concept to Pearce et al. (1989). The theoretical background of the CE has been slightly studied in the last few years (George et al., 2015; Ghisellini et al., 2016; Korhonen et al., 2018b), relating CE with economic growth theories, based on CE aims to create sustainable growth. Several authors affirm that CE operates around the neoclassical exogenous growth theory (Ghisellini et al., 2016; Bimpizas-Pinis et al., 2021; Donaghy, 2022), but this theory does not consider the limitation of natural resources availability as a constraint (Meadows et al., 1972; Kornafel and Telega, 2020), which is one of the main ideas underlying the concept of the CE. This limitation (Ghisellini et al., 2016) is contemplated in the steady-state economy theory (Daly, 1977), which aligns it with the CE philosophy. However, it lacks tools for dealing with environmental and ecological problems (Pin and Hutao, 2007). In contrast, the endogenous theory of growth, and in particular, the endogenous innovation in the theory of growth (Grossman and Helpman, 1994) states that “the best way to sustain the economic growth in the long run is by the improvements in technology by discovering ways to produce output while conserving those inputs that cannot be accumulated or regenerated.” Although the endogenous growth theory is not exempt from critics, for example, due to its operationality (Dinopoulos and Thompson, 1996), the CE could be a way to produce output while conserving inputs for at least a longer period and can be just one of many paths to be taken for the sustainable economic growth. The linkages between Industrial Ecology and CE have also been studied, concepts that although have different shades, should feed each other to enable and catalyze sustainable management of natural capital (Ghisellini et al., 2016; Saidani et al., 2020). In line with this idea, the European Commission remarks that the EU's economic prosperity and wellbeing are underpinned by its natural capital. Similarly, in 2021, the United Nations adopted a new statistical framework to complement the economic accounts with the natural capital accounts (Edens et al., 2022).
The positive aspect of CE is that the CBMs are considered enablers for CE implementation (Kirchherr et al., 2017). CBM is a class of generic sustainable business models (Bocken et al., 2013) suitable for CE application. The authors (Pieroni et al., 2019; Andreini et al., 2021) also looked into business model innovation (BMI), defined as “the design process for giving birth to a fairly new business model on the market, which is accompanied by an adjustment of the value proposition and/or the value constellation and aims at generation or securing sustainable competitive advantage,” for CE and sustainability. Both models still show constraints that are further discussed in the present study. Either CBM or BMI, tools to implement the CE to prompt a sustainable economic growth, should be accompanied by a circular supply chain management (CSCM) playing a crucial role in the transformation of a business model for the CE (Geissdoerfer et al., 2018).
Methods and data
This section is structured as follows: first, the EoL tuna net data collection is explained; second, empirical case studies are described, and finally, the selected methodology for the CBM application is defined.
EoL tuna net data collection
The estimation of the EoL tuna nets production by the tropical tuna purse seine fisheries focused on the Spanish tuna freezer companies operating in the Indian, Atlantic, and Pacific oceans. This study is focused on the Spanish tuna freezer sector because the need to valorize networks arose from the sector itself. Data for the estimation were collected by means of a survey process using a questionnaire (Appendix 1) where the companies were asked about the components (nets, thread, floating lines, eyebolts, etc.) of the fishing gears (quantitative and qualitative), the life span, and discarding frequency and fate of the nets (Zudaire et al., 2020). All Spanish companies were contacted, but not all of them responded at the time of writing this paper. The results of the questionnaire were extrapolated to the whole Spanish tuna freezer fishing fleet companies according to the number of vessels of each company.
Case studies
Three real implementation experiences or case studies (CS1, CS2, and CS3) were performed in collaboration with several stakeholders (ship owners, recyclers, textiles companies, fashion brands, and researchers) because the value creation processes for BMI involve actors across a wide variety of level spanning boundaries including not only organizational but also external actors (Andreini et al., 2021). Data were gathered during 2020–2021 to collect information on material flow, production costs, quality of the products, and logistic needs for the different value chain stages of the recovery and recycling of EoL tuna nets (Table 1). This information was then used to build the CBM of recycling EoL tuna nets of tropical tuna purse seine fleet that operated in the Indian Ocean.
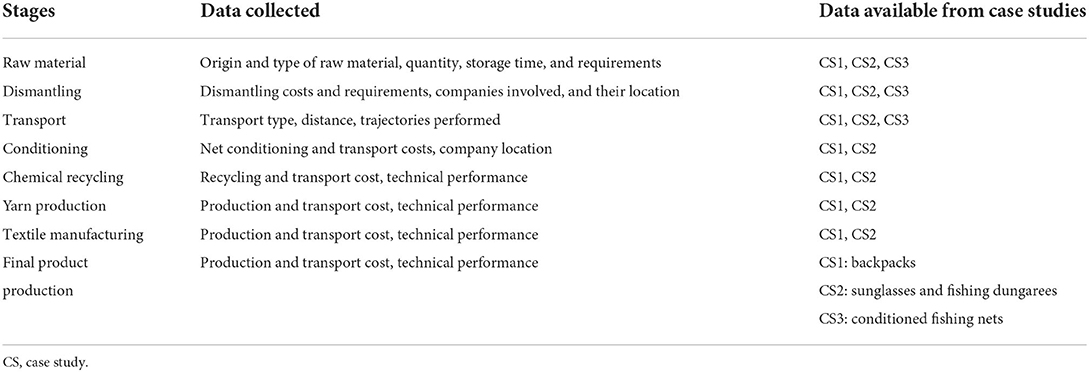
Table 1. Value chain stages of the EoL tuna net recycling, data collected from each stage, and data availability of each case study.
Not all the case studies went through the entire value chain. CS1 (involved 46 tons of EoL tuna nets) completed the entire value chain from the fishing nets collection, net conditioning for its transportation, transport, net dismantling for the recycling process, recycling process, yarn production, textile production, and final product for fishing sectors (backpacks and hats); CS2 (involved 2.5 tons of EoL tuna nets) developed same steps as CS1, but with different companies and different final products (dungarees for fishers and sunglasses); and CS3 (involved 32.6 tons of EoL tuna nets) stopped in the sale of conditioned fishing nets, and it was performed to verify some figures in dismantling process (Figure 2). The case studies (Figure 2) were only focused on the fleet operating in the Indian Ocean, whose nets have arrived at their end of life and are stored in Seychelles. Cost-related data are in relative terms based on a kilogram of recycled yarn. Costs in absolute terms are not presented due to confidentiality issues. The design of the case studies was performed together with the stakeholders (fishing companies, recyclers, textiles companies, and fashion brands) to cover all the stages. The CS2 and CS3 were developed to achieve data from different sources to calibrate data for each stage.
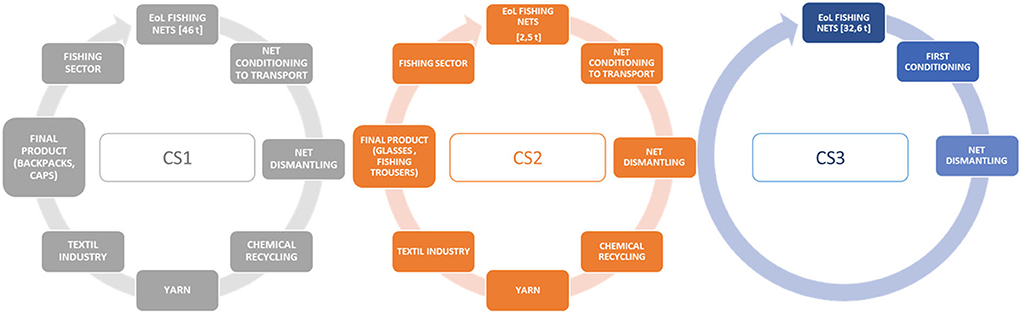
Figure 2. Flowchart of the three case studies (CS) that were focused on the chemical recycling of EoL tuna nets. Each colored rectangle represents the production stages achieved in each CS. The final stages of each CS are CS1: production of backpacks; CS2: production of glasses and fishing dungarees; CS3: selling fishing nets. CS1 and CS2 undertook the same production phases, but by different companies; in CS3, only the first three phases were analyzed.
