- 1Geosciences and Environmental Department, Faculty of Mines, National University of Colombia – Medellin, Medellin, Colombia
- 2Department of Green Chemistry and Technology, Ghent University, Ghent, Belgium
- 3Research Institute of Applied Earth Science, University of Miskolc, Miskolc, Hungary
Methodological tools such as Life Cycle Assessment (LCA), Exergy Analysis (ExA), and Emergy Analysis (EmA) that account for sustainability indicators in environmental, economic, and/or social dimensions, cannot provide an assessment under these three dimensions in a robust way by themselves. This research is proposing a sustainability assessment framework to obtain a unified performance metric (Integrated Sustainability Index, ISI) to assess the Triple Bottom Line – TBL. LCA, ExA, and EmA indicators are implemented in a complementary but not interchangeable manner, providing additional information for sustainability decision-making. The systematic approach is on a conceptual definition and calculation of sustainable environmental, social, and economic disaggregated indicators. These are then systematically combined into an Integrated Sustainability Index (ISI). EmA evaluates sustainability from a “donor-side” perspective, by assigning values to the environmental efforts and investment of nature to make and support flows, materials, and services; the system boundary is the geosphere. ExA evaluates sustainability through exergy efficiency under a “user-side” evaluation process (system boundary is the technosphere). LCA evaluates it based on the quantification of environmental impact by water, soil, and air emissions, caused by the use and processing of resources to provide products or services as a “user-side” method. The proposed sustainability index presents a comprehensible hierarchic structure supported by LCA, ExA, and EmA methodologies. The integration of social, environmental, and economic components into an index that also allows for the adjustment of externalities reducing the risk of subjectivity is a new approach to assessing sustainability.
Introduction
The word sustainable is a controversial concept because it has been defined in different ways under many different disciplines. However, as of the 1980s, the word is used to refer to appropriate management of natural resources, in such a way as to allow future generations access to adequate resources. This definition was articulated in the Brundtland Report (Brundtland commission, 1987). In the Brundtland Report, the idea of a sustainability analysis interrelating economic development and the environment was developed. Later, at the 2005 World Summit on Social Development, objectives of sustainable development were identified: economic development, social development, and environmental protection, also known as the three pillars of sustainability (United Nations General Assembly, 2005). Nearly all governments have in principle committed themselves to sustainable development by integrating economic welfare, environmental quality, and social coherence (Böhringer and Jochem, 2007). The preservation of the natural environment is a prerequisite for a well-functioning economy and social justice. Therefore, sustainable development means something more than a compromise between the natural environment and pursuit of economic growth (Reza et al., 2014). It means a definition of development that recognizes that sustainability is only possible within limits that have a structural origin both (economic) and natural.
Different methodological tools for sustainability assessment have been developed. Some are oriented only to environmental sustainability based not only on the environmental burden (generated in the Life Cycle Assessment production chain). These use direct and indirect resources provided by nature for manufacture, ending in a final product, and the investment and economic return that provides for social welfare (Emergy Analysis, EmA). Others include process efficiency in terms of entropy generated (Exergy Analysis, ExA). All these methodologies converge to the same point: tools that provide to the decision-makers environmental, economic, and/or social sustainability indicators for the formulation and implementation of public policies. Another meeting point of these analytical methods is that each one has exactly the same methodological limitations: joint production, commensuration, deliberation, setting system boundaries, and avoiding double counting. However, these methodological tools that account for sustainability indicators in environmental, economic, and/or social dimensions, cannot provide an assessment under these three dimensions in a robust way by themselves. Since each creates indicators representing a specific aspect of sustainability, it is necessary to implement them as a complement but not as interchangeable techniques.
In recent years, there has been an increasing tendency to “integrate” different accounting methods to have a more complete picture of impacts caused by the creation of a product or provision of a service. This avoids depending on one method that generally has a single criterion perspective (Kharrazi et al., 2014). These indicators can be taken as an individual or composite parameters; that is, synthetic aggregations of independent parameters, reflecting the stakeholders' values or considerations (Arbault et al., 2014). For example, Husgafvel et al. (2017b) proposed a sustainability index as the aggregation of each component (environmental, social and economic dimensions) (Husgafvel et al., 2017a,b). Regardless of whether individual or composite indicators are taken, a sustainable process should present energy and technical feasibility that generates economic gains for social welfare with an acceptable environmental burden. Hence, there is a need to make use of all tools for assessing this sustainability, not as exchangeable but as complementary, i.e., it is necessary to evaluate the sustainability of supply and value chains from a holistic view under an integrated approach from two points of view: “user-side” and “donor-side.” The user-side perspective analyzes final efficiency indicators of the process (environmental impacts per unit of product generated, or energy/exergy delivered per unit of energy/exergy inverted.) The donor-side perspective considers the work nature does to provide resources for the production of a good or service, and in turn, to assimilate the pollutant load released to the environment as an important component of sustainability assessment. The accounting of the economic return in relation to the investment made cannot be ignored; as well as the effects and welfare that project execution can bring to society (Arbault et al., 2014).
Detractors of this integration argue that it is a reductionist approach, which leads to an assessment of the three sustainability dimensions through non-correlated factors (Arbault et al., 2014; Kharrazi et al., 2014). Although a holistic approach may result in the loss of information about each individual dimension and the indicators that compose them, it is also true that creating a holistic system-level image of the interactions between the dimensions is critical for quantifying sustainability. The challenge is to build methodological tools that can quantitatively integrate three dimensions with as little loss of information as possible.
The research's aim here is to propose a sustainability assessment framework to obtain a unified performance metric (Integrated Sustainability Index, ISI) to assess Triple Bottom Line – TBL. To achieve this integration, Life Cycle Assessment (LCA) and Exergy Analysis (ExA) as user-side approach and Emergy Accounting (EmA) as a donor-side view are implemented. This is done even though each method differs on the basis of analysis and solves common methodological limitations differently. Some of the main issues faced by both designers and decision-makers are precision and uncertainty in the calculation of integrated indexes. This research presents systematically the proposed methodology to achieve the desired clarity and transparency. Methodological calculations supporting it can be found at Cano (2018) and Cano Londoño et al. (2019). The goal of this work is to describe the methodology in detail making it accessible to a wider scientific community, while the application of the methodology to specific case studies is the focus of a future paper. The document is divided into four sections covering the introduction, methodology, results, and conclusions.
Methodology
Accounting Methods Foundation: Life Cycle Assessment (LCA), Exergy Analysis (ExA), and Emergy Accounting (EmA)
LCA is a methodology standardized by the ISO 14040 (ISO, 1998). It is used to evaluate the environmental impacts of a process through its entire life cycle, including raw materials (extraction), manufacture, transport, use, disposal, and reuse (cradle to cradle) (Cano and Cabezas, 2021). LCA evaluates the concept of sustainability as the assessment of environmental burden from waste and emissions to water, air, and soil generated in the production chain by the use of energy resources, water resources, and other inputs. Note that it does not involve the economic dimension. From the social dimension, damage to human health can be considered one indicator. This topic will be expanded in later sections.