Circular business model
Recycling EoL tuna nets is especially focused on the value creation process of the sustainable BMI processes (Andreini et al., 2021). EoL tuna nets of PA6 used to be stored in ports, and in the presented business model, this secondary raw material provides higher environmental and economic value (Geissdoerfer et al., 2018). This business model aims to create value from waste (Bocken et al., 2014). Thus, the suitability of EoL tuna net recycling was assessed by applying a CBM approach based on Manninen et al. (2018) and considering the beginning of life (BoL: fishing nets), middle of life (MoL: lifespan of fishing nets in fisheries), and EoL (recycling tuna nets) (Figure 3). The description of the CBM followed the approach of five steps:
STEP 1: Environmental value proposition (EVP) definition: In line with the EVP given by Manninen (Manninen et al., 2018), the EVP considered the environmental value improvement of the EoL tuna net recycling process compared to the use of virgin material.
STEP 2: Stakeholder identification: The relevant stakeholders of the CBM in the whole value chain were defined, and their roles were determined.
STEP 3: Reference system and assessment of environmental impacts definition: The reference system is currently in the market. This reference system is the unit by which the proposed circular model is compared to different stages of the value chain. Depending on the stage of the value chain, the reference system is related to the non-recycled pellets or substitutive products for recycled yarn.
STEP 4: EVP verification: In this step, the environmental benefits related to different stakeholders were identified based on Step 3 and compared with the EVP defined in Step 1. To introduce circularity to the EoL fishing gears, the recycling process was quantitatively assessed. The material flow and the costs of each process were assessed. The life cycle phases of the raw material (EoL tuna nets) acquisition, transportation, and transformation were identified and quantified. To estimate the environmental benefits of this CBM, the benefit of preventing the creation of marine litter thanks to the recycling process and the benefit due to the reduction of CO2 were considered. The functional unit for the assessment was 1 kg of yarn.
STEP 5: Identification of improvement proposals: Possible improvement measures were proposed for the value chain of the business model to meet the EVP.
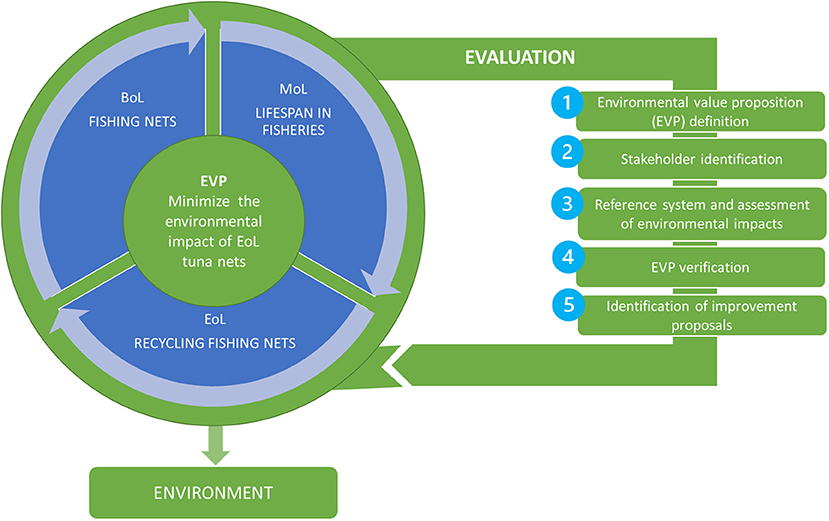
Figure 3. Environmental value proposition framework [adapted from Manninen et al., 2018]. The stages of the life cycle (BoL, beginning of life; MoL, middle of life; EoL, end of life) are in blue. The environmental value proposition (EVP) that impacts the environment is in green. This model is evaluated by the five steps defined on the right side of the figure.
Results
EoL tuna net data collection
At the time of this analysis in Spain were 10 tropical tuna purse seiner companies, all of them were surveyed, and five of them answered the questionnaire. The responses represented 63% of the Spanish tropical purse seiner vessels. According to the results, the weight of a tropical tuna purse seine net (Figure 4) is on average 93.2 tons with a standard deviation (SD) of ±16.2 tons. The net is made of nine components (Table 2). The netting, ropes, and flotation headlines are made of PA6, and the total mean weight of this material is around 64.2% of the total weight. The ethylene-vinyl acetate (EVA) floats are used in the upper part of the flotation headline and constitute 9.7% of the total mean net weight. The metallic components (chain, crowfoot, and ring bolts) are built of steel and correspond to 26.1% of the total mean weight of the whole fishing gear.
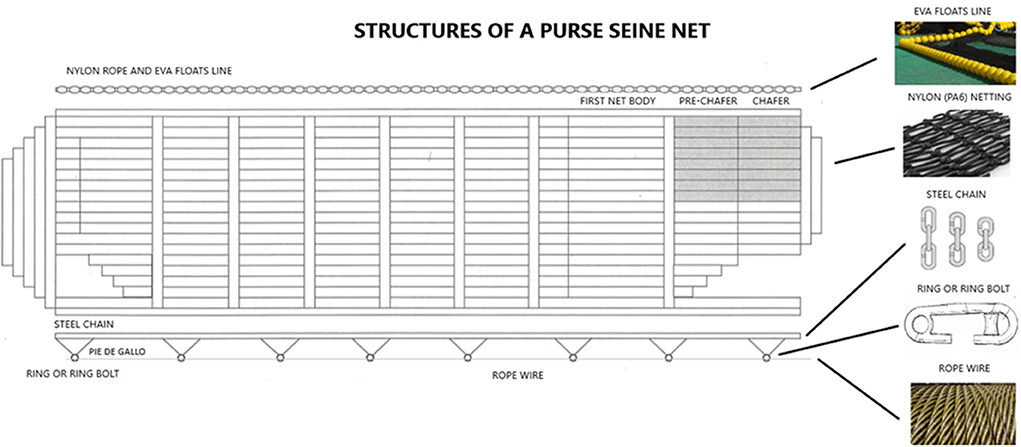
Figure 4. Tuna purse seine fishing net design including the different elements of which it is composed. On the right side of the figure, there are pictures of the main elements of the fishing gear.
The results showed high variability in the estimated life span of net components, with an average value of 3.2 and SD of ± 0.8 years for nets. According to the companies, one net is operative for at least 2 years, but it can also be used for up to 4 years. All components are susceptible to replacement or repair, which depends on the number of sets, life span, or observation of significant deterioration. For example, the netting is repaired in case of breakage and/or replaced after 12–14 months of use. These replaced net components are usually stored in Port of Victoria (Seychelles), Abidjan (Costa Marfil), Mindelo (Cabo Verde), and Posorja (Ecuador). According to the companies, some of the elements of the net (i.e., net, ropes, and chain) can be reused in new nets or as replacements and in the construction of dFAD. Despite these uses, significant amounts of nets can remain stored for years in these ports.
Case studies
The stages of the different case studies are described below, and Table 3 summarizes them.
STAGE 1. Raw material: The raw material considered for CS1, CS2, and CS3 was PA6 of the EoL tuna netting of the Spanish freezer tuna vessels. These EoL tuna nets were sold to recycling companies for 3.2–4.9% of the cost of one kg of recycled yarn. However, according to the interviewed stakeholders, not all the materials can be absorbed by this industry. Additionally, shipowners pay a fee (3.4–3.9% of the cost of 1 kg of recycled yarn) to rent the space for storing the fishing nets in port.
STAGE 2. Fishing net dismantling: Nets needed to be dismantled and then fitted into containers to be shipped to the conditioning plant. The dismantling was done by a local company (CS1) or by the tuna companies' own staff (CS2 and CS3). The cost of dismantling ranged from around 0.13–3.38% over the cost of 1 kg of recycled yarn.
STAGE 3. Secondary raw material transport: The transportation of containers from Seychelles to the dismantling company in Europe was done by ship for ocean transport and then by truck for road transport. In CS1, the nets were transported to a North European company, and in CS2, the nets were transported to a company located in the south of Europe. The transportation costs ranged from 2.6 to 5.1% over the cost of 1 kg of recycled yarn.
STAGE 4. Fishing net conditioning: In this process, the tuna nets were cleaned and prepared for its recycling. The conditioning process was undertaken by a North European company in CS1 and CS3. To contrast data obtained from this company, this stage was done with another company (South European) in the case study CS2. The estimated costs of net conditioning range between 2.5 and 9.9% over the total cost of 1 kg of recycled yarn. The CS3 traceability was finished at this stage.
STAGE 5. Net chemical recycling: Conditioned nets were chemically recycled. In CS1, the dismantling and recycling processes were done by North European companies, and the traceability of these processes was missing. In CS2, the net was recycled by a Portuguese company. The obtained recycled pellets were 100% made of EoL tuna nets. No additives were used in the process. The costs of chemical recycling ranged from 32.9 to 48.3% over the cost of 1 kg of recycled yarn. This cost included the transport from the dismantling company to the recycling company.
STAGE 6. Yarn production: In CS2, the recycled pellets were used for the yarn production, which was carried out in Spain. The cost of the yarn production phase was estimated from 27 to 51.5% over the total costs of recycled yarn.
STAGE 7. Textile manufacturing: The yarn was transported to the textile company located in Spain (CS1 and CS2) where the fabric was produced. The fabric production cost depends on the typology of the fabric. In CS2, the fabric was mixed with certain components such as elastomers to meet the requirements the final product had to present.
STAGE 8. Final products: The textile final products were backpacks and trucker caps (CS1) and dungarees for fishers (CS2), all produced by a Basque company. Additionally, in CS2, with the recycled pellet, sunglasses were produced in Italy.
Collected data and results were validated with stakeholders that are currently a part of this value chain, and who are coauthors of this contribution.
Circular bussines model
A CBM focuses on resources to check whether the model contributes to slowing, closing, or narrowing resource loops (Bocken et al., 2018). Using the EoL tuna nets instead of leaving them abandoned as a waste material means a circular economy solution (D'Amato and Korhonen, 2021), and accordingly, a circular business model. Figure 4 shows the scheme of the CBM for recycling EoL tuna nets.
STEP 1. Environmental value proposition (EVP) definition: The EVP is the result of three variables: first, the reduction of environmental impact caused by abandoned, lost, or otherwise discarded fishing gears (ALDFG) on the environment, especially if the recovering and recycling of EoL nets work as marine litter prevention mechanism; second, the reduction of petrol-based material extracted from the environment derived from recycling existing material; and third, the generation of a new business from the already existing and discarded raw material.
STEP 2. Stakeholder identification: For the recovery and recycling of EoL tuna nets, several are targeted as key stakeholders: (1) the public administration because the regulation is one of the main drivers for these types of business; (2) fishing companies as providers of raw material; (3) depending on the way nets are managed once they arrive to their EoL, the cost of the net recycling may vary; hence, net managers are also key for the cost efficiency of the process. For the transformation process, fishing net dismantling companies, recycling companies, textile companies and clothing or fashion companies have been identified and contacted. (4) Logistics and related stakeholders become pivotal, and several transport companies are involved along the whole value chain. Finally (5), research institutions also play a key role to improve the recycling process because currently chemical recycling of the PA6 EoL tuna nets is still costly and the price of the recycled PA6 pellet is still high compared to the virgin PA6 or other PA6 pellets from other recycled materials.
STEP 3. Reference system and assessment of environmental impact: In the case of raw material (PA6) and pellets, the reference system are the substitutive products existing in the market whose data are provided by the identified stakeholders. The reference system for the yarn was one that can be considered a substitutive product due to similar technical and market characteristics. Additionally, in the reference system, EoL tuna nets that are not collected, remain in the environment damaging it. In Figure 5, the values of the reference system compared to the CBM are represented.
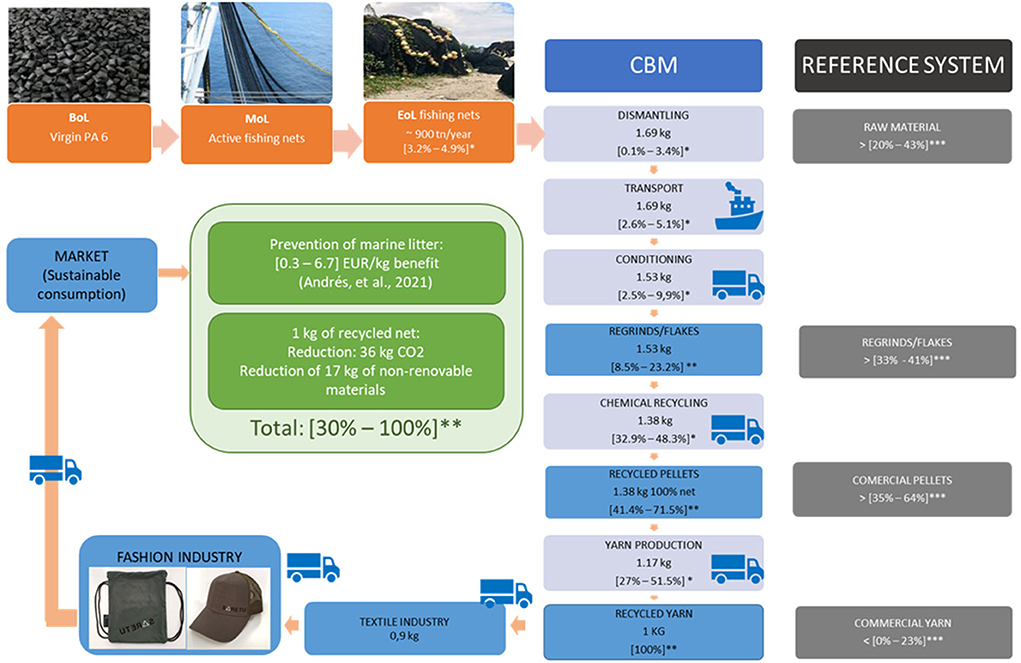
Figure 5. Value chain related to 1 kg of PA6 recycled yarn. Data [material flow in kilograms (kg) and costs in percentages] related to July 2021. The material flow is in orange, the CBM is in blue, the reference system is in gray, and the environmental value proposition of the CBM is in green. The subscript “*” represents the % of cost of the process (light blue) over the total costs of yarn production. Subscript “**” represents the total cost of production of intermediate products (dark blue) over the total costs of recycled yarn production. Subscript “***” represents the variation percentage of the CBM intermediate products compared to the products existing in the market (reference system).
STEP 4. Verification of the environmental value proposition (EVP): As Figure 5 shows, although the regrinds and pellets from virgin raw material (reference system) are 1.5 times less costly than pellets made of recycled material from EoL tuna nets, the recycled yarn can achieve a competitive value in the market. Additionally, the environmental benefit (15–140% over the market price of the recycled yarn) was estimated as a result of the recycling process.
STEP 5. Improvement proposals: Several improvement proposals were identified. First, the chemical recycling of the PA6 EoL tuna net was not optimal because difficulties were encountered during the yarn production. The recycling of nets needed to be improved, with one option being the use of additives to improve the viscosity of the materials during yarn production. Second, the EoL of the final products (textile final products) needed further assessment in order to establish the best use for this material at the end of its life because, for a more circular model, the final product needs to be done using a recycled material and it should be a recyclable material. For example, the recycled yarn used to manufacture the fabric of fisher dungarees had to be mixed with elastomer to improve the properties of the final product. This addition hampers the recycling of recycled fabric. Third, logistics and transportation are an issue in the tropical tuna purse seine nets recycling. The diverse locations of the agents involved in the value chain (Figure 6) from Seychelles to the dismantling plant (Lithuania or Spain), recycling plant (Turkey or Portugal), yarn production plant (Spain or Turkey), textile production (Spain), and finally the fashion company (Spain) can jeopardize the whole business model. Centralizing the processes would decrease the transport costs and CO2 emissions, improving the sustainability of the CBM. But for developing the whole value chain in just one region, the amount of recycling nets should be enough to justify the investment in the conditioning and recycling plants and facilities.