Exergy is a thermodynamic property that quantifies the useful working potential of a given amount of energy as it reaches equilibrium with the surroundings. It considers quality and quantity and applies to material and energy flows. ExA is the only methodology that can describe the degradation of natural capital with some rigor (Cano et al., 2021). ExA allows for evaluating sustainability as the environmental load associated with exergy destroyed. That is, it evaluates the sustainability based on the quantification of the loss of useful energy, due to both the inherent irreversibilities of the process (entropy generation) and the waste/emissions by technological inefficiencies, i.e., the energy that is not used and ends up in the environment as destroyed exergy (Dincer and Rosen, 2015; Velasquez et al., 2020; Cano et al., 2021). ExA is suitable for tracing the energy losses through the process, so it is beneficial for process improvements and for gauging ecosystem stability. Under ExA analysis only the environmental dimension is considered, and no indicator of social and economic dimensions is considered.
Emergy (EmA) assesses sustainability as the environmental load associated with direct and indirect raw material consumed (inputs) provided by the natural ecosystem for the product or service generation (Buonocore et al., 2015). EmA is an integrated evaluation of economic, ecological, technical, and energy systems (Cano et al., 2019, 2021). EmA considers apart from the environmental dimension, the human work and quality of life as indicators of social and economic dimensions, reinforcing the idea that it is a self-consistent method with high robustness (Giannetti et al., 2013). This subject is explained in later sections.
Figure 1 summarizes that EmA evaluates sustainability from a “donor-side” perspective, by assigning values to the environmental efforts and investment of nature to make and support flows, materials, and services. The system boundary is the geosphere. ExA evaluates sustainability through exergy efficiency under a “user-side” evaluation process (system boundary is technosphere). LCA evaluates it based on the quantification of environmental impact by water, soil, and air emissions, caused by the use and processing of resources to provide such product or service as a “user-side” method. By integrating these three analysis methodologies under a unified sustainability assessment methodology, the supply chain sustainability might be evaluated holistically as shown in Figure 1. That is, EmA involves Levels A + B + C, and ExA and LCA consider Levels B + C.
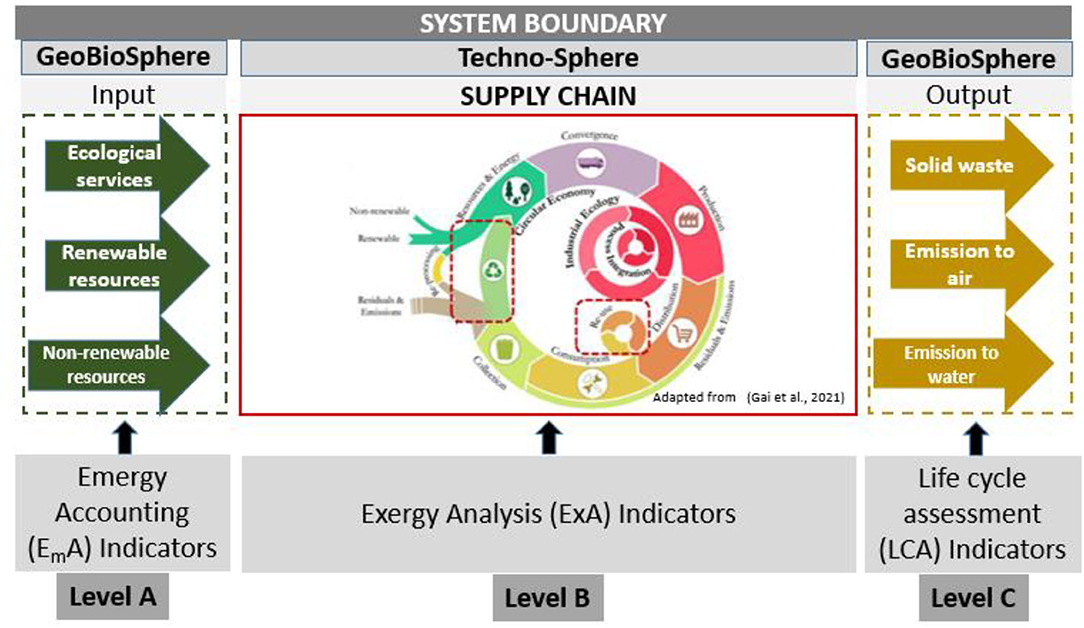
Figure 1. Sustainability integration evaluation by Life Cycle Assessment (LCA), Exergy Analysis (ExA) and Emergy Accounting (EmA) (Modified from Cano et al., 2021).
Supply chain challenges at Level A lie in the efficient use of the resources: (a) dematerialize production systems through reuse/recycling, close the material flow loop in the whole economic system (Stahel and Reday, 1976), (b) use functional substitutes, i.e., the substitution of resource for a less scarce one that fulfills the same functionality (Henckens et al., 2016a), and (c) promote the use of renewable resources to minimize dependence on non-renewables, making the system more productive (Odum, 2001). For supply chain optimization (level B), it is necessary to identify those stages where the greatest destroyed exergy is generated and able to make decisions for improvement: (a) change to new technology, and (b) reduce input resources (strictly using only resources necessary to carry out the process). Level C is a consequence of what happens at Level A and Level B, after efforts to prevent and reduce the waste/emissions. To add value to any residual streams, they should be considered as possible sources of raw material. This minimizes the waste generated and maximizes the resources used (Allwood, 2014; Henckens et al., 2016b; Londoño et al., 2022).
Concepts Definition Involved in the Integrated Sustainability Index (ISI) Methodology
• Indicators
Indicators can provide crucial guidance for decision-making in a variety of ways. They can translate physical and social science knowledge into manageable units of information to facilitate decision-making. They can help measure and calibrate progress toward Sustainable Development Goals (SDG). They can provide an early warning, raising the alarm in time to prevent economic, social, and environmental damage. They are also important tools for communicating ideas, thoughts, and values because “We measure what we value, and value what we measure” (UN, 2001).
• Categories and dimensions
Categories can be defined by the aggregation of different indicators under a well-developed and pre-determined methodology into more general and fewer categories. Categories are powerful and communicative tools that can be a significant aid to planners and decision-makers providing robust and reliable guidance (Gasparatos et al., 2008). In turn, these categories can also be aggregated into a smaller number of general groups called dimensions of sustainability.
• Integrated sustainability index (ISI)
Dimensions are grouped into a single number or a weighted final integrated index derived from different values of unweighted indicators. The advantage of this integrated indicator is that it summarizes multiple indicators through an easy and simple interpretation, facilitating the communication of results to the public. This leads to some criticism since it can fall into reductionism. This can possibly hide serious flaws in some dimensions and increase the difficulty of identifying appropriate corrective actions, and, perhaps, even promoting simplistic political conclusions. Hence, these can communicate misleading policy messages if they are poorly constructed, or can be used to support a preconceived policy if the construction is not transparent and lacks solid statistical or conceptual principles. As a result, indicators and weights selection can be the focus of political challenges (Gasparatos et al., 2008).