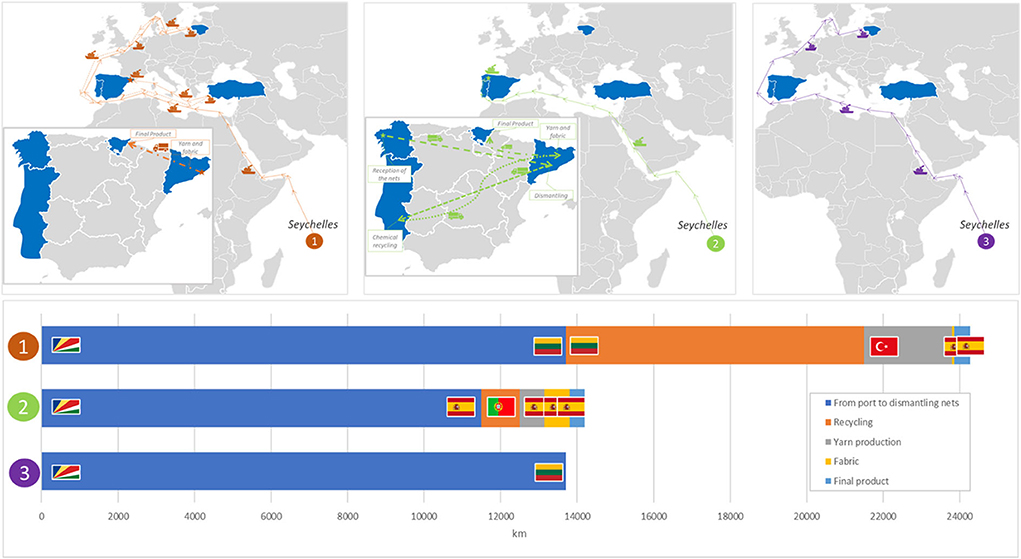
Figure 6. Transport required in the case studies to recycle EoL tuna nets, with a base port in Mahe, Seychelles. In the maps at the top of the figure the trajectories traveled by the EoL tuna nets from the country of origin to the final product processing are represented, and their transport methods (by vessel or by truck) are described with icons. The map in the top left (in orange) describes the CS1, the map in the top center (in green) represents the CS2, and the map in the top right (in purple) represents the CS3. In the graph below, the number of kilometers to reach the destination of each production phase for CS1, CS2, and CS3 is represented. The flag represents the country where the production phase indicated in the legend is performed.
Discussion
The recovery and revalorization of marine plastic within a CE are an attractive paradigm to increase global welfare while minimizing the environmental impacts of economic activities (Donati et al., 2020). This study has estimated, for the first time, the yearly contribution of Spanish tuna freezer purse seine companies to EoL fishing nets and assessed the viability of creating a CBM out of recycled tropical tuna purse seine EoL nets. This study allowed us to learn about the monetary and material flows, supply chains, stakeholders' perceptions, and the environmental impacts of upcycling polyamide nets. However, although the assessment and quantification of EoL tuna nets recycling CBM supported promising results, limitations in the business itself and in the methodology to assess the CBM have also been identified.
The first limitation when applying a CBM is to define the level of CE that needs to be analyzed. The high investment for recycling fishing nets should be addressed with a holistic perspective on a European-level basis by optimizing the location of the recycling plants according to the EoL fishing nets generation and logistic issues (i.e., macro level). This case study demonstrates the implementation of the circular economy of EoL tuna nets needs to implement the three levels of circular economy (Suárez-Eiroa et al., 2019): (i) micro level (recycling company), (ii) meso level (fisheries + recycling company + textile industries), and (iii) macro level (holistic perspective and European Regulation). While the CBM has been applied at the micro level, the meso and macro levels are affecting the CBM directly. Therefore, isolated CBM at the micro level should be accompanied by its related meso and macro CBMs. There is a need for innovative multi-level solutions (Madricardo et al., 2020), and how to relate these several CBMs at different levels needs further research.
Innovation seems to be a requirement along the whole value chain. EoL tuna nets are an important source of secondary raw material for its revalorization, even more, if this revalorization implies an environmental improvement. These nets are subjected to be recycled, although the process is more costly and requires adjustments in almost all stages of the process up to yarn production. This cost overrun can be balanced when high-value final products are produced (e.g., textile garments). The recycling of EoL tuna nets business cannot be understood without the BMI process. The processes of BMI (Andreini et al., 2021) linked to the recycling of EoL tuna nets are: (i) cognition processes of the stakeholder linked to the whole value chain, from fisher to textile and final consumers, developing a strategic sensitivity; (ii) understanding of the new way to produce and consume yarn in the textile industry considering the new technologies and innovations related to nets recycling; (iii) knowledge-shaping processes looking for a more sustainable solution for the EoL tuna nets; and (iv) value creation process with stakeholders' cooperation. The assessment and quantification of CBM shed light on the innovation requirement along the value chain. CE cannot, therefore, be understood without applying the BMI processes (Andreini et al., 2021).
Clothing production is associated with myriad environmental damages (Menke et al., 2021), and more sustainable production is required to minimize environmental impacts. The reduction of clothing consumption can be a part of the solution, but there could be attitudinal obstacles regarding clothing consumption (Kleinhückelkotten and Neitzke, 2019; Roba et al., 2021). Thus, sustainable clothes are the meanwhile solution. Circular supply chains contribute holistically to sustainable development, and they also have immediate effects in the ecological dimension that then spill over into the economic and social dimensions (Montag et al., 2021). Therefore, CE has emerged as a potential strategy for developing business practices based on sustainability concerns, especially in the fashion industry (Binet et al., 2021; Ostermann et al., 2021). Recycling fishing nets can also allow advancing in this direction and address both problems, the fashion industry and the marine litter driving the economy toward a sustainable growth.
The textile and fashion industries have adopted different strategies to make the use of marine plastic profitable. Consequently, the percentage of recycled marine plastic added to the final textile products is diverse. The presence of recycled marine litter products is increasing on the market, but there are some doubts about consumers' understanding of sustainability in the clothing industry. It is not the same as a fabric done with monofilament 100% recycled materials or with a lower percentage of recycled material. This sometimes generates confusing messages among consumers. The chemical recycling of mixed plastic waste has a 50% lower climate change impact and life cycle energy user than the energy recovery option, but chemical recycling has other higher impacts than mechanical recycling (Jeswani et al., 2021). Obtaining PA6 from EoL tuna nets seems to be valid for mechanical recycling, as they can be processed for different potential industrial applications without any remarkable loss of main properties (Mondragon et al., 2020). However, the experiences developed in this study showed chemical recycling of EoL tuna nets is more appropriate for the objective of the specific clothing design.
The production costs of the first stages of the value chain (raw material, regrinds/flakes, and pellet) could not be as competitive as the reference system, unless the regulatory framework will drive this kind of CBMs or demand change. Additionally, in terms of costs, the environmental benefits of recycled pellets could also offset the over-cost in comparison to the virgin material. In this sense, the conceptual framework of CBM has been widely studied (Geissdoerfer et al., 2020), but the quantification and comparison of the costs and benefits at economic, social, and environmental levels have not been still addressed. There is a need to create multidimensional indicators to measure the circularity of a business model in its totality, addressing all the components, including economic, social, and environmental dimensions (Rossi et al., 2020; Boldrini and Antheaume, 2021; Walzberg et al., 2021). The circularity needs to be assessed not only considering the whole life of the fishing nets: from the BoL, i.e. its design and construction, to the EoL, i.e. final products such as fisher trousers. But also the life cycle of the final products need to be assessed to determine the circularity. In line with this, textile recycling is also perceived as one of the key directions needed for a sustainable transition of the sector (Leal Filho et al., 2019), facilitating the manufacturing of recyclable textiles. In this paper, only a window of the CBM has been assessed, from the EoL tuna nets to the final product made by recycled nets. Nonetheless, the ‘real circularity will be assessed only by analysing the successive transformations of the raw material, that is, assessing the primary, secondary, tertiary and so on transformations of the raw materials. Considering the second law of thermodynamics, entropy (Georgescu-Roegen, 1971), every circular transformation or process should be analyzed for its (global) net environmental sustainability contribution (Korhonen et al., 2018a). As the material is neither created nor destroyed, it is only transformed, the circularity may be infinite, which makes difficult the assessment of the global net environmental contribution. The cyclic flow should be assessed in each loop. These loops should be supported only when they are socially desirable and eficient (Suárez-Eiroa et al., 2019), along all consecutive loops, because this is precisely the essence of the CE, the law of thermodynamics (Ghisellini et al., 2016). While perpetual loops may be desirable, there are several limitations (D'Amato and Korhonen, 2021). According to the entropy law, it seems unlikely that a fully circular economic system exists with product and energy turning back to raw material forever (Daly, 1977). Therefore, the CBM should be able to capture the value proposition of all consecutive loops, which could be a complex issue when the reuse of the final product done by recycled pellet has not been assessed, and so on. Existing models are not able to assess quantitatively the whole process of circularity, and this lack could entail biased estimation of the CBM outcomes. In this sense, further research is needed for addressing adequately (Nußholz, 2018).