Integrated Sustainability Index (ISI), Qualitative Methodology Based on LCA, ExA, and EmA
This section covers the quantitative method used to obtain the Integrated Sustainability Index (ISI) from the integration of LCA, ExA, and EmA indicators. Figure 2 summarizes the integration methodology. Sustainable Triple Bottom Line (TBL) is defined as the criteria of sustainability assessment that include environmental protection, economic prosperity, and social acceptability. Each sustainability dimension is approached from different categories derivate from LCA, ExA, and EmA indicators that are then aggregated into a single sustainable indicator.
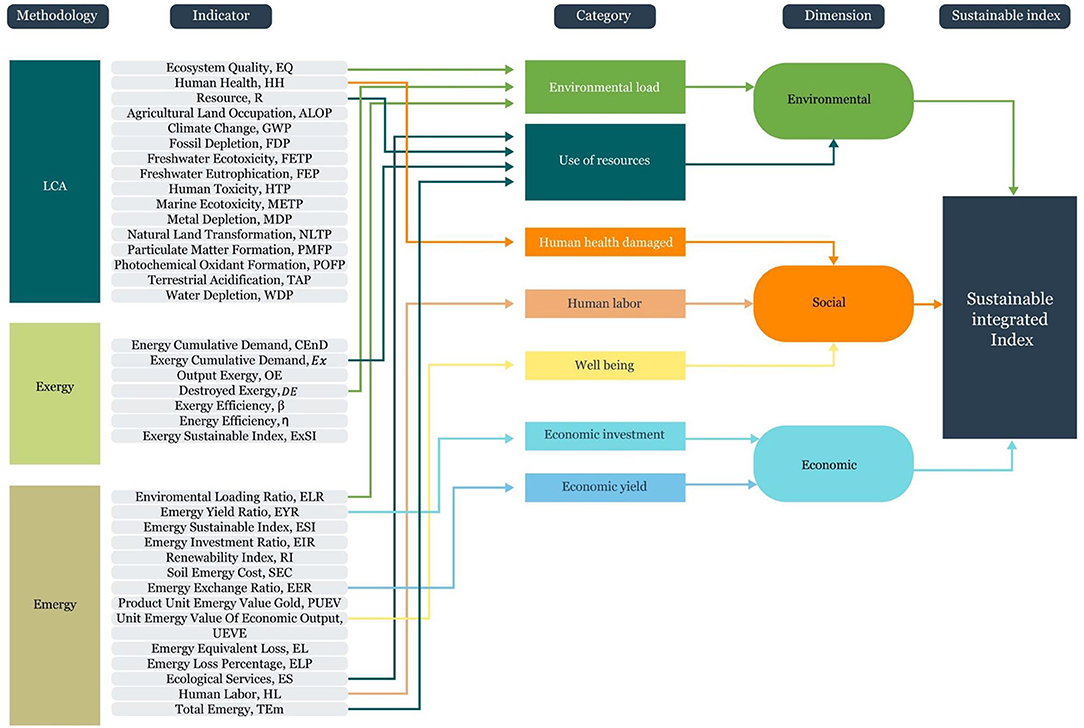
Figure 2. Structural model of Integrated Sustainability Index (ISI) calculation from the integration methodology proposed in this research.
Indicators Aggregation Into Four Categories and Their Contribution to Sustainability Dimensions
Classic methodologies have contributed a series of indicators that allow for evaluating the damage or impact on environmental, social, and/or economic dimensions (Triple Bottom Line), LCA, ExA, and EmA have generally been implemented in isolation, or at best, in a complementary manner. This is evidence of the need for a methodological tool that allows their integration to evaluate and select the best sustainable supply chain option, considering indices such as the use of resources, environmental burden, damage to human health, human labor, quality of life, economic investment, and economic performance. In addition, it is possible to quantify the contribution of each indicator to sustainability dimensions to offer a unified and useful index for better decision-making in policy formulation and monitoring of production activities.
The next sections explain the indicator aggregation process derived from LCA, ExA, and EmA from the following categories: use of resources, environmental burden, human health damage, human labor, quality of life, economic investment, and economic performance as shown in Table 1 and Figure 2. It is noteworthy that not all indicators calculated for each methodology, LCA (Cano, 2018), ExA (Cano et al., 2020), and EmA (Cano et al., 2019) summarized in the study were selected for the integration method since many of them presented double accounting.
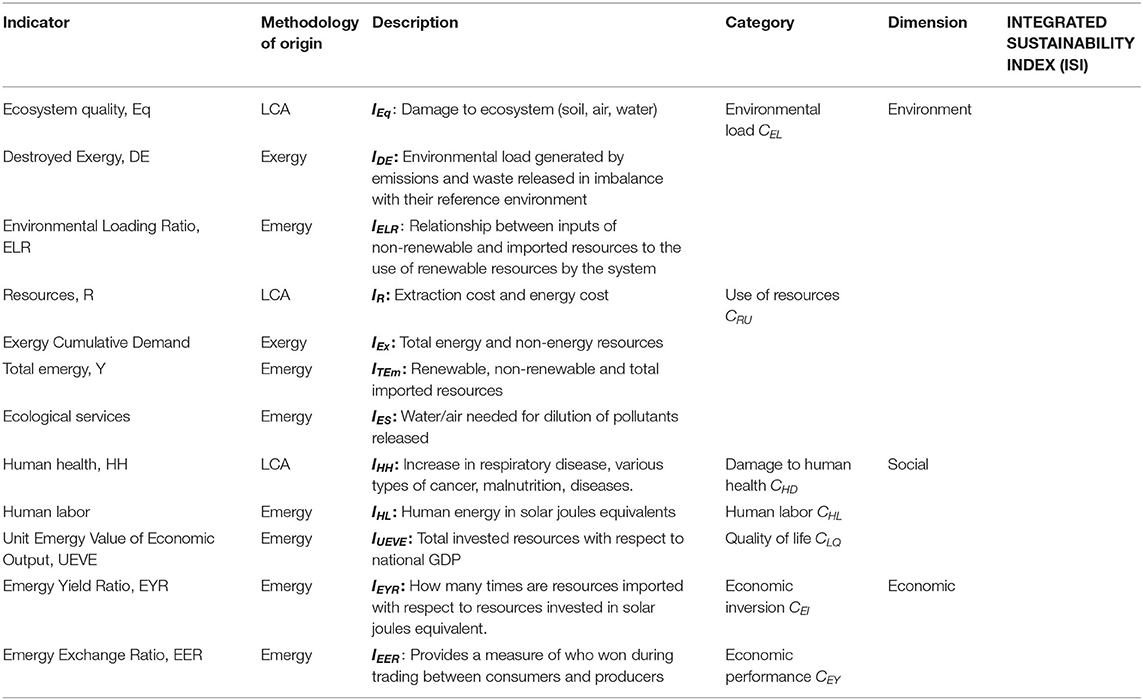
Table 1. Aggregation methodology indicators in categories, dimension, and Integrated Sustainability Index (ISI).
Categories Definition Involved in the Environmental, Economic, and Social Dimensions
The first question is why these categories were selected to be grouped in the environmental, social, and economic dimensions. In this section, this will be addressed by returning to the definition of sustainability as compatibility between the economic and environmental aspects of TBL: environmental protection, economic prosperity, and social acceptability. The selection of categories contributing to each dimension is limited to those generated by each analysis methodology (LCA, ExA, EmA) based on its fundamentals. The scope of this study is to integrate the categories, not to take indicators different from those calculated (Cano, 2018; Cano et al., 2019, 2020), or dissert all of the limitations or biases presented by each one.
• Environmental dimension
To promote the environmental sustainability of a supply chain, it is necessary to make efficient use of resources and minimize the waste/emissions generated in the process (Dincer and Rosen, 2015). This is done through different strategies such as process optimization, use of efficient technologies, and efficient use of resources, among others. Thus, the environmental dimension will depend both on the environmental load generated (waste/emissions) and the efficient use of resources.
- Environmental load CEL
For this methodology, the environmental load is considered to be the environmental impact caused by emissions and waste released into air, soil, and water, due to resources processing for the production of a good or service (Odum, 1996; Huijbregts et al., 2016; ReCiPe, 2016). The following describes how each analysis methodology considers and contributes to the environmental load generated.
From an LCA perspective, the ecosystem quality is affected by the environmental load caused for the generation of waste and emissions. Impact categories such as climate change (GWP), fossil depletion (FDP), freshwater ecotoxicity (FETP), etc. are not considered directly in this methodological proposal, since they are grouped into three damage categories: Ecosystem Quality, Human Health, and Resources. Otherwise, it would cause a double accounting of the impacts shown by LCA. The lower the value of the “Environmental Load” category of impact, the less environmental stress the process generates (Huijbregts et al., 2016; ReCiPe, 2016).
From the indicators calculated by ExA, destroyed Exergy is the one that represents the environmental load generated in the process (Cano, 2018; Cano et al., 2019). This methodology evaluates sustainability based on the quantification of the useful energy loss due to the inherent irreversibilities of the process and the non-use of energy outputs (waste/emissions). These again end in the environment as destroyed exergy contributing to environmental burden (Rosen et al., 2008; Dincer and Rosen, 2015). The less destroyed exergy produced, the lower the process environmental load.
EmA contributes to this category through the Environmental Loading Ratio (ELR). This is the default indicator in this methodology and contributes to environmental load as the relationship between inputs of non-renewable and imported resources to the use of renewable resources by the system (Odum, 1996, 2001). The high dependence on non-renewable and imported resources, and not on renewable resources, generates a larger environmental load (Cano, 2018; Cano et al., 2019).
- Use of resources, CRU
For purposes of this methodology, the use of resources is defined as the demand for all renewable, non-renewable, and imported resources required for generating a product or service, i.e., natural capital demand.
LCA represents the resources damaged category by default as it accounts for the extraction cost of mineral resources and fossil energy (oil, gas, coal) along the supply chain (Huijbregts et al., 2016; ReCiPe, 2016). Renewable resources are not taken into account since this methodology does not consider the work that nature had to do to provide them; it is a user-side method as described by Cano (2018) and Cano Londoño et al. (2019).
The Exergy Cumulative Demand indicator from ExA represents a user-side method, i.e., the total exergy consumption from nature to provide a given good or service, summing up the exergy of all resources required. Unlike the resources damaged category considered in LCA, ExA assesses the quality of energy demand and includes the exergy of energy carriers as well as that of non-energy materials (Szargut, 2005; Dincer and Rosen, 2007).
EmA quantifies the natural capital necessary for generating a product or service (Kharrazi et al., 2014), discretized in renewable, non-renewable, and imported sources. The sum of all of these is the Total Emergy (Y); providing emergy indexes to assess sustainability from an ecocentric perspective (bridges economic, and ecological parameters). In this category, ecological services provided by the environment (water and air resource) for the dilution of waterborne/airborne generated in the process are taken into account in terms of solar joules equivalents. It is important to remember that although ecological services are included in the calculation base of LCA, it is included in this category because the damage categories of LCA do not consider the ecological services of dilution. So, there is no double counting of impacts.
• Social dimension
This research considers that everything that promotes the improvement of the social conditions throughout the supply and value chain is considered social sustainability. This has the ultimate goal of achieving human wellbeing (UNEP/SETAC, 2020). Now, the question is what is human wellbeing? Many conceptualizations coexist in this aspect. The most common are quality of life, standards of life, and human development. But the following terms can also be used: wellbeing, satisfaction with life, the satisfaction of basic human needs, human development, happiness, and utility (UNEP/SETAC, 2009). For this reason, the dimensions here are diverse and cover many aspects ranging from knowledge, friendship, self-expression, affiliation, bodily integrity, health, economic security, human labor, freedom, affection, wealth, and leisure (Alkire, 2002).
As it can be seen, categories in this dimension can be quite numerous and generally valid. So, our selection is limited to the indicators generated by the three analysis methodologies implemented in this study. Only LCA and EmA provide indicators within this social dimension; human health in LCA, and human labor and Unit Emergy Value of Economic Output (UEVE) in EmA. It is important to clarify that in this dimension no distinction is made between the stakeholders for whom indices are calculated. Although, other methodologies such as Social Life Cycle Assessment (SLCA) do attempt to do so systematically (UNEP/SETAC, 2009; Wulf et al., 2019; SETAC, 2020).
- Damage to human health, CHD
This category is represented by the years of life lost to a person, or the years that a person is disabled due to a disease caused by emissions and waste generated in the production chain. In our work, this category only includes the human health damage category calculated from LCA in metric units of DALYs (Disability Adjusted Life Years) (ReCiPe, 2016). Additional information on this category can be found in the literature (Cano, 2018).
- Human labor, CHL
This category is represented by the human capital necessary to carry out the process in terms of the number of people hired per year. EmA addresses this indicator in terms of solar joules per year (seJ/yr). The calculation of this imported resource is shown in detail in the Appendix by Cano Londoño et al. (2019).
- Quality of life (Wellbeing), CLQ
It is clear that the GDP (Gross Domestic Product) of a nation as an indicator cannot measure the overall progress or wellbeing, because it does not take into account factors such as social equity and natural and human capital (Giannetti et al., 2015). It is necessary to integrate this economic indicator into other factors through different methodologies as described by Giannetti et al. (2015) to assess human wellbeing in a more objective way. Many governments and non-governmental organizations have taken the initiative and developed their indices for that purpose; most of them are composite indexes merging different measures into a single number consisting of GDP plus social and environmental concerns.