Another important issue detected in the case studies is the large number of kilometers that the material travels from the collection to the final product is achieved. An alternative logistics chain to accommodate abandoned, lost, or otherwise discarded fishing gear recycling would improve the costs and environmental impacts (van Giezen and Wiegmans, 2020), optimizing the location of the different stages at the meso level of the CE. From a simplified point of view, these business models depend on oil (natural resource) which is found in its different transformation products in the whole cycle, from fuel to transformed product. Furthermore, in a globalized economic system such as the one we have, kilometers are equally important and costly regardless of the business model, so the option to make them more local (micro or meso level) is important to minimize environmental impacts.
Regarding the environmental impacts, measuring the environmental value proposition (Das et al., 2022) is difficult in any CBM, but this study has taken one step forward by analyzing not only the reduction of CO2 emission but also the benefit of marine litter prevention. However, those are only a part of the ecosystem services that the environment can provide. Here arises one of the limitations of CBM when quantifying the environmental aspect of the value proposition. It is true that progress is currently being made in this direction in developing a Valuation for Natural Capital and Ecosystem Accounting (Badura et al., 2017), and this methodology should be coupled with the CBMs for the correct environmental value proposition evaluation.
The EoL tuna net CBM sustainability trade-off has been quantified using both, economic and environmental indicators. Regarding the social indicators, there is still a need for further research because in this study the social indicators were not addressed, and indeed social aspects were mentioned only in a third of CBM case studies in the literature (Dijkstra et al., 2020). How to quantify the social dimension of the CBM is another issue to be addressed in the future for a complete evaluation of the sustainability of the model. Note that differences between sustainability and CE were identified (Geissdoerfer et al., 2017), as CE prioritizes financial advantages for companies and less resource consumption and pollution for the environment. But monetarizing the environmental impacts, i.e., valuation of ecosystem services and its associated social welfare, may allow these dimensions into the model affecting directly to the financial performance of the business. In any case, CBM currently considers the three dimensions of sustainability, although the way of quantifying the impact on each dimension is still lacking general agreement. Thus, recycling fishing nets and textile garments seem to be a circular solution. But in the case of a textile garment recycling, it can lead to wrong incentives, because if a company or the society is able to recycle the textile products, consumers may be not interested in reducing the amount of waste (Gwehenberger et al., 2003). To mitigate trade-offs between raw material needs and other ecosystem services, solutions envisioned should include sustainable management practices (D'Amato and Korhonen, 2021), that need to be designed along the entire value chain.
There are more barriers identified when applying CBMs: high investment, complexity of the system, low consumer awareness or inherent irrationality of consumer behavior (Planing, 2015), lock-in supply chain agents, technological bottlenecks, reluctance within the organizations, and sustainability trade-offs were also identified as barriers of CBMs (Bishop et al., 2020). In this study, the pilot projects and the involvement of the stakeholders shed light on those barriers. From the third dimension of sustainability (society), consumer awareness seems to be already perceived by the fashion brands that are increasingly using recycled raw materials (Khandual and Pradhan, 2019). The supply chain is guaranteed due to the amount of EoL tuna nets generated by the Spanish tuna freezer fleet that has been quantified for the first time in this study. Regarding the technological bottlenecks, this study proved that the recycling process is possible, although some improvement in cleaning and sorting is needed (Vollmer et al., 2020). Regarding the reluctance within the organization, the regulation in force is driving this issue. BMI seems to be the process to follow for the CE.
In addition, currently, the profitability of the business presented in this study depends directly on the supply and demand of oil and the price that is imposed on it (Moutinho et al., 2017; Ghosh et al., 2021; Guo et al., 2021). There may be times when products derived directly from oil are cheaper, making the recycling processes unprofitable, as an example of what has happened because of the global pandemic of COVID. However, in the event of a variation in the price of oil, changes in the price of intermediate products could be estimated, making the price of the final product derived from oil and recycled products competitively priced. That is why it is important to focus on the environmental impact that both processes generate. Among these impacts, transportation could be assumed to be quite similar in both, deducing that the process dependent on natural resources has a greater impact than the recycling processes.
As a final remark, we can conclude that circular strategies are one option among others for sustainable economic growth (Geissdoerfer et al., 2017). Recycling EoL tuna nets seems to be a suitable strategy, although still need an efficiency improvements along the whole value chain. These improvements would have to be driven by BMI processes. But recycled materials for the fashion industry may lead to wrong incentives. This industry could be advocated for creative destruction (Scafidi, 2020) following the Schumpeterian theory. The way of production and consumption of clothes in first world countries is unsustainable. Thus a novel and most sustainable business model, driven by technological innovation, is required to substitute the current model. The innovation in the bussiness model will be a keystone to achieve sustainable fashion industry succeed commercially (Teece, 2010).
Conclusion
The CE is a suitable framework for the solution of EoL tuna nets. The valorization of EoL fishing nets involves not only a prevention measure for the marine litter but also a solution, at least a temporal solution, for the fashion industry. The empirical CBM was developed and quantified, production costs of each stage of the value chain and material flow, to recycle the EoL tuna nets into textile products. The Spanish tuna freezer companies yearly produce ~900 tons of tuna nets, and this waste material can transform into a secondary raw material for the textile industry. The production costs of the first stages of the value chain (raw material, regrind/flakes, and pellets) are higher than the reference system (which are those substitutive products in the market), but the cost of yarn may be competitive compared to the substitutive products. Even more so, if we consider that at the time of writing this paper the market price of polyamide is reaching its historical maximum. Logistics issues should be improved to build a more sustainable business model; thus, a local CBM seems to be an improvement in sustainable terms.
However, the CBM tool to evaluate the EoL tuna nets recycling leads to several limitations. First, there is a lack of methodologies to relate or integrate the three levels of the CE when designing the CBM. Although the micro level seems to be the most direct application, the meso and macro levels are implicitly involved in the micro level, and in a CBM, the micro level is difficult to be evaluated without considering the rest of the levels of the CE. Second, measuring the three levels of sustainability is not an easy issue since there are no explicit indicators for all dimensions; although there are advances in quantifying the natural capital, the application of CBM could be very case-specific making it more difficult for the measurement of the environmental dimension. Third, the EoL tuna net recycling for the textile industry can lead to wrong incentives for the fashion industry because society may not be interested in reducing the amount of waste. Thus, the CBM should be considered the consecutive loops of the material and business models to address real sustainability.
The CE paradigm could contribute to sustainable economic growth or at least can slow down the depletion of natural capital. Although this paradigm has the CBM as an enabler, social and environmental dimensions need to be further addressed to be implemented in the consecutive loops of the CBMs. If not, it will be difficult to assert that a CBM contributes to sustainable economic growth in the long term.
Data availability statement
The original contributions presented in the study are included in the article/Supplementary material, further inquiries can be directed to the corresponding author/s.
Ethics statement
Ethics review and approval/written informed consent was not required as per local legislation and institutional requirements.
Author contributions
MA, IZ, OB, and JL conceived of the presented idea. MA developed the theoretical formalism, performed the analytic calculations, and performed the numerical analysis. MA, OB, JL, and IZ wrote the manuscript with the support of AA and NG. MM, EU, NG, and AA provided data and revised the manuscript. All authors provided critical feedback and helped shape the research, analysis, and manuscript. All authors contributed to the article and approved the submitted version.
Funding
This research has been funded by the Basque Government through the EMFF funds (project SAREBIO, contract no 00011-IRB2019-33).
Acknowledgments
We would like to express our sincere gratitude to fishing companies and collaborators who have assisted in the data collection and the recycling process. We also would like to thank the reviewers for all their valuable comments and suggestions, which helped us to improve the quality of the manuscript. This paper is contribution no 1111 of AZTI, Marine Research, Basque Research and Technology Alliance (BRTA).
Conflict of interest
Authors MM and NG was employed by Antex and Edu Uribesalgo by Ternua Group.
The remaining authors declare that the research was conducted in the absence of any commercial or financial relationships that could be construed as a potential conflict of interest.
Publisher's note
All claims expressed in this article are solely those of the authors and do not necessarily represent those of their affiliated organizations, or those of the publisher, the editors and the reviewers. Any product that may be evaluated in this article, or claim that may be made by its manufacturer, is not guaranteed or endorsed by the publisher.