An arguable aspect of EmA is its approach to connecting environmental resources and their economic use (Giannetti et al., 2015). Real wealth derives from environmental resources while the income required for progress depends on how much real wealth (measured in emergy) is available. By dividing the emergy used by the GDP of an economy, it is possible to define the real buying power of money in a given country, and the optimal income to support progress and wellbeing (Odum, 1996). This indicator is the Unit Emergy Value of Economic Output, UEVE. It combines the renewable, non-renewable, and imported resources needed to carry out the process in terms of solar joules equivalents. While the GDP (Gross Domestic Product) measures the economic growth through production and final demand for goods and services in a country or region for a given period, the higher the UEVE, the less sustainable the system in socioeconomic terms. The reason is that it means that more resources need to be used to generate the same amount of money to provide for social welfare. This can be used in comparing two processes/alternatives.
• Economic dimension
Since the economy is an open system that dissipates energy and materials, its sustainability depends on the availability of the energy and materials it consumes (Lomas and Martín-lópez, 2005). For this method, economic sustainability is understood as a supply and value chain capable of self-sustaining economically. That is, an economically sustainable supply and value chain is one whose production costs are less than its profitability; the said profitability depends on the sale price of products. This can vary depending on the economy of the region where the supply and value chain is being executed (UNEP/SETAC, 2009; SETAC, 2020). The sale price of a product or service must support not only the economic supply chain investment it entails but also the mitigation and compensation for environmental and social impacts.
In this dimension, it is very important to consider the type of resources consumed. If society generates structures requiring large flows of energy from natural resources and large fossil energy storage, and if concentrations of these resources are consumed and exhausted, then society must dispense with these structures or face a forced decline. Sustainability in general will likely be better achieved by societies depending on the consumption of renewable energies and materials. In other words, the sustainability of a society or supply and value chain depends mainly on the types of resources or energies being used.
It is noteworthy that the economic dimension is only addressed from the producer's side. The categories that contribute to this dimension are investment and economic performance. These categories are the result of calculating emergy indicators since it is the only methodology including this dimension.
- Economic inversion, CEI
This indicator shows the dependence of the supply and value chain on imported resources where some have to be purchased and others are free resources found in nature (renewable and non-renewable). For free resources, the only cost is that of extraction (Cano et al., 2019). More sustainable supply and value chains are those with low dependence on imported resources. The product generated from a process with a high dependence on imported resources must have a high value in the market, which internalizes the low contribution of renewable natural capital in the process economy.
- Economic performance, CEY
This indicator evaluates economic return compared to the economic capital invested, showing economic benefit for producers vs. consumers. The ratio of the total invested emergy to a product's sale price is the emergy money ratio (also known as emergy-money or emergy exchange). It is the amount of emergy that can be purchased in one country with a unit of money in a specific year. Obtaining greater economic performance when less total emergy is invested points toward sustainability. This indicator can be extended in Cano (2018) and Cano et al. (2019).
Table 1 summarizes the aggregation methodology from LCA, EmA, and ExA indicators to categories and dimensions ending in the Integrated Sustainability Index (ISI).
Integrated Sustainability Index (ISI) Methodology Mathematical Description Based on LCA, ExA, and EmA
Seven categories were proposed; Environmental load CEL, Use of resources CRU, Damage to human health CHD, Human labor CHL, Quality of life, CLQ, Economic inversion CEI, and Economic profit CEY. Each of them is calculated based on indices coming from LCA, ExA, and EmA.
In the previous mathematical expressions Equations 1–7, each indicator has its units, according to its nature; to be able to group them, it is necessary to normalize using a reference value. In this case, the reference value for the series is the best value of the series, thus the constant ki is defined in Equation 8.
ki with i = Eq, DE, ELR, R, Ex, TEm, ES, HH, HL, UEVE, EYR, EER.
Category Calculation, Normalization, and Interpretation
Indicators derived from LCA, ExA, and EmA can be interpreted as Max and Min depending on how they affect sustainability:
- Max: corresponds to an indicator that positively affects sustainability when it is greater.
- Min: corresponds to an indicator that positively affects sustainability when it is lower.
Categories are proportional additions of carefully selected indicators with the same interpretation of Max or Min to avoid ambiguities. The characteristic of each category is the common factor from the indicators that constitute it. The characteristics for each indicator are presented in Table 1.
• Reference value of the category
Each category, used to compare several process or technology options, requires a reference value to establish a measurement scale. For this, it is assumed that if only one option is analyzed with this methodology, it will be the best one. In this case, the product of the factor in each category, which gives us the reference value for each category, is equal to the number of indicators that contribute to that category. The reference values for each category are presented in Table 2.
• Results interpretation by category
- Case 1: category with minimum characteristic
A category with Min characteristic is the case of environmental load, use of resources, damage to human health, quality of life, and economic profit. In the best case, it will take a value equal to the reference (number of indicators that contribute to that category), and in the worst case, it will be much higher than the reference value. This is because the best option for indices it contributes to in each category will always be the lowest value of the indexes among comparison options.
- Case 2: category with maximum characteristic
A category with Max characteristic as is the case of human labor and economic investment. In the best case, it will take a value equal to the reference (number of indicators that contribute to that category), and in the worst case, it will be close to zero. This is because the best option for indexes it contributes to each category will always be the largest index among comparison options.
• Normalization with Reference and redirection of categories
Since categories can have Max or Min characteristics according to their indicators, for aggregating them into sustainability dimensions, it is necessary to normalize them and eliminate the differences in analysis directions (Max or Min). We do so by the following procedure below:
- Case 1: category with minimum characteristic
A category with Min characteristic is one such as the case of environmental load, use of resources, damage to human health, quality of life, and economic profit. It is normalized and redirected (0 as the minimum value and 1 as the maximum value), determining the relationship between the reference value of CJ,Ref category and value of Cj category for each option Equation 9
- Case 2: category with maximum characteristic
A category with a Max characteristic is one such as the case of human labor and economic investment. It is normalized and redirected (0 as the minimum value and 1 as the maximum value), determining the relationship between the value of Cj category and the reference value of CJ,Ref category Equation 10
Categories Aggregation Into Sustainability Dimensions
As previously explained, categories are grouped into three dimensions: environmental, social, and economic.
- Environmental dimension
In the proposed methodology, the environmental dimension is defined as the equal percentage contribution of standardized categories of resource use and environmental burden. Equation 11
- Social dimension
In the proposed methodology, the social dimension is defined as the equal percentage contribution of normalized categories of human labor, damage to human health, and quality of life. Equation 12
- Economic dimension
In the proposed methodology, the economic dimension is defined as the equal percentage contribution of standardized categories of economic investment and economic profit. Equation 13
• Reference value of the dimensions
Each dimension is defined by the equal percentage contribution of several standardized categories. The maximum value that each dimension can take is equal to the number of categories that constitute it since they can take the unit as the maximum value (Table 3).
• Normalization of dimensions using a reference value
Dimensions are normalized using reference values. Equation 14
Integrated Sustainability Index (ISI) by Aggregation Dimensions
• Integrated sustainability index
The average sustainability index is calculated as the contribution of the three dimensions. Equation 15.
The Integrated Sustainability Index compares two processes using indicators from LCA, ExA, and EmA; however, this indicator does not take into account the changes in the Environmental, Social, and Economic baseline of the place where the project is established. To account for these influences, two alternatives to Integrated Sustainability Index are presented: the first is to use weighting factors for each dimension called as Weighted Average Sustainability Index (WASI). The second one is to include a category that quantifies the modifications to the environmental, social, and economic baseline in the calculation of each dimension, due to the presence of the process Average Adjusted Sustainability Index (AASI).
- Weighted Average Sustainability Index (WASI).
Weighted Average Sustainability Index was calculated using the weights for each dimension Equation 15 PEnviromental, PSocial, PEconomic
Weighting factors correspond to the importance of each dimension. These important factors must be a consensus for all stakeholders. For example: requirements of external regulatory bodies' influence on affected community, internal policies of a company, among others.
- Average Adjusted Sustainability Index (AASI).
The average Adjusted Sustainability Index was calculated by adding one new category in each dimension (Environmental Changes in Line Base CCBLEnv, Social changes in line base CCBLSoc, and Economic changes in line base CCBLEco) Equations 17–19.
The dimension normalized is defined as:
(DEnviroment,Ref = 3, DSocial,Ref = 4, DEconomic,Ref = 3) and Average Adjusted Sustainability Index as
To determine values between 0 and 1 of new categories that reflect the environmental, social, and economic change of the environment regarding a baseline, it is necessary to have a working group constituted by an interdisciplinary group of experts: Researchers, academics, government, environmental authorities as well as representatives of the community and the private firm owning the production process that can objectively assess changes in the baseline generated by the incursion of the project. A null modification with values equal to zero, and the highest modification of the environment with values equal to 1. Estimating these values is not within the scope of this study, but it is necessary to consider it.
Results of the Integrated Sustainability Index (ISI) Methodology: Illustration
Two of the three proposed methodologies (AASI and WASI) consider a degree of subjectivity in the weighting factors and the values allocated to the categories of changes in the baseline. However, the nature of weighting diminishes the rigorous analysis of categories constructed with the analysis of LCA, ExA, and EmA. In addition, WASI requires the relation of three categories and values agreed upon by a multidisciplinary and interdisciplinary group to have a representative value.
It should be noted that, in general, in integration methodologies, weighting scoring systems are often based on expert judgment and can be biased (Klöpffer and Grah, 2014). Moreover, weighting aggregation techniques usually ignore the fundamental essence and usefulness of different indicators that contribute to each category or dimension, which grouped together generate a single index of integrated sustainability. For this reason, in this study, to calculate the Integrated Sustainability Index (ISI) weights or values of importance were applied to the indicators, but not to categories nor to dimensions to minimize this bias. However, the possibility of assigning weights to each of these indicators/categories is left open. This weighting must be subject to as rigorous consensus as possible among all stakeholders intervening in the supply. Figure 3 shows systematically the implementation of this proposed integration methodology for the assessment of sustainability in development projects.
Note that for implementing this proposed integration methodology, independent of the economic sector, it is necessary to start calculating indicators from emergy, exergy, and LCA. That is, without calculating the appropriate indices, it is not possible to continue with the implementation of the proposed methodology. If exergy had been taken as a function of emergy, this complementary integration analysis could not have been done, since it would incur a double accounting of impacts or benefits if it were the case.
Resource efficiency, environmental burden, and process efficiency can be considered as one of the interpretations/consequences of Brundtland's definition of sustainable development. The integration methodology proposed here accounts for the use of all the resources required for the generation of a product or service. At the same time, it assesses the environmental degradation caused by waste and/or emissions inherent to the process as well as the management that can be given to them so that the process is economically profitable and socially viable, under parameters such as damage to human health, human labor, and quality of life. Hence the integration of LCA, ExA, and EmA; LCA evaluates process sustainability based on the environmental impacts generated by waste and emissions released to the environment, EmA based on the use of the necessary resources to carry out the process, and ExA based on process efficiency.
In addition, to evaluate the sustainability of a process, it is necessary to cover its life cycle as much as possible. The role of ecosystem goods and services must be taken into account since they form the basis of planetary activities and human welfare. Consequently, the system boundary must be large enough to account for all the ecosystem goods and services that support technological activities during the life cycle. Exergy and LCA do not consider the environmental contribution to the human economic system, but Emergy can consider all ecosystem services, hence the need to complement and integrate analysis methodologies. There are no exact limits to defining sustainability. In contrast, the border between sustainable and unsustainable is blurred, which means that it is often not possible to determine exact reference values for what is sustainable and what is not. That is why it is necessary to compare sustainability between alternate systems since it is rarely possible to determine that a process is sustainable with a simple number. For this reason, in this integration method, a value between 0 and 1 was defined, being 0 a 0% sustainable process, and 1 a 100% sustainable.
Process sustainability improvement lies in making efficient use of the resources together with the optimization of process efficiency, which leads to the reduction of emissions and waste generated, thus reducing the pollution in the environment. With these strategies, virgin resources are diminished, which implies a lower extraction. Waste that cannot be avoided because it is inherent to the process must have a new use through recycling and reuse strategies. To implement the methodology presented here, the following considerations are suggested:
- Compare the critical part of the different economic sectors such as the mining sector (energy and water) with other economic sectors that also make intensive use of these resources such as agriculture and livestock. To be a valid comparison, the scope and functional unit must be comparable.
- Apply theories of elasticity to calculate the global exergy efficiency of the process based on exergy efficiencies for individual processes. This would allow for reducing the calculation time.
- Exergy analysis based on the inventory of resources.
- Carry out another exergy scenario, where accumulated exergy demand is calculated as a function of the consumption of renewable, non-renewable, and imported resources, similar to how the cumulative emergy demand is calculated, but in terms of exergy not emergy.
- Evaluate possible methodologies for the objective assessment of weight importance of dimensions for the calculation of WASI, as well as those of impact categories for the calculation of AASI, where all stakeholders are participating throughout the entire productive cycle.
- To implement the proposed integration methodology by stages and not globally, to determine which stages of the process should have greater importance to improve their sustainability. This would involve making emergy analysis by stages.
Conclusions
It can be seen how EmA is an analytical methodology that connects the economy and ecological systems (Hau and Bakshi, 2004), allowing us to evaluate the contribution of environmental, economic, and social factors in the estimation of an unbiased Integrated Sustainability Index. While LCA is an analysis methodology that only contributes to environmental and social factors, and ExA to environmental factors, speaking in TBL terms. Through complementarity and integration of the three analysis methodologies under a common framework, an adequate evaluation of the system can be achieved, i.e., the environmental, economic, and social support provided by the biosphere and geobiosphere necessary for the generation of the product.