Supplementary material
The Supplementary Material for this article can be found online at: https://www.frontiersin.org/articles/10.3389/frsus.2022.929902/full#supplementary-material
References
Andreini, D., Bettinelli, C., Foss, N. J., and Mismetti, M. (2021). Business model innovation: a review of the process-based literature. J. Manag. Govern. 1–33. doi: 10.1007/s10997-021-09590-w
Androniceanu, A., Kinnunen, J., and Georgescu, I. (2021). Circular economy as a strategic option to promote sustainable economic growth and effective human development. J. Int. Stud. 14, 60–73. doi: 10.14254/2071-8330.2021/14-1/4
Arandes, J. M., Ereña, J., Azkoiti, M. J., López-Valerio, D., and Bilbao, J. (2004). Valorization by thermal cracking over silica of polyolefins dissolved in LCO. Fuel Process. Technol. 85, 125–140. doi: 10.1016/S0378-3820(03)00110-3
Argüello, G. (2020). Environmentally sound management of ship wastes: challenges and opportunities for European ports. J. Shipp. Trade 5, 12. doi: 10.1186/s41072-020-00068-w
Badura, T., Ferrini, S., Agarwala, M., and Turner, K. (2017). Valuation for Natural Capital and Ecosystem Accounting. Synthesis report for the European Commission. Centre for Social and Economic Research on the Global Environment, University of East Anglia. Norwich. Available online at: https://ec.europa.eu/environment/nature/capital_accounting/pdf/Valuation_for_natural_capital_and_ecosystem_acounting.pdf (accessed July 10, 2022).
Basurko, O. C., Markalain, G., Mateo, M., Peña, C., Mondragon, G., Larruskain, A., et al. (2022). End-of-life fishing gears in Spain: quantity and recyclability. Environ. Pollut.
Bertelsen, I. M. G., Ottosen, L. M, and Fischer, G. (2020). Influence of fibre characteristics on plastic shrinkage cracking in cement-based materials: A review. Construct. Build. Mater. 230, 116769 doi: 10.1016/j.conbuildmat.2019.116769
Bimpizas-Pinis, M., Bozhinovska, E., Genovese, A., Lowe, B., Pansera, M., Alberich, J. P., et al. (2021). Is efficiency enough for circular economy? Resour. Conserv. Recycl. 167, 105399. doi: 10.1016/j.resconrec.2021.105399
Binet, F., Saunier, F., and Margni, M. (2021). Assessing the mitigation potential of environmental impacts from circular economy strategies on an industrial sector and its value chain: a case study on the steel value chain in Quebec. Front. Sustain. 2, 738890. doi: 10.3389/frsus.2021.738890
Bishop, G., Styles, D., and Lens, P. N. L. (2020). Recycling of European plastic is a pathway for plastic debris in the ocean. Environ. Int. 142, 105893. doi: 10.1016/j.envint.2020.105893
Bocken, N., Schuit, C., and Kraaijenhagen, C. (2018). Experimenting with a circular business model: Lessons from eight cases. Environ. Innovat. Soc. Transit. 28, 79–95. doi: 10.1016/j.eist.2018.02.001
Bocken, N., Short, S., Rana, P., and Evans, S. (2013). A value mapping tool for sustainable business modelling. Corp. Govern. 13, 482–497. doi: 10.1108/CG-06-2013-0078
Bocken, N., Strupeit, L., Whalen, K., and Nußholz, J. (2019). A review and evaluation of circular business model innovation tools. Sustainability 11:2210. doi: 10.3390/su11082210
Bocken, N. M. P., Short, S. W., Rana, P., and Evans, S. (2014). A literature and practice review to develop sustainable business model archetypes. J. Clean. Prod. 65, 42–56. doi: 10.1016/j.jclepro.2013.11.039
Boldrini, J.-C., and Antheaume, N. (2021). Designing and testing a new sustainable business model tool for multi-actor, multi-level, circular, and collaborative contexts. J. Clean. Prod. 309, 127209. doi: 10.1016/j.jclepro.2021.127209
Boulding, K. (1966). “The economics of the coming spaceship earth,” in Environmental Quality in a Growing Economy, Resources for the Future, ed H. Jarrett (Baltimore, MD: Johns Hopkins University Press), 3–14.
Brodbeck, L. (2016). Mechanisms to Support the Recycling/Reuse of Fishing Gear and the Prevention of Gear Becoming Lost/Abandoned at Sea - Barrier assessment. Circular ocean, Report Type 10-2016. Trondheim, 43. Available online at: http://www.circularocean.eu/wp-content/uploads/2017/09/Barrier-assessment_FINAL.pdf (accessed July 5, 2022).
Busu, M., and Trica, C. L. (2019). Sustainability of circular economy indicators and their impact on economic growth of the European Union. Sustainability 11. doi: 10.3390/su11195481
Centobelli, P., Cerchione, R., Chiaroni, D., Del Vecchio, P., and Urbinati, A. (2020). Designing business models in circular economy: a systematic literature review and research agenda. Bus. Strat. Environ. 29, 1734–1749. doi: 10.1002/bse.2466
Corvellec, H., Stowell, A. F., and Johansson, N. (2022). Critiques of the circular economy. J. Ind. Ecol. 26, 421–432. doi: 10.1111/jiec.13187
Daly, H. E. (1977). The Steady-state Economy. The Sustainable Society: Implications for Limited Growth. New York, NY; London: Praeger, 107e114. Available online at: http://www.amalthys.com/greenpath/019steadystate.html (accessed March 03, 2015).
D'Amato, D., and Korhonen, J. (2021). Integrating the green economy, circular economy and bioeconomy in a strategic sustainability framework. Ecol. Econ. 188, 107143. doi: 10.1016/j.ecolecon.2021.107143
Das, A., Konietzko, J., and Bocken, N. (2022). How do companies measure and forecast environmental impacts when experimenting with circular business models? Sustain. Prod. Consumpt. 29, 273–285. doi: 10.1016/j.spc.2021.10.009
de Kwant, C., Rahi, A. B. M. F., and Laurenti, R. (2021). The role of product design in circular business models: An analysis of challenges and opportunities for electric vehicles and white goods. Sustain. Prod. Consumpt. 27, 1728–1742. doi: 10.1016/j.spc.2021.03.030
Deshpande, P. C., Skaar, C., Bratteb,ø, H., and Fet, A. M. (2020). Multi-criteria decision analysis (MCDA) method for assessing the sustainability of end-of-life alternatives for waste plastics: A case study of Norway. Sci. Total Environ. 719, 137353. doi: 10.1016/j.scitotenv.2020.137353
Dijkstra, H., van Beukering, P., and Brouwer, R. (2020). Business models and sustainable plastic management: a systematic review of the literature. J. Clean. Prod. 258, 120967. doi: 10.1016/j.jclepro.2020.120967
Dijkstra, H., van Beukering, P., and Brouwer, R. (2021). In the business of dirty oceans: overview of startups and entrepreneurs managing marine plastic. Mar. Pollut. Bull. 162, 111880. doi: 10.1016/j.marpolbul.2020.111880
Dinopoulos, E., and Thompson, P. (1996). A contribution to the empirics of endogenous growth. East. Econ. J. 22, 389–400.
Donaghy, K. P. (2022). A circular economy model of economic growth with circular and cumulative causation and trade. Netw. Spat. Econ. 1–28. doi: 10.1007/s11067-022-09559-8
Donati, F., Aguilar-Hernandez, G. A., Sigüenza-Sánchez, C. P., de Koning, A., Rodrigues, J. F. D., and Tukker, A. (2020). Modeling the circular economy in environmentally extended input-output tables: Methods, software and case study. Resour. Conserv. Recycling 152, 104508. doi: 10.1016/j.resconrec.2019.104508
Edens, B., Maes, J., Hein, L., Obst, C., Siikamaki, J., Schenau, S., et al. (2022). Establishing the SEEA ecosystem accounting as a global standard. Ecosyst. Serv. 54, 101413. doi: 10.1016/j.ecoser.2022.101413
European Commission (2021). Plastics in a Circular Economy. Available online at: https://ec.europa.eu/info/research-and-innovation/research-area/environment/circular-economy/plastics-circular-economy_en (accessed April 20, 2022).
Feary, D., Aranda, M., Russell, J., Cabezas, O., Rodríguez Climent, S., and Bremner, J. (2020). Study on Circular Design of the Fishing Gear for Reduction of Environmental Impacts. Brussels: European Commision. Available online at: https://data.europa.eu/doi/10.2826/548271 (accessed April 20, 2022).