The reductionism performed when presenting a single Integrated Sustainability Index has been one of the main criticisms presented by the methodologies that evaluate sustainability. However, as discussed in this document, there are advantages to having the holistic approach for the evaluation of a supply chain (environmental, economic, social). And, the ease of interpretation by the general public for decision-making is important. But it is necessary not to use these indexes indiscriminately. Making the analysis methodology strong, robust, and accurate can mitigate the risks of making decisions depending on policymakers preferences or priorities given the environmental, social, and economic interests.
In order not to fall into the reductionism described above, this methodology proposes to interpret the most general to the most specific. Comparing different scenarios to be evaluated from the Integrated Sustainability Index to have a general overview of alternatives/processes to be compared; followed by the analysis of dimensions (environmental, social, and economic) that allow addressing which dimensions are subject to improvements by determining those specific indices that make the process not so sustainable.
The proposed sustainability index presents a comprehensible hierarchic structure supported by established methodologies such as LCA, ExA, and EmA. The integration of social, environmental, and economic components into an index that allows the subjective adjustment of externalities reduces the risk of subjectivity overshadowing rigorous work. This is a new approach to assessing sustainability in development projects. The application of the methodology to specific real-life cases, particularly mining operations in Colombia, is beyond the scope of this study and will be the subject of a future study. But the proposed integration method could be used as a helpful methodological tool for sustainability assessment of various economic activities, facilitating decision-making by policymakers (government, environmental authorities, corporations, community, and companies). It can also help in proposing improvements, changes and key elements for the economic, energy, and especially environmental optimization of the process. The integration methodology is also suitable for sustainability reports according to the GRI (Global Reporting Initiative) guidelines as well as for comparisons between different production operations and other economic sub-sectors.
Data Availability Statement
The raw data supporting the conclusions of this article will be made available by the authors, without undue reservation.
Author Contributions
NC-L and CH: conceptualization, methodology, visualization, and investigation. NC-L, BK, and CH: writing—original draft preparation and writing—reviewing and editing. CH and BK: supervision. All authors contributed to the article and approved the submitted version.
Funding
Doctoral Program funded by the Department of Science and Technology of Colombia (COLCIENCIAS). National call for supporting research projects and artistic creation of the UNIVERSIDAD NACIONAL DE COLOMBIA 2017–2018. ENLAZA MUNDOS program 2017. Higher Education Agency of Medellín-SAPIENCIA and Mayor's Office of Medellín.
The research contribution by CH and BK was carried out in the framework of the GINOP-2.3.2-15-2016-00010 Development of enhanced engineering methods with the aim at utilization of subterranean energy resources project of the Research Institute of Applied Earth Sciences of the University of Miskolc in the framework of the Szechenyi 2020 Plan, funded by the European Union.
Conflict of Interest
The authors declare that the research was conducted in the absence of any commercial or financial relationships that could be construed as a potential conflict of interest.
Publisher's Note
All claims expressed in this article are solely those of the authors and do not necessarily represent those of their affiliated organizations, or those of the publisher, the editors and the reviewers. Any product that may be evaluated in this article, or claim that may be made by its manufacturer, is not guaranteed or endorsed by the publisher.
Acknowledgments
This project was carried out as part of the Doctoral Program funded by the Department of Science and Technology of Colombia (COLCIENCIAS). This research was supported by the 1) School of Mines at the National University of Colombia at Medellin; 2) Bioprocess and Reactive Flow Research Group.
References
Alkire, S. (2002). Dimensions of human development. World Dev. 30, 181–205. doi: 10.1016/S0305-750X(01)00109-7
Allwood, J. (2014). “Squaring the circular economy: the role of recycling within a hierarchy of material management strategies,” in Handbook of Recycling: State-of-the-Art for Practitioners, Analysts, and Scien- tists, eds M. Worrell, and E. Reuter, 445–4771. doi: 10.1016/B978-0-12-396459-5.00030-1
Arbault, D., Rugani, B., Tiruta-Barna, L., and Benetto, E. (2014). A semantic study of the emergy sustainability index in the hybrid lifecycle-emergy framework. Ecol. Indic. 43, 252–261. doi: 10.1016/j.ecolind.2014.02.029
Böhringer, C., and Jochem, P. E. P. (2007). No TitleMeasuring the immeasurable—A survey of sustainability indices. Ecol. Econ. 63, 1–8. doi: 10.1016/j.ecolecon.2007.03.008
Buonocore, E., Vanoli, L., Carotenuto, A., and Ulgiati, S. (2015). Integrating life cycle assessment and emergy synthesis for the evaluation of a dry steam geothermal power plant in Italy. Energy 86, 476–487. doi: 10.1016/j.energy.2015.04.048
Cano Londoño, N. A., Velásquez, H. I., and McIntyre, N. (2019). Comparing the environmental sustainability of two gold production methods using integrated emergy and life cycle assessment. Ecol. Indic. 107:105600. doi: 10.1016/j.ecolind.2019.105600
Cano, N. (2018). Sustainability Assessment of Alluvial and Open Pit Mining Systems in Colombia: Life Cycle Assessment, Exergy Analysis, and Emergy Accounting. Universidad Nacional de Colombia. Available online at: http://bdigital.unal.edu.co/71385/7/1020407602.2018.pdf.
Cano, N., and Cabezas, H. (2021). Perspectives on circular economy in the context of chemical engineering and sustainable development. Curr. Opin. Chem. Eng. 34:100738. doi: 10.1016/j.coche.2021.100738
Cano, N., Hasenstab, C., and Velasquez, H. (2019). “32nd International Conference on Efficiency, Cost, Optimization, Simulation and Environmental Impact of Energy Systems,” in Life-Cycle Assessment (LCA) of Exergy Indicators in the Colombian Gold Mining Sector: A Case Study in an Open-Pit and in an Alluvial Mining Processes, eds W. Stanek, P. Gładysz, S. Werle (Polonia: Institute of Thermal Technology). Available online at: http://www.s-conferences.eu/FTP/ECOS-files/ECOS2019_proceedings_v2.pdf.
Cano, N., Hasenstab, C., and Velasquez, H. I. (2020). Exergy life cycle assessment indicators in Colombian gold mining sector. J. Sustain. Mining 19:2. doi: 10.46873/2300-3960.1013
Cano, N., Natalia, S., Céspedes, S., Guerrero, C., and Gallego, D. (2021). Exergy and emergy: complementary tools for assessing the environmental sustainability use of biosolids generated in wastewater-treatment plant for energyproduction. Química Nova. 45, 1–12. doi: 10.21577/0100-4042.20170806
Dincer, I., and Rosen, M. (2015). “Exergy and its ties to the environment, economics, and sustainability, exergy analysis of heating, refrigerating and air conditioning,” in Methods and Applications. doi: 10.1016/B978-0-12-417203-6.00001-6
Dincer, I., and Rosen, M. A. (2007). Exergy, Energy, Environment and Sustainable Development. 1st. Edn. Marc: Elsevier. doi: 10.1016/B978-008044529-8.50006-9
Gasparatos, A., El-Haram, M., and Horner, M. (2008). A critical review of reductionist approaches for assessing the progress towards sustainability. Environ. Impact Assess. Rev. 28, 286–311. doi: 10.1016/j.eiar.2007.09.002
Giannetti, B. F., Agostinho, F., Almeida, C. M. V. B., and Huisingh, D. (2015). A review of limitations of GDP and alternative indices to monitor human wellbeing and to manage eco-system functionality. J. Cleaner Prod. 87, 11–25. doi: 10.1016/j.jclepro.2014.10.051
Giannetti, B. F., Bonilla, S. H., and Almeida, C. M. V. B. (2013). An emergy-based evaluation of a reverse logistics network for steel recycling. J. Cleaner Prod. 46, 48–57. doi: 10.1016/j.jclepro.2012.05.024
Hau, J. L., and Bakshi, B. R. (2004). Expanding exergy analysis to account for ecosystem products and services expanding exergy analysis to account for ecosystem products and services. Environ. Sci. Technol. 38, 3768–3777. doi: 10.1021/es034513s
Henckens, M. L. C. M., Driessen, P. P. J., Ryngaert, C., and Worrell, E. (2016a). The set-up of an international agreement on the conservation and sustainable use of geologically scarce mineral resources. Resour. Policy 49, 92–101. doi: 10.1016/j.resourpol.2016.04.010
Henckens, M. L. C. M., van Ierland, E. C., Driessen, P. P. J., and Worrella, E. (2016b). Mineral resources: geological scarcity, market price trends, and future generations. Resour. Policy 49, 102–111. doi: 10.1016/j.resourpol.2016.04.012
Huijbregts, M. A. J., Steinmann, Z. J. N., Elshout, P. M. F., Stam, G., Verones, F., Vieira, M., et al. (2016). ReCiPe2016: A harmonized life cycle impact assessment method at midpoint and endpoint level. Int. J. Life Cycle Assess. 22, 138–147. doi: 10.1007/s11367-016-1246-y
Husgafvel, R, Pajunen, N, Dahl, O, Heiskanen, K, Ekroos, A, and Virtanen, K. (2017b). “Environmental and economic sustainability metrics for metal production industry - experiences from university-industry cooperation,” in Sustainable Business, Management, and Economics. Frontiers in Sustainablilty, Vol. 2, eds L. Berger and M. M. Bergman. p. 98–122. doi: 10.3390/books978-3-03842-334-8-5
Husgafvel, R., Poikela, K., Honkatukia, J., and Dahl, O. (2017a). Development and piloting of sustainability assessment metrics for arctic process industry in Finland-The biorefinery investment and slag processing service cases. Sustainability 9:1693. doi: 10.3390/su9101693
ISO (1998). Environmental Management-Life Cycle Assessment-Principles and Framework. International Organisation for Standardisation, Geneva, Switzerland. Switzerland.
Kharrazi, A., Kraines, S., Hoang, L., and Yarime, M. (2014). Advancing quantification methods of sustainability: a critical examination emergy, exergy, ecological footprint, and ecological information-based approaches. Ecol. Indic. 37, 81–89. doi: 10.1016/j.ecolind.2013.10.003
Klöpffer, W., and Grah, B. (2014). Life Cycle Assessment (LCA): A Guide to Best Practice, ed. V. C. H. Wiley (John Wiley and Sons), 480. doi: 10.1002/9783527655625 (accessed April 21, 2014).
Lomas, P. L., and Martín-lópez, M. B. (2005). ‘El Sistema de Evaluación Emergética (Emergy Synthesis). Integrando Energía, Ecología y Economía', (January).
Londoño, N. C., Capaz, R. S., Hasenstab, C., Velásquez, H., McIntyre, N., Corder, G., et al. (2022). Life Cycle impacts assessment of two gold extraction systems in Colombia: Open-pit and alluvial mining. Int. J. Life Cycle Assess.
Odum, H. (1996). Environmental Accounting: Emergy and Environmental Decision Making. New York, NY: J. W. Sons.
ReCiPe (2016). A Harmonized Life Cycle Impact Assessment Method at Midpoint and Endpoint Level Report I: Characterization RIVM Report 2016-0104.
Reza, B., Sadiq, R., and Hewage, K. (2014). Emergy-based life cycle assessment (Em-LCA) for sustainability appraisal of infrastructure systems: a case study on paved roads. Clean Technol. Environ. Policy 16, 251–266. doi: 10.1007/s10098-013-0615-5
Rosen, M. A., Dincer, I., and Kanoglu, M. (2008). Role of exergy in increasing efficiency and sustainability and reducing environmental impact. Energy Policy 36, 128–137. doi: 10.1016/j.enpol.2007.09.006
SETAC (2020). Guidelines for social life cycle assessment of products. Management 15:104. Available online at: http://www.unep.fr/shared/publications/pdf/DTIx1164xPA-guidelines_sLCA.pdf.
Stahel, W. R., and Reday, G. (1976). The Potential for Substituting Manpower for Energy, Report to the Commission of the European Communities, Brussels.
UN (2001). Indicators of Sustainable Development: Guidelines and Methodologies. New York, NY. Available online at: http://www.un.org/esa/sustdev/publications/indisd-mg2001.pdf.
UNEP/SETAC (2009). Guidelines for Social Life Cycle Assessment of Products. Paris: United Nations Environment Programme.
Velasquez, H. I., Orozco Loaiza, C. A., Hasenstab, C., and Cano, N. (2020). Evaluation of the extractive gold process: open-pit mining through exergy analysis. J. Sustain. Mining 19. doi: 10.46873/2300-3960.1014
Keywords: Integrated Sustainability Assessment (ISA), Life Cycle Assessment (LCA), Exergy Analysis (ExA), Emergy Analysis (EmA), single indicator, sustainability framework
Citation: Cano-Londoño N, Heriberto C and Krisztián B (2022) Integrated Sustainability Assessment: Exergy, Emergy, Life Cycle Assessment. Front. Sustain. 3:921874. doi: 10.3389/frsus.2022.921874
Received: 16 April 2022; Accepted: 17 June 2022;
Published: 26 July 2022.
Edited by:
Karina Angelica Ojeda Delgado, University of Cartagena, ColombiaReviewed by:
Beatriz Belmonte, University of Santo Tomas, PhilippinesRoope Husgafvel, Aalto University, Finland
Copyright © 2022 Cano-Londoño, Heriberto and Krisztián. This is an open-access article distributed under the terms of the Creative Commons Attribution License (CC BY). The use, distribution or reproduction in other forums is permitted, provided the original author(s) and the copyright owner(s) are credited and that the original publication in this journal is cited, in accordance with accepted academic practice. No use, distribution or reproduction is permitted which does not comply with these terms.
*Correspondence: Natalia Cano-Londoño, bmFjYW5vbCYjeDAwMDQwO3VuYWwuZWR1LmNv