Foschi, E., and Bonoli, A. (2019). The commitment of packaging industry in the framework of the European strategy for plastics in a circular economy. Administr. Sci. 9. doi: 10.3390/admsci9010018
Gao, C., Gao, C., Song, K., and Fang, K. (2020). Pathways towards regional circular economy evaluated using material flow analysis and system dynamics. Resour. Conserv. Recycling 154, 104527. doi: 10.1016/j.resconrec.2019.104527
Garrido, J., Sáez, J., Armesto, J. I., Espada, A. M., Silva, D., Goikoetxea, J., et al. (2020). 3D printing as an enabling technology to implement maritime plastic circular economy. Proc. Manuf. 51, 635–641. doi: 10.1016/j.promfg.2020.10.089
Geissdoerfer, M., Morioka, S. N., de Carvalho, M. M., and Evans, S. (2018). Business models and supply chains for the circular economy. J. Clean. Prod. 190, 712–721. doi: 10.1016/j.jclepro.2018.04.159
Geissdoerfer, M., Pieroni, M. P. P., Pigosso, D. C. A., and Soufani, K. (2020). Circular business models: a review. J. Clean. Prod. 277, 123741. doi: 10.1016/j.jclepro.2020.123741
Geissdoerfer, M., Savaget, P., Bocken, N. M. P., and Hultink, E. J. (2017). The circular economy – a new sustainability paradigm? J. Clean. Prod. 143, 757–768. doi: 10.1016/j.jclepro.2016.12.048
George, D. A. R., Lin, B. C.-A., and Chen, Y. (2015). A circular economy model of economic growth. Environ. Modell. Softw. 73, 60–63. doi: 10.1016/j.envsoft.2015.06.014
Georgescu-Roegen, N. (1971). The Entropy Law and the Economic Process. Cambridge, MA: Harvard University Press.
Ghisellini, P., Cialani, C., and Ulgiati, S. (2016). A review on circular economy: the expected transition to a balanced interplay of environmental and economic systems. J. Clean. Prod. 114, 11–32. doi: 10.1016/j.jclepro.2015.09.007
Ghosh, T., Sahu, S., and Chattopadhyay, S. (2021). “Inflation expectations of households in India: role of oil prices, economic policy uncertainty, and spillover of global financial uncertainty.” Bull. Econ. Res. 73, 230–251. doi: 10.1111/boer.12244
Grossman, G. M., and Helpman, E. (1994). Endogenous innovation in the theory of growth. J. Econ. Perspect. 8, 23–44. doi: 10.1257/jep.8.1.23
Guldmann, E., and Huulgaard, R. D. (2020). Barriers to circular business model innovation: a multiple-case study. J. Clean. Prod. 243, 118160. doi: 10.1016/j.jclepro.2019.118160
Guo, Z., Long, B., Gao, S., Luo, J., Wang, L., Huang, X., et al. (2021). Carbon nanofiber based superhydrophobic foam composite for high performance oil/water separation. J. Hazard. Mater. 402, 123838. doi: 10.1016/j.jhazmat.2020.123838
Gwehenberger, G., Erler, B., and Schnitzer, H. (2003). “In a multi e strategy approach to zero emissions,” in Article Presented at Technology Foresight Summit, Budapest 27e29 March 2003. United Nations Industrial Development Organization. Available online at: http://www.unido.org/ (accessed April 20, 2022).
ICCAT (2006–2016). ICCAT Manual. International Commission for the Conservation of Atlantic Tuna. ICCAT Publications.
IOTC-2021-WGFAD02-INF02 (2021). Resolution 19/02 Procedures on a Fish Aggregating Devices (FADs) Management Plan. Information papers. IOTC ad hoc Working Group on FADs (WGFAD).
Jeswani, H., Krüger, C., Russ, M., Horlacher, M., Antony, F., Hann, S., et al. (2021). Life cycle environmental impacts of chemical recycling via pyrolysis of mixed plastic waste in comparison with mechanical recycling and energy recovery. Sci. Total Environ. 769, 144483. doi: 10.1016/j.scitotenv.2020.144483
Joyce, A., and Paquin, R. L. (2016). The triple layered business model canvas: a tool to design more sustainable business models. J. Clean. Prod. 135, 1474–1486. doi: 10.1016/j.jclepro.2016.06.067
Kamimura, A., Shiramatsu, Y., and Kawamoto, T. (2019). Depolymerization of polyamide 6 in hydrophilic ionic liquids. Green Energy Environ. 4, 166–170. doi: 10.1016/j.gee.2019.01.002
Khandual, A., and Pradhan, S. (2019). “Fashion brands and consumers approach towards sustainable fashion,” in Fast Fashion, Fashion Brands and Sustainable Consumption, ed S.S. Muthu (Singapore: Springer Singapore), 37–54.
Kirchherr, J., Reike, D., and Hekkert, M. (2017). Conceptualizing the circular economy: an analysis of 114 definitions. Resour. Conserv. Recycling 127, 221–232. doi: 10.2139/ssrn.3037579
Kleinhückelkotten, S., and Neitzke, H. P. (2019). Social acceptability of more sustainable alternatives in clothing consumption. Sustainability 11:6194. doi: 10.3390/su11226194
Klun, U., and Kržan, A. (2000). Rapid microwave induced depolymerization of polyamide-6. Polymer. 41, 4361–4365. doi: 10.1016/S0032-3861(99)00658-8
Korhonen, J., Honkasalo, A., and Seppälä, J. (2018a). Circular economy: the concept and its limitations. Ecol. Econ. 143, 37–46. doi: 10.1016/j.ecolecon.2017.06.041
Korhonen, J., Nuur, C., Feldmann, A., and Birkie, S. E. (2018b). Circular economy as an essentially contested concept. J. Clean. Prod. 175, 544–552. doi: 10.1016/j.jclepro.2017.12.111
Kornafel, M., and Telega, I. (2020). Dynamics of natural capital in neoclassical growth model. Int. J. Sustain. Econ. 12, 1–24. doi: 10.1504/IJSE.2020.107862
Leal Filho, W., Ellams, D., Han, S., Tyler, D., Boiten, V. J., Paço, A., et al. (2019). A review of the socio-economic advantages of textile recycling. J. Clean. Prod. 218, 10–20. doi: 10.1016/j.jclepro.2019.01.210
Liu, J., Wu, P., Jiang, Y., and Wang, X. (2021). Explore potential barriers of applying circular economy in construction and demolition waste recycling. J. Clean. Prod. 326, 129400. doi: 10.1016/j.jclepro.2021.129400
Lüdeke-Freund, F., Gold, S., and Bocken, N. M. P. (2019). A review and typology of circular economy business model patterns. J. Ind. Ecol. 23, 36–61. doi: 10.1111/jiec.12763
Luo, Y., and Deng, K. (2021). Sustainable fashion innovation design for marine litter. J. Phys. Conf. Ser. 1790, 012098. doi: 10.1088/1742-6596/1790/1/012098
Madricardo, F., Ghezzo, M., Nesto, N., Mc Kiver, W. J., Faussone, G. C., Fiorin, R., et al. (2020). How to deal with seafloor marine litter: an overview of the state-of-the-art and future perspectives. Front. Mar. Sci. 7, 505134. doi: 10.3389/fmars.2020.505134
Manninen, K., Koskela, S., Antikainen, R., Bocken, N., Dahlbo, H., and Aminoff, A. (2018). Do circular economy business models capture intended environmental value propositions? J. Clean. Prod. 171, 413–422. doi: 10.1016/j.jclepro.2017.10.003
Meadows, D. H., Meadows, D. L., Randers, J., and Behrens, W. W. (1972). The Limits To Growth: a Report for the Club of Rome's Project on the Predicament of Mankind. New York, NY: Universe Books, 205.
Menke, C., Hüsemann, M., and Siems, E. (2021). Stakeholder influence on sustainable supply chain management: a case study of a German apparel frontrunner. Front. Sustain. 2, 735123. doi: 10.3389/frsus.2021.735123
Mondragon, G., Kortaberria, G., Mendiburu, E., González, N., Arbelaiz, A., and Peña-Rodriguez, C. (2020). Thermomechanical recycling of polyamide 6 from fishing nets waste. J. Appl. Polym. Sci. 137, 48442. doi: 10.1002/app.48442
Montag, L., Klünder, T., and Steven, M. (2021). Paving the way for circular supply chains: conceptualization of a circular supply chain maturity framework. Front. Sustain. 2, 781978. doi: 10.3389/frsus.2021.781978
Moutinho, V., Bento, J. P. C., and Hajko, V. (2017). Price relationships between crude oil and transport fuels in the European Union before and after the 2008 financial crisis. Util. Policy 45, 76–83. doi: 10.1016/j.jup.2017.02.003
Núñez-Cacho, P., Molina-Moreno, V., Corpas-Iglesias, F. A., and Cortés-García, F. J. (2018). Family businesses transitioning to a circular economy model: the case of “mercadona”. Sustainability 10, 538. doi: 10.3390/su10020538
Nußholz, J. L. K. (2017). Circular business models: defining a concept and framing an emerging research field. Sustainability 9, 1810. doi: 10.3390/su9101810
Nußholz, J. L. K. (2018). A circular business model mapping tool for creating value from prolonged product lifetime and closed material loops. J. Clean. Prod. 197, 185–194. doi: 10.1016/j.jclepro.2018.06.112
Ostermann, C. M., Nascimento, L. D. S., and Zen, A. C. (2021). Business model innovation for circular economy in fashion industry: a startups' perspective. Front. Sustain. 2, 766614. doi: 10.3389/frsus.2021.766614
Osterwalder, A., and Pigneur, Y. (2010). Business Model Generation: A Handbook for Visionaries, Game Changers, and Challengers, Vol. 1. John Wiley & Sons.
Pearce, D. W., and Turner, R. K. (1989). Economics of Natural Resources and the Environment. London: Hemel Hempstead, Harvester Wheatsheaf.
Peña-Rodriguez, C., Mondragon, G., Mendoza, A., Mendiburu-Valor, E., Eceiza, A., and Kortaberria, G. (2021). “Recycling of marine plastic debris”. in Recent Developments in Plastic Recycling. Composites Science and Technology, eds J. Parameswaranpillai, S. Mavinkere Rangappa, A. Gulihonnehalli Rajkumar, S. Siengchin (Singapore: Springer).
Pieroni, M. P. P., McAloone, T. C., and Pigosso, D. C. A. (2019). Business model innovation for circular economy and sustainability: a review of approaches. J. Clean. Prod. 215, 198–216. doi: 10.1016/j.jclepro.2019.01.036
Pieroni, M. P. P., Pigosso, D. C. A., and McAloone, T. C. (2018). Sustainable qualifying criteria for designing circular business models. Proc. CIRP 69, 799–804. doi: 10.1016/j.procir.2017.11.014
Pin, X., and Hutao, Y. (2007). Re-reading steady-state economy: calm thinking on hot circular economy. China Popul Resour. Environ. 17, 20–23. doi: 10.1016/S1872-583X(07)60012-6
Planing, P. (2015). Business model innovation in a circular economy reasons for non-acceptance of circular business models. Open J. Bus. Model Innovat. 1, 1–11. Available online at: https://mycourses.aalto.fi/pluginfile.php/1187882/mod_label/intro/Planing_2015_Business%20Model%20Innovation%20in%20a%20Circular%20Economy.pdf
Ramos, A., Berzosa, J., Clarens, F., Marin, M., and Rouboa, A. (2020). Environmental and socio-economic assessment of cork waste gasification: life cycle and cost analysis. J. Clean. Prod. 249, 119316. doi: 10.1016/j.jclepro.2019.119316
Reim, W., Parida, V., and Sjödin, D. R. (2019). Circular business models for the bio-economy: a review and new directions for future research. Sustainability 11, 2558. doi: 10.3390/su11092558
Richardson, K., Hardesty, B. D., Vince, J. Z., and Wilcox, C. (2021). Global causes, drivers, and prevention measures for lost fishing gear. Front. Mar. Sci. 8, 690447. doi: 10.3389/fmars.2021.690447
Roba, J., Kuppens, T., Janssens, L., Smeets, A., Manshoven, S., and Struyven, K. (2021). Serious games in secondary education to introduce circular economy: experiences with the game EcoCEO. Front. Sustain. 2. doi: 10.3389/frsus.2021.690232
Rosa, P., Sassanelli, C., and Terzi, S. (2019). Towards circular business models: a systematic literature review on classification frameworks and archetypes. J. Clean. Prod. 236, 117696. doi: 10.1016/j.jclepro.2019.117696
Rossi, E., Bertassini, A. C., Ferreira, C. D. S., Neves do Amaral, W. A., and Ometto, A.R. (2020). Circular economy indicators for organizations considering sustainability and business models: plastic, textile and electro-electronic cases. J. Clean. Prod. 247, 119137. doi: 10.1016/j.jclepro.2019.119137
Saidani, M., Yannou, B., Leroy, Y., Cluzel, F., and Kim, H. (2020). “How circular economy and industrial ecology concepts are intertwined? A bibliometric and text mining analysis,” in Online Symposium on Circular Economy and Sustainability, 1-3 July 2020 (Alexandroupolis). Available online at: https://arxiv.org/ftp/arxiv/papers/2007/2007.00927.pdf
Scafidi,. S. (2020). “Creation, Destruction, and the Future of Fashion”. 30 Fordham Intell. Prop. Media & Ent. L.J. 393 (2020). Available online at: https://ir.lawnet.fordham.edu/iplj/vol30/iss2/1 (accessed April 20, 2022).
Sherrington, C., Darrah, C., Hann, S., Cole, G., and Corbin, M. (2016). Study to Support the Development of Measures to Combat a Range of Marine Litter Sources. Report for European Commission DG Environment by Eunomia, 432.
Suárez-Eiroa, B., Fernández, E., Méndez-Martínez, G., and Soto-Oñate, D. (2019). Operational principles of circular economy for sustainable development: linking theory and practice. J. Clean. Prod. 214, 952–961. doi: 10.1016/j.jclepro.2018.12.271
Teece, D. J. (2010). Business models, business strategy and innovation. Long Range Plann. 43, 172–194. doi: 10.1016/j.lrp.2009.07.003
van Giezen, A., and Wiegmans, B. (2020). Spoilt - Ocean Cleanup: alternative logistics chains to accommodate plastic waste recycling: An economic evaluation. Transport. Res. Interdiscipl. Perspect. 5, 100115. doi: 10.1016/j.trip.2020.100115
Vollmer, I., Jenks, M. J. F., Roelands, M. C. P., White, R. J., van Harmelen, T., de Wild, P., et al. (2020). Beyond mechanical recycling: giving new life to plastic waste. Angew. Chem. Int. Ed. 59, 15402–15423. doi: 10.1002/anie.201915651
Walzberg, J., Lonca, G., Hanes, R. J., Eberle, A. L., Carpenter, A., and Heath, G. A. (2021). Do we need a new sustainability assessment method for the circular economy? A critical literature review. Front. Sustain. 1, 620047. doi: 10.3389/frsus.2020.620047
Williams, A. T., and Rangel-Buitrago, N. (2022). The past, present, and future of plastic pollution. Mar. Pollut. Bull. 176, 113429. doi: 10.1016/j.marpolbul.2022.113429
Keywords: end of life fishing gear, circular business model, value chain, textile industry, plastics recycling, circular economy
Citation: Andrés M, Zudaire I, Larreta J, Asueta A, González N, Molist M, Uribesalgo E and Basurko OC (2022) Nuts and bolts of tropical tuna purse seine nets recycling: A circular business model. Front. Sustain. 3:929902. doi: 10.3389/frsus.2022.929902
Received: 27 April 2022; Accepted: 12 July 2022;
Published: 17 August 2022.
Edited by:
Idiano D'Adamo, Sapienza University of Rome, ItalyReviewed by:
A. B. M. Fazle Rahi, University of Gävle, SwedenJingkuang Liu, Guangzhou University, China
Copyright © 2022 Andrés, Zudaire, Larreta, Asueta, González, Molist, Uribesalgo and Basurko. This is an open-access article distributed under the terms of the Creative Commons Attribution License (CC BY). The use, distribution or reproduction in other forums is permitted, provided the original author(s) and the copyright owner(s) are credited and that the original publication in this journal is cited, in accordance with accepted academic practice. No use, distribution or reproduction is permitted which does not comply with these terms.
*Correspondence: Marga Andrés, bWFuZHJlcyYjeDAwMDQwO2F6dGkuZXM=